- 1School of Automation, Hangzhou Dianzi University, Hangzhou, China
- 2Wenzhou Academy of Agricultural Sciences, Wenzhou, China
- 3Fair Friend Institute of Intelligent Manufacturing, Hangzhou Vocational and Technical College, Hangzhou, China
- 4Jinhua Academy of Agricultural Sciences, Jinhua, China
Dendrobium candidum (DC) is an agricultural product for both food and medicine. It has a variety of beneficial effects on the human body with antioxidant, anti-inflammatory, antitumor, enhancing immune function, and other pharmacological activities. Due to less natural distribution, harsh growth conditions, slow growth, low reproduction rate, and excessive logging, wild DC has been seriously damaged and listed as an endangered herbal medicine variety in China. At present, the quality of DC was uneven in the market, so it is very necessary to detect its quality. This article summarized the methods of DC quality detection with traditional and rapid nondestructive, and it also expounded the correlation between DC quality factor and endophytes, which provides a theoretical basis for a variety of rapid detection methods in macromolecules. At last, this article put forward a variety of rapid nondestructive detection methods based on the emission spectrum. In view of the complexity of molecular structure, the quality correlation established by spectral analysis was greatly affected by varieties and environment. We discussed the possibility of DC quality detection based on the molecular dynamic calculation and simulation mechanism. Also, a multimodal fusion method was proposed to detect the quality. The literature review suggests that it is very necessary to understand the structure performance relationship, kinetic properties, and reaction characteristics of chemical substances at the molecular level by means of molecular chemical calculation and simulation, to detect a certain substance more accurately. At the same time, several modes are combined to form complementarity, eliminate ambiguity, and uncertainty and fuse the information of multiple modes to obtain more accurate judgment results.
Introduction
Dendrobium candidum (DC) is a precious agricultural product, both food and medicine, known as “life-saving fairy grass.” It has the effect of “moistening throat, warming stomach and brightening eyesight, tonifying kidney and prolonging life.” Modern chemical composition and pharmacological studies show that DC also has many functions, such as antitumor, anti-aging, enhancing body immunity, and effectively reducing liver injury (Wang and Li, 2014). Wild DC has less natural distribution, strict requirements for growth conditions, slow growth, low reproduction rate, and excessive logging. At present, it is listed as an endangered traditional herbal medicine variety. With the improvement of people's health awareness, herbal medicinal materials similar to DC for both food and medicine have attracted more and more attention in China, east Asian countries, and regions. Now in China, the central government and local departments attach great importance to the traditional herbal medicine industry, and relevant policies have been issued (as shown in Table 1).
At present, the artificial cultivation of DC has developed rapidly, but the quality is mainly judged by human experience in the market, which has become an important technical bottleneck and restricting the realization of intelligent processing and accurate directional regulation of DC processing technology. Therefore, to realize the objective, quantitative, real-time, fast, convenient, and practical scientific evaluation detection of DC quality, then automatically optimize the differentiated and standardized primary process parameters on the production according to the properties of raw materials, and then to make machine that replaces the human experience, it is necessary to find an effective method to promote traditional herbal medicine industry to intelligent manufacturing.
Dendrobium candidum is a perennial herb belonging to Orchidaceous Dendrobium (as shown in Figure 1). Due to its strict requirements for growth environment, such as altitude, humidity, and light, its industrial distribution is regional. Wild DC is mainly distributed in east Asia, southeast Asia, and Australia (Li and Li, 2017). At present, there is no wild DC in the Chinese market as the state has banned picking, so they are all planted in greenhouses or imitation wild environments. It is mainly produced in several provinces in China, such as Anhui, Zhejiang, Yunnan, and Taiwan. In the recent years, the medicinal and food healthcare value has been continuously excavated, which has led to the continuous expansion of its industrial planting scale (Wang and Shi, 2019).
After years of herbal industry development, there are a number of well-known leading enterprises, which provide unique conditions for DC industry. The chain of the traditional herbal medicine industry is long, and quality is the core factor. In this article, DC quality detection research is described from several angles and its development trend prospects.
Dendrobium candidum quality grade and evaluating index
Dendrobium candidum quality grade
There is no unified grade standard for DC quality. Different scholars have different methods (Table 2). For example, classification standard (Wang et al., 2021) has been built for fresh DC according to appearance, taste, middle internode length, internode number, and middle diameter as main evaluation indexes, which is divided into three grades in total, including DC planted on the cliff, under the tree, and in the greenhouse. A variety of cultivation methods (Xu et al., 2018) was classified according to the living environment, such as tree imitating wild, dead tree imitating wild, greenhouse imitating wild, stone imitating wild, rock wall imitating wild, forest imitating wild, and pure wild. The main quality factors are the contents of polysaccharides, mannose, alcohol-soluble extract, flavonoids, polyphenols, and crude fiber. It is considered that pure wild DC has the highest content for all quality indexes and significantly higher than any other cultivation methods. There are unwritten regulations in the market that DC is divided into five grades: they are special grade I, special grade II, grade I, grade II, and grade III (Table 3); however, this description is vague, and it is only distinguished from growth environment, planting process, growth characteristics, planting conditions, and planting mode. The main evaluation index is to compare the growth environment and features with wild DC.
The international standard ISO 21370: 2019 traditional Chinese herbal medicine Dendrobium candidum submitted by Zhejiang Shouxiangu Pharmaceutical Co., Ltd [a very famous company in the herbal medicine industry (Chai, 2019)] regulates the sensory indexes, physical and chemical indexes, agricultural residue heavy metal indexes, detection methods, packaging, storage, and transportation requirements. The main quality indexes are water contents, total ashes, heavy metals, pesticide residues, and polysaccharides. This standard covers many contents, including the whole process of DC, but the quantitative standard on quality is not obvious. Lu (2014) supplemented the quality indexes of DC and compared the differences in chemical components from different places. Zhang et al. (2018) measured 14 indexes such as the pseudobulb length of DC and used character variation analysis to clarify the quality differences between different regions.
In short, there are many factors affecting the quality of DC, external morphological features are easy to detect by image process, internal physical and chemical indexes are direct factors reflecting quality, and the evaluation criteria should be detected by different methods.
Dendrobium candidum quality index
As a typical herbal medicinal material for both food and medicine, DC has many substances beneficial to the human body, so the quality index concerned in different eating occasions is different (refer to Table 4). According to the (Pharmacopoeia Commission of the People's Republic of China, 2010), it takes polysaccharides and mannose as the quality standard, but it is relatively simple and cannot describe the quality fully and accurately. Tang et al. (2015) believed that polysaccharide, dendrobine, and flavonoids are the main pharmacodynamic components, and it is directly related to the quality of DC. The content of the main components is different with different growers in different regions and different growth ages, and even the content is very different in the same planted region. Polysaccharides are closely related to the efficacy of DC, such as enhancing immune function, antitumor, anti-aging, and antifatigue and reducing blood sugar. Wang H. et al. (2019) has done relevant work about polysaccharide extraction and antioxidant activity. Pharmacological effects are mainly cardiovascular, gastrointestinal, and antipyretic, and the contents of dendrobine and polysaccharides were determined in DC (Ge et al., 2015). Flavone is a compound that can reduce inflammation and virus, improve sleep, and protect the heart and brain. It has the functions of regulating body function, anti-aging, improving blood circulation, and promoting metabolism. Wu et al. (2011) tested the total amount of flavone. Huang et al. (2014) studied the antioxidant activity of different polar extracts of DC in vitro and its correlation with the content of polyphenols and flavonoids. Lv et al. (2017) determined 10 flavonoids in DC, compared the content of flavonoids from different producing areas, carried out a systematic cluster comprehensive analysis combined with pharmacopeia indexes, and analyzed the composition and content of polysaccharides at the same time.
It can be seen from the above literature that the quality evaluation standards of DC mostly focus on polysaccharides, dendrobines, and flavonoids. Generally, the three indexes can comprehensively reflect DC quality.
In addition to directly detecting these parameters for weighing DC quality, some scholars indirectly reflect the quality by measuring the substances closely related to the quality parameters, for example, to reveal the relationship between endophytic fungi and the functional components. Yu et al. (2016) measured polysaccharides and flavonoids in the mycelium of 21 kinds of endophytic fungi of DC and the extract of fermentation broth, it also provided a theoretical basis for the quality determination by endophytic bacteria, and it is another idea for DC quality detection.
Methods of Dendrobium candidum quality detection
There are many methods for DC quality detection, most of which are traditional destructive methods. For example, Wang et al. (2015) compared the differences between chemical components and their counterfeits by combining fingerprint and naringin content, analyzed DC and its counterfeits by HPLC, determined naringin content, and compared the similarity of a fingerprint with traditional herbal medicine chromatographic evaluation system, indicating that there is a great similarity between the fingerprints of authentic DC. Besides, there are also some methods including DNA and near-infrared technology to detect the quality, origin-producing place and authenticity of DC.
Sensory evaluation of Dendrobium candidum quality
Many factors have a great impact on the efficacy, taste, and value, such as planting place, variety, picking period, and other factors of DC. Therefore, it is most common to evaluate DC quality through sensory evaluation (refer to Table 5). Some scholars indirectly reflected internal elements' content through sensory features, especially predicting polysaccharide content. For example, He (2017) made a stepwise regression analysis between structure indexes (hardness, shear force, and shear displacement) and polysaccharide content of DC. It was found that the most influential factor on polysaccharide content was hardness, followed by shear force. According to the stepwise regression analysis between structure indexes (viscosity and consistency) and polysaccharide contents, the biggest factor affecting polysaccharide content is viscosity, followed by consistency, which indirectly reflects polysaccharide content according to sensory features. Zhong et al. (2020) compared hardness, shear force, shear displacement, viscosity, consistency, sensory score, and color difference among three kinds of DC; polysaccharide content, molecular weight, monosaccharide composition, flavonoid content, and alkaloid content were used to explore the relationship between quality and Dendrobium structure before and after beating. The results showed that there were significant differences in hardness, shear force, polysaccharide molecular weight, consistency, and color difference among three Dendrobium samples, and there were significant differences for DC quality detection at the same origin-producing places, same series, and different varieties. This can be used as the basis for quality, variety, and origin-producing place evaluation.
Lan et al. (2017) took cultivated DC species in different growth environments as the research sample, compared morphological features, yield, disease resistance, and polysaccharide content, and finally determined that the DC with high polysaccharide content and good disease resistance is more suitable to be processed into a product. Luo (2018) took five Dendrobium species as research samples from Longling County, Baoshan, Yunnan Province, and compared the differences in pharmacodynamic active components, sensory qualities, and structure indexes of different Dendrobium species, and the researcher established a correlation between structure and active components and carried out stepwise regression analysis between physical property indexes (hardness, shear force, and shear displacement) and Dendrobium polysaccharide content; it can be seen that hardness has the greatest influence on the content of Dendrobium polysaccharide. The stepwise regression analysis among viscosity, consistency, and polysaccharide content showed that the most important factor for Dendrobium polysaccharide content was viscosity, followed by consistency.
Jiang et al. (2019) carried out DC local cultivation from three different producing areas, sensory evaluation and polysaccharide content were determined, the results showed that viscosity was significantly different, and the viscosity of Zhejiang and Jiangsu strains was significantly higher than those of Yunnan strains. In the experiment, five persons with a healthy physique, sound mental quality, no bad habits, and no partial eclipse were selected as sensory evaluation participants to evaluate the sensory taste of fresh DC materials (the evaluation criteria are shown in Table 6). After a taste, three stem segments were taken for repetition and recorded the taste evaluation scores. The evaluation criteria refer to morphology and quality description in national standards for DC quality, as well as evaluation indexes, which are used in health care and consumption. To make the evaluation intuitive and reliable, quantitative scoring was used to judge which criteria were the indicators biased toward.
According to this standard, fresh DC sensory evaluations are shown in Table 7, and it can be found that the smaller the score, the better the effect.
Chen Y. L. et al. (2020) analyzed the correlation among polysaccharides, active components, and the structure of DC in different regions and established a new evaluation system, and the result shows that the content of polysaccharides can be detected by structure features.
Vision technology was expanding the cognition of human eye. The quality of DC can be detected through vision technology. Xin (2011) compared microscopic features between tube plantlets and commercial DC with digital photography, among these microscopic features, the powder of commercial DC has obvious features, including stem epidermal cells, nonglandular hairs, and calcium oxalate needle crystal bundles, the powder features of tube plantlets have small calcium oxalate needle crystal bundles, and there were no obvious microscopic features in embryo powder. Yin (2014) used modern microscopy technology with ordinary light and fluorescence to observe DC and counterfeit that were easy to be confused in the market and established a microscopic feature database of Dendrobium species for the first time. DC can be detected quickly and accurately according to microscopic features in cross-section by observing ordinary light and fluorescence.
Wang (2012) not only showed obvious differences in seed germination of DC, but also in the growth of test tube plantlets. In addition, the seed germination and growth of different germplasm were better than their parents, the result indicated that heterosis exists in DC, and the variety improvement can be carried out by hybridization. On the growth features of DC, there were great differences in plant height, stem diameter, and leaf type from different native places.
Application of DNA technology in quality detection
The DNA technology has more and more application scenarios due to its detection accuracy. There are a variety of research cases on the quality of DC (Table 5). Polymerase chain reaction (PCR) is a molecular biology technology to amplify specific DNA fragments. It can be regarded as a special DNA replication in vitro. The most important feature of PCR is that it can greatly increase a small amount of DNA. In identifying the medicinal properties of DC, Geng et al. (2015) used PCR to amplify the mitochondrial genes of 39 individuals in 17 species; meanwhile, suitable gene fragments were selected to study the joint detection. Three wild Dendrobium species from Huoshan were successfully detected based on nrDNA. ITS+nad 1-intron two combined fragment using an unweighted pair group method with arithmetic mean, and the accuracy rates are 100%. Shi et al. (2006) studied the DNA of DC and seven other Dendrobium species, and ribosomal 5S sequence was amplified by polymerase chain reaction. It was inferred that different Dendrobium species could be distinguished by 5S sequencing.
The sequence length and species of ITS vary greatly. RFLP labeling or sequence analysis of its amplicons can be used for detecting different biotypes, species and genera, even for distinguishing closely related species. Chen X. Y. et al. (2020) took 101 Dendrobium and Phalaenopsis samples as test materials and selected ITS as the most ideal DNA bar code, and it was found that 25 samples could be effectively detected. Xu (2011) established a rapid and accurate herbal medicinal material detection method based on its sequence alignment by combining real-time quantitative PCR with an amplification mutation system. This method can effectively detect DC materials from adulterants. It is suitable for fresh and dry materials, especially for deep-processing herbal medicine. Chen (2021) took 12 DC from Zhejiang Province and 10 Dendrobium from other provinces as experimental materials, selected DNA barcode ITS2, and find a rapid detection method of single-nucleotide polymorphism (SNP) sites based on high-resolution melting curve (HRM). Liu et al. (2014) analyzed ITS2 sequence differences between DC and its common adulterants and established a rapid detection method for DC authenticity. Yang et al. (2018) studied the genetic diversity of DC at the molecular level and discussed the genetic relationship among different DC varieties. It provided the scientific basis for the detection of DC varieties, offspring differentiation, new varieties, and utilization and protection of germplasm resources.
The results obtained by DNA are accurate, including sequences of many organisms. However, this method is complex, time-consuming, and expensive compared with sensory evaluation methods, so it is not suitable for large-scale and real-time detection.
Biosensor detection
Biosensor detection is a device that monitors and transmits information about a life process, a device consisting of a biological component (such as enzyme or bacterium) that reacts with a target substance and an electrochemical or optical component that detects the resulting products or by-products and emits a signal (Jiang et al., 2018).
There are few cases of using biosensor technology to detect the quality of DC, but there are similarities in herbal medicine. For instance, the endophytic fungi from Fritillaria thunbergii were used as raw materials to successfully synthesize nanosilver by biosynthesis and silver nitrate solution, and the synthesis process was optimized by changing the reaction conditions. Then, the antibacterial activity and antitumor activity of nanosilver were studied. It was found that the endophytic fungi CBY4 and CBY13 isolated from Fritillaria thunbergii could synthesize nanosilver, a reaction condition suitable for the synthesis of nanosilver was optimized, and the synthesized silver nanoparticles have certain antibacterial and antitumor activities (Deng, 2020).
In addition, biosensors are combined with electrochemical and electrochemiluminescence methods to detect heavy metals in traditional Chinese herbal medicine. For example, by synthesizing nanomaterials, preparing biometric probes, and designing electrochemical biosensors based on deoxyribonucleic acid (DNA) hybridization chain reaction, host guest recognition, and gold sulfur bond (Au-S) self-assembly technology, the biosensors have a good linear response to hg~ (2+) in the range of 0.04–800 ng/ml, and the detection limit is 0.02 ng/ml(S/N = 3) (Kong, 2019).
The toxic substances were also detected in traditional Chinese medicine. Combined with a new nano-sensing membrane material, the monoclonal antibody against aflatoxin BL (afbl) was used as a biometric element. By investigating the effect of the interaction between AFB1 and the antibody on the [Fe(CN)6]3−/[Fe(CN)6]4−([Fe(CN)6]3−/4−) redox system in the test substrate, an electrochemical biosensor for the rapid detection of AFB1 in traditional Chinese medicine was constructed. Under the optimum conditions, the linear response range to AFB1 concentration was 0.1–100 pmol/L, and the detection limit was 14.7 fmol/L. The method was applied to determine the content of AFB1 in jujube, coix seed, and cassia seed, and the recovery of the known sample was 95.3%−109.5% (Wei et al., 2021).
The characteristic of biosensors is that immobilized bioactive substances are used as catalysts, and expensive reagents can be reused many times, which overcomes the shortcomings of the high cost of enzyme analysis reagents and complex chemical analysis in the past; it has strong specificity, only reacts to specific substrates, and is not affected by color and turbidity; the analysis speed is fast, and the results can be obtained in 1 min; the accuracy is high, and the general relative error can reach 1%; the operating system is relatively simple and easy to realize automatic analysis; the cost is low, and the cost of each measurement is lower in continuous use; some biosensors can reliably indicate the status of oxygen supply and the production of by-products in the microbial culture system. In the actual control, many complex physical and chemical sensors and displacement sensors can be used to obtain the information (Aquino and Conte-Junior, 2020).
This method also has some limitations, and the technology is not ideal in terms of the stability of the test results. The test results are easily affected by the physical and chemical environmental, resulting in the deviation of the test results and distortion of the final results (Rathee et al., 2016).
Rapid nondestructive internal quality detection
Given the similarity between DC and other herbal medicinal materials, many herbal medicinal material detection methods can be used for DC nondestructive quality detection, such as X-ray, computer vision, nuclear magnetic resonance, near-infrared spectroscopy, hyperspectral imaging, terahertz spectroscopy, and Raman spectroscopy technology (Jin, 2018).
Spectral analysis based on different modes
In general, DC rapid nondestructive detection was mainly based on spectral analysis in practical application. This method has many subdivisions, according to the essence of electromagnetic radiation, and it can be divided into atomic spectrometry and molecular spectrometry. Atomic spectrometry was divided into atomic emission, atomic absorption, atomic fluorescence, and X-ray fluorescence; molecular spectroscopy was divided into six methods: UV-vis spectroscopy, visible infrared spectroscopy, molecular fluorescence spectroscopy, molecular phosphorescence spectroscopy, nuclear magnetic resonance spectroscopy, and chemiluminescence spectroscopy. According to electromagnetic transmission mode, it was divided into absorption spectroscopy, emission spectroscopy, and scattering spectroscopy (Qiao et al., 2019). For DC, most of the quality detection methods used at present were visible/near-infrared spectroscopy technology, which was also the easiest to be applied in practice. The key was that visible/near-infrared sensor was small, affordable, and easy to carry (Villar et al., 2012). Nuclear magnetic resonance technology was expensive and large (Wishart, 2019), so it was not practical to be used in actual DC quality detection. X-ray detection speed was fast, which can realize herbal medicine internal visual analysis, but X-ray equipment was expensive and produces radiation to the human body (Meo et al., 2006).
Rapid nondestructive quality detection based on visible/near-infrared technology
At present, DC rapid nondestructive detection was mainly realized by visible near-infrared, which was called “black box” technology because it only required testers to be familiar with the instruments and data processing software, and was easy to train and predict, and it was the fastest developing method in the recent 10 years (Tang et al., 2020). Many scholars used this technology to detect origin-producing place or quality for food or agricultural products (see Table 5). Dendrobium Huoshan, Dendrobium Yunnan, and Dendrobium Zhejiang were the three most important producing areas in China. Gu et al. (2016) established a prediction model using near-infrared diffuse reflectance spectroscopy combined with soft independent modeling of class analogy (SIMCA) and conducted a rapid detection study on DC from five producing areas. The spectral data were smoothed by savitzky Golay, first-order savitzky Golay (S-G), derivative, mean centralization combined spectral preprocessing, and band selection optimization, and the accuracy and rejection rate of SIMCA model were 100%.
It was relatively easy to detect authenticity of fresh DC, but it was not easy to detect dried one. For example, DC, Dendrobium nobile, and other fake DC have been used as DC in the market. Liu (2015) used micro-near-infrared spectrometer to detect three kinds of easily confused DC from seven producing areas, the accuracy and rejection rate of models established for complete and crushed samples were 100%, and both stability and accuracy were high. Because the difference between producing areas was smaller than that between varieties, the detection of producing areas of DC was more difficult than that of varieties.
In terms of origin-producing place detection, Jiang et al. (2017) collected 231 Huoshan and Henan Dendrobium samples from a portable near-infrared spectrometer and obtained good results by partial least squares discriminant analysis (PLS-DA). The accuracy, sensitivity, and specificity models of the test set, validation set, and prediction set were 100%, respectively. In terms of DC quality detection, Hu et al. (2014) studied three different species with 5 grades. After spectral pretreatment, band selection, and principal component fraction optimization, the accuracy and rejection rate of SIMCA reached 100%. Tang (2014) collected 100 Huoshan Dendrobium samples from six categories: primary and secondary Dendrobium moniliform, primary and secondary DC, and rice Dendrobium and Dendrobium flower, and the detection was studied by Fourier transform near-infrared spectrometer with high precision and resolution.
Liu et al. (2014) have done a lot of work on a comprehensive analysis of DC. They collected samples from five main DC planting provinces in Yunnan, Guangdong, Guangxi, Zhejiang, and Guizhou and analyzed different parts, different years, different producing areas, and different drying types by infrared spectroscopy combined with traditional chemical methods, and the samples of different decoction pieces and extracted intermediate products were analyzed qualitatively and quantitatively. At the same time, an infrared quantitative calibration model for polysaccharide and mineral elements content was established.
Problems of Dendrobium candidum quality detection
Lack of quality influencing factor weight analysis
The interaction between quality indexes was not clear, the detection of endophytic bacteria focuses on species separation, the accuracy of quality influencing factors was a lack of relevant literature, and the quality grading was rough without a clearly quantitative index.
Quality components detection methods were miscellaneous
Traditional detection methods have high requirements for laboratory instruments and equipment, skilled technicians, reagent quality, and monitoring. Besides, many instruments were expensive, easy pollution of laboratory and environment, various processes, time-consuming, and laborious.
Limitations of detection methods
There were many detection methods, but each method has its focus. As the detection index of individual parameters, it was feasible, but as a comprehensive quality index, more systematic research was needed, such as multimodal analysis. Therefore, it was needed for a convenient, simple, low-cost, real-time, nondestructive, rapid, and accurate detection method.
Interaction mechanism between spectral data and quality factors was not clear enough
Spectral analysis was used to predict quality mainly through the correlation between spectral features and quality, but it was greatly affected by the variety and environment of samples, and the relationship between the molecular structure of quality factors and spectral information has not been solved from the mechanism.
Possibility of other rapid nondestructive detection methods for Dendrobium candidum quality detection
Quality detection by endophytic bacteria
Endophytic bacteria were microbial groups that survive in healthy plant tissues for a certain period or all periods without causing obvious infection symptoms in host plants. It mainly includes fungi, bacteria, and actinomycetes. A large number of studies have shown that plant endophytes can form a mutually beneficial symbiotic relationship with host plants, play an important role in their growth, and were one of the important factors affecting their drug properties (Xu, 2015). Gong et al. (2017) summarized in detail on the diversity of endophytic bacteria of DC and the effect of endophytic bacteria on the host. At present, a variety of fungi and bacteria have been isolated from DC in different environments, and some strains have significant growth-promoting effects on plants. Liu (2017) believed that the endophytic bacteria of DC promoted active components accumulation by inducing key enzymes expression in the synthesis pathway of Dendrobium polysaccharides and alkaloids, DC has a unique eco-type and health care due to long-term adaptation to various stresses, and therefore, the quality can be determined by endophytic bacteria. Chen et al. (2017) found that Pseudomonas and Acidovorax were dominant bacteria in roots, stems, and leaves. Ruminococcus, fecal bacterium, methylobacterium, and Shewanella showed tissue specificity, and the top 10 genera in relative abundance at the genus level were selected, to generate a species relative abundance distribution map. Abundance cluster and principal component analysis showed that the community structure of endophytic bacteria in roots and leaves was the most similar. Leaves were easier to collect and experiment with than roots; therefore, leaves could be detected directly.
Dendrobium candidum plants were rich in bacterial resources, and their distribution was affected by tissue structure and composition factors. This also showed that there was a great correlation between the content of endophytic bacteria and the quality of DC.
Relationship between macromolecules and spectra
As a macromolecule, endophytic bacteria widely exist in a variety of traditional herbal medicinal plants. Chen (2016) studied the antitumor and immune activities of saffron with the structure of endophytic bacteria polysaccharide. After biological macromolecules absorb energy, the energy of the whole macromolecule was increased to a new energy state. The energy state of molecules was closely related to the form of molecular motion, including the electron's motion, the atomic nuclei vibration, and molecule rotation. The excited state is the change in the electronic energy level. The excited state of a biological macromolecule was the electronically excited state after the biological macromolecule absorbs energy, and it was an important part of molecular biophysics. Biological macromolecules can produce various changes after absorbing energy. Therefore, according to these changes, the internal substances of DC can be analyzed qualitatively or quantitatively. The whole process was mainly divided into two steps: one was the absorbing energy process and the second was releasing energy process. The former was mainly visible/near-infrared absorption spectrum, and this technology allows atoms or molecules in the ground state and low excited state to absorb light in a certain wavelength and transmit it to each excited state to form a dark line or dark band spectrum arranged. These dark band spectra can be used as a basis for qualitative and quantitative analyses of a certain substance. This was also a technology that has been studied more at present. The latter was typically represented by fluorescence or phosphorescence spectrum, that was, the spectrum formed by releasing excess energy from atoms or molecules when they were transferred from high-energy level to low-energy level. At higher energy levels, the excited atoms and molecule transition to lower energy levels emit photons at a certain frequency.
Compared with the absorption spectrum, the emission spectrum has higher sensitivity in the detection of traditional Chinese herbal medicine. For example, the detection limit of fluorescence analysis was at least two orders of magnitude lower than that of absorbance analysis; that was, the sensitivity was more than two orders of magnitude higher.
Fluorescence and phosphorescence in Dendrobium candidum quality detection
There was a competitive relationship between phosphorescence and fluorescence. According to the spin forbidden transition rule, fluorescence was easier to produce than phosphorescence. If a substance emits both fluorescence and phosphorescence, it was easier to identify them according to their respective spectral features, attenuation dynamics, and properties of singlet and triplet states (Jin, 2018).
The horizontal black lines show the energy levels of a molecule (Figure 2), and the energy level is increasing along the vertical axis in the diagram. The bold lines show the lowest vibrational level of each electronic state, with the higher vibrational levels represented by thinner lines. The vibrational levels become more closely spaced as energy increases and eventually form a continuum; for clarity, only a subset of these vibrational levels was represented on the diagram (Ke and Dong, 1998).
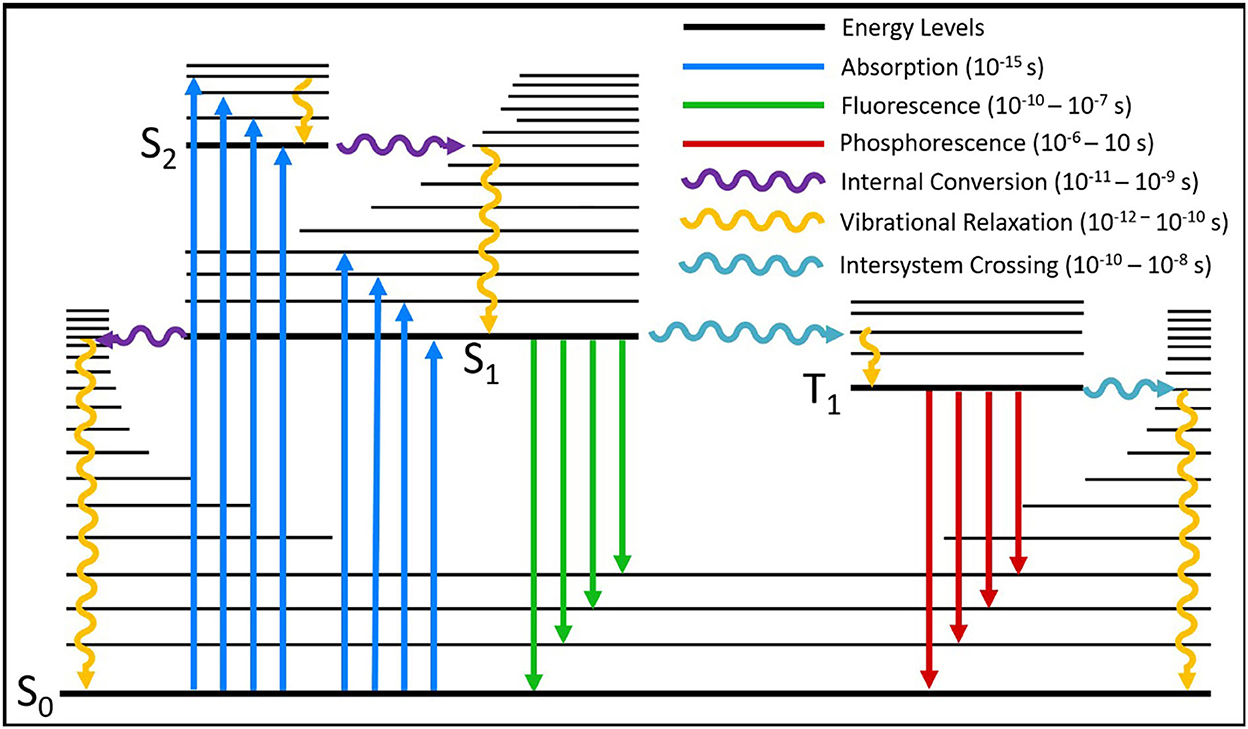
Figure 2. A typical Jablonski diagram showing the possible radiative and non-radiative transitions (IUPAC, 1997).
The naming of electronic states is based on the spin angular momentum configuration of each state. Singlet states (a total spin angular momentum of zero) are denoted by an S and triplet states (a total spin angular momentum of one) by T:
• S0 is the singlet ground state of the molecule.
• S1 is the first excited singlet state and Sn is the nth excited singlet state.
• T1 is the first excited triplet state and Tn is the nth excited triplet state.
Because the endophytic bacteria in DC contain a lot of organic substances, such as proteins, carbohydrates, and lipids, these substances contain a large number of chromophore structures, such as C=C, C=O, C=C, C-H, –N=O, –N=N–, and C=N, which provides a theoretical basis for the emission spectrum analysis quality of DC.
Fluorescence spectroscopy for quality detection
Fluorescence spectroscopy has the characteristics of high sensitivity, strong selectivity, less sample dosage, simple method, and more physical parameters. These characteristics determine that fluorescence analysis is a very effective spectrochemical analysis method and will play an important role in the field of spectral analysis (refer to Table 5). At present, there are few kinds of the literature on rapid nondestructive detection of DC by fluorescence.
Fluorescence qualitative analysis: different fluorescent substances have different excitation spectra and emission spectra, so fluorescence can be used to detect a certain substance. Compared with absorption spectroscopy, the fluorescence method has higher selectivity.
Fluorescence quantitative analysis: using the relationship, the fluorescence intensity is directly proportional to the material contained at a lower concentration. It is commonly used to detect the content of amino acids, proteins, and nucleic acids. One advantage of fluorescence quantitative determination is its high sensitivity. For example, the determination limit of vitamin B2 can reach 1 ng/ml, which greatly reduces the amount of sample required for detection.
The influencing factors of fluorescence intensity: (1) the influence of solvent. They are polarity, hydrogen bonds, and the formation of coordination bonds; (2) the influence of temperature. Fluorescence is sensitive to temperature, and the probability of inactivation increases with the increase of temperature; (3) the pH value of the solution has a great influence on the acid-base compounds; (4) internal filtering and self-absorption. In internal filtering, there are exciting or fluorescent substances in the solution, such as potassium dichromate; (5) self-absorption phenomenon: the short wavelength end of the fluorescence emission spectrum of compounds overlaps with the long wavelength end of their absorption, resulting in self-absorption, such as anthracene compounds (Gao, 1979; Edinburgh Instruments, 1997).
A fixed wavelength light is selected to irradiate the sample, which absorbs the excitation light and emits fluorescence, and detected fluorescence intensity at different emission wavelengths. Through the fluorescence emission spectrum, the emission wavelength corresponding to the strongest fluorescence can be obtained under a fixed wavelength excitation. Xu et al. (2002) observed the spontaneous fluorescence of hyphae, conidia, and fruiting bodies of three different fungi. The results showed that the spontaneous fluorescence of fungi was species-specific and affected by factors such as culture medium, while the culture time had no significant effect on fluorescence intensity and category. The chemical reagent test showed that the structure and composition of spontaneous fluorescence substances in different fungal cells could lead to a change in fluorescence properties. The occurrence of cotton verticillium wilt is closely related to endophytes, to understand the temporal and spatial dynamic changes for the number of cotton verticillium wilt endophytic fungi and its relationship with the number of verticillium wilt pathogens. Shi et al. (2018) measured endophytic fungi number of cotton verticillium wilt by TaqMan probe real-time fluorescence quantitative PCR and analyzed the relationship between cotton endophytic fungi number and the number of verticillium wilt pathogens. Ma et al. (2017) found that fungi spontaneous fluorescence during the real-time observation of citrus leaves infected by ulcer bacteria. Yu (2010) found that when arbuscular mycorrhizal fungi infect the roots of camptothecin acuminata seedlings to form arbuscular mycorrhiza, there was camptothecin spontaneous fluorescence in the vascular bundle, epidermis, root tip, root hair, and other parts, and there was also camptothecin spontaneous fluorescence in the parts where the arbuscular mycorrhizal structure was formed (hyphae, arbuscles and vesicles). After the formation of arbuscular mycorrhizal, the spontaneous fluorescence of camptothecin in the formation area of arbuscular mycorrhizal increased with the increase of arbuscular structure. To a certain extent, this proves the correlation between fungi and alkaloids. A fungus of DC may have a similar correlation with dendrobine; Jiang et al. (2015) labeled endophytic bacteria of corn with green fluorescent protein, under the fluorescence microscope, and it can be observed that a single cell emits strong green fluorescence. Cui et al. (2019) studied the response of DC ingredients by UV-B continuous radiation under different carbon and nitrogen ratios, and the results showed that UV-B radiation increased the defoliation rate, the content of carotenoids, total polysaccharides, total alkaloids, and the activity of antioxidant enzymes. Wu (2009) detected the fluorescence spectrum of Lactobacillus Plantarum, which was a kind of bacteria, and it was found that the strain emits fluorescence after absorbing ultraviolet light. In the range of 300–650 nm, the fluorescence spectrum of Lactobacillus Plantarum has four peaks, which were located near 338, 387, 465, and 538 nm, respectively. These four peaks correspond to the fluorescence peaks of the main substances in the cell and provide relevant information inside the cell.
Since the chemical reaction is related to the concentration of reactants and, in some cases, the concentration of catalysts (sometimes including activators, inhibitors, or demulsifiers), the content of the substance can be detected by measuring the reaction rate, which is the basis for the legal measurement of kinetic analysis, so this method is also called reaction rate. With the research deepening, people gradually realize that there is a wealth of information in the fluorescence induction kinetic curve. There were many studies on fluorescence in agricultural products. Yuan et al. (2018) used four wheat varieties with different drought resistances as materials and systematically studied the effects of dry matter quality and chlorophyll fluorescence parameters of wheat at seedling stage of normal, mild drought, severe drought, and rewatering after drought using indoor hydroponic culture method. The results showed that the dry matter quality of each wheat variety decreased significantly under drought stress, drought stress changed the rapid chlorophyll fluorescence kinetic curve, and the minimum fluorescence intensity increased. Huang et al. (2019) carried out double factor control experiment on soil water and nitrogen level with the grape variety “Hongti” as the test material, and the results showed that the dynamic change curve of rapid fluorescence induction in different observation stages of grape leaves at the seedling stage was significantly different at different characteristic point positions (OJIP) with the decrease of soil water and nitrogen level, the higher the water and nitrogen level, the greater the maximum fluorescence value of grape leaves. Marques da Silva et al. (2020) used chlorophyll fluorescence dynamics to classify grape gene types, when dark adapted leaves were irradiated with saturated light, a rapid multiphase rise of fluorescence emission (Kautsky effect) was observed, and the curve shape depends on the molecular structure of the photochemical device, which is an interaction function between genotype and environment. Wyber et al. (2018) used high-time-resolution photoinduced fluorescence dynamic technology to evaluate the effect of photosynthesis monitoring of mature potted Perseus outer crown leaves. The results show that under high dynamic light conditions, fluorescence dynamics can monitor photosynthesis remotely, which may improve the canopy photosynthesis model and estimate crop yield.
Photosynthetic phenotypes require a rapid description of dynamic characteristics when measuring the number of a large number of plants in a fluctuating environment; Keller et al. (2019) evaluated the ability of the light-induced fluorescence transient (LIFT) method to quickly generate fluorescence parameters within a distance of 0.6 m, under hypoxia or chemical inhibitors, and spinach leaves and thylakoids showed the same result with chlorophyll fluorescence parameters under controlled conditions. Stirbet et al. (2018) used another way to extract the information from fluorescence transients, which was to synthesize biomarker reactions, and this method can be used to combine chlorophyll A fluorescence data with other characterizations of CO2, which was convenient for a more plant performance comprehensive evaluation.
In addition to molecules that can excite fluorescence, they can also excite fluorescence on ions; Sun et al. (2019) successfully realized continuous, reversible, wide range, and high stability luminescence color regulation in single CsPbCl3 perovskite microcrystal doped with positive divalent manganese ion (Mn2+) by changing excitation conditions and found the fluorescence kinetic regulation mechanism of manganese ion-doped perovskite single crystal.
It can be seen from the above literature that endophytic bacteria of DC are macromolecules and have the conditions to produce fluorescence. Therefore, the quality of DC can be analyzed quantitatively and qualitatively by inducing endophytic bacteria to stimulate fluorescence.
Phosphorescence spectroscopy for quality detection
Molecular phosphorescence originates from the lowest triplet state of molecules. We can understand the molecular triplet information by observing the triplet energy transfer or electron transfer between molecules and establish a sensitive sensor system. At present, few kinds of literature have found that phosphorescence is used to detect DC quality or other herbal medicines, and it was more used for material analysis, such as Zhang et al.'s (2003) research on a certain luminescent material. Xu and Zhu (2009) conducted quantitative analysis on plant hormones. Du (1997) studied room temperature phosphorescence spectral properties of 1-bromonaphthalene induced by linear fatty alcohol in β-cyclodextrin solution, which showed that n-pentanol has the greatest sensitization to phosphorescence, then, the phosphorescence intensity gradually decreased from n-hexanol to n-octanol, the composition and stability constant of inclusion complex were measured, it was found that the binding strength of 1-BrN and A:β-CD inclusion complex was the decisive factor for the phosphorescence intensity of ternary inclusion complex, and the effect of alcohol molecular size on room temperature phosphorescence was explained from the possible structure of ternary inclusion complex.
Most of the research work focuses on the development of quantum dot-fluorescent sensors, but there is less research on the phosphorescence characteristics and potential phosphorescence detection ability of quantum dots. He et al. (2008) studied phosphorescence characteristics of Mn-doped ZnS quantum dots and established a simple, rapid, economic, sensitive, and selective room temperature phosphorescence (RTP) method for enoxacin detection in biological solution. Weinberger et al. (1982) studied the effectiveness of micellar stabilized room temperature phosphorescence (MSRTP) in aromatic molecule detection and quantification by high-performance liquid chromatography (HPLC). Although the sensitivity in this article is lower than that of classical fluorescence detection, by carefully selecting the excitation and emission wavelength according to the “total” luminescence curve, the selectivity has been significantly improved in the analysis of β-naphthol, biphenyl, and phenanthrene mixtures. Traviesa-Alvarez et al. (2007) designed a fast and simple flow optical sensor with online coupling phosphorescence for the direct screening of tetracycline antibiotics (tetracycline, oxytetracycline, aureomycin, and doxycycline) in water and milk samples. Rojas-Duran et al. (2006) introduced a visual screening method of aspergillus flavus strains based on room temperature phosphorescence (RTP), methyl β-cyclodextrin and bile salt (0.6% sodium deoxycholate) were added to the medium widely used in food mycology, and after incubation at 28°C for 3 days, the production of aflatoxin can be easily detected by RTP emission of aflatoxin mycelium observed under UV irradiation. After an experiment on 32 aspergillus samples by this method, it can be found that this phosphorescence phenomenon was reproduced in vitro after aflatoxin B1 was immobilized on ion exchange resin microspheres.
It can be seen from the above literature that phosphorescence is feasible for molecule detection, especially for macromolecules in DC flavonoids and polysaccharides. However, at present, there is no case of phosphorescence spectrum used in the detection of traditional herbal medicine from the existing literature.
Terahertz for quality detection
Terahertz (THz) is a new and important cross-cutting-edge technology with many outstanding advantages (Dhillon et al., 2017). In recent years, with the rapid development of ultrafast THz laser hardware, a stable THz radiation source has gradually formed, which makes THz technology an effective spectral detection technology and has considerable development potential and application prospects in the field of biological detection (Mittleman, 2017).
The application of THz technology in the field of biological detection mainly stems from its penetrability, which can penetrate plastics, ceramics, and other substances, and can also detect the biological tissue information under the epidermis; it has very low photon energy, will not produce ionization effect like X-ray, and will not damage the body and biological tissue; high sensitivity to polar substances; THz signal not only has better time resolution but also has better spatial resolution compared with microwave and millimeter wave.
Terahertz is sensitive to the interaction and structural changes of biological macromolecules. The absorption spectrum of biological macromolecules, such as proteins, has no obvious absorption peak and is not easy to identify directly. However, the structural information of different molecules is detected through the overall absorption change or phase change, so as to show the material law of the interaction between macromolecules and cells from the microbiological field and finally explain the life phenomenon (Bowen, 2018; Hao et al., 2018; Qu et al., 2018). THz spectroscopy has unique advantages in studying the molecular spatial structure, intermolecular reactions and interactions, and the interaction between molecules and the environment. It provides fingerprint features for identifying the physical properties of molecules, such as configuration, conformation, and environmental impact, and provides a theoretical basis for the application of THz spectroscopy in biological information detection.
Terahertz radiation frequency has a certain correlation with the weak interaction between molecules, such as hydrogen bonds, and lattice low-frequency vibration (El Haddad et al., 2013). Based on the fingerprint spectrum, we can study the structural features of substances. The fingerprint spectrum here is the spectral features of molecules with different polarities exposed to THz radiation, which is mainly related to the absorption characteristics of molecules in this band. More scholars have joined the research in this field (Pliński and Plińska, 2012). In addition, the feature absorption peaks of peptide molecules in THz band are typical. Based on this, we can effectively identify the fingerprint features, which is also an important research direction. THz spectroscopy can be applied to the detection and quality control of impurities in food. The research confirmed that THz spectrum can be applied to the fields of environment and food detection, which has obvious advantages over traditional detection technology (Dorney et al., 2001). Jordan realized the detection of sundries in food based on this technology. During the experiment, glass slag and small metal blocks were added to food, detected by THz spectroscopy technology, and obtained more accurate detection results (Scheller et al., 2008). Double pulses are detected in the THz pulse waveform with impurities. Based on this, the THz image is processed and analyzed to accurately detect the glass slag impurities mixed in it, and then, the effectiveness of this method is verified.
This technology can also be applied to the detection of food corruption, which can detect the moisture contained in food. Some scholars have confirmed its detection effect through experiments. Chua et al. (2005) realized the effective detection of wheat flour moisture based on THz in their research. For milled wheat flour, in the range of 0.1–2.0 THz, the model is constructed based on the THz wave attenuation caused by wheat flour. The wheat flour is mainly divided into four groups, and the water content has obvious differences, which are set as dry, 12, 14, and 18%, respectively. The adulteration of vegetable oil has a long history, and some researchers have put forward different detection methods. Yu et al. (2018) conducted a lot of research on the problem of oil adulteration, carried out detection and analysis based on THz spectrum, obtained the corresponding parameters combined with the measured pulse time-domain waveform, built a model on this basis, and predicted and obtained more accurate detection results. At present, there are residual pesticides in many agricultural products. The application effect of THz technology has been confirmed in some studies. Yan et al. (2008) measured and analyzed the refractive index spectra of different pesticides (mancozeb and imidacloprid) at 0.2–2.0 THz based on THz TDS technology. It was found that there were great differences in refractive index between them.
In addition to the above research and application, THz technology has also been adopted in the field of microdetection, which can detect viruses and other microorganisms, showing great application value in food detection and disease diagnosis and treatment. Choi et al. (2002) obtained the reflection coefficient and other information of Bacillus powder in the research process. In the experiment, the reflection and projection methods were mainly used. The research results show that a variety of substances can be effectively identified. Globus et al. (2004) focused on the identification and analysis of a variety of bacteria. Lu et al. (2005) realized the detection of molecules based on THz spectrum. In their research, they integrated this technology with biochip technology, made full use of their advantages, and obtained relatively reliable detection results. Later, the drug components such as amphetamine were detected and analyzed, and it was found that the corresponding feature spectrum could still be obtained in the case of a small number of drugs. Therefore, the above research verifies the application value of THz technology in the field of microdetection and can effectively detect harmful components, viruses, and biomolecules. Therefore, terahertz technology also has great application prospects in the quality detection of traditional Chinese medicine, such as DC.
Molecular dynamics calculation and simulation
Nondestructive detection of optical properties of DC and other similar agricultural products is a new technology in recent years. It is an integrated technology of light, machinery, and electricity. At present, the rapid nondestructive detection of internal quality mainly adopts the methods of the spectrum or hyperspectral image. Optical detection technology is used in the appearance of agricultural products (surface defects, color, and the like) and internal components (soluble solid sugar, firmness, acidity, dry matter content, and so on). External quality inspection is easy to understand, and for internal quality detection, there are three main methods: regular reflection light, diffuse reflection light, and transmission light method. In practice, the rapid nondestructive detection effect is good at a few material elements, but the detection result is disturbed under more material elements by many factors, such as environment, the interaction between substances, light intensity, and band range. The current practice was to analyze spectra and establish a correlation between spectra and measured substances according to empirical and statistical methods; however, due to the influence of spectral superposition, the effect is not good in the practical application of agricultural products. Therefore, it is very necessary to explain the direct influence of spectrum on various substances from a molecular basis, that is to find the eigen spectrum and determine the substance to be detected according to the eigen spectrum.
Molecular dynamic simulation (MDS) has been developed for more than 50 years since 1966. With the continuous improvement in computational methodology and rapid development of computer computing power, MDS technology has continuously penetrated into biology, chemistry, pharmacy, and other related fields and has been widely used in recent years; at present, it has become the third analysis method besides theoretical analysis and experimental research (Li and Liu, 2001; Ouyang et al., 2005; Li et al., 2020; Zhang B. et al., 2021).
Computational simulation has become an important means of chemical and biology research. On the one hand, the existing methods are often indirect detection, which needs theoretical and computational interpretations. On the other hand, due to the limitation of experimental conditions, the ideal experimental conditions cannot be truly realized. By means of molecular chemistry calculation and simulation, we can understand the structure performance relationship, kinetic properties, and reaction characteristics of chemical substances at the molecular level, which are difficult to achieve through the traditional laboratory at the macrolevel.
Molecular dynamic simulation can not only get trajectory of atoms but also carry out various observations, such as experiments, especially microscopic details related to atoms that cannot be obtained in actual experiments. It can be observed conveniently in MDS. Compared with the experiments, MDS has the following advantages: (1) low cost; (2) reduces the incidence of experimental accidents and has high safety; (3) it is helpful to better understand and obtain some molecular level structural and kinetic data that are difficult to obtain experimentally and effectively supplement the experimental data (Zhou et al., 2020).
At present, there are few studies on the combination of MDS and spectral characteristics. Lv et al. (2019) measured spectral changes of myoglobin (MB) by UV absorption spectroscopy, fluorescence spectroscopy, and circular dichroism and analyzed the secondary structure, content of MB, protein carbonyl, sulfhydryl group, and surface hydrophobicity of MB. Based on this, combined with MDS, the structural changes of MB under the action of ozone were explored to provide a theoretical basis for raw meat color protection technology. The simulation results are consistent with real results.
Liu (2020) searched for potential type II 5α-reductase (5αR2) inhibitors from active components of traditional herbal medicine based on molecular docking and MDS methods and verified biological activity through in vitro micro-enzyme reaction system, indicating that MDS results are consistent with the docking results (as shown in Figure 3).
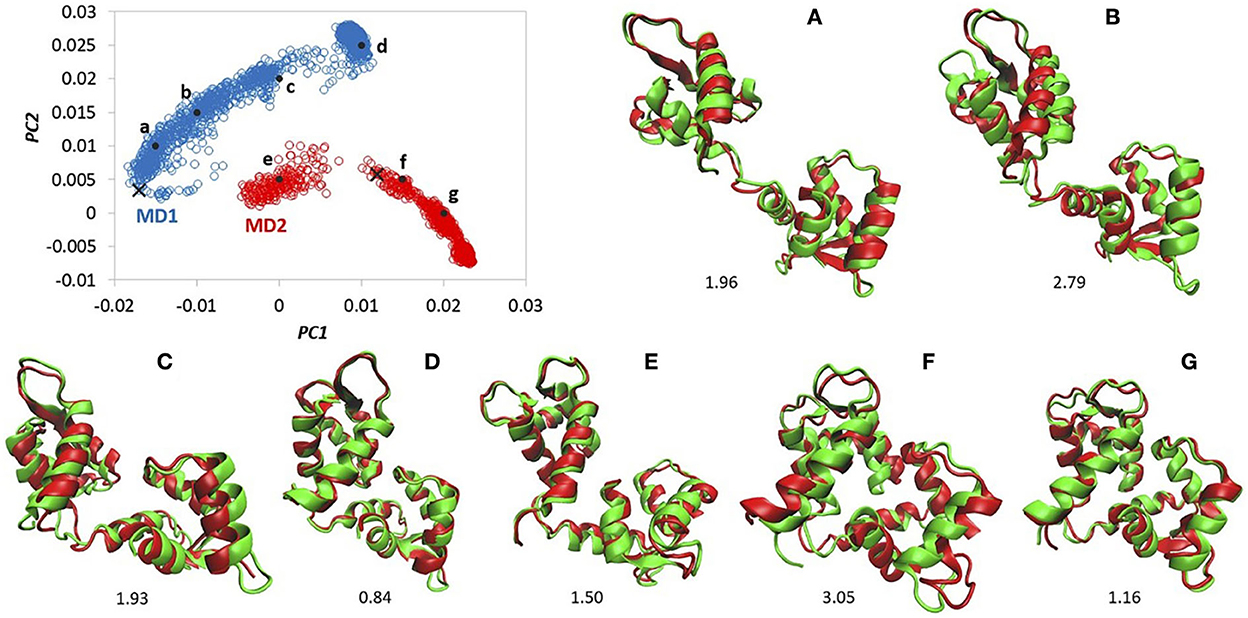
Figure 3. Textile predictions of CaM. Top left: PC2 vs PC1 plot from the 2D RMSD matrix constructed from two MDS (blue and red circles), with points a–g (black dots) used for testing. The black crosses belong to the starting textiles for each simulation. For each textile, testing only included the MD simulation that the textile was not taken from (MD2 for a–d, MD1 for e–g). Overlays of the original textile (green) with the predicted textile (red) are shown for each point (A–G), with the RMSD shown below.
Jin et al. (2021) presented a proof-of-principle method of combining MDS with machine learning to explore protein conformational space. For seven predicted structures, the average RMSD relative to the target structure was 1.89 ± 0.81, and even for the worst predictions, the overall gross structural features were successfully predicted. We can find that the authenticity of MDS is very accurate. According to this mechanism, there can be a good prediction between the molecular structure of DC and its possible characteristic spectrum.
Some scholars (Qu et al., 2018) compared the results of theoretical spectra and experimental spectra of several pesticides in the detection of pesticide residues and found that the theoretical spectra and experimental spectra have high consistency in peak position matching except for slight frequency shift and less loss of absorption peaks (as shown in Figure 4).
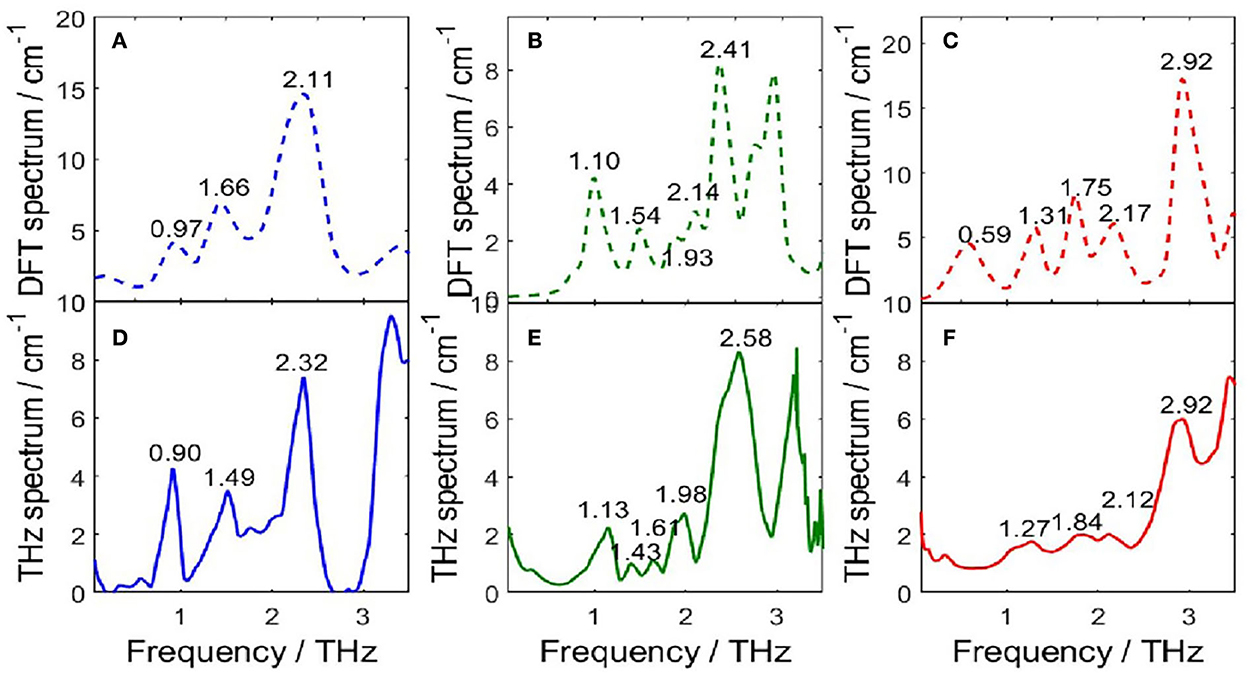
Figure 4. Comparison of experimental and theoretical spectra of three pyrethroid pesticides. (A–C) Density functional theory (DFT) spectrum of deltamethrin, fenvalerate and beta-cypermethrin, respectively. (D–F) THz spectrum of deltamethrin, fenvalerate, and beta-cypermethrin respectively.
In this way, we can also see that it is very convenient to explain the significance of the spectrum through the dynamic simulation of molecular structure.
Because of the characteristics of molecular dynamics, the intrinsic spectrum of each index to be measured can be obtained, which can provide a theoretical basis for other spectral detection methods, such as fluorescence spectrum, phosphorescence spectrum, and terahertz spectrum.
Multimodal (cross-modal) method for Dendrobium candidum quality detection
Research status of multimodal technology
Multimodal detection refers to the integration or fusion of two or more detection technologies, making use of its unique advantages of multiple detection technologies and combining data fusion technology to make the authentication and detection process more accurate.
Multimodal technology is applied earlier in medicine because there are many kinds of images in medicine, such as magnetic resonance imaging (MRI), ultrasound, and computed tomography (CT). There is great complementarity between different imaging modes. For example, X-ray computed tomography (XCT) and MRI can provide structural information, but cannot be used for pathological diagnosis; single photon emission computed tomography (SPECT), positron emission tomography (PET), and optical imaging technology can provide changes in cell function and metabolism, but cannot accurately locate diseased tissue. Lu (2012) studied these methods, and they were calibration methods of XCT geometric parameters based on the multimodal imaging system, XCT accurate reconstruction algorithm, and XCT/DOT dual-mode tomography technology. In addition to medical image technology, there was also sequence detection, such as electrocardiograph (Schulte et al., 2014; Johnson et al., 2015; Pimentel et al., 2015).
In terms of agricultural products, Lin et al. (2019) proposed a method of soybean appearance quality detection based on a low-rank sparse representation framework of visible spectrogram multimodal dictionary features, so as to accurately determine soybean quality grade. Wang et al. (2015) developed a multimodal machine vision system to comprehensively and nondestructive evaluate onions' quality factors. The system integrates hyperspectral imaging, 3D, and X-ray imaging sensors, obtaining color image, spectral image, depth image, and X-ray image of onion and detecting the weight. Based on collected multimodal data, an algorithm was developed for calculating the maximum diameter, volume, and density and detecting potential defects of onions. Finally, three groups of sweet onions were tested with good results.
In agricultural remote sensing, multisource data fusion was carried out with multiple data of satellite, UAV, and ground remote sensing detection (Jia et al., 2000). Because satellite remote sensing focuses on macro- and large-scale information, and the detailed information should be supplemented by UAV and ground remote sensing, it was also more and more used in practice (Shan et al., 2019; Wang H. et al., 2019; Zhang Z. et al., 2021; Zhao et al., 2021). It can be seen from Figure 5 that multimodal data are the very important prerequisite for obtaining accurate ground object information.
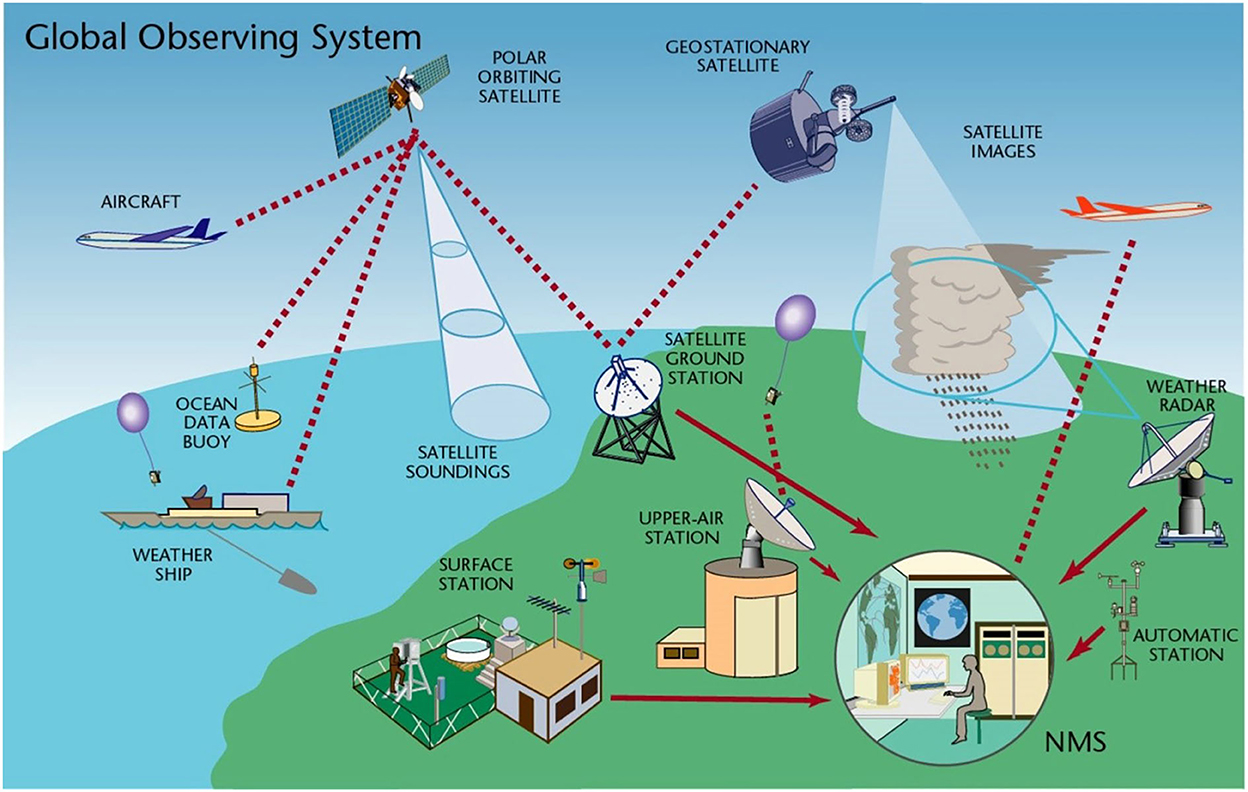
Figure 5. Analysis of multi-source remote sensing data (Robert FitzRoy, 2010).
There are some methods for rapid and nondestructive quality detection of DC, but each method has its focus. Under multimodal technology, one mode can supplement the weakness of another mode, such as micro-image is sensitive to the variety and growth time of DC, fluorescence spectrum is sensitive to molecular structure, and near-infrared spectrum is sensitive to hydrogen-containing groups. The combination of these modes can form complementarity, eliminate ambiguity and uncertainty, integrate the information of multiple modes, and obtain more accurate results. If the modes are complementary, the quality of DC can be perceived quickly, accurately, and effectively.
Key points of multimodal fusion technology
Similar to traditional data analysis, multimodal data have high capacity, diversity, and different structures. The diversity of multimodal data is more prominent as it composes several modes. These modes contain partial descriptions of the same things involved in the distribution independent of each mode, and there are complex correlations between modes. The complete model of fusion representation hidden in modes and cross-modes can further improve the performance of various multimodal applications. Also, multimodal data cannot be processed directly and can produce different datasets connected with each other.
Deep learning is a new research direction in the field of machine learning. Deep learning is learning the intrinsic rules and representation levels of sample data and deriving information from them. This information is useful for the interpretation of data, such as text, images, and sounds. Deep learning neural networks mimic the human brain through a combination of data inputs, weights, and biases. These elements work in concert to accurately identify, classify, and describe objects in the data. Deep neural networks consist of multiple layers of interconnected nodes, with each layer building on top of the previous one to refine and optimize predictions or classifications. This computational process performed through the network is called forward propagation. The input and output layers of a deep neural network are called visible layers. The input layer is where the deep learning model ingests data for processing, and the output layer is where the final prediction or classification is made. Another process called backpropagation uses algorithm, such as gradient, descent to calculate the error in the prediction and then adjusts the weights and biases of the function by moving the layers backward to train the model. Together, forward and backward propagation enable neural networks to make predictions and correct any errors accordingly. Over time, the algorithm gradually becomes more accurate (Hao et al., 2016). Ashtiani et al. (2021) using convolutional neural networks for the classification of mulberry fruit ripening stages demonstrates high accuracy of deep learning.
With the development of deep learning technology, there are also some pioneering deep learning models in the field of multimodal data fusion, such as cross-pattern retrieval, image annotation, and auxiliary diagnosis. Yang (2019) designed the structure of a multimodal target detection network, proposed a step-by-step network training method, and achieved good detection results.
Although some progress has been made in the deep learning model of multimodal data fusion, it is still in the preliminary stage. Zhang et al. (2020) reviewed the relevant literature on data fusion methods based on deep learning in recent years and analyzed the data fusion methods based on deep learning from three aspects: data fusion methods based on deep learning feature extraction, deep learning fusion, and the whole process of deep learning. There are many methods and scales for multimodal integration, mainly including stage-based and integration strategy, feature-based and semantic-based model fusion (Yu et al., 2020). Table 8 summarized the comparison of different model fusion methods.
However, there are also the following problems in multimodal data fusion:
• Heterogeneous data fusion. How to complete the association and intersection of heterogeneous data and finally obtain the correlation between data is the focus of heterogeneous data fusion research.
• Complex methods have good data processing ability (such as deep learning methods), but they also bring a series of problems. For example, the training model needs a lot of data, the training model has high requirements for the computing power of the equipment, and the training model takes a long time.
• Data fusion evaluation system. At present, most data fusion methods are evaluated based on simulation or idealized assumptions, which makes it difficult to evaluate the practical application effect of the model. Evaluating the model from the actual scene will make researchers pay more attention to the performance of the model under ideal conditions and ignore the performance of the model in the actual application scene, which will hinder the development and application of data fusion technology.
• Data fusion model security. In the special application field of data fusion, such as the military field, there is an urgent need for a secure model to complete the fusion of military data.
• Sensor limitations. At present, much data are limited by the performance of sensors, so the collected data cannot meet actual production requirements, such as response sensitivity, environmental adaptability, data accuracy, response speed, and the like.
Therefore, to make detection results credible, we should work hard on software and hardware at the same time.
Conclusion
With the deepening of agricultural artificial intelligence, there are a variety of methods for traditional herbal medicine quality detection using fast nondestructive quality perception technology. This review summarized and combed the application of various methods in the quality detection of DC from traditional methods, DNA methods, sensory methods, and near-infrared spectroscopy and puts forward the development direction in the future. At present, the quality of DC is uneven in the market, and there are a variety of detection methods, but there is still a long way from practical application. Affected by the complexity of planting environment and varieties, the reliability of monitoring results is low, and the accuracy is difficult to ensure. To solve these problems, we need to effectively integrate agronomy and planting experience knowledge, new and innovative detection methods, and multimodal information and detection methods, Which makes the technology and method mature in the application.
Author contributions
FC, ZC, and YH contributed to the conception and first draft preparation. HS, JZ, KW, and SZ reviewed, analyzed, and provided interpretation. All authors contributed to the article and approved the submitted version.
Funding
This work was supported by the Zhejiang Province Key Research and Development Program [Grant number: 2021C02011 and 2021C02016], Zhejiang Province Public Welfare Technology Application Research Project [Grant number: LGN18F030002], the Major Science and Technology Projects of Breeding New Varieties of Agriculture in Zhejiang Province [Grant number: 2021C02074], WenZhou Key Scientific and Technological Innovation Project [Grant Number: ZN2022006], and the Project of Hangzhou Science and Technology Bureau [Grant number: 20201203B116/20201203B97].
Acknowledgments
The authors would like to thank all the members of the research group for the valuable discussions about the ideas and technical details presented in this article.
Conflict of interest
The authors declare that the research was conducted in the absence of any commercial or financial relationships that could be construed as a potential conflict of interest.
Publisher's note
All claims expressed in this article are solely those of the authors and do not necessarily represent those of their affiliated organizations, or those of the publisher, the editors and the reviewers. Any product that may be evaluated in this article, or claim that may be made by its manufacturer, is not guaranteed or endorsed by the publisher.
References
Aquino, A., and Conte-Junior, C. A. (2020). A systematic review of food allergy: nanobiosensor and food allergen detection. Biosensors 10, 194. doi: 10.3390/bios10120194
Ashtiani, S. H. M., Javanmardi, S., Jahanbanifard, M., Martynenko, A., and Verbeek, F. J. (2021). Detection of mulberry ripeness stages using deep learning models. IEEE Access 9, 100380–100394. doi: 10.1109/ACCESS.2021.3096550
Bowen, J. W. (2018). “Terahertz nanoscale science and technology,” in Quantum Nano-Photonics. NATO 2017. NATO Science for Peace and Security Series B: Physics and Biophysics, eds B. Di Bartolo, L. Silvestri, M. Cesaria, and J. Collins (Springer). doi: 10.1007/978-94-024-1544-5_7
Chai, Y. (2019). ISO international standards for Ganoderma and Dendrobium officinale are released, and standardization is the driving force for Chinese medicine to go international. TCM Healthy Life Nurturing 5, 76–77.
Chen, W. H. (2016). Study on the Structure and Anti-tumor and Immune Activity of a Saffron Endophytic Fungus Polysaccharide. Guangdong: Guangdong Pharmaceutical University.
Chen, W. Q., Wang, X. F., Chen, X. Y., Peng, C., Xu, J. F., and Cai, J. (2021). A preliminary study on the identification of Dendrobium candidum from Zhejiang Province based on ITS2 and SNP technology. Acta Agric. Zhejiangensis. 33, 69–76. doi: 10.3969/j.issn.1004-1524.2021.01.09
Chen, X. Y., Liu, Y. Y., Tan, Q. Q., Ren, J. R., Jiang, X. X., Jiang, X. M., et al. (2020). Application of DNA barcode and real-time fluorescence quantitative PCR in the identification of Dendrobium candidum. J. Nucl. Agric. Sci. 34, 41–51. doi: 10.11869/j.issn.100-8551.2020.08.1655
Chen, Y. (2021). A transfer learning model with multi-source domains for biomedical event trigger extraction. BMC Genomics 22, 31. doi: 10.1186/s12864-020-07315-1
Chen, Y. L., Zhong, C. F., Xu, Y. N., Du, B., Ren, Y. H., and Li, P. (2020). Study on the quality difference of Dendrobium candidum in different regions. Food Ferment. Ind. 8, 123–130. doi: 10.13995/j.cnki.11-1802/ts.022986
Chen, Z., Li, B., Gao, X., Wang, D., Jin, S., Guo, L., et al. (2017). High-throughput analysis of endophytic bacterial communities in different parts of Dendrobium candidum. J. Henan Agric. Sci. 46, 104–108.+118.
China Food and Drug Administration. (2019). Announcement on the Release of “Health Food Raw Materials Catalogue (I)” and “Catalogue of Health Functions Allowed to be Claimed in Health Food (I). Available online at: http://www.samr.gov.cn/tssps/tzgg/zjwh/201903/t20190313_291950.html (accessed March 13, 2019).
China state Administration for Market Regulation. (2019). Health Food Raw Material Catalogue and Health Function Catalogue Management Measures. Available online at: http://www.lst.gov.cn/lst/spypjg/202012/4fc2a45309664b969a4dfd38b8e2d6fe.shtml
Choi, M. K., Taylor, K., Bettermann, A., and Van der Weide, D. W. (2002). Broadband 10-300 GHz stimulus-response sensing for chemical and biological entities. Phys. Med. Biol. 47, 3777–3787. doi: 10.1088/0031-9155/47/21/316
Chua, H. S., Upadhya, P. C., Haigh, A. D., Obradovic, J., Gibson, A. A., Linfield, E. H., et al. (2005). “Terahertz time-domain spectroscopy of crushed wheat grain,” in IEEE MTT-S International Microwave Symposium Digest (IEEE), 2103–2106. doi: 10.1109/MWSYM.2005.1517162
Cui, D., Mo, Y., Zeng, L., Feng, K., Feng, X., Huang, J., et al. (2019). Response of dendrobium officinale Kimura et Migo, a prized medicinal plant, to continuous UV-B irradiation at different C/N ratios. J. Plant Growth Regul. 39, 358–369. doi: 10.1007/s00344-019-09987-w
Deng, K. L. (2020). Biosynthesis and Activity Analysis of Silver Nanoparticles Mediated by endophytic fungi from Fritillaria thunbergii. Ya'an city: Sichuan Agricultural University.
Dhillon, S. S., Vitiello, M. S., Linfield, E. H., Davies, A. G., Hoffmann, M. C., Booske, J., et al. (2017). The 2017 terahertz science and technology roadmap. J. Phys. D Appl. Sci. 50, 043001. doi: 10.1088/1361-6463/50/4/043001
Dorney, T. D., Baraniuk, R. G., and Mittleman, D. M. (2001). Material parameter estimation with terahertz time-domain spectroscopy. J. Opt. Soc. Am. A: Opt. Image Sci. Vis. 18, 1562–1571. doi: 10.1364/JOSAA.18.001562
Du, X. Z. (1997). Simultaneous-guided room temperature phosphorescence spectra of 1-bromonaphthalene induced by linear fatty alcohols in the presence of β-cyclodextrin. Chem. J. Chin. Univ. 18, 1935–1938.
Edinburgh Instruments. (1997). What is a Jablonski Diagram (Perrin-Jablonski Diagram). Available online at: https://www.edinst.com/blog/jablonski-diagram/ (accessed April 05, 1997).
El Haddad, J., Bousquet, B., Canioni, L., and Mounaix, P. (2013). Review in terahertz spectral analysis. TrAC Trends Anal. Chem. 44, 98–105. doi: 10.1016/j.trac.2012.11.009
Food Safety Standards and Monitoring and Evaluation Division. (2020). Interpretation of the “on the Dangshen and Other Nine Substances in Accordance with the Traditional Both Food and Herbal Substances Management Pilot Notice”. Available online at: http://www.nhc.gov.cn/sps/s7886/202001/cd36fe79f7cb4313a99d9ef0772b37bf.shtml (accessed January 07, 2020).
Ge, Y. H., Wang, J., Zhou, C., Tong, Y. L., and Ren, Z. M. (2015). Determination of dendrobine and polysaccharides in Dendrobium candidum. Chin. J. Tradit. Med. Sci. Technol. 22, 527–528, 602.
General Office of the People's Government of Yunnan Province. (2019). Notice of the General Office of the Yunnan Province on Issuing the Action Plan for Quality Improvement in 9 Fields including Special Agricultural Products. Available online at: http://www.yn.gov.cn/zwgk/zfgb/2019/2019d13q/szfbgtwj/201907/t20190711_180841.html (accessed July 12, 2019).
General Office of the State Council. (2017). Notice of the General Office of the State Council on the issuance of the National Nutrition Plan (2017-2030). Available online at: http://www.gov.cn/zhengce/content/2017-07/13/content_5210134.htm (accessed June 30, 2017).
General Office of the State Council. (2021). The General Office of the State Council issued “Several Policies and Measures to Accelerate the Development of the Characteristics of Traditional Chinese Medicine”. Available online at: http://www.gov.cn/zhengce/content/2021-02/09/content_5586278.htm (accessed February 9, 2021).
Geng, L. X., Zheng, R., Reng, J., Niu, Z. T., Sun, Y. L., Xue, Q. Y., et al. (2015). Significance of a new combination fragment: nrDNA ITS+nad 1-intron2 in the identification of Fengdou Dendrobium. Acta Pharm. Sin. 50, 1060–1067.
Globus, T., Woolard, D. L., Khromova, T., Partasarathy, R., Majewski, A., Abreu, R., et al. (2004). Terahertz signatures of biological-warfare-agent simulants. Proc. SPIE Int. Soc. Opt. Eng. 5411, 25–32. doi: 10.1117/12.549128
Gong, Y., He, B. W., Wang, S. Z., and Mao, B. Z. (2017). Research progress on endophytes of Dendrobium candidum. J. Zhejiang Agric. Sci. 58, 1372–1375. doi: 10.16178/j.issn.0528-9017.20170825
Gu, Y. Q., Liu, R. T., Shou, G. Z., Zhang, W. Y., and Zhao, D. X. (2016). Using near infrared spectroscopy to quickly identify the producing area of Dendrobium candidum. Jiangsu Agric. Sci. 5, 365–368. doi: 10.15889/j.issn.1002-1302.2016.05.107
Hao, S. B., Huang, H. C., Ma, Y. Y., Tang, W. C., Gao, H., Gao, L., et al. (2018). “Characteristics of the Calamine analyzed by terahertz time-domain technology,” in Tenth International Conference on Information Optics and Photonics. doi: 10.1117/12.2504745
Hao, X., Zhang, G., and Ma, S. (2016). Deep learning. Int. J. Semant. Comput. 10, 417–439. doi: 10.1142/S1793351X16500045
He, K. N. (2017). Quality Research and Evaluation Model Establishment of Dendrobium Dendrobium of Different Productions. Guangzhou: South China Agricultural University.
He, Y., Wang, H. F., and Yan, X. P. (2008). Exploring Mn-doped ZnS quantum dots for the room-temperature phosphorescence detection of enoxacin in biological fluids. Anal. Chem. 80, 3832–3837. doi: 10.1021/ac800100y
Hu, T., Tang, L. M., Tang, Q., Zhang, H., Nie, L., Li, L., et al. (2014). Rapid identification of Dendrobium huoshanense species by miniature near- infrared spectroscopy. Spectrosc. Spectr. Anal.. 10, 2808–2814. doi: 10.3964/j.issn.1000-0593(2014)10-2808-07
Huang, Q., Shen, Y. X., Zhang, C. J., Luo, A. X., and Fan, Y. J. (2014). The content of polyphenols and flavonoids of Dendrobium candidum and their correlation with antioxidant activity. Chin. J. Appl. Environ. Biol. 20, 438–442. doi: 10.3724/sp.j.1145.2014.04028
Huang, Q. Q., Yang, Z. Q., Li, J. S., Li, J. J., Zheng, Q. T., and Ding, Y. H. (2019). Effect of water and nitrogen coupling on the dynamic characteristics of grape leaf rapid fluorescence induction. Chin. J. Agrometeorol. 40, 557–573.
IUPAC. (1997). Compendium of Chemical Terminology, 2nd ed. (the “Gold Book”); Compiled by A. D. McNaught and A. Wilkinson. Hoboken, NJ: Blackwell Scientific Publications.
Jia, Y. H., Li, D. R., and Sun, J. B. (2000). Multi-source remote sensing image data fusion. Remote Sens. Technol. Appl. 15, 4. doi: 10.3969/j.issn.1004-0323.2000.01.010
Jiang, B., Peng, P., Ni, X. Q., and Shen, X. G. (2019). Comparative study on sensory evaluation and polysaccharide content of different Dendrobium candidum cultivated strains. J. Changshu Inst. Technol. 2, 105−107.
Jiang, C., Lan, L., Yao, Y., Zhao, F., and Ping, J. (2018). Recent progress in application of nanomaterial-enabled biosensors for ochratoxin A detection. TrAC Trends Anal. Chem. 102, 236–249. doi: 10.1016/j.trac.2018.02.007
Jiang, M. Z., Han, X. B., Yan, H., Jia, J. Q., Tu, J., and Ma, Y. K. (2017). Fast and non-destructive identification of Dendrobium Huoshan and Dendrobium Henan by portable near-infrared spectrometer. Chem. Ind. For. Prod. 5, 101–106. doi: 10.3969/j.issn.0253-2417.2017.05.013
Jiang, X. L., He, P. F., Wang, Y. L., Guo, L. W., Wu, Y. X., Yang, J., et al. (2015). Fluorescence labeling of corn endophytic bacteria Y19 and its colonization in corn. J. Maize Sci. 23, 50–56.
Jin, Y., Johannissen, L. O., and Hay, S. (2021). Predicting new protein conformations from molecular dynamics simulation conformational landscapes and machine learning. Proteins Struct. Funct. Bioinform. 89, 915–921. doi: 10.1002/prot.26068
Johnson, A. E., Behar, J., Andreotti, F., Clifford, G. D., and Oster, J. (2015). Multimodal heart beat detection using signal quality indices. Physiol. Meas. 36, 1665. doi: 10.1088/0967-3334/36/8/1665
Ke, Y. K., and Dong, H. R. (1998). Analytical Chemistry Handbook: Volume III Spectral Analysis [M]. Chemical Industry Press.
Keller, B., Vass, I., Matsubara, S., Paul, K., Jedmowski, C., Pieruschka, R., et al. (2019). Maximum fluorescence and electron transport kinetics determined by light- induced fluorescence transients (LIFT) for photosynthesis phenotyping. Photosyn. Res. 140, 221–233. doi: 10.1007/s11120-018-0594-9
Kong, D. R. (2019). Study on a New Electrochemical Sensing Method for Gene Identification and Heavy Metal Detection of Traditional Chinese Medicine, Jiangxi University of Traditional Chinese Medicine. Nanchang.
Lan, F., Li, L. L., Wei, X. Y., Huang, R. S., and Feng, P. (2017). Comparison of the morphological characteristics of three kinds of Dendrobium officinale and the quality of its medicinal materials. West China J. Pharm. Sci. 32, 615–617. doi: 10.13375/j.cnki.wcjps.2017.06.017
Li, C. H., and Li, K. C. (2017). Propagation and greenhouse production of Dendrobium. China Flowers Horticult. 16, 27−31.
Li, J., Ying, S., Ren, H., Dai, J., Zhang, L., Liang, L., et al. (2020). Molecular dynamics study on the encapsulation and release of anti-cancer drug doxorubicin by chitosan. Int. J. Pharm. 580, 119241. doi: 10.1016/j.ijpharm.2020.119241
Li, Y. G., and Liu, J. C. (2001). Molecular simulation and chemical engineering. Mod. Chem. Ind. 21, 10–13. doi: 10.16606/j.cnki.issn0253-4320.2001.07.003
Lin, P., He, J. Q., Zhou, Z. Y., and Chen, Y. M. (2019). Judgment method of soybean appearance quality based on visible spectrum. Acta Opt. Sin. 39, 200–207. doi: 10.3788/AOS201939.0815002
Liu, R. T. (2015). Research and Application of Rapid Identification of Dendrobium Plants based on Near-infrared Spectroscopy. Hangzhou: Zhejiang AandF University.
Liu, W., Zhang, C., Yu, B., and Li, Y. (2019). “A general multi-source data fusuion framework, in International Conference on Machine learning and Computing, 285–289. doi: 10.1145/3318299.3318394
Liu, W. -H. (2017). Isolation of endophytic fungi from Dendrobium officinale and preliminary evaluation of in vitro biological activity. Chin. Herb. Med. 24, 533–538. doi: 10.7501/j.issn.0253-2670.2017.03.018
Liu, X. Y. (2020). Screening of Type II 5α-reductase inhibitors from traditional chinese medicine based on molecular docking and molecular dynamics simulation. Chin. Tradit. Herbal Drugs 51, 232–240.
Liu, Y. L., Feng, S. G., He, R. F., Zhao, M. Y., Wu, H., Cui, Y. Q., et al. (2014). A preliminary study on molecular identification of Dendrobium officinale and its fakes based on ITS2 barcode. J. Hangzhou Normal Univ. 13, 35−41. doi: 10.3969/j.issn.1674-232X.2014.01.007
Liu, Z., Liu, H., Huang, W., Wang, B., and Sun, F. (2020). Audiovisual cross-modal material surface retrieval. Neural Comput. Appl. 32, 14301–14309. doi: 10.11992/tis.201804030
Lu, J. Y., Piprek, J., Chen, L. J., Kao, T. F., Chang, H. H., and Liu, A. S. (2005). Optoelectronic Devices: Physics, Fabrication, and Application Ii - Terahertz Biochip Based on Optoelectronic Devices. Boston, MA: SPIE. doi: 10.1117/12.630033
Lu, Q. F. (2014). Quality Evaluation of Dendrobium officinale and Research on Drying Process. Guangzhou: Guangzhou University of Chinese Medicine.
Lu, Y. B. (2012). Research on X-ray CT Imaging Technology and Multi-modal Tomography Technology. Beijing: Peking University.
Luo, X. F. (2018). Evaluation of Texture and Active Ingredients of Different Varieties of Dendrobium and Their Correlation. Guangzhou: South China Agricultural University.
Lv, C. G., Yang, J., Kang, C. Z., Li, Z. H., Ma, Z. H., Guo, L. P., et al. (2017). Determination of 10 flavonoids in Dendrobium candidum by UPLC-MS/MS and analysis of polysaccharide composition conten. Chin. J. Exp. Tradit. Med. 55−60. doi: 10.13422/j.cnki.syfjx.2017170047
Lv, F., Zhang, J., Chen, X. L., Liu, J. H., and Ding, Y. T. (2019). Spectroscopy combined with molecular dynamics simulation to study the mechanism of action of ozone on the structure of myoglobin. Spectrosc. Spectr. Anal. 39, 3115–3121.
Ma, X. F., Liu, L. P., and Deng, Z. N. (2017). Real-time observation of canker bacteria infecting citrus leaves using laser confocal scanning microscope. Acta Laser Biol. Sin. 26, 143–150. doi: 10.3969/j.issn.1007-7146.2017.02.009
Marques da Silva, J., Figueiredo, A., Cunha, J., Eiras-Dias, J. E., Silva, S., Vanneschi, L., et al. (2020). Using rapid chlorophyll fluorescence transients to classify vitis genotypes. Plants 9, 174. doi: 10.3390/plants9020174
Meo, S. A., Al Drees, A. M., Zadi, S. Z., Damgh, S. A., and Al-Tuwaijri, A. S. (2006). Hazards of X-ray radiation on the quantitative and phagocytic functions of polymorphonuclear neutrophils in X-ray technicians. J. Occup. Health 48, 88–92. doi: 10.1539/joh.48.88
Milella, A., Reina, G., and Nielsen, M. (2019). A multi-sensor robotic platform for ground mapping and estimation beyond the visible spectrum. Precis. Agri. 20, 423–444. doi: 10.1007/s11119-018-9605-2
Mittleman, D. M. (2017). Perspective: Terahertz science and technology. J. Appl. Phys. 122, 230901. doi: 10.1063/1.5007683
Ngiam, J., Khosla, A., Kim, M., Nam, J., Lee, H., Ng, A. Y., et al. (2011). “Multimodal deep learning,” in Proceedings of International Conference on Machine Learning, Washington, 689–696.
Ouyang, F. P., Xu, H, and Guo, A. M. (2005). Molecular simulation method and its application in molecular biology. Chin. J. Bioinform. 3, 33–36. doi: 10.3969/j.issn.1672-5565.2005.01.009
Pharmacopoeia Commission of the People's Republic of China. (2010). Acopoeia of the People's Republic of China. Beijing: China Medical Science Press, 245–266.
Pimentel, M. A., Santos, M. D., Springer, D. B., and Clifford, G. D. (2015). Heart beat detection in multimodal physiological data using a hidden semi-Markov model and signal quality indices. Physiol. Meas. 36, 1717. doi: 10.1088/0967-3334/36/8/1717
Pliński, E. F., and Plińska, S. (2012). Sensing with terahertz radiation of pharma-and bio-materials. Proc. Eng. 47, 929–932. doi: 10.1016/j.proeng.2012.09.298
Qiao, S. C., Jiang, J. Q., He, K., Song, S. Y., and Tian, Y. W. (2019). Research progress of non-destructive testing technology for quality of chinese medicinal materials. J. Chin. Med. Mater. 42, 2468–2472. doi: 10.13863/j.issn1001-4454.2019.10.050
Qu, F., Lin, L., Cai, C., Dong, T., He, Y., Nie, P., et al. (2018). Molecular characterization and theoretical calculation of plant growth regulators based on terahertz time-domain spectroscopy. Appl. Sci. 8, 420. doi: 10.3390/app8030420
Rathee, K., Dhull, V., Dhull, R., and Singh, S. (2016). Biosensors based on electrochemical lactate detection: a comprehensive review. Biochem. Biophy. Rep. 5, 35–54. doi: 10.1016/j.bbrep.2015.11.010
Robert FitzRoy. (2010). World Meteorological Organization 60th Anniversary. Available online at: https://blog.metservice.com/node/903 (accessed March 22, 2010).
Rojas-Duran, T. R., Fente, C. A., Vazquez, B. I., Franco, C. M., Sanz-Medel, A., Cepeda, A., et al. (2006). Study of a room temperature phosphorescence phenomenon to allow the detection of aflatoxigenic strains in culture media. Int. J. Food Microbiol. 115, 149–158. doi: 10.1016/j.ijfoodmicro.2006.10.018
Scheller, M., Jordens, C., Breitenstein, B., Selmar, D., and Koch, M. (2008). “Effective permittivity and scattering model for the evaluation of the leaf water status,” in 2008 33rd International Conference on Infrared, Millimeter and Terahertz Waves (IEEE), 1–2. doi: 10.1109/ICIMW.2008.4665826
Schulte, R., Krug, J., and Rose, G. (2014). Identification of a signal for an optimal heart beat detection in multimodal physiological datasets. Comput. Cardiol. IEEE 1–4, 273–276. Available online at: https://ieeexplore.ieee.org/document/7043032
Shan, W., Jin, X. B., Meng, X. S., Yang, X. Y., Xu, Z. G., Gu, Z. M., et al. (2019). Dynamic monitoring of ecological environment quality of land rehabilitation based on multi-source remote sensing data. Trans. Chin. Soc. Agric. Eng. 35, 234–242. doi: 10.11975/j.issn.1002-6819.2019.01.029
Shi, Y. W., Chu, M., Yang, H. M., Gao, Y., Zhang, T., Zeng, J., et al. (2018). Fluorescence quantitative detection and time-space dynamic analysis of endophytic fungi from cotton verticillium wilt in Xinjiang. Microbiol. China 45, 376–385. doi: 10.13344/j.microbiol.china.170275
Shi, Z. R., Zhang, Y. B., and Tong, Y. (2006). “Application of 5S rDNA gene microarray technology to identify Chinese medicinal material Fengdou Shihu (English),” in The 3rd International Conference on Chinese Medicine Engineering. p. 83–89. Available online at: http://cpfd.cnki.com.cn/Article/CPFDTOTAL-OGSR200612001018.htm
Snoek, C. G. M., and Worring, M. (2005). Multimodal video indexing: a review of the state-of-the-art. Multimed. Tools Appl. 25, 5–35. doi: 10.1023/B:MTAP.0000046380.27575.a5
State Administration for Market Regulation. (2021). Health Food Record Management to Promote the Healthy Development of the Industry Review. Available online at: http://www.samr.gov.cn/tssps/sjdt/gzdt/202103/t20210317_326996.html (accssed March 17, 2021).
Stirbet, A., Lazár, D., and Kromdijk, J. (2018). Chlorophyll a fluorescence induction: can just a one-second measurement be used to quantify abiotic stress responses. Photosynthetica 56, 86–104. doi: 10.1007/s11099-018-0770-3
Sun, Q., Wang, S., Zhao, C., Leng, J., Tian, W., Jin, S., et al. (2019). Excitation-dependent emission color tuning from an individual Mn-doped perovskite microcrystal. J. Am. Chem. Soc. 141, 20089–20096. doi: 10.1021/jacs.9b09143
Tang, L., Li, J., Long, H., and Li, G. (2015). Differences of total polysaccharides, total alkaloids and total flavonoids in stems and leaves of Dendrobium candidum at different growth ages. Guangdong Agric. Sci. 42, 17–21. doi: 10.16768/j.issn.1004-874x.2015.08.019
Tang, Q. (2014). Near-infrared Spectroscopy Analysis Technology to Quickly Identify the Modeling Research Class of Dendrobium huoshanense. Jinan: Shandong University.
Tang, X. P., Liu, S. L., Guo, X. H., Weng, L. J., and Qu, D. (2020). Research progress of near infrared spectroscopy in tea quality analysis. Guangxi J. Light Ind. 36, 258.
Traviesa-Alvarez, J. M., Costa-Fernández, J. M., Pereiro, R., and Sanz-Medel, A. (2007). Direct screening of tetracyclines in water and bovine milk using room temperature phosphorescence detection. Anal. Chim. Acta 589, 51–58. doi: 10.1016/j.aca.2007.02.063
Villar, A., Gorritxategi, E., Aranzabe, E., Fernández, S., Otaduy, D., Fernández, L. A., et al. (2012). Low-cost visible–near infrared sensor for on-line monitoring of fat and fatty acids content during the manufacturing process of the milk. Food Chem. 135, 2756–2760. doi: 10.1016/j.foodchem.2012.07.074
Wang, F., and Shi, H. Q. (2019). Research on the development of Dendrobium officinale Industry in my country. China For. Econ. 88–90, 135.
Wang, H. (2012). Study on the Biological Characteristics of Dendrobium candidum. Beijing: University of Chinese Academy of Sciences.
Wang, H., Luo, G. P., Wang, W. S., Pachikin, K., Li, Y. M., Zheng, H. W., et al. (2019). Retrieval of farmland soil moisture in the middle and lower reaches of the xier river based on multi-source remote sensing data. J. Nat. Resour. 34, 229–243. doi: 10.31497/zrzyxb.20191218
Wang, H. Y., and Li, M. (2014). Research progress on the medicinal functions of Dendrobium polysaccharide. Glob. Chin. Med. 7, 817–820. doi: 10.3969/j.issn.1674-1749.2014.10.030
Wang, L. X., Liu, M. Z., Wang, F., and Zhang, H. (2019). Study on extraction and antioxidant activity of polysaccharides from Dendrobium candidum. China Food Addit. 2, 85–90. doi: 10.3969/j.issn.1006-2513.2019.02.006
Wang, S. P., Sun, Y. M, Jiang, Y, Xia, Z. Y., and Wang, Z. A. (2015). Identification of Dendrobium officinale and counterfeit products based on fingerprint and naringenin content. Acta Agric. Zhejiangensis 27, 2199–2205. doi: 10.3969/j.issn.1004-1524.2015.12.24
Wang, X. Q., He, X. Q., and Zeng, H. T. (2021). Preliminary study on the classification standard of fresh JiangXi Dendrobium. Pract. Clin. J. Integr. Tradit. Chin. West. Med. 21, 154–156.
Wei, X. H., You, J. K., and Wand, D. (2021). Electrochemical biosensor for rapid detection of AFB1 in Chinese herbal medicine. Chin. J. Anal. Lab. 40, 130–134. doi: 10.13595/j.cnki.issn1000-0720.2020.082601
Weinberger, R., Yarmchuk, P., and Love, L. J. C. (1982). Liquid chromatographic phosphorescence detection with micellar chromatography and postcolumn reaction modes. Anal. Chem. 54, 1552–1558. doi: 10.1021/ac00246a022
Wishart, D. S. (2019). NMR metabolomics: a look ahead. J. Magn. Reson. 306, 155–161. doi: 10.1016/j.jmr.2019.07.013
Wu, H. (2009). Study on the Fluorescence Spectra Characteristics of Probiotics. Wuxi: Jiangnan University.
Wu, J. W., Bao, J. L., Lv, Y. P., Zhong, X. L., Xiang, L., and Xu, A. L. (2011). Determination of total flavonoids of Dendrobium candidum in Yueqing City. J. Anhui Agric. Sci. 39, 10856–10884. doi: 10.3969/j.issn.0517-6611.2011.18.050
Wyber, R., Osmond, B., Ashcroft, M. B., Malenovsk,ý, Z., and Robinson, S. A. (2018). Remote monitoring of dynamic canopy photosynthesis with high time resolution light-induced fluorescence transients. Tree Physiol. 38, 1302–1318. doi: 10.1093/treephys/tpx161
Xin, T. (2011). Quality Evaluation of Tissue Cultured Dendrobium candidum. Shenyang: Liaoning University Of Traditional Chinese Medicine.
Xinhua News Agency. (2019). Release of 2019 No. 1 Central Document. Available online at: https://baijiahao.baidu.com/s?id=1625902536484630675&wfr=spider&for=pc (accessed January 03, 2019).
Xinhua News Agency. (2020). Anhui Province Promotes the Construction of “Top Ten Anhui Medicines” Industrial Demonstration Bases. Available online at: http://www.ah.xinhuanet.com/2020-10/19/c_1126630341.htm (accessed February 20, 2019).
Xu, H. J. (2011). Study on the Identification of Dendrobium officinale Based on Real-time PCR and ARMS Technology. Nanjing: Nanjing Normal University.
Xu, H. L., Zhou, X., Zheng, W. R., Li, T. T., He, Z. F., Zhan, F. Y., et al. (2018). Comparison of multiple quality indexes of different imitated wild cultivated Dendrobium candidum. J. Zhejiang Agric. Sci. 59, 1253–1257.
Xu, X. S., Zhang, Y. C, Liu, Q. M., Dong, H. P., and Wang, X. F. (2002). Study on the autofluorescence phenomenon of fungi. Acta Laser Biol. Sin. 11, 32–36. doi: 10.3969/j.issn.1007-7146.2002.02.006
Xu, Y. P. (2015). Research progress on endophytic fungi of Dendrobium candidum. J. Microbiol. 35, 108–112. doi: 10.3969/j.issn.1005-7021.2015.05.020
Xu, W. T, and Zhu, R. H. (2009). Simultaneous-derivative solid-substrate room temperature phosphorescence spectrometry for rapid and simultaneous analysis of a-naphthaleneacetic acid and 6-benzylaminopurine. Chin. J. Anal. Lab. 3, 99–102. doi: 10.3969/j.issn.1000-0720.2009.03.026
Yan, Z. G., Hou, D. B., Cao, B. H., Zhang, G. X., and Zhou, Z. K. (2008). Terahertz spectroscopic investigation of riboflavin and nicotinic acid. J. Infrared Millim. Waves 27, 326–329. doi: 10.3969/j.issn.1004-1699.2009.03.004
Yang, L. Y., Yang, Z., Cai, Y. M., Zhang, Y. C., and Wang, Y. C. (2018). Application of SRAP molecular markers in identification of progeny varieties of Dendrobium candidum. Mol. Plant Breed. 2018, 5690–5695. doi: 10.13271/j.mpb.016.005690
Yin, H. M. (2014). Study on quality control of Dendrobium officinale. Zhejiang University of Technology. doi: 10.27463/d.cnki.gzgyu.2014.000081
Yu, H., Liang, Z. T., and Yan, Y. C. (2020). Review on multi-source and multi-modal data fusion and integration. Inf. Stud. Theory Appl. 43, 169–178. doi: 10.16353/j.cnki.1000-7490.2020.11.027
Yu, M. M., Wu, L. S., Si, J. P., Liu, J. J., Yu, Z. X., Wang, H. C., et al. (2016). Screening of superior endophytic fungi of Dendrobium candidum based on polysaccharides and flavonoids. China J. Chin. Mater. Med. 41, 2208–2212. doi: 10.4268/cjcmm20161206
Yu, Q., Zhong, P. P., and Wang, Y. X. (2018). Progress in adulteration identification of edible vegetable oil. China Oils Fats 043, 81–84, 111. doi: 10.3969/j.issn.1003-7969.2018.06.018
Yu, Y. (2010). Correspondence Analysis of Arbuscular Mycorrhiza Formation and Camptothecin Content in Tree Seedlings. Harbin: Northeast Forestry University.
Yuan, J. L., Ma, C., Feng, Y. L., Zhang, J., Yang, F. Q., and Li, Y. J. (2018). Response of the kinetic curve of rapid chlorophyll fluorescence induction in different drought resistance wheat to drought and rewatering. Plant Physiol. J. 54, 1119–1129.
Yuan, N. J., Zheng, Y., Xie, X., Wang, Y., Zheng, K., Xiong, H., et al. (2015). Discovering urban functional zones using latent activity trajectories. IEEE Trans. Knowl. Data Eng. 27, 712–725. doi: 10.1109/TKDE.2014.2345405
Zhang, B., Kang, Z., Zhang, J., Kang, Y., Liang, L., Liu, Y., et al. (2021). Simultaneous binding mechanism of multiple substrates for multidrug resistance transporter P-glycoprotein. Phys. Chem. Chem. Phys. 23, 4530–4543. doi: 10.1039/D0CP05910B
Zhang, H., Cheng, C. Q., Xu, Z. G., and Li, J. J. H. (2020). A survey of research on data fusion methods based on deep learning. Comput. Eng. Appl. 56, 7–17. doi: 10.3778/j.issn.1002-8331.2007-0475
Zhang, J. F., He, S. M., and Xie, Q. (2018). Quality analysis and evaluation index determination of Dendrobium. Nat. Prod. Res. Dev. 12, 30–38. doi: 10.16333/j.1001-6880.2018.6.012
Zhang, Y. Q., Zheng, Z. G., Huang, J. M., Zhou, Z. M., Luo, D. H., and Tang, L. (2003). Phosphorescence spectroscopy analysis of SrAl2O4, Eu2.+, Dy3+ and its room temperature phosphorescence. Chin. J. Inorg. Chem. 19, 1129–1132. doi: 10.3321/j.issn:1001-4861.2003.10.019
Zhang, Z., Xu, W., Shi, Z., and Qin, Q. (2021). Establishment of a comprehensive drought monitoring index based on multisource remote sensing data and agricultural drought monitoring. IEEE J. Sel. Top. Appl. Earth Obs. Remote Sens. 14, 2113–2126. doi: 10.1109/JSTARS.2021.3052194
Zhao, J. H., Zhang, C. Y., Min, L., Li, N., and Wang, Y. L. (2021). Retrieval for soil moisture in farmland using multi-source remote sensing data and feature selection with GA-BP neural network. Nongye Gongcheng Xuebao/Trans. Chin. Soc. Agric. Eng. 37, 112–120. doi: 10.11975/j.issn.1002-6819.2021.11.013
Zhejiang Provincial Development and Reform Commission. (2021). Provincial Development and Reform Commission, Provincial Department of Economy and Information, Provincial Department of Agriculture and Rural Affairs, Provincial Health and Health Commission, Provincial Administration of Traditional Chinese Medicine Notice on Printing and Distributing the “Fourteenth Five-Year Plan for the Development of Traditional Chinese Medicine in Zhejiang Province”. Available online at: http://www.zj.gov.cn/art/2021/6/30/art_1229203592_2307194.html (accessed June 30, 2021).
Zhong, C. F., Chen, Y. L., Ren, Y. H., Li, P., Cha, Y. H., and Du, B. (2020). Comparative study on quality of different Dendrobium candidum Hongxin varieties. Sci. Technol. Food Ind. 452, 47–52.+61. doi: 10.13386/j.issn1002-0306.2020.12.007
Keywords: Dendrobium candidum, quality factors, molecular dynamics, multimodal, rapid detection
Citation: Chen F, Chen Z, Sun H, Zhu J, Wu K, Zhou S and Huang Y (2022) Dendrobium candidum quality detection in both food and medicine agricultural product: Policy, status, and prospective. Front. Sustain. Food Syst. 6:1042901. doi: 10.3389/fsufs.2022.1042901
Received: 13 September 2022; Accepted: 24 October 2022;
Published: 05 December 2022.
Edited by:
Chu Zhang, Huzhou University, ChinaReviewed by:
Kang Huang, The University of Auckland, New ZealandSeyed-Hassan Miraei Ashtiani, Ferdowsi University of Mashhad, Iran
Copyright © 2022 Chen, Chen, Sun, Zhu, Wu, Zhou and Huang. This is an open-access article distributed under the terms of the Creative Commons Attribution License (CC BY). The use, distribution or reproduction in other forums is permitted, provided the original author(s) and the copyright owner(s) are credited and that the original publication in this journal is cited, in accordance with accepted academic practice. No use, distribution or reproduction is permitted which does not comply with these terms.
*Correspondence: Yun Huang, 25154994@qq.com