- 1Cardamom Research Station, Kerala Agricultural University, Pampadumpara, India
- 2ICAR-Indian Institute of Spices Research, Kozhikode, India
- 3Biological Systems Engineering, College of Agriculture and Food Sciences, Florida State A&M University, Tallahassee, FL, United States
- 4Department of Materials Engineering, Indian Institute of Science, Bangalore, India
- 5Horticulture College and Research Institute, Periyakulam, India
In this study, we investigated climatic parameters and predicted future changes in precipitation and atmospheric temperature levels based on RCP4. 5/8.5 scenarios in all cardamom-coffee hot spots of southern India. Our results showed more precipitation levels and patterns occurred in Cardamom hills (Kerala), followed by lower Puleny hills in Tamil Nadu. The least variation in precipitation levels has been noticed for temperate upper Puleny hills and Kodagu hills in Karnataka. RCP4.5/8.5 scenario analysis showed greater variability in precipitation, up to 180% increase and 90% decrease for all hot sports. The scenario analysis also predicted extreme temperature variations ranging from 0.5 to 8.5°C increase for the entire study region. A significant change in coffee yield and quality has been recorded over the last 30 years. Increased yield trends in coffee were noticed for Cardamom hills (CH) and Kodagu hills, but significantly lower coffee production was observed for lower Pulney hills. The mixed response of yield variability in coffee has been primarily attributed to the ongoing changing climatic factors. Ecophysiological studies of coffee, cardamom, and black pepper have proved that coffee would adapt well to future challenging climatic conditions, closely followed by cardamom and black pepper. Since all the coffee-cardamom hot spots in southern India undergoes considerable change in precipitation levels and pattern, necessary precautions, including water and irrigation management strategies, must be given utmost priority to increase the crop yield sustainability of these delicate cardamom-coffee hot spots in India.
Introduction
Indian cardamom-coffee hot spots are one of the two unique and vital tropical mountain ecosystems for a growing variety of crops, e.g., spices, fruits, and vegetables. The other is located in Guatemala, where maize and tapioca are also cultivated along with cardamom and coffee. Generally, farmers' livelihoods in tropical mountain agriculture in southern India depend mainly on growing high-value spice crops and seasonal vegetables and fruit crops. These hot spots in India have peculiar environments mainly due to altitudinal variations and specific geographical locations concerning the Indian monsoon and its seasonal winds. Agroecosystems in tropical mountains are highly sensitive and vulnerable to climatic change, thereby reduction in crop yields. Agriculture across the world is under stress leading to diminishing returns and productivity. The relative rates of yield increase for all major cereal crops and plantation crops are already declining (Ainsworth and Ort, 2010; Murugan et al., 2012).
The world's coffee-cardamom sector provides employment and livelihood for over 60 million people in the tropics. It is considered the second largest employment sector in the world after the oil industry. Correspondingly, India is the sixth and second-largest coffee and cardamom producing country globally, accounting for over 4 and 40% of the world's production. More than 92% of the Indian coffee and cardamom production comes from Karnataka, Kerala, and Tamil Nadu. The area, production, and productivity of coffee are maximum for Karnataka, followed by Kerala and Tamil Nadu. Kerala is the country's leading producer of small cardamom, chased by Karnataka and Tamil Nadu. India's major share of coffee and cardamom production originates from small to medium farms, which are more vulnerable to climatic change and variability (Murugan et al., 2009a). Both cardamom and coffee prefer elevation-induced natural forest ecosystems for sustainable production. Cardamom being more closely related to the rain forest environment; sufficient surface soil moisture is indispensable. Coffee, which requires cooler subtropical climatic conditions, is relatively less sensitive to drought under optimum natural forest shade. Hence, these crops are predominantly cultivated in the high ranges and the high uplands of the Western Ghats (WG) in southern India (Murugan et al., 2022). Except for the Upper Pulney hills (UPH), where many temperate fruits and vegetables are mainly farmed, the other hot spots are cultivated with only high-value spices and plantation crops. Most coffee farms in these states are concentrated in the WG high uplands. The rich history of coffee cultivation in Pulney hills is ancient, whereas it is relatively new and peculiar to Cardamom hills (CH). Both Arabica and Robusta spoil ~50% share in acreage.
Upper Pulney hills (UPH) are the largest highland massif in southern India (Raith et al., 1997). Geographically, the unique Pulney hills form a part of the widest portion in the entire WG, which are considered one of the Global Biodiversity hot spots. Fyson et al. (1915) first published authentic and science-based information about the biotic richness of flowering plants of the temperate Kodaikanal hills. One of the Global Atmospheric Watch (GAW) stations were positioned in Kodaikanal hills considering the geographical superiority over other hills in India5. South of the Himalayas, the longest high-plateau (7,700 feet MSL) is supposed to have more diversified agricultural/sylvicultural/silvopastoral and socio-economic significance and activities than the other hot-spots.
According to (Smith, 1895), the overall climate of the UPH used to be a splendid one, combining many of the advantages of tropical and temperate regions; for instance, the mean relative humidity varied from 47% in March to 83% in August and the mean for the year being 72%. The actual annual total rainfall around 1,895 was 47.5 inches at the observatory site. Still, slightly increased rainfall (20% more) was reported for a station little away from the observatory at higher elevation cliffs (61 inches). The rainfall was spread over all months, highest for August (21 days) and lowest for March (3 days). There were 2,065 h of sunshine annually. The impact of orography characterizes the rainfall pattern over these high ridges and valleys. Hence the rainfall activity spread across all the seasons rather than a single season. The western high ridge plateau of the hills experiences a temperate environment. At the same time, the lesser elevated eastern (1,000–2,000 MSL) slopes enjoy the tropical and subtropical climate, which mostly comes under Lower Pulney hills (LPH). Overall, the average annual total rainfall, 41, 35, and 24%, correspondingly contributed by the northeast and southwest monsoon and summer months. Thus, the entire region has a magnificent climate with many advantages over tropical and temperate regions. That is why a variety of crops ranging from temperate fruits (Pear and Plum) to vegetables (Cole crops, Beans), further to tubers (Potato and Carrot), and plantation crops (coffee and pepper) are successfully cultivated in these hills throughout the year. And as such, any change in the patterns of climate parameters, for instance, temperature and rainfall, attracts value amongst researchers and agriculturalists concerned (Anandhi et al., 2022; Murugan et al., 2022).
The main factors that control the growth and development of diseases in plants are temperature and precipitation (water) by affecting factors such as the plant pathogen's survival, vigor, rate of multiplication, sporulation, direction, the distance of dispersal of inoculums, and rate of spore germination and penetration (Yáñez-López et al., 2014). Hence, studies assessing the impact of climate change in agriculture have mainly focused on the effects of temperatures and precipitation while considering agricultural production and the impact of insects, pests, and diseases. Increases in the growing season change the evapotranspiration and affect the water demand in managed ecosystems (Anandhi et al., 2013a,b). Specific stages of growth (e.g., flowering, pollination, and grain filling) are susceptible to weather conditions and critical for final yield (Lavalle et al., 2009). As global temperature rises, crops will increasingly experience failure in traditional production regions, especially if climate variability increases and precipitation lessens or becomes more variable. Atmospheric temperature and rainfall variability significantly affect crop growth and yield.
Climate change in managed ecosystems is likely to alter the availability and distribution of freshwater (e.g., floods, droughts) while simultaneously increasing the demand for water from rivers and impacting groundwater availability (Allan et al., 2013). In addition, rising temperatures have been shown to impact the phenology of plants and insects [shifts in the timing of plant and insect activity] (Anandhi et al., 2016a). Plant response to climate change is realized through complex interactions among CO2 concentration, temperature, solar radiation, and precipitation. Temperature, precipitation, sunlight, and air humidity are also primary climate factors controlling crop growth and the development of insects, pests, and diseases (Esbjerg and Sigsgaard, 2014). However, data on climate variables such as solar radiation and humidity for these hot spots are sparse compared with rainfall and temperature (Anandhi et al., 2012; Murugan et al., 2013; Anandhi et al., 2016b).
The production physiology of coffee is unique among crops. The crop prefers a sub-tropical (cool dry) condition for growth, with optimum temperature being 18–26°C. However, it requires another condition of inductive stress followed by a medium to heavy rainfall that triggers the entire flowering process. The tricky physiology of coffee flowering has been probed, and on which convincing results are available. The term blossom showers give a meaningful representation of how much summer showers mean to productivity in coffee, and this could also be why nature has placed the original habitat of coffee in an area with tropical showers blended with cool winters.
The flowering aspects of coffee have been extensively studied and documented (Huxley, 1970; Browning, 1975) and extensively reviewed by Cannell (1985). This points to the summer stress and summer showers as pre determinants of flowering requirements. The crux of these works revolves around the production of hydrolytic enzymes brought about by a favorable balance of hormones, namely GA/Auxins, that initiates flowering. There is also compelling evidence to suggest a reduction in abscisic acid levels, which releases the dormancy of flower buds with the receipt of the first rains (Barros et al., 1978; Rena et al., 1994). Thus, the three critical requirements of inductive stress, the blossom showers, and backing rains, are the most critical aspects that govern flowering and productivity in coffee. The timely receipt of showers and the intensity to break down the dormancy of flower buds are the points of immediate concern in the WG. Limited water availability can cause an increase in crop failure (defined as the complete loss of crops on a farm). The LPH where coffee is grown is now seen to have a prolonged drought in summer, posing a challenge to the very existence of the crop there. The case in CH is that the summer shower has become erratic and subjected to cause increased stress often (Murugan et al., 2013). Wynad district in Kerala, where coffee is mainly grown as a sole crop, climate change has been felt, and coffee production and productivity have been rendered vulnerable. This has led to an agrarian crisis, with farmers resorting to suicide as it is the mainstay of livelihood. The neighboring Coorg district in Karnataka has not yet frequently witnessed climate change compared to Kerala and Tamil Nadu, mostly because large-scale deforestation is yet to occur.
The cardamom sector is much more organized with very high inputs, but this Zingiberaceous crop is very sensitive to climatic vagaries. This is another crop that requires little stress during early February and with the onset of profusely monsoon tillers under rainfed conditions. It is from the base of such healthy tillers or stout tillers that the panicle arises. Longer wet spells drive the production of panicles from the previous season tillers. Another important aspect is that the lengthening of the panicles bearing the flowers and the consequent fruit set is again dependent on the length of wet spells or otherwise has to be managed or supplemented by irrigation.
Thus, we have two typical clear-cut crop stages with distinct requirements dependent on environmental conditions.
1. Inductive summer stress followed by summer showers for floral induction of coffee followed by backing rains for berry set and development and
2. Stress is followed by rains for tillering (emergence of new shoots) and panicle initiation from the base of stout tillers, and panicle growth and flowering and fruit set with persistent rains in cardamom.
Any limitation to this sequence or change in the intensity and calendar of events like prolonged or frequent drought, non-receipt of timely rainfall, and delayed and non-optimal receipt of continued rainfall could offset the entire production cycle, leading to reduced crop yields. Thus, the critical environmental conditions have paved the way for the further development of both crops. Relative rates of yield increase for all of the major cereal crops are already declining. The assumptions, such as yield improvements from the latter half of the 20th century, may not be sound because they are based on historical temperature-crop yield relationships, potential ceilings to crop yields and limitations to the expansion of agricultural lands. Under climate change conditions and increased human activity, the stresses and vulnerabilities associated with a water supply and water demand will increase significantly worldwide (Allan et al., 2013).
Agriculture is vulnerable to climate change and variability through direct (i.e., abiotic) effects on crop development and yield (e.g., changes in temperature or precipitation) as well as through indirect effects arising from changes in the severity of pest, insect, and disease pressures; availability of pollination services; and performance of other ecosystem services that affect agricultural productivity (Walthall, 2012; Anandhi and Blocksome, 2017). However, long-term data on climate variables such as solar radiation and humidity are sparse compared with rainfall and temperature (Anandhi et al., 2012, 2016b). Present and future climatic change may present unprecedented challenges to coffee-cardamom cultivations by influencing crop distribution and production and increasing the economic and environmental risks associated with the production systems. Therefore, critical analysis and insights into the observed climatic variability and yield of crops could be helpful for scientific and farming communities to manage the delicate Indian cardamom-coffee hot spots better.
Materials and methods
Long-term climate data from four representative climate stations located in three south Indian states and productivity data of select crops were subjected to statistical analyses. Future rainfall variability and patterns for all three montane hot spots were also studied (Table 1). The future climate of small-scale tropical mountains is uncertain and unknown. Global Climate Models (GCMs) are among the most advanced tools that simulate climatic conditions hundreds of years into the future. The scenarios are often used in investigating the potential consequences of anthropogenic climate change and natural climate variability. A scenario is a coherent, internally consistent, and plausible description of a possible future state of the world. A farmer's survey was also conducted to understand the grower's perception of climate change implications.
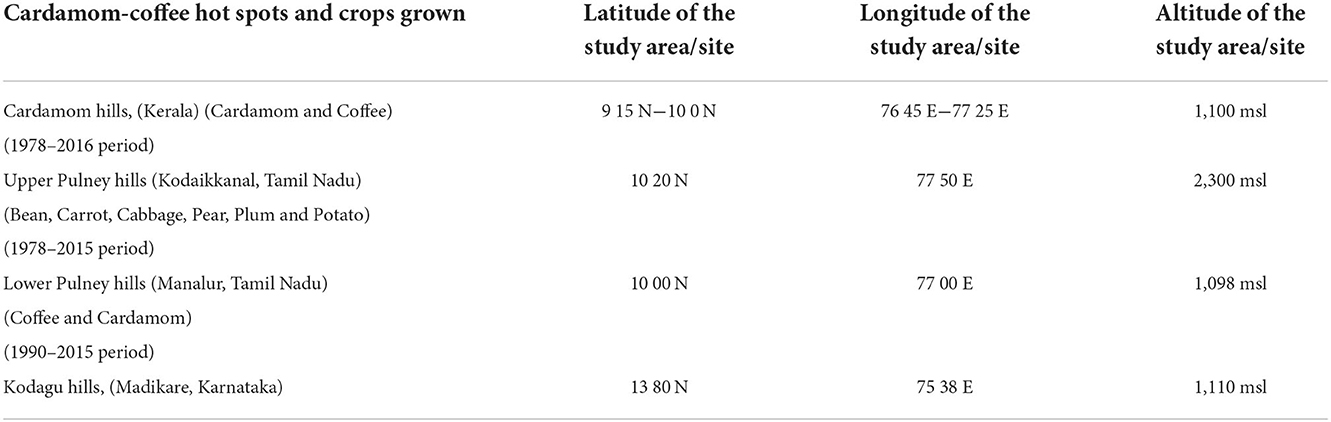
Table 1. Name of the metrological station and geographical location details of cardamom-coffee hot spots.
Data used
The fifth phase of the Climate Model Intercomparison Project (CMIP5), a freely available state-of-the-art multi-model dataset (multiple GCMs and representative concentration pathway-RCP), was designed to advance our knowledge of climate variability and climate change. Monthly simulations of precipitation and temperature from 35 GCMs that participated in the CMIP5 were used in this study. Results from historical (1986–2005) and future (2006–2099 and 2006–2099, RCP4.5 and RCP8.5) experiments were investigated. Further information on the experiments and models is available on the CMIP5 website (http://cmippcmdi.llnl.gov/cmip5/). The data were extracted and interpolated to a common 1.5° grid square using bilinear interpolation.
Simulations from 35 GCMs participating in CMIP5 for two future emission scenarios (RCP4.5 and RCP8.5) provide a wide range of potential climate change outcomes (Anandhi and Bentley, 2018). Moreover, the results could be later used to access both mitigation and adaptation alternatives to reduce vulnerabilities in managed ecosystems (agriculture and urban systems) and water resources. This paper tried to explain and explore how current climate change and variability have impacted the yields of select crops grown in these delicate mountain ecosystems by statistical analysis and comparison. Regression models were used to explain the crop yields' variability using growing season climate data.
Developing incremental scenario
Scenarios can be created using simple downscaling methods such as change factor methodology (Anandhi et al., 2011). The scenarios thus obtained were also referred to as synthetic scenarios (Anandhi et al., 2018).
Step 1: Grids were identified: 11 grids cover the study region (Table 2). Each grid has 70 monthly time series for (35 GCMs/runs for rcp 4.5/rcp 8.5). Therefore the 770-time series (70 monthly time series each for 11 grids) were analyzed.
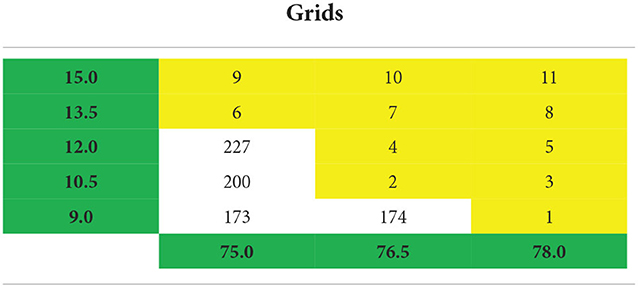
Table 2. Geographical grid details used in the study region highlighted in yellow (for interpretation of the references to color in this figure, the reader is referred to the web version of this article).
Step 2: All the 770 time-series were plotted for each variable.
Step 3: 24 month moving averages from each of the 770 time-series were plotted for each variable.
Step 4: Change using Equation 1 for Precipitation and Equation 2 for temperature was estimated and plotted.
Step 5: All 11 grids' time series for RCP 4.5 scenarios were combined for a variable and then estimated the percentiles 5, 10, 15, 25, 50, 75, 90, and 95 percentiles and plotted them. Did the same for RCP 8.5.
Step 6: Boundary of the scenario funnels was developed. Estimated the 24-month moving average of the percentiles 5, 10, 15, 25, 50, 75, 90, and 95 percentiles and plotted them. The same was repeated for RCP 8.5. Thus, each RCP had three funnels with six boundaries (blue, red, and yellow).
Step 7: The funnel boundaries were plotted for the two RCP in a single plot and the changes were observed.
Ecological physiology of shade grown cardamom, coffee and black pepper
Experiments were conducted during a warm-weather condition in March 2019 at the research farm, ICAR-Indian Institute of Spices Research, Regional Station, Madikeri. The study was carried out on yielding coffee, cardamom, and black pepper plants under natural conditions (natural forest). All the observations were carried out in 10 days to avoid seasonal changes. Newly expanded mature leaves, i.e., the third leaf in the experimental plants, were studied.
Leaf gas exchange
Gas exchange measurements were obtained using a portable photosynthetic system (LCpro-SD Advanced Photosynthesis Measurement System, England). Net photosynthetic rate (Anet, μmol CO2/m−2 s−1), stomatal conductance (gs, mol H2O/m−2 s−1) and transpiration (E, mmol/m−2 s−1), instantaneous water-use efficiency (WUEi) was calculated as the ratio of net assimilation (Anet) to transpiration (E). Diurnal variation of gas exchange parameters was collected in the field-grown plants during the summer season at 2-h intervals from 7:00 to 17:00 h over two consecutive days per population. The measurements were recorded when both Anet and gs were stable.
In addition to the ecophysiological measurements, micro-environmental parameters at the natural stands of the select sample populations were recorded. Light availability was measured as photosynthetic photon flux density (PPFD) (μmol/m−2 s−1), reflecting ambient conditions at the specific measurement date. The difference in the water vapor pressure (VPD; mbar) was also computed. The leaf temperature (oC) and Ci- intercellular CO2 concentration (ppm) were also measured.
Chlorophyll fluorescence
In vivo chlorophyll fluorescence was measured using a chlorophyll fluorometer (Os-30p) in 10–15 min of dark-adapted leaves on the same plants as those used for leaf gas exchange measurements. The maximum PS II quantum yield [Fv/Fm = (Fm – Fo)/Fm] was determined in dark-adapted leaves at 2 h intervals from 7:00 to 17:00 h local time, according to Strasser et al. (1995). Gas exchange and chlorophyll fluorescence were analyzed on a typical sunny day. All measurements were performed on the fully expanded leaves with 30 observations.
Results and discussion
Observed climatic variability in coffee-cardamom hot spots
All hot spots have registered climatic variability, but the hotspots' levels vary depending on the specific geographic location (Figures 1, 2). Rainfall variability was maximum for CH, followed by LPH. Temperate UPH and Kodagu hills (KH) have experienced little variation in rainfall. Interestingly UPH has recorded increased rainfall amounts over the study period. Extreme variability in air temperature and rainfall was notified for LPH and UPH. Temperature showed a downward trend in upper Pulney hills. KH showed increased rainfall during the first two seasons, whereas the rainfall during October and December showed decreasing trend. Increasing rainfall trends were registered at cardamom hills for the first two seasons. The third season rainfall recorded a declining tendency.
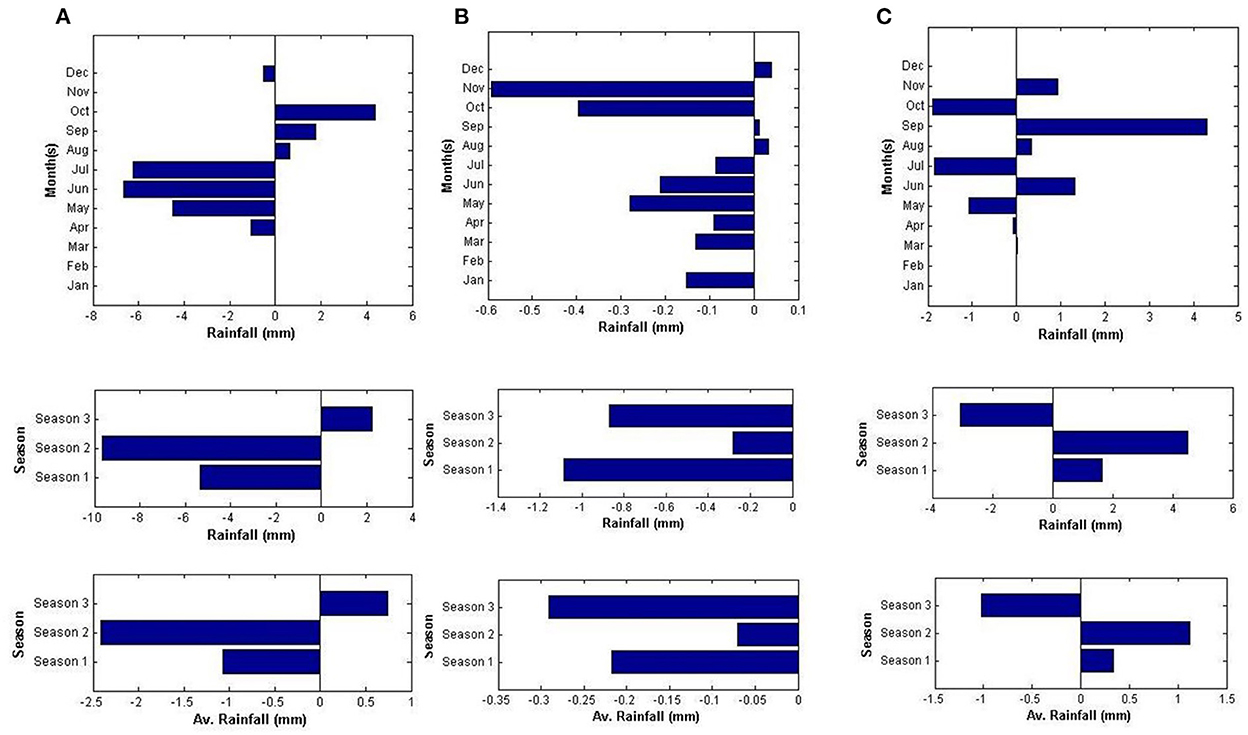
Figure 2. Sen slopes of trends in climatic variability in (A), CH, Kerala; (B), LPH (Manalur), Tamil Nadu; (C), KH, Karnataka.
The observed variability in precipitation levels on seasonal and monthly scales was the greatest for LPH and UPH (Tamil Nadu), followed by CH (Kerala) and KH (Karnataka) (Murugan et al., 2008, 2009a). Variation in the amounts and pattern of seasonal rainfall has not changed much during the period (1978–2014) at higher altitude UPH (Kodaikkanal station). Rainfall during the summer months (Jan–May) contributed 24.08% (372.06 mm) while the first (June–Sep) and second monsoon months (Oct–Sep) had respectively given 34.64 (541.3 mm) and 41.28% (649.23 mm). Annual total effective sunshine hours (SSH) declined over the study period; its range was 1,775.7–2,005.3 h. Minimum monthly SSH ranged from 14.8 to 70.3 hours. July recorded the least, while February was the sunniest month. The rainfall distribution had not changed much in UPH, but other climatic factors had changed dramatically from the earlier observations (Smith, 1895). Increased rainfall amounts have been recorded in UPH for the study period on seasonal scales, besides a significant variability in atmospheric air temperature and relative humidity. Maximum and minimum temperatures across the seasons declined, except in the summer months. A notable reduction in diurnal temperatures was registered for all seasons. Atmospheric relative humidity showed positive trends in all seasons in UPH. Similar findings and observations were also made in the previous analysis of Rangan et al. (2010).
The observed atmospheric air temperature (T min) in the regions consistently increased, causing significant challenges in the water management of these sensitive crops. The productivity of the crops was highly variable with hotspots. Coffee yields have decreased significantly over time in LPH, while a considerable increase was observed for the other two hotspots, CH & KH. Even a 40% decrease in annual total rainfall did not affect the yields of coffee in CH during 2017–2018 but reduced the cardamom yield to nearly 50%. Increasing atmospheric air temperatures coupled with high precipitation amounts could further stress groundwater reservoirs of the region, leading to withdrawal rates that become even more unsustainable for the mountainous regions. High precipitation variability (with a higher propensity for localized intense rainfall events) observed in the region can be a crucial factor in these ecosystems' water management. In the future, the results could be used to develop and plan mitigation and adaptation alternatives to reduce vulnerabilities and increase yield sustainability of the hot spot.
Future climate change based on RCP 4.5/8.5 (precipitation and temperature)
Agriculture is vulnerable to climate change and variability through direct (i.e., abiotic) effects on crop development and yield (e.g., changes in temperature or precipitation) as well as through indirect effects arising from changes in the severity of pest, insect, and disease pressures; availability of pollination services; and performance of other ecosystem services that affect agricultural productivity Walthall (2012). In tropical regions, heavy rainfall and strong winds are destructive to crops (Lansigan et al., 2000). Studies have shown that high rainfall events over parts of India are increasing (Ramesh and Goswami, 2014; Karmakar et al., 2015). This can impact agricultural production and urban water management. Heavy rainfall just before harvesting decreases yields in many regions of the world. Mean temperature, mean precipitation, and the number of days with precipitation were correlated to changes in insect phenology, date of the first appearance, changes in insect generation time, number of generations per season, and geographical location distribution (Esbjerg and Sigsgaard, 2014). Changing temperatures can change the crop development stage, which can generally change the crop water requirement and cause water stress to plant growth, which may impact yield. These can have important implications for water resource management in the region. Our results showed high variability in the future change in precipitation and temperature in all hot spots (Figures 3, 4). High variability in the future change in precipitation and temperature was noticed for the entire region. Variability in rainfall and its unique pattern (with a higher propensity for localized extreme rainfall events) was observed for the region, which can be a key factor for these ecosystems, mainly crop water management of these sensitive, delicate mountain regions.
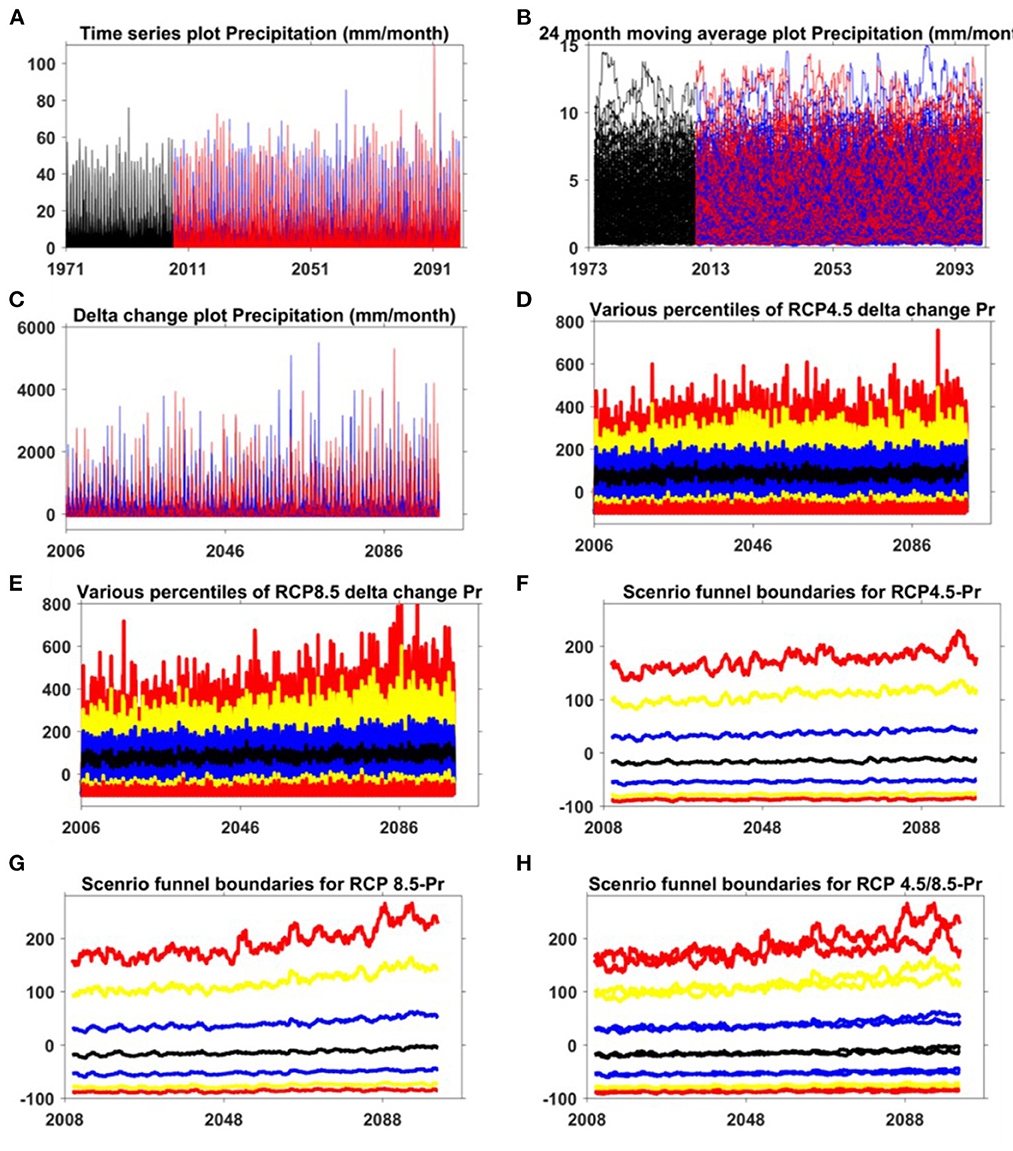
Figure 3. The plot of various steps in developing the scenario funnel for precipitation [Pr, (A–H)] by data-analysis, (A) time-series plot of raw precipitation data, (B) time-series plot of 24 month moving average precipitation data, (C) time-series plot of delta change estimated for precipitation, (D) various percentile values for RCP 4.5 for precipitation (red: 5th, 95th; yellow:10th, 90th, blue: 25th, 75th, black: 50th percentiles), (E) various percentile values for RCP 8.5 for precipitation (red: 5th, 95th; yellow:10th, 90th, blue: 25th, 75th, black: 50th percentiles), (F) Developing scenario funnel boundaries for RCP 4.5 from 24 month moving average of the percentiles (red: 5th, 95th; yellow:10th, 90th, blue: 25th, 75th, black: 50th percentiles), (G) Developing scenario funnel boundaries for RCP 8.5 from 24 month moving average of the percentiles (red: 5th, 95th; yellow:10th, 90th, blue: 25th, 75th, black: 50th percentiles), (H) scenario funnels for precipitation. In (A–C), red and blue color represents the RCP 8.5 and RCP 4.5, respectively. (For interpretation of the references to color in this figure legend, the reader is referred to the web version of this article).
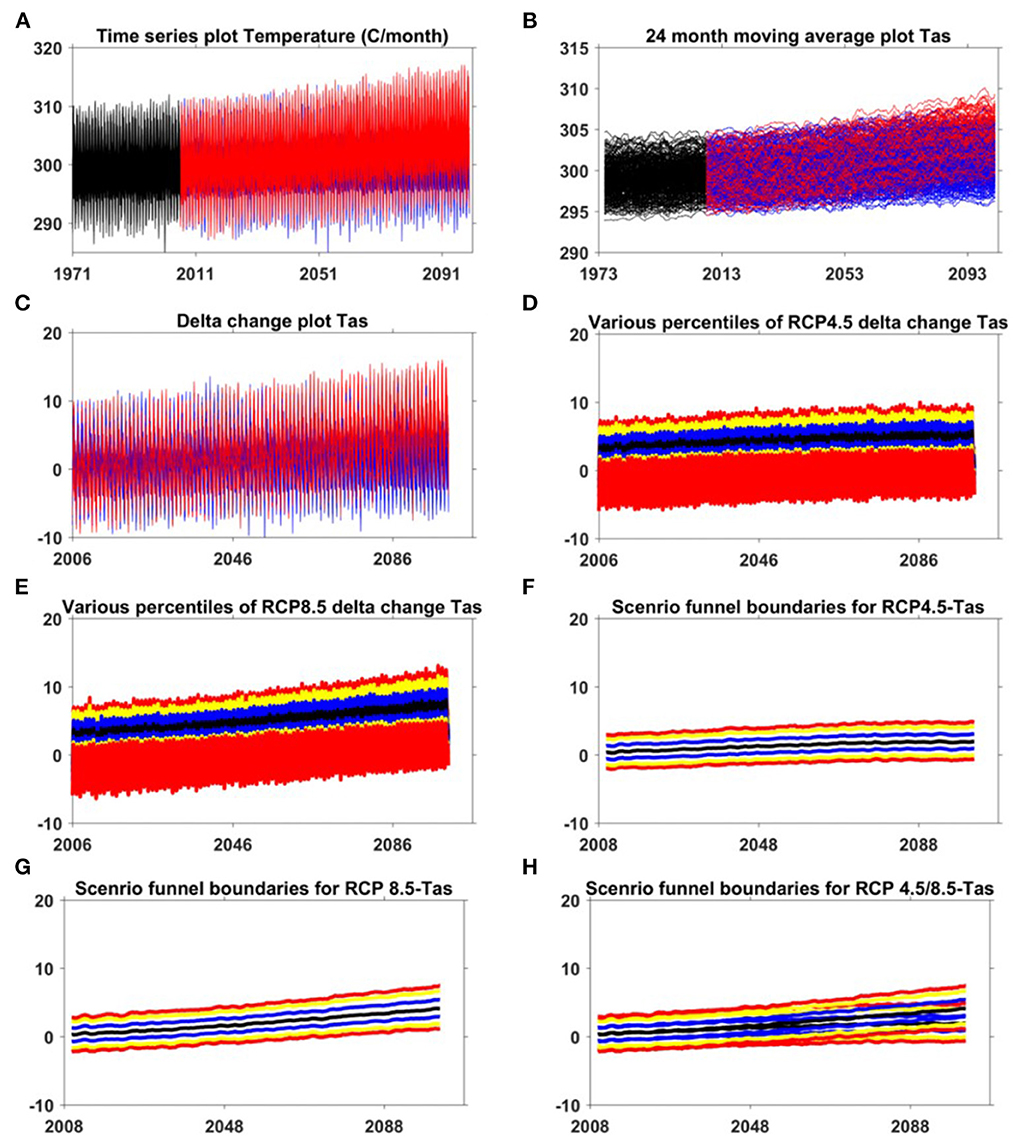
Figure 4. The plot of various steps in developing the scenario funnel for average surface temperature [T as, (A–H)] by data-analysis, (A) time-series plot of raw temperature data, (B) time-series plot of 24 month moving average temperature data, (C) time-series plot of delta change estimated for temperature, (D) various percentile values for RCP 4.5 for temperature (red: 5th, 95th; yellow:10th, 90th, blue: 25th, 75th, black: 50th percentiles), (E) various percentile values for RCP 8.5 for temperature (red: 5th, 95th; yellow:10th, 90th, blue: 25th, 75th, black: 50th percentiles), (F) Developing scenario funnel boundaries for RCP 4.5 from 24 month moving average of the percentiles (red: 5th, 95th; yellow:10th, 90th, blue: 25th, 75th, black: 50th percentiles), (G) Developing scenario funnel boundaries for RCP 8.5 from 24 month moving average of the percentiles (red: 5th, 95th; yellow:10th, 90th, blue: 25th, 75th, black: 50th percentiles), (H) scenario funnels for temperature. In (A–C), red and blue color represents the RCP 8.5 and RCP 4.5, respectively. (For interpretation of the references to color in this figure legend, the reader is referred to the web version of this article).
Based on global scenarios, the Intergovernmental Panel on Climate Change (IPCC) predicted an increase in the mean global climate ranging from 1.4 to 5.8°C by the end of this century (Intergovernmental Panel on Climate Change, 2007). Climate change is also projected to cause more frequent and intense El-Nino-Southern Oscillation (ENSO) events leading to widespread drought in some areas and extensive flooding. Consequently, such events will negatively impact the availability of water resources, food, agricultural security, human health, and biodiversity (Wara et al., 2005). Presently, studies on the impact of night time (minimum) temperature on tropical crops have been significantly improved, particularly in Southeast Asia and India (Nagarajan et al., 2010; Rao et al., 2014; Murugan et al., 2017). Indeed, the global scale minimum temperatures have increased twice as fast as the maximum temperature (Vose et al., 2005; María et al., 2013). Recently, South African scientists have demonstrated a significant negative influence of night time minimum temperature on coffee productivity in Coffea arabica (Craparo et al., 2015). Scenario analysis based on the Representative Concentration Pathway (RCP) (6.0) for Tanzanian high lands also predicted that there would be a drastic change in the future minimum temperature that will be very severe in the interior parts also where the major Arabica growing regions are located (Meinshausen et al., 2011).
Global average air temperature is predicted to increase by ~6.0°C by 2,100. The CO2 concentration is expected to increase to 940 μmol/mol and the precipitation to increase by 20.4% from existing conditions under the RCP 8.5 CC scenario. The Representative Concentration Pathway (RCP 4.5/8.5) predicts moderate to extreme variation in the temperature range from 0.5 to 8°C for the entire study location. Planters cultivate coffee under natural forests (shade-grown coffee), which is a purely sun-grown crop in eastern Africa. Under a modest climate change scenario (RCP 4.5), coffee can tolerate and yield reasonably because of the natural forest's buffering and shading effect. Approximately 8°C has been predicted for the Indian coffee hot spots under the RCP 8.5 climate change scenario. The hot spots will experience extreme temperatures coupled with the tremendous change in the precipitation levels (180% increased and 90% decreased) will have severe implications and negative impacts on the growth, development, and yield of coffee, cardamom, and pepper plants.
Under unusually low precipitation (90% decreases) scenarios, the coffee plants can be affected severely by disruption of photosynthesis, flowering and fruit set, and other physiological abnormalities. Thereby, the yield and quality of coffee berries will be negatively impacted under the changing climate scenario. The surface and subsurface soils become extremely complex due to extremely low rainfall, and the complete withering of young coffee plants can be anticipated depending on the slope gradient. High-intensity rainfall (180% increase) will cause the shedding of harvestable berries (matured coffee berries) besides flower-dropping during the blossom period. Honeybee activity is highly disturbed by incessant rainfall, affecting pollination and reducing the cardamom yield by up to 40% (the case of 2018). In pepper, pollination and berry development in the spikes are affected due to continuous rain. In some of the past years, the hot spots across the states have received even 30 inches of rainfall in a day during October-November that, caused 50% crop loss in coffee. Scientific evidence and information on climate change impacts based on RCP scenarios for coffee are very scanty. Still, published information on similar RCP scenarios for crops like hot pepper showed extreme negative impacts on physiological disorders and morphological deformities of Chillies (Capsicum) (Lee et al., 2017).
Variability in climatic factors and coffee yield in the hot-spots
India is the leading producer of coffee in Asia, where Arabica and Robusta are grown under significant acreage. Spatio-temporal variability in coffee yields was exhibited among Indian cardamom and coffee hotspots. Year-to-year variation in coffee yield was observed among the regions depending on the precipitation pattern and temperature influenced by ENSO of the respective years. The localized phenomenon, particularly the altitude and slope gradients, is also associated with the coffee yield variability. Declining and increasing trends were observed correspondingly for Tamil Nadu and Kerala hot spots, while the productivity was more or less static for the Karnataka region (Figure 5).
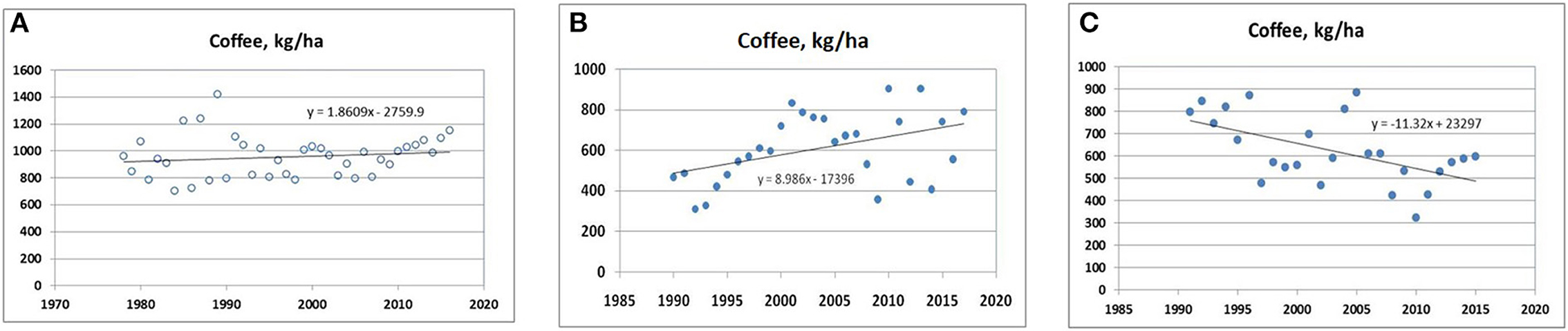
Figure 5. Coffee productivity trend. (A) Kodagu hills (Karnataka); (B) Cardamom hills (Kerala); (C) Lower Pulney hills (Tamil Nadu).
Insufficient rainfall during the flowering phase (Feb–Mar) and heavy intense rainfall during the maturity to ripening stage (Nov–Dec) caused yield losses in coffee. Such damage has been more pronounced in Arabica than in Robusta. Precipitation pattern before and after the first monsoon plays a significant role in deciding the yield of coffee in all three hot spots. Most of the coffee plantations in LPH (Tamil Nadu) are located on the lee side of the southwest monsoon; therefore, the region enjoys lesser annual rainfall. The minimum temperature levels were very high for the summer months (Jan–May), which, along with the poor distribution of summer months' rain, could have caused the decline in production. Recently, smallholding coffee farmers in southern India tend to abandon coffee fields because of the unfavorable market price. Many growers follow cost-cutting practices, including inputs like fertilizers, chemicals, and manures. As far as biotic stresses are concerned, white stem borer is a severe problem in Karnataka coffee-growing areas, but in other regions in Kerala and Tamil Nadu, the pest incidence is minimal. At present, Robusta coffee's productivity is increasing due to its tolerance to insect pests and diseases as well as environmental stress. Arabica coffee is experiencing stagnant productivity as well as lower market price.
The ongoing climate warming and precipitation change in these hot spots are two critical factors affecting coffee and cardamom yields. In coffee, the Arabica type is on the higher side of the risk than the Robusta. The early maturity of coffee has both positives and negatives. For example, a better market price can be anticipated for Arabica, but at the same time, heavy downpours of up to 30 inches of rainfall in a day can destroy the crop during the second monsoon months like October–November. Such intense rainfall during November in the Karnataka region is less expected, so it is advantageous there. However, in Kerala and Tamil Nadu, where the northeast monsoon is still active, during which time the matured crop can get destroyed, it is not benevolent for Kerala and Tamil Nadu planters. Early summer showers (February) in all regions lead to early crop, but atmospheric warming, intense rainfall, and strong surface winds significantly destroy coffee and cardamom. Extension of the dry period by a month (March–April) leads to late crop, which coincides with heavy rainfall during winter monsoon (November–December) and damages the coffee crop severely in Kerala and Tamil Nadu regions. Overall, it is observed that the production of coffee is at increased risk in all coffee-growing hotspots in southern India. Therefore, planters need extra care to cope with unfavorable climatic conditions and variability.
Recommended adaption strategies and farmers perspectives
There has been a significant change in the forest tree species composition of cardamom-coffee hot spots over the last three decades. At least 50% of trees in the CH hot spot are composed of only three species, namely Vernonia arborea (Karana), Artocarpus heterophyllus (Jack), and Toonaciliata (Cedar). The population of notified native forest trees is declining, and they are all ancient and facing severe elimination. To maintain uniform and required shade level, the planters must increase tree density and diversity significantly. The other two major species, except for Jack, are highly vulnerable to wind damage. Maintaining good shade levels in coffee plantations in Kerala and Tamil Nadu delays early maturity; the berries ready to harvest will not suffer from a heavy downpour during the winter monsoon period.
Interestingly, the Karnataka regions escape from this problem since the monsoon rain never damages the matured coffee berries in November–December. Planters also use chemicals (Cobalt sulfate 375 g/ha) to delay the maturity and ripening of coffee berries by at least 15–20 days so that the damage and loss of mature coffee beans due to heavy rain can be thwarted. Anticipating the extreme temperature and precipitation levels as given by the RCP climate change scenario, multiple canopy levels are recommended with various tree species, mainly species with broad leaves, because needle-shaped leaves (Grevillea robusta) allow more sunlight to fall on coffee and cardamom canopy, which will have serious implication under future warming and precipitation levels. Our objective is to reduce air and crop canopy temperature levels for which tropical evergreen species are the most preferred. Planters in Tamil Nadu and Kerala's lower rainfall areas go for subsoil irrigation and fertigation to safeguard the younger coffee plants from drought and water scarcity. Most planters and growers in the hot spots perceive and recognize drought and climate change as the most critical aspect affecting the productivity of long-duration and perennial crops, followed by irrigation water availability and scarcity and insect pests and disease problems. Nearly 60% of farmers rank unfavorable market price as an essential factor affecting agricultural sustainability, followed by problems with pesticides (39%) and water quality degradation (28%) in the hot spot areas. These are concerned with the present and future health externalities. Shade level, forest degradation (27%), and loss of topsoil and degradation (15%) rank as the penultimate and ultimate problems of the planters (Table 3).
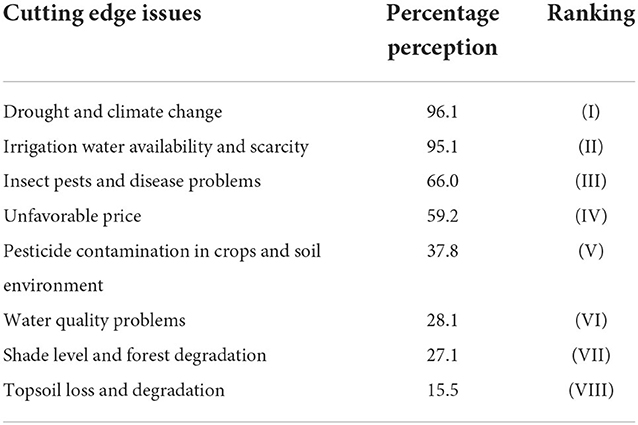
Table 3. Planters' perception on the cutting-edge issues including drought and climate change affecting sustainability of cardamom farming (n = 103).
Ecological physiology of cardamom, coffee and black pepper
Key physiological parameters of cardamom, coffee, and black pepper those are common to all cardamom-coffee hotspots in India are presented in Figure 6. Anet in C. robusta and Elettaria cardamomum have shown single peak curves during the day, while Piper nigrum showed two-peak curves (Figure 6A). But the time when the Anet peak occurred was different between the crops, being at 1,100 h for C. robusta and at 09:00 h for both P. nigrum and E. cardamomum. The diurnal patterns of gs in two crops coffee (robusta) and small cardamom were showed one-peak curves, while P. nigrum showed two-peak curves. A midday depression of gs was exhibited in all three crops, but the stomata did not close entirely. The time when the depression occurred was 11:00 h for cardamom and pepper and 13:00 h for coffee. Midday depression of leaf Anet is usually found in C3 plants such as rice (Ishihara and Saitoh, 1987), and soybean (Huck et al., 1983). We also found midday depression of Anet in the all C3 crops (Figure 6B), although it was not so typical. Many researchers have reported that stomatal closure was the main cause of the reduction in the photosynthetic rate (Hirasawa et al., 1989; Wakabayashi et al., 1996). A close positive correlation between Anet and gs (r2 = 0.776**, r2 = 0.723** and r2 = 0.544** for E. cardamomum, C. robusta, and P. nigrum, respectively) had indicated that the midday depression of Anet in all three species might be due to stomatal closure (Figure 6C). But the increase in gs was always procession by a decrease in Ci in C. robusta and E. cardamomum, but in P. nigrum the increased gs was always accompanied by an increase in Ci (Figure 6D). The low Ci values upon high gs values indicated that the midday depression of Anet was not caused primarily by the lower gs, but rather by non-stomatal factors in C. robusta and E. cardamomum and depression of Anet was caused primarily by the lower gs, relatively by stomatal factors.
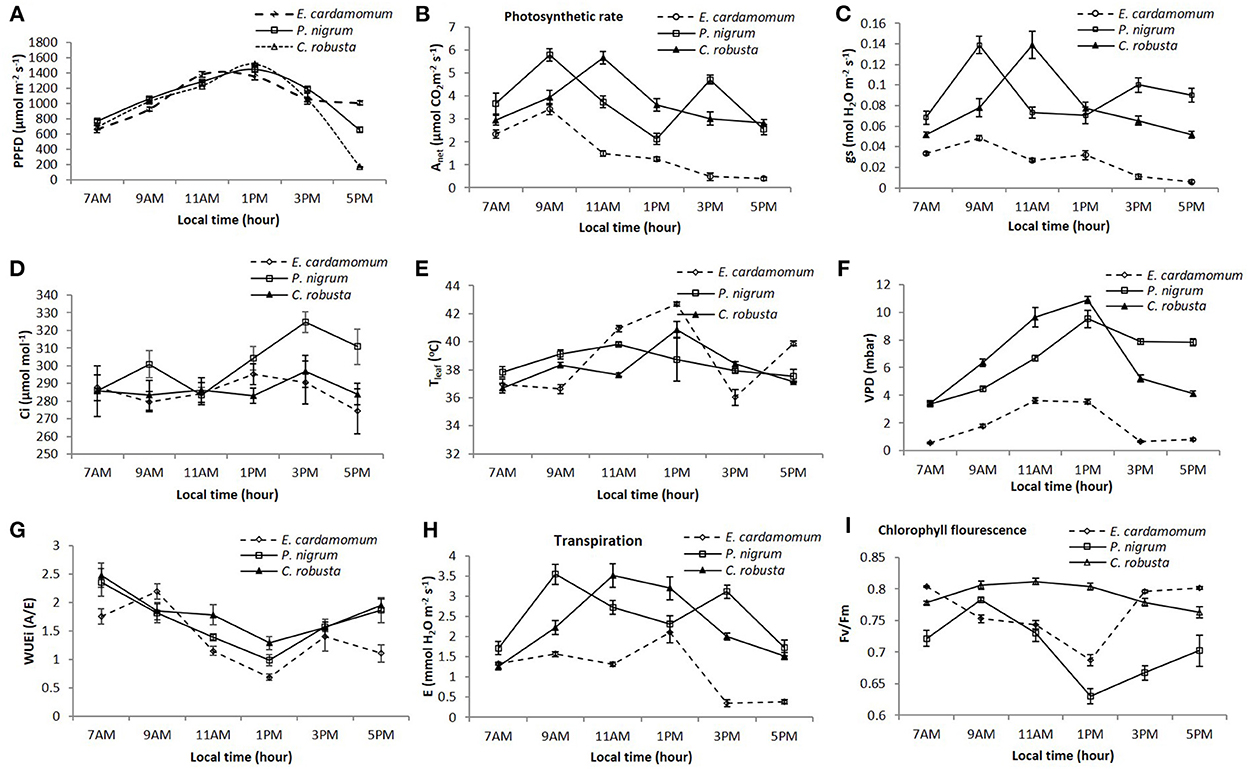
Figure 6. Eco-physiological parameters of cardamom, black pepper, and coffee. (A) Photosynthetic photon flux density (PPFD). (B) Net photosynthetic rate (Anet). (C) Stomatal conductance (gs). (D) Internal CO2 concentration (Ci). (E) Leaf temperature (Tleaf). (F) Vapor pressure deficit (VPD). (G) Intrinsic water use efficiency (WUEi). (H) Transpiration rate (E). (I) Chlorophyll fluorescence (Fv/Fm).
Like many other tropical plant species C. robusta, and E. cardamomum experience a pronounced midday depression in net photosynthesis (Anet) and stomatal conductance (gs) under tropical Indian climatic conditions during summer. P. nigrum did show a slight recovery after midday depression in Anet and gs but the overall pattern is different from the other two species investigated. These differences could be due to differences in leaf types with complex interactions with environmental variables. Similar differences in diurnal patterns were reported earlier for C. robusta, and E. cardamomum (Murugan et al., 2013; Alagupalamuthirsolai et al., 2018). The depressions in Anet and gs could also be due to diurnal variation in leaf temperature (36–42°C) in all these crops, but the temperature variation during peak photosynthesis and depression is 3°C (37–40°C) in C. robusta, 1°C (38–39°C) in P. nigrum and 6°C (36–42°C) in E. cardamomum during the days under observation. The diurnal changes in temperature and Anet also shows that temperature variation may not have a direct effect on afternoon decline in Anet in C. robusta, and P. nigrum but its E. cardamomum may have slight direct effect on Anet during 09:00 to 13:00 h.
We observed the peak values of Anet occurred at 11:00 h for C. robusta and P. nigrum and at 09:00 h for E. cardamomum. Associating with PPFD (Figures 6B,E), it was shown that Anet declined after 11:00 h (Tleaf 37.7°C) in C. robusta, the 1st declines after 09:00 h (Tleaf 39.1oC) and the 2nd decline after 15:00 h (Tleaf 38°C) in P. nigrum and also Anet declined after 09:00 h (Tleaf 36.6°C) in E. cardamomum, whereas the PPFD still increased higher than 15:00 μmol/m−2 s−1 between 11:00 and 13:00 h (Figure 6A). This is exactly the temperature at which Rubisco would be deactivated. The conditions with Anet peak are in fact the optimal combination of multiple environmental factors for leaf photosynthesis. Between 09:00 and 15:00 h, the ambient temperature was higher than 30°C. High temperature affects the photosynthetic functions of crop plants by its effects on the rate of chemical reactions and on structural organization (Pastenes and Horton, 1996). In C3 plants, ribulose-1,5 bisphosphate carboxylase/oxygenase (Rubisco) activity is limited by the CO2 concentration. As temperature increases, the affinity of the enzyme for CO2 and the solubility of CO2 decrease. Together with Rubisco deactivation with temperature increasing, Anet declines at leaf temperatures greater than 32°C in C3 plants (Crafts-Brandner and Salvucci, 2002). So, the effects of temperature on Rubisco activity without other compensation mechanisms could be a reason rather than for Anet in E. cardamomum and P. nigrum being lower beyond 30°C and in C. robusta beyond 32°C. By comparing PPFD at 09:00 h (928 and 1,061 μmol/m−2 s−1 in E. cardamomum and P. nigrum respectively) and 1,100 h (1,230 μmol/m−2 s−1 in C. robusta), we give evidence that leaf photosynthesis of cardamom and black pepper was less adaptive to high irradiance and atmospheric temperature than that of C. robusta.
Leaf temperature increased throughout the day until respective maximum values of 40.8, 38.7, and 42.6°C and values of VPD 1.08, 0.95, and 0.36 mbar were reached in C. robusta, P. nigrum, and E. cardamomum, respectively (Figure 6F). The decline in Anet and gs was associated with high PPFD and substantial increase in VPD from 09:00 to 13:00 h in P. nigrum and E. cardamomum, and 11:00 to 13:00 h in C. robusta. The potential effects of leaf temperature on the diurnal pattern of the photosynthetic rates for a given temperature at saturation PPFD (>900 μmol/m−2 s−1), the Anet measured in the afternoon was always lower than that in the morning in C. robusta and E. cardamomum, but in P. nigrum it was higher at 15:00 h than that of the morning. This shows that a circadian component was not essential for the development of a midday depression of Anet and gs in P. nigrum which is apparent from the experiments in which the PPFD and VPD were reached high (Figure 6F). However, in C. robusta and E. cardamomum, it appears that high PPFD and high VPD may not disturb the overall pattern of diurnal variation in Anet and/or gs during entire day which in turn may have been governed by circadian components. Therefore, we presume that crops like P. nigrum grown under low VPD conditions (monsoon) when exposed to a relatively dry environment (summer) show strong stomatal response to dry air atmosphere with double peaked diurnal activity. In the case of C. robusta and E. cardamomum the circadian component like leaf folding mechanism to some extent endows the leaf with mechanisms that reduce the exposing area to the irradiance and conserve water without undergoing large stomatal changes. This may be the reason for the one peak response in C. robusta and E. cardamomum.
Leaves of all the three species would withstand much higher PPFD (up to 1,600 μmol/m−2 s−1) than their saturation limit (500–800 μmol/m−2 s−1) without damaging the photosynthesis significantly, when VPD was favorable. In P. nigrum the effect of high VPD was instantaneous and gas exchange rates remained low as long as high VPD was maintained. The gas exchange rates were improved only when VPD was lowered and results provided enough evidence for the dominant role of VPD in P. nigrum. In the second, the reduction in Anet and gs in P. nigrum due to high VPD (40%−50%) was higher as compared to that of high PPFD (10%−20%) during 09:00 to 13:00 h. And also, reduction in Anet and gs in E. cardamomum due to high VPD influencing (103%) than PPFD (48%) only during 09:00 to 11:00 h, after 11:00 h E. cardamomum undergoes circadian leaf folding mechanism during midday to reduce the exposure to irradiance without undergoing large stomatal chances. However, in C. robusta it appears that high PPFD and high VPD may not disturb the overall pattern of diurnal variation in Anet and gs which in turn may have been rather governed by circadian components like leaf folding mechanism to reduce irradiance exposure. It has been shown in trees Acacia auriculiformis (growing in foot hills of the WG) that as long as VPD was held constant, the photosynthetic capacity and stomatal conductance were almost insensitive to changes in temperature (in the range 27–38°C) and photosynthesis rate began to decline with increasing temperature only after threshold VPD was exceeded. In our observations the temperature variations were not large and a decline in photosynthesis set in only after a critical VPD had been exceeded. This threshold VPD may be different for different leaf types and may be influenced by growth conditions. Thus C. robusta might have a highest critical VPD of 9 mbar as compared to 4.45 and 1.78 mbar for P. nigrum and E. cardamomum respectively (Figure 6F). From these observations it appears that leaves of P. nigrum and E. cardamomum are susceptible to light and/or VPD stress to a varying extent compared to C. robusta which was relatively less affected when exposed to the above-mentioned stress conditions.
The potential photosynthetic capacity of C. robusta leaves is relatively high (30 μmol CO2/m−2 s−1) and could be even greater than that of P. nigrum and E. cardamomu (Murugan et al., 2009b; Alagupalamuthirsolai et al., 2018) and also other crops such as wheat and spinach (Parry et al., 2011). However, their actual Anet is relatively low (typically in the range of 4–11 μmol/m−2 s−1) at saturating light and current atmospheric [CO2] when compared with many others tropical tree crops (DaMatta, 2004). Given these facts, it is clear that such a low Anet is due mainly to large limitations to CO2 diffusion from the atmosphere to the chloroplasts (Batista et al., 2012). This is in good agreement with the low values of gs and gm (mesophyll conductance, i.e., the conductance to CO2 from the intercellular air spaces to the chloroplast carboxylation sites). Such large diffusional limitations to Anet suggest that C. robusta might benefit from increasing [CO2] relatively more than P. nigrum and E. cardamomum with low diffusional limitations to photosynthesis.
C. robusta has evolved in shaded environments and its photosynthetic apparatus becomes saturated at relatively low PPFD (DaMatta, 2004). Therefore, when the plant is grown in open fields, leaves can absorb more light energy than can be used in photosynthesis, which could increase the probability of formation of highly reactive oxygen species (ROS) and triplet chlorophyll production. Under future climate warning as well as elevated [CO2], more light energy is required to saturate photosynthesis (Ramalho et al., 2013), thus a proportional lower energy level will be available to promote cell oxidative conditions; furthermore, the anticipated lower production of ROS (e.g., hydrogen peroxide) via decreases in photorespiration rates under enhanced [CO2] should also contribute to decrease the oxidative stress and, ultimately, promoting a better physiological and agronomic performance under elevated [CO2].
The differences in WUEi during 07:00 to 11:00, 09:00 to 15:00, and 11:00 to 13:00 h were mainly due to their specific ability to maintain Anet rather than changes in transpirational water loss in P. nigrum, E. cardamomum, and C. robusta respectively (Figures 6G,H). Differences in WUEi during 11:00 to 15:00 and 07:00 to 09:00 h were mainly due to their specific ability in changes in E rather than their capability to maintain Anet in E. cardamomum and P. nigrum respectively and also during 07:00 to 11:00 and 13:00 to 17:00 h in C. robusta that showed more influence of E. cardamomum than Anet. The results of changes in WUEi of C. robusta and small cardamom which were mostly influenced by E during diurnal pattern. The reason that C. robusta avoids heat load deposition on leaves from 11:00 h onwards by doing partial leaf folding mechanism to reduce transpirational water loss. Clearly, it is a tendency of higher WUEi in C. robusta plants, providing a promising mechanism for the maintenance of productivity, and hence, fitness advantage in water deficit environments (Farquhar et al., 1989). In combination with gas exchange, it can be suggested that higher WUEi resulted primarily from the maintenance of high photosynthetic capacity and down-regulation of stomatal conductance and transpiration controlling the internal plant water status (Scartazza et al., 1998).
Leverenz et al. (1990) reported that a decrease in maximum carboxylation rate occurs at the primary stage of photoinhibition. In this study, Fv/Fm of three species decreased with the increased PPFD in the morning, then recovered during the afternoon when PPFD decreased (Figure 6I). These reversible changes suggest that a down-regulation of PSII might happen in photosynthetically active leaves. This type of response may reflect a protective mechanism in avoiding photodamage to the photosynthetic apparatus under excess irradiance (Demmig-Adams and Adams, 1992). For leaves under high irradiance at midday, along with depressed photosynthetic rates, the excessive excited energy must be safely dissipated; otherwise, the photosynthetic system will suffer photoinhibitory damage unless compensatory mechanisms alleviate photoinhibition are operational (Mattos et al., 1997; Graßes et al., 2002). The maximum PSII efficiency Fv/Fm is widely used as an indicator of photoinhibition (Ort and Baker, 2002). In this study, reversible change in Fv/Fm was found in black pepper and cardamom during the day, suggesting photoprotection rather than photodamage occurred. The decrease in Fv/Fm is likely due to the reversible inactivation or downregulation of PSII, rather than the photodamage to PSII or loss of D1 protein (Demmig-Adams et al., 1996).
Conclusion
Our results from the RCP scenario analyses for the hot spots indicate that future air temperature and precipitation levels will vary highly. An increase in air temperature by 8°C and precipitation change by 80% (reduction) is likely to cause significant changes in all physiological parameters, including WUE of all these species (coffee, cardamom, and black pepper), an increase in disease incidences, and intensities. The observed historical data also showed reduced productivity of these crops depending on each hot spot's temperature and precipitation pattern. Therefore, the growth, development, and yield of cardamom, coffee, and black pepper in these hot spots would be vulnerable and impacted negatively, warranting the prioritization of climate mitigation and adaptation strategies. Only then, the livelihoods of Milhous of farmers and laborers can be protected.
Data availability statement
The raw data supporting the conclusions of this article will be made available by the authors, without undue reservation.
Author contributions
MM and AA developed the methodology and conceived the work. RR, KA, KK, JR, and MD collected and complied data. MM, AA, and MA analyzed the data and wrote the manuscript. All authors contributed to the article and approved the submitted version.
Funding
This article was based upon work partially supported by National Science Foundation Grant no. 1735235 awarded as part of the National Science Foundation Research Traineeship and USDA-NIFA capacity building grant 2017-38821-26405.
Acknowledgments
This paper is dedicated in the loving memory of Prof. Rangaswamy Ramesh, Climatologist, NISER, Odisha whose inspiration made the senior author publish this paper.
Conflict of interest
The authors declare that the research was conducted in the absence of any commercial or financial relationships that could be construed as a potential conflict of interest.
Publisher's note
All claims expressed in this article are solely those of the authors and do not necessarily represent those of their affiliated organizations, or those of the publisher, the editors and the reviewers. Any product that may be evaluated in this article, or claim that may be made by its manufacturer, is not guaranteed or endorsed by the publisher.
References
Ainsworth, E. A., and Ort, D. R. (2010). How do we improve crop production in a warming world? Plant Physiol. 154, 526–530. doi: 10.1104/pp.110.161349
Alagupalamuthirsolai, M., Ankegowda, S. J., and Aravind, S. (2018). Factors determining the midday depression of photosynthesis in small cardamom (Elettaria cardamomum Maton) under summer climate. Multilogic Sci. 7,526–527.
Allan, C., Xia, J., and Pahl-Wostl, C. (2013). Climate change and water security: challenges for adaptive water management. Curr. Opin. Environ. Sustain. 5, 625–632. doi: 10.1016/j.cosust.2013.09.004
Anandhi, A., Allan, F., Donald, C., Pierson, E., Schneiderman, M., Mark, S. Z., et al. (2011). Examination of change factor methodologies for climate change impact assessment. Water Resour. Res. 47, W03501. doi: 10.1029/2010WR009104
Anandhi, A., Anjali, S., and Siera, S. (2018). Can meta-analysis be used as a decision-making tool for developing scenarios and causal chains in eco-hydrological systems? Case study in Florida. Ecohydrology 11, e1997. doi: 10.1002/eco.1997
Anandhi, A., and Bentley, C. (2018). Predicted 21st century climate variability in southeastern U.S. using downscaled CMIP5 and meta-analysis. Catena 170, 409–420. doi: 10.1016/j.catena.2018.06.005
Anandhi, A., and Blocksome, C. (2017). Developing adaptation strategies using an agroecosystem indicator: variability in crop failure temperatures. Ecol. Indic. 76, 30–41. doi: 10.1016/j.ecolind.2016.12.013
Anandhi, A., Omani, N., Chaubey, I., Horton, R., Bader, D. A., and Nanjundiah, R. S. (2016a). Synthetic scenarios from CMIP5 model simulations for climate change impact assessments in managed ecosystems and water resources: case study in south asian countries. Transl. Am. Soc. Agric. Biol. Eng. 59, 1715–1731. doi: 10.13031/trans.59.11585
Anandhi, A., Perumal, S., Gowda, P. H., Knapp, M., Hutchinson, S., John, H. J., et al. (2013b). Long-term spatial and temporal trends in frost indices in Kansas, USA. Clim. Change 120, 169–181. doi: 10.1007/s10584-013-0794-4
Anandhi, A., Puneet, S., Rabi, H. M., Richard, G. L., Sumit, S., and Jasmeet, L. (2022). Methodologies and principles for developing nexus definitions and conceptualizations: lessons from few nexus studies. J. ASABE. doi: 10.13031/ja.14539. [Epub ahead of print].
Anandhi, A., Srinivas, V., Kumar, D. N., and Nanjundiah, R. S. (2012). Daily relative humidity projections in an Indian riverbasin for IPCC SRES scenarios. Theor. Appl. Climatol. 108, 85–104. doi: 10.1007/s00704-011-0511-z
Anandhi, A., Steiner, J., and Bailey, N. A. (2016b). System's approach to assess the exposure of agricultural production to climate change and variability. Clim. Change 136, 647–659. doi: 10.1007/s10584-016-1636-y
Anandhi, A., Zion, M. S., Gowda, P. H., Pierson, D. C., Lounsbury, D., and Allan, F. (2013a). Past and future changes in frost day indices in Catskill Mountain region of New York. Hydrol. Process. 27, 3094–3104. doi: 10.1002/hyp.9937
Barros, R. S., Maestri, M., and Coons, M. P. (1978). The physiology of flowering in coffee: a review. J. Coffee Res. 8, 29–73.
Batista, K. D., Araújo, W. L., Antunes, W. C., Cavatte, P. C., Moraes, G. A. B. K., Martins, S. C. V., et al. (2012). Photosynthetic limitations in coffee plants are chiefly governed by diffusive factors. Trees 26, 459–468. doi: 10.1007/s00468-011-0606-2
Browning, G. (1975). ‘Shoot growth in Coffea arabica L. I. Response to rainfall when the soil moisture status and gibberellin supply are not limiting'. J. Horticul. Sci. 50, 1–1. doi: 10.1080/00221589.1975.11514596
Cannell, M. G. R. (1985). “Physiology of the coffee crop,” in Coffee, eds M. N. Clifford and K. C. Willson (Boston, MA: Springer). p. 108–134. doi: 10.1007/978-1-4615-6657-1_5
Crafts-Brandner, S. J., and Salvucci, M. E. (2002). Sensitivity of photosynthesis in a C4 plant, maize, to heat stress. Plant Physiol. 129, 1773–1780. doi: 10.1104/pp.002170
Craparo, A. C. W., Van Astenb, P. J. A., Läderachc, P., Jassogneb, L. T. P., and Graba, S. W. (2015). Coffea arabica yields decline in Tanzania due to climate change: global implications. Agr. Forest Meteorol. 207, 1–10. doi: 10.1016/j.agrformet.2015.03.005
DaMatta, F. M. (2004). Ecophysiological constraints on the production of shaded and unshaded coffee: a review. Field Crops Res. 86, 99–114. doi: 10.1016/j.fcr.2003.09.001
Demmig-Adams, B., and Adams, W. W. (1992). Photoprotection and other responses of plants to high light stress. Annu. Rev. Plant Physiol. Plant Mol. Biol. 43, 599–626. doi: 10.1146/annurev.pp.43.060192.003123
Demmig-Adams, B., Adams, W. W, Barker, D. H., Logan, B. A., Bowling, D. R., and Verhoeven, A. S. (1996). Using chlorophyll fluorescence to assess the fraction of absorbed light allocated to thermal dissipation of excess excitation. Physiol. Plant. 98, 253–264. doi: 10.1034/j.1399-3054.1996.980206.x
Esbjerg, P., and Sigsgaard, L. (2014). Phenology and pest status of Agrotis segetum in a changing climate. Crop Prot. 62, 64–71. doi: 10.1016/j.cropro.2014.04.003
Farquhar, G. D., Ehleringer, J. R., and Hubick, K. T. (1989). Carbon isotope discrimination and photosynthesis. Annu. Rev. Plant Physiol. 40, 503–537. doi: 10.1146/annurev.pp.40.060189.002443
Fyson, P. F., Bourne, L., and Fyson, F. (1915). The flora of the Nilgri and Pulney Hill-tops [PhD Thesis]. Presidency College, Madras.
Graßes, T., Pesaresi, P., Schiavon, F., Varotto, C., Salamini, F., Jahans, P., et al. (2002). The role of ΔpH-dependent dissipation of excitation energy in protecting photosystem II against light induced damage in Arabidopsis thaliana. Plant Physiol. Biochem. 40, 41–49. doi: 10.1016/S0981-9428(01)01346-8
Hirasawa, T., Iida, Y., and Ishihara, K. (1989). Dominant factors in reduction of photosynthetic rate affected by air humidity and leaf water potential in rice plants. Jpn. J. Crop Sci. 58, 383–389. doi: 10.1626/jcs.58.383
Huck, M. G., Ishihara, K., Peterson, C. M., and Ushijima, T. (1983). Soybean adaptation to water stress at selected stages of growth. Plant Physiol. 73, 422–427. doi: 10.1104/pp.73.2.422
Huxley, P. A. (1970). “Some aspects of the physiology of Arabica coffee - the central problems and the need for a synthesis,” in Physiology of Tree Crops, eds L. C. Luckwill and C. V. Cutting (London, New York and San Francisco: Academic Press), 253–268.
Intergovernmental Panel on Climate Change. (2007). “Summary for policymakers,” in Climate Change 2007 - Mitigation of Climate Change: Working Group III contribution to the Fourth Assessment Report of the IPCC (Cambridge: Cambridge University Press), 1–24. doi: 10.1017/CBO9780511546013.003
Ishihara, K., and Saitoh, K. (1987). Diurnal courses of photosynthesis, transpiration, and diffusive conductance in the single-leaf of the rice plants grown in the paddy field under submerged condition. Jpn. J. Crop Sci. 56, 8–17. doi: 10.1626/jcs.56.8
Karmakar, N., Chakraborty, A., and Nanjundiah, R. S. (2015). De-creasing intensity of monsoon low-frequency intraseasonal var-iability over India. Environ. Res. Lett. 10:054018. doi: 10.1088/1748-9326/10/5/054018
Lansigan, F., De Los Santos, W., and Coladilla, J. (2000). Agronomic impacts of climate variability on rice production in the Philippines. Agric. Ecosyst. Environ. 82, 129–137. doi: 10.1016/S0167-8809(00)00222-X
Lavalle, C., Micale, F., Houston, T. D., Camia, A., Hiederer, R., Lazar, C., et al. (2009). Climate change in Europe. 3. Impact on agriculture and forestry. A review. Agron. Sustain. Dev. 29, 433–446. doi: 10.1051/agro/2008068
Lee, S. G., Kim, S. K., Lee, H. J., Lee, H. S., and Lee, J. H. (2017). Impact of moderate and extreme climate change scenarios on growth, morphological features, photosynthesis, and fruit production of hot pepper. Ecol. Evol. 201, 197–206. doi: 10.1002/ece3.3647
Leverenz, J. W., Falk, S., Pilström, C. M., and Samuelsson, G. (1990). The effects of photoinhibition on the photosynthetic light-response curve of green plant cells (Chlamydomonas reinhardtii). Planta. 182, 161–168. doi: 10.1007/BF00197105
María, M. S. M., Brunet, M., Sigró, J., Aguilar, E., Groening, J. A. A., Bentancur, O. J., et al. (2013). Warming and wetting signals emerging from analysis of changes in climate extreme indices over South America. Glob. Planet. Change 100, 295–307. doi: 10.1016/j.gloplacha.2012.11.004
Mattos, E. A., de Grams, T. E. E., Ball, E., Franco, A. C., Haag-Kerwer, A., Herzog, B., et al. (1997). Diurnal patterns of chlorophyll a fluorescence and stomatal conductance in species of two types of coastal tree vegetation in southeastern Brazil. Trees 11, 363–369. doi: 10.1007/PL00009680
Meinshausen, M., Smith, S. J., Calvin, K., Daniel, J. S., Kainuma, M. L. T., Lamarque, J. F., et al. (2011). The RCP greenhouse gas concentrations and their extensions from 1765 to 2300. Clim. Change. 213. doi: 10.1007/s10584-011-0156-z
Murugan, M., Ashokkumar, K., Alagupalamuthirsolai, M., Anandhi, A., Ravi, R., Dhanya, M. K., et al. (2022). Understanding the effects of cardamom cultivation on its local environment using novel systems thinking approach-the case of Indian Cardamom Hills. Front. Sustain. Food Syst. 6, 728651. doi: 10.3389/fsufs.2022.728651
Murugan, M., Mukund, V., Ramesh, R., Hiremath, M. B., Josephrajkumar, A., and Shetty, P. K. (2008). Centennial rainfall variation in semi arid and tropical humid environments in the cardamom hill slopes, southern Western Ghats, India. Caspian J. Environ. Sci. 6, 31–39. doi: 10.54207/bsmps1000-2008-27J960
Murugan, M., Ravi, R., Anandhi, A., Sajan, K., and Dhanya, M. K. (2017). Pesticide use in Indian cardamom needs change in cultivation practices. Curr. Sci. 113, 1058–1063. doi: 10.18520/cs/v113/i06/1058-1063
Murugan, M., Shetty, P. K., Anandhi, A., and Ravi, R. (2013). Present and future climate change in Indian Cardamom Hills: implications for cardamom production and sustainability. Br. J. Environ. Climate Change 2, 368–390. doi: 10.9734/BJECC/2012/1495
Murugan, M., Shetty, P. K., Anandhi, A., Ravi, R., Alappan, S., Vasudevan, M., et al. (2009a). Rainfall changes over tropical montane cloud forests of southern Western Ghats, India. Curr. Sci. 97, 1755–1760.
Murugan, M., Shetty, P. K., Ravi, R., Anandhi, A., and Rajkumar, A. J. (2012). Climate change and crop yields in the Indian Cardamom Hills, 1978–2007 CE. Clim. Change 110, 737–753. doi: 10.1007/s10584-011-0115-8
Murugan, M., Shetty, P. K., Ravi, R., and Subbiah, A. (2009b). The physiological ecology of cardamom (Elettaria cardamomum M) in Cardamom Agroforestry system. Int. J. Environ. Res. 3, 35–44.
Nagarajan, S., Jagadish, S. V. K., Hari Prasad, A. S., Thomar, A. K., Anand, A., Pal, M., et al. (2010). Local climate affects growth, yield and grain quality of aromatic and non-aromatic rice in northwestern India. Agric. Ecosyst. Environ. 138, 274–281. doi: 10.1016/j.agee.2010.05.012
Ort, D. R., and Baker, N. R. (2002). A photo protective role for O2 as an alternative electron sink in photosynthesis? Curr. Opin. Plant Biol. 5, 193–198. doi: 10.1016/S1369-5266(02)00259-5
Parry, M. A., Reynolds, M., Salvucci, M. E., Raines, C., Andralojc, P. J., Zhu, X. G., et al. (2011). Raising yield potential of wheat. II. Increasing photosynthetic capacity and efficiency. J. Exp. Bot. 62, 453–467. doi: 10.1093/jxb/erq304
Pastenes, C., and Horton, P. (1996). Effects of high temperature on photosynthesis in beans. I. Oxygen evolution and chlorophyll fluorescence. Plant Physiol. 112, 1245–1251. doi: 10.1104/pp.112.3.1245
Raith, M., Srikantappa, C., Buhl, D., and Koehler, H. (1997). Ultra-high temperature metamorphism and multistage decompression evolution of sapphirine granulites from Palni Hills, South India. J. Metamorph. Geol. 15, 379–399. doi: 10.1111/j.1525-1314.1997.00027.x
Ramalho, J. C., Fortunato, A. S., Goulao, L. F., and Lidon, F. C. (2013). Cold-induced changes in mineral content in leaves of Coffea spp. Identification of descriptors for tolerance assessment. Biol. Plant. 57, 495–506. doi: 10.1007/s10535-013-0329-x
Ramesh, K. V., and Goswami, P. (2014). Assessing reliability of regional climate projections: the case of Indian monsoon. Sci. Rep. 4, 04071. doi: 10.1038/srep04071
Rangan, H., Christian, A. K., and Lisa, A. (2010). Forest plantation, water availability, and regional climate change: controversies surrounding Acacia mearnsii plantations in the upper Palni Hills, southern India. Reg. Environ. Change 10, 103–117. doi: 10.1007/s10113-009-0098-4
Rao, B., Santhibhushan, C. P., Sandeep, V. M., Rao, V. U. M., and Venkateswarlu, B. (2014). Rising minimum temperature trends over India in recent decades: implications for agricultural production. Glob. Planet. Change 117, 1–8. doi: 10.1016/j.gloplacha.2014.03.001
Rena, A. B., Barros, R. S., Maestri, M., and Söndahl, M. R. (1994). “Coffee,” in Handbook of Environmental Physiology of Tropical Fruit Crops: Sub-Tropical and Tropical Crops, eds B. Schaffer, P. C. Andersen, Vol. II (Boca Raton, FL: CRC Press), 101–22.
Scartazza, A., Lauteri, M., Guido, M., and Brugnoli, E. (1998). Carbon isotope discrimination in leaf and stem sugars, water-use efficiency and mesophyll conductance during different developmental stages in rice subjected to drought. Funct. Plant Biol. 25, 489–498. doi: 10.1071/PP98017
Smith, M. (1895). The Kodaikkanal solar physics laboratory in India. Publ. Astron. Soc. Pac. 7, 113–116. doi: 10.1086/121008
Strasser, R. J., Ivastava, A., and Govindjee (1995). Polyphasic chlorophyll a fluorescence transient in plants and cyanobacteria. Photochem. Photobiol. 61, 32–42. doi: 10.1111/j.1751-1097.1995.tb09240.x
Vose, R. S., Easterling, D. R., and Gleason, B. (2005). Maximum and minimum temperature trends for the globe: an update through 2004. Geophys. Res. Lett. 32, 1–5. doi: 10.1029/2005GL024379
Wakabayashi, K., Hirasawa, T., and Ishihara, K. (1996). Analysis of photosynthesis depression under low leaf water potential by comparison of CO2 exchange and O2 evolution rates. Jpn. J. Crop Sci. 65, 590–598. doi: 10.1626/jcs.65.590
Walthall, C. L. (2012). Climate change and agriculture in the United States: effects and adaptation. USDA Tech. Bull. 1935, 1–186
Wara, M. W., Ravelo, A. C., and Delaney, M. L. (2005). Permanent El Nino-like conditions during the Pliocene warm period. Science 309, 758–761. doi: 10.1126/science.1112596
Keywords: tropical mountains, Representative Concentration pathway (RCP), spices and plantation agriculture, ecophysiology, climate change scenarios, India
Citation: Murugan M, Alagupalamuthirsolai M, Ashokkumar K, Anandhi A, Ravi R, Rajangam J, Dhanya MK and Krishnamurthy KS (2022) Climate change scenarios, their impacts and implications on Indian cardamom-coffee hot spots; one of the two in the world. Front. Sustain. Food Syst. 6:1057617. doi: 10.3389/fsufs.2022.1057617
Received: 29 September 2022; Accepted: 18 November 2022;
Published: 08 December 2022.
Edited by:
Abid Hussain, International Centre for Integrated Mountain Development, NepalReviewed by:
Nasir Mahmood, Pir Mehr Ali Shah Arid Agriculture University, PakistanMohammad Hossain, International Rice Research Institute (IRRI), Philippines
Copyright © 2022 Murugan, Alagupalamuthirsolai, Ashokkumar, Anandhi, Ravi, Rajangam, Dhanya and Krishnamurthy. This is an open-access article distributed under the terms of the Creative Commons Attribution License (CC BY). The use, distribution or reproduction in other forums is permitted, provided the original author(s) and the copyright owner(s) are credited and that the original publication in this journal is cited, in accordance with accepted academic practice. No use, distribution or reproduction is permitted which does not comply with these terms.
*Correspondence: Muthusamy Murugan, bXV0aHVwZXlhbkBnbWFpbC5jb20=; Kaliyaperumal Ashokkumar, YmlvdGVjaC5hc2hva0BnbWFpbC5jb20=