- 1Department of Agricultural Microbiology, Laboratory of Soil Microbiology, Institute of Agricultural Microbiology and Agricultural Production NAAS of Ukraine, Kyiv, Ukraine
- 2Department of Agroecology and Biosafety, Laboratory of Environmental Assessment of Agroecosystems, Institute of Agroecology and Environmental Management NAAS of Ukraine, Kyiv, Ukraine
Studies conducted in a stationary lysimeter experiment in the conditions of the washing water regime have shown that the use of PGPR for pre-sowing seed inoculation of agricultural crops reduces vertical migration of biogenic nutrients and water-soluble organic matter down the soil profile. The effect of seed inoculation with PGPR on the reduction of nutrient losses was not specific to the type of rhizobacteria and was similar for crops grown on different mineral fertilizers backgrounds (spring barley and winter rye seeds were inoculated with the nitrogen-fixing bacteria—Azospirillum brasilense 410 and A. brasilense 18-2, respectively, while maize seeds were inoculated with the phosphate-mobilizing Paenibacillus polymyxa KB). Seed inoculation has decreased nitrogen leaching down the soil profile by 4–9 kg/ha, phosphorus compounds—by 0.5–3.0 kg/ha, potassium—by 0.6–3.0 kg/ha, calcium—by 6–42 kg/ha, magnesium—by 3.0–6.0 kg/ha, water-soluble organic matter—by 0.8–8.0 kg/ha, subject to crop and norms of mineral fertilizers. Maize seeds inoculated with phosphorous-mobilizing P. polymyxa KB under crop cultivation on the cattle manure background did not affect the intensity of nutrient migration. On the other hand, the combination of green manure (narrow-leaved lupine as an intermediate crop) with pre-sowing seed inoculation had significantly reduced nutrient losses beyond the root zone soil layer. It is concluded that the use of PGPR in crop production on mineral and green manure backgrounds contributes to the preservation of soil fertility by limiting biogenic nutrients and water-soluble organic matter leaching with the water drainage down the soil profile. Pre-sowing seed inoculation had no significant effect on the vertical migration of nutrients in the soil on the background of cattle manure, due to the highly competitive environment created with the introduction of microorganisms from organic fertilizer, preventing the establishment of close interactions between PGPR and plants.
Highlights
- Plant growth promoting rhizobacteria (PGPR) reduce nutrients and water-soluble organic matter leaching with the drainage water down the soil profile under the cultivation of spring barley, maize and winter rye on mineral and green manure backgrounds.
- No reduction of nutrients along the soil profile was observed under maize cultivation on the background of 40 t/ha cattle manure.
- Seed treatment with PGPR improves crop yields grown on mineral and green manure backgrounds but has no effect on maize productivity grown on a cattle manure background.
Introduction
Soil bacteria and fungi play an integral part and are irreplaceable within the “soil-microorganism-plant” system (Curl and Truelove, 1986; Hamilton and Frank, 2001; Herman et al., 2006; Landi et al., 2006; Bulgarelli et al., 2013; Zhalnina et al., 2018; Berg et al., 2020). An optimal set of rhizospheric microorganisms provides plants with the proper mineral nutrition, protection from pathogens, and improved yield potential of the crops (Watt et al., 2006; Marschner, 2012; Fasusi et al., 2021). This indicates that crop-growing technologies should foresee the optimization of microbiological processes in the soil, including the adaptation of the composition of groups of microorganisms in the root spheres of plants. It is especially relevant for modern agriculture, which is significantly affected by the anthropogenic load on the soil with the subsequent negative impact on the soil biota.
Pre-sowing seed inoculation with the selected strains of agronomically-valuable microorganisms named as Plant Growth-Promoting Rhizobacteria (PGPR) (Kloepper and Schroth, 1978; Bashan and Holguin, 1998), has become a significant technological mean used for the optimization of the processes of formation of groups of soil microorganisms and improvement of the agricultural crops' technologies. Today many countries and companies are producing microbial preparations based on nitrogen-fixing, phosphate-mobilizing, growth-stimulating microorganisms and antagonists of phytopathogens. There is no doubt about their positive influence on the growth and development of crops, possessing either direct or indirect influence on their productivity. The effect of PGPR is largely associated with the processes of molecular nitrogen fixation, solubilization of phosphorus compounds, production of siderophores, physiologically active substances, including plant hormones, enhancement of nutrient uptake by plants, improvement of the general plant health by the synthesis of 1-aminocyclopropane-1-carboxylate (ACC) deaminase, which contributes to the crop tolerance to drought, soil salinity and heavy metal contamination (Bashan and Holguin, 2002; Volkogon et al., 2006; Lugtenberg and Kamilova, 2009; Babalola, 2010; Martínez-Viveros et al., 2010; O'Callaghan, 2016; Di Benedetto et al., 2017; Olanrewaju et al., 2017; Di Salvo et al., 2018; Jeyanthi and Kanimozhi, 2018; Khanna et al., 2019; Ilyas et al., 2020; Trivedi et al., 2020; Santoyo et al., 2021; Yasmin et al., 2021, 2022; Adedayo et al., 2022; Figueiredo et al., 2022; Volpiano et al., 2022). However, most studies of the PGPR do not question their effect on soil fertility in terms of the formation of nutrient reserves, as it is a priori believed that inoculation contributes to the improvement of soil fertility. There are practically no reports that could characterize the transformation of biogenic nutrients in the soil profile during the cultivation of bacterized plants.
Lysimeters provide a unique opportunity to study these issues, however, they are not often used due to the limitations of the relevant installations. The results obtained in lysimeters can be highly indicative and informative when determining the influence of various factors on the character of the vertical migration of compounds of biogenic elements.
Using the lysimeters we studied the effect of PGPR during the cultivation of various agricultural crops, namely spring barley, maize, and winter rye on their productivity and extent of the losses of biogenic nutrient compounds, namely nitrogen (NO3/NH4), phosphorous (P2O5), potassium (K2O), calcium (CaO) and magnesium (MgO) as well as water-soluble organic matter losses with the water drainage down the soil profile.
Materials and methods
The research was conducted in the stationary lysimeter installed at the Institute of Agricultural Microbiology and Agro-Industrial Production of the National Academy of Agrarian Sciences of Ukraine (Chernihiv). The lysimeter site is located in the zone of the washing water regime (Northern Ukraine, Polissya climate zone) and has 48 sections-lysimeters, placed in two parallel rows of 24 lysimeters each. Receiver vessels are installed underneath the lysimeters to collect infiltrate. Lysimeters are concrete, bulk type in design and simulate the profile of sod-podzolic sandy soil [organic matter content−1.1%; pHsalt−5.0; hydrolytic soil acidity−2.5 mg-eq./100 g; easily hydrolyzed nitrogen-−45 mg/kg of soil; phosphorous (P2O5) content−170.0 mg/kg of soil, potassium (K2O)−62.0 mg/kg of soil], starting from the mother rock and considering the sizes of genetic horizons (see Figure 1). The soil was tested for the depth of the arable layer of 0–23 cm, using the standard soil analysis methods (Fomin and Fomin, 2000). The planting area of each lysimeter is 3.8 m2, the soil depth is 155 cm, the soil weight is 10.5 tons/lysimeter.
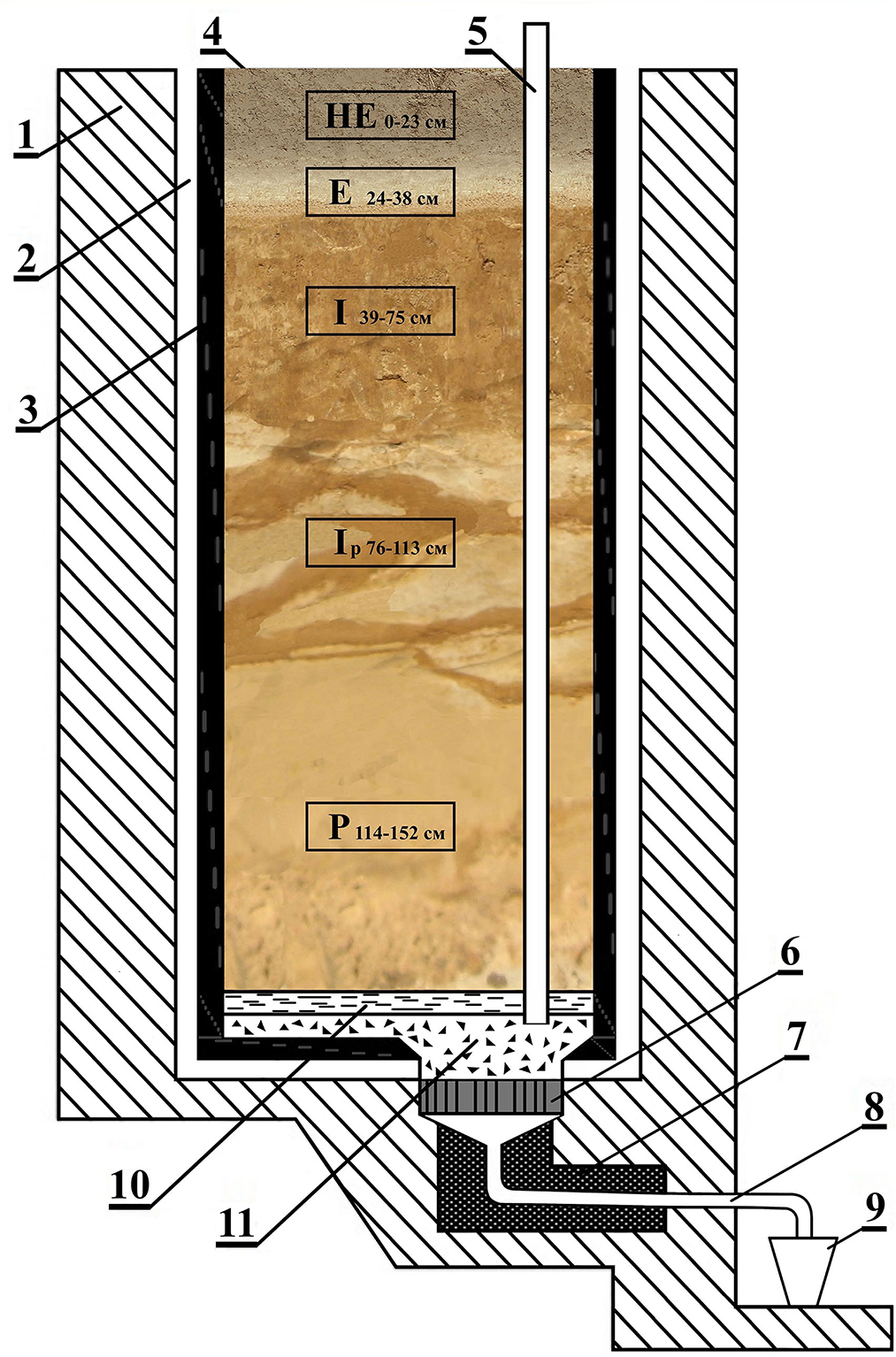
Figure 1. The scheme of vertical cut of lysimeter packed with soil. 1—outer wall (concrete); 2—hydro isolation (3 cm); 3—inner isolation wall (4 cm); 4—soil (considering the depth of genetic horizons); 5—drainage vertical pipe; 6—drainage disk; 7—mineral wool; 8—drainage pipe; 9—container for leachate collection; 10—river sand; 11—crushed glass.
Spring barley
Spring barley of Nosivskiy variety was grown during April–August, 2016, under the different mineral fertilizers backgrounds (see the experimental design scheme below) and seed inoculation with Azospirillum brasilense 410 (Volkogon et al., 1991). Bacterial suspension at the rate of 0.5 million cells per seed was used for pre-sowing seed inoculation, taking into the account the planting rates (4.5 million seeds/ha) and the area of the lysimeter (3.8 m2). Seed inoculation was carried out manually in the laboratory by adding water (2.0% of the seed weight) to the bacterial suspensions for uniform distribution of bacterial cells on the seeds. Bacterized seeds were planted immediately after inoculation. Mineral fertilizers were applied to the soil prior to spring barley planting.
Spring barley experimental design scheme:
I. Without inoculation
1. Control, without fertilizers.
2. N60P60K60 (applied pre-planting).
3. N120P120K120 (applied pre-planting).
II. Inoculation with A. brasilense 410:
4. Control, without fertilizers.
5. N60P60K60 (applied pre-planting).
6. N120P120K120 (applied pre-planting).
Maize
To equalize soil mineral background in 2017 all lysimeters were planted with the oats grown without fertilizers. After harvesting oats (mid-July 2017) narrow-leaved lupine was planted in corresponding plots as an intermediate green manure crop. By the end of November 2017, the aboveground mass of lupine (equivalent to 13 t/ha) was embedded manually into the soil. Cattle manure (equivalent to 40 t/ha) was embedded into the soil in the corresponding test plots also by the end of November 2017.
Maize plants of Petrovskiy 169 CB hybrid were grown in 2018 under different mineral fertilizers backgrounds (see the experimental design scheme below) and seeds inoculation with Paenibacillus polymyxa KB (Tokmakova, 1997). The same rate (0.5 million cells per seed) of bacterial suspension was used for pre-sowing maize seed inoculation, taking into the account the planting rates (70 thousand seeds/ha) and the area of the lysimeter (3.8 m2). Seed inoculation was carried out manually in the laboratory by adding water (2.0% of the seed weight) to the bacterial suspensions for uniform distribution of bacterial cells on the seeds. Bacterized seeds were planted immediately after inoculation. Mineral fertilizers in corresponding test plots were applied prior to maize planting.
Maize experimental design scheme:
I. Without inoculation
1. Control, without fertilizers.
2. Green manure (equivalent to 13 t/ha).
3. N90P90K90 (applied pre-planting).
4. Green manure + N90P90K90 (applied pre-planting).
5. Cattle manure (equivalent to 40 t/ha).
II. With inoculation P. polymyxa KB:
6. Control, without fertilizers.
7. Green manure (equivalent to 13 t/ha).
8. N90P90K90 (applied pre-planting).
9. Green manure + N90P90K90 (applied pre-planting).
10. Cattle manure (equivalent to 40 t/ha).
Winter rye
In 2019 all lysimeters plots were planted with oats to equalize soil mineral background (grown without fertilizers). Winter rye of Borot'ba variety was planted in September 2019 under the different mineral fertilizers backgrounds (see the experimental design scheme below) and seeds inoculation with A. brasilense 18-2 (Nadkernychna, 2003). The inoculation rate of 0.5 million cells per seed was used for pre-sowing winter rye seed inoculation taking into the account the planting rates (4 million seeds/ha) and the area of the lysimeter (3.8 m2). Seed inoculation was carried out manually in the laboratory by adding water (2.0% of the seed weight) to the bacterial suspensions for uniform distribution of bacterial cells on the seeds. Bacterized seeds were planted immediately after inoculation. The split application of mineral fertilizers was used.
Winter rye experimental design scheme:
I. Without inoculation
1. Control, without fertilizers.
2. N30P30K30 (applied at planting).
3. N60P60K60 (split application: N30P30K30–at planting, N30P30K30–at dormancy break).
4. N90P90K90 (split application: N30P30K30–at planting, N30P30K30—at dormancy break, N30P30K30–at elongation stage).
II. With inoculation A. brasilense 18-2:
5. Control, without fertilizers.
6. N30P30K30 (applied at planting).
7. N60P60K60 (split application: N30P30K30–at planting, N30P30K30–at dormancy break).
8. N90P90K90 (split application: N30P30K30–at planting, N30P30K30–at dormancy break, N30P30K30–at elongation stage).
All experimental variants across the years of study were repeated four times.
Bacterial culture
Azospirillum brasilense 410 was previously isolated by Volkogon et al. (1991) from the rhizoplane of meadow ryegrass as an active associative nitrogen fixer and producer of phytohormones. The strain actively progresses in the root zones of meadow ryegrass, spring barley, potatoes, and oats and enhances the productivity of these crops (Volkogon, 1999). Azospirillum brasilense 18-2 was isolated by Nadkernychna (2003) from buckwheat rhizoplane as an active associative nitrogen fixing bacteria, forming close associations with the buckwheat and winter rye plants. Azospirillum brasilense 410 and A. brasilense 18-2 are deposited in the Depository of Microorganisms of the Institute of Microbiology and Virology of the National Academy of Sciences of Ukraine under numbers IMV B-7222 and IMV B-7221, respectively. Paenibacillus polymyxa KB was isolated from sod-podzolic soil and is characterized with high ability to colonize the rhizosphere of many types of plants, significantly improving their phosphorus nutrition (Tokmakova, 1997). Paenibacillus polymyxa KB is deposited in the Depository of Microorganisms of the All-Russian Institute of Agricultural Microbiology under the number VNIAM 324D. The efficiency of this strains was tested in laboratory and field experiments confirming crop productivity increase and output quality improvement at pre-sowing inoculation of the corresponding types of agricultural crops (Volkogon et al., 2006, 2020). All three rhizobacteria were used as an active agents for bacterial preparations created and commercialized in Ukraine, namely Microhumin for spring barley (A. brasilense 410), Diazobacterin (A. brasilense 18-2) and Polymyxobacterin (P. polymyxa KB).
Bacteria were grown for 72 h under periodic culture conditions on a microbiological shaker (180 shakes/min). Azospirilla were cultivated on a liquid medium with malate and ammonium sulfate (Gerhardt, 1981), while P. polymyxa KB—on a liquid medium of the following composition (g/L): molasses-−40.0; Maize extract−20.0; K2HPO4−0.38; KH2PO4−0.5; MgSO4−0.5; NaCl−0.5; CaCO3−5.0. The pH was adjusted to 6.8 using NaOH. Titers of bacterial suspensions were determined prior to the application by direct microscopic counting using hemocytometer followed by aqueous dilutions cultures of the suspensions on the appropriate media: A. brasilense was cultivated on a semi-liquid medium with malate (Gerhardt, 1981), using the acetylene test (Villemin et al., 1974); P. polymyxa was cultivated on meat-peptone agar (Gerhardt, 1981). Cell titers for A. brasilense 410 were 3.5 × 109, for A. brasilense 18-2−3.0 × 109, for P. polymyxa KB−5.0 × 109.
Analysis of drainage waters
Analysis of drainage water volumes was carried out during the crops' growing season. Analysis of drainage water for nitrates, ammonium nitrogen, water-soluble P2O5, K2O, CaO, MgO, and water-soluble organic matter was performed by standard methods (Fomin and Fomin, 2000). Nutrient losses were calculated on a “per hectare” basis (considering that the area of one lysimeter is 3.8 m2).
Crop harvest
Crops were harvested directly from each test plot individually and weighted. Yield data is calculated on a “per hectare” basis.
Statistical analysis
The analysis of variance (ANOVA) was used to analyze the differences among means of biogenic nutrients and water-soluble organic matter losses. The probability values <0.05 (p-values ≤ 0.05) were used to distinguish statistically significant differences (Statistica 6.0, StatSoft Inc., USA).
Results
Significant losses of mineral nitrogen under the spring barley cultivation on sod-podzolic soil in washing water regime conditions were observed in all variants, including control. The application of mineral fertilizers caused additional leaching of nitrate nitrogen. However, the use of A. brasilense 410 for the pre-sowing treatment of barley seeds reduced nutrients leaching beyond the root zone of the soil by 21%−33%, subject to the fertilization norms. Seed inoculation led to the reduction of potassium washing down the soil profile by 11%−41%. No significant difference in phosphorous (P2O5) loss reduction was observed, except for the variants with the highest dose of mineral fertilizers. The substantial drop in leaching of calcium (by 24%−34%) and magnesium (by 25%−50%) compounds was observed under the use of A. brasilense 410 on various fertilizers backgrounds. Losses of water-soluble organic matter also decreased by 25%−43% (Table 1).
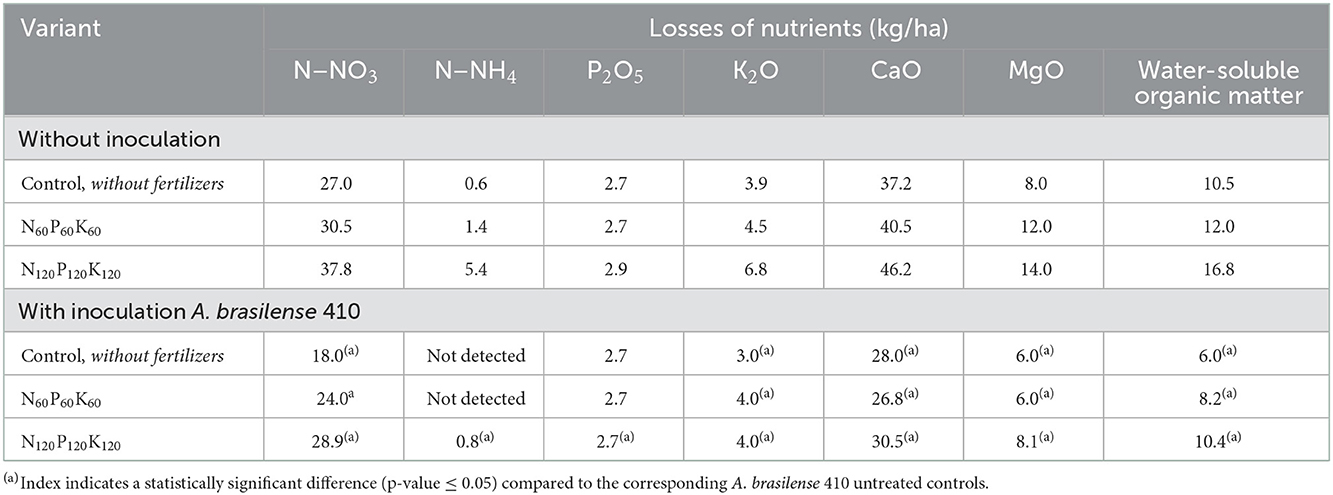
Table 1. The effect of fertilizers and pre-sowing spring barley seeds inoculation with A. brasilense 410 on losses of nutrients and water-soluble organic matter with drainage waters (April–August 2016).
The yield of spring barley increased with the introduction of mineral fertilizers. Pre-sowing inoculation promoted a significant increase in the productivity of the crop grown without fertilizers and with the mineral fertilizers applied at N60P60K60 rate (Table 2). No significant response in crop yield was obtained from inoculation under the increase of fertilizers norms to N120P120K120.
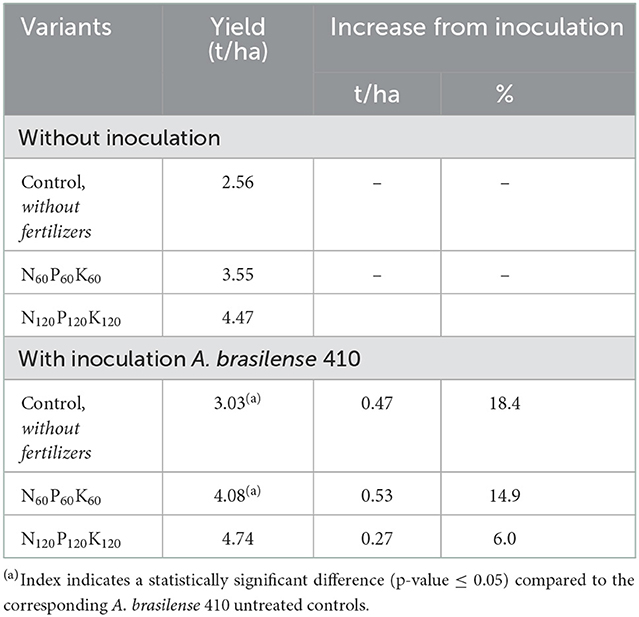
Table 2. The effect of fertilizers and pre-sowing seeds inoculation with A. brasilense 410 on spring barley yield (prorated to “per hectare” basis).
Inoculation of maize seeds with the phosphate-mobilizing bacterium P. polymyxa KB had a similar effect: depending on the agricultural background, the reduction of nitrate loss compared to controls was 8%−25%, CaO-−12%−39%, MgO-−8%−18% (Table 3).
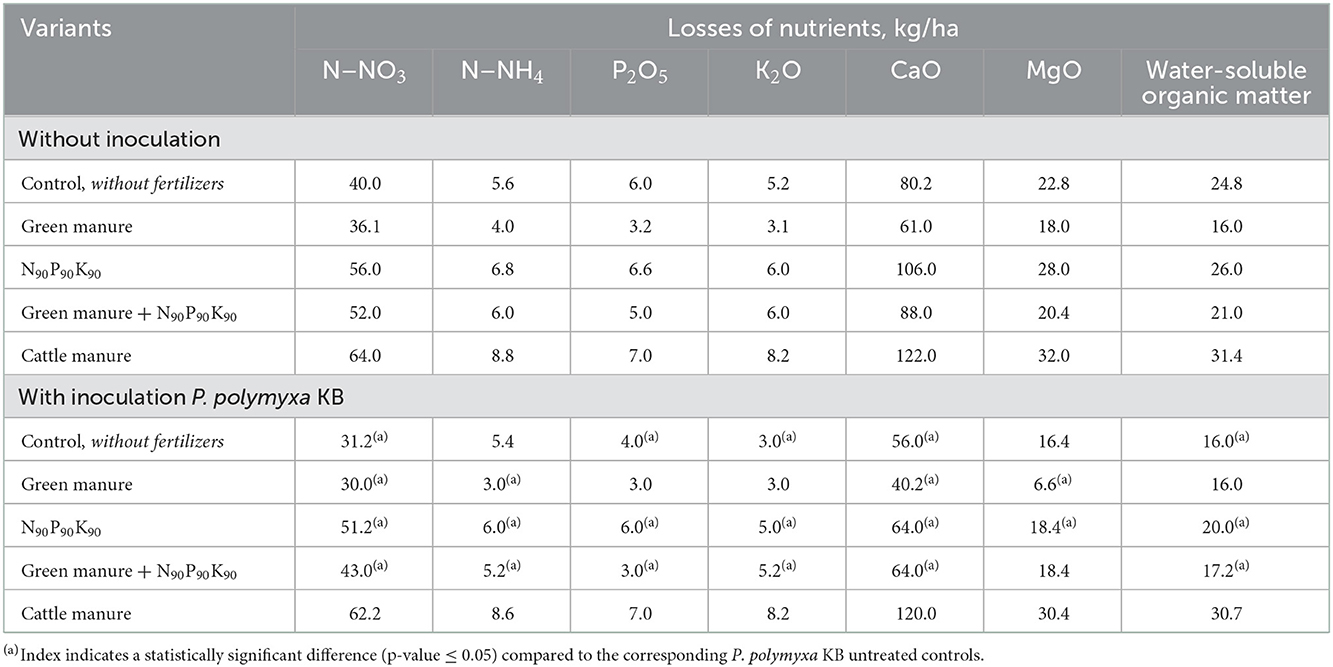
Table 3. The effect of fertilizers and pre-sowing maize seeds inoculation with P. polymyxa KB on losses of nutrients and water-soluble organic matter with drainage waters (May–September 2018).
Noteworthy is the lack of effect of maize seeds inoculation in the test plots with cattle manure and the significant efficiency of inoculation in the variants with green manure (lupine).
The highest losses of water-soluble organic matter were observed in the test plots with mineral fertilizers and cattle manure (Table 3). The use of the intermediate green manure crop (narrow-leaved lupine) reduced the losses of water-soluble organic matter to values lower than the ones observed in the control (variant without fertilizers). Moreover, the combination of green manure with mineral fertilizers in the N90P90K90 norm also significantly limited the leaching of water-soluble organic compounds. The lowest losses were observed in the plots with seeds inoculation with P. polymyxa KB and the use of mineral fertilizers. The combination of factors “green manure + N90P90K90 + P. polymyxa KB” was shown to be the most efficient, ensuring the reduction of water-soluble organic matter leaching lower than the absolute control values.
Maize grown under the use of green manure and mineral fertilizers background had the highest grain productivity both in variants with and without seeds treatment with P. polymyxa KB, while seeds inoculation with P. polymyxa KB had no significant effect on maize yield under the crop cultivation on cattle manure background (Table 4).
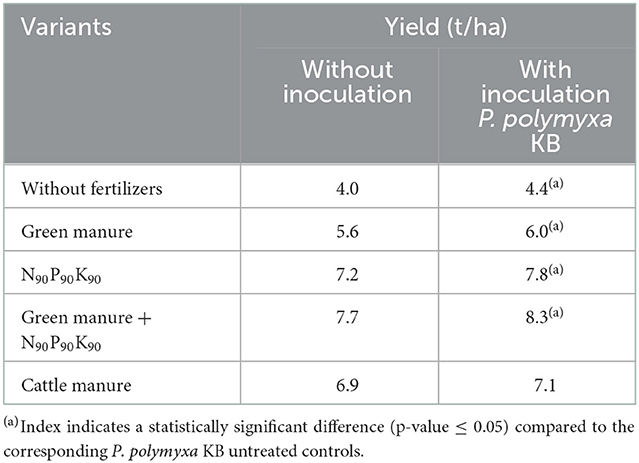
Table 4. The effect of fertilizers and pre-sowing seeds inoculation with P. polymyxa KB on maize yield (prorated to “per hectare” basis).
With the increasing doses of mineral fertilizers the productivity of winter rye had also increased up to the application of the N60P60K60 rate. However, further increase of fertilizers norms (N90P90K90) had shown less return in yield per applied fertilizer norms (Table 5).
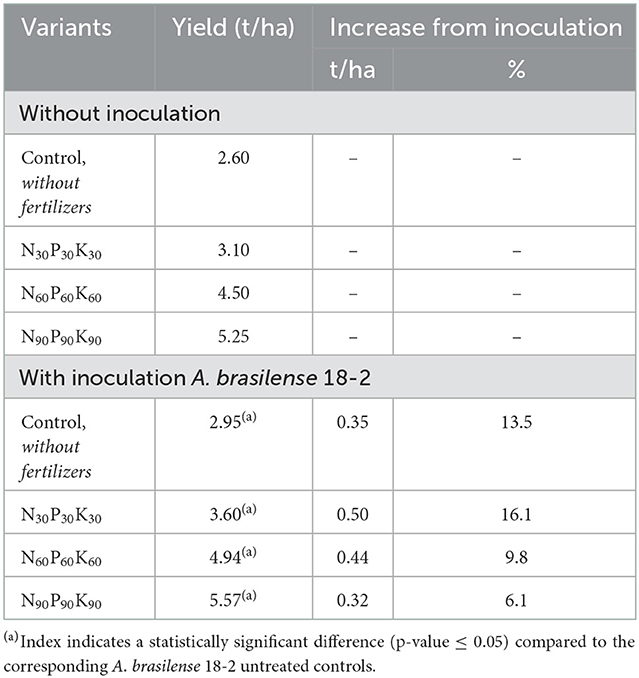
Table 5. The effect of fertilizers and pre-sowing seeds inoculation with A. brasilense 18-2 on winter rye yield (prorated to “per hectare” basis).
Pre-sowing treatment of winter rye seeds with A. brasilense 18-2 increased winter rye grain productivity in all test plots, including control without fertilizers (13.5% yield increase). Although the seeds treatment with A. brasilense 18-2 resulted in a reliable yield increase the increment was the lowest across the experiment (Table 5).
Improvement of crop yield in variants with seeds inoculation with A. brasilense 18-2 is supported by the analysis of the lysimeter drainage waters showing the reduction of nutrients leaching from the soil profile (Table 6). Seeds treatment reduced the losses of all studied compounds, as well as water-soluble organic matter.
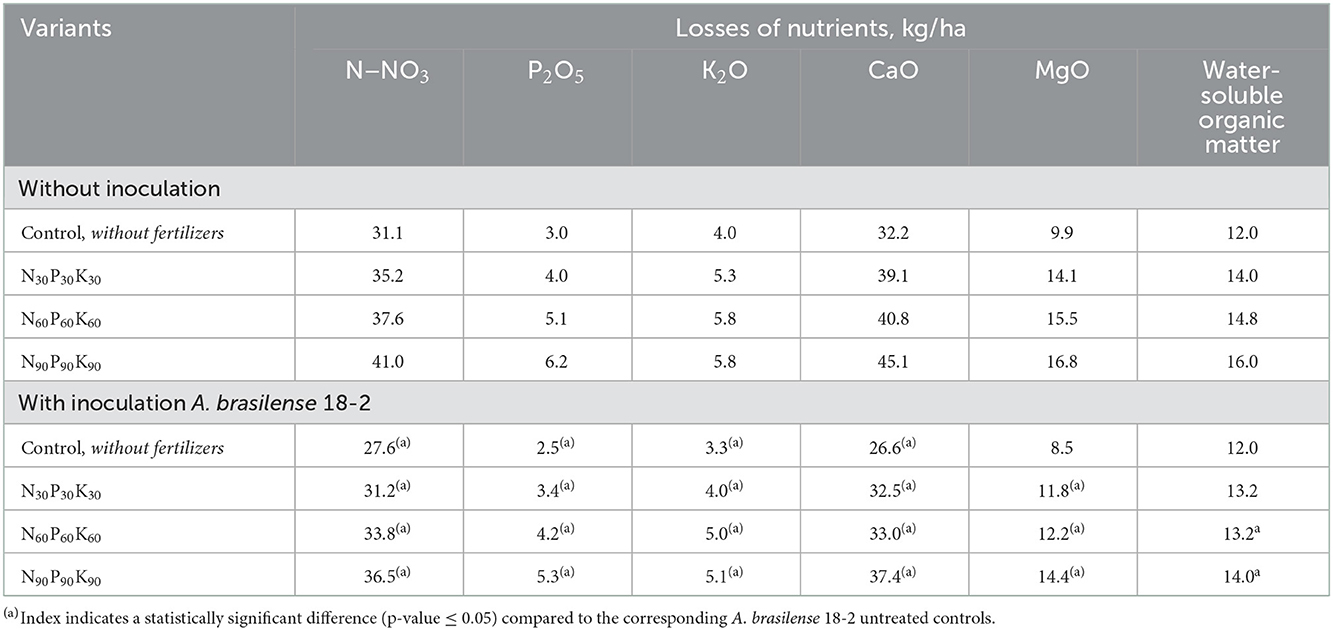
Table 6. The effect of fertilizers and pre-sowing winter rye seeds inoculation with A. brasilense 18-2 on losses of nutrients and water-soluble organic matter with drainage waters (March–July 2020).
Discussion
Modern approaches to agriculture foresee the extensive use of fertilizers to provide plants with the necessary nutrients. However, the excessive use of fertilizers can cause significant environmental impacts, as certain nutrients can be leached from the soil with downstream water runoff caused by rain or irrigation, resulting in eutrophication and water pollution (Adesemoye and Kloepper, 2009; Yu et al., 2019). These threats designate the need to develop measures for effective management of crop nutrition systems, taking into account environmental protection. A promising approach aimed at increasing the efficiency of fertilizer use and environmental protection is the use of PGPR for the inoculation of agricultural crops.
All studied crops grown on a mineral fertilization background following pre-sowing seeds inoculation with PGPR had a similar pattern effect preventing nutrients from leaching down the soil profile. Statistically significant reduction of nutrient losses were observed in most of the studied plots. Variants, shown no reliable changes, still had a clear tendency to decrease nutrients leaching with the drainage waters compared to the corresponding controls. These findings confirm the significant influence of PGPR on the degree of nutrients assimilation by plants, including those from fertilizers, described previously in the literature (Lin et al., 1983; Okon et al., 1998; Zaidi and Khan, 2005; Abbasi et al., 2011; Sharma et al., 2011; Pii et al., 2015; Backer et al., 2018). Higher assimilation levels are accompanied by increased removal of biogenic elements with the crop, resulting in a reduction of nutrients losses from the soil. And although the mechanisms of influence of the studied PGPR on the production process of agricultural crops may be different, the consequences of the functioning of artificially created bacterial-plant associations were similar.
Part of the nutrients is retained in the crops' root system, which in turn has increased as a result of seed inoculation. It is known that physiologically active substances produced by inoculants contribute to the optimization of rhizogenesis (Jacoud et al., 1998; Dobbelaere et al., 2001; El Zemrany et al., 2006). The bacteria used in our research, in addition to the ability to fix nitrogen and mobilize phosphate, are also active producers of auxins, cytokinins and gibberellins, which promote changes in the architecture of the root system and its scopes. Accordingly, the enlarged root system can temporarily retain significant amounts of nutrients in organic form. After the root residue mineralization, these nutrients can benefit the following crops in the crop rotation or be used at de novo synthesis of soil organic matter.
The reduction of calcium and magnesium compounds' leaching down the root zone of bacterized plants is another essential characteristic of the mechanism of the effect of biological preparations on both the productivity of agricultural crops and soil fertility. With calcium playing an important role in the processes of soil formation (Mazur, 2008) and metabolic regulation in both eukaryotes and prokaryotes (Smith, 1995), the retention of calcium compounds in soil from leaching is a positive indicator. Magnesium takes an active part in the chloroplasts' biogenesis and is part of certain enzymes in the plant organism (Kwon et al., 2019; Alejandro et al., 2020). Magnesium deficiency in plants results in leaf chlorosis, while severe cases result in stunted growth (Bang et al., 2021). A decrease in magnesium compounds' losses under the use of PGPR for seed inoculation is an unambiguously confident consequence. However, the observed significant reduction of calcium and magnesium compounds losses as a result of seed inoculation cannot be explained only by the increase in the level of their assimilation by bacterized plants and requires additional research.
The decrease in the leaching of water-soluble organic matter in test plots with pre-sowing seed inoculation was somewhat unexpected. Subject to the fertilization background, barley seed inoculation with A. brasilense 410 contributes to the reduction of the water-soluble organic matter losses by 25%−43% compared to the corresponding variants without inoculation. Maize growing in control variants (without fertilizers) and on a mineral background, N90P90O90 followed by sed inoculation with P. polymyxa KB ensured a drop of water-soluble organic matter leaching by 35% and 30%, respectively. Slightly lower values were observed for the winter rye crop, with seeds inoculated with A. brasilense 18-2.
It can be assumed that part of water-soluble organic matter is mineralized and assimilated by plants due to the bacterial hydrolytic enzyme activity. Mineralization processes can be enhanced in the rhizosphere of plants due to the phytostimulatory effect of PGPR and an increased amount of readily available carbon compounds, which contributes to the growth of the number and activity of heterotrophic microorganisms (Oger et al., 2004; Blagodatskaya et al., 2010). At the same time, the production of hydrolytic enzymes can be conditioned not only by the development of the bacteria introduced into the rhizosphere but also by the indirect effect of inoculation on the development of the resident microbiota in the rhizosphere (Hu et al., 2021). Recent studies using molecular biological methods (Ramakrishnan et al., 2017; Jiménez et al., 2020) confirm the effect of PGPR inoculation on the development of resident microbiota in the rhizosphere of plants.
The ability of plants to absorb both mineral and organic substances can also be affected by the reduction in the intensity of water-soluble organic matter leaching. Information about the assimilation of organic compounds with low molecular weight by plants is not new (Hutchinson and Miller, 1912), however, it was questioned until recently when the importance of this process for crop yield formation has been shown (Farzadfar et al., 2021). The size of the root system is considered the most promising mechanism for the absorption of organic substances by plants (Farzadfar et al., 2021), so the ability of PGPR to influence the rhizogenesis of plants can stimulate the assimilation of not only mineral compounds, but also organic compounds.
Altogether the prevention of the nutrient and water-soluble organic matter losses in the rhizosphere of crops inoculated with PGPR and the potential involvement of increased plant biomass in the processes of “synthesis-mineralization” indicate the importance of the PGPR on soil fertility.
The results obtained relate in the first place to the conditions of the washing water regime, typical for the soil-climatic zone of Polissia of Ukraine. However, it may also be important to take into account these PGPR effects on the processes of nutrient migration in the soil under irrigation.
Fertilization had a significant influence on the PGPR effect on crop yield. Relatively low doses of mineral fertilizers increased the efficiency of seed inoculation. In our opinion, low rates of fertilizers can lie within the physiological optimum both for the development of plants and bacteria (Volkogon, 2013). It is under such conditions that biological and mineral resources can be synergistic in providing plants with nutrients and biological functions congenial to PGPR.
High doses of mineral fertilizers applied to spring barley and winter rye crops had significantly reduced the effect of inoculation on crop productivity, indicating their redundancy within the course of biological processes in the “soil-plant-bacteria” system. The obtained results confirm the conclusions of Ozturk et al. (2003), who conducted research with wheat and barley. A similar situation is also described for other artificially created plant-bacterial associations. Thus, Shaharooma et al. (2008) reported an increased nitrogen use efficiency by wheat crops in response to Pseudomonas fluorescens inoculation, but to the different extents subject to mineral fertilizer rates, which provided 115, 52, 26, and 27% increases in yield compared to the uninoculated control with nitrogen, phosphorus, and potassium applied at 25, 50, 75, and 100% of the recommended rates, respectively.
The results of our research support the conclusion on seed inoculation as an important strategy for sustainable soil management and lessening environmental problems due to the reduction of the chemical fertilizers load (Santa et al., 2008; Adesemoye et al., 2009; Hungria et al., 2010). However, in contrast to publications promoting PGPR as the direct replacement for mineral fertilizers, in our assessments of the prospects of inoculation, we share the views focused on the effective combination of mineral and biological factors for agricultural crops fertilization.
Noteworthy is the lack of effect of PGPR P. polymyxa KB used for pre-sowing seed inoculation of maize against a manure background. It can be explained by non-specific soil bacterization with a vast amount of microorganisms introduced into the soil with cattle manure, which largely neutralizes the effect of pre-sowing seed inoculation due to the creation of a competitive environment. A similar effect was also observed in our studies of the PGPR effects (Volkogon et al., 2021). Recent research conducted in our laboratory on the peculiarities of the development of genetically marked PGPR strains in the root zone of agricultural crops grown on different mineral fertilization backgrounds and application of cattle manure confirms these findings. After manure application, the introduction of selected agronomically valuable bacteria into the root zones of plants was ineffective (Sydorenko et al., 2020).
At the same time, significant effectiveness of seed inoculation is observed when growing crops on green manure background. Unlike cattle manure, the sideral mass is less contaminated with microorganisms, which do not interfere with the formation of effective interaction of introduced bacteria and plants. Besides the mineralization of green manure biomass can serve as an additional source of nutrients for P. polymyxa KB.
Lupine, as green manure, promotes nutrient loss reduction when used in combination with mineral fertilizers. Under these conditions, P. polymyxa KB shows significant effectiveness when applied as a seed treatment. Considering the high grain productivity achieved in test plots for this combination and the preservation of soil resources, the combination of seed inoculation with P. polymyxa KB, mineral fertilizers and green manure is considered to be the most promising for maize cultivation on sod-podzolic soils.
Conclusions
PGPR promote the reduction of biogenic elements and water-soluble organic matter losses with the drainage water flow down the soil profile upon the cultivation of spring barley, maize, and winter rye on mineral and organic fertilization backgrounds. However, the application of cattle manure decreases the observed effect. The observed peculiarities are particularly useful for crop cultivation in zones with a washing hydrological regime, as well as under irrigation.
As shown by the results seed inoculation with PGPR stimulates nutrient assimilation, thus explaining the mechanism of the positive effect of pre-sowing seed bacterization on crop productivity and soil fertility.
Data availability statement
The raw data supporting the conclusions of this article will be made available by the authors, without undue reservation.
Author contributions
VV substantiated the research idea, carried out the microbiological part of the work, analyzed the experimental data, and worked on the manuscript. LP worked with the lysimeters. MV assisted with data interpretation and manuscript preparation. All authors read and approved the final manuscript.
Acknowledgments
The authors are thankful to the employees of the Institute of Agricultural Microbiology and Agro-Industrial Production of the National Academy of Agrarian Sciences of Ukraine Nadkernychna O.V. and Tokmakova L.M. for provided bacterial strains A. brasilense 18-2 and P. polymyxa KB. The authors are sincerely grateful to the reviewers and the editor for their advice and corrections, which made it possible to significantly improve the manuscript.
Conflict of interest
The authors declare that the research was conducted in the absence of any commercial or financial relationships that could be construed as a potential conflict of interest.
Publisher's note
All claims expressed in this article are solely those of the authors and do not necessarily represent those of their affiliated organizations, or those of the publisher, the editors and the reviewers. Any product that may be evaluated in this article, or claim that may be made by its manufacturer, is not guaranteed or endorsed by the publisher.
References
Abbasi, M., Sharif, S., Kazmi, M., Sultan, T., and Aslam, M. (2011). Isolation of plant growth promoting rhizobacteria from wheat rhizosphere and their effect on improving growth, yield and nutrient uptake of plants. Plant Biosyst. 145, 159–168. doi: 10.1080/11263504.2010.542318
Adedayo, A. A., Babalola, O. O., Prigent-Combaret, C., Cruz, C., Stefan, M., Kutu, F., et al. (2022). The application of plant growth promoting rhizobacteria in Solanum lycopersicum production in the agricultural system: a review. Peer J. 10, e13405. doi: 10.7717/peerj.13405
Adesemoye, A. O., and Kloepper, J. W. (2009). Plant-microbes interactions in enhanced fertilizer-use efficiency. Microbiol. Biotechnol. 85, 1–12. doi: 10.1007/s00253-009-2196-0
Adesemoye, A. O., Torbert, H. A., and Kloepper, J. W. (2009). Plant growth-promoting rhizobacteria allow reduced application rates of chemical fertilizers. Microb. Ecol. 58, 921–929. doi: 10.1007/s00248-009-9531-y
Alejandro, S., Holler, S., Meier, D., and Peiter, E. (2020). Manganese in plants: from acquisition to subcellular allocation. Front. Plant Sci. 11, 300. doi: 10.3389/fpls.2020.00300
Babalola, O. O. (2010). Beneficial bacteria of agricultural importance. Biotechnol. Lett. 32, 1559–1570. doi: 10.1007/s10529-010-0347-0
Backer, R., Rokem, J. S., Ilangumaran, G., Lamont, J., Praslickova, D., Ricci, E., et al. (2018). Plant growth-promoting rhizobacteria: context, mechanisms of action, and roadmap to commercialization of biostimulants for sustainable agriculture. Front. Plant Sci. 9, 1473. doi: 10.3389/fpls.2018.01473
Bang, T. C., Husted, S., Laursen, K. H., Persson, D. P., and Schjoerring, J. K. (2021). The molecular-physiological functions of mineral macronutrients and their consequences for deficiency symptoms in plants. New Phytol. 229, 2446–2469. doi: 10.1111/nph.17074
Bashan, Y., and Holguin, G. (1998). Proposal for the division of plant growthpromoting rhizobacteria into two classifications: biocontrol-PGPB (plant growth-promoting bacteria) and PGPB. Soil Biol. Biochem. 30, 1225–1228. doi: 10.1016/S0038-0717(97)00187-9
Bashan, Y., and Holguin, G. (2002). Plant growth-promoting bacteria: a potential tool for arid mangrove reforestation. Trees 16, 159–166. doi: 10.1007/s00468-001-0152-4
Berg, G., Rybakova, D., Fischer, D., Cernava, T., Vergès, M-C. C., Charles, T., et al. (2020). Microbiome definition re-visited: old concepts and new challenges. Microbiome 8, 1–22. doi: 10.1186/s40168-020-00875-0
Blagodatskaya, E., Littschwager, J., Laurer, M., and Kuzyakov, Y. (2010). Growth rates of rhizosphere microorganisms depend on competitive abilities of plants and N supply. Plant Biosyst. 144, 408–413. doi: 10.1080/11263501003718596
Bulgarelli, D., Schlaeppi, K., Spaepen, S., Van Themaat, E. V. L., and Schulze-Lefert, P. (2013). Structure and functions of the bacterial microbiota of plants. Ann. Rev. Plant Biol. 64, 807–838. doi: 10.1146/annurev-arplant-050312-120106
Curl, E. A., and Truelove, B. (1986). The Rhizosphere. (Advanced Series in Agricultural Sciences, Vol. 15). Berlin-Heidelberg-New York-Tokyo: Springer-Verlag, 288. doi: 10.1007/978-3-642-70722-3
Di Benedetto, N. A., Corbo, M. R., Campaniello, D., Pia Cataldi, M., Bevilacqua, A., Sinigaglia, Z., et al. (2017). The role of plant growth promoting bacteria in improving nitrogen use efficiency for sustainable crop production: a focus on wheat. AIMS Microbiol. 3, 413–434. doi: 10.3934/microbiol.2017.3.413
Di Salvo, L. P., Cellucci, G. C., Carlino, M. E., and Garcia de Salamone, I. E. (2018). Plant grows-promoting rhizobacteria inoculation and nitrogen fertilization increase maize (Zea mays L.) grain yield and modified rhizosphere microbial communities. Agric. Ecosyst. Environ. Appl. Soil Ecol. 126, 113–120. doi: 10.1016/j.apsoil.2018.02.010
Dobbelaere, S., Croonenborghs, A., Thys, A., Ptacek, D., Vanderleyden, J., Dutto, P., et al. (2001). Responses of agronomically important crops to inoculation with Azospirillum. Aust. J. Plant Physiol. 28, 871–879. doi: 10.1071/PP01074
El Zemrany, H., Cortet, J., Lutz, M. P., Chabert, A., Baudoin, E., Haurat, J., et al. (2006). Field survival of the phytostimulator Azospirillum lipoferum CRT1 and functional impact on maize crop, biodegradation of crop residues, and soil faunal indicators in a context of decreasing nitrogen fertilisation. Soil Biol. Biochem. 38, 1712–1726. doi: 10.1016/j.soilbio.2005.11.025
Farzadfar, S., Knight, J. D., and Congreves, K. A. (2021). Soil organic nitrogen: an overlooked but potentially significant contribution to crop nutrition. Plant Soil. 462, 7–23. doi: 10.1007/s11104-021-04860-w
Fasusi, O. A., Cruz, C., and Babalola, O. O. (2021). Agricultural sustainability: microbial biofertilizers in rhizosphere management. Agriculture 11, 163. doi: 10.3390/agriculture11020163
Figueiredo, M. V. B., Seldin, L., de Araujo, F. F., and Mariano, R. (2022). “Plant growth promoting rhizobacteria: fundamentals and applications,” in Plant Growth and Health Promoting Bacteria, ed D. K. Maheshwari (Berlin Heidelberg: Springer-Verlag), 21–36. doi: 10.1007/978-3-642-13612-2_2
Fomin, G. S., and Fomin, A. G. (2000). The Soil. Quality Control and Environmental Safety in Accordance with International Standards. Moskow: Protector [russian].
Gerhardt, P. (1981). Manual of Methods for General Bacteriology. Washington, DC: American Society for Microbiology.
Hamilton, E. W., and Frank, D. A. (2001). Can plants stimulate soil microbes and their own nutrient supply? Evidence from a grazing tolerant grass. Ecology 82, 2397–2402. doi: 10.1890/0012-9658(2001)082(2397:CPSSMA)2.0.CO;2
Herman, D. J., Johnson, K. K., Jaeger, C. H., Schwartz, E., and Firestone, M. K. (2006). Root influence on nitrogen mineralization and nitrification in Avena barbata rhizosphere soil. Soil Biol. Biochem. 70, 1504–1511. doi: 10.2136/sssaj2005.0113
Hu, J., Yang, T., Friman, V. P., Kowalchuk, G. A., Hautier, Y., Li, M., et al. (2021). Introduction of probiotic bacterial consortia promotes plant growth via impacts on the resident rhizosphere microbiome. Proc. R. Soc. B. 288, 20211396. doi: 10.1098/rspb.2021.1396
Hungria, M., Rubens, J., Campo, R. J., Souza, E. M., and Pedrosa, F. O. (2010). Inoculation with selected strains of Azospirillum brasilense and A. lipoferum improves yields of maize and wheat in Brazil. Plant Soil. 331, 413–425. doi: 10.1007/s11104-009-0262-0
Hutchinson, H. B., and Miller, N. H. J. (1912). The direct assimilation of inorganic and organic forms of nitrogen by higher plants. J. Agric. Sci. 4, 282–302. doi: 10.1017/S0021859600001386
Ilyas, N., Mazhar, R., Yasmin, H., Khan, W., Sumera Iqbal, S., Hesham El Enshasy, H. E., et al. (2020). Rhizobacteria isolated from saline soil induce systemic tolerance in wheat (Triticum aestivum L.) against salinity stress. Agronomy 10, 989. doi: 10.3390/agronomy10070989
Jacoud, C., Faure, D., Wadoux, P., and Bally, R. (1998). Development of a strain-specific probe to follow inoculated Azospirillum lipoferum CRT1 under field conditions and enhancement of maize root development by inoculation. FEMS Microb. Ecol. 27, 43–51. doi: 10.1111/j.1574-6941.1998.tb00524.x
Jeyanthi, V., and Kanimozhi, S. (2018). Plant growth promoting rhizobacteria (PGPR) - prospective and mechanisms: a review. J. Pure and App. Microbiol. 12, 733–749. doi: 10.22207/JPAM.12.2.34
Jiménez, J. A., Novinscak, A., and Filion, M. (2020). Inoculation With the plant-growth-promoting rhizobacterium Pseudomonas fluorescens LBUM677 impacts the rhizosphere microbiome of three oilseed crops. Front. Microbiol. 11, 569366. doi: 10.3389/fmicb.2020.569366
Khanna, K., Jamwal, V. L., Sharma, A., Gandhi, S. G., Ohri, P., Bhardwaj, R., et al. (2019). Supplementation with plant growth promoting rhizobacteria (PGPR) alleviates cadmium toxicity in Solanum lycopersicum by modulating the expression of secondary metabolites. Chemosphere 230, 628–639. doi: 10.1016/j.chemosphere.2019.05.072
Kloepper, J. W., and Schroth, M. N. (1978). “Plant growth promoting rhizobacteria on radishes,” in Proceedings of the 4th International Conference on Plant Pathogenic Bacteria, ed Station de Pathologic Vegetal et Phytobacteriologic, Vol. 2. (Angers, France), 879–882.
Kwon, M. C., Kim, Y. X., Lee, S., Jung, E. S., Singh, D., Sung, J., et al. (2019). Comparative metabolomics unravel the effect of magnesium oversupply on tomato fruit quality and associated plant metabolism. Metabolites 9, 231. doi: 10.3390/metabo9100231
Landi, L., Valori, F., Ascher, J., Renella, G., Falchini, L., Nannipieri, P., et al. (2006). Root exudate effects on the bacterial communities, CO2 evolution, nitrogen transformations and ATP content of rhizosphere and bulk soils. Soil Biol. Biochem. 38, 509–516. doi: 10.1016/j.soilbio.2005.05.021
Lin, W., Okon, Y., and Hardy, R. W. R. F. (1983). Enhauced mineral uptake by Zea mays and Sorgum bicolor roots inoculated with Azospirillum brasilense. Appl. Environ. Microbiol. 45, 1775–1779. doi: 10.1128/aem.45.6.1775-1779.1983
Lugtenberg, B., and Kamilova, F. (2009). Plant-growth-promoting rhizobacteria. Annu. Rev. Microbiol. 63, 541–556. doi: 10.1146/annurev.micro.62.081307.162918
Marschner, P. (2012). “Rhizosphere biology,” in Marschner's Mineral Nutrition of Higher Plants, ed P. Marschner, 3rd ed. (Amsterdam: Elsevier), 369–388. doi: 10.1016/B978-0-12-384905-2.00015-7
Martínez-Viveros, O., Jorquera, M. A., Crowley, D. E., Gajardo, G., and Mora, M. L. (2010). Mechanisms and practical considerations involved in plant growth promotion by rhizobacteria. J. Soil Sci. Plant Nutr. 10, 293–319. doi: 10.4067/S0718-95162010000100006
Mazur, G. A. (2008). Reproduction and Regulation of Fertility Of Light Soils. Kiev: Agrarian Science [ukrainian].
Nadkernychna, O. V. (2003). Ability of diazotrophs to form associative systems with winter rye plants. Agroecological J. 3, 17–20.
O'Callaghan, M. (2016). Microbial inoculation of seed for improved crop performance: issues and opportunities. Appl. Microbiol. Biotechn. 100, 5729–5746. doi: 10.1007/s00253-016-7590-9
Oger, P. M., Mansouri, H., Nesme, X., and Dessaux, Y. (2004). Engineering root exudation of Lotus toward the production of two novel carbon compounds leads to the selection of distinct microbial populations in the rhizosphere. Microb. Ecol. 47, 96–103. doi: 10.1007/s00248-003-2012-9
Okon, Y., Bloemberg, G. V., and Lugtenberg, B. J. J. (1998). “Biotechnology of biofertilization and phytostimulation,” in Agricultural Biotechnology, ed A. Altman (New York, NY: Marcel Dekker), 327–349. doi: 10.1201/9781420049275.pt2a
Olanrewaju, O. S., ·Bernard, R., Glick, B. R., and Babalola, O. O. (2017). Mechanisms of action of plant growth promoting bacteria. World J. Microbiol. Biotechnol. 33, 1–16. doi: 10.1007/s11274-017-2364-9
Ozturk, A., Caglar, K., and Sahin, F. (2003). Yield response of wheat and barley to inoculation of plant growth promoting rhizobacteria at various levels of nitrogen fertilization. J. Plant Nutr. Soil Sci. 166, 262–266. doi: 10.1002/jpln.200390038
Pii, Y., Mimmo, T., Tomasi, N., Terzano, R., Cesco, S., and Crecchio, C. (2015). Microbial interactions in the rhizosphere: beneficial influences of plant growth promoting rhizobacteria on nutrient acquisition process. A review. Biol. Fertil. Soils 51, 403–415. doi: 10.1007/s00374-015-0996-1
Ramakrishnan, B., Kaur, S., Prasanna, R., Ranjan, K., Kanchan, A., Hossain, F., Shivay, Y. S., and Nain, L. (2017). Microbial inoculation of seeds characteristically shapes the rhizosphere microbiome in desi and kabuli chickpea types. J. Soils Sediments 17, 2040–2053. doi: 10.1007/s11368-017-1685-5
Santa, O. R. D., Santa, H. S. D., Fernándes, R., Michelena, G., and Junior, P. R. (2008). Influence of Azospirillum sp. inoculation in wheat, barley and oats. Ambiência 4, 197–207.
Santoyo, G., Urtis-Flores, C. A., Loeza-Lara, P. D., Orozco-Mosqueda, M. C., and Glick, B. R. (2021). Rhizosphere colonization determinants by plant growth-promoting rhizobacteria (PGPR). Biology 10, 475. doi: 10.3390/biology10060475
Shaharooma, B., Naveed, M., Arshad, M., and Zahir, Z. A. (2008). Fertilizer-dependent efficiency of Pseudomonads for improving growth, yield, and nutrient use efficiency of wheat (Triticum aestivum L.). Appl. Microbiol. Biotechnol. 79, 147–155. doi: 10.1007/s00253-008-1419-0
Sharma, S. K., Johri, B. N., Ramesh, A., Joshi, O. P., and Prasad, S. S. (2011). Selection of plant growth-promoting Pseudomonas spp. that enhanced productivity of soybean-wheat cropping system in central India. J. Microbiol. Biotechnol. 21, 1127–1142. doi: 10.4014/jmb.1012.12018
Smith, R. J. (1995). Calcium and bacteria. Adv. Microb. Physiol. 37, 83–133. doi: 10.1016/S0065-2911(08)60144-7
Sydorenko, V. P., Volkohon, V. V., Dimova, S. B., Volkohon, K. I., Lutsenko, N. V., Shtanko, N. P., et al. (2020). Efficiency of pre-sowing inoculation in cultivation of agricultural crops under different organic agrarian backgrounds. Sil′s′kohospodars′ka Mikrobiolohiya 32, 18–34. doi: 10.35868/1997-3004.32.18-34
Tokmakova, L. N. (1997). ?acillus polymyxa and Achromobacter album strains - the basis for the creation of bacterial preparations. Microbiol. J. 4, 131–138.
Trivedi, P., Leach, J. E., Tringe, S. G., Sa, T., and Singh, B. K. (2020). Plant-microbiome interactions: from community assembly to plant health. Nat. Rev. Microbiol. 18, 607–621. doi: 10.1038/s41579-020-0412-1
Villemin, G., Balandreau, J., and Dommergues, Y. (1974). Utilization du test de reduction de l'acetylene pour la numeration des bacteries libres fixatuces d'azote. Ann. Microbiol. Ensimol. 24, 87–94. doi: 10.3406/reper.1974.1243
Volkogon, V., Dimova, S., Volkohon, K., and Sydorenko, V. (2020). The efficiency of microbial preparations in different systems of fertilizing crops. Visnyk Ahrarnoyi Nauky (Herald of Agrarian Science) 6, 5–14. doi: 10.31073/agrovisnyk202006-01
Volkogon, V. V. (1999). Nitrogen-fixing microorganisms of the root zone and seeds of cereal grasses. Bul. Inst. Agricult. Microbiol. 4, 6–11.
Volkogon, V. V. (2013). Biological Nitrogen Transformation. The Direction of Processes at Different Levels of Crop Fertilizer. Saarbrucken: Palmarium Academic Publishing [in russian].
Volkogon, V. V., Dimova, S. B., Volkogon, K. I., Sydorenko, V. P., and Volkogon, M. V. (2021). Biological nitrogen fixation and denitrification in rhizosphere of potato plants in response to the fertilization and inoculation. Front. Sustain. Food Syst. 5, 606379. doi: 10.3389/fsufs.2021.606379
Volkogon, V. V., Khalchytskiy, A. E., Minyailo, V. G., Onishchenko, L. I., and Lemeshko, S. V. (1991). Nitrogen-fixing microorganisms of the root zone of ryegrass and smooth brome. Microbiol. J. 6, 3–8.
Volkogon, V. V., Nadkernichnaya, O. V., Kovalevskaya, T. M., ToκMaκova, L. M., Kopilov, E. P., Kothar, S. F., et al. (2006). Microbial Preparations for Farming. Theory and Practice, ed V. V. Volkogon. Kiev: Agrarian Science [ukrainian].
Volpiano, C. G., Lisboa, B. B., São José, J. F. B, Beneduzi, A., Granada, C. E., and Vargas, L. K. (2022). Soil-plant-microbiota interactions to enhance plant growth. Rev. Bras. Cienc. Solo. 46, e0210098. doi: 10.36783/18069657rbcs20210098
Watt, M., Kirkegaard, J. A., and Passioura, J. B. (2006). Rhizosphere biology and crop productivity. Aust. J. Soil Research. 44, 299–317. doi: 10.1071/SR05142
Yasmin, H., Bano, A., Wilson, N. L., Nosheen, A., Naz, R., Hassan, M. N., et al. (2022). Drought-tolerant Pseudomonas sp. showed differential expression of stress-responsive genes and induced drought tolerance in Arabidopsis thaliana. Physiol. Plant 174, e13497. doi: 10.1111/ppl.13497
Yasmin, H., Mazher, J., Azmat, A., Nosheen, A., Naz, R., Hassan, M. N., et al. (2021). Combined application of zinc oxide nanoparticles and biofertilizer to induce salt resistance in safflower by regulating ion homeostasis and antioxidant defense responses. Ecotoxicol. Environ. Saf. 218, 112262. doi: 10.1016/j.ecoenv.2021.112262
Yu, C., Huang, X., Chen, H., Godfray, H. C. J., Wright, J. S., Hall, J. W., et al. (2019). Managing nitrogen to restore water quality in China. Nature 567, 516–520. doi: 10.1038/s41586-019-1001-1
Zaidi, A., and Khan, S. (2005). Interactive effect of rhizotrophic microorganisms on growth, yield, and nutrient uptake of wheat. J. Plant Nutr. 28, 2079–2092. doi: 10.1080/01904160500320897
Keywords: inoculation, PGPR, Azospirillum brasilense, Paenibacillus polymyxa, mineral fertilizers, lysimeter study, vertical migration, water-soluble organic matter
Citation: Volkogon VV, Potapenko LV and Volkogon MV (2023) Vertical migration of nutrients and water-soluble organic matter in the soil profile under pre-sowing seed treatment with plant growth promoting rhizobacteria. Front. Sustain. Food Syst. 6:1054113. doi: 10.3389/fsufs.2022.1054113
Received: 26 September 2022; Accepted: 21 December 2022;
Published: 10 January 2023.
Edited by:
Duraisamy Saravanakumar, The University of the West Indies St. Augustine, Trinidad and TobagoReviewed by:
Marta Susana Dardanelli, National University of Río Cuarto, ArgentinaMuthusamy Karthikeyan, Tamil Nadu Agricultural University, India
Humaira Yasmin, COMSATS University, Islamabad Campus, Pakistan
Copyright © 2023 Volkogon, Potapenko and Volkogon. This is an open-access article distributed under the terms of the Creative Commons Attribution License (CC BY). The use, distribution or reproduction in other forums is permitted, provided the original author(s) and the copyright owner(s) are credited and that the original publication in this journal is cited, in accordance with accepted academic practice. No use, distribution or reproduction is permitted which does not comply with these terms.
*Correspondence: Mykola V. Volkogon, mvolkogon@gmail.com