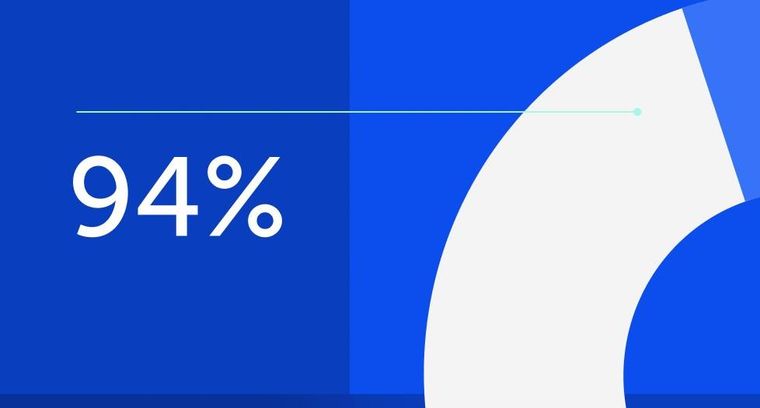
94% of researchers rate our articles as excellent or good
Learn more about the work of our research integrity team to safeguard the quality of each article we publish.
Find out more
ORIGINAL RESEARCH article
Front. Sustain. Food Syst., 18 January 2023
Sec. Sustainable Food Processing
Volume 6 - 2022 | https://doi.org/10.3389/fsufs.2022.1071821
This article is part of the Research TopicSustainable Processing and Preservation of Underutilized Indigenous FoodsView all 7 articles
Introduction: Ukwa (Treculia africana) is an indigenous edible seed. It is a strategic store of vital food nutrients that are available during a specific crucial time of the year when reliable sources of these nutrients are under cultivation and extremely scarce. In the past, only poor rural residents used to eat it, but today it is not only a specialty meal enjoyed by Nigeria's elite and metropolitan residents but also a source of foreign exchange.
Methods: In this study, Ukwa was processed into whole, dehulled, malted and defatted flours. Maize and coconut flours were blended with the respective Ukwa flours at the ratio of 0:95:5, 20:75:5, 25:70:5, 30:65:5, 35:60:5 and 95:0:5 for Ukwa flour:Maize flour:Coconut grits respectively for development of snack bars. A two-factor factorial experiment in a completely randomized block design was applied for the study of the effect of processing, substitution levels of Ukwa flour and the interaction of the two variables on the responses analyzed. Anti-nutrient content and proximate compositions of the flours were determined using standard procedures.
Results: Processing significantly reduced the anti-nutrient content and increased protein content of Ukwa flours. Up to 51.72% reduction of tannin was obtained by dehulling. Defatting, malting and dehulling resulted in 18.75, 34.37 and 65.62% reduction respectively in oxalate content. Highest reduction was obtained by dehulling, and was 70.69% in phytate, 79.95% in saponin, and 48.17% in trypsin inhibitor activity. Crude protein content of snack bars had 16.16 to 25.46% substitution main effect, and 19.43 to 22.65% processing main effect. In-vitro protein and starch digestibility increased with processing and decreased with increase in substituted level of Ukwa in the blend. Improvement, up to 9.97% by dehulling, 9.86% by malting and 8.64% by defatting, was recorded in in-vitro protein digestibility. An increase of 3.00 to 24.10% by defatting, 5.90 to 29.09% by malting and 9.70 to 31.80% by dehulling was recorded in in-vitro starch digestibility.
Discussion: Our study revealed that, all the processing methods adopted reduced the anti-nutrient content of ukwa flours. Protein content and total essential amino-acid (TEAA) showed significant increase with increased substituted level of ukwa flour. In-vitro protein and starch digestibility decreased with increase substituted level of ukwa flour. Snack bars formulated with 20% Ukwa showed the highest in-vitro starch and protein digestibility irrespective of the method of processing. Malted Ukwa based snack bars recorded the highest values of TEAA. Processing of this nutritious seeds and use of its flour to develop snack bars could enhance utilization and give convenience to consumers and encourage extensive farming of the crop.
Food processing enhances the quality of food because, in the process, foodstuffs are made into forms that are more acceptable and different completely from the original food in appearance, taste, flavor, texture, and aroma. All food processing unit operations are procedures to achieve the desired changes in the raw materials. The combination of operations determines the nature of the final products (Brennan et al., 1970). Food processing is basically aimed at extending shelf-life by the use of preservatives to inhibit the growth of microbiological or occurrence of biochemical changes; eliminating anti-nutrient factors; removing contaminants from food; making available a wide variety of food products with attractive flavors, colors, aroma, and texture; and providing nutrients that are required for healthy living (Fellows, 1990; Enwere, 1998). Enhancing the qualities of food can be achieved through various means, such as drying, blanching, defatting, dehulling, fermenting, sprouting, or malting among other methods.
Convenience food is an eclectic category of processed food, manufactured for mass consumption, including chilled, dried, and canned foods; confectionary, snacks, and beverages; and processed meat, pasta, and cheese, ready to eat, ready to cook, and ready to serve. The very first definition of convenience food was proffered by Raj et al. (2021). They added that any food which has work prepared outside the home can be regarded as convenience food. According to Jabs and Devine (2006), convenience food is deemed as domestic outsourcing of food planning, preparation, and cooking. They also stressed that convenience food required very little preparation related to home cooking.
Ukwa (Treculia africana), commonly known as African breadfruit, is an evergreen tree with great potential as a source of nutrients to man (Osabor et al., 2009).
Ukwa is a rich source of protein (17–20%), carbohydrates (40%), oil (10%), and minerals such as magnesium, potassium, zinc, iron, calcium, sodium, copper, and vitamins (Osabor et al., 2009). Its protein has a fairly balanced composition of amino acids, with a lysine content higher than that in wheat (Nwabueze, 2007). In Nigeria, Ukwa is popular with the Igbo communities, and the seeds are eaten as snacks when toasted or roasted and as porridge when cooked with ingredients (Nwabueze et al., 2008). Its flour could be used as a soup thickener (Iwe and Ngoddy, 2000). As snacks, toasted Ukwa could be eaten with fresh maize or mature coconut. Runsewe et al. (2001) reported that Ukwa porridge improved the health conditions of malnourished children without any adverse effects. Despite its dense nutrient composition, Ukwa is under-utilized, partly because of the current lifestyle and demand for convenience, and the laborious steps and time involved in its processing. Expanding applications of Ukwa would increase its utility and versatility. One such application is processing into high fiber snack bars (Edima-Nyah et al., 2019), extruded snacks (Nwabueze et al., 2008), and malt for use in alcoholic beverages, and ethanol production (Nwabueze and Uchendu, 2011).
Maize (Zea mays L.) is a cereal with the highest production worldwide (Gwirtz and Garcia-Casal, 2014) and is extensively cultivated in Nigeria. Maize is considered a staple food generally in Africa, particularly in Nigeria. It feeds ~50% of the population. Maize is an important source of carbohydrates, proteins, and minerals such as iron and the B vitamins. There are ~40 different ways of maize consumption recorded in African countries (Nago et al., 1990).
Coconut is respected in history because of its nutritious nature. It is rich in fiber, vitamins, and minerals. Due to the numerous health benefits derived from its nutritional content, coconut is regarded as a “functional food.” Many food uses or products exist for coconut. The primary product is copra, and the white “meat” is found adhering to the inner wall of the shell. It can be dried to 2.5% moisture content, shredded, and used in candies and cakes, and other confections (Okafor and Ugwu, 2014). Coconut oil is expressed from copra, which is used in a variety of cooked foods and margarine production. Coconut is a source of quick energy for physical performance and athletic exercises (Bruce-Fife, 2010).
In view of the increasing demand for convenience foods, the objective of this study was to investigate the effect of processing and substituted levels of Ukwa (Treculia africana) on the anti-nutrient factors, proximate composition, in vitro protein digestibility, in vitro starch digestibility, and total essential amino acid content of the developed snack bars made from Ukwa, maize, and coconut to deliver a nutritious health product. The outcome of this study would help in determining the suitability of these developed snack bars as good complementary diets, increase utilization, and consumption beyond traditional ceremonies and attempt to make Ukwa-based snacks available to interested consumers.
Ukwa was purchased from Ndoro market, Umudike, and Nigeria. Maize (white dent variety) was obtained from Uyo main market. Coconuts were obtained from a local farmer in Uyo, Nigeria.
Ukwa seeds were cleaned, parboiled for 15 min at 100°C, drained through stainless steel sieve, and allowed to cool. Parboiled Ukwa seeds were dried for 5 h at 60°C and toasted for 20 min at 150°C in an oven (Precision Compact, Model: PR305225M). Toasted seeds were milled using a Colombian Grain Mill (Victoria, Model: 530025) to flour. The whole Ukwa flour was stored in a clean container with a secured lid at room temperature (27 ± 2°C).
Ukwa seeds, previously washed and drained, were parboiled for 15 min at 100°C (for facilitating seed coat separation from the endosperm). Draining of parboiled seeds was done using stainless steel sieve and allowed to stand for 20 min to effect cooling and also soften the seed coat. Dehulling was performed by cracking the grains using Grain Mill (Victoria, Model: 530025, Colombia) and sorting to obtain clean dehulled seeds. Dehulled seeds were dried for 5 h at 60°C in an oven (Precision Compact, Model: PR305225M), and their temperature increased to 150°C for the toasting of the seeds for 20 min. Toasted seeds were milled to flour with a manual mill (Victoria Grain Mill, Model Ref: 530025), packaged, and stored at ambient temperature (27 ± 2°C) in a clean plastic container with a secured cover.
Processing procedures to obtain malted Ukwa flour (MUF) were carried out according to the method described by Edima-Nyah et al. (2022). The seeds were washed with potable water and steeped for 24 h, changing the liquor at 8-h intervals to reduce microbial load. This would prevent the embryo from suffocating due to the depletion of oxygen. Steeping was terminated by draining the liquor and spreading the seeds on a sterilized jute bag, placed on a laboratory bench. Germination was carried out at room temperature for 7 days and was terminated by kilning in an oven at 45°C for 12 h. The temperature was later increased to 60°C for 6 h for drying. The dried malted seeds were then toasted in the oven for 20 min at 150°C and milled to flour using a manual mill (Victoria Grain Mill, Model: 530025, Colombia). The flour was stored at ambient temperature (27 ± 2°C) in a clean, dry plastic container with a secured lid.
Clean Ukwa was parboiled, drained, dried, toasted, and milled to flour using the same conditions described for whole Ukwa flour (WUF). The flour was defatted using the method described by Nwabueze and Iwe (2010) for defatting soybean flour. Food grade ethanol was used to soak the flour in the ratio of 1:3 for 3 h and centrifuged for 15 min at 4,000 rpm. The supernatant was decanted off leaving the semi-solid mass, after standing for some time. The semi-solid flour mass was then spread in pans and placed under the fan for 30 min to reduce the ethanol concentration therein. It was further dried for 24 h in an oven at 60°C to completely desolventizing the ethanol residue in the flour. The dried defatted flour was milled using a manual mill (Victoria Grain Mill, Model: 530025, Colombia) to break flour lumps. The dry defatted Ukwa flour (FUF) was packaged in a clean dry container with a secured lid, labeled, and stored at ambient temperature.
Maize grains were processed into flour according to the procedures outlined by Edima-Nyah et al. (2019).
Mature coconuts were harvested, dehusked, and cracked. Coconut flesh was removed manually from the hard endocarp using a stainless steel knife, with a sharp and pointed edge. The procedure, described by Edima-Nyah et al. (2019), was adopted for processing coconut grits.
Processed Ukwa flour, maize flour, and coconut grits were blended in the ratio of 0:95:5, 20:75:5, 25:70:5, 30:65:5, 35:60:5, and 95:0:5.
Six snack bar samples were prepared, each based on each of the composite flours previously blended. From each composite flour, 100 g of flour was weighed out. In addition, 25 g of caramel, 15 g of margarine, 10 g of coconut oil, 5 g of milk powder, 2 g of baking powder, 2 g of nutmeg, and 0.2 g of common salt were blended with 100 g of each composite flour. Each blend was mixed with 40 g of potable water.
Production of snack bars was according to the procedures described by Edima-Nyah et al. (2019). The flour and other baking ingredients were mixed manually in a stainless steel bowl for ~3 min, for a uniform mixture. The liquid ingredients (coconut oil and caramel) were added next and mixed for another 3 min, and then, water was slowly added until the whole dough was mixed to obtain a uniform dough. The dough was placed in greased pans and compressed with a spatula to obtain a uniform mass and then covered. The dough was oven-baked at 150°C for 25 min. Products were cooled (~60°C), de-panned, cut into bars (5 cm × 3 cm × 2 cm), dried to reduce the moisture content in an air-circulation oven for 6 h at 60°C, and packaged and stored at ambient temperature (27 ± 2°C) in the laboratory for further analyses.
Tannin, phytate, and trypsin inhibitor activity contents of flour samples were determined by standard spectrophotometric methods of Pearson (1976). Oxalate and tannin determinations were determined by methods of Oberleas (1973) and Onwuka (2005), respectively.
The proximate composition of raw materials and snack bars was determined using standard methods (AOAC, 2005) for moisture content, and crude fat, crude protein, total ash, crude fiber, and carbohydrate and calorific (energy) values were calculated according to the method described by Osborne and Voogt (1978).
An enzymatic method described by Kanu et al. (2009) was adopted for the determination of in vitro protein digestibility of snack bars produced.
The method of analysis described by Singh et al. (2012), using enzymes, was used for the determination of in vitro starch digestibility of snack bars.
The amino acid profile of Snack bars produced was determined by the method of Benitez (1989). The samples were dried to a constant weight and hydrolyzed after defatting. Evaporation was carried out in a rotary evaporator, before loading into an applied bio-system (PTH) amino acid analyzer (model: 120A PTH, England). The total essential amino acid was calculated as the sum of cysteine, histidine, isoleucine, leucine, lysine, methionine, phenylalanine, threonine, tryptophan, tyrosine, and valine, as measured in g/100 g protein.
Data obtained were analyzed using IBM SPSS software, version 20, with two-way analysis of variance (ANOVA) for the determination of significance (P < 0.05) between means, and separated with the Duncan multiple range test.
Anti-nutrient contents of flours are presented in Table 1. Tannin content ranged from 0.27% to 0.58%, with whole Ukwa flour (WUF) showing the highest and dehulled (DUF) showing the least concentrations, respectively. Processing significantly (P < 0.05) lowered tannin content. ~32.76% reduction in tannin concentration of flour was recorded by defatting, 43.10% reduction by malting, and 51.72% reduction by dehulling. Percentage reduction by dehulling was higher than those reported in the literature by Alonso et al. (2000) in Faba bean (35% reduction in Shambat 616; 43% reduction in SML 85/1/1). The reduction in tannins by the dehulling process could be due to the removal of the hulls since most tannins are located in the hulls. The concentrations of tannins in the flour recorded in this study posed no health risk since the accepted lethal dose reported is 0.09% (Ifie and Emeruwa, 2011; Maseta et al., 2016). Tannins are high oligomeric molecules of polyphenol that occur in plants naturally (Adeoti et al., 2017). They inhibit digestive enzymes by binding with carbohydrates and proteins, thereby reducing their bioavailability (Ayodele and Kigbu, 2003).
Table 1. Anti-nutrient content of whole, dehulled, malted, and defatted Ukwa flours, maize flour, and coconut grits.
Oxalate content in flours ranged from 0.11 mg/100 g to 0.33 mg/100 g (Table 1). Dehulled Ukwa flour showed the lowest value (0.11 mg/100 g) followed by the malted (0.21 mg/100 g) and defatted flour (0.26 mg/100 g) in increasing order. There was no significant difference between the defatted flour (FUF) and coconut grits (COF). WUF had the highest concentration (0.33 mg/100 g) of oxalate. Dehulling, malting, and defatting processes resulted in 65.62%, 34.37%, and 18.75% reduction in oxalate content of Ukwa flours. The amount of oxalate in the processed flours could not be toxic under meal portions since they were lower than the safe level (15–30 g/100 g food consumed) reported in the literature for a man (Coe et al., 2005).
The Phytate content of flours ranged from 2.09 mg/100 g to 7.42 mg/100 g. A significant difference (p < 0.05) existed in the phytate contents of all the flour samples. Maize flour (WMF) had the highest value (7.42 mg/100 g), followed by whole Ukwa flour (WUF), while dehulled flour (DUF) had the lowest value. ~70.69% reduction was obtained by dehulling, while 52.73% and 21.18% reduction was obtained by malting and defatting processes, respectively. Thapliyal et al. (2014) reported a 14.39–20.87% reduction for chickpeas, and Wang et al. (2008) reported a decrease of 5.3–8.0% in field peas due to dehulling. The concentrations of phytate in the flours were lower than the considered lethal amount to human health, 250 mg/100 g, reported in the literature (Nagel, 2010; Maseta et al., 2016). According to Kumar et al. (2010), phytates, when at high levels in human foods, may limit the bioavailability and utilization of minerals such as iron, calcium, magnesium, and manganese by forming compounds that are insoluble and indigestible.
The saponin content of flours ranged from 2.23% to 11.12%. Dehulled Ukwa flour showed the lowest value, followed by the defatted flour (3.61%) and the malted (3.83%) in decreasing order. A significant difference existed in the saponin contents of all the flour samples. Whole Ukwa flour showed the highest value (11.12%), followed by maize flour (10.21%). The processing treatments resulted in a 79.95% reduction in saponin by dehulling, a 69.15% reduction by malting, and a 67.54% reduction by defatting. Adeoti et al. (2017) reported saponin content of 9.54–18.50 mg/100 g for Akee apple seed and ariel flour, while 5.20 mg/g was reported for flour from seeds of M. utilis (Seena, 2006). Saponin is known to have both bad and good effects on human health. By reacting with sterols on the membranes of erythrocytes, saponin shows hemolytic activities (Bauman et al., 2000) and has hypocholesterolemic properties (Oakfenfall and Sidu, 1990).
Trypsin inhibitor activity in the flours ranged from 0.95 TIU/mg to 13.76 TIU/mg. Coconut grits (COF) had the lowest (0.95 TIU/mg) concentration, followed by maize flour and WMF (1.03 TIU/mg) in increasing order. Whole Ukwa flour (WUF) had a significantly higher value (13.76 TIU/mg), followed by the MUF (10.81 TIU/mg) and FUF (10.04 TIU/mg) in decreasing order. Processing of Ukwa resulted in a 48.17% reduction by dehulling, 27.25% reduction by malting, and 27.11% reduction by defatting trypsin inhibitor factors. Thapliyal et al. (2014) reported a 7.72%−16.72% reduction after dehulling chickpea, while Wang et al. (2008) recorded a 5.3%−13.1% reduction in trypsin inhibitor after dehulling field pea. Steinkraus (2002) reported a decrease in the toxic effect of trypsin inhibitors due to sprouting.
Moisture, ash, crude fat, crude fiber, crude protein, carbohydrate, and the energy value of the whole, dehulled, malted, and defatted Ukwa flours, maize flour, and coconut grits are shown in Table 2. The moisture content of Ukwa flour ranged from 3.48% to 3.80%, with dehulled flour having the lowest value. Coconut grits (COF) had the highest moisture, 4.86%. Coconut has been shown to have hygroscopic or water-absorbing properties (Wasserman, 2016), and this could be the reason for having the highest moisture content. The moisture content obtained in this study was lower than those reported by earlier researchers: 9.00% for unmalted and 11.00% for malted African breadfruit seed flour by Nwabueze and Atuonwu (2007); 8.00% and 6.00% for unmalted and malted African breadfruit seed flour, respectively, by Nwabueze and Uchendu (2011), and 10.72% for raw, 9.64% for cooked, 7.70% for parboiled, and 6.80% for toasted African breadfruit seed flour by Emenonye (2016). Moisture content lower than 10% is considered within the standard category (James, 1995). The moisture content of flour samples may be of advantage in prolonged storage, with proper packaging (Rodge et al., 2012; Feili et al., 2013).
Table 2. Proximate composition and energy values of the whole, dehulled, malted, and defatted Ukwa flours, maize flour, and coconut grits.
The Ash content of maize flour was 1.83%, while that of coconut grits was 6.28%. Malted Ukwa flour (MUF) showed the highest ash content (3.52%), followed by the dehulled (DUF, 3.29%), defatted (FUF, 3.07%), and whole (WUF, 3.14%). Dehulling, malting, and defatting significantly (P < 0.05) increased the ash content of Ukwa. Ash content is an estimation of the composition of minerals in a sample (Emenonye, 2016). Ash contents recorded in the study were higher than the results of earlier researchers: 2.50% and 2.75% for unmalted and malted African breadfruit seed flour reported by Nwabueze and Atuonwu (2007), 2.26% by Osabor et al. (2009), 2.80% for unmalted and 2.40% for malted reported by Nwabueze and Uchendu (2011), and 2.79% and 2.88% for raw and toasted seeds, respectively, as reported by Emenonye (2016). However, values close to those of this research were obtained by Nwabueze (2007) for raw African breadfruit seed flour (3.00%) and Emenonye (2016) for parboiled (3.22%) and cooked (3.52%) African breadfruit seed flour.
Crude fat content ranged from 5.83% to 11.52% in Ukwa flours. The fat contents of 11.52% for malted, 11.24% for whole, 11.09% for dehulled, and 5.83% for defatted samples were obtained. ~a 48% reduction in fat was recorded by the defatting process. These fat contents were lower compared to those reported by Emenonye (2016) for raw (12.49%), parboiled (13.24%), and toasted (16.24%) African breadfruit seed flour. It was the same (11.45%) as reported by Nwabueze (2007). Maize flour showed the least value (4.79%), while coconut grits (42.12%) were the highest in fat content. The percentage of the crude fat content of coconut was highest because it was used as full-fat, not defatted.
Crude fiber content ranged from 7.73% to 20.18%. Maize flour had the lowest value (7.73%), followed by dehulled Ukwa flour (DUF) (7.77%) and coconut grits (10.67%). Gwirtz and Garcia-Casal (2014) reported about the same fiber value (7.3%) for maize flour. Malted Ukwa flour was observed to have the highest crude fiber content (20.18%). The high fiber contents of the Ukwa flour could have been a result of using the whole seeds (without removing the hulls) in the flour production. These values were higher than those reported in the literature: 3.00% by Nwabueze (2007) for raw dehulled seed flour; 3.30% for malted and 2.80% for unmalted African breadfruit seed flour by Nwabueze and Atuonwu (2007); 1.46% and 1.90% for malted and unmalted African breadfruit seed flour, respectively, by Nwabueze and Uchendu (2011); and 1.31% for raw, 1.38% for cooked, 4.96% for parboiled, and 6.79% for toasted African breadfruit seed flour by Emenonye (2016). The values obtained in this research were desirable since high fiber foods are said to benefit the heart, lower the risk of blocked arteries, heart attack, and stroke, as well as reduce appetite, thus protecting against obesity (Schill and Munz, 2011), whereas low fiber diets are not desirable since they may cause constipation and are implicated with the disease of the colon such as pile, hemorrhoids, appendicitis, and even cancer (O'Keefe, 2019). Coconut fiber stands out as more important than other fiber sources because it has no phytic acid, and so prevents the removal of minerals but increases their absorption in the body. Coconut fiber decreases the rate of emptying the stomach of foods, thereby allowing food a long time in the stomach for mineral release, and subsequent high mineral availability for body absorption (Wasserman, 2016).
Crude protein content ranged from 20.48% to 23.32% for Ukwa flours. The protein contents obtained in this research were higher compared to those reported (15.76%) by Nwabueze (2007) and 15.10%, 16.30%, and 18.00% reported by Emenonye (2016) for cooked, parboiled, and toasted African breadfruit seed flour, respectively. Maize flour showed the least crude protein content (9.65%), while the coconut grits showed the highest (27.11%). The protein content of maize flour was higher than that reported (6.9%) by Gwirtz and Garcia-Casal (2014). The difference could be due to varietal differences in the maize used. The crude protein and crude fat content of all the Ukwa flours could qualify them as valuable sources of nutrients. The results are in line with previous reports in the literature (Iwe and Ngoddy, 2000; Odemelam, 2000; Nwabueze, 2007; James and Nwabueze, 2013a). These results suggest that Ukwa could be important for a developing country like Nigeria.
The Carbohydrate content of maize flour was significantly highest (72.18%), while that of coconut grits was lowest (10.95%) among all the samples. The Carbohydrate content of Ukwa flours ranged from 37.70% to 52.14%, with DUF showing the highest value, while the MUF had the lowest. Activities of endogenous enzymes leading to the release of bound carbohydrates from the non-nutritive complexes of the seeds could increase the carbohydrate content of flour (Ugwu and Oranye, 2006; Emenonye, 2016). The carbohydrate contents of DUF and maize flour (WMF) were lower than those reported by Nwabueze (2007): 60.59% for dehulled raw seed flour and 73.58% for maize flour. Emenonye (2016) reported carbohydrate contents of 52.69%, 54.58%, 60.23%, and 49.30% for raw, parboiled, cooked, and toasted African breadfruit seed flours, respectively.
The energy content of flours ranged from 302.76 Kcal/100 g to 531.41 Kcal/100 g. The energy value of coconut grits (COF) was significantly higher, while that of malted Ukwa flour (MUF) was lower than in other samples. The amount of energy in food, required for the maintenance of basic body functions such as physical exercise, breathing, regulation of body temperature, and circulation of blood, are represented in energy value (Edima-Nyah et al., 2019).
Table 3 shows the effect of treatments employed in the processing of Ukwa flours and the level of substitution on the protein content of maize–coconut snack bars. The protein content of snack bars ranged from 16.16% to 27.15% and increased with the increasing substituted level of Ukwa in the snack bar formulations. Processing increased the protein content of snack bars. The malted and the dehulled Ukwa snack bars showed the highest values of protein at different levels of blending interchangeably. Snack bars produced from whole Ukwa had the least values of protein at all levels of substitution, followed by those from defatted Ukwa. Significant differences (P < 0.05) also existed in the processing method's main effect and the composition's main effect. Processing resulted in a significant increase in the protein content of all the snack bars. The increase could be attributed to the increase in proteolytic activities and the accumulation of microbial mass. The breakdown of complex compounds, during the germinating process, into a simple form may have caused the increase. Protein increase by malting could also be a result of the solubilization of compounds in the flour and changes in the structure of storage protein (glutelins and prolamins) exposing them to enzymatic reactions (Nwabueze and Atuonwu, 2007). The increase in protein content by the defatting process could be attributed to the breakdown of protein–lipid linkages, and subsequent release of protein molecules, which eventually increases the value of crude. Proteins are important in tissue building. All the snack bar formulations show good protein content and may be regarded as a good protein source.
Table 3. Effect of processing and substituted levels of Ukwa flours on crude protein content (%) of maize–coconut snack bars.
Analysis of variance, developed from factorial experiment, shows the effect of the model terms and the reliability of the model in modeling and predicting the crude protein response. All model terms (treatments, substitution of African breadfruit seed flour, and their interactions) were significant (P < 0.05), showing that the crude protein contents of the snack bars were significantly affected by processing, level of substitution, and their interactions. The model was significant, showing that the combination of these model terms can reliably predict the crude protein content. This is evident in the R2 value of 1.00, which implies that 100% of the variability in the response is accounted for by the model terms. The adjusted R2 value of 1.00 is a result of the lack of insignificant term(s) in the model, making the model accurate.
Table 4 shows the effect of processing treatments and levels of substitution of Ukwa flour on the in vitro protein digestibility (IVPD) of snack bars. A general decrease in in vitro protein digestibility (IVPD) with an increase in substituted levels was observed in all Ukwa-based snack bars. Processing significantly (P < 0.05) increased the IVPD of snack bars from 63.93% to 88.34%. IVPD of snack bars produced with dehulled Ukwa flour recorded the highest value followed by those produced with malted, defatted, and whole Ukwa flour, respectively, in decreasing order. Improvement of ~7.17% by dehulling, 5.92% by malting, and 4.30% by defatting was achieved in the IVPD of the snack bars. Alonso et al. (2000) reported an increase of IVPD in Faba and kidney beans by 2.4% and 5.14%, respectively, after dehulling. Rahim (2004) reported a change in IVPD of the SML cultivar of the Faba bean from 69.78% to 82.31% due to dehulling. Improvement of IVPD of dehulled Ukwa-based snack bars could be attributed to the reduction in anti-nutrients and insoluble fiber, which are found in the seed coat (hulls). High concentration of anti-nutrients and insoluble fiber in food products from cereals and legumes as protein sources results in poor protein digestibility (Gilani et al., 2005). This is probably the reason the snack bars with hulls (whole, defatted, and malted) recorded lower IVPD compared to the dehulled samples.
Table 4. Effect of processing and substituted levels of African breadfruit seed flours on% in vitro protein digestibility (IVPD) of maize–coconut snack bars.
Malting and defatting also significantly (P < 0.05) increased the IVPD of snack bars. These could have been possible with reduced anti-nutrient content (Table 1), which led to subsequent improvement of IVPD (Table 4) of the snack bars, as reported by Alonso et al. (2000). Rahim (2004) reported improvement from 69.78% to 89.98% in faba beans due to germination. Yadav and Bhatnagar (2016) equally reported an improvement in IVPD from 12.50 g in control to 25.60 g in defatted soy-enriched cereal bars. Azzollini et al. (2018) reported higher values of 76% to 92% IVPD in insect-rich extruded snacks, while James and Nwabueze (2013b) recorded 70% to 72% IVPD in extruded soy snacks.
Analysis of variance of the effect of processing treatments and the levels of substitution Ukwa flour on the in vitro protein digestibility (IVPD) of snack bars showed that a significant (P < 0.05) main and interactive effect existed. The significant model implies that the model terms (processing treatments and substitution levels) can reliably predict the response (IVPD). The R2 value of 1.00 and adjusted R2 value of 1.00 show 100% variability in the response is accounted for by the model terms, without insignificant terms, making the model accurate.
Dehulling, malting, and defatting processes generally resulted in increased in vitro protein digestibility of the snack bars. According to Alonso et al. (2000) and Rahim (2004), the quality of food protein can be defined by the composition of amino acids and their digestibility, and the degree of digestibility would determine the number of amino acids available. This, therefore, implies that there could be increased availability of the amino acids in the snack bars when consumed.
Table 5 shows the results of the effect of processing and levels of substitution of Ukwa on in vitro starch digestibility (IVSD) of snack bars produced with maize and coconut blends. IVSD of snack bars ranged from 29.59% to 57.48%, higher than the values (11.6–13.4%) reported by Flores-Silva et al. (2015) for snacks produced with blends of chickpea, maize, and unripe plantain, while Azzollini et al. (2018) reported IVSD of 34–57% in extruded insect-rich snacks.
Table 5. Effect of processing and substituted levels of Ukwa flours on the in vitro starch digestibility of maize–coconut snack bars.
Processing of Ukwa had a significant (P < 0.05) effect on the IVSD of snack bars. The highest effect was achieved by dehulling, followed by malting and defatting processes in decreasing order. IVSD decreased with an increased substituted level of Ukwa flour in the snack bars. The reduction in in vitro starch digestibility due to the increase in Ukwa flour could probably be due to a corresponding increase in fat (lipid) content and consequently limited starch transformation. Xiaoli (2008) recorded in the literature that fat (~5%) could reduce mechanical energy, melting temperature, prevent moisture absorption, and gelatinization by forming a hydrophobic layer around starch granules, thereby limiting the degree of starch digestibility (Altan et al., 2009).
Improvement in starch digestibility due to dehulling (up to 31%) could be a result of the reduction in fiber, phytic acid, and tannin present in the hulls, which could inhibit α-amylase activity and consequently reduce starch digestibility. Improvement of ~29% by malting and 24% by defatting in the IVSD content of snack bars was achieved. This could be due to enzyme action in breaking down complex molecules to simpler forms and also the reduction in the anti-nutrient content of the snack bars. The reduction in anti-nutrients (such as tannins and phytic acids) is suggested to create a large space in the matrix of the food, causing an increase in enzymatic attack, and so increases the digestibility of starch (Rehman and Shah, 2005). This may explain why the snack bars from dehulled African breadfruit showed the highest starch digestibility among the snacks developed. Roopa and Premavalli (2008) in their research showed that germination among other processing methods (such as autoclaving, pressure cooking, and puffing) increased starch digestibility by 35%−40%.
Analysis of variance, developed from the factorial experiment, showed the effect of the model terms and the reliability of the model in modeling and predicting the response (in vitro starch digestibility). From Table 5, all model terms (treatments, substitutions of Ukwa flour, and interaction of the two variables) were significant (P < 0.05), showing that the IVSD content of snack bars was significantly affected by processing, level of substitution, and the interaction of the two independent variables. The model was significant, showing that the combination of these model terms can reliably predict the response (IVSD). This is evident in the R2 value of 1.00, which implies that 100% of the variability in the response is accounted for by the model terms. The adjusted R2 value of 1.00 is a result of the lack of insignificant term(s) in the model, making the model accurate.
Table 6 shows the effect of dehulling, malting, and defatting, and levels of Ukwa flour on the total essential amino acid (TEAA) of the snack bars from maize and coconut blends. TEAA content ranged from 30.09 g/100 g to 39.05 g/100 g of protein. Significant (P < 0.05) differences existed in the TEAA content of snack bars. Dehulling, malting, and defatting processes significantly (P < 0.05) increased the TEAA content of snack bars. Snack bars made from malted Ukwa flour had the highest TEAA, followed by snack bars produced with dehulled and defatted flours in decreasing order. The improvement in TEAA observed could be a result of the reduction in the anti-nutritional factors and the consequent increase in digestibility of the processed foods. The process of dehulling reduces anti-nutrients through the removal of the hull and concentrates the nutrients. Malting is said to improve the composition of essential amino acids and increase the density of nutrients (Nwabueze and Atuonwu, 2007). Increased amino acids due to malting are attributed to the solubilization of flour and changes in structures of storage protein (glutelins and prolamins), causing their availability for enzymatic attack (Alonso et al., 2000; Nwabueze and Atuonwu, 2007). The process of defatting results in the breakdown of protein–lipid complexes, releasing the protein and making them available for digestion.
Table 6. Effects of processing and substituted levels of Ukwa flours on total essential amino acid (TEAA) content of maize–coconut snack bars.
ANOVA for the effect of treatments and levels of substitution of African breadfruit seed flour on the total essential amino-acid content of snack bar showed significant (P < 0.05) main and interactive effect existed in the treatments and substituted levels of Ukwa flour. Significant (P < 0.05) main and interactive effects existed in the treatments and substituted levels of Ukwa flour. The model developed from the data explained 100% variation (R2 = 1.00, adjusted R2 = 1.00) in the TEAA content of the snack bars.
The study has shown that processing treatments will reduce the anti-nutrients and increase the nutrient contents of Ukwa. The use of dehulled, malted, and defatted Ukwa, in composite with maize–coconut flour blends in the development of snack bars, showed increased availability of nutrients and increased digestibility of protein and starch. Utilization of local food crops in the production of snack bars would help in the reduction in wheat importation and consequent reduction in foreign exchange, and diversification of products thereby making available varieties to consumers. Successful application of these composite flours in snack bars production would have the potential of increasing the protein intake of the consumers of these products, increase the utilization of Ukwa, increase farmer's income, and thereby encourage commercial farming of these indigenous crops Treculia africana (Ukwa), maize, and coconut.
The raw data supporting the conclusions of this article will be made available by the authors, without undue reservation.
AE-N: conceptualization, investigation, methodology, data curation, software analysis, writing—original draft, and writing review. PO and TN: supervision, project administration, conceptualization, investigation, methodology, data curation, software analysis, and review and editing original draft. VN and ME: writing—original draft preparation, data curation, visualization, investigation, software analysis, methodology, formal analysis, and reviewing. All authors have read and agreed to the published version of the manuscript.
The authors declare that the research was conducted in the absence of any commercial or financial relationships that could be construed as a potential conflict of interest.
All claims expressed in this article are solely those of the authors and do not necessarily represent those of their affiliated organizations, or those of the publisher, the editors and the reviewers. Any product that may be evaluated in this article, or claim that may be made by its manufacturer, is not guaranteed or endorsed by the publisher.
Adeoti, O. A., Alabi, A. O., Adedokun, S. O., Jimoh, K. O., and Elutilo, O. O. (2017). Influence of processing methods on the nutrient, anti-nutrient, mineral compositions and functional properties of Akee apple (Blighia sapida Konig) seed and aril flour. J. Hum. Nutr. Food Sci. 5, 1101.
Alonso, R., Aguirre, A., and Marzo, F. (2000). Effect of extrusion and traditional processing methods on anti-nutrients and in vitro digestibility of protein and starch in faba and kidney beans. Food Chem. 68, 159–165. doi: 10.1016/S0308-8146(99)00169-7
Altan, A., McCarthy, K. L., and Maskan, M. (2009). Effect of extrusion cooking on functional properties and in vitro starch digestibility of barley-based extrudates from fruits and vegetables by-products. J. Food Sci. 74, 77–86. doi: 10.1111/j.1750-3841.2009.01051.x
AOAC (2005). Official Methods of Analysis, 18th Edn. Washington, DC: Association of Official Analytical Chemists.
Ayodele, J. I., and Kigbu, P. E. (2003). Some anti-nutritional factors in Cajanus cajan, Sterculia setgera and Vigna dikindtiana. Biol. Environ. Sci. J. Trop. 2, 43–45.
Azzollini, D., Derossi, A., Fogliano, V., Lakemond, C. M. M., and Severini, C. (2018). Effect of formulation and process conditions on microstructure, texture and digestibility of extruded insect-riched snacks. Innov. Food Sci. Emerg. Technol. 45, 344–353. doi: 10.1016/j.ifset.2017.11.017
Bauman, E., Stoya, G., Volkner, W., Lenke, C., and Liuss, W. (2000). Hemolysis of human erythrocyte with saponins affects the membrane structure. Acta Histochem. 102, 21–35. doi: 10.1078/0065-1281-00534
Benitez, L. V. (1989). “Amino acid and fatty acid profiles in aquaculture nutrition studies,” in Fish Nutrition Research in Asia. Proceedings of The Third Asian Fish Nutrition Network Meeting Asian Fish Society Special Publication, ed S. S. De Silva (Manila), 23–35.
Brennan, J. G., Butters, J. R., Cowell, N. D., and Lilly, A. E. V. (1970). Food Engineering Operations. 2nd edition. London: Applied Science Publication Ltd.
Bruce-Fife, N. D. (2010). Coconut Dietary Fiber: A New Dietary Supplement. Colorado: Piccadilly Books, Ltd.
Coe, F. L., Evan, A., and Worcester, E. (2005). Kidney stone disease. J. Clin. Investig. 115, 2598–2608. doi: 10.1172/JCI26662
Edima-Nyah, A. P., Nwabueze, T. U., and Ojimelukwe, P. C. (2019). Development and quality evaluation of snack bars from African breadfruit (Treculia Africana), maize (Zea mays) and coconut (Cocos nucifera) blends. J. Scient. Eng. Res. 6, 74–83.
Edima-Nyah, A. P., Udo, M. E., Ntukidem, V. E., Ojimelukwe, P. C., and Nwabueze, T. U. (2022). Effect of malted African breadfruit (Treculia africana) seed flour inclusion on the in-vitro glycemic index, starch and protein digestibility of fibre rich snack bars. European J. Nutr. Food Saf. 14, 28–40.
Emenonye, A. G. (2016). Extent of processing effect on the proximate and mineral composition of African breadfruit (Treculia africana) seed. Int. J. Sci. Technol. 6, 6–10.
Enwere, N. J. (1998). Foods of Plant Origin. Nsukka: Afro-Orbis Publishing Company Limited, 182–299.
Feili, R., Zzaman, W., Abdullah, W. N. W., and Yang, T. A. (2013). Physical and sensory Analysis of High Fibre Bread incorporated with Jackfruit rind flour. J. Food Sci. Technol. 1, 30–36. doi: 10.13189/fst.2013.010203
Fellows, P. (1990). Food Processing Technology (Principles and Practice). Bodmin, Cornel: Harnolls Publication Limited.
Flores-Silva, P. C., Rodriguez-Ambriz, S. L., and Bello-Perez, L. A. (2015). Gluten-free snacks using plantain-chickpea and maize blend: chemical composition, starch digestibility and predicted glycemic index. J. Food Sci. 80, C961–C966. doi: 10.1111/1750-3841.12865
Gilani, G. S., Cockell, K. A., and Sepehr, E. (2005). Effect of anti-nutritional factors on protein digestibility and amino-acid availability in foods. J. Assoc. Off. Anal. Chem. Int. 88, 967–987. doi: 10.1093/jaoac/88.3.967
Gwirtz, J. A., and Garcia-Casal, M. N. (2014). Processing maize flour and corn meal food products. Ann. NY Acad. Sci. 1312, 66–75. doi: 10.1111/nyas.12299
Ifie, I., and Emeruwa, C. (2011). Nutritional and anti-nutritional characteristics of the larva of Oryctes monoceros. Agric. Biol. J. North Am. 1992, 42–46. doi: 10.5251/abjna.2011.2.1.42.46
Iwe, M. O., and Ngoddy, P. O. (2000). Effect of extrusion on trypsin inhibitor contents of soy-sweet potato mixtures. J. Process. Preserv. 24, 453–463. doi: 10.1111/j.1745-4549.2000.tb00434.x
Jabs, J., and Devine, C. M. (2006). Time scarcity and food choices and overview. Appetite 47, 196–204. doi: 10.1016/j.appet.2006.02.014
James, C. S. (1995). Analytical Chemistry of Foods. London: Chapman and Hall Publishers. doi: 10.1007/978-1-4615-2165-5
James, S., and Nwabueze, T. U. (2013a). Quality evaluation of extruded full fat blend of African Breadfruit-soybean-corn snack. Int. J. Sci. Technol. Res. 2, 212–216.
James, S., and Nwabueze, T. U. (2013b). Effect of extrusion condition and defatted soybean inclusion on the physio-chemical, in vitro digestibility and sensory acceptability of African breadfruit (Treculia africana) blends. Int. J. Sci. Technol. Res. 2, 207–211.
Kanu, J. k., Sandy, E. H., and Kandeh, B. A. J. (2009). Production and evaluation of breakfast cereal-Based porridge mixed with sesame and pigeon peas for Adults. Pakistan J. Nutr. 8, 1335–1343.
Kumar, V., Sinha, A. K., Makkar, H. P. S., and Becker, K. (2010). Dietary roles of phytate and phytase in human nutrition: a review. Food Chem. 120, 945–959. doi: 10.1016/j.foodchem.2009.11.052
Maseta, E., Mosha, T. C. E., Laswai, H., and Nyaruhucha, C. N. (2016). Nutritional quality, mycotoxins and antinutritional factors in quality protein maize-based supplementary foods for children in Tanzania. Int. J. Sci. 5, 37–44. doi: 10.18483/ijSci.1082
Nagel, R. (2010). Living with phytic acid: wise traditions in food, farming and the healing arts. Q. J. Weston. Available online at: www.westonaprice.org
Nago, C. M., Devautour, H., and Munchnic, J. (1990). Technical resources of food processing micro enterprises in Benin. Agritrop. 14, 7–11.
Nwabueze, T. U. (2007). Effects of process variables on trypsin inhibitor activity (TIA), phytic acid and tannin content of extruded African breadfruit-soy-corn mixtures: a response surface analysis. J. Food Sci. Technol. 40, 21–29. doi: 10.1016/j.lwt.2005.10.004
Nwabueze, T. U., and Atuonwu, A. C. (2007). Effect of malting African Breadfruit (respectively, Treculia africana) seeds on flour properties and biscuit sensory and quality characteristics as composite. J. Food Technol. 5, 42–48.
Nwabueze, T. U., and Iwe, M. O. (2010). Residence time distribution (RTD) in a single screw extrusion of African breadfruit mixtures. Food Bioprocess Technol. 3, 135–145. doi: 10.1007/s11947-008-0056-z
Nwabueze, T. U., Iwe, M. O., and Akobundu, E. N. T. (2008). Physical characteristics and acceptability of extruded African breadfruit-based snacks. J. Food Qual. 31, 142–155.
Nwabueze, T. U., and Uchendu, C. B. (2011). African breadfruit (Treculia africana) seed as adjunct in ethanol production. Eur. J. Food Res. Rev. 1, 15–22.
Oakfenfall, D., and Sidu, G. (1990). Could saponin be a useful treatment for hypercholesterolemia? Eur. J. Clin. Nutr. 44, 79–88.
Oberleas, D. (1973). Toxicants Occurring Naturally in Foods. Washington, DC: National Academy of Science. p. 363–371.
Odemelam, S. A. (2000). Functional properties of African breadfruit (Treculia africana) flour as influenced by processing. Glob. J. Pure Appl. Sci. 6, 389–393.
Okafor, G. I., and Ugwu, F. C. (2014). Production and evaluation of cold extruded and baked ready-to-eat snacks from blends of breadfruit (Treculia africana), cashew (Anacardium occidentale) and coconut (Cocos nucifera). Food Sci. Qual. Manag. 23, 65–77.
O'Keefe, S. J. (2019). The association between dietary fiber deficiency and high income lifestyle associated diseases: Burkitt's hypothesis revisited. Lancet Gastroenterol. Hepatol. 4, 984–996. doi: 10.1016/52468-1253(19)30257-2
Onwuka, G. I. (2005). Food Analysis and Instrumentation: Theory and Practice. Lagos: Naphtali Prints. p. 19 - 38.
Osabor, V. N., Ogar, D. A., Okafor, P. C., and Egbung, G. F. (2009). Profile of the African breadfruit (Treculia africana). Pak. J. Nutr. 8, 1005–1008. doi: 10.3923/pjn.2009.1005.1008
Osborne, D. R., and Voogt, P. (1978). Analysis of Nutrients in Foods. New York, NY: Academic Press. p. 237.
Rahim, S. I. A. (2004). Effect of Processing on Anti-Nutritional Factors and in Vitro Protein Digestibility of Faba Beans (Vicia faba) (Thesis). Department of Food Science and Technology, University of Khartoum, 128.
Raj, S., Suvadarshini, A., and Mishra, B. B. (2021). Role, relevance and significance of convenience food—a literature review approach. Glob. Med. J. 19, 41.
Rehman, Z. U., and Shah, W. R. (2005). Thermal heat processing effects on anti-nutrients, protein and starch digestibility of food legumes. Food Chem. 91, 327–331. doi: 10.1016/j.foodchem.2004.06.019
Rodge, A. B., Sonkamble, S. M., Salve, R. V., and Hashmi, S. I. (2012). Effect of hydrocolloid (Guar gum) incorporation on the quality characteristics of bread. J. Food Process. Technol. 63, 721–725. doi: 10.4172/2157-7110.1000136
Roopa, S., and Premavalli, K. S. (2008). Effect of processing on starch fractions in different varieties of finger millet. Food Chem. 106, 875–882. doi: 10.1016/j.foodchem.2006.08.035
Runsewe, A. I., Olowu, A. O., Olanrewaju, D. M., and Akesode, F. A. (2001). Efficacy of the African breadfruit (Treculia africana) in the nutritional rehabilition of children with protein energy malnutrition. Niger. J. Paediatr. 28, 128–134.
Schill, C., and Munz, K. (2011). Spentgrains—a valueable raw material for a high fibre food snack. J. Org. Chem. 76, 8428–8431.
Seena, L. P. (2006). Anti-nutritional and functional properties of great northern bean (Phaseolus vulgaricus L.) protein. J. Food Sci. 46, 71–73.
Singh, A., Yadav, N., and Sharma, S. (2012). Effect of fermentation on physicochemical properties and in vitro starch and protein digestibility of selected cereals. Int. J. Agric. Food Sci. 2, 66–70.
Steinkraus, K. H. C. (2002). Fermentation in world food processing. An inaugural issue of comprehensive reviews in food science and food safety. Inst. Food Technol. 1, 23–32. doi: 10.1111/j.1541-4337.2002.tb00004.x
Thapliyal, P., Sehgal, S., and Kawatra, A. (2014). In vitro digestibility and antinutrients as affected by soaking, dehulling and pressure cooking of chickpea (Cicer avietinum) varieties. Asian J. Dairy Food Resour. 33, 131–135. doi: 10.5958/0976-0563.2014.00589.2
Ugwu, F. M., and Oranye, N. A. (2006). Effects of some processing methods on the toxic components of African breadfruit (Treculia africana). Afr. J. Biotechnol. 5, 2329–2333.
Wang, C., Jia, G., Zhi, H., Niu, Z., Chai, Y., Li, W., Wang, Y., Li, H., Lu, P., and Zhao, B. (2008). In vitro starch and protein digestibility of common snacks from cereals. Genes Genom. Genet. 2, 769–777. doi: 10.1534/g3.112.002907
Wasserman, R. (2016). Properties of Coconut Fiber. Available online at: http://www.livestrong.com/article/249254-properties-of-coconut-fiber/ (accessed August 19, 2016).
Xiaoli, X. (2008). In Vitro Digestibility of Starch in Sorghum Differing in Endosperm Hardness and Flour Particle Size (M. Sc. Thesis). Department of Grain Science and Industry, Kansas State University, Kansas, 61–78.
Keywords: dehulling, sprouting, defatting, amino-acids, digestibility
Citation: Edima-Nyah AP, Ntukidem VE, Ekanem MC, Ojimelukwe PC and Nwabueze TU (2023) Effect of processing and substitution levels of Ukwa (Treculia africana) on the anti-nutrient factors, in-vitro starch and protein digestibility and total essential amino-acid content of snack bars. Front. Sustain. Food Syst. 6:1071821. doi: 10.3389/fsufs.2022.1071821
Received: 17 October 2022; Accepted: 22 December 2022;
Published: 18 January 2023.
Edited by:
Philippa Chinyere Ojimelukwe, Michael Okpara University of Agriculture, NigeriaReviewed by:
Bin Wang, Shaoguan University, ChinaCopyright © 2023 Edima-Nyah, Ntukidem, Ekanem, Ojimelukwe and Nwabueze. This is an open-access article distributed under the terms of the Creative Commons Attribution License (CC BY). The use, distribution or reproduction in other forums is permitted, provided the original author(s) and the copyright owner(s) are credited and that the original publication in this journal is cited, in accordance with accepted academic practice. No use, distribution or reproduction is permitted which does not comply with these terms.
*Correspondence: Anne P. Edima-Nyah, ZWRpbWFhbm5lcEBnbWFpbC5jb20=
Disclaimer: All claims expressed in this article are solely those of the authors and do not necessarily represent those of their affiliated organizations, or those of the publisher, the editors and the reviewers. Any product that may be evaluated in this article or claim that may be made by its manufacturer is not guaranteed or endorsed by the publisher.
Research integrity at Frontiers
Learn more about the work of our research integrity team to safeguard the quality of each article we publish.