- 1The Molecular Microbiology Laboratory, The Agricultural Biotechnology Division, School of Agriculture and Rural Development, Ramakrishna Mission Vivekananda Educational and Research Institute, Ramakrishna Mission Ashrama-Narendrapur, Kolkata, India
- 2All India Network Programme on Organic Farming-Narendrapur Centre, Ramakrishna Mission Vivekananda Educational and Research Institute, Ramakrishna Mission Ashrama-Narendrapur, Kolkata, India
- 3The Agriculture and Rural Development Division, School of Agriculture and Rural Development, Ramakrishna Mission Vivekananda Educational and Research Institute, Ramakrishna Mission Ashrama-Narendrapur, Kolkata, India
- 4Sasya Shyamala Krishi Vigyan Kendra, Ramakrishna Mission Vivekananda Educational and Research Institute, Ramakrishna Mission Ashrama-Narendrapur, Kolkata, India
- 5Department of Agricultural Biotechnology, Faculty of Agriculture, Bidhan Chandra Krishi Viswavidyalaya, Mohanpur, India
- 6The Quality Testing Laboratory, Ramakrishna Mission Vivekananda Educational and Research Institute, Ramakrishna Mission Ashrama-Narendrapur, Kolkata, India
- 7Institute of Agricultural Science, University of Calcutta, Kolkata, India
- 8Department of Agronomy, Faculty of Agriculture, Bidhan Chandra Krishi Viswavidyalaya, Mohanpur, India
- 9All India Network Programme on Organic Farming-Umiam Centre, ICAR Research Complex for NEH Region, Umiam, India
- 10ICAR-Indian Institute of Farming Systems Research, Meerut, India
India's oldest documented manure, most commonly referred to as Kunapajala, has a long history of over 1,000 years in crop cultivation. Kunapajala is primarily an in-situ decomposition technology of animal waste and can potentially provide an eco-friendly pipeline for recycling bio-waste into essential plant nutrients. This traditional animal manure, in addition, also contains dairy excreta (e.g., feces and urine), dairy products (e.g., milk and ghee), natural resources (e.g., honey), broken seeds or grains, and their non-edible by-product waste. Here, we aimed to assess the waste recycling and plant biostimulant potential of Kunapajala prepared from livestock (e.g., Black Bengal goats) or fish (e.g., Bombay duck) post-processed wastes over different decomposition periods, e.g., (0, 30, 60, and 90-days). In this study, an in-situ quantification of livestock- (lKPJ) and fish-based Kunapajala (fKPJ) reveals a dynamic landscape of essential plant primary nutrients, e.g., (0.70 > NH4-N < 3.40 g•L−1), (100.00 > P2O5 < 620.00 mg•L−1), and (175.00 > K2O < 340.00 mg•L−1), including other physico-chemical attributes of Kunapajala. Using correlation statistics, we find that the plant-available nutrient content of Kunapajala depicts a significant (p < 0.0001) transformation over decomposition along with microbial dynamics, abundance, and diversities, delineating a microbial interface to animal waste decomposition and plant growth promotion. Importantly, this study also reports the indole 3-acetic acid (IAA) content (40.00 > IAA < 135.00 mg•L−1) in Kunapajala. Furthermore, the bacterial screening based on plant growth-promoting traits and their functional analyses elucidate the mechanism of the plant biostimulant potential of Kunapajala. This assay finally reports two best-performing plant growth-promoting bacteria (e.g., Pseudomonas chlororaphis and Bacillus subtilis) by the 16S ribotyping method. In support, in-planta experiments have demonstrated, in detail, the bio-stimulative effects of Kunapajala, including these two bacterial isolates alone or in combination, on seed germination, root-shoot length, and other important agronomic, physio-biochemical traits in rice. Together, our findings establish that Kunapajala can be recommended as a source of plant biostimulant to improve crop quality traits in rice. Overall, this work highlights Kunapajala, for the first time, as a promising low-cost microbial technology that can serve a dual function of animal waste recycling and plant nutrient recovery to promote sustainable intensification in agroecosystems.
1. Introduction
To meet the increasing global demands for better quality food, intensified crop production in combination with large-scale livestock farming has been contributing to on-farm residue generation, including animal waste, at an astonishingly rapid growth rate in the modern era. India, as an example, produces about 683 million tones of crop residues per year of 10 major Indian crops (Bhattacharjya et al., 2019), while rice and wheat alone contributed around 300 million tones of residue generation recorded in the year 2017–2018 (Venkatramanan et al., 2021). The data on livestock, on the other hand, estimates ~135 million tones of animal excreta generation per year in India, of which more than 90% are from cattle and buffalo sources (Bhattacharjya et al., 2019). In addition, India also accounts for 11% of goat meat and nearly 8% of fish production, ranking as the world's second and third largest producers, respectively (Norris and Smith, 2020). According to the 20th Livestock census data, livestock industries, including fisheries, contribute more than 25% of the total agriculture GDP in India, with an overall increase of 4.6% in production in the last 7 years. The post-processed by-products generated daily in these growing livestock and fish industries in India and the rest of the world, in turn, have led to a gigantic bio-waste accumulation of serious environmental concerns and seek immediate attention for careful management. Recent studies evaluated and discussed the scope of available biomass residues, including leftover agricultural residues and animal and municipal waste, in bioenergy production in India (Singh et al., 2022). In addition, biomass conversion into manures and compost can be an alternate mode of bio-waste management with a possible application in agriculture. Several studies confirmed that bio-waste, including crop residues and animal excreta, are potential sources of essential plant nutrients (e.g., N, P, K) and plant biostimulants (e.g., plant growth-promoting microbes, phytohormones, protein hydrolysates) and can be suitably recycled back into the agroecosystems (Huang et al., 2021). Therefore, the technological services in agriculture and allied sectors have become increasingly instrumental in transforming the circular economy associated with bio-waste management in recent times (Paes et al., 2019; Bakan et al., 2022). At present, advanced technologies, such as anaerobic digestion (AD), composting, algal-based sewage treatment and resource recovery systems (STaRR), are commercially available to promote the recycling of “waste-turn-into-wealth” and are reported to have tremendous potential to minimize the menace of waste hazards, posing environmental and public health risks (Onwosi et al., 2017; Abeysiriwardana-Arachchige et al., 2021; Cremonez et al., 2021). On a similar goal, the technologies that recycle crop residues, animal wastes, and human excreta can provide alternate options to convert bio-degradable solid waste into agricultural inputs in a sustainable way (Ahuja et al., 2020; Kelova et al., 2021; Greff et al., 2022). The main aim of these technologies, in general, is to maximize nutrient recovery and the fertilizer potential of derived by-products. In addition, there are also reports of indirect application-based robust methods for safe recycling in domestic sewage treatment, municipal, and agro-industrial bio-waste for further use in agriculture (Gross et al., 2021; Lin et al., 2021; Ravindran et al., 2021). These technologies, in summary, promote the waste recycling process, create an alternate nutrient cycle, and maintain soil health in agricultural lands, and as a result, can significantly reduce the adverse loads of synthetic fertilizers and pesticides in the agroecosystems.
In India, several traditional formulations have been documented for their application in crop farming for over a 1,000 years. Kunapajala (a Sanskrit word meaning filthy fluid) is an ancient innovation of animal waste recycling into agricultural inputs. This liquid animal manure, narrated originally in “Vrikshayurveda” by Surapala around 1,000 AD, is a formulation of decomposed animal waste such as bones, viscera, fins, and scales from fish waste or waste of crushed bones, skins, and flesh derived from livestock including cattle, goats, pigs, and sheep (Nene, 2018). In addition, locally available resources such as dairy excreta (e.g., cow dung and cow urine), broken or damaged pulse seeds (e.g., green gram or black gram), crop residues, or non-edible by-products obtained after oil extraction (e.g., rice husks and oil cakes), natural or forest resources (e.g., honey), dairy products (e.g., milk and ghee) are also used either as raw or processed materials and most commonly considered as enrichment agents or bulking materials in the Kunapajala preparation (Nene, 2012). Based on end-product trend analyses of animal waste decomposition (Ahuja et al., 2020; Brod and Øgaard, 2021), this low-cost animal manure is assumed to provide a rich source of plant nutrients, the majority of which are in the form of N, P, and K (Chakraborty et al., 2019). Hence, this technology of great potential can serve a dual purpose of sustainable animal waste management and an alternate mode of nutrient cycling in agriculture (Sorathiya et al., 2014). On the other hand, manure composition is dynamic and varies with changing farming circumstances and higher eco-social requirements (e.g., conventional vs. organic farming) (He et al., 2016). Thus, updated knowledge of the oldest manure of India is needed to optimize its recycling potential.
Kunapajala is also an abundant source of plant growth-promoting bacteria (PGPB) that could offer various benefits to its host plants, including nutrient availability, plant growth promotion, and control of pests and diseases (Chakraborty et al., 2019). Therefore, it has been recommended widely as a foliar spray or soil drenching for several crops, such as rice (Oryza sativa), mustard (Brassica campestris), and black gram (Vigna mungo), however with more emphasis on vegetables in India, such as okra (Abelmoschus esculentus), tomato (Solanum lycopersicum), chili (Capsicum annuum), and cowpea (Vigna unguiculata) (Mishra, 2007; Ali et al., 2012; Deshmukh et al., 2012; Sarkar et al., 2014; Kavya and Ushakumari, 2020). In addition, the Kunapajala formulation also showed a significant impact on the growth, physiological, biochemical, yield, and quality attributes of medicinal plants, Ashwagandha (Withania sominifera), and Kalamegha (Andrographis paniculata) (Ankad et al., 2017, 2018). However, despite in-depth physico-chemical and microbiological characterization of Kunapajala (Jani et al., 2017; Chakraborty et al., 2019) and its positive effect on plant growth, no attempt has been made, to date, to understand the population dynamics of microbes in Kunapajala and their functions in animal waste decomposition, nutrient recycling, and mineralization processes. In addition, functional screening of the PGPB isolates, owing to their combinatorial, bio-stimulative impact on the Kunapajala formulation in relation to plant growth and development, remains largely unexplored.
The present study describes the trend analyses of livestock- (lKPJ) and fish-based Kunapajala (fKPJ) throughout decomposition (e.g., 0, 30, 60, and 90-days) to maximize the recovery of resources in terms of available plant nutrients, plant growth regulator content, and as a source of the microbial niche contributing to plant growth-promoting traits such as IAA-production, N-fixation, P- and K-solubilization. We then screened bacteria for their efficiency in plant growth promotion and reported the best-performing isolates using the standard 16S ribotyping method. Finally, this study also assessed the plant biostimulant potential of Kunapajala, including these two bacterial isolates, on different growth stages of rice in a pot-based assay.
2. Materials and methods
2.1. Livestock and fish waste-derived Kunapajala preparation
In order to assess and compare various quality attributes, the preparation of Kunapajala and its components used in this study has been adopted based on the recommendation of Nene, a modified version of the ancient formulation (Jani et al., 2017; Nene, 2018). Here, the Kunapajala samples were prepared separately from two different sources of waste: livestock waste (e.g., Black Bengal goats: Capra hircus) collected from the slaughterhouse; and fish waste (e.g., Bombay duck: Harpadon nehereus) from a local fish market. Subsequently, these wastes were boiled and mixed with other ingredients to prepare the Kunapajala formulation (see Supplementary Table A1 for details). Briefly, livestock and fish waste (having a fresh weight of about 1.25 kg) with crushed bones, fins, skins, and marrows were boiled in 2.5 L of water at 100°C for an hour in two separate containers. After cooling down, the liquid residue was added to 5.0 L of water along with rice husks, available oil cakes (e.g., Mustard: Brassica campestris), and broken or damaged pulse seeds (e.g., Green gram: Vigna radiata). The other ingredients, such as cow dung, cow urine, cow milk, ghee, and honey, were then serially added in the amounts specified to these preparations and were adjusted with water to 50.0 L. The mixture was then kept in the container under shade at room temperature (25–30°C), stirred twice daily during the incubation, and the mouth of the container was further closed with a cloth to facilitate aeration, the prevention of houseflies from laying eggs, and uniform decomposition of the components. Finally, the liquid manures were collected with the help of a fine net and diluted to 100.0 L to prepare the Kunapajala formulation. In this study, the samples were collected for analysis at 30-day intervals, starting from 0-day to 90-days of incubation, e.g., (0, 30, 60, and 90-days).
2.2. The pH, electrical conductivity, organic C, total soluble protein, and macro-nutrient concentrations in the Kunapajala manure
The physico-chemical attributes of the Kunapajala preparation were determined by following the standard methods. In brief, after mixing with deionized water at a 1:100 (v/v) ratio, the pH and the electrical conductivity (EC) of the Kunapajala samples were measured using pH meter (Systronics Digital) and conductivity electrode, respectively. It is important to note that the deionized water has poor conductivity, typically in the range of 10−5-10−6 mS•cm−1, and is therefore assumed to have no influence on the EC measurement. The wet oxidation (Walkley and Black, 1934) and the Lowry (Waterborg, 2009) methods were used to estimate oxidizable organic C and total soluble protein (TSP) content, respectively.
The available N (NH4-N) of Kunapajala was then determined using the standard alkaline-based Kjeldahl technique (Subbiah and Asija, 1956). Similarly, available P (P2O5) and K (K2O) were determined by Olsen et al.'s (1954) and by the flame photometric (Jackson, 1973) methods, respectively.
2.3. The diversity analysis of the microbial community
To determine the microbial population, Kunapajala was serially diluted at the desired concentration and plated with three replications per Petri plate on appropriate culture media so that microbes grow as distinct colonies. Then, the colonies were counted according to standard microbiological norms to study and calculate the microbial dynamics (Mukherjee et al., 2022). The specific growth media for a diverse group of microbes, including their standard cultural conditions, are further enumerated in Supplementary Table A2. The bacteria that appeared as single colonies on specific growth media were streaked further to ensure the purity of cultures for various plant beneficial trait assays.
2.4. The efficacy assay of free-living N-fixers and P-solubilizing bacteria
Free-living N-fixers (FNFs) and P-solubilizing bacteria (PSB), thus obtained as pure colonies on culture plates, were cultured again in nutrient broth to study their plant beneficial traits. Now, to assess the ability of FNFs to fix atmospheric N, the bacteria were grown on a Luria-Bertani culture medium overnight, and then the freshly grown culture was inoculated again to Jansen's media. Finally, 8 mL of grown culture was collected after 7-days of incubation at 30°C for acid digestion to estimate the total N content by the Kjeldahl method (Subbiah and Asija, 1956). On the other hand, to determine the efficiency of PSB in solubilizing insoluble P sources, the diameter of the P-solubilization zone was taken as an indicative measurement during their growth on Pikovskaya's agar media at 30°C (Pikovskaya, 1948; Mukherjee et al., 2022). Furthermore, the efficiency of P-solubilization was again validated quantitatively by Olsen's extraction method (Olsen et al., 1954).
The best-performing FNF and PSB among these two classes of beneficial bacteria obtained in this screening were scored and compared based on their highest N-fixing and P-solubilizing attributes, respectively, and characterized further by subsequent 16S ribotyping and in-planta experiments.
2.5. Estimation of IAA production
The IAA content of the Kunapajala formulation was detected and quantified by the high-performance thin-layer chromatography (HPTLC) method (Goswami et al., 2015). Briefly, the Kunapajala extractants were first isolated using the ethyl acetate solvent. Then, the extractants were loaded on the TLC plate and air-dried. The spots thus formed on the TLC plate were scanned using Scanner 3 (Camag) in absorbance-reflectance mode at a 256 nm wavelength. In the case of the plant beneficial microbes, the bacterial isolates were grown on Luria-Bertani culture media with 0.10 g•L−1 of L-tryptophan. Then, 10 mL of grown culture was collected after 6- and 9-days of incubation to determine the total indole content by the standard Salkowski method (Salkowski, 1885).
2.6. Molecular identification of plant beneficial bacteria
Genomic DNA was extracted from two best-performing plant beneficial bacteria by the lysozyme-mediated lysis method (Moore et al., 2004) with a minor modification. This protocol, however, did not include a preheated NaCl/CTAB solution. Then, using the primers [Forward primer (27F): 5′AGAGTTTGATYMTGGCTCAG3′; Reverse primer (1492R): 5′TACCTTGTTAYGACTT3′], PCR was performed to amplify the 16S rDNA region of the selected bacteria (Edwards et al., 1989). Next, the PCR-amplified products were gel purified according to the manufacturer's protocol (QIAGEN). The purified rDNA fragments thus obtained were sequenced by the Sanger di-deoxy method, and the generated nucleotide sequences were further run to search for homology by the NCBI Nucleotide BLAST program (https://blast.ncbi.nlm.nih.gov/Blast.cgi).
2.7. A plant-based bioassay of Kunapajala and derived bacterial isolates of plant beneficial traits
Seeds of rice (Oryza sativa) from the Shatabdi (IET4786) variety were used in this experiment. Notably, Shatabdi is a popular, high-yielding rice variety cultivated in different agro-climatic zones of West Bengal, India. Studies on germination percentage and root-shoot length were executed by employing a plant-based assay based on the Kunapajala formulation and derived bacterial isolates. In this experiment, the selected seeds were first subjected to surface sterilization by stepwise washing with 70% ethanol and 0.5% hydrogen peroxide solutions for 3 min each, followed by 5 rinses in sterile distilled water. Next, to determine the effect of Kunapajala on germination and other agronomic parameters, sterilized seeds were further soaked and treated with seed priming agents for 6 h. The seed priming agents used in this study were 1% lKPJ and fKPJ formulations after different days of decomposition (e.g., 0, 30, 60, and 90-days), best FNF, and PSB isolated from the Kunapajala preparation, and sterile distilled water as a control. In the case of the best FNF and PSB, a fixed number of bacterial cells was counted (~3 × 1010 cells in 30 mL of sterilized distilled water) and used to treat the seeds. It is important to note that the experiment comprises five replications with 100 seeds per replication. The study on germination percentage was performed on Petri plates under 10 h of light and 14 h of darkness for 7 consecutive days at 25–30°C. The emergence of root-shoot length, including the germination percentage, was carefully observed, recorded, and measured during this period.
The plant growth-promoting abilities of Kunapajala and derived bacterial isolates were evaluated on transplanted rice seedlings in a pot-based experiment. In this experiment, three healthy rice seedlings with a root length of about 3 mm were selected randomly from Petri plates and transplanted further in each earthen pot filled with 2.5 kg of air-dried and autoclaved soil. The details of the nutrient content and physico-chemical and microbiological properties of the agricultural soils used in this study are available in Supplementary Table A3. In this study, we followed a foliar spray of the Kunapajala formulation (e.g., @1% v/v) every 15-day interval up to the flowering stage of rice while considering the plant nutrient content and an earlier recommendation of Kunapajala (Mishra, 2007).
2.8. Agronomic and physico-biochemical attributes of rice seedlings
Shoot height, root length, fresh weight, and dry weight of shoots and roots of a tiller were recorded at every 15-day interval starting from 45-days after transplanting to till harvesting. In addition, other standard agronomic attributes such as the number of tillers per plant, the number of active tillers per plant, the number of grains per panicle, the chaffy: filled grain ratio, the 1,000 seed weight, and yield per plant after harvest were also examined and recorded.
Further, the content of the photosynthetic pigments, such as chlorophyll-a, chlorophyll-b, and total chlorophyll content, was estimated using the standard spectrophotometric method. Briefly, 50 mg of freshly chopped leaves were dipped in 80% acetone in dark conditions for 72 h at 10°C, followed by their intensity measurement at 645 and 663 nm wavelengths. All the quantitative calculations to estimate chlorophyll content follow the standard formula (Tao et al., 2022).
On the other hand, to determine the total carbohydrate and soluble protein content of different plant parts, roots, shoots, and leaves were collected and finely chopped into smaller pieces. Then, 50 mg of finely chopped plant parts were further acid-hydrolyzed to estimate the carbohydrate content by the standard colorimetric method (Jain et al., 2017). In the case of total protein content estimation, the same amount of plant parts was dissolved in phosphate-buffered saline (PBS) and further analyzed by following the Lowry method (Lowry et al., 1951).
2.9. Statistical analysis
Samples collected after different incubation periods were analyzed, with n = 3 in each case unless otherwise specified in the in-planta experiment (i.e., n = 5). The study used Dunnett's tests as a multiple comparison procedure for a significant One-way ANOVA. When the data breached test assumptions (e.g., non-normal data), we employed the non-parametric Kruskal-Wallis test as an alternative to One-way ANOVA. In that case, pairwise comparisons using Dunn's test were used to compare statistical differences among treatments. Significance values were further adjusted by the Bonferroni correction for multiple tests. Analyses were performed in SPSS for Windows 25.0 (IBM SPSS Statistics for Windows, Version 25.0. Armonk, NY: IBM Corp.). Similarly, Pearson's correlation coefficient analysis was performed by a computer-based STAR program (http://bbi.irri.org/products).
3. Results
3.1. Biomass-degrading bacteria: An implication in the Kunapajala technology to recycle animal waste
To explore the microbial action of livestock and fish waste degradation in Kunapajala, we initially studied the microbial dynamics of proteolytic, lipolytic, and starch-hydrolyzing bacteria, henceforth named biomass-degrading bacteria, based on their colony-forming unit (CFU) on appropriate nutrient media (Supplementary Table A2). The idea to examine the dynamics of the starch-hydrolyzing bacterial isolates is due to the crop residues, including rice husks and cow dung, which are present in the Kunapajala formulation. In this study, we observed an elevated level of the biomass-degrading bacterial population (Table 1). However, till 90-days of incubation, there was no reduction observed in the microbial population specifically for this class of bacteria, except for the starch-hydrolyzing bacteria, which showed a decline in the population (Table 1). Overall, our data reveal that the trend in the population dynamics of the biomass-degrading bacteria is amazingly similar in lKPJ and fKPJ.
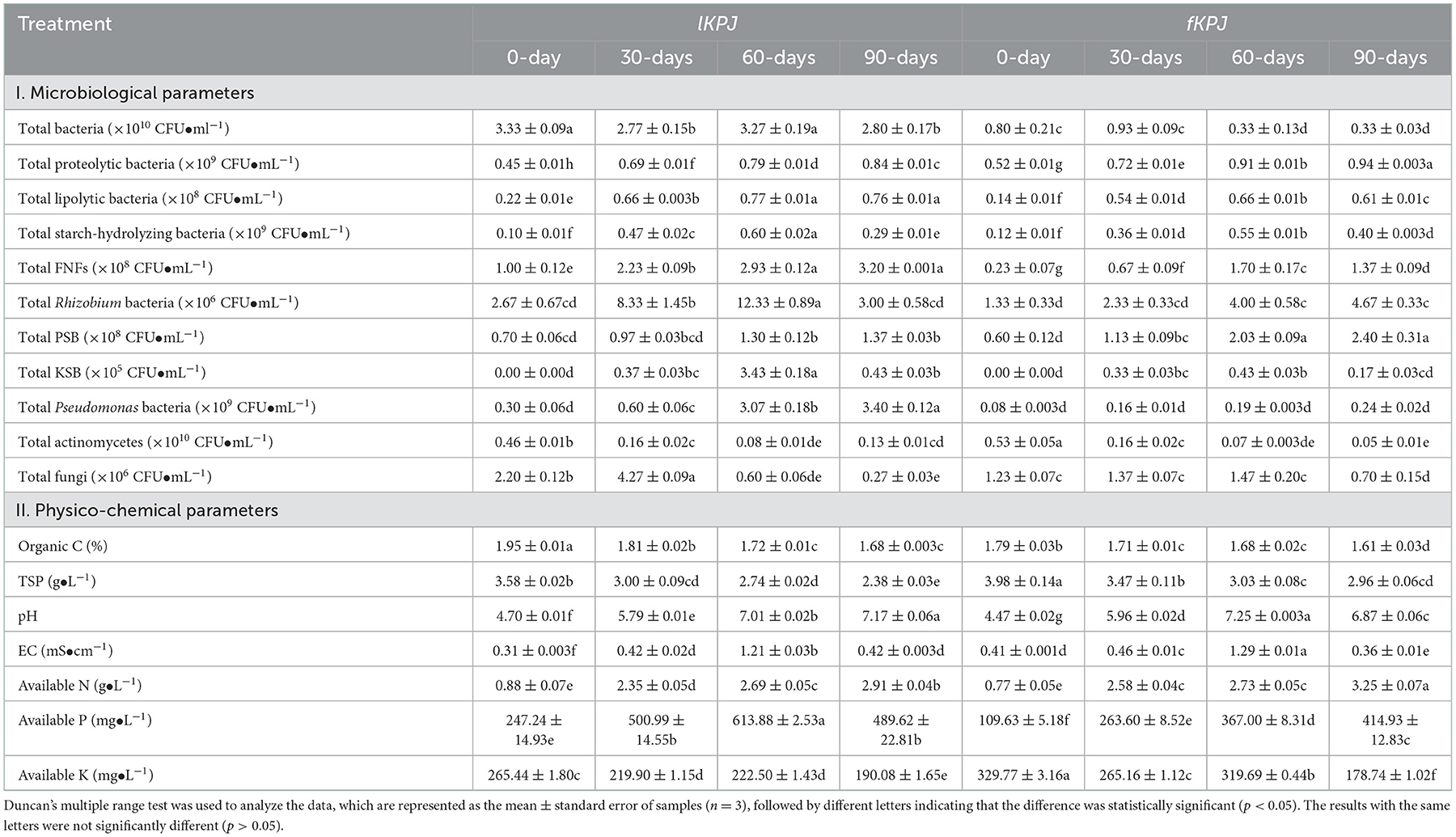
Table 1. The microbiological and physico-chemical properties of Kunapajala after different days of incubation.
To monitor the decomposition process and depolymerization of organic matter subsequently, organic C and TSP were estimated at every 30-day interval till 90-days of incubation. The result indicates that the level of organic C is gradually depleted significantly (p < 0.0001) over time, at least up to 60-days, in both lKPJ and fKPJ (Table 1). It is important to note that the initial concentration of the organic C (in percent value) in two different Kunapajala formulations is in nearly identical ranges (lKPJ: 1.95 ± 0.01%, and fKPJ: 1.79 ± 0.03%). Similarly, the initial TSP content (g•L−1) of the lKPJ and fKPJ formulations ranges between 3.58 ± 0.02 and 3.98 ± 0.14, respectively, and it also shows a similar trend of gradual depletion over a period of incubation. The correlation statistics further establish a positive interaction between microbial growth and animal waste decomposition in the Kunapajala technology (Supplementary Table A4). Together, these data indicate a possible role of microbial action as evident in the abundance of biomass-degrading bacteria in the initial steps of animal waste degradation in Kunapajala.
Besides, other factors such as pH, EC, moisture content, aeration, and temperature are critical in regulating microbial activity and thus may determine the waste decomposition rate in the Kunapajala system (Rastogi et al., 2020). The study revealed that the pH in both of these formulations increased significantly (p < 0.0001) from the initial acidic pH (lKPJ: 4.70 ± 0.01, and fKPJ: 4.47 ± 0.02) to nearly neutral (lKPJ: 7.17 ± 0.06, and fKPJ: 6.87 ± 0.06) over decomposition (Table 1). The EC data (mS•cm−1) in lKPJ and fKPJ, on the other hand, range from 0.31 ± 0.003 to 1.21 ± 0.03 and 0.36 ± 0.01 to 1.29 ± 0.01, respectively, and show no adverse fluctuation to a higher value over incubation (Table 1). Based on physico-chemical and microbiological analyses of Kunapajala, this study highlights for the first time a comprehensive nutrient-microbe network and further reports an amazingly uniform decomposition pattern in Kunapajala, irrespective of animal waste source and heterogeneity.
3.2. Kunapajala is the source of essential plant nutrients: An in-situ resource recycling mode to promote the nitrogen, phosphorus, and potash cycles in agroecosystems
In this study, we further assessed the bio-fertilizer potential of Kunapajala in terms of its plant nutrient concentration. An earlier report indicates Kunapajala formulation as a rich source of plant nutrients based on 40-days of decomposition (Chakraborty et al., 2019). Here, we have extended the earlier observation and evaluated, in particular, the plant nutrient content (e.g., available N, P, and K) of Kunapajala at every 30-day sample till 90-days of incubation. Our data indicate that the concentration of NH4-N and P2O5 increases significantly (p < 0.0001) at 60-days of waste decomposition in both the lKPJ and the fKPJ (Table 1). In comparison over different incubation periods, our study established that the overall concentration of NH4-N, P2O5, and K2O reaches its optimum range at 60-days of incubation in lKPJ and fKPJ (Table 1). These data, in fact, correlate with the uniform animal waste decomposition, including the population dynamics of biomass-degrading bacteria (Supplementary Table A4). These results support a previous report (Chakraborty et al., 2019) that the Kunapajala manure is a rich source of plant nutrients, especially as a plant-available N, P, K (0.70 > NH4-N < 3.40 g•L−1; 100.00 > P2O5 < 620.00 mg•L−1; and 175.00 > K2O < 340.00 mg•L−1). In summary, this study presents Kunapajala as a natural resource restoration technology that may offer enormous potential to provide an alternate source of plant primary nutrients in modern-day agriculture, considering an incremental mining operation followed by the gradual exhaustion of natural resource-based rock phosphate and potash mines and their limited availability in recent years.
3.3. Screening and performance of plant beneficial bacteria isolated from the Kunapajala formulation
We next sought to study the growth dynamics of PGPB, such as FNFs and symbiotic N-fixers (e.g., Rhizobium species), along with PSB and K-solubilizing bacteria (KSB). We observed that the PGPB population ranges between 107 and 108 CFU•mL−1 in the Kunapajala formulation, except for the KSB (Table 1). The population density of Pseudomonas species was reported to be in the 108-109 CFU•mL−1 range, while the KSB and Rhizobium species, on the other hand, were in the ranges of 104-105 and 106 CFU•mL−1 count, respectively (Table 1). In most cases, there is a significant (p < 0.0001) and highest bacterial population observed at 60-days of decomposition, irrespective of the source of animal waste. In addition, our data also confirm that the microbial population of plant beneficial traits exhibits temporal variation in the effects of animal waste source and their decomposition over different days of incubation (Table 1).
The plant beneficial bacteria that appeared were further purified and screened based on their abilities to fix atmospheric N and P-solubilization. We also assume that these PGPB isolates are the most abundant species, given their appearance on their respective culture media after serial dilutions of at least the 10−7 range. In this study, 16 different bacteria (e.g., 9 FNFs and 7 PSB) were screened and selected based on their N-fixation or P-solubilization and evaluated further for their performance owing to other plant growth promotion abilities. We observed that 6 FNFs (designated as KP49, KP52N, KP60, KP82A, KP85, and KP113) showed the highest level of N-fixation (1.05 ± 0.18 > NH4-N < 2.87 ± 0.12 mg•g−1), and the rest 3 bacterial isolates (e.g., KP51, KP109A, and KP109B), however, failed to fix above 1.00 mg•NH4-N•g−1 of sugar consumed (Figure 1). In the case of the PSB, 4 bacterial isolates, such as KP2, KP52P, KP38, and KP19, show a maximum level of inorganic P-solubilization in vitro in both qualitative and Olsen's methods. These bacterial isolates can solubilize inorganic P within the range of 46.39 ± 6.25 > P2O5 < 240.69 ± 18.28 mg•mL−1 after 14 days of their growth in the culture media (Figure 1). Finally, we report two best-performing PGPB (e.g., KP85 and KP19) among these 16 isolates based on their highest N-fixing and P-solubilization abilities. These data, together, reflect a microbial pool of plant beneficial bacteria and their contribution to the plant nutrient niche of Kunapajala.
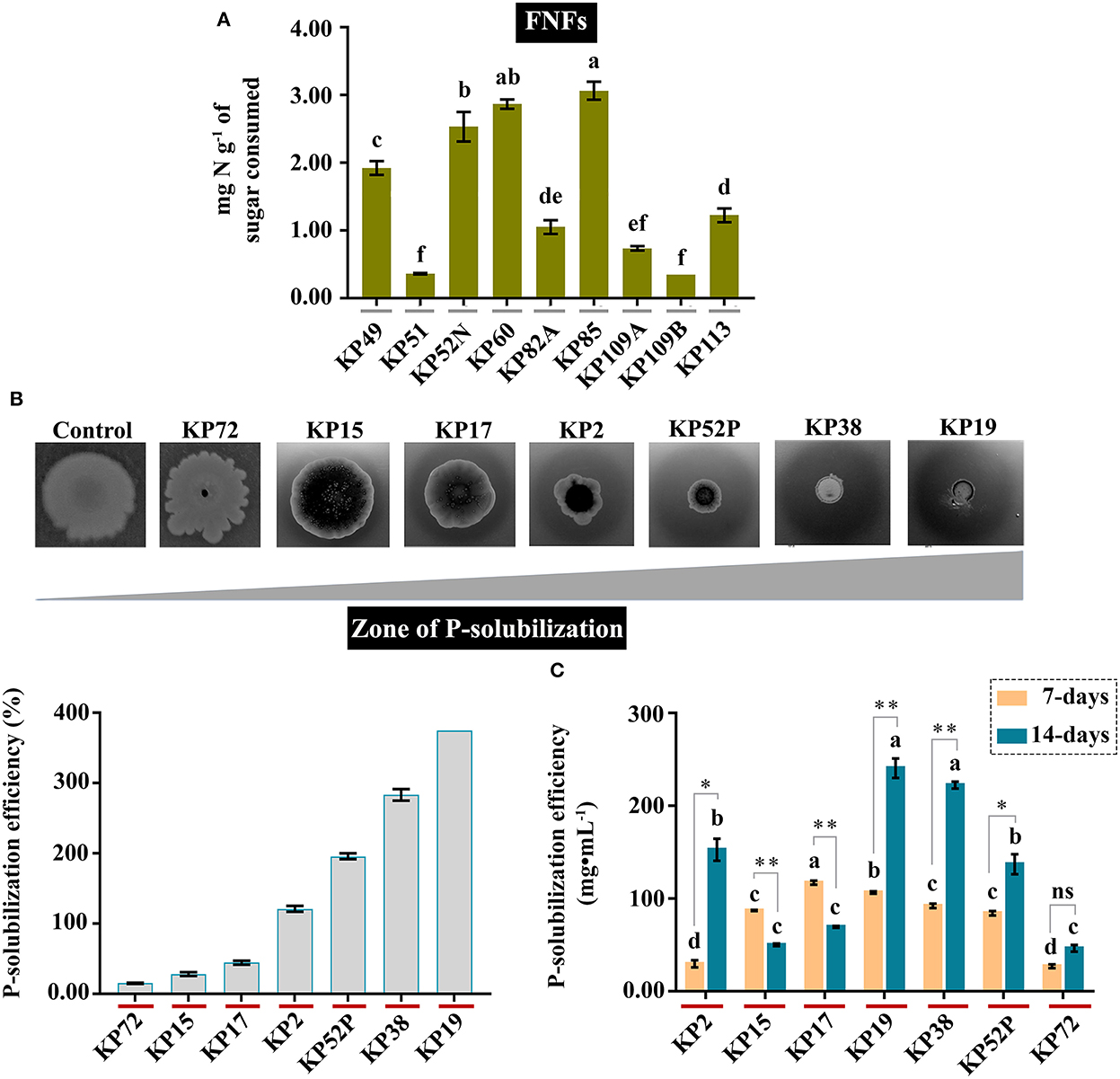
Figure 1. An assay to determine the N-fixing and P-solubilizing efficiency of PGPB isolates from the Kunapajala formulation. (A) The ability of various FNFs to fix N from the atmosphere. The bacterial isolates were grown in Jensen's media and measured further for their N-fixing efficiency by the Kjeldahl method. The y-axis denotes the amount of total N fixed in mg•g−1 of sucrose consumed. (B, C) The P-solubilization ability of PSB. (B) The bacterial isolates were spotted on Pikovskaya's media and incubated for 21 days to measure the zone of P-solubilization. The zone of P-solubilization efficiency (in percent value) was calculated further and plotted. The colonies in the figure are not drawn in scales. (C) The bacterial isolates were grown in liquid Pikovskaya's media, and their P-solubilizing efficiency was measured by the standard spectrophotometric method. The y-axis denotes the amount of phosphorus in mg•mL−1. Here, different letters indicate a statistically significant difference (p < 0.05), and asterisks indicate statistical significance. *p < 0.05; **p < 0.01; ns, non-significant.
3.4. Kunapajala and derived plant beneficial bacterial isolates are efficient sources of IAA production
In this study, we report that Kunapajala is also a potent source of IAA (41.00 ± 1.00 > IAA < 132.33 ± 1.53 mg•L−1), and the concentration of IAA in the lKPJ and fKPJ formulations reached the highest peak after 30- and 60-days of incubation, respectively (Figure 2). The dynamic plot revealed that the IAA concentration depletes sharply after 30-days of decomposition in lKPJ. In the case of fKPJ, however, the concentration of IAA gradually increases up to 60-days of incubation and then declines upon further incubation (Figure 2). Further, we also observed that the IAA concentration and its dynamics are not directly related to the soluble protein content of Kunapajala and the population of proteolytic bacteria that decompose proteins into peptides and free amino acids (Supplementary Table A4).
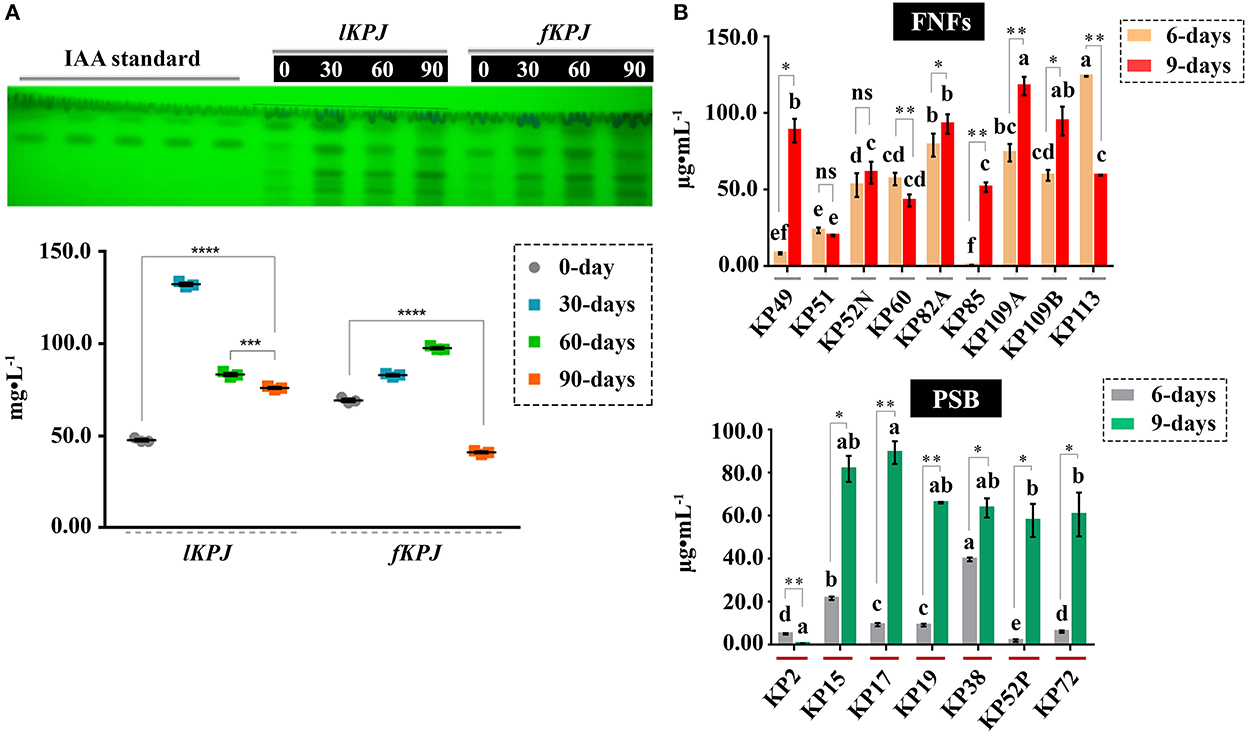
Figure 2. Kunapajala may act as a potential source of IAA. (A) A dynamic plot of the IAA content of Kunapajala. The IAA concentration (in mg•L−1) was estimated from the Kunapajala formulation after different incubation periods by the HPTLC technique. The y-axis denotes the value of three replicates with a standard error of the mean (SEM). (B) The indolic compound synthesis ability of FNFs and PSB. The total indolic compound (in μg•mL−1) was analyzed with three replicates by growing the FNFs and PSB on LB media with 0.10 g•L−1 of tryptophan after 6- and 9-days of incubation. In this figure, different letters indicate a statistically significant difference (p < 0.05), and asterisks indicate statistical significance. *p < 0.05; **p < 0.01; *** p< 0.001; ****p < 0.0001; ns, non-significant.
The other source of IAA in the Kunapajala formulation could be microbial in-vivo IAA synthesis, as bacteria and fungi can also produce IAA besides plants (Spaepen et al., 2007). To elucidate the role of bacteria in IAA production in the Kunapajala formulation, we studied the selected FNFs and PSB to evaluate their ability to synthesize IAA. All 16 bacterial isolates (designated KP49, KP51, KP52N, KP60, KP82A, KP85, KP109A, KP109B, and KP113 from FNFs; and KP2, KP15, KP17, KP19, KP38, KP52P, and KP72 from PSB) were confirmed to have indolic compound synthesis ability in vitro (Figure 2). However, upon screening 16 different bacterial isolates, there was no bias in the distribution of the magnitude of the IAA-production ability, irrespective of their N-fixation and P-solubilization attributes. These bacterial isolates were studied further for their ability to produce IAA (in μg•mL−1) after 6- and 9-days of growth in LB culture media with 0.10 g•L−1 of L-tryptophan (see section Materials and methods). It confirms an overall improvement in IAA synthesis (either p < 0.05 or p < 0.01) after 9-days of growth in most bacterial strains (Figure 2). These results strongly indicate that the bacterial isolates from the class of FNFs and PSB also contribute to the IAA reservoir of the Kunapajala formulation.
3.5. Molecular identification of the best FNF and PSB isolated from Kunapajala
The two best-performing bacterial isolates mentioned earlier were identified further by the 16S rDNA amplicon sequencing technique (Table 2). In this method, the Sanger sequencing reads were obtained for their high-quality sequence coverage by both primers running reversibly. The sequence data thus obtained were joined together to have at least 1.3 kb rDNA sequence coverage by assembling overlapping sequence reads. Finally, the NCBI BLAST program revealed that the best FNF (KP85) and PSB (KP19) show strong sequence homologies with Pseudomonas chlororaphis subsp. aurantiaca and Bacillus subtilis subsp. subtilis, respectively (Table 2).

Table 2. Molecular identification of the best performing bacterial isolates reported from the Kunapajala formulation.
3.6. The biostimulant effects of the Kunapajala formulation on rice seedlings: An agronomic and physio-biochemical trait analysis approach
This study discussed the Kunapajala technology so far in terms of its roles in waste recycling, contribution to nutrient cycles, as a source of plant nutrients, plant growth regulators, and plant beneficial bacteria. These observations stimulate immediate follow-up experiments on the biostimulant effects of this technology on the whole-plant and tissue levels, addressing various agronomic and physio-biochemical traits of rice seedlings. Here, we analyzed, in total, 11 agronomic and 3 physio-biochemical traits in rice, including germination percentage, root-shoot height and weight, yield, total carbohydrates, total proteins, and chlorophyll content. The results revealed a significant difference (p < 0.0001) observed among the treatments of the lKPJ and fKPJ formulations prepared during different decomposition periods (Table 3). We further showed that rice seedlings treated with Kunapajala formulations after 60-days of incubation perform better in their crop quality traits, including yield (Table 3). A trend in the dynamics of almost 14 agronomic and physio-biochemical traits in rice, irrespective of animal waste source, reflects a gradient in the plant biostimulant potential of Kunapajala formulations at least up to 60-days of incubation. This study, in support, indicates a uniform decomposition pattern and microbial dynamics in both lKPJ and fKPJ, owing to their combinatorial impacts on plant biostimulant potential. However, the fKPJ formulation, in essence, acts better to improve the agronomic traits (Table 3). We next sought to study the plant biostimulant potential of the two best-performing bacterial isolates from the Kunapajala formulation. These isolates, alone or in combination, show the best result in crop yield and further quality improvement in rice (Table 3). In conclusion, our study establishes that the Kunapajala formulation, in addition to the derived bacterial strains, could be recommended as a source of plant biostimulants in rice fields.
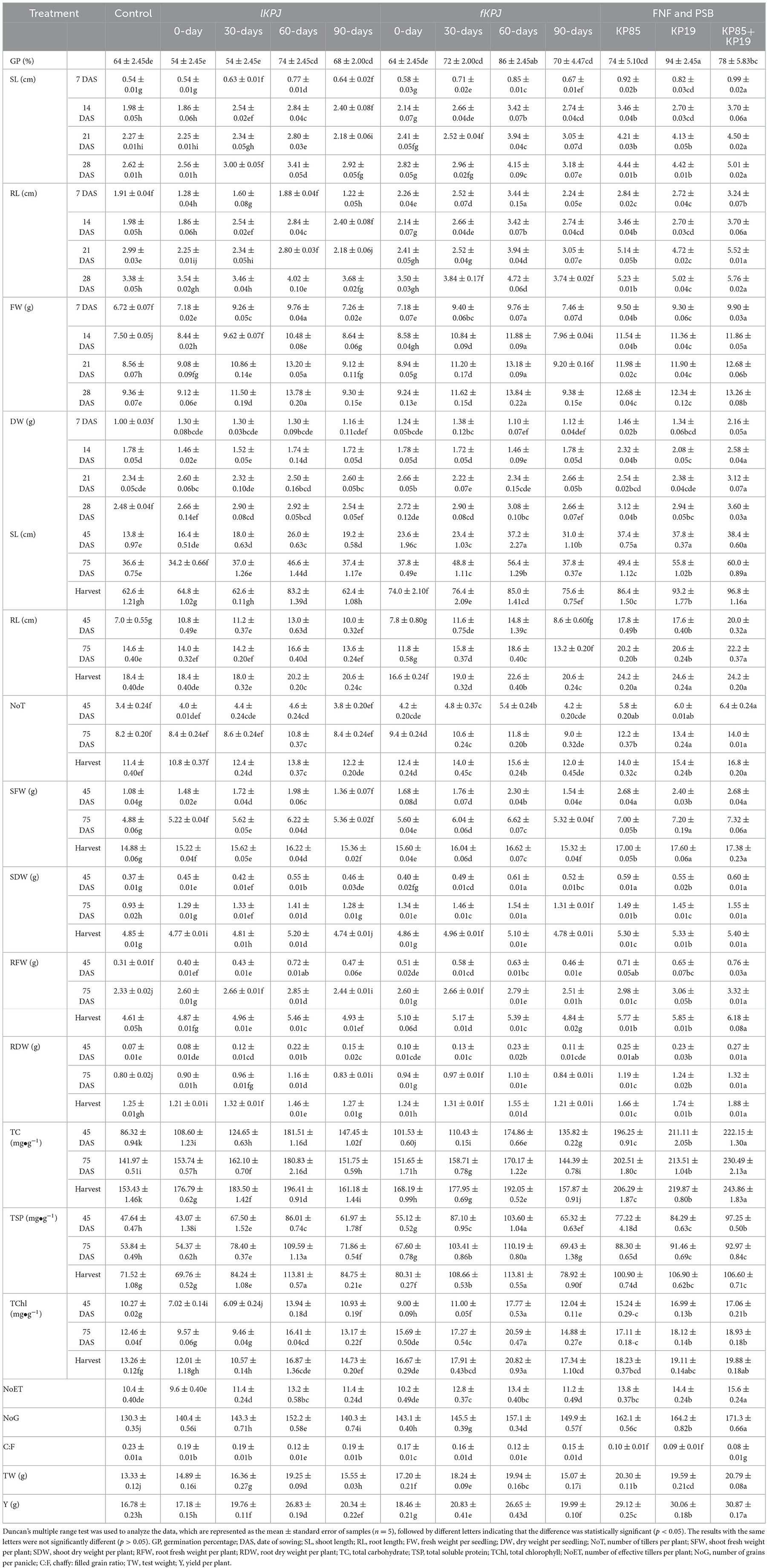
Table 3. The effects of the Kunapajala formulation and two bacterial isolates on various agronomic and physio-biochemical traits of rice.
4. Discussion
4.1. Biodegradable solid waste management and the nutrient potential of Kunapajala
The biomass of livestock and fish tissues is in huge global demand as a high-value nutritive source in the human diet. However, the vast amounts of waste generated due to the processing of meat or fish products, either in the fish market or slaughterhouses, respectively, is a growing problem in the urban-based modern world. It recently appeared that 70 and 60% of processed fish and meat products lead to abattoir waste not fit for human consumption (Natalia et al., 2022). The waste management practice generally employs a segregation strategy that converts a substantive portion of this animal waste into the rendering process as poultry or fish feed (Ominski et al., 2021). The remaining waste, in general, is often disposed of in incineration or landfills, concerning serious hygienic issues and public health. An estimation of over 40 tones of animal waste of daily disposal in India seeks an immediate strategic plan to address the daunting task of solid waste management. In this paper, we have explored the benefits of microbial application in animal waste recycling while revisiting the oldest Kunapajala manure technology. Our results have shown that the presence of biomass-degrading bacteria in the Kunapajala technology speeds up the animal waste degradation process, causing 10–14% organic C depletion and 25–35% TSP decomposition after 90-days of incubation. To support this further, we also observed an overall consistent enrichment of biomass-degrading bacteria within the 107-108 range (CFU•mL−1) throughout the decomposition period. This bacterial niche is, in fact, likely to release several hydrolytic enzymes (e.g., starch-hydrolyzing, proteolytic, and lipolytic) to favor decomposition (Table 1). In support, Bacillus subtilis subsp. subtilis, isolated and characterized from Kunapajala in this study, has been previously reported to secrete amylases, proteases, and lipases (Latorre et al., 2016; Su et al., 2020). Together, it reflects the microbial degradative capacity in Kunapajala, which has turned out to be crucial at the initial stages of the decomposition process. Based on these data, we assume that the Kunapajala technology might provide promising applications in animal waste management.
Animal wastes in the Kunapajala preparation, as stated in other animal manures (Chen et al., 2019), should include complex biomasses of carbohydrates, proteins, lignin, and fat. Consequently, the hydrolysis of these complex bio-molecular fractions often generates a valuable and concentrated source of water-soluble plant nutrients and biologically stable humic substances, which can be added directly to agricultural fields as soil amendments (Huang et al., 2021). In this study, we report the bio-fertilizer potential of the Kunapajala manure in terms of plant-available nutrient conversion recovery and its overall content. In addition, the EC profile in Kunapajala, along with neutral pH after 60-days of decomposition, indicates that it may positively stimulate soil processes, biological activity, and overall impact on ecological health when applied to soils (Kumar and Sharma, 2020). This technology, therefore, could provide an eco-friendly pipeline to create a nutrient cycle both in-situ and ex-situ (Figure 2). The nutrient cycle, in general, is a natural mode of resource management that becomes crucial to balance nutrient recycling and ecosystem health (Harindintwali et al., 2021). Not surprisingly, nutrient mineralization, mobilization, further uptake by crops, and return to soil organic matter provide a foundation for soil health and sustainable crop cultivation. According to IFOAM-Organics International (https://www.ifoam.bio/sites/default/files/2020-03/poa_english_web.pdf), organic farming practices nurture these naturally occurring cycles and boost their ecological benefits, either through agronomic practices or various improved technologies, to achieve sustainable nutrient management (Lorenz and Lal, 2023). The Kunapajala technology as a low-cost valorization approach of animal waste into high-value plant biostimulant may thus appear to act significantly in maintaining soil health and promoting sustainable agriculture.
The safety of the direct application of animal manure to agricultural fields also depends on the toxicity level of heavy metal contamination. Studies predict a high chance of heavy metals cross-transfer to crops, as the concentration above the threshold level could be an alarming issue in animal waste management (da Rosa Couto et al., 2018; Zheng et al., 2022). However, the bio-availability of heavy metals in composts and manures was reportedly lesser in concentration than in anaerobic digestate (Zheng et al., 2022). Therefore, immediate follow-up studies are needed to explore at least two practical aspects of the Kunapajala formulation: (i) periodic monitoring of the quality testing parameter of harmful substances, in particular, heavy metal contamination in the Kunapajala samples; and (ii) accelerate the decomposition rate of animal waste in combination with physical, chemical, or biological treatments. In continuation, future studies may look at the possibility of the black soldier (Hermetia illucens) fly larvae (Lalander et al., 2019; Bortolini et al., 2020) as a biological tool in the Kunapajala formulation to speed up the decomposition process.
4.2. The plant biostimulant potential of Kunapajala and modes of plant growth promotion
The eco-friendly approach of crop cultivation to improve plant biomass and yield is a top priority worldwide in modern-day agriculture. The use of microbial plant biostimulants, such as the beneficial PGPB, to promote climate-resilient crop technology has become widely popular in recent years (Du Jardin, 2015; Fadiji et al., 2022). In this study, we showed Kunapajala as a potential source of the beneficial PGPB, which includes their roles in N-fixation, P-solubilization, and IAA production, owing to their cumulative beneficial impact on the formulation to crop growth (Figures 1, 2; Table 3). We also report the molecular identification of the best-performing bacterial isolates from the Kunapajala source. One of the best-performing isolates, namely Pseudomonas chlororaphis subsp. aurantiaca is known to inhibit the in-planta growth of several important bacterial and fungal phytopathogens (Jain and Pandey, 2016). In addition, these two bacterial species with multiple plant benefits are also non-pathogenic to crop plants and humans (Anderson and Kim, 2018; Su et al., 2020). In this work, we have further established their fitness, alone or in combination, as a biostimulant on rice seedlings. We observed better performance of these bacterial isolates individually or in combination to improve plant performance on yield and other physio-biochemical traits in rice. It seems enigmatic compared to the whole microbial consortium, such as the Kunapajala formulation (Table 3). In the microscopic community, the bacterial species often interact with other microbes and form a complex microbial network that adjoins all possible metabolic interactions among microbes, including antagonisms and symbioses (Han and Yoshikuni, 2022). This interactive microbial metabolic network ultimately deciphers various niche-specific ecological functions, including multiple plant-related benefits to crops. The beneficial microbial consortia are known to improve the performance of agricultural crops while maintaining a healthy agroecological niche and the micro-environment. In recent years, the beneficial PGPB have been considered a promising biotechnological tool to improve crop productivity and enhance crop quality traits irrelevant to its nutrient content (Oleńska et al., 2020). Studies also revealed that the active root zone is the site of abundant microbial diversity based on its composition and function (Ray et al., 2020). Therefore, plant-associated microbiome engineering as bio-fertilizers and bio-pesticides has been a sustainable approach, with added benefits in boosting soil biological activity and fertility (Chojnacka et al., 2020; Mitter et al., 2021). These data, together, establish the earlier recommendation of Kunapajala as a soil drenching. When applied to soils, microbial communities of plant benefits, in essence, build a mutual relation with niche crops and act cooperatively as a cohort of “healthy-peaceful-societies” to circumvent detrimental fluctuations in crop farming, specifically under challenging conditions.
On the other hand, the microbial consortium often shows a lesser effect, as observed in this study, which may indicate the prevalence of antagonistic interactions over the best-performing single or a few beneficial bacterial isolates. Therefore, the combination of effective strains with better stability of similar genera and additive effects on plant growth promotion often termed synthetic microbial communities (e.g., SynComs), becomes more robust and is increasingly recognized in modern agriculture (Yin et al., 2022). In this line of evidence, our results strongly encourage the development of an effective bio-fertilizer derived from these two reported bacteria and a few more similarly effective isolates in the near future. Overall, Kunapajala technology can integrate plants, microbes, and nutrient relationships to maintain ecological harmony and promote natural resource-based sustainable farming.
4.3. Future perspectives on the scaling-up potential of the Kunapajala technology
Kunapajala, as an example, represents an ancient indigenous innovation of India. However, the question remains poised about the practices of such traditional knowledge for adaptation, overall impacts, and vulnerability to the modern economic world. The Kunapajala technology provides two main advantages: first, it requires low-cost investment for infrastructural development and encourages the circular bio-economy of agricultural farms; and second, an animal-based agroecosystem may facilitate its sustainable integration to promote inclusive agriculture and rural socio-economic transformation. This study nevertheless also discusses its pivotal role in animal waste recycling. In recent years, the Swachh Bharat (Clean India; https://swachhbharat.mygov.in/) Mission has been gearing up to generate public awareness and policies about the waste disposal and segregation mechanisms followed by their management into potential applications like energy, fertilizer, animal feed, chemical, and leather industries. The Government of India also launched a flagship initiative, Startup India (https://www.startupindia.gov.in/), in order to foster innovative ideas and passion for building up an inclusive ecosystem among entrepreneurs. This industry-academia knowledge exchange platform may aid in planning ideas according to local needs, industrial viability, and national policy plans. The Kunapajala technology, in this context, would be more appropriate and socially viable to add up in animal waste management, particularly in the Indian context. In addition, the technological operation of Kunapajala, starting with animal waste management to broader applications in agriculture, would also create unique linkages for its strategic implementation, especially in agriculture-based nations.
In India and other parts of the world, organic farming has been growing stupendously as an alternate model of crop cultivation aiming to achieve greater economic viability and environmental sustainability (Meemken and Qaim, 2018; Das et al., 2020). The principle of organic farming practices often emphasizes the safe and efficient recycling of natural resources, including on-farm residues, to counterbalance the antagonistic effects of agrochemicals, maintain ecological harmony, and conserve biodiversity. In order to substitute the chemical inputs, which are essential resources in conventional agriculture, organic agriculture relies on composts and manures of “true” organic standards and origin. In this study, cow dung and urine, the potential sources of organic matter and microbial consortia, have been collected from the in-house dairy farm, practicing conventional (non-organic) animal farming and therefore fails to qualify as “true” organic manure. Hence, organic animal farming, including poultry and fish, has become an integrated part of this ecological farming system (He, 2020). To monitor the “true” organic standards in complex food chains, the certification process of organic products and farms continues to evolve in the United States and other countries, including India. Therefore, stating terms such as organic manure, organic agriculture, and alike must be carefully considered and discussed. Hence, the Kunapajala formulation, often widely coined as organic manure in the past in India, must now qualify for the organic standards and pass through the certification process before being termed organic manure for commercial purposes and scientific agricultural practices.
India is, however, now on the verge of introducing chemical-free natural farming based on on-farm resource recycling and dairy excreta-based microbial formulations (https://www.niti.gov.in/natural-farming-niti-initiative). Surely, Kunapajala technology would get wider acceptance as a regenerative input under the new Indian agricultural policy. On the other hand, according to the United Nations' Sustainable Development Goals (https://sdgs.un.org/goals), our planet would experience almost 90% of global deforestation primarily due to the expansion of agricultural practices, including crop farming and livestock grazing. It consequently leads to rapid natural resource depletion, agro-pollution, biodiversity loss, unpredictable climate vulnerability, and eventually severe crop loss and yield, having short- and long-term negative impacts on the planet and human health. Therefore, sustainable intensification and ecosystem services in agriculture grab immediate global attention while strengthening the traditional culture and practices to maintain agroecosystem resilience, along with the “inclusive” strategic integration of natural resource utilization and scientific implementation of niche-specific innovations and technologies (Jhariya et al., 2021). For instance, careful animal waste utilization generated from animal farms into recycled animal manure will be far more effective for environment-friendly, sustainable agriculture (He et al., 2020). Therefore, traditional innovations, such as Kunapajala technology, could be revisited and assessed to promote sustainable intensification and address ecosystem services across diverse agroecological and socio-economic domains. Hopefully, in the coming years, a more holistic inter-sectoral approach to building a resilience system on the scaling-up potential of Kunapajala at par commercial standards would contemplate viable solutions in the socio-economic development encompassing agriculture-environment-public health nexus.
5. Conclusions
In this study, we revisited and assessed the waste recycling potential of Kunapajala manures prepared from livestock or fish wastes over different decomposition periods. We have further shown Kunapajala to be a dynamic formulation of essential plant primary nutrients and a rich source of PGPB and their metabolic network. This study also elucidates the mechanism of plant growth promotion in Kunapajala, including the molecular identification of two plant beneficial bacteria. Based on the 16S ribotyping method, the two best-performing bacterial isolates show strong sequence homology with Pseudomonas chlororaphis subsp. aurantiaca and Bacillus subtilis subsp. subtilis. Overall, this work provides the first mechanistic insight into the plant biostimulant potential of Kunapajala and its further in-planta validation from seed germination to sprouting shoots and roots to grain production in rice.
Data availability statement
The generated nucleotide sequences have been deposited into the NCBI database under the accession 538 numbers: OM698822 (KP19) and OM698823 (KP85).
Author contributions
SM: conceptualization, methodology, validation, investigation, resources, and writing—review and editing. AB and AC: methodology, validation, investigation, resources, and writing—review and editing. RG: methodology, formal analysis, and writing—review and editing. KR, MNA, HB, and JL: formal analysis and writing—review and editing. SS: methodology and resources. AKH and ST: methodology, resources, and writing—review and editing. ASP: writing—review and editing, project administration, and fund acquisition. NR: conceptualization, writing—review and editing, project administration, and fund acquisition. MAA: writing—review and editing and project administration. GC: conceptualization, methodology, formal analysis, investigation, supervision, writing—original draft, project administration, and fund acquisition. All authors contributed to the article and approved the submitted version.
Funding
This work was supported by the All India Network Programme on Organic Farming (AINP-OF), funded by the Indian Council of Agricultural Research (ICAR) (Ref. No. F. No. 1-42/NPOF/201), and the Government of India.
Acknowledgments
RG, KR, AKH, and GC acknowledged the Ramakrishna Mission Vivekananda Educational and Research Institute (RKMVERI). The facilities offered by the RKMVERI and the ICAR to accomplish this study are duly acknowledged.
Conflict of interest
The authors declare that the research was conducted in the absence of any commercial or financial relationships that could be construed as a potential conflict of interest.
Publisher's note
All claims expressed in this article are solely those of the authors and do not necessarily represent those of their affiliated organizations, or those of the publisher, the editors and the reviewers. Any product that may be evaluated in this article, or claim that may be made by its manufacturer, is not guaranteed or endorsed by the publisher.
Supplementary material
The Supplementary Material for this article can be found online at: https://www.frontiersin.org/articles/10.3389/fsufs.2022.1073010/full#supplementary-material
References
Abeysiriwardana-Arachchige, I. S. A., Samarasingha, N., Rosalez, R., Munasinghe-Arachchige, S. P., Delanka-Pedige, H. M. K., Brewer, C. E., et al. (2021). Maximizing phosphorus recovery as biofertilizer in an algal wastewater treatment system. Resour. Conserv. Recycl. 170, 105552. doi: 10.1016/j.resconrec.2021.105552
Ahuja, I., Dauksas, E., Remme, J. F., Richardsen, R., and Løes, A. K. (2020). Fish and fish waste-based fertilizers in organic farming – with status in Norway: a review. Waste Manag. 115, 95–112. doi: 10.1016/j.wasman.2020.07.025
Ali, M. N., Chakraborty, S., and Paramanik, A. (2012). Enhancing the shelf life of Kunapajala and Shasyagavya and their effects on crop yield. Int. J. Bioresour. Stress Manag. 3, 289–294.
Anderson, A. J., and Kim, Y. C. (2018). Biopesticides produced by plant-probiotic Pseudomonas chlororaphis isolates. Crop Protect. 105, 62–69. doi: 10.1016/j.cropro.2017.11.009
Ankad, G. M., Hiremath, J., Patil, R. T., Pramod, H. J., and Hegde, H. V. (2017). Evaluation of Kunapa jala and Pancha gavya on plant quality, physiology, biochemistry, yield and growth attributes - a case study of Withania somnifera Dun. J. Ayurv. Integr. Med. 8, 247–251. doi: 10.1016/j.jaim.2017.01.004
Ankad, G. M., Hiremath, J., Patil, R. T., Pramod, H. J., and Hegde, H. V. (2018). Nutrient analysis of Kunapa jala and Pancha gavya and their evaluation on germination of Ashwagandha and Kalamegha seeds: a comparative study. J. Ayurv. Integr. Med. 9, 13–19. doi: 10.1016/j.jaim.2017.01.011
Bakan, B., Bernet, N., Bouchez, T., Boutrou, R., Choubert, J. M., Dabert, P., et al. (2022). Circular economy applied to organic residues and wastewater: research challenges. Waste Biomass Valor. 13, 1267–1276. doi: 10.1007/s12649-021-01549-0
Bhattacharjya, S., Sahu, A., Manna, M. C., and Patra, A. K. (2019). Potential of surplus crop residues, horticultural waste and animal excreta as a nutrient source in the central and western regions of India. Curr. Sci. 116, 1314–1323. doi: 10.18520/cs/v116/i8/1314-1323
Bortolini, S., Macavei, L. I., Saadoun, J. H., Foca, G., Ulrici, A., Bernini, F., et al. (2020). Hermetia illucens (L.) larvae as chicken manure management tool for circular economy. J. Clean. Prod. 262,121289. doi: 10.1016/j.jclepro.2020.121289
Brod, E., and Øgaard, A. F. (2021). Closing global P cycles: the effect of dewatered fish sludge and manure solids as P fertiliser. Waste Manag. 135, 190–198. doi: 10.1016/j.wasman.2021.08.041
Chakraborty, B., Sarkar, I., Maitra, S., Khan, A. M., Bandyopadhyay, S., and Sinha, A. K. (2019). Nutritional and microbial analytical study of Vedic liquid organic manure cum pesticide Kunapajala with different storage time interval. Int. J. Pharmacog. 6, 209–215.
Chen, X., Liu, R., Hao, J., Li, D., Wei, Z., Teng, R., et al. (2019). Protein and carbohydrate drive microbial responses in diverse ways during different animal manures composting. Bioresour. Tech. 271, 482–486. doi: 10.1016/j.biortech.2018.09.096
Chojnacka, K., Moustakas, K., and Witek-Krowiak, A. (2020). Bio-based fertilizers: a practical approach towards circular economy. Bioresour. Tech. 295, 122223. doi: 10.1016/j.biortech.2019.122223
Cremonez, P. A., Teleken, J. G., Meier, T. R. W., and Alves, H. J. (2021). Two-stage anaerobic digestion in agroindustrial waste treatment: a review. J. Environ. Manag. 281, 111854. doi: 10.1016/j.jenvman.2020.111854
da Rosa Couto, R., Comin, J. J., Souza, M., Ricachenevsky, F. K., Lana, M. A., Gatiboni, L. C., et al. (2018). Should heavy metals be monitored in foods derived from soils fertilized with animal waste? Front. Plant Sci. 9, 732. doi: 10.3389/fpls.2018.00732
Das, S., Chatterjee, A., and Pal, T. K. (2020). Organic farming in India: a vision towards a healthy nation. Food Qual. Saf. 4, 69–76. doi: 10.1093/fqsafe/fyaa018
Deshmukh, R. S., Patil, N. A., and Nikam, T. D. (2012). Influence of Kunapajala treatment from vrikshyaurveda on leaves of tomato (Lycopersicon esculentum l. Cv. Selection 22) and its comparison with conventional farming and organic farming. IOSR J. Pharmacy 2, 55–63. doi: 10.9790/3013-25405563
Du Jardin, P. (2015). Plant biostimulants: definition, concept, main categories and regulation. Scientia Horti. 196, 3–14. doi: 10.1016/j.scienta.2015.09.021
Edwards, U., Rogall, T., Blocker, H., Emde, M., and Bottger, E. C. (1989). Isolation and direct complete nucleotide determination of entire genes. Characterization of a gene coding for 16S ribosomal RNA. Nucl. Acids Res. 17, 7843–7853. doi: 10.1093/nar/17.19.7843
Fadiji, A. E., Babalola, O. O., Santoyo, G., and Perazzolli, M. (2022). The potential role of microbial biostimulants in the amelioration of climate change-associated abiotic stresses on crops. Front. Microbiol. 12, 829099. doi: 10.3389/fmicb.2021.829099
Goswami, D., Thakker, J. N., and Dhandhukia, P. C. (2015). Simultaneous detection and quantification of indole-3-acetic acid (IAA) and indole-3-butyric acid (IBA) produced by rhizobacteria from L-tryptophan (Trp) using HPTLC. J. Microbio. Methods 110, 7–14. doi: 10.1016/j.mimet.2015.01.001
Greff, B., Szigeti, J., Nagy, Á., Lakatos, E., and Varga, L. (2022). Influence of microbial inoculants on co-composting of lignocellulosic crop residues with farm animal manure: a review. J. Environ. Manag. 302, 114088. doi: 10.1016/j.jenvman.2021.114088
Gross, T., Breitenmoser, L., Kumar, S., Ehrensperger, A., Wintgens, T., and Hugi, C. (2021). Anaerobic digestion of biowaste in Indian municipalities: effects on energy, fertilizers, water and the local environment. Resour. Conserv. Recycl. 170, 105569. doi: 10.1016/j.resconrec.2021.105569
Han, S. W., and Yoshikuni, Y. (2022). Microbiome engineering for sustainable agriculture: using synthetic biology to enhance nitrogen metabolism in plant-associated microbes. Curr. Opin. Microbiol. 68, 102172. doi: 10.1016/j.mib.2022.102172
Harindintwali, J. D., Zhou, J., Muhoza, B., Wang, F., Herzberger, A., and Yu, X. (2021). Integrated eco-strategies towards sustainable carbon and nitrogen cycling in agriculture. J. Environ. Manag. 293, 112856. doi: 10.1016/j.jenvman.2021.112856
He, Z. (2020). “Organic animal farming and comparative studies of conventional and organic manures,” in Animal Manure: Production, Characteristics, Environmental Concerns, and Management, Vol. 67, eds H. M. Waldrip, P. H. Pagliari, Z. He, 165–182.
He, Z., Pagliari, P. H., and Waldrip, H. M. (2016). Applied and environmental chemistry of animal manure: a review. Pedosphere 26, 779–816. doi: 10.1016/S1002-0160(15)60087-X
He, Z., Pagliari, P. H., and Waldrip, H. M. (2020). “Advances and outlook of manure production and management,” in Animal Manure: Production, Characteristics, Environmental Concerns, and Management, Vol. 67, eds H. M. Waldrip, P. H. Pagliari, Z. He, 373–383.
Huang, S., Zheng, X., Luo, L., Ni, Y., Yao, L., and Ni, W. (2021). Biostimulants in bioconversion compost of organic waste: A novel booster in sustainable agriculture. J. Clean. Prod. 319, 128704. doi: 10.1016/j.jclepro.2021.128704
Jain, R., and Pandey, A. (2016). A phenazine-1-carboxylic acid producing polyextremophilic Pseudomonas chlororaphis (MCC2693) strain, isolated from mountain ecosystem, possesses biocontrol and plant growth promotion abilities. Microbiol. Res. 190, 63–71. doi: 10.1016/j.micres.2016.04.017
Jain, V. M., Karibasappa, G. N., Dodamani, A. S., and Mali, G. V. (2017). Estimating the carbohydrate content of various forms of tobacco by phenol-sulfuric acid method. J. Educ. Health Promot. 6, 90. doi: 10.4103/jehp.jehp_41_17
Jani, S., Prajapati, P. K., Harisha, C. R., and Patel, B. R. (2017). Kunapajala a liquid organic manure: preparation and its quality parameters. World J. Pharma. Pharmaceut. Sci. 6, 1989–2000. doi: 10.20959/wjpps20178-9865
Jhariya, M. K., Banerjee, A., Meena, R. S., Kumar, S., and Raj, A. (2021). “Sustainable intensification for agroecosystem services and management: an overview,” in Sustainable Intensification for Agroecosystem Services and Management, eds M. K. Jhariya, A. Banerjee, R. S. Meena, S. Kumar, A. Raj (Singapore: Springer), 1–35.
Kavya, S. R., and Ushakumari, K. (2020). Effect of organic liquid manure of Kunapajala on growth and yield of Bhindi [Abelmoschus esculentus (L.) Moench.]. Agril. Science Digest. 40, 270–274. doi: 10.18805/ag.D-5083
Kelova, M. E., Eich-Greatorex, S., and Krogstad, T. (2021). Human excreta as a resource in agriculture – evaluating the fertilizer potential of different composting and fermentation-derived products. Resour. Conserv. Recycl. 175, 105748. doi: 10.1016/j.resconrec.2021.105748
Kumar, P., and Sharma, P. K. (2020). Soil salinity and food security in India. Front. Sustain. Food Syst. 4, 533781. doi: 10.3389/fsufs.2020.533781
Lalander, C., Diener, S., Zurbrügg, C., and Vinnerås, B. (2019). Effects of feedstock on larval development and process efficiency in waste treatment with black soldier fly (Hermetia illucens). J. Clean. Prod. 208, 211–219. doi: 10.1016/j.jclepro.2018.10.017
Latorre, J. D., Hernandez-Velasco, X., Wolfenden, R. E., Vicente, J. L., Wolfenden, A. D., Menconi, A., et al. (2016). Evaluation and selection of Bacillus species based on enzyme production, antimicrobial activity, and biofilm synthesis as direct-fed microbial candidates for poultry. Front. Vet. Sci. 3, 95. doi: 10.3389/fvets.2016.00095
Lin, X., Li, S., Wei, Z., Chen, Y., Hei, L., and Wu, Q. T. (2021). Indirect application of sludge for recycling in agriculture to minimize heavy metal contamination of soil. Resour. Conserv. Recycl. 166, 105538. doi: 10.1016/j.resconrec.2020.105358
Lorenz, K., and Lal, R. (2023). “Introduction to organic agriculture,” in Organic Agriculture and Climate Change, eds K. Lorenz and R. Lal (Cham: Springer), 1-38.
Lowry, O. H., Rosebrough, N. J., Farr, A. L., and Randall, R. J. (1951). Protein measurement with the folin phenol reagent. J. Biol. Chem. 193, 265–275. doi: 10.1016/S0021-9258(19)52451-6
Meemken, E., and Qaim, M. (2018). Organic agriculture, food security, and the environment. Annu. Rev. Resour. Econ. 10, 39–63. doi: 10.1146/annurev-resource-100517-023252
Mishra, P. K. (2007). Effects of Kunapajalam Vrikshayurveda on growth of paddy. Ind. J. Tradit. Know. 6, 307–310.
Mitter, E. K., Tosi, M., Obregón, D., Dunfield, K. E., and Germida, J. J. (2021). Rethinking crop nutrition in times of modern microbiology: innovative biofertilizer technologies. Front. Sustain. Food Syst. 5, 606815. doi: 10.3389/fsufs.2021.606815
Moore, E., Arnscheidt, A., Krüger, A. S., Strömpl, C., and Mau, M. (2004). “Simplified protocols for the preparation of genomic DNA from bacterial cultures,” in Molecular Microbial Ecology Manual, eds G. A. Kowalchuk, F. J. de Bruijn, I. M. Head, A. D. L. Akkermans, J. D. van Elsas (London: Kluwer Academic Publishers), 1.01, 3–18.
Mukherjee, S., Sain, S., Ali, M. N., Goswami, R., Chakraborty, A., Ray, K., et al. (2022). Microbiological properties of Beejamrit, an ancient Indian traditional knowledge, uncover a dynamic plant beneficial microbial network. World J. Microbiol. Biotech. 38, 111. doi: 10.1007/s11274-022-03296-3
Natalia, Q. P., Cristina, R. T., and Germán, B. E. (2022). Lipolytic effect of Staphylococcus warneri for obtaining high-quality fishmeal from fish waste fermentation. Waste Biomass Valor. 13, 2519–2530. doi: 10.1007/s12649-021-01668-8
Nene, Y. L. (2012). Potential of some methods described in Vrikshayurvedas in crop yield increase and disease management. Asian Agri History 16, 45–54.
Nene, Y. L. (2018). The concept and formulation of Kunapajala, the world's oldest fermented liquid organic manure. Asian Agri History 22, 8–14. doi: 10.18311/aah/2018/v22i1/18292
Norris, A. B., and Smith, W. B. (2020). “Farming characteristics and manure management of small ruminant and cervid livestock,” in Animal Manure: Production, Characteristics, Environmental Concerns, and Management, Vol. 67, eds H. M. Waldrip, P. H. Pagliari, and Z. He (John Wiley & Sons Ltd.), 129–144.
Oleńska, E., Małek, W., Wójcik, M., Swiecicka, I., Thijs, S., and Vangronsveld, J. (2020). Beneficial features of plant growth-promoting rhizobacteria for improving plant growth and health in challenging conditions: a methodical review. Sci. Total Environ. 743, 140682. doi: 10.1016/j.scitotenv.2020.140682
Olsen, S. R., Cole, C. V., Watanabe, F. S., and Dean, L. A. (1954). Estimation of Available Phosphorus in Soils by Extraction With Sodium Bicarbonate, Vol. 939. Washington, DC: US Department of Agriculture, 19.
Ominski, K., McAllister, T., Stanford, K., Mengistu, G., Kebebe, E. G., Omonijo, F., et al. (2021). Utilization of by-products and food waste in livestock production systems: a Canadian perspective. Anim. Front. 11, 55–63. doi: 10.1093/af/vfab004
Onwosi, C. O., Igbokwe, V. C., Odimba, J. N., Eke, I. E., Nwankwoala, M. O., Iroh, I. N., et al. (2017). Composting technology in waste stabilization: on the methods, challenges and future prospects. J. Environ. Manag. 190, 140–157. doi: 10.1016/j.jenvman.2016.12.051
Paes, L. A. B., Bezerra, B. S., Deus, R. M., Jugend, D., and Battistelle, R. A. G. (2019). Organic solid waste management in a circular economy perspective–a systematic review and SWOT analysis. J. Clean. Prod. 239, 118086. doi: 10.1016/j.jclepro.2019.118086
Pikovskaya, R. I. (1948). Mobilization of phosphorus in soil connection with the vital activity of some microbial species. Microbiol. 17, 362–370.
Rastogi, M., Nandal, M., and Khosla, B. (2020). Microbes as vital additives for solid waste composting. Heliyon 6, e03343. doi: 10.1016/j.heliyon.2020.e03343
Ravindran, B., Karmegam, N., Yuvaraj, A., Thangaraj, R., Chang, S. W., Zhang, Z., et al. (2021). Cleaner production of agriculturally valuable benignant materials from industry generated bio-wastes: a review. Bioresour. Tech. 320, 124281. doi: 10.1016/j.biortech.2020.124281
Ray, P., Lakshmanan, V., Labb,é, J. L., and Craven, K. D. (2020). Microbe to microbiome: a paradigm shift in the application of microorganisms for sustainable agriculture. Front. Microbiol. 11, 622926. doi: 10.3389/fmicb.2020.622926
Salkowski, E. (1885). Uber das verhalten der skatolcarbonsaure im organismus. Z. Physiol. Chem. 9, 23–33. doi: 10.1515/bchm1.1885.9.1.23
Sarkar, S., Kundu, S. S., and Ghorai, D. (2014). Validation of ancient liquid organics - Panchagavya and Kunapajala as plant growth promoters. Ind. J. Tradit. Know. 13, 398–403.
Singh, A. D., Gajera, B., and Sarma, A. K. (2022). Appraising the availability of biomass residues in India and their bioenergy potential. Waste Manag. 152, 38–47. doi: 10.1016/j.wasman.2022.08.001
Sorathiya, L. M., Fulsoundar, A. B., Tyagi, K. K., Patel, M. D., and Sing, R. R. (2014). Eco-friendly and modern methods of livestock waste recycling for enhancing farm profitability. Int. J. Recycl. Org. Waste Agricult. 3, 50. doi: 10.1007/s40093-014-0050-6
Spaepen, S., Vanderleyden, J., and Remans, R. (2007). Indole-3-acetic acid in microbial and microorganism-plant signaling. FEMS Microbiol. Rev. 31, 425–448. doi: 10.1111/j.1574-6976.2007.00072.x
Su, Y., Liu, C., Fang, H., and Zhang, D. (2020). Bacillus subtilis: a universal cell factory for industry, agriculture, biomaterials and medicine. Microb. Cell Fact. 19, 173. doi: 10.1186/s12934-020-01436-8
Subbiah, B. V., and Asija, G. L. (1956). A rapid procedure for the estimation of available nitrogen in soils. Curr. Sci. 25, 259–260.
Tao, L., Qiuhong, L., Fuqiang, Y., Shuhui, Y., Suohui, T., and Linyuan, F. (2022). Plant growth-promoting activities of bacterial endophytes isolated from the medicinal plant Pairs polyphylla var. yunnanensis. World J. Microbiol. Biotechnol. 38, 15. doi: 10.1007/s11274-021-03194-0
Venkatramanan, V., Shah, S., Rai, A. K., and Prasad, R. (2021). Nexus between crop residue burning, bioeconomy and sustainable development goals over North-Western India. Front. Energy Res. 8, 614212. doi: 10.3389/fenrg.2020.614212
Walkley, A., and Black, I. A. (1934). An examination of the Degtjareff method for determining soil organic matter, and a proposed modification of the chromic acid titration method. Soil Sci. 37, 29–38. doi: 10.1097/00010694-193401000-00003
Waterborg, J. H. (2009). “The Lowry method for protein quantitation,” in The Protein Protocols Handbook, ed J. M. Walker (Totowa, NJ: Humana Press), 7–10.
Yin, C., Hagerty, C. H., and Paulitz, T. C. (2022). Synthetic microbial consortia derived from rhizosphere soil protect wheat against a soilborne fungal pathogen. Front. Microbiol. 13, 908981. doi: 10.3389/fmicb.2022.908981
Keywords: Kunapajala, animal waste, waste recycling, nutrient recovery, plant growth regulators (PGRs), plant growth-promoting bacteria (PGPB)
Citation: Mukherjee S, Basak A, Chakraborty A, Goswami R, Ray K, Ali MN, Santra S, Hazra AK, Tripathi S, Banerjee H, Layek J, Panwar AS, Ravisankar N, Ansari MA and Chatterjee G (2023) Revisiting the oldest manure of India, Kunapajala: Assessment of its animal waste recycling potential as a source of plant biostimulant. Front. Sustain. Food Syst. 6:1073010. doi: 10.3389/fsufs.2022.1073010
Received: 18 October 2022; Accepted: 28 December 2022;
Published: 19 January 2023.
Edited by:
Sanjay Singh Rathore, Indian Agricultural Research Institute (ICAR), IndiaReviewed by:
Sowmyalakshmi Subramanian, McGill University, CanadaPravin Kumar Upadhyay, Indian Agricultural Research Institute (ICAR), India
Zhongqi He, Agricultural Research Service (USDA), United States
Copyright © 2023 Mukherjee, Basak, Chakraborty, Goswami, Ray, Ali, Santra, Hazra, Tripathi, Banerjee, Layek, Panwar, Ravisankar, Ansari and Chatterjee. This is an open-access article distributed under the terms of the Creative Commons Attribution License (CC BY). The use, distribution or reproduction in other forums is permitted, provided the original author(s) and the copyright owner(s) are credited and that the original publication in this journal is cited, in accordance with accepted academic practice. No use, distribution or reproduction is permitted which does not comply with these terms.
*Correspondence: Meraj A. Ansari, bWVyYWppYXJpQGdtYWlsLmNvbQ==; Gautam Chatterjee,
Z2F1dGFtY2hhdHRlcmplZTg0QGdtYWlsLmNvbQ==
†Present addresses: Sayantan Santra, Department of Agricultural and Food Engineering, Indian Institute of Technology Kharagpur, Kharagpur, India
Alok Kumar Hazra, G.D. Pharmaceuticals Pvt. Ltd., Kolkata, India
Sudipta Tripathi, School of Environment and Disaster Management, Ramakrishna Mission Vivekananda Educational and Research Institute, Ramakrishna Mission Ashrama-Narendrapur, Kolkata, India
‡These authors have contributed equally to this work and share first authorship