- 1Center of Food Science and Technology, Chaudhary Charan Singh Haryana Agricultural University Hisar, Hisar, Haryana, India
- 2Department of Plant Pathology, Chaudhary Charan Singh Haryana Agricultural University Hisar, Hisar, Haryana, India
- 3Department of Vegetable Science, Chaudhary Charan Singh Haryana Agricultural University Hisar, Hisar, Haryana, India
- 4Faculty of Agricultural Sciences, Institute of Applied Sciences & Humanities, GLA University, Mathura, Uttar Pradesh, India
- 5Department of Agronomy, Acharya Narendra Deva University of Agriculture and Technology, Ayodhya, India
- 6Department of Plant Pathology, Krishi Vigyan Kendra (KVK), Panchkula (Chaudhary Charan Singh Haryana Agricultural University Hisar), Hisar, India
- 7Department of Agronomy, Chaudhary Charan Singh Haryana Agricultural University Hisar, Hisar, Haryana, India
Nanotechnology emerged as a revolutionary technology in various fields of applied sciences, such as biomedical engineering and food technology. The pivotal roles of nanocompounds have been explored in various fields, such as food protection, preservation, and enhancement of shelf life. In this sequence, metallic nanoparticles (MNPs) are proven to be useful in developing products with antimicrobial activity and subsequently improve the shelf life of agrifoods. The major application of MNPs has been observed in the packaging industry due to the combining ability of biopolymers with MNPs. In recent years, various metal nanoparticles have been explored to formulate various active food packaging materials. However, the method of production and the need for risk evaluation are still a topic of discussion among researchers around the world. In general, MNPs are synthesized by various chemical and physical means, which may pose variable health risks. To overcome such issues, the green synthesis of MNPs using microbial and plant extracts has been proposed by various researchers. In this review, we aimed at exploring the green synthesis of MNPs, their properties and characterization, various ways of utilizing MNPs to extend their shelf life, and, most importantly, the risk associated with these along with their quality and safety considerations.
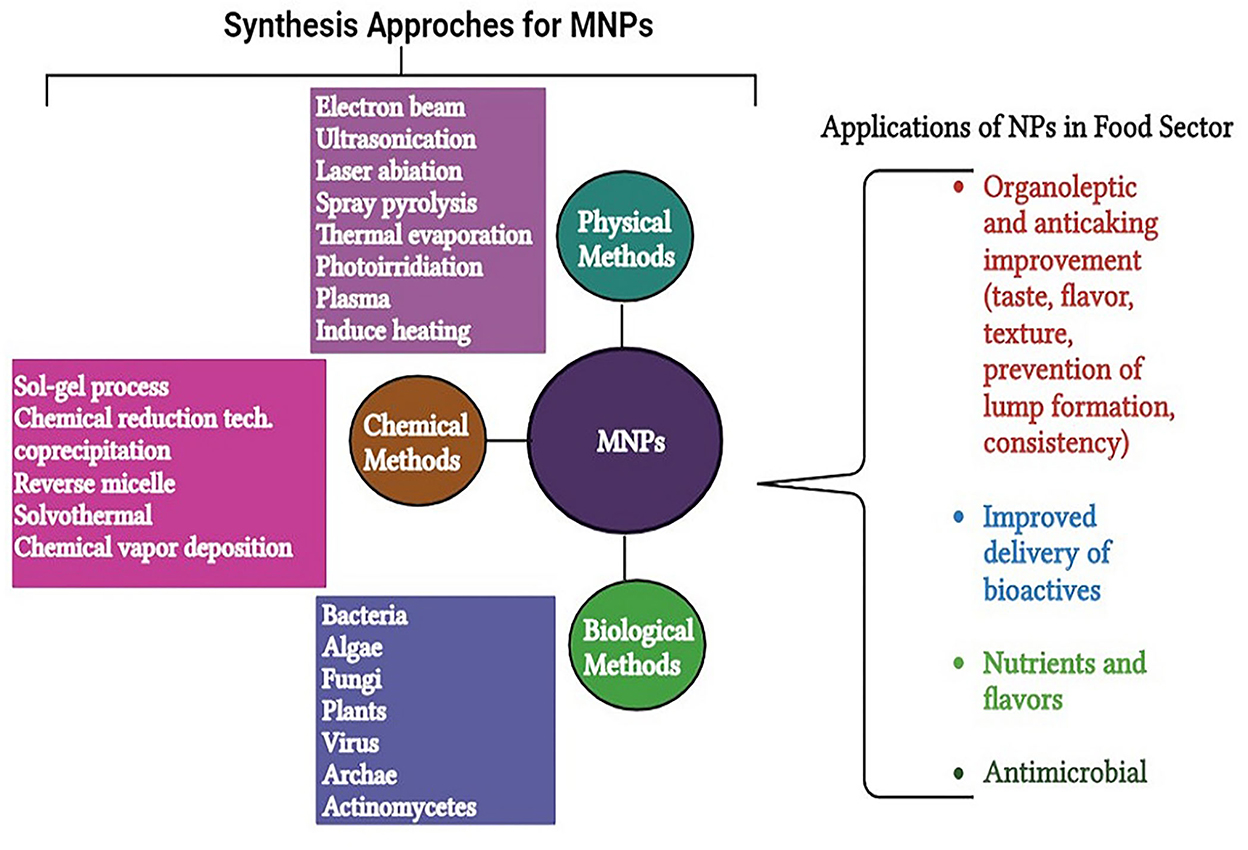
Graphical Abstract. This figure is representation of nanoparticles synthesis and their application in food sector.
Introduction
In the modern era of industrialization, especially in the food industry, the emergence of nanomaterials is gaining widespread interest of researchers in the field of food nanotechnology. This rapid emergence of nanotechnology in the food sector is due to improved properties (mechanical resistance, diffusivity, optical properties, and solubility) of materials formulated through these nano-techniques, which led to their utilization in all stages of the food chain, including processing, production, packaging, storage, and transport (Bang et al., 2019). The estimated role of nanotechnology in the shelf-life enhancement of agrifoods is >40% of estimated food losses around the world. Brazil is the largest producer as well as consumer of NPs (especially Ag NPs), followed by India (Ijaz et al., 2020).
Although there are several techniques to enhance the shelf life of agrifoods, such as modification of atmospheric gas composition, mild heat treatments, combined gas atmosphere treatments, and cold storage, these treatments are less efficient and expensive to operate (Saravanakumar et al., 2020). Due to this, NPs, particularly metallic nanoparticles (MNPs), became a valuable class of nanoparticles owing to their key role in preserving, protecting, and extending the shelf stability of foods (Iderawumi and Yusuff, 2021). MNPs can assist in reducing postharvest losses using active packing elements with enhanced mechanical and gas permeation performances, which ultimately influence the quality of agrifoods (Fadiji et al., 2022). Among all the major strategies toward shelf-life enhancement of foods, incorporation of MNPs into food packaging systems is a widely accepted strategy that improves vital characteristics such as mechanical properties, permeability (to atmospheric gases), and antimicrobial activity, which tends to maintain the freshness and also enhances the shelf life of foods. On the other hand, MNPs assist in the development of microfluidic devices or nanosensors, which are emerging as modern methods of food analysis (Couto and Almeida, 2022).
Each technology has its pros and cons. Due to the enhanced utilization of MNPs, various environmental and ecological issues have originated due to its traditional system of synthesis, which explores a variety of harmful chemicals. To overcome these concerns, the synthesis route of metallic nanocomposites should be altered. As a result, the green synthesis route of NP production is gaining widespread attention worldwide (Zhao et al., 2021). Green synthesis excludes toxic chemicals and organic solvents as reducing agents and only allows using biological systems (microorganisms and plant extracts) to synthesize NPs. Biological systems possess a variety of biochemicals, including flavonoids, terpenoids, alkaloids, and polyphenols, which are potent reducing agents and stabilizing agents (Chi et al., 2019).
From these instances, it is clear that emerging metal nanotechnology possesses both positive and negative impacts and a wide range of applications from agriculture to food processing and packaging. However, various types of safety concerns may arise, especially regarding human health. Therefore, complete information about safety and risk associated with the use of MNPs in food systems is crucial. Keeping these things in view, this review is designed to assess the recent developments in the production and utilization of MNPs, their properties, green synthesis technology, their major roles in the shelf-life enhancement of agrifoods, and their toxicity and health concerns.
MNPs: Synthesis, properties, and characterization
Synthesis of MNPs
As mentioned earlier in this review, there is a remarkable increase in nanotechnology tools owing to their widely use in the field of food processing and preservation, etc. (Dikshit et al., 2021); therefore, studying the mode of its synthesis is more important. NPs are naturally occurring on earth since their origin in soil, water, minerals, volcanic dust, etc. (Luzala et al., 2022). In addition to their natural origin, NPs can also be synthesized through several methods (chemical and biological). In this sequence, there are two types of approaches employed for the formulation of MNPs, that is, bottom-up and top-down methods, which depends upon the initial raw material. In the top-down approach, the large particulate material is converted into nano-sized particles by mechanical milling (dry/wet), laser ablation, and ion sputtering (Loza et al., 2020). The aforementioned methods are easy to operate but inadequate to reduce the particle size to very small. Conversely, in the bottom-up approach, the formation of MNPs is based on joining atoms and molecules to construct nano-sized particles. Methods of the bottom-up approach include electrodeposition, supercritical fluid precipitation, ultrasound, and microwave-assisted techniques (Zhang et al., 2020). Hence, physical methods belong to the category of the top-down approach, while chemical and biological methods follow the bottom-up approach for the NPs synthesis (Sharma and Gupta, 2020). Among all, the biological processes of NP synthesis are considered cheaper, simpler, and ecologically safer as compared to chemical techniques (Dikshit et al., 2021). Nevertheless, low production rates, expensive operations, and high energy consumption are the major limitations of physical processes. Chemical synthesis methods including chemical reduction, electrochemical, microemulsion/colloidal, and thermal decomposition are the traditional and most widely used methods for the synthesis of MNPs. In the chemical method, the chemical reduction of respective metal salt (precursors) is carried out by adding particular reducing agents to produce NPs. Various reducing agents, such as sodium borohydride (Banne et al., 2017) and stabilizing agents, such as dodecyl benzyl sulfate (Akbarzadeh and Dehghani, 2017) and polyvinyl pyrrolidone (Pandey et al., 2018), are used for the synthesis of NPs. The stability of NPs is also a major concern for researchers. The science behind the stabilization of NPs advocates the adsorption of high-molecular weight compounds, which form a layer around the particle surface and prevent aggregation among them (Couto and Almeida, 2022). According to various researchers, the stability of NPs depends on competition between weak van der Waals attractive forces and electrostatic repulsion (Kowsalya et al., 2019). Resorcinol is an interesting reductant as it can be used as both a reducing and a stabilizing agent for the synthesis of NPs. The chemical approaches are economical for large-scale production of NPs; nevertheless, the use of toxic chemicals and the production of harmful by-products cause environmental damage (Gupta and Xie, 2018; Saratale et al., 2018). Due to these major concerns, biological reductants are gaining widespread interest among researchers for the fabrication of NPs and the synthesis of NPs through biological means, which is also termed “green synthesis” (Chopra et al., 2022).
Green synthesis of NPs
Nowadays, green synthesis of NPs is a widely used technique as it is an energy-efficient technique for NP synthesis (Shafiq et al., 2021). In addition, the synthesis of NPs using microorganisms and plants is considered a cost-effective, biologically safe, and eco-friendly substitute (Uzair et al., 2020). It is also considered an important tool to reduce the adverse side effects of NPs produced by traditional synthesis methods (Mukherjee et al., 2021). In recent years, green synthesis is becoming an exciting and upcoming technology possessing a greater scope in the synthesis of NPs (Khanna et al., 2019). For the green synthesis of NPs, bacteria, cyanobacteria, actinomycetes, algae, fungi, and some higher plants are being utilized (Figure 1) (Ramrakhiani and Ghosh, 2018; Mohamed, 2020). Particularly, the synthesis of NPs from plant extracts is termed “phytosynthesis” (Ríos-Corripio et al., 2019). Plants and microorganisms possess the ability to consume and accumulate inorganic metal ions from their corresponding niche (Zhang et al., 2020). In addition, these microbes and plants contain various biochemicals (such as organic acids, alkaloids, polysaccharides, vitamins, amino acids, terpenoids, flavonoids, and polyphenols) that play an important role as reductants and as stabilizers of metal ions (Patil et al., 2018). NPs produced by biological processes possess a greater catalytic activity and a larger surface area because of the improved contact between reducing enzymes and the metal ion (Sharma et al., 2020). However, green production of metal NPs is time-consuming and requires practical microbiological experience to ensure cell culture under aseptic conditions (Kumar et al., 2020). Several studies have been conducted on the use of plants and microbes for NP synthesis, as mentioned in Table 1.
Properties of NPs
As discussed previously, NPs possess altered physical and chemical properties in comparison to their larger dimension counterparts. The following sections explain the important properties that we persuade at the nanoscale.
Mechanical properties
This section describes the most important properties concerning the packaging development process. Mechanical properties of NPs are a function of various other properties, such as strength, hardness, toughness, plasticity, elasticity, rigidity, and yield stress (Sun et al., 2000). Mostly, all the inorganic and nonmetallic materials are brittle and do not possess the required level of toughness, plasticity, and ductility. NPs show differential mechanical properties owing to their surface properties and quantum effects in comparison to bulk materials. For instance, micro-sized FeAl (size >4 μm) is brittle, and on the other hand, the nano-sized FeAl alloy powder possesses better strength, ductility, and plasticity (Pithawalla et al., 2001). This happens due to the diverse interaction forces between NPs and other surfaces. When the size of any particle decreases, the surface forces start playing a major role in contact, adhesion, and deformation.
Thermal properties
Heat transfer primarily depends on energy conduction due to electrons and photons (Savage and Rao, 2006). Thermal properties of nanomaterials are the function of thermal conductivity, heat capacity, thermoelectric power, and thermal stability, and the size of NP directly impacts the thermal and the electrical conductivity of NPs. As their size decreases, the ratio of their surface area to their volume increases (Andrievski, 2014). As the conduction of electrons is mainly responsible for heat transfer, the higher surface-to-volume ratio provides an increased amount of electrons for heat transfer in comparison to the bulk (Qiu et al., 2020).
On the other hand, NPs exhibit a huge decrease in the melting point (MP) of materials because the liquid–vapor interface energy is lower than that of the solid–vapor interface energy (Gülseren et al., 1995). As their size decreases, their surface-to-volume ratio increases, and subsequently, the MP drops down (Shim et al., 2002). For example, the MP of Au NPs is lower than that of gold by 300 degrees. In addition, the composition of NPs plays a paramount role in thermal stability. For example, the thermal stability of gold NPs is higher than that of pure gold (Mottet et al., 2005).
Catalytic properties
NPs also termed nano-catalysis as it induces enhanced catalytic properties i.e., reactivity and selectivity as compared to their normal analogous material. This property of NPs highly depends on their shape, size, composition, oxidation state, interparticle spacing, and support of the NPs. As the size of NPs decreases, their catalytic activity becomes more prominent. On the other hand, the shape of NP also affects its reactivity and selectivity. The hemispherical shape of NPs was found more functional than the spherical shape for the oxidation of CO by Au NPs (Xu et al., 2006). The main reason for these alterations in properties is the increase or decrease in catalytically active surface facets (Henry, 2005). It has also been reported that the use of alloys in NPs can improve their catalytic activity as alloys can alter their electronic properties, decrease the poisoning effect, and provide distinct selectivity (Cuenya, 2010).
Characterization of NPs
The MNPs possess improved characteristics such as high plasmon excitation efficiencies, high surface energies, and exceptional optical properties, and their physicochemical properties mainly depend on free surface electrons (Couto and Almeida, 2022). To monitor the proper synthesis and incorporation of MNPs in the target matrix, several modern techniques are deployed. The techniques employed to study the properties and characteristics of NPs include dynamic light scattering (DLS), the Brunauer–Emmett–Teller (BET) method, atomic force microscopy (AFM), infrared and UV-Vis spectrophotometry, X-ray diffraction (XRD), X-ray photoelectron spectroscopy (XPS), scanning electron microscopy (SEM), transmission electron microscopy (TEM), energy-dispersive X-ray analysis (EDAX), zeta potential (ZP), Raman spectroscopy (R), field emission scanning electron microscopy (FESEM), and high-resolution transmission electron microscopy (HRTEM) (Shifa et al., 2019). A detailed description of these techniques has been given in the following section.
Zeta potential and particle size
These parameters are used to evaluate the stability of NPs utilizing a zeta potentiometer. It assesses the stability of NPs corresponding to their pH. Zeta potential increases with an increase in pH. It has been observed that synthesized Ag NPs are stable within a pH range of 6–12. However, at pH 12, Ag NPs are observed as more stable (Shifa et al., 2019). The high negative zeta potential values of Ag NPs indicate their excellent stability in an aqueous solution (Shankar et al., 2021). On the other hand, dynamic light scattering (DLS) or photon correlation spectroscopy (PCS) is the most common method for determining particle size and distribution. The particle size and size distribution of the sample are also investigated for a size range of 0.1–1,000 nm by laser diffractometry (Wang et al., 2020).
Fourier transfer infrared (FTIR) spectroscopy
It is the most commonly used technique that provides information regarding the functional groups as well as structural variation and also identifies the interactions among them (Jayakumar et al., 2022). It provides an overview of the absorption band corresponding to different molecules and the bonds between them. Furthermore, it gives information about the responsible biomolecule for capping and stabilization (Wang et al., 2020). Different NPs exhibit different types of absorption peak, which are considered the characteristic peaks of that particular NP. ZnO–SiO2 imparts its peak at an absorption band of 3,291.56 cm−1, which represents –NH and –OH vibration stretch, confirming the formation of ZnO—SiO2 NPs (Al-Tayyar et al., 2020). In the case of ZnO NPs, the peak found at 460 cm−1 is attributed to the formation of ZnO NPs, and the peak at 796 cm−1 indicates the formation of SiO2 NPs. Similarly, the peaks at 600 and 1,630 cm−1 correspond to the Cu–O vibration and stretching, confirming the formation of CuO NPs (Francis et al., 2022). Raman spectroscopy is an advanced tool of NP characterization, which is carried out to obtain information about the biocomponent precursors being used for the biosynthesis of NPs, that is, the interaction of polyphenols with S+ ions during NP formation (Shifa et al., 2019).
UV–visible spectroscopy
UV-Vis spectroscopy is mainly used to characterize NPs just after their synthesis in the wavelength range of 300–800 nm as most of the MNPs present their specific peak in the UV or visible range. It also assesses the concentration of NPs that are formed during synthesis (Kavakebi et al., 2021). In addition, it offers instant qualitative information regarding the size of NPs (Fierascu et al., 2019). It also provides information on monitoring the changes in the properties of NPs with time. The absorption peaks in the spectrophotometer vary with different NPs. The difference in absorption peaks mainly depends on particle size, chemical surroundings, and the dielectric medium of the NPs. Furthermore, if the size of NPs is increased due to the aggregation of particles, then there will be a broadening of the plasmonic band toward larger wavelengths in a sensible way (Sharifan et al., 2022). For example, a change of color to brownish-yellow due to the formation of Ag NPs is confirmed when a broad surface plasmon resonance band is observed around 450–470 nm, the peak absorption band for Cu NPs is obtained in the range of 550–600 nm, and the peak absorption band for ZnO NPs can be noticed between a range of 348 and 380 nm (Soniya et al., 2015; Lomate et al., 2018). This change in coloration is observed due to the surface plasmon vibrations in MNPs (Kowsalya et al., 2019). However, changing the composition of MNPs by adding them to another matrix may bring changes to its peak due to the surface plasmon resonance of MNPs. Ag NPs impart a peak at 420 nm when incorporated in the gel matrix of films (Bang et al., 2019). Due to the high concentration of NPs, it can show a secondary peak due to quadrupole resonance. Therefore, the peak width, peak wavelength, and secondary resonances form a unique spectral fingerprint for a particular NP. Hence, the peak intensity profile is considered an important characteristic of NPs, which is, in general, calculated using Scherer's formula (Shifa et al., 2019).
X-ray diffraction
This technique is used to assess the crystal structure and recognize coatings, stresses, lattice parameters, and crystallinity of NPs (Jayakumar et al., 2022). It works on the principle of Bragg's equation, which is a description of the reflection of the collided X-ray beam on a crystal plane of the target sample. It is based on wide-angle elastic scattering and is widely used in case of ordered crystalline materials. In this technique, a beam of X-ray is passed through the sample, which is simultaneously scattered/diffracted by the atoms/molecules present in the sample. This interference is observed by applying Bragg's law and a corresponding detector (Raval et al., 2018). Strong and distinctive diffraction couriers can be observed at different angles of the material sample, which corresponds to respective crystalline levels and gives the exact information about the formation and purity of the formed NPs. For example, strong couriers are observed at the 2θ angles of 38, 44, 64, and 77 degrees corresponding to the crystalline levels of 111, 200, 220, and 311, respectively, which confirms the presence of silver and also provides the information about the shape and structure of formed NP (Azari et al., 2020). Out of all peaks, the 2θ value at 38°, which corresponds to the 111 lattice plane, particularly, represents the formation of crystalline Ag NPs (Bang et al., 2019). In the case of Cu NPs, the characteristic peaks are observed at 43°, 50°, and 73°, which correspond to 111, 200, and 220 planes of the crystal structure of Cu NPs (Lomate et al., 2018; Sooch and Mann, 2021). A variety of peaks are observed owing to the difference in their crystallinity (Singh, 2022). For example, the ZnO–SiO2 nanocomposite possessing sharp and narrow peaks shows good crystallinity and also indicates the effects of different parameters on the nucleation (Al-Tayyar et al., 2020).
Scanning electron microscopy and transmission electron microscopy
These are widely used techniques to assess the shape, size, microstructures, and overall morphology of prepared NPs, and spherical and well-shaped particles are identified as good NPs in most instances. However, MgO NPs impart cubic morphology, which is an important characteristic of MgO NPs. Based on size and morphology, the level of agglomeration within NPs can be assessed (Al-Tayyar et al., 2020). Prepared NPs impart different colors and textures in the SEM image in the total matrix. For example, Ag NPs are seen as white dots in SEM images (Azari et al., 2020). The surface morphology of CuO and ZnO NPs is, in general, observed as roughly spherical in SEM images, while CuS NPs are observed as granular protrusions due to the aggregation of CuS NPs (Rasul et al., 2022). On the other hand, TEM is a more effective technique than SEM in terms of assessing morphology, size, composition, shape, crystal defects, surface structures, and electronic states of NPs. It assesses the shape and particle distribution of MNPs with a better resolution than SEM (Azari et al., 2020).
Electron paramagnetic resonance, energy-dispersive X-ray spectroscopy, and atomic force microscopy
Electron paramagnetic resonance is another type of spectroscopy that is used to confirm the superoxide generation during the formation of the NPs. Various reports concluded that during the formation of NPs, phenolic groups transfer electrons to O2, which carries out the reduction of NPs. It creates different peaks for different types of treatments, provides different peaks, and detects photogeneration of MNPs (Azari et al., 2020). Energy-dispersive X-ray spectroscopy assesses the presence and purity of formed NPs in packaging and films. It is used to investigate the dispersion of NPs in the nanocomposite, which demonstrates that MNPs are well distributed in the starch film matrix with no agglomeration (Peighambardoust et al., 2019). Atomic force microscopy is a powerful tool applied in investigating the fine structure information of food materials and molecular interaction at the nanoscale. AFM images of the sample reveal a distribution of extended chain-like molecules, directly visualizing a small number of branched macromolecules. AFM can be applied for nanorheological and nanotribological measurements of biopolymers (Rasul et al., 2022).
Antimicrobial activity of MNPs
Microbial contamination not only causes economic loss but also imparts a risk to human health. Therefore, there is an urge to develop safer antimicrobials to control foodborne microorganisms (Zhao et al., 2021). In recent years, nanomaterials have been developed and explored as promising antimicrobial agents to target drug-resistant microorganisms (Chaubey et al., 2017). The antimicrobial property of NPs depends on various factors, and they possess an efficient mechanism in microbial inactivation. NPs attach to the microbial cell wall and can easily pass through it. As a result, there could be possible damage to the cell membranes, and the cytoplasmic content may leak (Qiu et al., 2022). In addition, NPs can interact with other cellular structures and biomolecules (DNA) and subsequently affect the ATP synthesis machinery, which can result in cell apoptosis owing to DNA damage and lipid peroxidation (Tirado-Kulieva et al., 2022; Akyüz et al., 2023). NPs can also interact with biomolecules such as amino and carboxyl groups of peptidoglycan in the cell wall and generate oxidative stress, which hinders DNA replication and subsequently collapses the proton motive force across the cell membrane (Figure 2) (Lazić et al., 2020). The rate of inhibition of the growth of microbes by NPs is highly dependent on the particle size and the concentration of NPs (Videira-Quintela et al., 2021).
Among various types of metallic and metallic oxide NPs, Ag NPs are observed as the most potent antimicrobial agent due to their extremely small particle size, which result in efficient cell penetration; however, their application is limited due to a risk of potential toxicity to humans, particularly at high concentrations (Dehghani et al., 2021). On the other hand, Cu NPs also possess a good status as an antimicrobial as it possesses the ability to bind electrons; hence, it is capable of catalyzing oxidation and reduction reactions. An oxidized form of copper can interfere with the active site of enzymes, nucleic acids, and cell wall components, which causes cell death. Cu NPs are highly deteriorative against both Gram positive and Gram negative bacteria and also possess antifungal activity. Zn NPs have antimicrobial properties similar to Ag NPs and have advantages such as white appearance, low cost, and resistance to UV radiation in comparison to silver (Ebrahimi et al., 2019). Furthermore, TiO2 MNPs are used as a photocatalytic agent, which decays the microorganisms and certain organic molecules (Mesgari et al., 2021). These MNPs can generate reactive oxygen species in the presence of ultraviolet light, water, and oxygen molecules. As a result, the oxidation of the cellular plasma of microorganisms gets oxidized, causing cell death (Madhusha et al., 2021).
Applications of NPs in food and food products
Food demand is at its peak, which also induces food safety concerns. Nanotechnology is emerging as an innovative technology in the field of food and agriculture. Nanomaterials can be used in agrifood in several ways, such as crop improvement, protection against diseases, nanodevices in genetic engineering, plant disease diagnosis, etc. (Prasad et al., 2017). MNPs have broad applications in food systems, including food processing, preservation, and packaging (Singh et al., 2017) (Table 2). In preservation, nanopreservatives, nanoencapsulated food additives, and toxin detectors are involved. On the other hand, nanosensor preparation, nanocoating, edible coating of NPs, and nanocomposites are studied under food packaging applications (Dikshit et al., 2021). The application of MNPs in polymers, which are applied on the surface of food products, extends the shelf life of foods by slowing down the enzymatic processes concerning postharvest ripening and restricting the development of many physiological diseases (Rodino et al., 2019). For the preservation and enhancing the shelf life of soft fruits, the application of NPs in the food sector can be mainly divided into two groups, one is food nanosensing and the other is food nanostructuring. In food processing, food nanostructures can be used as food additives that carry anticaking agents, antimicrobial agents, and nutrient delivery systems, and used for the durability of the packaging materials; on the other hand, food nanosensing is used for better food quality and safety evaluation of foods (Singh et al., 2017). Foodborne pathogens can cause fatalities, and this further increases the resistance of microbial strains (Ahmed and Al-Zubaidy, 2020). In this concern, MNPs play an important role in detection and control. The MNPs are also reported to develop semiconductors cheaply and efficiently, which can be useful to enhance the conductivity of sensors (dos Santos et al., 2020). These sensors can also monitor the temperature and pH of agrifoods to prevent microbial contamination (Powell and Kanarek, 2006).
The major application of MNPs is to improve the functionality of packaging material, especially biodegradable films. MNPs including silver, copper oxide, and zinc oxide significantly improve the performance of biodegradable films due to their large surface area and antimicrobial activity against a range of microbes (e.g., fungi, bacteria, and molds) (Ahari et al., 2021). However, MNPs are an expensive affair, and their injudicious use may cause toxicity, which limits their application in food packaging systems (Rai et al., 2019). Second, MNPs are used at lower concentrations, and none of the antimicrobial agents is effective against all pathogens at lower concentrations (Kumar et al., 2021). Hence, a combined treatment of multiple MNPs is recommended to obtain effective results against microbes (Zhai et al., 2022). Another aspect of MNPs in food packaging is to improve the mechanical properties of packaging films. For instance, starch-based materials are better at degradation properties but worst at mechanical properties and water-holding characteristics (Zare et al., 2019). MNPs impart good mechanical properties even at very lower levels (< 5%). Improvement in these film properties is owing to their high surface ratio and uniform distribution of MNPs (Abdolsattari et al., 2020). In some reports, SiO2 and ZnO NPs are proven to provide better mechanical strength and warm dependability to plastic films (Sothornvit, 2019). Especially, SiO2 NPs are supposed to be more suitable for this particular purpose due to their higher surface movements, which enable them to assimilate different atoms. These two are also approved by the FDA under recommended limited proportions (Al-Tayyar et al., 2020). In addition, these MNPs enhance the gas barrier properties of polymers, which ultimately aims at enhancing the shelf life of food (Chadha et al., 2022).
Nanomaterials are also highly promising in plant protection induced by the mechanism of genetic modification of plants to induce disease resistance (Nair et al., 2010). On the other hand, nanoencapsulation is considered more efficient and safer concerning the handling of fertilizers, pesticides, and vaccines (Yaktine and Pray, 2009). Macro- and micronutrients possess very low bioavailability; therefore, we can enhance their bioavailability by decreasing their particle size and increasing their surface area, which increases their absorption rates in the digestive system (Sonkaria et al., 2012). For example, nano drops are used in canola oil, which encapsulates minerals, vitamins, and phytochemicals and passes them through the digestive system (Chu et al., 2019). Iron deficiency is the most common deficiency, which causes anemia (Tkaczyszyn et al., 2018). A major possibility to combat this situation is fortification; however, it seriously alters the sensorial characteristics of food. On the other hand, nanoiron was found not to alter the sensorial properties of food (Zimmermann et al., 2007).
Toxicity and health concerns of MNPs
Currently, advanced nanomaterial innovations in food applications are adding new possibilities for improving food quality. However, a major drawback of these NPs is toxicity, which can be caused by their injudicious use. These can pose danger to both environments and human health (Mathew and Radhakrishnan, 2022). Both inorganic and organic MNPs are applied in nanofood and packaging systems, with the main aim to enhance the shelf life by providing antimicrobial protection against food pathogens; however, there is a chance of migration of these MNPs into food and subsequently to the human body, which later on tend to accumulate (Chadha et al., 2022; Mallia et al., 2022). The potential toxicologic effects of MNPs on human health have been widely studied by researchers using in vitro and in vivo approaches (Couto and Almeida, 2022). The effect certainly depends on the type or nature of the packaging matrix, degree of migration, toxicity of the used nanomaterial, and uptake rate of the particular food. Higher amounts of such compounds absorbed through the skin pose a major risk to human health, particularly in terms of long-term toxicity (Sahoo et al., 2022). These NPs are reported to build up in several organs, including the stomach, small intestine, kidneys, liver, and spleen (McClements and Xiao, 2017). Several reports are available on the toxicity of NPs (Table 3) to humans, such as kidney damage, lung damage, and hepatic injury, which could occur due to the intake of NPs (Mathew and Radhakrishnan, 2022).
Currently, researchers are more focused on the fast development and applications of nanotechnology due to its attractive impact without concerning its toxicology (Mathew and Radhakrishnan, 2022). Nevertheless, there is a requirement for validated proof regarding the interaction between NPs and cells or tissues, particularly concerning possible threats to human health. Most importantly, NP synthesis by various chemical approaches has negative consequences and produces harmful by-products, which cause severe environmental pollution (Khalil et al., 2021). In addition, regulatory policies, risk assessment programs, and biosafety concerns must be taken into consideration during the processing, packaging, and consumption of nano-based food products (Bajpai et al., 2018).
Many regulatory agencies, including the FDA, USEPA, and IFAS, have initiated protocols to deal with the potential risks related to the use of NPs and nano-based products. Since 2006, the FDA has been working to identify sources of nanomaterials, estimating the environmental impact of nanomaterials and their risks on human, animal, and plant health (Jeevanandam et al., 2018). The FDA constituted a Nanotechnology Task Force in 2006, which is charged with developing supervisory approaches to nano-based products that will ensure safety and efficacy and also help in beneficial technological innovation (Zabihzadeh Khajavi et al., 2019). The FDA, the EU, and other international regulatory authorities provide detailed guidance and information to evaluate the safety of NPs applied in food packaging, and also on the development of standardized procedures to analyze the risk of NPs on human health and on the environment (Kumar et al., 2022).
Conclusion
In the present review, we have studied that MNPs have a wider range of applications in food processing and preservation, such as targeted delivery of nutrients, increased absorption, packaging to extend shelf life, sensors to improve food safety, and antimicrobials to inactivate microorganisms. These materials can also improve product texture, flavor, and composition. Among aforesaid applications, they are vitally used in the food packaging matrix due to their efficient interaction with packaging materials. They impart antimicrobial and enhanced functional properties of packaging films. We have also used modern tools to monitor the synthesis and stability of nanoparticles, which, in turn, are very important to assess the information about the formation and properties of MNPs. In addition to their major applications, they possess potential risks to human health owing to their toxicity and their absorption in the human body. Researchers are developing these materials without evaluating the risk associated with these NPs. Innovation without precaution is not beneficial to society; also, it is deleterious to the human race. Future trends in NP development should be in line with the toxicological evaluation and risk assessment of NPs.
Author contributions
Conceptualization and writing-original draft preparation: AnK, AbK, and CV. Review and editing: PS, PC, RC, and KC. Figures creation: VK and Abhineet. All authors have read and approved it for publication.
Conflict of interest
The authors declare that the research was conducted in the absence of any commercial or financial relationships that could be construed as a potential conflict of interest.
Publisher's note
All claims expressed in this article are solely those of the authors and do not necessarily represent those of their affiliated organizations, or those of the publisher, the editors and the reviewers. Any product that may be evaluated in this article, or claim that may be made by its manufacturer, is not guaranteed or endorsed by the publisher.
References
Abdolsattari, P., Peighambardoust, S. H., Pirsa, S., Peighambardoust, S. J., and Fasihnia, S. H. (2020). Investigating microbial properties of traditional Iranian white cheese packed in active LDPE films incorporating metallic and organoclay nanoparticles. Chem Rev Lett 3, 168–174. doi: 10.22034/crl.2020.231587.1059
Adewale, O. B., Egbeyemi, K. A., Onwuelu, J. O., Potts-Johnson, S. S., Anadozie, S. O., Fadaka, A. O., et al. (2020). Biological synthesis of gold and silver nanoparticles using leaf extracts of Crassocephalum rubens and their comparative in vitro antioxidant activities. Heliyon 6, e05501. doi: 10.1016/j.heliyon.2020.e05501
Ahari, H., Anvar, A. A., Ataee, M., and Naeimabadi, M. (2021). Employing nanosilver, nanocopper, and nanoclays in food packaging production: a systematic review. Coatings 11, 1–29. doi: 10.3390/coatings11050509
Ahmed, H. M., and Al-Zubaidy, A. M. A. (2020). Exploring natural essential oil components and antibacterial activity of solvent extracts from twelve Perilla frutescens L. Genotypes. Arab. J. Chem. 13, 7390–7402. doi: 10.1016/j.arabjc.2020.08.016
Ajarem, J. S., Maodaa, S. N., Allam, A. A., Taher, M. M., and Khalaf, M. (2022). Benign synthesis of cobalt oxide nanoparticles containing red algae extract: antioxidant, antimicrobial, anticancer, and anticoagulant activity. J. Clust. Sci. 33, 717–728. doi: 10.1007/s10876-021-02004-9
Ajdary, M., Moosavi, M. A., Rahmati, M., Falahati, M., Mahboubi, M., Mandegary, A., et al. (2018). Health concerns of various nanoparticles: A review of their in vitro and in vivo toxicity. Nanomaterials 8. doi: 10.3390/nano8090634
Akbarzadeh, R., and Dehghani, H. (2017). Sodium-dodecyl-sulphate-assisted synthesis of Ni nanoparticles: electrochemical properties. Bull. Mater. Sci. 40, 1361–1369. doi: 10.1007/s12034-017-1500-3
Akyüz, G., Kaymazlar, E., Ay, H., Andaç, M., and Andaç, Ö. (2023). Use of silver nanoparticles loaded locust bean gum coatings to extend the shelf-life of fruits. Biointerface Res. Appl. Chem. 13, 1–16. doi: 10.33263/BRIAC133.289
Al'Abri, A. M., Abdul Halim, S. N., Abu Bakar, N. K., Saharin, S. M., Sherino, B., Rashidi Nodeh, H., et al. (2019). Highly sensitive and selective determination of malathion in vegetable extracts by an electrochemical sensor based on Cu-metal organic framework. J. Environ. Sci. Heal. - Part B Pestic. Food Contam. Agric. Wastes 54, 930–941. doi: 10.1080/03601234.2019.1652072
Albelda, J. A. V., Uzunoglu, A., Santos, G. N. C., and Stanciu, L. A. (2017). Graphene-titanium dioxide nanocomposite based hypoxanthine sensor for assessment of meat freshness. Biosens. Bioelectron. 89, 518–524. doi: 10.1016/j.bios.2016.03.041
Alsamhary, K. I. (2020). Eco-friendly synthesis of silver nanoparticles by Bacillus subtilis and their antibacterial activity. Saudi J. Biol. Sci. 27, 2185–2191. doi: 10.1016/j.sjbs.2020.04.026
Al-Tayyar, N. A., Youssef, A. M., and Al-Hindi, R. R. (2020). Antimicrobial packaging efficiency of ZnO-SiO2 nanocomposites infused into PVA/CS film for enhancing the shelf life of food products. Food Packag. Shelf Life 25, 100523. doi: 10.1016/j.fpsl.2020.100523
Andrievski, R. A. (2014). Review of thermal stability of nanomaterials. J. Mater. Sci. 49, 1449–1460. doi: 10.1007/s10853-013-7836-1
Azari, A., Anvar, S. A. A., Ahari, H., Sharifan, A., and Motallebi Moghanjoghi, A. A. (2020). Study on Nanosilver-TiO2 photocatalytic nanocomposite coating with extrusion technique for increasing shelf life of Nile Tilapia (Oreochromis niloticus). Iran. J. Fish. Sci. 19, 2618–2633. doi: 10.22092/ijfs.2020.122566
Badawy, M. E. I., Lotfy, T. M. R., and Shawir, S. M. S. (2019). Preparation and antibacterial activity of chitosan-silver nanoparticles for application in preservation of minced meat. Bull. Natl. Res. Cent. 43, 1–14. doi: 10.1186/s42269-019-0124-8
Bajpai, V. K., Kamle, M., Shukla, S., Mahato, D. K., Chandra, P., Hwang, S. K., et al. (2018). Prospects of using nanotechnology for food preservation, safety, and security. J. Food Drug Anal. 26, 1201–1214. doi: 10.1016/j.jfda.2018.06.011
Bang, Y. J., Shankar, S., and Rhim, J. W. (2019). In situ synthesis of multi-functional gelatin/resorcinol/silver nanoparticles composite films. Food Packag. Shelf Life 22, 100399. doi: 10.1016/j.fpsl.2019.100399
Banne, S. V., Patil, M. S., Kulkarni, R. M., and Patil, S. J. (2017). Synthesis and Characterization of Silver Nano Particles for EDM Applications. Mater. Today Proc. 4, 12054–12060. doi: 10.1016/j.matpr.2017.09.130
Bittar, D. B., Catelani, T. A., Nigoghossian, K., Barud, H. da S., Ribeiro, S. J. L., Pezza, L., et al. (2017). Optimized synthesis of silver nanoparticles by factorial design with application for the determination of melamine in milk. Anal. Lett. 50, 829–841. doi: 10.1080/00032719.2016.1196213
Bouafia, A., Laouini, S. E., Tedjani, M. L., Ali, G. A. M., and Barhoum, A. (2022). Green biosynthesis and physicochemical characterization of Fe3O4 nanoparticles using Punica granatum L. fruit peel extract for optoelectronic applications. Text. Res. J. 92, 2685–2696. doi: 10.1177/00405175211006671
Camas, M., Sazak Camas, A., and Kyeremeh, K. (2018). Extracellular synthesis and characterization of gold nanoparticles using mycobacterium sp. BRS2A-AR2 isolated from the aerial roots of the ghanaian mangrove plant, rhizophora racemosa. Indian J. Microbiol. 58, 214–221. doi: 10.1007/s12088-018-0710-8
Chadha, U., Bhardwaj, P., Selvaraj, S. K., Arasu, K., Praveena, S., Pavan, A., et al. (2022). Current trends and future perspectives of nanomaterials in food packaging application. J. Nanomater. 2022, 1–32. doi: 10.1155/2022/2745416
Chaicherd, S., Killingsworth, M. C., and Pissuwan, D. (2019). Toxicity of gold nanoparticles in a commercial dietary supplement drink on connective tissue fibroblast cells. SN Appl. Sci. 1, 1–8. doi: 10.1007/s42452-019-0354-2
Chaubey, K. K., Singh, S. V., Gupta, S., Singh, M., Sohal, J. S., Kumar, N., et al. (2017). Mycobacterium avium subspecies paratuberculosis – An important food borne pathogen of high public health significance with special reference to india: An update. Vet. Q. 37, 282–299. doi: 10.1080/01652176.2017.1397301
Chi, H., Song, S., Luo, M., Zhang, C., Li, W., Li, L., et al. (2019). Effect of PLA nanocomposite films containing bergamot essential oil, TiO 2 nanoparticles, and Ag nanoparticles on shelf life of mangoes. Sci. Hortic. (Amsterdam). 249, 192–198. doi: 10.1016/j.scienta.2019.01.059
Chopra, H., Bibi, S., Singh, I., Hasan, M. M., Khan, M. S., Yousafi, Q., et al. (2022). Green metallic nanoparticles: biosynthesis to applications. Front. Bioeng. Biotechnol. 10:548. doi: 10.3389/fbioe.2022.874742
Chu, Y., Jing, S., Liu, D., Liu, J., and Zhao, Y. (2019). Morphological control and kinetics in three dimensions for hierarchical nanostructures growth by screw dislocations. Acta Mater. 162, 284–291. doi: 10.1016/j.actamat.2018.10.007
Couto, C., and Almeida, A. (2022). Metallic nanoparticles in the food sector: a mini-review. Foods 11, 402. doi: 10.3390/foods11030402
Cuenya, B. R. (2010). Synthesis and catalytic properties of metal nanoparticles: size, shape, support, composition, and oxidation state effects. Thin Solid Films 518, 3127–3150. doi: 10.1016/j.tsf.2010.01.018
Cunha, F. A., Cunha, M. da C. S. O., da Frota, S. M., Mallmann, E. J. J., Freire, T. M., Costa, L. S., et al. (2018). Biogenic synthesis of multifunctional silver nanoparticles from Rhodotorula glutinis and Rhodotorula mucilaginosa: antifungal, catalytic and cytotoxicity activities. World J. Microbiol. Biotechnol. 34, 1–15. doi: 10.1007/s11274-018-2514-8
Dairi, N., Ferfera-Harrar, H., Ramos, M., and Garrigós, M. C. (2019). Cellulose acetate/AgNPs-organoclay and/or thymol nano-biocomposite films with combined antimicrobial/antioxidant properties for active food packaging use. Int. J. Biol. Macromol. 121, 508–523. doi: 10.1016/j.ijbiomac.2018.10.042
Dehghan, Z., Ranjbar, M., Govahi, M., and Khakdan, F. (2022). Green synthesis of Ag/Fe3O4 nanocomposite utilizing Eryngium planum L. leaf extract and its potential applications in medicine. J. Drug Deliv. Sci. Technol. 67, 102941. doi: 10.1016/j.jddst.2021.102941
Dehghani, S., Peighambardoust, S. H., Peighambardoust, S. J., Fasihnia, S. H., Khosrowshahi, N. K., Gullón, B., et al. (2021). Optimization of the amount of ZnO, CuO, and Ag nanoparticles on antibacterial properties of Low-Density Polyethylene (LDPE) films using the response surface method. Food Anal. Methods 14, 98–107. doi: 10.1007/s12161-020-01856-7
Dikshit, P. K., Kumar, J., Das, A. K., Sadhu, S., Sharma, S., Singh, S., et al. (2021). Green synthesis of metallic nanoparticles: applications and limitations. Catalysts 11, 902. doi: 10.3390/catal11080902
Din, M. I., Nabi, A. G., Rani, A., Aihetasham, A., and Mukhtar, M. (2018). Single step green synthesis of stable nickel and nickel oxide nanoparticles from Calotropis gigantea: Catalytic and antimicrobial potentials. Environ. Nanotechnology, Monit. Manag. 9, 29–36. doi: 10.1016/j.enmm.2017.11.005
dos Santos, C. A., Ingle, A. P., and Rai, M. (2020). The emerging role of metallic nanoparticles in food. Appl. Microbiol. Biotechnol,. 104, 2373–2383. doi: 10.1007/s00253-020-10372-x
Dutra-Correa, M., Leite, A. A. B. V., de Cara, S. P. H. M., Diniz, I. M. A., Marques, M. M., Suffredini, I. B., et al. (2018). Antibacterial effects and cytotoxicity of an adhesive containing low concentration of silver nanoparticles. J. Dent. 77, 66–71. doi: 10.1016/j.jdent.2018.07.010
Ebrahimi, Y., Peighambardoust, S. J., Peighambardoust, S. H., and Karkaj, S. Z. (2019). Development of antibacterial carboxymethyl cellulose-based nanobiocomposite films containing various metallic nanoparticles for food packaging applications. J. Food Sci. 84, 2537–2548. doi: 10.1111/1750-3841.14744
El Domany, E. B., Essam, T. M., Ahmed, A. E., and Farghali, A. A. (2018). Biosynthesis physico-chemical optimization of gold nanoparticles as anti-cancer and synergetic antimicrobial activity using Pleurotus ostreatus fungus. J. Appl. Pharm. Sci. 8, 119–128. doi: 10.7324/JAPS.2018.8516
Enescu, D., Dehelean, A., Gonçalves, C., Cerqueira, M. A., Magdas, D. A., Fucinos, P., et al. (2020). Evaluation of the specific migration according to EU standards of titanium from Chitosan/Metal complexes films containing TiO2 particles into different food simulants. A comparative study of the nano-sized vs micro-sized particles. Food Packag. Shelf Life 26, 579. doi: 10.1016/j.fpsl.2020.100579
Eramabadi, P., Masoudi, M., Makhdoumi, A., and Mashreghi, M. (2020). Microbial cell lysate supernatant (CLS) alteration impact on platinum nanoparticles fabrication, characterization, antioxidant and antibacterial activity. Mater. Sci. Eng. C. 117, 111292. doi: 10.1016/j.msec.2020.111292
Fadiji, A. E., Mthiyane, D. M. N., Onwudiwe, D. C., and Babalola, O. O. (2022). Harnessing the known and unknown impact of nanotechnology on enhancing food security and reducing postharvest losses: constraints and future prospects. Agronomy 12, 1657. doi: 10.3390/agronomy12071657
Fierascu, I., Fierascu, I. C., Dinu-Pirvu, C. E., Fierascu, R. C., Anuta, V., Velescu, B. S., et al. (2019). A short overview of recent developments on antimicrobial coatings based on phytosynthesized metal nanoparticles. Coatings 9, 1–25. doi: 10.3390/coatings9120787
Francis, D. V., Thaliyakattil, S., Cherian, L., Sood, N., and Gokhale, T. (2022). Metallic nanoparticle integrated ternary polymer blend of PVA/Starch/Glycerol: a promising antimicrobial food packaging material. Polymers (Basel). 14:1379. doi: 10.3390/polym14071379
Ganesan, R. M., and Gurumallesh Prabu, H. (2019). Synthesis of gold nanoparticles using herbal Acorus calamus rhizome extract and coating on cotton fabric for antibacterial and UV blocking applications. Arab. J. Chem. 12, 2166–2174. doi: 10.1016/j.arabjc.2014.12.017
Gülseren, O., Ercolessi, F., and Tosatti, E. (1995). Premelting of thin wires. Phys. Rev. B 51, 7377–7380. doi: 10.1103/PhysRevB.51.7377
Gupta, R., and Xie, H. (2018). Nanoparticles in daily life: applications, toxicity and regulations. J. Environ. Pathol. Toxicol. Oncol. 37, 209–230. doi: 10.1615/JEnvironPatholToxicolOncol.2018026009
Hassan, S. E. D., Fouda, A., Radwan, A. A., Salem, S. S., Barghoth, M. G., Awad, M. A., et al. (2019). Endophytic actinomycetes Streptomyces spp mediated biosynthesis of copper oxide nanoparticles as a promising tool for biotechnological applications. J. Biol. Inorg. Chem. 24, 377–393. doi: 10.1007/s00775-019-01654-5
Henry, C. R. (2005). Morphology of supported nanoparticles. Prog. Surf. Sci. 80, 92–116. doi: 10.1016/j.progsurf.2005.09.004
Huq, M. A., and Akter, S. (2021). Bacterial mediated rapid and facile synthesis of silver nanoparticles and their antimicrobial efficacy against pathogenic microorganisms. Materials (Basel). 14, 2615. doi: 10.3390/ma14102615
Iderawumi, A. M., and Yusuff, M. A. (2021). Effects of nanoparticles on improvement in quality and shelf life of fruits and vegetables. J. Plant Biol. Crop Res. 4, 2–7.
Ijaz, M., Zafar, M., Afsheen, S., and Iqbal, T. (2020). A review on Ag-nanostructures for enhancement in shelf time of fruits. J. Inorg. Organomet. Polym. Mater. 30, 1475–1482. doi: 10.1007/s10904-020-01504-x
Ilahi, N., Haleem, A., Iqbal, S., Fatima, N., Sajjad, W., Sideeq, A., et al. (2022). Biosynthesis of silver nanoparticles using endophytic Fusarium oxysporum strain NFW16 and their in vitro antibacterial potential. Microsc. Res. Tech. 85, 1568-1579. doi: 10.1002/jemt.24018
Jayakumar, A., Radoor, S., Kim, J. T., Rhim, J. W., Nandi, D., Parameswaranpillai, J., et al. (2022). Recent innovations in bionanocomposites-based food packaging films – a comprehensive review. Food Packag. Shelf Life 33, 100877. doi: 10.1016/j.fpsl.2022.100877
Jeevanandam, J., Barhoum, A., Chan, Y. S., Dufresne, A., and Danquah, M. K. (2018). Review on nanoparticles and nanostructured materials: history, sources, toxicity and regulations. Beilstein J. Nanotechnol. 9, 1050–1074. doi: 10.3762/bjnano.9.98
Jiang, X., Wu, Y., Gray, P., Zheng, J., Cao, G., Zhang, H., et al. (2018). Influence of gastrointestinal environment on free radical generation of silver nanoparticles and implications for their cytotoxicity. NanoImpact. 10, 144–152. doi: 10.1016/j.impact.2018.04.001
Joseph Kirubaharan, C., Fang, Z., Sha, C., and Yong, Y. C. (2020). Green synthesis of Ag and Pd nanoparticles for water pollutants treatment. Water Sci. Technol. 82, 2344–2352. doi: 10.2166/wst.2020.498
Kadam, D., Momin, B., Palamthodi, S., and Lele, S. S. (2019). Physicochemical and functional properties of chitosan-based nano-composite films incorporated with biogenic silver nanoparticles. Carbohydr. Polym. 211, 124–132. doi: 10.1016/j.carbpol.2019.02.005
Kambale, E. K., Nkanga, C. I., Mutonkole, B. P. I., Bapolisi, A. M., Tassa, D. O., Liesse, J. M. I., et al. (2020). Green synthesis of antimicrobial silver nanoparticles using aqueous leaf extracts from three Congolese plant species (Brillantaisia patula, Crossopteryx febrifuga and Senna siamea). Heliyon 6, e04493. doi: 10.1016/j.heliyon.2020.e04493
Kanimozhi, S., Durga, R., Sabithasree, M., Kumar, A. V., Sofiavizhimalar, A., Kadam, A. A., et al. (2022). Biogenic synthesis of silver nanoparticle using Cissus quadrangularis extract and its invitro study. J. King Saud Univ. - Sci. 34, 101930. doi: 10.1016/j.jksus.2022.101930
Katata-Seru, L., Moremedi, T., Aremu, O. S., and Bahadur, I. (2018). Green synthesis of iron nanoparticles using Moringa oleifera extracts and their applications: Removal of nitrate from water and antibacterial activity against Escherichia coli. J. Mol. Liq. 256, 296–304. doi: 10.1016/j.molliq.2017.11.093
Kavakebi, E., Anvar, A., Ahari, H., and Motalebi, A. (2021). The effect of PVA coated film containing silver nanoparticles synthesized from aqueous Satureja rechingeri extract on shelf life of rainbow trout (Oncorhynchus mykiss) fillet. J. Food Bioprocess Eng. 4, 135–145. doi: 10.22059/jfabe.2021.325907.1094
Khalil, A. T., Iqbal, J., Shah, A., Haque, M. Z., Khan, I., Ayaz, M., et al. (2021). The bio-nano interface as an emerging trend in assembling multi-functional metal nanoparticles. SPR Nanosci. 7, 1–24. doi: 10.1039/9781839163791-00001
Khani, R., Roostaei, B., Bagherzade, G., and Moudi, M. (2018). Green synthesis of copper nanoparticles by fruit extract of Ziziphus spina-christi (L.) Willd.: Application for adsorption of triphenylmethane dye and antibacterial assay. J. Mol. Liq. 255, 541–549. doi: 10.1016/j.molliq.2018.02.010
Khanna, P., Kaur, A., and Goyal, D. (2019). Algae-based metallic nanoparticles: synthesis, characterization and applications. J. Microbiol. Methods 163, 105656. doi: 10.1016/j.mimet.2019.105656
Kora, A. J., and Rastogi, L. (2018). Green synthesis of palladium nanoparticles using gum ghatti (Anogeissus latifolia) and its application as an antioxidant and catalyst. Arab. J. Chem. 11, 1097–1106. doi: 10.1016/j.arabjc.2015.06.024
Kowsalya, E., Mosachristas, K., Balashanmugam, P., Tamilselvi, A., and Rani, J. C. (2019). Biocompatible silver nanoparticles/poly(vinyl alcohol) electrospun nanofibers for potential antimicrobial food packaging applications. Food Packag. Shelf Life 21, 100379. doi: 10.1016/j.fpsl.2019.100379
Kumar, S., Basumatary, I. B., Sudhani, H. P. K., Bajpai, V. K., Chen, L., Shukla, S., et al. (2021). Plant extract mediated silver nanoparticles and their applications as antimicrobials and in sustainable food packaging: a state-of-the-art review. Trends Food Sci. Technol. 112, 651–666. doi: 10.1016/j.tifs.2021.04.031
Kumar, S., Mudai, A., Roy, B., Basumatary, I. B., Mukherjee, A., and Dutta, J. (2020). Biodegradable hybrid nanocomposite of chitosan/gelatin and green synthesized zinc oxide nanoparticles for food packaging. Foods 9:1143. doi: 10.3390/foods9091143
Kumar, S., Mukherjee, A., Kalita, S., Singh, N., Katiyar, V., Mitra, A., et al. (2022). Nanomaterials in food packaging. Biopolym. Food Packag., 336–367. doi: 10.1002/9781119702313.ch10
Lazić, V., Vivod, V., Peršin, Z., Stoiljković, M., Ratnayake, I. S., Ahrenkiel, P. S., et al. (2020). Dextran-coated silver nanoparticles for improved barrier and controlled antimicrobial properties of nanocellulose films used in food packaging. Food Packag. Shelf Life 26:100575. doi: 10.1016/j.fpsl.2020.100575
Lebaschi, S., Hekmati, M., and Veisi, H. (2017). Green synthesis of palladium nanoparticles mediated by black tea leaves (Camellia sinensis) extract: Catalytic activity in the reduction of 4-nitrophenol and Suzuki-Miyaura coupling reaction under ligand-free conditions. J. Colloid Interface Sci. 485, 223–231. doi: 10.1016/j.jcis.2016.09.027
Li, L., Zhao, C., Zhang, Y., Yao, J., Yang, W., Hu, Q., et al. (2017). Effect of stable antimicrobial nano-silver packaging on inhibiting mildew and in storage of rice. Food Chem. 215, 477–482. doi: 10.1016/j.foodchem.2016.08.013
Lian, S., Diko, C. S., Yan, Y., Li, Z., Zhang, H., Ma, Q., et al. (2019). Characterization of biogenic selenium nanoparticles derived from cell-free extracts of a novel yeast Magnusiomyces ingens. 3 Biotech 9, 1–8. doi: 10.1007/s13205-019-1748-y
Liang, T., Qiu, X., Ye, X., Liu, Y., Li, Z., Tian, B., et al. (2020). Biosynthesis of selenium nanoparticles and their effect on changes in urinary nanocrystallites in calcium oxalate stone formation. 3 Biotech 10, 1–6. doi: 10.1007/s13205-019-1999-7
Liao, C., Li, Y., and Tjong, S. C. (2019). Bactericidal and cytotoxic properties of silver nanoparticles. Int. J. Mol. Sci. 20, 449. doi: 10.3390/ijms20020449
Lin, Z., Weng, X., Owens, G., and Chen, Z. (2020). Simultaneous removal of Pb(II) and rifampicin from wastewater by iron nanoparticles synthesized by a tea extract. J. Clean. Prod. 242, 118476. doi: 10.1016/j.jclepro.2019.118476
Lomate, G. B., Dandi, B., and Mishra, S. (2018). Development of antimicrobial LDPE/Cu nanocomposite food packaging film for extended shelf life of peda. Food Packag. Shelf Life 16, 211–219. doi: 10.1016/j.fpsl.2018.04.001
Lopez-Chaves, C., Soto-Alvaredo, J., Montes-Bayon, M., Bettmer, J., Llopis, J., and Sanchez-Gonzalez, C. (2018). Gold nanoparticles: Distribution, bioaccumulation and toxicity. In vitro and in vivo studies. Nanomed. Nanotechnol. Biol. Med. 14, 1–12. doi: 10.1016/j.nano.2017.08.011
Lotha, R., Shamprasad, B. R., Sundaramoorthy, N. S., Nagarajan, S., and Sivasubramanian, A. (2019). Biogenic phytochemicals (cassinopin and isoquercetin)capped copper nanoparticles (ISQ/CAS@CuNPs)inhibits MRSA biofilms. Microb. Pathog. 132, 178–187. doi: 10.1016/j.micpath.2019.05.005
Loza, K., Heggen, M., and Epple, M. (2020). Synthesis, structure, properties, and applications of bimetallic nanoparticles of noble metals. Adv. Funct. Mater. 30:1909260. doi: 10.1002/adfm.201909260
Luzala, M. M., Muanga, C. K., Kyana, J., Safari, J. B., Zola, E. N., Mbusa, G. V., et al. (2022). A critical review of the antimicrobial and antibiofilm activities of green-synthesized plant-based metallic nanoparticles. Nanomaterials 12, 1841. doi: 10.3390/nano12111841
Madhavan, A. A., Qotainy, R., and Nair, R. (2019). Synthesis of functionalized silver nanoparticles and its application as chemical sensor. 2019 Adv. Sci. Eng. Technol. Int. Conf. ASET 2019. doi: 10.1109/ICASET.2019.8714300
Madhusha, C., Munaweera, I., and Kottegoda, N. (2021). Functional nanomaterials as smart food packaging: A brief review. African J. Agric. Food Sci. 4, 58–78. Available online at: https://abjournals.org/ajafs/wp-content/uploads/sites/16/journal/published_paper/volume-4/issue-1/AJAFS_RB6P4LAU.pdf
Mallia, J. D. O., Galea, R., Nag, R., Cummins, E., Gatt, R., and Valdramidis, V. (2022). Nanoparticle food applications and their toxicity: current trends and needs in risk assessment strategies. J. Food Prot. 85, 355–372. doi: 10.4315/JFP-21-184
Mathew, S., and Radhakrishnan, E. K. (2022). Health and safety issues of nanotechnology in food applications. Nano-Innov. Food Packag. (Boca Raton: Apple Academic Press) 247–276. doi: 10.1201/9781003277422-12
Mathew, S., S, S., Mathew, J., and Radhakrishnan, E. K. (2019). Biodegradable and active nanocomposite pouches reinforced with silver nanoparticles for improved packaging of chicken sausages. Food Packag. Shelf Life 19, 155–166. doi: 10.1016/j.fpsl.2018.12.009
McClements, D. J., and Xiao, H. (2017). Is nano safe in foods? Establishing the factors impacting the gastrointestinal fate and toxicity of organic and inorganic food-grade nanoparticles. npj Sci. Food 1, 1–13. doi: 10.1038/s41538-017-0005-1
Menon, S., Shrudhi, S. D., Agarwal, H., and Shanmugam, V. K. (2019). Efficacy of biogenic selenium nanoparticles from an extract of ginger towards evaluation on anti-microbial and anti-oxidant activities. Colloids Interface Sci. Commun. 29, 1–8. doi: 10.1016/j.colcom.2018.12.004
Mesgari, M., Aalami, A. H., and Sahebkar, A. (2021). Antimicrobial activities of chitosan/titanium dioxide composites as a biological nanolayer for food preservation: a review. Int. J. Biol. Macromol. 176, 530–539. doi: 10.1016/j.ijbiomac.2021.02.099
Mohamed, M. A. (2020). Myco-engineered gold nanoparticles from Jahnula aquatica coated with ampicillin/amoxicillin and their antibacterial and anticancer activity against cancer cells. Biotechnol. Lett. 42, 151–170. doi: 10.1007/s10529-019-02764-5
Monowar, T., Rahman, S., Bhore, S. J., Raju, G., and Sathasivam, K. V. (2018). Silver nanoparticles synthesized by using the endophytic bacterium pantoea ananatis are promising antimicrobial agents against multidrug resistant bacteria. Molecules 23, 3220. doi: 10.3390/molecules23123220
Motahharifar, N., Nasrollahzadeh, M., Taheri-Kafrani, A., Varma, R. S., and Shokouhimehr, M. (2020). Magnetic chitosan-copper nanocomposite: A plant assembled catalyst for the synthesis of amino- and N-sulfonyl tetrazoles in eco-friendly media. Carbohydr. Polym. 232, 115819. doi: 10.1016/j.carbpol.2019.115819
Mottet, C., Rossi, G., Baletto, F., and Ferrando, R. (2005). Single impurity effect on the melting of nanoclusters. Phys. Rev. Lett. 95:035501. doi: 10.1103/PhysRevLett.95.035501
Mukherjee, A., Sarkar, D., and Sasmal, S. (2021). A review of green synthesis of metal nanoparticles using algae. Front. Microbiol. 12:693899. doi: 10.3389/fmicb.2021.693899
Musa, S. F., Yeat, T. S., Kamal, L. Z. M., Tabana, Y. M., Ahmed, M. A., El Ouweini, A., et al. (2018). Pleurotus sajor-caju can be used to synthesize silver nanoparticles with antifungal activity against Candida albicans. J. Sci. Food Agric. 98, 1197–1207. doi: 10.1002/jsfa.8573
Musial, J., Krakowiak, R., Mlynarczyk, D. T., Goslinski, T., and Stanisz, B. J. (2020). Titanium dioxide nanoparticles in food and personal care products-what do we know about their safety? Nanomaterials 10, 1–23. doi: 10.3390/nano10061110
Nair, R., Varghese, S. H., Nair, B. G., Maekawa, T., Yoshida, Y., and Kumar, D. S. (2010). Nanoparticulate material delivery to plants. Plant Sci. 179, 154–163. doi: 10.1016/j.plantsci.2010.04.012
Nasrollahzadeh, M., Issaabadi, Z., and Sajadi, S. M. (2018). Green synthesis of Pd/Fe3O4 nanocomposite using Hibiscus tiliaceus L. extract and its application for reductive catalysis of Cr(VI) and nitro compounds. Sep. Purif. Technol. 197, 253–260. doi: 10.1016/j.seppur.2018.01.010
Omole, R. K., Torimiro, N., Alayande, S. O., and Ajenifuja, E. (2018). Silver nanoparticles synthesized from Bacillus subtilis for detection of deterioration in the post-harvest spoilage of fruit. Sustain. Chem. Pharm. 10, 33–40. doi: 10.1016/j.scp.2018.08.005
Pandey, G., Singh, S., and Hitkari, G. (2018). Synthesis and characterization of polyvinyl pyrrolidone (PVP)-coated Fe3O4 nanoparticles by chemical co-precipitation method and removal of Congo red dye by adsorption process. Int. Nano Lett. 8, 111–121. doi: 10.1007/s40089-018-0234-6
Patil, M. P., Kim, G., and Do (2018). Marine microorganisms for synthesis of metallic nanoparticles and their biomedical applications. Colloids Surf. B Biointerfaces 172, 487–495. doi: 10.1016/j.colsurfb.2018.09.007
Peighambardoust, S. J., Peighambardoust, S. H., Mohammadzadeh Pournasir, N., and Pakdel, P. (2019). Properties of active starch-based films incorporating a combination of Ag, ZnO and CuO nanoparticles for potential use in food packaging applications. Food Packag. Shelf Life 22, 100420. doi: 10.1016/j.fpsl.2019.100420
Pithawalla, Y. B., El-Shall, M. S., Deevi, S. C., Ström, V., and Rao, K. V. (2001). Synthesis of magnetic intermetallic FeAl nanoparticles from a non-magnetic bulk alloy. J. Phys. Chem. B 105, 2089–2090. doi: 10.1021/jp002354i
Pongrac, I. M., Ahmed, L. B., Mlinarić, H., Jurašin, D. D., Pavičić, I., Marjanović Čermak, A. M., et al. (2018). Surface coating affects uptake of silver nanoparticles in neural stem cells. J. Trace Elem. Med. Biol. 50, 684–692. doi: 10.1016/j.jtemb.2017.12.003
Powell, M. C., and Kanarek, M. S. (2006). Nanomaterial health effects - Part 2: Uncertainties and recommendations for the future. Wis. Med. J. 105, 18–23.
Pragathiswaran, C., Violetmary, J., Faritha, A., Selvarani, K., and Nawas, P. M. A. (2021). Photocatalytic degradation, sensing of Cd2+ using silver nanoparticles synthesised from plant extract of cissus quadrangularis and their microbial activity. Mater. Today Proc. 45, 3348–3356. doi: 10.1016/j.matpr.2020.12.656
Prasad, R., Kumar, M., and Kumar, V. (2017). Nanotechnology: An Agricultural Paradigm. p. 1–372. doi: 10.1007/978-981-10-4573-8
Qiao, Z. P., Wang, M. Y., Liu, J. F., and Wang, Q. Z. (2022). Green synthesis of silver nanoparticles using a novel endophytic fungus Letendraea sp. WZ07: Characterization and evaluation of antioxidant, antibacterial and catalytic activities (3-in-1 system). Inorg. Chem. Commun. 138, 109301. doi: 10.1016/j.inoche.2022.109301
Qiu, L., Zhang, M., Bhandari, B., and Yang, C. (2022). Shelf life extension of aquatic products by applying nanotechnology: a review. Crit. Rev. Food Sci. Nutr. 62, 1521–1535. doi: 10.1080/10408398.2020.1844139
Qiu, L., Zhu, N., Feng, Y., Michaelides, E. E., Zyła, G., Jing, D., et al. (2020). A review of recent advances in thermophysical properties at the nanoscale: from solid state to colloids. Phys. Rep. 843, 1–81. doi: 10.1016/j.physrep.2019.12.001
Rabiee, N., Bagherzadeh, M., Kiani, M., and Ghadiri, A. M. (2020). Rosmarinus officinalis directed palladium nanoparticle synthesis: Investigation of potential anti-bacterial, anti-fungal and Mizoroki-Heck catalytic activities. Adv. Powder Technol. 31, 1402–1411. doi: 10.1016/j.apt.2020.01.024
Radini, I. A., Hasan, N., Malik, M. A., and Khan, Z. (2018). Biosynthesis of iron nanoparticles using Trigonella foenum-graecum seed extract for photocatalytic methyl orange dye degradation and antibacterial applications. J. Photochem. Photobiol. B Biol. 183, 154–163. doi: 10.1016/j.jphotobiol.2018.04.014
Rai, M., Ingle, A. P., Gupta, I., Pandit, R., Paralikar, P., Gade, A., et al. (2019). Smart nanopackaging for the enhancement of food shelf life. Environ. Chem. Lett. 17, 277–290. doi: 10.1007/s10311-018-0794-8
Raja, K., Balamurugan, V., Selvakumar, S., and Vasanth, K. (2022). Striga angustifolia mediated synthesis of silver nanoparticles: Anti-microbial, antioxidant and anti-proliferative activity in apoptotic p53 signalling pathway. J. Drug Deliv. Sci. Technol. 67, 102945. doi: 10.1016/j.jddst.2021.102945
Rajesh, K. M., Ajitha, B., Reddy, Y. A. K., Suneetha, Y., and Reddy, P. S. (2018). Assisted green synthesis of copper nanoparticles using Syzygium aromaticum bud extract: Physical, optical and antimicrobial properties. Optik (Stuttg). 154, 593–600. doi: 10.1016/j.ijleo.2017.10.074
Ramrakhiani, L., and Ghosh, S. (2018). Metallic nanoparticle synthesised by biological route: Safer candidate for diverse applications. IET Nanobiotechnol. 12, 392–404. doi: 10.1049/iet-nbt.2017.0076
Rasul, N. H., Asdagh, A., Pirsa, S., Ghazanfarirad, N., and Sani, I. K. (2022). Development of antimicrobial/antioxidant nanocomposite film based on fish skin gelatin and chickpea protein isolated containing Microencapsulated Nigella sativa essential oil and copper sulfide nanoparticles for extending minced meat shelf life. Mater. Res. Express 9:025306. doi: 10.1088/2053-1591/ac50d6
Raval, N., Maheshwari, R., Kalyane, D., Youngren-Ortiz, S. R., Chougule, M. B., and Tekade, R. K. (2018). “Importance of physicochemical characterization of nanoparticles in pharmaceutical product development,” in Basic Fundamentals of Drug Delivery (Academic Press), 369–400. doi: 10.1016/B978-0-12-817909-3.00010-8
Razavi, R., Molaei, R., Moradi, M., Tajik, H., Ezati, P., and Shafipour Yordshahi, A. (2020). Biosynthesis of metallic nanoparticles using mulberry fruit (Morus alba L.) extract for the preparation of antimicrobial nanocellulose film. Appl. Nanosci. 10, 465–476. doi: 10.1007/s13204-019-01137-8
Ríos-Corripio, R. C. M. A., López-Díaz, A. S., Ramírez-Corona, N., López-Malo, A., and Palou, E. (2019). Metallic Nanoparticles: Development, Applications, and Future Trends for Alcoholic and Nonalcoholic Beverages. The Science of Beverages (Elsevier Inc).
Rodino, S., Butu, M., and Butu, A. (2019). Application of biogenic silver nanoparticles for berries preservation. Dig. J. Nanomater. Biostructures 14, 601–606.
Rolim, W. R., Pieretti, J. C., Renó, D. L. S., Lima, B. A., Nascimento, M. H. M., Ambrosio, F. N., et al. (2019). Antimicrobial activity and cytotoxicity to tumor cells of nitric oxide donor and silver nanoparticles containing PVA/PEG films for topical applications. ACS Appl. Mater. Interfaces. doi: 10.1021/acsami.8b19021
Sahoo, M., Panigrahi, C., Vishwakarma, S., and Kumar, J. (2022). A review on nanotechnology : applications in food industry, future opportunities, challenges and potential risks. J. Nanotechnol. Nanomater. 3, 28–33. doi: 10.33696/Nanotechnol.3.029
Salih, T. A., Hassan, K. T., Majeed, S. R., Ibraheem, I. J., Hassan, O. M., and Obaid, A. S. (2020). In vitro scolicidal activity of synthesised silver nanoparticles from aqueous plant extract against Echinococcus granulosus. Biotechnol. Reports 28, e00545. doi: 10.1016/j.btre.2020.e00545
Saratale, R. G., Saratale, G. D., Shin, H. S., Jacob, J. M., Pugazhendhi, A., Bhaisare, M., et al. (2018). New insights on the green synthesis of metallic nanoparticles using plant and waste biomaterials: current knowledge, their agricultural and environmental applications. Environ. Sci. Pollut. Res. 25, 10164–10183. doi: 10.1007/s11356-017-9912-6
Saravanakumar, K., Hu, X., Chelliah, R., Oh, D. H., Kathiresan, K., and Wang, M. H. (2020). Biogenic silver nanoparticles-polyvinylpyrrolidone based glycerosomes coating to expand the shelf life of fresh-cut bell pepper (Capsicum annuum L. var. grossum (L.) Sendt). Postharvest Biol. Technol. 160, 111039. doi: 10.1016/j.postharvbio.2019.111039
Saravanan, M., Gopinath, V., Chaurasia, M. K., Syed, A., Ameen, F., and Purushothaman, N. (2018). Green synthesis of anisotropic zinc oxide nanoparticles with antibacterial and cytofriendly properties. Microb. Pathog. 115, 57–63. doi: 10.1016/j.micpath.2017.12.039
Satpathy, S., Patra, A., Ahirwar, B., and Hussain, M. D. (2020). Process optimization for green synthesis of gold nanoparticles mediated by extract of Hygrophila spinosa T. Anders and their biological applications. Phys. E Low-Dimensional Syst. Nanostructures 121, 113830. doi: 10.1016/j.physe.2019.113830
Savage, T., and Rao, A. M. (2006). Thermal properties of nanomaterials and nanocomposites. Therm. Conduct. 261–284. doi: 10.1007/0-387-26017-X_12
Shafiq, T., Uzair, M., Iqbal, M. J., Zafar, M., Hussain, S. J., and Shah, S. A. A. (2021). Green synthesis of metallic nanoparticles and their potential in bio-medical applications. Nano Biomed. Eng. 13, 191–206. doi: 10.5101/nbe.v13i2.p191-206
Shankar, S., Khodaei, D., and Lacroix, M. (2021). Effect of chitosan/essential oils/silver nanoparticles composite films packaging and gamma irradiation on shelf life of strawberries. Food Hydrocoll. 117, 106750. doi: 10.1016/j.foodhyd.2021.106750
Sharifan, H., Noori, A., Bagheri, M., and Moore, J. M. (2022). Postharvest spraying of zinc oxide nanoparticles enhances shelf life qualities and zinc concentration of tomato fruits. Crop Pasture Sci. 73, 22–31. doi: 10.1071/CP21191
Sharma, R., and Gupta, H. (2020). Green synthesis of silver, copper and gold nanoparticles using terminalia arjuna bark and their effect on seed germination. Nanosci. Nanotechnol. Asia 11, 243–247. doi: 10.2174/2210681210999200521131404
Sharma, R., Jafari, S. M., and Sharma, S. (2020). Antimicrobial bio-nanocomposites and their potential applications in food packaging. Food Control 112, 107086. doi: 10.1016/j.foodcont.2020.107086
Shifa, S., Begum, T., Afroze, F., and Kaisarunnahar Shraboni, M. (2019). Preliminary phytochemical screening, antibacterial activity and cytotoxic activity of leaves extract of Carissa carandas Linn. ~ 801 ~ J. Pharmacogn. Phytochem. 8, 801–804.
Shim, J. H., Lee, B. J., and Cho, Y. W. (2002). Thermal stability of unsupported gold nanoparticle: a molecular dynamics study. Surf. Sci. 512, 262–268. doi: 10.1016/S0039-6028(02)01692-8
Singh, A. K. (2022). Flower extract-mediated green synthesis of bimetallic Cu[sbnd]Zn oxide nanoparticles and its antimicrobial efficacy in hydrocolloid films. Bioresour. Technol. Rep. Int. Dev. Res. Cent. Can. 18, 101034. doi: 10.1016/j.biteb.2022.101034
Singh, T., Shukla, S., Kumar, P., Wahla, V., and Bajpai, V. K. (2017). Application of nanotechnology in food science: perception and overview. Front. Microbiol. 8:1501. doi: 10.3389/fmicb.2017.01501
Soliman, H., Elsayed, A., and Dyaa, A. (2018). Antimicrobial activity of silver nanoparticles biosynthesised by Rhodotorula sp. strain ATL72. Egypt. J. Basic Appl. Sci. 5, 228–233. doi: 10.1016/j.ejbas.2018.05.005
Soniya, P., Kumaresn, K., Parthiban, D., Arun, N., and Kumaravel, P. (2015). Effect of Zinc oxide Nanoparticles on Artemia salina. Res. J. Pharm. Dos. Forms Technol. 7, 103. doi: 10.5958/0975-4377.2015.00015.4
Sonkaria, S., Ahn, S. H., and Khare, V. (2012). Nanotechnology and its impact on food and nutrition: a review. Recent Patents Food Nutr. Agric. 4, 8–18. doi: 10.2174/1876142911204010008
Sooch, B. S., and Mann, M. K. (2021). Nanoreinforced biodegradable gelatin based active food packaging film for the enhancement of shelf life of tomatoes (Solanum lycopersicum L.). Food Control 130, 108322. doi: 10.1016/j.foodcont.2021.108322
Sothornvit, R. (2019). Nanostructured materials for food packaging systems: new functional properties. Curr. Opin. Food Sci. 25, 82–87. doi: 10.1016/j.cofs.2019.03.001
Srinath, B. S., Namratha, K., and Byrappa, K. (2018). Eco-friendly synthesis of gold nanoparticles by bacillus subtilis and their environmental applications. Adv. Sci. Lett. 24, 5942–5946. doi: 10.1166/asl.2018.12224
Sun, S., Murray, C. B., Weller, D., Folks, L., and Moser, A. (2000). Monodisperse FePt nanoparticles and ferromagnetic FePt nanocrystal superlattices. Science 287, 1989–1992. doi: 10.1126/science.287.5460.1989
Suriyakala, G., Sathiyaraj, S., Babujanarthanam, R., Alarjani, K. M., Hussein, D. S., Rasheed, R. A., et al. (2022). Green synthesis of gold nanoparticles using Jatropha integerrima Jacq. flower extract and their antibacterial activity. J. King Saud Univ. - Sci. 34, 101830. doi: 10.1016/j.jksus.2022.101830
Thangamani, N., and Bhuvaneshwari, N. (2019). Green synthesis of gold nanoparticles using Simarouba glauca leaf extract and their biological activity of micro-organism. Chem. Phys. Lett. 732, 136587. doi: 10.1016/j.cplett.2019.07.015
Tirado-Kulieva, V. A., Sánchez-Chero, M., Jimenez, D. P. P., Sánchez-Chero, J., Cruz, A. G. Y. S., Velayarce, H. H. M., et al. (2022). A critical review on the integration of metal nanoparticles in biopolymers: an alternative for active and sustainable food packaging. Curr. Res. Nutr. Food Sci. 10, 1–18. doi: 10.12944/CRNFSJ.10.1.01
Tkaczyszyn, M., Comín-Colet, J., Voors, A. A., van Veldhuisen, D. J., Enjuanes, C., Moliner-Borja, P., et al. (2018). Iron deficiency and red cell indices in patients with heart failure. Eur. J. Heart Fail. 20, 114–122. doi: 10.1002/ejhf.820
Tunesi, M. M., Kalwar, N., Abbas, M. W., Karakus, S., Soomro, R. A., Kilislioglu, A., et al. (2018). Functionalised CuO nanostructures for the detection of organophosphorus pesticides: A non-enzymatic inhibition approach coupled with nano-scale electrode engineering to improve electrode sensitivity. Sensors Actuators, B Chem. 260, 480–489. doi: 10.1016/j.snb.2018.01.084
Uzair, B., Liaqat, A., Iqbal, H., Menaa, B., Razzaq, A., Thiripuranathar, G., et al. (2020). Green and cost-effective synthesis of metallic nanoparticles by algae: Safe methods for translational medicine. Bioengineering 7, 1–22. doi: 10.3390/bioengineering7040129
Verkhovskii, R., Kozlova, A., Atkin, V., Kamyshinsky, R., Shulgina, T., and Nechaeva, O. (2019). Physical properties and cytotoxicity of silver nanoparticles under different polymeric stabilizers. Heliyon 5, e01305. doi: 10.1016/j.heliyon.2019.e01305
Videira-Quintela, D., Martin, O., and Montalvo, G. (2021). Recent advances in polymer-metallic composites for food packaging applications. Trends Food Sci. Technol. 109, 230–244. doi: 10.1016/j.tifs.2021.01.020
Vijaya Kumar, P., Mary Jelastin Kala, S., and Prakash, K. S. (2019). Green synthesis of gold nanoparticles using Croton Caudatus Geisel leaf extract and their biological studies. Mater. Lett. 236, 19–22. doi: 10.1016/j.matlet.2018.10.025
Wang, D., Xue, B., Wang, L., Zhang, Y., Liu, L., and Zhou, Y. (2021). Fungus-mediated green synthesis of nano-silver using Aspergillus sydowii and its antifungal/antiproliferative activities. Sci. Rep. 11, 1–9. doi: 10.1038/s41598-021-89854-5
Wang, W., Yu, Z., Alsammarraie, F. K., Kong, F., Lin, M., and Mustapha, A. (2020). Properties and antimicrobial activity of polyvinyl alcohol-modified bacterial nanocellulose packaging films incorporated with silver nanoparticles. Food Hydrocoll. 100, 105411. doi: 10.1016/j.foodhyd.2019.105411
Wu, Z., Zhou, W., Pang, C., Deng, W., Xu, C., and Wang, X. (2019). Multifunctional chitosan-based coating with liposomes containing laurel essential oils and nanosilver for pork preservation. Food Chem. 295, 16–25. doi: 10.1016/j.foodchem.2019.05.114
Xu, R., Wang, D., Zhang, J., and Li, Y. (2006). Shape-dependent catalytic activity of silver nanoparticles for the oxidation of styrene. Chem. Asian J. 1, 888–893. doi: 10.1002/asia.200600260
Yaktine, A., and Pray, L. (2009). Nanotechnology in Food Products: Workshop Summary. National Academies Press. p. 59–71. doi: 10.1201/9781315370859-16
Yazhiniprabha, M., and Vaseeharan, B. (2019). In vitro and in vivo toxicity assessment of selenium nanoparticles with significant larvicidal and bacteriostatic properties. Mater. Sci. Eng. C 103, 109763. doi: 10.1016/j.msec.2019.109763
Yu, Z., Wang, W., Kong, F., Lin, M., and Mustapha, A. (2019). Cellulose nanofibril/silver nanoparticle composite as an active food packaging system and its toxicity to human colon cells. Int. J. Biol. Macromol. 129, 887–894. doi: 10.1016/j.ijbiomac.2019.02.084
Zabihzadeh Khajavi, M., Mohammadi, R., Ahmadi, S., Farhoodi, M., and Yousefi, M. (2019). Strategies for controlling release of plastic compounds into foodstuffs based on application of nanoparticles and its potential health issues. Trends Food Sci. Technol. 90, 1–12. doi: 10.1016/j.tifs.2019.05.009
Zare, M., Namratha, K., Ilyas, S., Hezam, A., Mathur, S., and Byrappa, K. (2019). Smart Fortified PHBV-CS biopolymer with ZnO-Ag nanocomposites for enhanced shelf life of food packaging. ACS Appl. Mater. Interfaces 11, 48309–48320. doi: 10.1021/acsami.9b15724
Zhai, X., Zhou, S., Zhang, R., Wang, W., and Hou, H. (2022). Antimicrobial starch/poly(butylene adipate-co-terephthalate) nanocomposite films loaded with a combination of silver and zinc oxide nanoparticles for food packaging. Int. J. Biol. Macromol. 206, 298–305. doi: 10.1016/j.ijbiomac.2022.02.158
Zhang, C., Li, W., Zhu, B., Chen, H., Chi, H., Li, L., et al. (2018). The quality evaluation of postharvest strawberries stored in nano-Ag packages at refrigeration temperature. Polymers (Basel). 10, 894. doi: 10.3390/polym10080894
Zhang, D., Ma, X. L., Gu, Y., Huang, H., and Zhang, G. W. (2020). Green synthesis of metallic nanoparticles and their potential applications to treat cancer. Front. Chem. 8, 799. doi: 10.3389/fchem.2020.00799
Zhao, X., Wang, K., Ai, C., Yan, L., Jiang, C., and Shi, J. (2021). Improvement of antifungal and antibacterial activities of food packages using silver nanoparticles synthesized by iturin A. Food Packag. Shelf Life 28, 100669. doi: 10.1016/j.fpsl.2021.100669
Keywords: agrifoods, green synthesis, MNPs, nanoparticles, shelf-life
Citation: Kumar A, Kumar A, Vats C, Sangwan P, Kumar V, Abhineet, Chauhan P, Chauhan RS and Chaudhary K (2022) Recent insights into metallic nanoparticles in shelf-life extension of agrifoods: Properties, green synthesis, and major applications. Front. Sustain. Food Syst. 6:1025342. doi: 10.3389/fsufs.2022.1025342
Received: 22 August 2022; Accepted: 11 November 2022;
Published: 07 December 2022.
Edited by:
Mónica L. Chávez-González, Universidad Autónoma de Coahuila, MexicoReviewed by:
Mahesh Kumar Samota, Central Institute of Post-Harvest Engineering and Technology (ICAR), IndiaSantosh Kumar, Central Institute of Technology Kokrajhar, India
Copyright © 2022 Kumar, Kumar, Vats, Sangwan, Kumar, Abhineet, Chauhan, Chauhan and Chaudhary. This is an open-access article distributed under the terms of the Creative Commons Attribution License (CC BY). The use, distribution or reproduction in other forums is permitted, provided the original author(s) and the copyright owner(s) are credited and that the original publication in this journal is cited, in accordance with accepted academic practice. No use, distribution or reproduction is permitted which does not comply with these terms.
*Correspondence: Abhishek Kumar, abhishekpathology9511@gmail.com; Chaman Vats, vashisth1997@gmail.com