- 1State Key Laboratory for Managing Biotic and Chemical Treats to the Quality and Safety of Agroproducts, Key Laboratory of Biotechnology in Plant Protection, Ministry of Agriculture and Rural Affairs, Institute of Plant Protection and Microbiology, Zhejiang Academy of Agricultural Sciences, Hangzhou, China
- 2State Key Laboratory of Rice Biology, Ministry of Agriculture Key Lab of Molecular Biology of Crop Pathogens and Insects, Institute of Insect Sciences, Zhejiang University, Hangzhou, China
- 3Key Laboratory of Natural Enemy Insects, Ministry of Agriculture and Rural Affairs, Institute of Plant Protection, Chinese Academy of Agricultural Sciences, Beijing, China
Introduction: Orius spp. are generalist predators released in horticultural and agricultural systems to control thrips. Understanding the effects of temperature on the development, predation rate, and population dynamics of Orius is essential for identifying the optimal timing of Orius release for establishing an adequate population to facilitate synchrony with thrips population growth and to prevent thrips outbreaks. The biological control efficiency of natural enemies as well as predator–prey relationships can be precisely described by integrating life table parameters and the predation rate.
Methods: In this study, the demographic features of Orius strigicollis fed on 2nd instar nymphs of western flower thrips (WFT), Frankliniella occidentalis, were compared at 18.5, 23.5, 27, and 33°C using the TWOSEX-MSChart program. The CONSUME-MSChart program was used to examine predation rates under different temperatures (18.5, 23.5, and 27°C).
Results: The results showed no significant difference in fecundity among those reared at 18.5, 23.5, and 27°C, but fecundity at these temperatures was significantly higher than that at 33°C. The intrinsic rate of increase (r), finite rate of increase (λ), and net reproduction rate (R0) were the highest at 27°C. The net predation rate (C0) and transformation rate (Qp) were significantly higher at 18.5°C (C0 = 168.39 prey/predator, Qp = 8.22) and 23.5°C (C0 = 140.49 prey/predator, Qp = 6.03) than at 27°C (C0 = 138.39 prey/predator, Qp= 3.81); however, the finite predation rate (ω) showed the opposite trend. In addition to temperature, the stage of O. strigicollis at release can affect population dynamics.
Discussion: Our study showed that temperature influenced the demographic traits and predation rates of O. strigicollis. When planning a release, the stage of O. strigicollis and temperature should be taken into account to establish an adequate population for the control of WFT.
Introduction
The western flower thrips (WFT), Frankliniella occidentalis Pergrande, is a notorious agricultural and horticultural insect pest worldwide; this species harms plants directly through feeding and oviposition and indirectly through the transmission of plant viruses (Reitz et al., 2011, 2020; Gao et al., 2012; Mouden et al., 2017; He et al., 2020; Wu et al., 2021). Damage by WFT may reduce crop quantity and quality, resulting in severe economic losses for farmers (He et al., 2020; Reitz et al., 2020; Avellaneda et al., 2021). The WFT is a typical r-strategist insect pest with a small size, cryptic behavior, and a rapid reproduction rate, and these characteristics make WFT develop resistance to chemical pesticides (Reitz et al., 2011, 2020; Gao et al., 2012; Wu et al., 2021). Moreover, long-term and inappropriate use of chemical pesticides often results in food safety problems, pesticide resistance, and negative impacts on non-target species (Bielza, 2008; Gao et al., 2012; Mouden et al., 2017). Ecology-based methods to reduce insect pest injury in agricultural systems are essential to achieve integrated pest management (Lewis et al., 1997; Demirozer et al., 2012; Mouden et al., 2017). Hence, alternative measures to reduce reliance on chemical insecticides, such as the release of biological control agents, have become important (Mouden et al., 2017; Reitz et al., 2020; Wu et al., 2021).
As biological control agents of key pests that infest cultivated crops, insect predators and parasitoids provide valuable ecosystem services (Landis et al., 2000; Lu et al., 2012; Zhao et al., 2017). The periodic release of natural enemies, also known as augmentative biological control (ABC), is commonly used in greenhouse biological control programs (Messelink et al., 2014; van Lenteren et al., 2018). Minute pirate bugs, Orius spp., are useful natural enemies that prey on small and soft-bodied insect pests of agricultural and horticultural crops (De Clercq et al., 2014; Bonte et al., 2017; Zhao et al., 2017; Peterson et al., 2018). Feeding on non-prey food, such as pollen, allows Orius to survive when prey are scarce or absent, promoting sustainable insect pest control (Hinds and Barbercheck, 2020; Mendoza et al., 2021, 2022). Accordingly, these generalist predators are preferred in ABC programs and are now mass-reared for pest control purposes, particularly thrips control (Mouden et al., 2017; van Lenteren et al., 2018; Hinds and Barbercheck, 2020; Reitz et al., 2020; Mendoza et al., 2021). Indeed, releasing O. insidiosus at a 1:40 WFT ratio can nearly eliminate a WFT population within days (Funderburk et al., 2000). Thus, a comprehensive understanding of the characteristics of Orius is crucial for the development and promotion of this natural enemy as a method of thrips control.
Temperature is an important environmental factor that influences both the biological and ecological characteristics of natural enemies (Wallner, 1987; Sørensen et al., 2013; Helgadóttir et al., 2017) as well as the effectiveness of biological control (Montserrat et al., 2013; Boukal et al., 2019; Bai et al., 2022). In mass-rearing programs, natural enemies are reared indoors at an optimum and constant temperature to ensure rapid and efficient production, but these conditions considerably differ from those of crop systems in the field or greenhouse (Montserrat et al., 2013; Sørensen et al., 2013; Helgadóttir et al., 2017), in which temperatures change with seasonal oscillations and diel activity rhythms. As the temperature has a direct effect on the survival, development, and reproduction of natural enemies (Helgadóttir et al., 2017), population dynamics will be affected by temperature fluctuations (Barton and Schmitz, 2009; Montserrat et al., 2013). Thus, the development, predatory ability, and population dynamics of natural enemies in response to various temperatures must be systematically assessed; these results will facilitate predictions of the ecological effects of climate change on predator-prey interactions (Boukal et al., 2019).
Numerous studies have assessed the influence of temperature on the development, fecundity, and functional response of Orius species (Nagai and Yano, 1999; Ohta, 2001; Baniameri et al., 2005; Ballal et al., 2017). Experimental studies on the integrated life table traits and predatory rates of Orius species in response to temperature can precisely elucidate the biological control efficiency of Orius and predator-prey relationships (Ding et al., 2021). Orius strigicollis is a major natural enemy of pests among agroecosystems in southern China, Korea, and Japan (Musolin et al., 2004; Cho et al., 2005; Musolin and Ito, 2008; Ding et al., 2021) that has been mass-reared for thrips control (van Lenteren, 2012; Tuan et al., 2016; van Lenteren et al., 2018). Releasing this biological control agent and establishing an adequate population promotes synchronization with thrips population growth and prevents thrips outbreaks. In this study, to fully understand the effect of temperature on the population dynamics and development of O. strigicollis, the demographic characteristics (including development, reproduction, survival, and longevity) of O. strigicollis reared at constant temperatures of 18.5–33°C and fed 2nd instar WFT nymphs were quantitatively described using the life table analysis program (TWOSEX-MSChart, Chi, 2022a), which can precisely describe stage differentiation and correctly evaluate the fitness of populations (Chi et al., 2020). The predation rates of O. strigicollis fed WFT under different temperature conditions (18.5–27°C) were also evaluated with the CONSUME-MSChart computer program (Chi, 2022b). Additionally, the population growth of O. strigicollis under different temperature conditions was projected using the TIMING-MSChart program (Chi, 2022c).
Materials and methods
Insects
Western flower thrips were collected from Cucumis melo L. grown in the greenhouse at the Institute of Vegetables and Flowers, Chinese Academy of Agricultural Sciences (39.53 °N, 116.70 °E) in Beijing, China, in 2007 and reared on bean pods (Phaseolus vulgaris L.) under laboratory conditions at 26±1°C, with relative humidity (RH) of 60 ± 10% and an L14:D10 photoperiod. Orius strigicollis were collected from thrips-damaged pepper (Capsicum annuum L.) plants grown in the greenhouse at the Yangdu Experiment Station of Zhejiang Academy of Agricultural Sciences (30.53 °N, 120.68 °E) in Jiaxing, Zhejiang Province, China, in 2016 and reared on WFT and bean pods, which were used as a water source and oviposition substrate for O. strigicollis. To avoid the negative effects of inbreeding, both WFT and O. strigicollis were collected every year from the field to propagate with the laboratory colony.
Life table and predation rate of O. strigicollis under various temperature conditions
Orius strigicollis eggs oviposited for 24 h on bean pods were selected and randomly separated into four groups, with 82, 98, 78, and 104 eggs per group. The egg groups were maintained at 18.5, 23.5, 27, and 33°C, respectively, with 60 ± 10% RH and an L16:D8 photoperiod. Every 24 h, the eggs were checked, and for viable eggs, the demographic traits were accurately determined (Mou et al., 2015; Ding et al., 2021). In total, 74 (18.5°C), 88 (23.5°C), 71 (27°C), and 89 (33°C) eggs were used as the initial samples. Newly hatched nymphs were individually transferred to Petri dishes (6 cm in diameter) with a fine brush. Two holes (1 cm in diameter) covered with a fine-mesh screen in the lid of the Petri dish allowed for ventilation. A piece of fresh bean pod (2–3 cm in length) was provided as a water source, and different numbers of 2nd instar WFT were provided as food for O. strigicollis nymphs (Ding et al., 2021). To prevent the escape of the WFT from the Petri dish, each dish was sealed with Parafilm. Every 24 h, the developmental stage of the nymphs was observed, and fresh bean pods and WFT were replaced in the Petri dish. Since predatory ability varies with developmental stage in O. strigicollis, different numbers of WFT were provided daily: 10 WFT for 1st (N1) and 2nd instar (N2), 15 WFT for 3rd (N3) and 4th instar (N4), and 20 WFT for 5th instar (N5) O. strigicollis every day (Ding et al., 2021). The number of WFT provided for O. strigicollis every day under various temperature conditions was sufficient. The numbers of WFT consumed by O. strigicollis reared at 18.5–27°C were recorded every 24 h.
When the adults emerged, sex was determined. Females and males were paired, and each adult pair was moved into a Petri dish (9 cm in diameter) with a 3 cm-diameter hole on the lid covered with a fine-mesh screen. A fresh bean pod (~6 cm in length) was used as the oviposition substrate, and a sufficient number of thrips (30 WFT) were provided as prey. Fecundity, longevity, and survival were recorded every 24 h, and fresh bean pods and WFT were provided daily. If the number of females exceeded that of males or a paired male died, additional males of the same age from the laboratory colony were paired with the females, but the longevity and the number of consumed WFT were not recorded. If the number of males exceeded that of females or a paired female died, additional females of the same age from the laboratory colony were paired with the males, but female fecundity, longevity, and the number of consumed WFT were not recorded (Ding et al., 2021). The numbers of WFT consumed by adult O. strigicollis reared at 18.5–27°C were recorded, and a male-to-female ratio of 1:2 was used to determine the consumption rate of paired adults (Ding et al., 2021).
Data analysis
Life table analysis
The demographic characteristics of O. strigicollis fed on WFT in response to temperature were analyzed using the computer program TWOSEX-MSChart (Chi, 2022a) based on the age-stage, two-sex life table theory (Chi and Liu, 1985; Chi, 1988; Chi et al., 2020). The age-stage-specific survival rate (sxj) (where x indicates age and j indicates stage), age-specific survival rate (lx), age-specific fecundity (mx), net maternity (lxmx), age-stage-specific fecundity (fxj), intrinsic rate of increase (r), net reproduction rate (R0), finite rate of increase (λ), and mean generation time (T) were calculated according to Chi and Liu (1985). The age-stage life expectancy (exj) was calculated according to Chi and Su (2006), and the age-stage reproductive value (vxj) was calculated according to Tuan et al. (2014a,b). R0 was calculated as follows:
The interactive bisection method and the Euler-Lotka equation (Goodman, 1982) were used to estimate r:
λ and T were calculated as follows:
The variances and standard errors of all of the parameters were estimated with 100,000 resamplings in the TWOSEX-MSChart program. Significant differences in the parameters under different temperature conditions were determined using the paired bootstrap test based on the 95% confidence intervals.
Predation rate analysis
The predation rates of O. strigicollis at different temperatures (18.5, 23.5, and 27°C) were calculated according to Chi and Yang (2003) using the CONSUME-MSChart computer program (Chi, 2022b). The age-specific predation rate (kx, the number of prey consumed by the surviving O. strigicollis at age x) was calculated as follows:
where cxj is the number of 2nd instar nymphs of WFT consumed by O. strigicollis at age x and stage j, which was calculated as follows:
where dxj,i is the number of WFT consumed by the ith O. strigicollis at age x and stage j, and nxj is the number of O. strigicollis surviving to age x and stage j. The age-specific net predation rate (qx) was calculated as follows:
The cumulative net predation rate (Cx) (the number of prey consumed per predator from birth to age x) and the net predation rate (C0) (the total number of prey consumed per predator over its lifetime) were calculated as follows:
The transformation rate (Qp) indicates the number of prey consumed to produce one offspring and was calculated as follows:
Following Ding et al. (2021), the predation rate of each predator in stage j (Pj) was calculated as follows:
where pij is the number of prey killed by predator i in stage j and nj is the number of predators that survived in stage j.
The daily predation rate per predator in stage j (Dj) (Ding et al., 2021) was calculated as follows:
where a and b indicate the first and last ages of stage j, respectively. The finite predation rate (ω) (Yu J. K., et al., 2013) was calculated as follows:
where axj indicates the proportion of individuals at age x and stage j.
Significant differences in the parameters under different temperature conditions were determined using the paired bootstrap test in the TWOSEX-MSChart program (Chi, 2022a).
Population projection
The population growth of O. strigicollis under different temperature conditions was projected using the computer program TIMING-MSChart (Chi, 2022c). All of the figures were drawn with GraphPad Prism software (8.0 version).
Results
Life table of O. strigicollis under different temperatures
The temperature had a strong effect on the developmental duration of O. strigicollis, as the developmental duration shortened with an increase in temperature (Table 1). At 18.5°C, the developmental durations of both O. strigicollis eggs and nymphs were the longest, followed by those at 23.5°C and 27°C. At 33°C, the developmental durations of O. strigicollis eggs and nymphs were the shortest, indicating a fast development rate (Supplementary Table 1). The adult longevity of O. strigicollis was not significantly different between the 23.5 and 27°C treatments; both were significantly lower than that at 18.5°C but longer than that at 33°C.
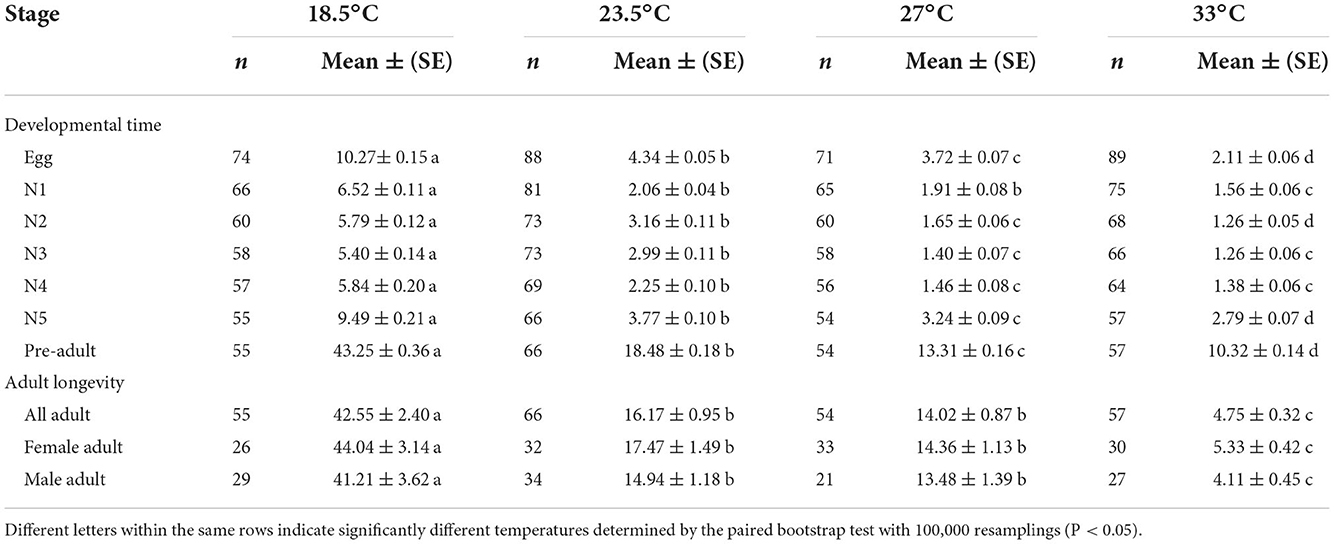
Table 1. Developmental times (d) and adult longevities (d) of Orius strigicollis fed on Frankliniella occidentalis at different temperatures.
The age-stage survival rate (sxj) of O. strigicollis under the different temperature conditions is shown in Figure 1; obvious overlaps existed among the stages at all of the tested temperatures. The survival rates of both eggs and nymphs varied with temperature; the survival rates were higher at 18.5, 23.5, and 27°C (Figures 1A–C) and lower at 33°C (Figure 1D).
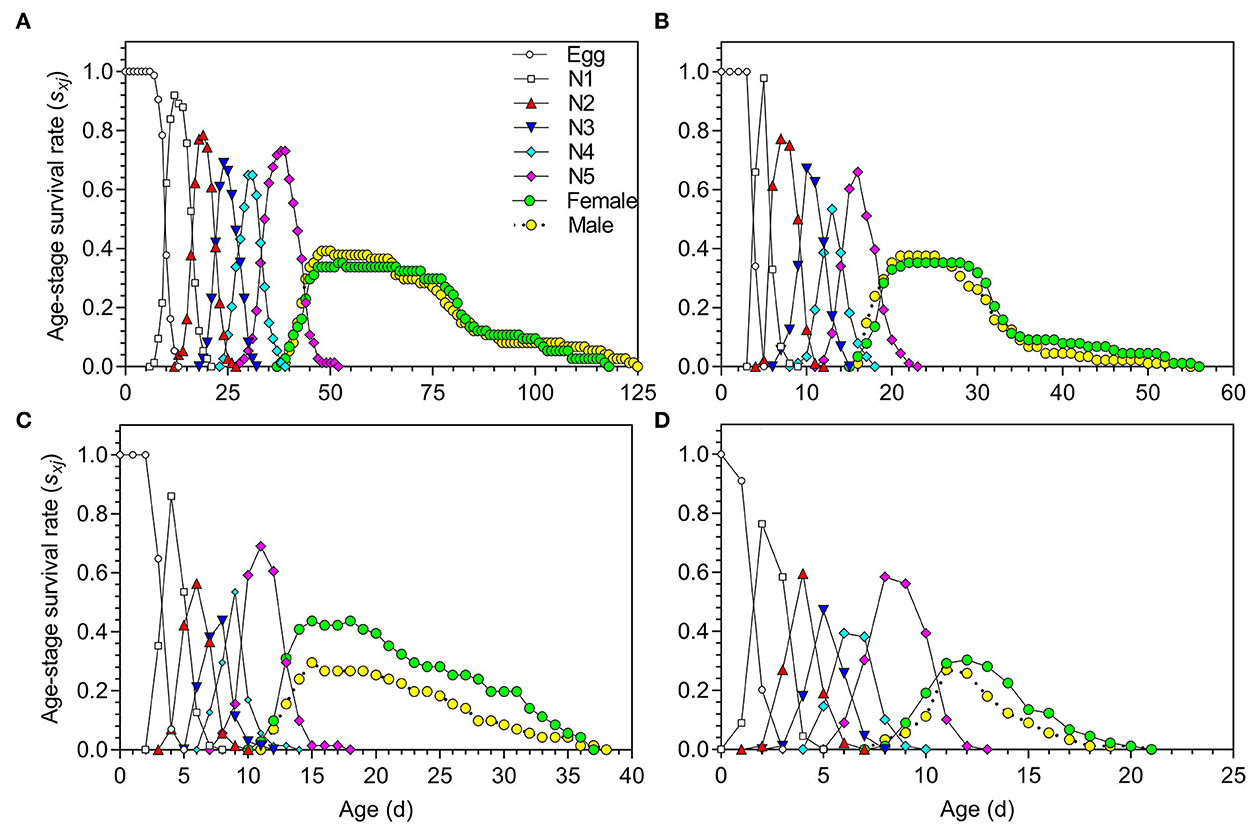
Figure 1. Age-stage survival rate (sxj) of Orius strigicollis fed on Frankliniella occidentalis under 18.5°C (A), 23.5°C (B), 27°C (C), and 33°C (D).
The adult preoviposition period (APOP), total preoviposition period (TPOP), and oviposition days (Od) were significantly longer at 18.5°C than those at 23.5, 27, and 33°C (Table 2). The parameters of APOP and Od did not significantly differ between the 23.5°C (APOP = 4.73 days, Od = 10.67 days) and 27°C (APOP = 3.60 days, Od = 9.23 days) treatments, but both were significantly longer than those at 33°C (APOP = 1.78 days, Od = 3.04 days). The mean fecundity (F) of O. strigicollis showed no significant difference among the 18.5°C (58.31 eggs/female), 23.5°C (64.03 eggs/female), and 27°C (78.09 eggs/female) treatments but was obviously higher than that at 33°C (17.87 eggs/female). However, the number of eggs laid per female per day (f ) at 27°C (5.28 eggs) was obviously larger than those at 23.5°C (3.57 eggs), 33°C (2.93 eggs), and 18.5°C (1.32 eggs). Among the tested temperatures, the female age-specific fecundity (fx7) first increased rapidly, peaked, and then decreased (Figure 2). The peak fx7 of O. strigicollis was 7.83 eggs/female at 27°C, which was higher than that at 23.5°C (6.55 eggs/female), 33°C (5.25 eggs/female), and 18.5°C (2.28 eggs/female). Fecundity (mx) and net maternity (lxmx) are plotted in Figure 2.
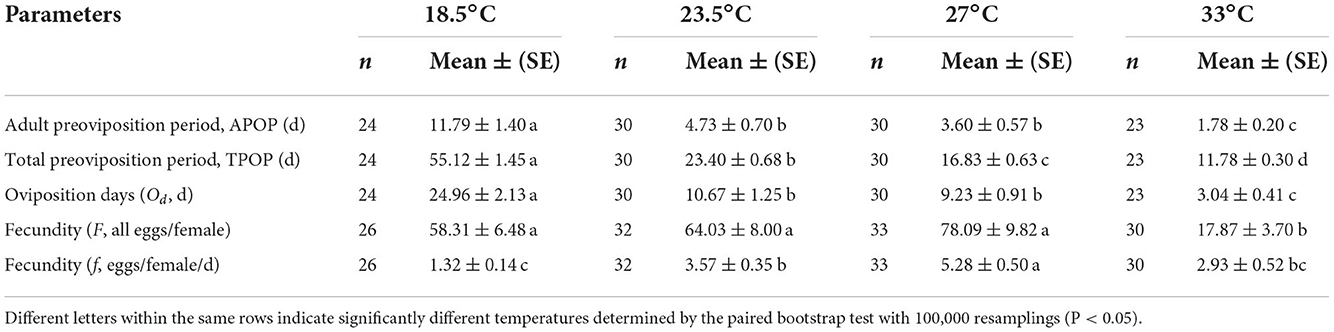
Table 2. Preoviposition period, oviposition period, and fecundity of Orius strigicollis preying on Frankliniella occidentalis at different temperatures.
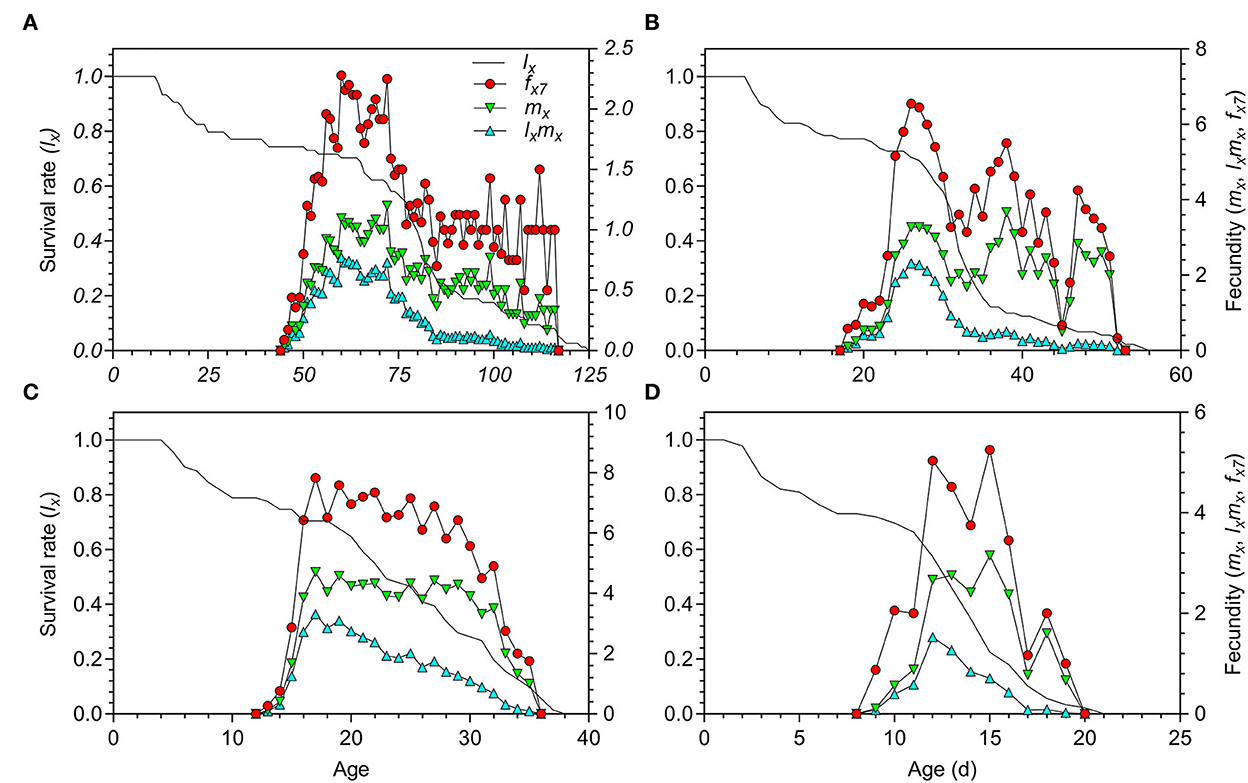
Figure 2. Age-specific survival rate (lx), fecundity (mx), net maternity (lxmx), and female age-specific fecundity (fx7) of Orius strigicollis fed on Frankliniella occidentalis under 18.5°C (A), 23.5°C (B), 27°C (C), and 33°C (D).
The life expectancy (exj) of O. strigicollis decreased with increasing temperature (Figure 3). The highest reproductive values (vxj) of O. strigicollis at 18.5, 23.5, 27, and 33°C were 29.43, 34.60, 35.78, and 13.57 day−1, respectively (Figure 4).
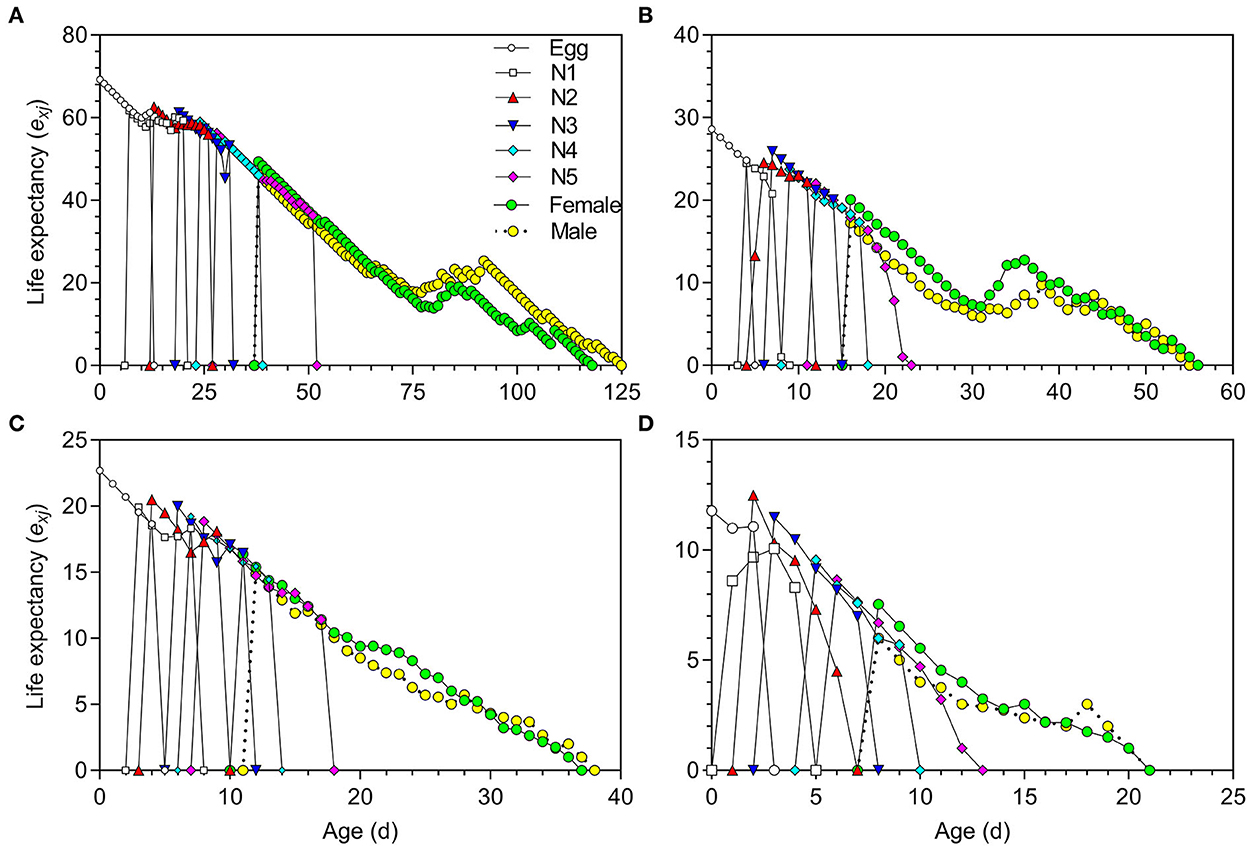
Figure 3. Life expectancy (exj) of Orius strigicollis fed on Frankliniella occidentalis at 18.5°C (A), 23.5°C (B), 27°C (C), and 33°C (D).
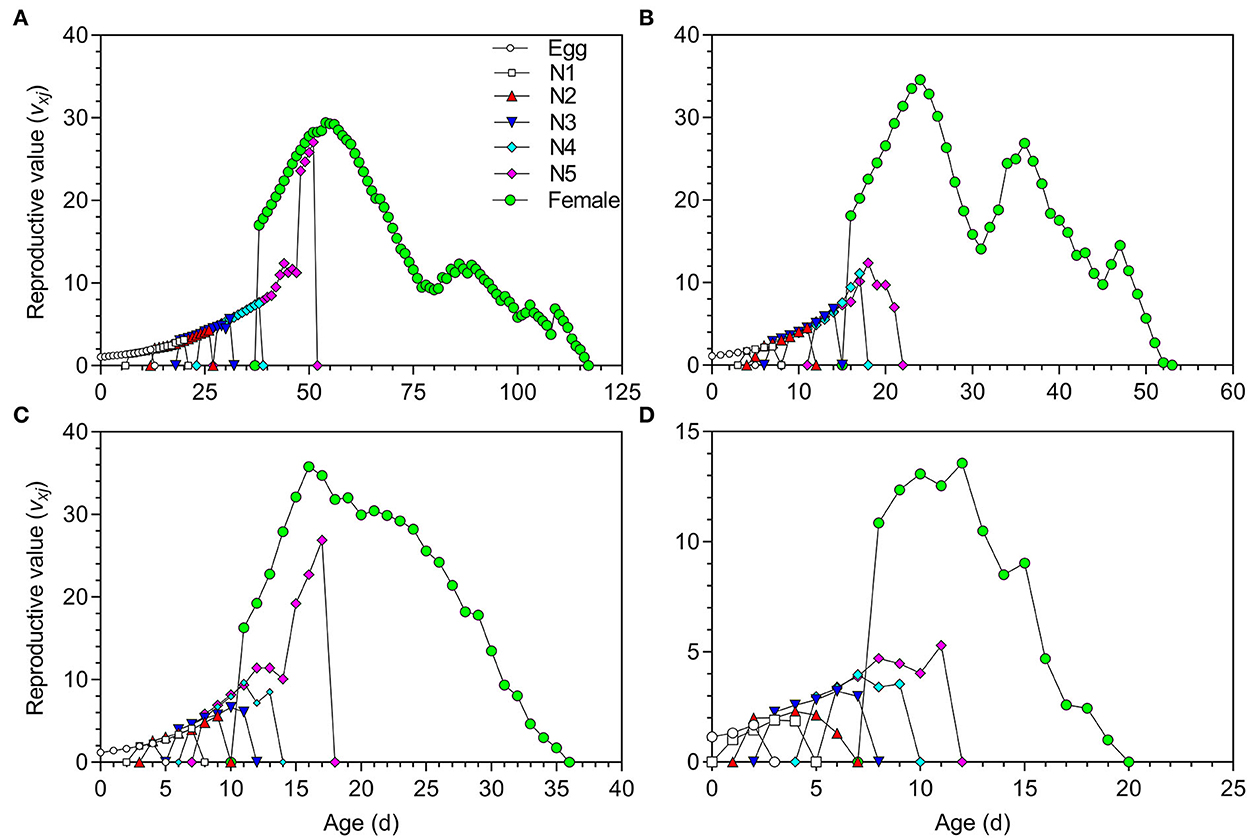
Figure 4. Reproductive value (vxj) of Orius strigicollis fed on Frankliniella occidentalis at 18.5°C (A), 23.5°C (B), 27°C (C), and 33°C (D).
The intrinsic rate of increase (r) and finite rate of increase (λ) of O. strigicollis were highest at 27°C (r = 0.1682 day−1, λ = 1.1833 day−1) but did not differ significantly from those of at 33°C (r = 0.1298 day−1, λ = 1.1387 day−1) (Table 3); those at 23.5°C (r = 0.1094 day−1, λ = 1.1157 day−1) and 18.5°C (r = 0.0457 day−1, λ = 1.0467 day−1) were lower. The net reproductive rate (R0) of O. strigicollis at 27°C (36.29 offspring/individual) was not significantly different from that at 23.5°C (23.28 offspring/individual), but the R0 values at both of these temperatures were significantly higher than those at 18.5°C (20.48 offspring/individual) and 33°C (6.02 offspring/individual). The mean generation time (T) of O. strigicollis decreased as the temperature increased. The longest generation time was observed at 18.5°C (66.06 days), while the shortest generation time was observed at 33°C (13.83 days).
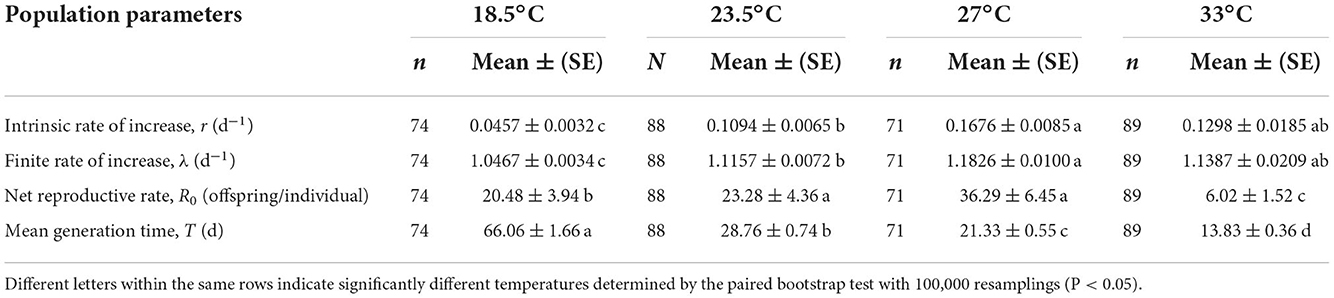
Table 3. Population parameters of Orius strigicollis preying on Frankliniella occidentalis at different temperatures.
Predation rate
The daily predation rate (Dj) of O. strigicollis increased as the temperature increased within the tested temperature range (Table 4), and the Dj of each stage was the highest at 27°C. For O. strigicollis female adults, the Dj showed no significant difference between 27°C (9.88 prey/predator) and 23.5°C (9.44 prey/predator), but these Dj values were significantly higher than that at 18.5°C (4.40 prey/predator). The total numbers of WFT consumed (Pj) during the preadult stage were 69.96, 63.61, and 60.28 per predator at 18.5, 23.5, and 27°C, respectively (Table 5). The adult Pj at 18.5°C was higher than those at 23.5 and 27°C. The Pj of female adults was the highest at 18.5°C (198.87 prey/predator), followed by 23.5°C (164.92 prey/predator) and 27°C (141.93 prey/predator).
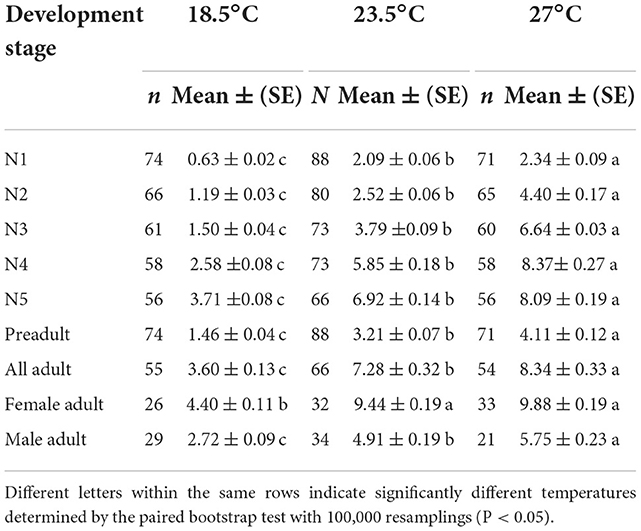
Table 4. Predation rate for daily (Dj) of Orius strigicollis fed on Frankliniella occidentalis at different temperatures.
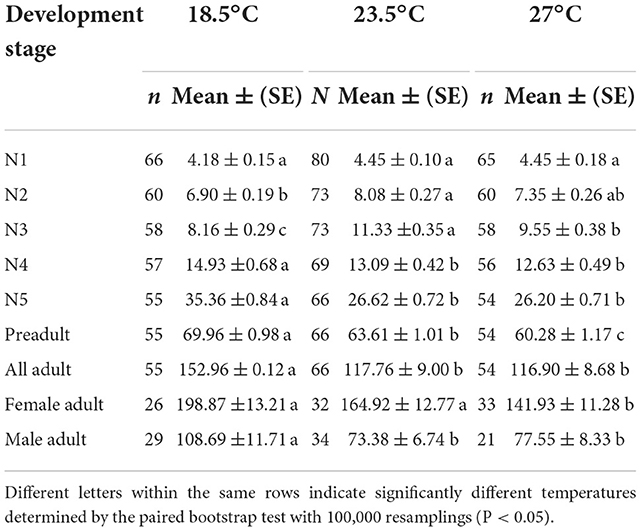
Table 5. Predation rate for the stage of Orius strigicollis survived (Pj) fed on Frankliniella occidentalis at different temperatures.
The first peaks of the age-specific predation rate (kx) and age-specific net predation rate (qx) in O. strigicollis fed on WFT at 18.5°C occurred at the age of 39.00 days in the N5 stage; these rates then decreased until adult emergence at the age of 44.00 days (Figure 5A). The peaks occurred significantly later than those at 23.5°C (15.00 days) (Figure 5B) and 27°C (10.00 days) (Figure 5C).
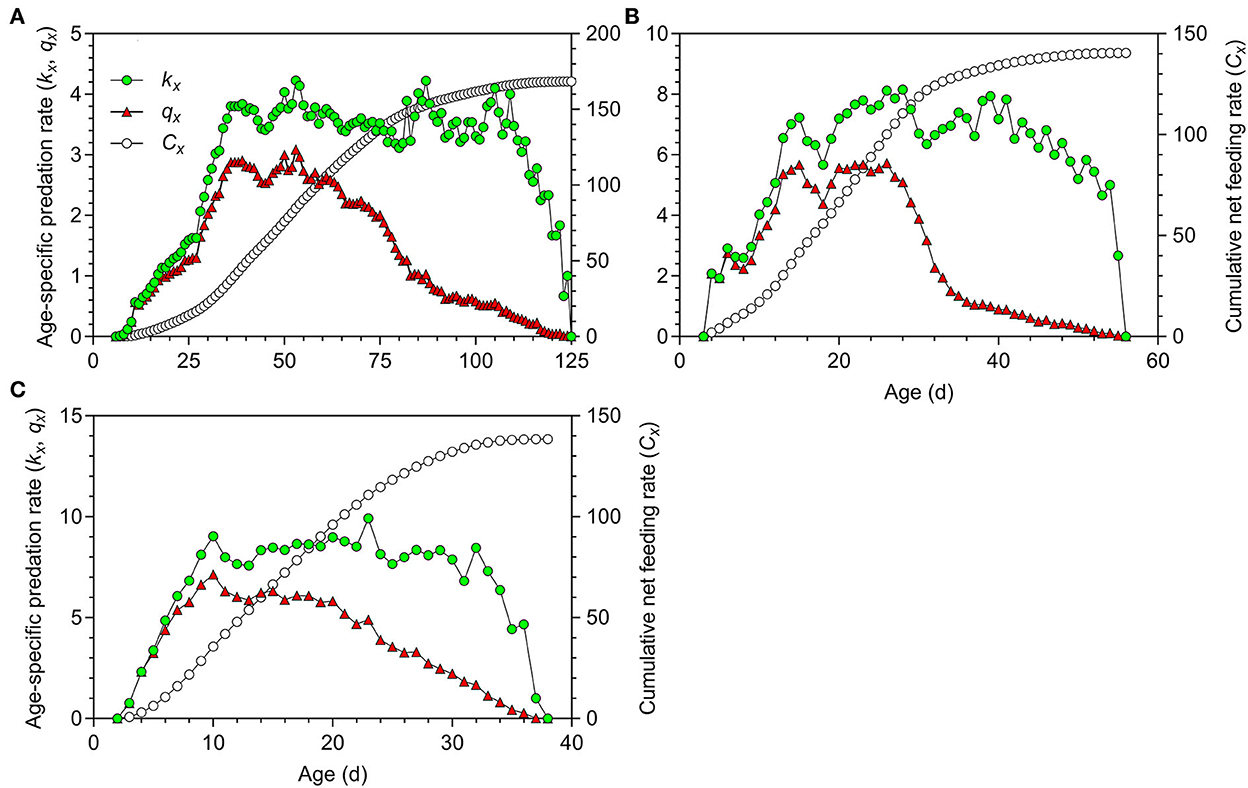
Figure 5. Age-specific predation rate (kx), age-specific net predation rate (qx) and cumulative predation rate (Cx) of Orius strigicollis fed on Frankliniella occidentalis at 18.5°C (A), 23.5°C (B), and 27°C (C).
The net predation rate (C0) varied significantly among temperature conditions (Table 6). Each O. strigicollis consumed an average of 168.39 prey over its lifetime when reared at 18.5°C, which was significantly higher than the numbers of prey consumed at 23.5°C (140.49 prey/predator) and 27°C (138.39 prey/predator). The finite predation rate (ω) increased as the temperature increased. The transformation rate (Qp) indicated that at 18.5°C, O. strigicollis needed to consume 8.22 WFTs to produce one egg, which was not significantly different from that at 23.5°C (6.03); however, both were higher than that at 27°C (3.81).
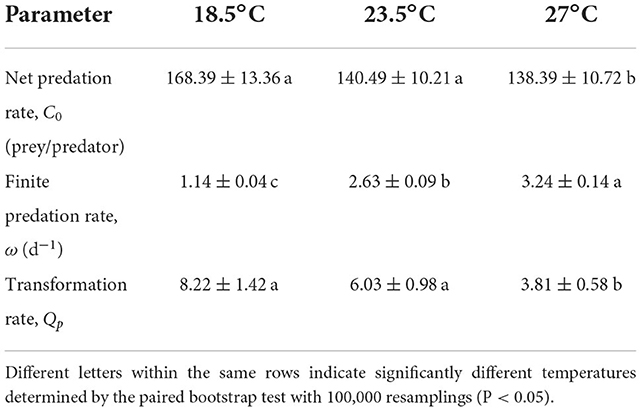
Table 6. Parameters of Orius strigicollis preying (Mean ± SE) on Frankliniella occidentalis at different temperatures.
Population projections of O. strigicollis in response to temperature
Data from the life table study were used to project the population growth of O. strigicollis with an initial number of 10 viable eggs (Figure 6). The population increased faster at 27°C than at 18.5, 23.5, and 33°C. After 40 days, the total numbers of O. strigicollis produced under 18.5, 23.5, 27, and 33°C conditions were 8 (0 eggs, 6 nymphs, 1 female adult, and 1 male adult), 181 (21 eggs, 152 nymphs, 4 female adults and 4 male adults), 3,308 (2,014 eggs, 1,115 nymphs, 109 female adults, and 70 male adults), and 855 (430 eggs, 299 nymphs, 73 female adults, and 53 male adults), respectively.
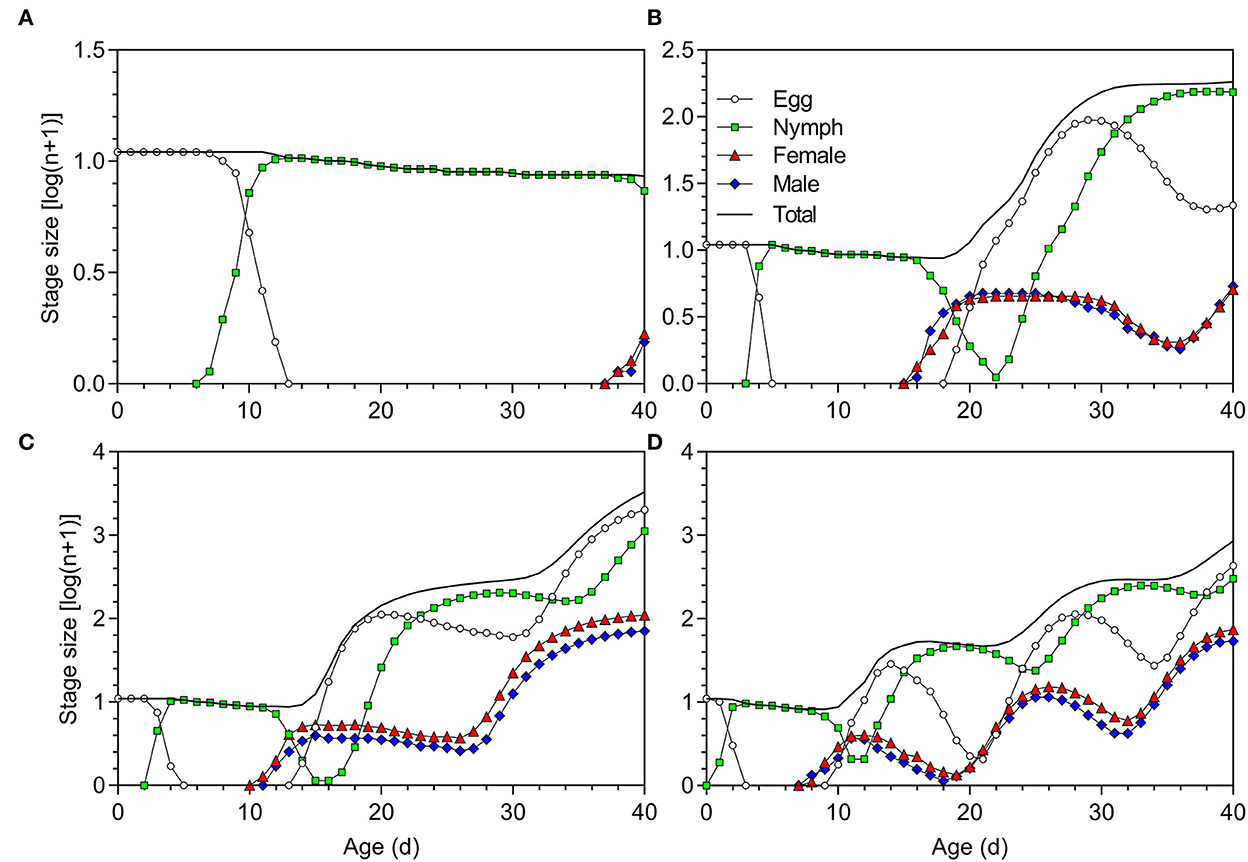
Figure 6. Population projection of Orius strigicollis fed on Frankliniella occidentalis at (A) 18.5°C, (B) 23.5°C, (C) 27°C, and (D) 33°C starting with an initial population of 10 viable eggs.
With an initial population of five pairs of newly hatched adults, after 40 days, the total numbers of O. strigicollis produced at 18.5, 23.5, 27, and 33°C were 223 (79 eggs, 137 nymphs, 4 female adults, and 3 male adults), 2,926 (1,695 eggs, 1,062 nymphs, 87 female adults, and 82 male adults), 12,888 (5,380 eggs, 5,936 nymphs, 986 female adults, and 586 male adults), and 3,085 (564 eggs, 2,195 nymphs, 184 female adults, and 142 male adults), respectively (Figure 7).
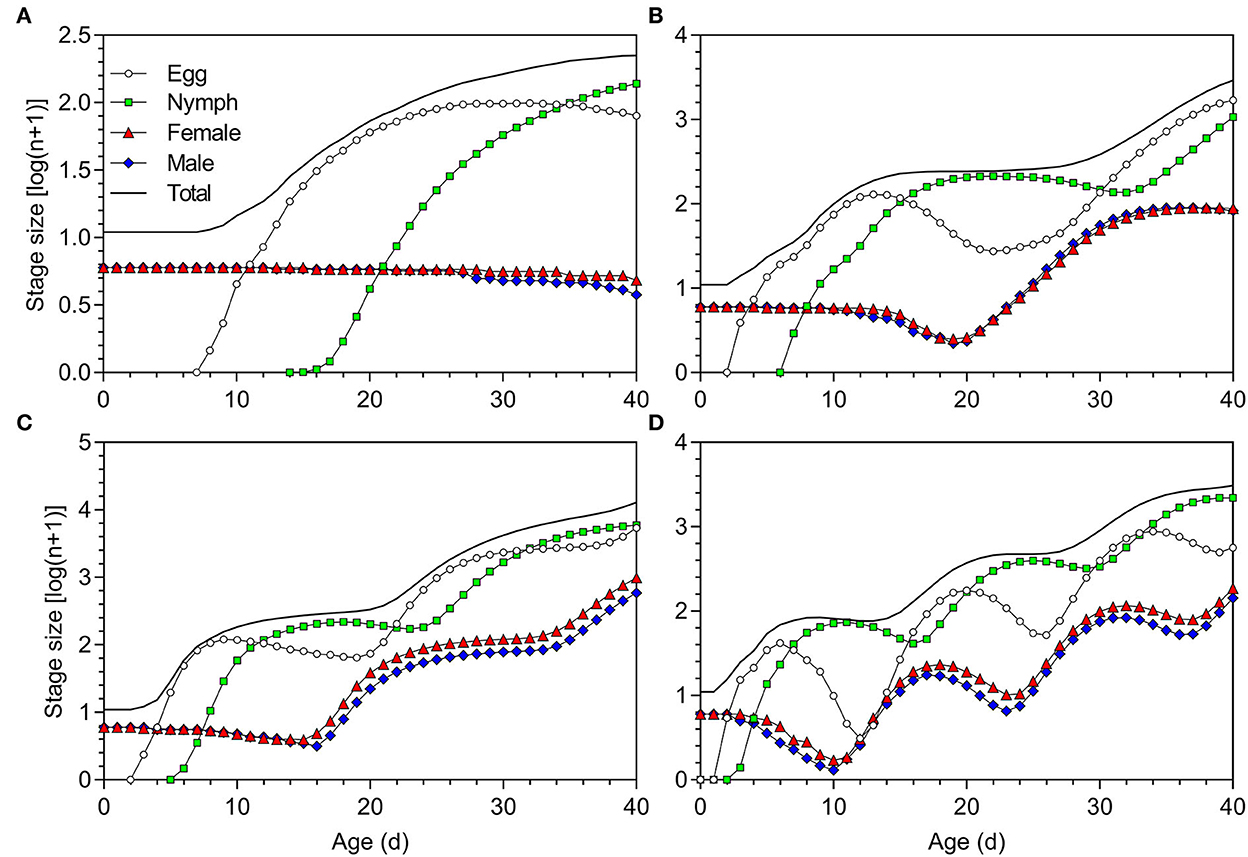
Figure 7. Population projection of Orius strigicollis fed on Frankliniella occidentalis at (A) 18.5°C, (B) 23.5°C, (C) 27°C, and (D) 33°C starting with an initial population of five paired adults.
Discussion
Effect of temperature on the development and fecundity of O. strigicollis
Variability in temperature conditions can directly affect the physiology, survival, fecundity, behavior, and other characteristics of insects (Harrison et al., 2012; González-Tokman et al., 2020), ultimately affecting population dynamics (Bai et al., 2022). The development rate of insects is temperature-dependent (Bai et al., 2022), and the developmental duration of Orius can be significantly affected by this abiotic factor. Consistent with these findings, our results showed that temperature had a significant effect on the developmental duration of O. strigicollis. The prolongation of developmental duration observed at decreased temperatures may be due to a decrease in insect metabolism at lower temperatures (Howe, 1967; Brown et al., 2004; Garcia et al., 2007; Williams III and Roane, 2007; González-Tokman et al., 2020). Thus, the adult preoviposition period (APOP), total preoviposition period (TPOP), oviposition days (Od), and mean generation time (T) exhibited temperature dependence (Table 2).
Low temperatures resulted in fewer eggs laid per day by O. strigicollis, similar to Bicyclus anynana, which laid fewer eggs per day at lower temperatures than at higher temperatures (Geister et al., 2008). However, the mean number of eggs laid per O. strigicollis female over a lifetime revealed no significant difference between 18.5, 23.5, and 27°C when considering the longevity and survival of adults. The fecundity of insects is limited under high-temperature conditions (Harrison et al., 2012). For example, when the temperature was 32–36°C, the fecundity of Orius tantillus decreased substantially (Ballal et al., 2017), and the fecundity of both Orius laevigatus and Orius albidipennis decreased substantially as the temperature was increased to 35°C (Cocuzza et al., 1997; Sanchez and Lacasa, 2002). As a result of physiological trade-offs, insects may need to reduce their fecundity to ensure high-quality egg production (Berger et al., 2008). Fand et al. (2015) also reported reduced adult longevity at high temperatures, as well as a shortening of the reproductive phase with decreased oviposition. In the current study, the high temperature had an obvious effect on the fecundity of O. strigicollis, resulting in a significantly low net reproductive rate (R0 = 6.02 offspring/individual at 33°C).
Effect of temperature on the population parameters of O. strigicollis
Previous studies by Tuan et al. (2016) and Ding et al. (2021) showed that the intrinsic rate of increase (r) and finite rate of increase (λ) of O. strigicollis fed on Cadra cautella (r = 0.1677 day−1, λ = 1.1826 day−1) and Frankliniella intonsa nymphs (r = 0.1437 day−1, λ = 1.1546 day−1) at 25°C were similar, and these rates were also similar to those of O. strigicollis fed on WFT nymphs at 27°C in this study (r = 0.1682 day−1, λ = 1.1833 day−1). The temperature had obvious effects on developmental duration, longevity, survival, and fecundity; thus, both the population parameters of r and λ were also temperature dependent (Yu J. K., et al., 2013; Ali et al., 2020). For O. strigicollis reared at 18.5°C and 23.5°C, a slow developmental rate (Supplementary Table 1) was the primary factor resulting in the low rate of population increase. However, despite the low fecundity of O. strigicollis at 33°C, the short preadult duration and adult longevity enhanced population growth, resulting in large increases in these rates (r = 0.1298 day−1, λ = 1.1387 day−1). These findings are similar to the results of a previous study, where the recorded values were 0.12 day−1 (r) and 1.13 day−1 (λ) for O. strigicollis reared on eggs of Pectinophora gossypiella at 31°C (Ali et al., 2020).
Effects of temperature on the predation rates of O. strigicollis
Temperature strongly affects the predation ability of predaceous insects, as frequently confirmed by the functional response (Sørensen et al., 2013; Ge et al., 2018; Rehman et al., 2020; Bai et al., 2022). As the temperature increases within a tolerable temperature range, physiological metabolism increases, and predators consume more prey to meet these energetic demands (Schwarz and Frank, 2019; González-Tokman et al., 2020). Similarly, the mean predation rates per day (Dj) of both nymphs and adults of O. strigicollis on WFT increased as the temperature increased (Table 4). The finite predation rate (ω) can be used to assess the potential predation of natural enemies (Chi et al., 2011; Yu J. K., et al., 2013); this rate showed an increase with increasing temperature in the current study, similar to trends in previous studies (Sørensen et al., 2013; Helgadóttir et al., 2017; Ge et al., 2018). When considering the survival rate, longevity, and predation rate, higher net predation rates (C0) were observed at 18.5 and 23.5°C than at 27°C, similar to the result reported by Yu J. K., et al. (2013). Additionally, when developing at low temperatures, some insects can physiologically adapt by accumulating energy reserves to improve cold stress tolerance (Denlinger and Lee, 2010). Thus, O. strigicollis reared at 18.5°C may need to accumulate more energy reserves for survival by increasing the predation rate. Accordingly, the transformation rate (Qp) was highest at the lowest temperature (18.5°C), allowing individuals to maximize fitness at this temperature.
Population projections and release
The computer simulation in this study showed the stage structure and population dynamics of O. strigicollis in response to different temperatures, providing a reference for the field application of this natural enemy under various environmental temperature conditions. The optimal predator stage, release time, and release ratio of natural enemies at various temperatures can be determined based on population projections (Yu L. Y., et al., 2013; Mou et al., 2015; Ding et al., 2021) to ensure the establishment of sufficient natural enemy populations for pest population suppression (Janssen and Sabelis, 2015; Mendoza et al., 2021). Obviously, the developmental stage of natural enemies released can affect population dynamics, and the O. strigicollis population increased faster when initiated using five pairs of adults than when using 10 viable eggs, similar to the results reported by Ding et al. (2021). As the predation rate was also age-dependent (Table 4), the release of adults or nymphs of O. strigicollis with a high predation rate is favorable for achieving immediate pest control (van Lenteren et al., 2018; Ding et al., 2021). In addition, the preventative release of natural enemies (one aspect of ABC) by introducing natural enemies in the greenhouse first and supporting natural enemy establishment before pest arrival has also been proposed (Messelink et al., 2014; van Lenteren et al., 2018; Pijnakker et al., 2020). This method facilitates the establishment of an adequate population of natural enemies, allowing predator synchronization with pest population growth. Thus, in the preventative release of this natural enemy, both the effects of environmental temperatures and the release stage of O. strigicollis on the population dynamics should be considered to determine the timing of release to prevent thrips outbreaks.
Integrated analysis of the demography and predation rate of the natural enemy in response to temperature can facilitate the biocontrol efficacy of natural enemy release for pest control. This study comprehensively described the demographic characteristics and predation rate of O. strigicollis in response to temperature. Increasing temperature promoted the development and shortened the longevity of O. strigicollis. At 27°C and 33°C, O. strigicollis exhibited rapid population growth; in contrast, relatively low temperatures, especially 18.5°C, led to slow population growth. The predation rates of O. strigicollis in various developmental stages varied greatly at different temperatures. Consequently, when releasing O. strigicollis in the field to control WFT, both environmental temperature and developmental stage should be taken into consideration to establish sufficient populations.
Data availability statement
The data analyzed in this study is subject to the following licenses/restrictions: The datasets generated and/or analyzed during the current study are available from the corresponding author on reasonable request. Requests to access these datasets should be directed to luybcn@163.com.
Author contributions
XR, LZ, and YL conceived the research, designed experiments, and wrote the manuscript. XL, JH, and ZZ collected and prepared material. XR, JZ, LC, and SZ performed experiments and collected data. XR, XL, and MH analyzed data. All authors read and approved the manuscript.
Funding
This study was supported by the Zhejiang Provincial Key Research and Development Plan (2020C02001-08, 2021C02003), the National Key Research and Development Program of China (2017YFD0201000), the National Natural Science Foundation of China (31901885), and the Demonstration and Extension Program of Science and Technology Achievements of Zhejiang Academy of Agricultural Sciences (TG2022008).
Conflict of interest
The authors declare that the research was conducted in the absence of any commercial or financial relationships that could be construed as a potential conflict of interest.
Publisher's note
All claims expressed in this article are solely those of the authors and do not necessarily represent those of their affiliated organizations, or those of the publisher, the editors and the reviewers. Any product that may be evaluated in this article, or claim that may be made by its manufacturer, is not guaranteed or endorsed by the publisher.
Supplementary material
The Supplementary Material for this article can be found online at: https://www.frontiersin.org/articles/10.3389/fsufs.2022.1026115/full#supplementary-material
References
Ali, S., Zhu, Q., Jaleel, W., Rehman, S. U., Rasheed, M. A., Khan, M. M., et al. (2020). Determination of fitness traits of Orius strigicollis Poppius (Hemiptera: Anthocoridae) on Pectinophora gossypiella (Lepidoptera: Gelechiidae) using two-sex life table analysis. PeerJ. 8, e9594. doi: 10.7717/peerj.9594
Avellaneda, J., Díaz, M., Coy-Barrera, E., Rodríguez, D., and Osorio, C. (2021). Rose volatile compounds allow the design of new control strategies for the western flower thrips (Frankliniella occidentalis). J. Pest Sci. 94, 129–142. doi: 10.1007/s10340-019-01131-7
Bai, Y., Quais, M. K., Zhou, W., and Zhu, Z. R. (2022). Consequences of elevated temperature on the biology, predation, and competitiveness of two mirid predators in the rice ecosystem. J. Pest Sci. 95, 901–916. doi: 10.1007/s10340-021-01414-y
Ballal, C. R., Gupta, T., and Joshi, S. (2017). Effect of constant temperature regimes on the biological parameters of an anthocorid predator Orius tantillus (Motsch). J. Biol. Control 31, 146–158. doi: 10.18311/jbc/2017/16431
Baniameri, V., Soleiman-nejadian, E., and Mohaghegh, J. (2005). Life table and age-dependent reproduction of the predatory bug Orius niger Wolff (Heteroptera: Anthocoridae) at three constant temperatures: a demographic analysis. Appl. Entomol. Zool. 40, 545–550. doi: 10.1303/aez.2005.545
Barton, B. T., and Schmitz, O. J. (2009). Experimental warming transforms multiple predator effects in a grassland food web. Ecol. Lett. 111, 2599–2604. doi: 10.1111/j.1461-0248.2009.01386.x
Berger, D., Walters, R., and Gotthard, K. (2008). What limits insect fecundity? Body size and temperature-dependent egg maturation and oviposition in a butterfly. Funct. Ecol. 22, 523–529. doi: 10.1111/j.1365-2435.2008.01392.x
Bielza, P. (2008). Insecticide resistance management strategies against the western flower thrips, Frankliniella occidentalis. Pest Manag. Sci. 64, 1131–1138. doi: 10.1002/ps.1620
Bonte, J., van de Walle, A., Conlong, D., and De Clercq, P. (2017). Eggs of Ephestia kuehniella and Ceratitis capitata, and motile stages of the astigmatid mites Tyrophagus putrescentiae and Carpoglyphus lactis as factitious foods for Orius spp. Insect Sci. 24, 613–622. doi: 10.1111/1744-7917.12293
Boukal, D. S., Bideault, A., Carreira, B. M., and Sentis, A. (2019). Species interactions under climate change: connecting kinetic effects of temperature on individuals to community dynamics. Curr. Opin. Insect Sci. 35, 88–95. doi: 10.1016/j.cois.2019.06.014
Brown, J. H., Gillooly, J. F., Allen, A. P., Savage, V. M., and West, G. B. (2004). Toward a metabolic theory of ecology. Ecology 85, 1771–1789. doi: 10.1890/03-9000
Chi, H. (1988). Life-table analysis incorporating both sexes and variable development rates among individuals. Environ. Entomol. 17, 26–34. doi: 10.1093/ee/17.1.26
Chi, H. (2022a). TWOSEX-MSChart: A Computer Program for the Age-Stage, Two-Sex Life Table Analysis. Available online at: http://140.120.197.173/Ecology/ (accessed August 2 2022).
Chi, H. (2022b). CONSUME-MSChart: A Computer Program for Consumption Rate Based on the Age-Stage, Two-Sex Life Table Analysis. Available online at: http://140.120.197.173/Ecology (accessed August 2, 2022).
Chi, H. (2022c). TIMING-MSChart: A Computer Program for Population Projection Based on the Age-Stage, Two-Sex Life Table Analysis. Available online at: http://140.120.197.173/Ecology/ (accessed August 2, 2022).
Chi, H., Huang, Y. B., Allahyari, H., Yu, J. Z., Mou, D. F., Yang, T. C., et al. (2011). Finite predation rate: a novel parameter for the quantitative measurement of predation potential of predator at population level. Nat. Prec. doi: 10.1038/npre.2011.6651.1
Chi, H., and Liu, H. (1985). Two new methods for the study of insect population ecology. Bull. Inst. Zool. Academia Sinica 24, 225–240.
Chi, H., and Su, H. Y. (2006). Age-stage, two-sex life tables of Aphidius gifuensis (Ashmead) (Hymenoptera: Braconidae) and its host Myzus persicae (Sulzer) (Homoptera: Aphididae) with mathematical proof of the relationship between female fecundity and the net reproductive rate. Environ. Entomol. 35, 10–21. doi: 10.1603/0046-225X-35.1.10
Chi, H., and Yang, T. C. (2003). Two-Sex life table and predation rate of Propylaea japonica Thunberg (Coleoptera: Coccinellidae) fed on Myzus persicae (Sulzer) (Homoptera: Aphididae). Environ. Entomol. 32, 327–333. doi: 10.1603/0046-225X-32.2.327
Chi, H., You, M., Atlihan, R., Smith, C. L., Kavousi, A., Özgökçe, M. S., et al. (2020). Age-stage, two-sex life table: an introduction to theory, data analysis, and application. Entomol. Gen. 40, 103–124. doi: 10.1127/entomologia/2020/0936
Cho, J. R., Kim, J. H., Lee, M., and Kim, H. S. (2005). Induction and termination of the reproductive diapause in the minute pirate bug Orius strigicollis Poppius (Hemiptera: Anthocoridae). J. Asia-Pac. Entomol. 8, 167–174. doi: 10.1016/S1226-8615(08)60088-6
Cocuzza, G. E., De Clercq, P., Lizzio, S., Van de Veire, M., Tirry, L., Degheele, D., et al. (1997). Life tables and predation activity of Orius laevigatus and O. albidipennis at three constant temperatures. Entomol. Exp. Appl. 85, 189–198. doi: 10.1046/j.1570-7458.1997.00249.x
De Clercq, P., Coudron, T. A., and Riddick, E. W. (2014). “Production of heteropteran predators,” in Mass Production of Beneficial Organisms: Invertebrates and Entomopathogens, eds J. A. Morales-Ramos, M. G. Rojas, and D. I. Shapiro-Ilan (USA, Academic Press), 57–100.
Demirozer, O., Tyler-Julian, K., Funderburk, J., Leppla, N., and Reitz, S. R. (2012). Frankliniella occidentalis (Pergande) integrated pest management programs for fruiting vegetables in Florida. Pest Manag. Sci. 68, 1537–1545. doi: 10.1002/ps.3389
Denlinger, D. L., and Lee, R. (2010). Low Temperature Biology of Insects. New York, NY, Cambridge University Press.
Ding, H. Y., Lin, Y. Y., Tuan, S. J., Tang, L. C., Chi, H., Atlihan, R., et al. (2021). Integrating demography, predation rate, and computer simulation for evaluation of Orius strigicollis as biological control agent against Frankliniella intonsa. Entomol. Gen. 41, 179–196. doi: 10.1127/entomologia/2020/1082
Fand, B. B., Sul, N. T., Bal, S. K., and Minhas, P. S. (2015). Temperature impacts the development and survival of common cutworm (Spodoptera litura): simulation and visualization of potential population growth in India under warmer temperatures through life cycle modelling and spatial mapping. PLoS ONE 10, e0124682. doi: 10.1371/journal.pone.0124682
Funderburk, J., Stavisky, J., and Olson, S. (2000). Predation of Frankliniella occidentalis (Thysanoptera: Thripidae) in field peppers by Orius insidiosus (Hemiptera: Anthocoridae). Environ. Entomol. 29, 376–382. doi: 10.1093/ee/29.2.376
Gao, Y., Lei, Z., and Reitz, S. R. (2012). Western flower thrips resistance to insecticides: detection, mechanisms and management strategies. Pest Manag. Sci. 68, 1111–1121. doi: 10.1002/ps.3305
Garcia, E. S., Ratcliffe, N. A., Whitten, M. M., Gonzalez, M. S., and Azambuja, P. (2007). Exploring the role of insect host factors in the dynamics of Trypanosoma cruzi–Rhodnius prolixus interactions. J. Insect Physiol. 53, 11–21. doi: 10.1016/j.jinsphys.2006.10.006
Ge, Y., Camara, I., Wang, Y., Liu, P., Zhang, L., Xing, Y., et al. (2018). Predation of Aphis craccivora (Hemiptera: Aphididae) by Orius sauteri (Hemiptera: Anthocoridae) under different temperatures. J. Econ. Entomol. 111, 2599–2604. doi: 10.1093/jee/toy255
Geister, T. L., Lorenz, M. W., Meyering-Vos, M., Hoffmann, K. H., and Fischer, K. (2008). Effects of temperature on reproductive output, egg provisioning, juvenile hormone and vitellogenin titres in the butterfly Bicyclus anynana. J. Insect Physiol. 54, 1253–1260. doi: 10.1016/j.jinsphys.2008.06.002
González-Tokman, D., Córdoba-Aguilar, A., Dáttilo, W., Lira-Noriega, A., Sánchez-Guillén, R. A., and Villalobos, F. (2020). Insect responses to heat: physiological mechanisms, evolution and ecological implications in a warming world. Biol. Rev. 95, 802–821. doi: 10.1111/brv.12588
Goodman, D. (1982). Optimal life histories, optimal notation, and the value of reproductive value. Am. Nat. 119, 803–823. doi: 10.1086/283956
Harrison, J. F., Woods, H. A., and Roberts, S. P. (2012). Ecological and Environmental Physiology of Insects. New York, NY, Oxford University Press, 64–97.
He, Z., Guo, J., Reitz, S. R., Lei, Z., and Wu, S. (2020). A global invasion by the thrip, Frankliniella occidentalis: current virus vector status and its management. Insect Sci. 27, 626–645. doi: 10.1111/1744-7917.12721
Helgadóttir, F., Toft, S., and Sigsgaard, L. (2017). Negative effects of low developmental temperatures on aphid predation by Orius majusculus (Heteroptera: Anthocoridae). Biol. Control 114, 59–64. doi: 10.1016/j.biocontrol.2017.08.002
Hinds, J., and Barbercheck, M. E. (2020). Diversified floral provisioning enhances performance of the generalist predator, Orius insidiosus (Hemiptera: Anthocoridae). Biol. Control 149, 104313. doi: 10.1016/j.biocontrol.2020.104313
Howe, R. W. (1967). Temperature effects on embryonic development in insects. Annu. Rev. Entomol. 12, 15–42. doi: 10.1146/annurev.en.12.010167.000311
Janssen, A., and Sabelis, M. W. (2015). Alternative food and biological control by generalist predatory mites: the case of Amblyseius swirskii. Exp. Appl. Acarol. 65, 413–418. doi: 10.1007/s10493-015-9901-8
Landis, D. A., Wratten, S. D., and Gurr, G. M. (2000). Habitat management to conserve natural enemies of arthropod pests in agriculture. Annu. Rev. Entomol. 45, 175–201. doi: 10.1146/annurev.ento.45.1.175
Lewis, W. J., van Lenteren, J. C., Phatak, S. C., and Tumlinson, J. H. (1997). A total system approach to sustainable pest management. P. Natl. Acad. Sci. USA 94, 12243–12248. doi: 10.1073/pnas.94.23.12243
Lu, Y. H., Wu, K. M., Jiang, Y. Y., Guo, Y. Y., and Desneux, N. (2012). Widespread adoption of Bt cotton and insecticide decrease promotes biocontrol services. Nature 487, 362–365. doi: 10.1038/nature11153
Mendoza, J. E., Balanza, V., Cifuentes, D., and Bielza, P. (2021). Genetic improvement of Orius laevigatus for better fitness feeding on pollen. J. Pest Sci. 94, 729–742. doi: 10.1007/s10340-020-01291-x
Mendoza, J. E., Balanza, V., Rodríguez-Gómez, A., Cifuentes, D., and Bielza, P. (2022). Enhanced biocontrol services in artificially selected strains of Orius laevigatus. J. Pest Sci. 95, 1597–1608. doi: 10.1007/s10340-022-01539-8
Messelink, G. J., Bennison, J., Alomar, O., Ingegno, B. L., Tavella, L., Shipp, L., et al. (2014). Approaches to conserving natural enemy populations in greenhouse crops: current methods and future prospects. BioControl 59, 377–393. doi: 10.1007/s10526-014-9579-6
Montserrat, M., Guzmán, C., Sahún, R. M., Belda, J. E., and Hormaza, J. I. (2013). Pollen supply promotes, but high temperatures demote, predatory mite abundance in avocado orchards. Agr. Ecosyst. Environ. 164, 155–161. doi: 10.1016/j.agee.2012.09.014
Mou, D. F., Lee, C. C., Smith, C. L., and Chi, H. (2015). Using viable eggs to accurately determine the demographic and predation potential of Harmonia dimidiata (Coleoptera: Coccinellidae). J. Appl. Entomol. 139, 579–591. doi: 10.1111/jen.12202
Mouden, S., Sarmiento, K. F., Klinkhamer, P. G. L., and Leiss, K. A. (2017). Integrated pest management in western flower thrips: past, present and future. Pest Manag. Sci. 73, 813–822. doi: 10.1002/ps.4531
Musolin, D. L., and Ito, K. (2008). Photoperiodic and temperature control of nymphal development and induction of reproductive diapause in two predatory Orius bugs: interspecific and geographic differences. Physiol. Entomol. 33, 291–301. doi: 10.1111/j.1365-3032.2008.00628.x
Musolin, D. L., Tsytsulina, K., and Ito, K. (2004). Photoperiodic and temperature control of reproductive diapause induction in the predatory bug Orius strigicollis (Heteroptera: Anthocoridae) and its implications for biological control. Biol. Control 31, 91–98. doi: 10.1016/j.biocontrol.2004.04.001
Nagai, K., and Yano, E. (1999). Effects of temperature on the development and reproduction of Orius sauteri (Poppius) (Heteroptera: Anthocoridae), a predator of Thrips palmi Karny (Thysanoptera: Thripidae). Appl. Entomol. Zool. 34, 223–229. doi: 10.1303/aez.34.223
Ohta, I. (2001). Effect of temperature on development of Orius strigicollis (Heteroptera: Anthocoridae) fed on Frankliniella occidentalis (Thysanoptera: Thripidae). Appl. Entomol. Zool. 36, 483–488. doi: 10.1303/aez.2001.483
Peterson, J. A., Burkness, E. C., Harwood, J. D., and Hutchison, W. D. (2018). Molecular gut-content analysis reveals high frequency of Helicoverpa zea (Lepidoptera: Noctuidae) consumption by Orius insidiosus (Hemiptera: Anthocoridae) in sweet corn. Biol. Control 121, 1–7. doi: 10.1016/j.biocontrol.2018.02.006
Pijnakker, J., Vangansbeke, D., Duarte, M., Moerkens, R., and Wäckers, F. L. (2020). Predators and parasitoids-in-first: from inundative releases to preventative biological control in greenhouse crops. Front. Sustain. Food S. 4, 595630. doi: 10.3389/fsufs.2020.595630
Rehman, S. U., Zhou, X., Ali, S., Rasheed, M. A., Islam, Y., Hafeez, M., et al. (2020). Predatory functional response and fitness parameters of Orius strigicollis Poppius when fed Bemisia tabaci and Trialeurodes vaporariorum as determined by age-stage, two-sex life table. PeerJ 8, e9540. doi: 10.7717/peerj.9540
Reitz, S. R., Gao, Y., Kirk, W. D. J., Hoddle, M. S., Leiss, K. A., and Funderburk, J. E. (2020). Invasion biology, ecology, and management of western flower thrips. Annu. Rev. Entomol. 65, 17–37. doi: 10.1146/annurev-ento-011019-024947
Reitz, S. R., Gao, Y., and Lei, Z. (2011). Thrips: pests of concern to China and the United States. Agr. Sci. China 10, 867–892. doi: 10.1016/S1671-2927(11)60073-4
Sanchez, J. A., and Lacasa, A. (2002). Modelling population dynamics of Orius laevigatus and O. albidipennis (Hemiptera: Anthocoridae) to optimize their use as biological control agents of Frankliniella occidentalis (Thysanoptera: Thripidae). B. Entomol. Res. 92, 77–88. doi: 10.1079/BER2001136
Schwarz, T., and Frank, T. (2019). Aphid feeding by lady beetles: higher consumption at higher temperature. BioControl 64, 323–332. doi: 10.1007/s10526-019-09931-7
Sørensen, C. H., Toft, S., and Kristensen, T. N. (2013). Cold-acclimation increases the predatory efficiency of the aphidophagous coccinellid Adalia bipunctata. Biol. Control 65, 87–94. doi: 10.1016/j.biocontrol.2012.09.016
Tuan, S. J., Lee, C. C., and Chi, H. (2014a). Population and damage projection of Spodoptera litura (F.) on peanuts (Arachis hypogaea L.) under different conditions using the age-stage, two-sex life table. Pest Manag. Sci. 70, 805–813. doi: 10.1002/ps.3618
Tuan, S. J., Lee, C. C., and Chi, H. (2014b). Erratum: Population and damage projection of Spodoptera litura (F.) on peanuts (Arachis hypogaea L.) under different conditions using the age-stage, two-sex life table. Pest Manag. Sci. 70, 1936. doi: 10.1002/ps.3920
Tuan, S. J., Yang, C. M., Chung, Y. T., Lai, W. H., Ding, H. Y., Saska, P., et al. (2016). Comparison of demographic parameters and predation rates of Orius strigicollis (Hemiptera: Anthocoridae) fed on eggs of Tetranychus urticae (Acari: Tetranychidae) and Cadra cautella (Lepidoptera: Pyralidae). J. Econ. Entomol. 109, 1529–1538. doi: 10.1093/jee/tow099
van Lenteren, J. C. (2012). The state of commercial augmentative biological control: plenty of natural enemies, but a frustrating lack of uptake. BioControl 57, 1–20. doi: 10.1007/s10526-011-9395-1
van Lenteren, J. C., Bolckmans, K., Köhl, J., Ravensberg, W. J., and Urbaneja, A. (2018). Biological control using invertebrates and microorganisms: plenty of new opportunities. BioControl 63, 39–59. doi: 10.1007/s10526-017-9801-4
Wallner, W. E. (1987). Factors affecting insect population dynamics: differences between outbreak and non-outbreak species. Annu. Rev. Entomol. 32, 317–340. doi: 10.1146/annurev.en.32.010187.001533
Williams III, L., and Roane, T. M. (2007). Nutritional ecology of a parasitic wasp: food source affects gustatory response, metabolic utilization, and survivorship. J. Insect Physiol. 53, 1262–1275. doi: 10.1016/j.jinsphys.2007.06.017
Wu, S., Xing, Z., Ma, T., Xu, D., Li, Y., Lei, Z., et al. (2021). Competitive interaction between Frankliniella occidentalis and locally present thrips species: a global review. J. Pest Sci. 94, 5–16. doi: 10.1007/s10340-020-01212-y
Yu, J. Z., Chi, H., and Chen, B. H. (2013). Comparison of the life tables and predation rates of Harmonia dimidiata (F.) (Coleoptera: Coccinellidae) fed on Aphis gossypii Glover (Hemiptera: Aphididae) at different temperatures. Biol. Control 64, 1–9. doi: 10.1016/j.biocontrol.2012.10.002
Yu, L. Y., Chen, Z. Z., Zheng, F. Q., Shi, A. J., Guo, T. T., Yeh, B. H., et al. (2013). Demographic analysis, a comparison of the jackknife and bootstrap methods, and predation projection: a case study of Chrysopa pallens (Neuroptera: Chrysopidae). J. Econ. Entomol. 106, 1–9. doi: 10.1603/EC12200
Keywords: predator, temperature, release, augmentative biological control, thrips
Citation: Ren X, Li X, Huang J, Zhang Z, Hafeez M, Zhang J, Chen L, Zhou S, Zhang L and Lu Y (2022) Linking life table and predation rate for evaluating temperature effects on Orius strigicollis for the biological control of Frankliniella occidentalis. Front. Sustain. Food Syst. 6:1026115. doi: 10.3389/fsufs.2022.1026115
Received: 23 August 2022; Accepted: 02 November 2022;
Published: 14 December 2022.
Edited by:
Wen Xie, Insititute of Vegetables and Flowers (CAAS), ChinaReviewed by:
Shihao Zhou, Hainan University, ChinaNing Di, Beijing Academy of Agriculture and Forestry Science, China
Copyright © 2022 Ren, Li, Huang, Zhang, Hafeez, Zhang, Chen, Zhou, Zhang and Lu. This is an open-access article distributed under the terms of the Creative Commons Attribution License (CC BY). The use, distribution or reproduction in other forums is permitted, provided the original author(s) and the copyright owner(s) are credited and that the original publication in this journal is cited, in accordance with accepted academic practice. No use, distribution or reproduction is permitted which does not comply with these terms.
*Correspondence: Yaobin Lu, luybcn@163.com; Lisheng Zhang, zhangleesheng@163.com