- 1Division of Agronomy, ICAR-Indian Agricultural Research Institute, New Delhi, India
- 2Division of Nematology, ICAR-Indian Agricultural Research Institute, New Delhi, India
- 3Division of Agricultural Chemicals, ICAR-Indian Agricultural Research Institute, New Delhi, India
- 4Division of Environment Science, ICAR-Indian Agricultural Research Institute, New Delhi, India
- 5Division of Agricultural Physics, ICAR-Indian Agricultural Research Institute, New Delhi, India
- 6C. P. College of Agriculture, Sardarkrushinagar Dantiwada Agricultural University, Dantiwada, India
Direct-seeded rice (DSR) can be a resource-efficient alternative to puddled transplanted rice (PTR), but weeds and nematodes pose severe challenges. Conservation agriculture (CA)-based DSR may inhibit/influence weeds/nematodes, which can be further intensified by adopting better weed control. Hence, this experiment was undertaken. Five CA-based DSR practices involving zero tillage, residue retention, brown manuring, and superimposed with four weed control/herbicide options were compared with PTR in a split-plot design replicated three times. All DSRs encountered more weeds and plant parasitic nematodes (PPNs) than PTR. Root-knot nematodes (RKN) infested five among 14 weeds present in rice and was first time found in Dinebra retroflexa. A CA-based zero till (ZT)DSR+ mungbean residue – ZT wheat + rice residue – ZT mungbean+wheat residue system reduced weeds significantly. It reduced RKN galls in Echinochloa colona, Echinochloa crusgalli, and rice plants by 72, 58, and 56%, respectively. In soil too, RKN and other PPNs, namely, Tylenchorhynchus brevilineatus and Pratylenchus thornei were reduced by 39%, 32%, and 26%, respectively, which gave a 6.3–22.7% higher yield in this CA practice than other DSRs. Pyrazosulfuron-ethyl, cyhalofop-butyl, and bispyribac-Na applied sequentially reduced weeds and PPNs, increased rice yield by 176.1%, and were at below detectable levels in soil, rice grains, and straw, and were safe for rotational crops. The above ZT-based triple cropping with residue supplemented with herbicides through better weed and nematode control would be an alternative to PTR in the North-wester Indo-Gangetic Plains of India and in similar agroecologies of the tropics/sub-tropics. This study would help farmers and policymakers to design integrated weed and nematode management modules using tillage, crop residue, and herbicides/pesticides for higher DSR yield and income.
Introduction
Recently, the sustainability of puddled transplanted rice (PTR)–conventional till wheat (CTW) cropping system, the most dominant system practiced in nearly 10.5 million ha in the Indo-Gangetic Plains (IGP) of India (Ladha et al., 2009; Das et al., 2018) is threatened due to a host of problems, mainly associated with PTR. PTR is less labor-, water-, time-, energy-, and carbon-efficient and more cost-intensive (Gupta et al., 2016; Nawaz et al., 2017b; Raj et al., 2017; Das et al., 2020b; Sen et al., 2021). Puddling done in PTR affects soil structures and reduces subsurface permeability by forming hard pans (Mondal et al., 2019). PTR delays wheat sowing and can reduce 8–9% yield of wheat (Kumar and Ladha, 2011; Bhattacharyya et al., 2015). New resource-efficient and climate-smart management approaches are required to ensure food production in Indian IGP and make a substantial contribution to the food security of South-East Asia. Conservation Agriculture (CA) is a viable alternative to tillage-intensive agriculture (Kassam et al., 2018; FAO, 2020), which can improve biodiversity and above- and below-ground biological processes (Ghosh et al., 2019), and leads to higher use efficiencies of water and nutrients and sustainable crop production (FAO, 2020). Direct-seeded rice (DSR) is an alternate rice production technology and can be a potential alternative to PTR (Farooq et al., 2011; Kumar and Ladha, 2011). Under CA, zero till DSR (ZTDSR) is adopted in the rice–wheat system. The ZTDSR – ZT wheat (~ZTW) system with residue has advantages over transplanting: earlier rice maturity, lower water (Nawaz et al., 2017b; Mohammad et al., 2018) and labor requirement, timely/early sowing of wheat, and higher economic returns (Bhattacharyya et al., 2015; Nawaz et al., 2017b; Raj et al., 2017). But DSR is heavily infested with weeds and nematodes, irrespective of climates and soils due to changes in ecology (Rao et al., 2007; Kyndt et al., 2014; Chauhan et al., 2015; Khan, 2015). DSR yield loss due to weeds varies across locations depending on management practices (Chauhan and Opena, 2012; Raj et al., 2016). It could be even 100% in a certain situation (Awan et al., 2015). Globally, the potential yield loss in rice due to various pests has been estimated to be around 77%, of which weeds contributed the highest 37.1% loss (Oerke, 2006). The losses caused by animal pests (insects, mites, nematodes, rodents, slugs/snails, birds, etc), pathogens, and viruses were 24.7, 13.5, and 1.7%, respectively. In India, the actual economic loss in rice due to weeds is 4,420 million US$ annually, the highest among the losses caused by 10 major crops (Gharde et al., 2018). The ZTDSR, residue retention, and brown manuring (Nawaz et al., 2017a; Behera et al., 2018; Behera and Das, 2019; Das et al., 2020a) crop intensification with a legume (Das et al., 2020b) highly influences dominance and diversity of weeds by altering weed seeds recruitment/dispersal across the depth of soil (Chauhan and Opena, 2012; Chauhan et al., 2015). In a long-term experiment, the conventional tilled DSR (~CTDSR) - ZTW system was followed for the first 4 years (from 2010 to 2013), and weed management was studied in CTDSR in 3rd and 4th years (2012 and 2013). The experiment was fully modified to a CA system with three principles (Kassam et al., 2018) by adopting ZTDSR with residue in 2014, which led to a weed shift to annual grassy weeds [Dactyloctenium aegyptium (L.) Willd., Dinebra retroflexa (Vahl) Panz., and Leptochloa chinensis (L.) Nees], and perennial sedges (Cyperus esculentus L., Cyperus rotundus L.). Bispyribac-Na (hereafter referred to as bispyribac) recommended could not control these weeds. This prompted us to design this experiment in 2018 (9th year) and 2019 (10th year) to evaluate afresh weed control practices in ZTDSR and their associated effects on nematodes. Newer herbicides and combinations, such as the sequential applications of pre-emergence pyrazosulfuron-ethyl (hereafter referred to as pyrazosulfuron) as a substitute of pendimethalin, followed by (~fb) post-emergence bispyribac and cyhalofop-butyl (hereafter referred to cyhalofop) as supplementary to bispyribac, were studied to control weed better and prevent/arrest weed dynamics. Pendimethalin, a broad-spectrum, but a grass-killer exclusively pre-emergence herbicide inhibits microtubule assembly in cell division (Das and Das, 2018). Pyrazosulfuron is a pre-emergence, broad-spectrum herbicide and inhibits acetolactate synthase (~ALS). Bispyribac is also a broad-spectrum herbicide, inhibiting ALS, but post-emergence and less effective against certain grassy weeds (Sen et al., 2021). Cyhalofop-butyl is an acetyl coenzyme A carboxylase (ACCase) inhibitor herbicide, post-emergence, and kills exclusively grassy weeds.
Recently, there has been a considerable increase in plant-parasitic nematodes (PPNs) in crops worldwide (Mantelin et al., 2017; Devaraja et al., 2018). The changes in agroecology, tillage, and management practices influenced nematodes' community/species structure and their interactions with hosts (Kyndt et al., 2014; Pankaj et al., 2015; Liu et al., 2019). The PPNs can reduce rice yield by 10–25% (Bridge et al., 2005) or even more based on location and initial inoculum level (Ornat and Sorribas, 2008). Worldwide annual economic losses due to nematodes in crops are estimated to be US$ 173 billion (Elling, 2013). Kumar et al. (2020) reported that PPNs caused 21.3% crop losses amounting to US$ 1.58 billion per year in India. The economic loss in rice crops due to root-knot nematode (Meloidogyne graminicola Golden & Birchfield) alone was INR 23.3 billion (US$ 0.29 billion) annually. Among the top 10 PPNs of the world, root-knot nematode (RKN), cyst nematode (Heterodera oryzae Luc & Berdon), root-lesion nematode (Pratylenchus thornei Sher and Allen), and rice white-tip nematode (Aphelenchoides besseyi Christie) can cause damage to rice (Jones et al., 2013).
Several researchers (Chauhan and Opena, 2012; Chauhan et al., 2015; Abbas et al., 2019; Pandey and Kandel, 2020; Sen et al., 2021) have reported variable effects of the varying combinations of tillage, crop residue, and herbicides on weeds in DSR across locations. Puddling could significantly reduce nematodes in PTR, while nematodes such as RKN, Meloidogyne triticoryzae, and Tylenchorhynchus mashoodi were higher in DSR (Gaur and Singh, 1993; Chandel et al., 2002). Similarly, Suong et al. (2019) found higher root-parasitic nematodes in rice under direct-seeded mulch-based cropping system than in conventional plow-based tillage in Cambodia. The populations of Tylenchorhynchus brevilineatus and Pratylenchus spp were significantly higher in ZT than in CT fields (Pankaj et al., 2006). In contrast, Yadav et al. (2021) reported lesser RKN and PPNs in DSR than in PTR. However, the effect of herbicides on nematodes is less/negligibly studied. Zhang et al. (2010) highlighted that acetochlor and carbofuran reduced total nematodes and PPN in soybean. Weeds act as alternate hosts of these PPNs in the presence/absence of crops and are sources of inoculums for the next crops (Rich et al., 2009; Baghel et al., 2020). All these studies having combinations of tillage, residue, crop rotation, herbicides, etc. were different leading to variable effects on weeds and nematodes. In fact, the CA effect is location-specific, depending on soil type, prevailing climate, weed and nematode species distribution, etc., which suggests that studies need to be carried out to validate its impact on these pests across locations. There are gaps in location-specific comprehensive studies encompassing tillage (ZT and CT), nature/kind and amount of residue (cereal, legume, and brown manure crop residue), cropping (double or triple cropping with legume intervention), herbicides rotation (arresting weed dynamics) on weeds, and PPNs, especially RKN (most devastating to rice). Identifying new emerging weeds as alternate hosts of these nematodes in rice is also lacking. This provides opportunities for multidisciplinary integrated weed and nematode management research in DSR involving CA and weed management/herbicides. We hypothesized that the CA-based DSR supplemented with herbicides may lead to better weed and nematode management. The objectives were: to evaluate CA and weed management/herbicides' effects on weeds, nematodes, and productivity of rice; and to develop an effective weed management strategy for DSR under a CA-based rice–wheat system.
Materials and methods
Experimental sites and treatments
Experiments were conducted in the 9th (~2018) and 10th (~2019) years of a long-term conservation agriculture (CA)-based rice–wheat system (mentioned in Section Introduction) at ICAR-Indian Agricultural Research Institute, New Delhi (28°35′ N; 77°12′ E; 228 m above mean sea level). Six main plot treatments, involving tillage and crop residue, and four sub-plot treatments involving weed control/herbicides (Table 1) were laid out in a split-plot design with three replications. A triple cropping system involving a legume crop mungbean, which is usually not followed by the farmers, was taken as a treatment for comparison with the CA-based double cropping systems and conventional PTR–CTW system. Unweeded control (UWC) was a natural uninhibited weed infestation. The soil (order Inceptisol, Typic Haplustept) was clayey loam on the surface and loam below.
Crop sowing and agronomic practices
For ZTDSR, rice hybrid (Arize 6129 Gold) was sown by using a happy seeder with 25 kg seed ha−1 in rows 20 cm apart at 2–3 cm depth of soil. For PTR, transplanting was done manually at 20 cm × 10 cm spacing with 25 days old seedlings. For ZTW, wheat was sown by using a happy seeder in rows 20 cm apart at 3–5 cm depths of soil with 100 kg seed ha−1. For triple cropping treatments, mungbean was sown after wheat harvest during summer using a happy seeder at 20 cm × 10 cm spacing. Recommended doses of 150 kg N, 26.2 kg P, and 33.1 kg K ha−1 were applied to rice and wheat. A 30% recommended dose of N and full doses of P and K were applied as basal, and the rest of N was applied in equal halves at active tillering and panicle initiation stages of rice and wheat. Diammonium phosphate at 100 kg ha−1 was applied to mungbean as basal.
Weeds density, rice yield, and economics
Two central rows of rice (~0.40 m) up to a length of 0.5 m were selected randomly from two locations in each plot. Weeds were collected, counted species-wise, and categorized into grassy, broad-leaved, and sedge weeds, which were summed up as total weeds. A net plot area comprising 16 rows of rice up to a length of 2.8 m (~3.2 m × 2.8 m) was harvested for grain yield recorded at 12% moisture. The common cost of all treatments was the sum total of the prevailing costs of inputs/operations such as seed, fertilizer, irrigation, plant protection (excluding herbicide), harvesting, and threshing. The cost of treatment constituted the costs of tillage (ZT/CT/puddling), sowing (DSR/nursery), transplanting, brown manuring, crop residue, and herbicide as applicable to the treatment. The common cost plus treatment cost constituted the total cost of treatment. Minimum support price for rice grains of the Government of India was used for calculating economics. Gross returns (GR), net returns (NR), and net benefit:cost (NB:C) were estimated as per Das and Das (2018). The exchange rates of November 2018 and 2019 were considered for converting Indian Rupees (~INR) to US$ (X-rates, 2017).
Nematodes population
Soil samples were collected from five locations in each plot using a tube auger (5-cm diameter) at 60 DAS. These five cores soils were composited and mixed thoroughly, and a sample core of 200 cc was taken in a polyethylene bag and washed. Then, muddy water suspension was poured on double-folded tissue paper superimposed on wire mesh placed on the top of the Petri dish and placed for incubation at 25°C−29°C for 48 h. In the second-stage juveniles (J2s), adult nematodes passing through the tissue paper to the Petri dishes having clear water suspension were observed under the stereoscopic binocular microscope. Ten J2s and adult nematodes were killed by mild heating and prepared temporary slides to identify nematode species (Pokharel et al., 2007). Standard procedures were followed for determining nematode populations (Southey, 1986), root-knot nematode galls in rice and weed plants (Coyne et al., 2007), and gall index (Pederson and Windham, 1989).
Herbicide residue estimation in rice grains and straw and soil
Residues of bispyribac, cyhalofop, pendimethalin, and pyrazosulfuron in rice grains, straw, and soil were estimated using QuEChERS (Quick, Easy, Cheap, Effective, Rugged, and Safe) method and subsequent analysis by liquid chromatography-mass spectroscopy/mass spectroscopy (LC-MS/MS) (Schenck and Hobbs, 2004).
Extraction and cleanup of herbicides from rice grains and soil
Soil and rice grains at harvest were collected from each treatment and their representative samples (100 g each) were prepared by quartering. Rice grains were homogenized in a mixer grinder, and soil samples were dried, ground by pestle mortar, and sieved through a 2-mm sieve. An aliquot of 5 g each for grains and soil was taken in 50 ml centrifuge tubes separately. Then, 2.5 ml of water was added to it and kept for half an hour. After that, 5 ml of acetonitrile, 2 g of anhydrous magnesium sulfate, and 0.75 g of NaCl were added into the centrifuge tube and were mixed thoroughly with a vortex mixer for 2 min. It was then centrifuged at 5,000 RPM for 5 min at a temperature of 27±1°C. After centrifugation, 1 ml of supernatant was taken in a 1.8 ml microcentrifuge tube and subjected to cleanup by dispersive solid-phase extraction using primary secondary amine (PSA) sorbent (50 mg) and anhydrous magnesium sulfate (150 mg) and vortexed for 1 min. It was then centrifuged for 5 min at 5,000 RPM in a microcentrifuge. After clean-up, the supernatant extract was filtered through a syringe filter (0.22 μm) and analyzed in LC-MS/MS.
Extraction and cleanup of herbicides from rice straw
Representative samples of rice straw (100 g) prepared by quartering were homogenized in a Willy mill straw crusher. An aliquot of 2.5 g was taken in a 50-ml centrifuge tube and 2.5 ml of water was added to it and kept for half an hour. Then, 20 ml of acetonitrile, 1 g of anhydrous magnesium sulfate, and 250 mg of NaCl were added to the centrifuge tube and were mixed thoroughly with a vortex mixer for 2 min. It was centrifuged at 5,000 RPM for 5 min at a temperature of 27±1°C. After centrifugation, 4 ml of supernatant was taken, which was evaporated to dryness by a rotary vacuum evaporator and reconstituted with 1 ml of acetonitrile. The 1-ml reconstituted supernatant was taken in a 1.8-ml microcentrifuge tube and subjected to cleanup by dispersive solid-phase extraction using primary secondary amine (PSA) sorbent (50 mg) and anhydrous magnesium sulfate (150 mg) and vortexed for 1 min. It was then centrifuged for 5 min at 5,000 RPM in a microcentrifuge, and the supernatant extract was filtered through a syringe filter (0.22 μm) and analyzed in LC-MS/MS.
Instrumental analysis of herbicide residue
The LC-MS/MS method for identification and quantification of bispyribac, cyhalofop, pendimethalin, and pyrazosulfuron was developed through optimizing LC and MS instrumental parameters in the Shimadzu LCMS/MS-8030 instrument equipped with Zorbax Eclipse Plus C18 column (Agilent make) of dimension 3 mm i.d., 10 cm length with 3.5 μm column coating. In electrospray ionization (ESI) with positive mode having DL temperature of 250°C, heat block temperature of 400°C, nebulizing gas flow of 3 ml/min, drying gas flow of 15 ml/min, multiple reaction monitoring (MRM) was optimized for selection of the best products for identification and quantification of each herbicide. The MRM optimization parameters, i.e., collision energy (CE), Q1 pre-bias and Q3 pre-bias, dwell time, and pause time for each event, were optimized according to the sensitivity of the compound. The mobile phase 10:90 water (5mM ammonium formate): methanol was used for eluting these four herbicides in the 6-min run and the flow of solvent was maintained at 0.2 ml/min. Herbicide standards in the concentration range of 0.1 to 1.0 ppm were injected to obtain a 5-point linearity curve within the detection range. As per the sensitivity of the analytes, the Limit of Detection [LOD] of the herbicides was found to be 0.01 μg/ml (signal: noise ratio ≥3:1), and the Limit of Quantification [LOQ] was found to be 0.05 μg/ml (signal: noise ratio ≥10:1). From the C18 column in 6 min run time, the retention time (RT) of bispyribac, cyhalofop, pendimethalin, and pyrazosulfuron were found to be 2.27, 3.31, 4.81, and 2.24 min, respectively (Table 2). The most intense MRM transition of each herbicide was designated as quantifier ion transition and used for quantification of the herbicides through Chrome Browser software associated with LC-MS/MS using system generated calibration curve (Figure 1). The quantifier MRM transitions were m/z 430.65>274.90 for bispyribac; m/z 374.75>255.90 for cyhalofop; m/z 281.90>211.80 for pendimethalin; and m/z 415.10>182.10 for pyrazosulfuron, respectively. Other less intense MRM transitions were used as qualifier ion transitions.
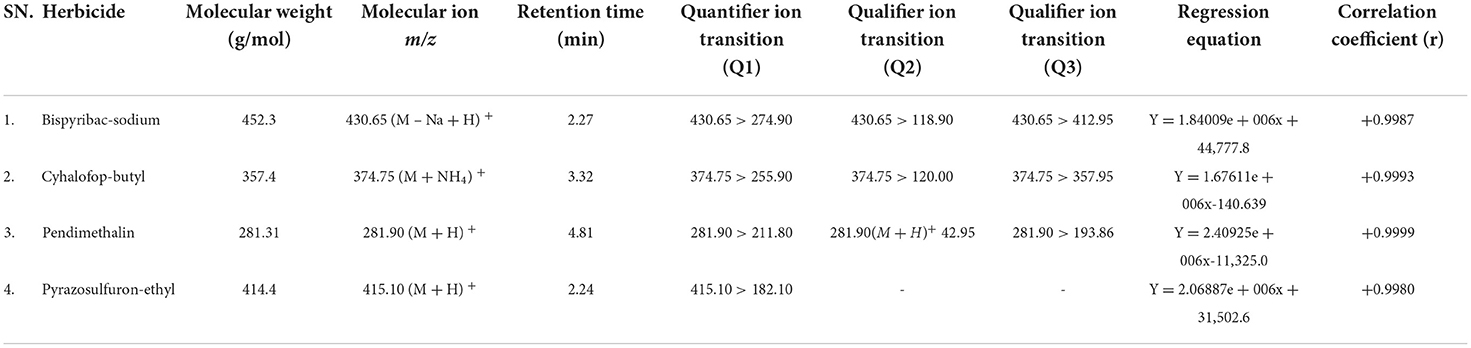
Table 2. LC-MS/MS quantifier and qualifier MRM transitions with regression equation of the herbicides.
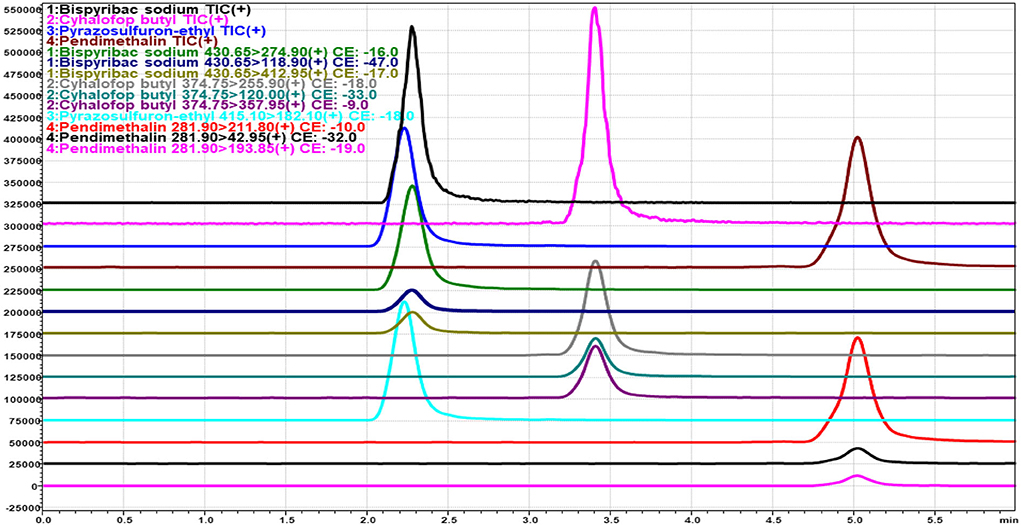
Figure 1. Base shift chromatogram showing multiple reaction monitoring (MRM) transition of bispyribac sodium, cyhalofop-butyl, pyrazosulfuron-ethyl, and pendimethalin herbicides.
Recovery study
For the recovery of bispyribac, cyhalofop, pendimethalin, and pyrazosulfuron, herbicide-free rice grains, straw, and soil were fortified with 0.05 mg/kg (~0.05 ppm) of the respective herbicide and analyzed following the above-mentioned procedure. The recoveries of bispyribac, cyhalofop, pendimethalin, and pyrazosulfuron were 86.6, 118.3, 72.8, and 87.5% (from soil); 62.5, 81.9, 78.5, and 113.9% (from grains); and 57.6, 114.2, 71.6, and 77.6% (from straw), respectively. All the recoveries of herbicides from the soil, grains, and straw were in the acceptable range of 70–120%, except the recovery of bispyribac, which was relatively lower from rice grains and straw.
Statistical analysis
Data on weed, nematode, and rice were analyzed by the analysis of variance (ANOVA) technique for a split-plot design using PROC GLM in SAS 9.3 (SAS Institute, Cary, NC). Weed and nematode populations were transformed through the square-root method [(x+0.5)½] before ANOVA to reduce higher variation. The species-wise populations of weeds and nematodes and rice grain yield were subjected to Levene's test for homogeneity of variance. The error variances for almost all parameters (i.e., weed and nematode population, rice grain yield) were homogeneous over the years, indicating that the uniformity in error variance was significant. Hence, pooled analysis was done to find out the effects of the year (Y), and interactions between Y × conservation agriculture (C), Y × weed control (W), C × W, and Y × C × W on the studied variables of weed, nematode and rice, and data are presented year-wise. The significance of treatment means was appraised using Tukey's honest significant difference (HSD) test at p ≤ .05.
Results
Effects on weeds diversity, dynamics, and interference
A total of 14 weeds (Table 3), comprising six grassy (Table 4), four broad-leaved (~BLW) (Table 5), and four sedge weeds (Table 6) were observed in rice under UWC. The pooled ANOVA reflected the significant difference between the years in densities of eight weeds, namely, Echinochloa colona (L.) Link., Dactyloctenium aegyptium (L.) Willd., Dinebra retroflexa (Vahl) Panz., Leptochloa chinensis (L.) Nees, Eclipta alba L., Trianthema portulacastrum L., Cyperus esculentus L. and Cyperus difformis L. weeds with higher densities in the second year than in the 1st year. In contrast, there was no significant yearly difference in the densities of the rest of the six weeds, namely, Echinochloa crusgalli (L.) Beauv, Eleusine indica (L.) Gaertn., Phyllanthus niruri L., Alternanthera philoxeroides (Mart.) Griseb, Cyperus rotundus L. and Cyperus iria L. (Table 3). The pooled mean effect of CA and weed control practices and their interactions were significant for all 14 weeds observed in rice (Table 3). The DSRs encountered higher infestations of Echinochloa colona, Dactyloctenium aegyptium, Dinebra retroflexa, Leptochloa chinensis, and Eleusine indica than the PTR, which, on the contrary, had a higher density of Echinochloa crusgalli (Table 4). The triple ZT system with three crops residue (C5) among the DSRs (C1-C5) led to the lowest densities of D. aegyptium, D. retroflexa and L. chinensis. PTR (C6) system was not infested with these weeds but had the highest density of E. crusgalli. Broad-leaved weeds Eclipta alba, Phyllanthus niruri, and Trianthema portulacastrum infested DSRs (C1-C5) but not PTR (C6) except E. alba in 2019 (Table 5). Eclipta alba decreased while T. portulacastrum increased (in C4&C5) in the second year under DSRs. The C5 caused a significant reduction of these weeds compared to C4, having the highest densities. Alternenthera philoxeroides were found in C6 and C1, the former having a significantly higher density than the latter. Perennial sedges Cyperus esculentus L. and Cyperus rotundus L. had larger densities in CA-based DSRs (C1-C5) and were absent in PTR (C6), whereas annual sedges Cyperus difformis L., Cyperus iria L. were observed in PTR and absent in DSRs in both the years (Table 6). Among DSRs, C4 had the highest densities of C. esculentus and C. rotundus. The pyrazosulfuron fb cyhalofop fb bispyribac treatment resulted in significantly lower densities of all grassy weeds except E. indica (Table 4) and all broad-leaved weeds than UWC and pyrazosulfuron fb tank-mix cyhalofop + bispyribac (Table 5). This herbicide treatment also led to lower densities of C. esculentus, C. rotundus, C. difformis, and C. iria than other treatments in both years (Table 6).
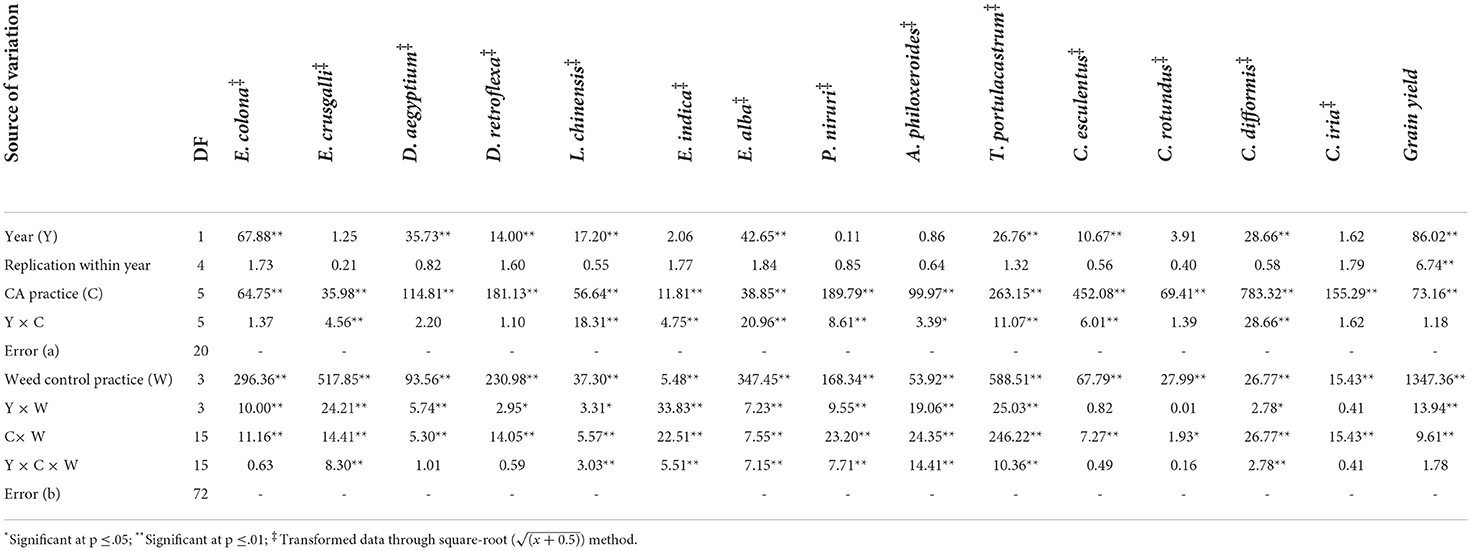
Table 3. Pooled analysis of variance (ANOVA) of Echinochloa colona, Echinochloa crusgalli, Dactyloctenium aegyptium, Dinebra retroflexa, Leptochloa chinensis, Eleusine indica, Eclipta alba, Phyllanthus niruri, Alternanthera philoxeroides, Trianthema portulacastrum, Cyperus esculentus, Cyperus rotundus, Cyperus difformis, Cyperus iria and grain yield showing the effects of years, CA and weed control practices and their interactions.
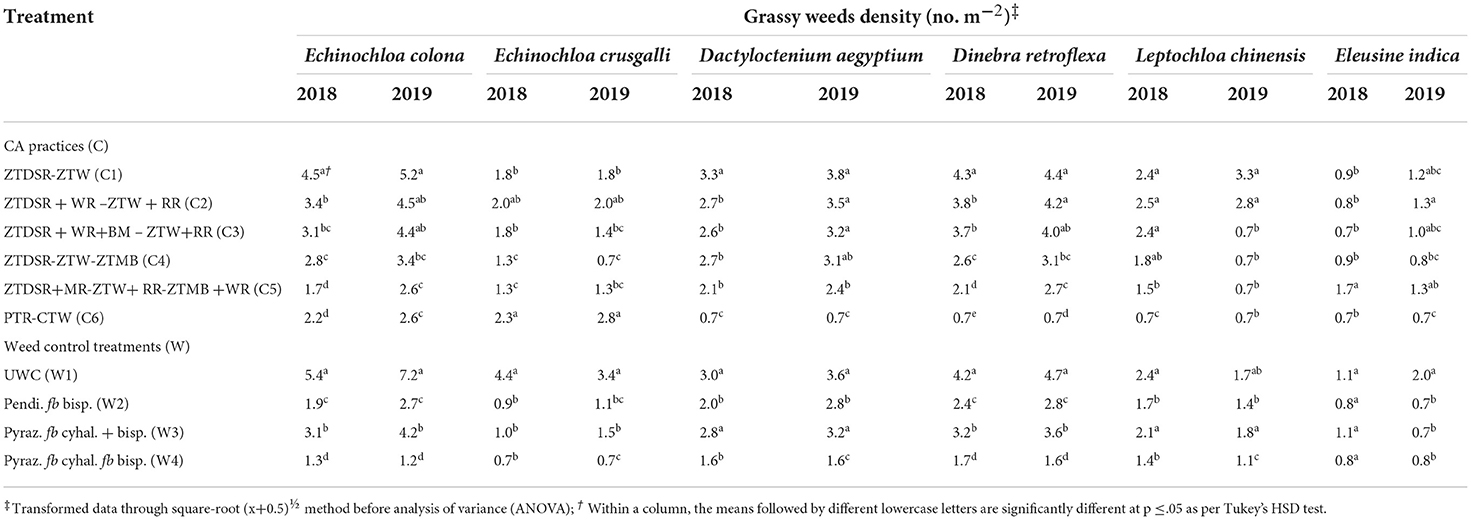
Table 4. Species-wise grassy weeds density (no. m−2) in rice as influenced by CA and weed control treatments at 60 DAS/DAT during 2018 and 2019.
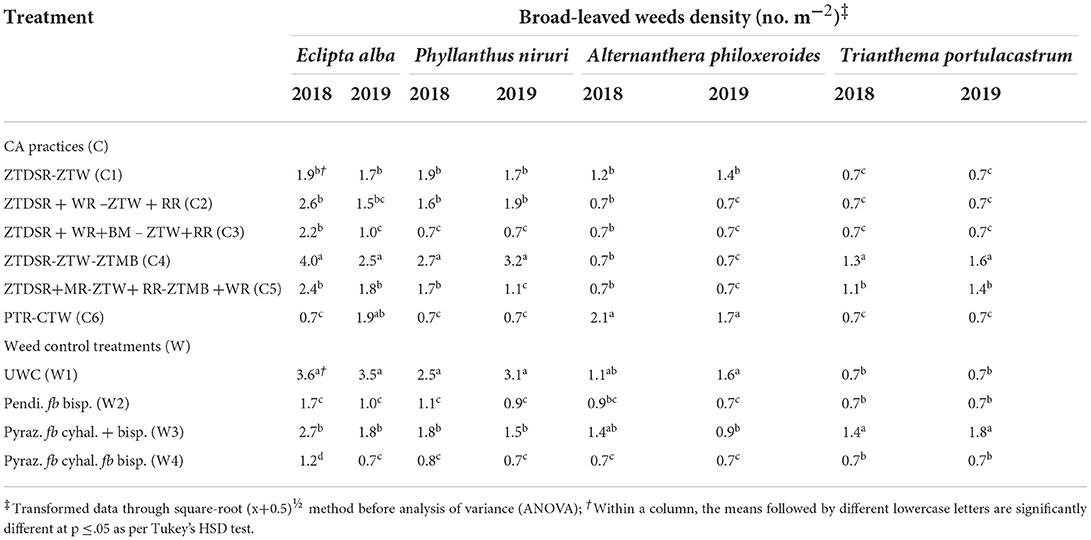
Table 5. Species-wise broad-leaved weeds density (no. m−2) in rice as influenced by CA and weed control treatments at 60 DAS/DAT during 2018 and 2019.
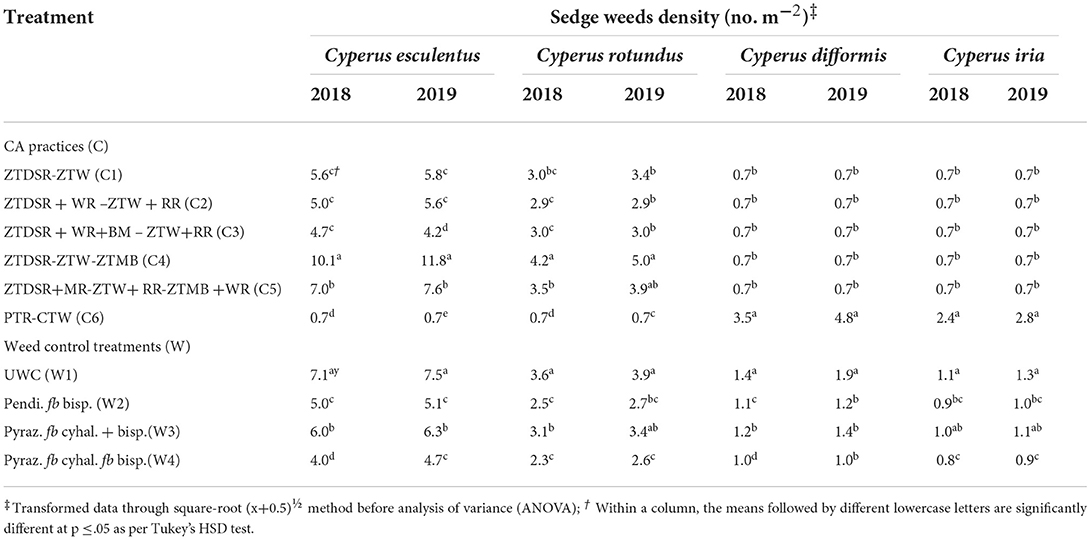
Table 6. Species-wise sedge weeds density (no. m−2) in rice as influenced by CA and weed control treatments at 60 DAS/DAT during 2018 and 2019.
Effects on species-wise and total nematodes
RKN galls were found in four grassy weeds (E. colona, E. crusgalli, D. retroflexa, E. indica) and one broad-leaved weed (E. alba) among 14 weeds present in this study. CA significantly influenced RKN galls in weeds and rice. RKN galls were significantly higher in E. colona, E. crusgalli, and rice in CA-based DSRs than PTR-CTW (Table 7). Among DSRs, the brown manuring (C3) had the highest, whereas the triple ZT with three crops residue (C5) had the lowest RKN galls and gall index (GI) of E. colona, E. crusgalli, and rice. The C5 led to reduction in RKN galls by 72, 60, 68, and 58% in E. colona; 58, 57, 52, and 34% in E. crusgalli; and 56, 50, 48, and 27% in rice compared to C3, C1, C2, and C4, respectively. CA practices also significantly influenced plant parasitic nematodes (PPNs) (Table 9). DSRs had higher densities of RKN, T. brevilineatus and P. thornei than PTR, which, on the contrary, had higher H. oryzeae. The C5 led to a reduction in RKN by 30% and T. brevilineatus by 27% compared to C3 and had the lowest total PPNs (mean of 2 years). Contrarily, C1 had significantly higher total PPNs (Table 9) than other treatments. The application of pyrazosulfuron fb cyhalofop fb bispyribac led to a reduction in RKN galls by 73.7% in rice plants by reducing weed density by 82.6% and had significantly lower RKN galls in rice than in other treatments (Table 8). Also, this had the lowest total PPNs (Table 9). It could reduce RKN, T. brevilineatus, P. thornei, and H. oryzeae by 27%, 71%, 82%, and 53% during 2018 and 19%, 65, 81, and 47% during 2019, respectively, compared to UWC. The RKN galls of weeds (~E. colona, E. crusgalli) and rice plants (Figures 2A,B) were significantly (p ≤ .01) positively correlated (r = 0.88**, 0.89**; R2 = 0.78, 0.79, respectively; n = 18), but the relationship was inverse (Figures 3A,B) between PPNs and rice grain yield [r = −0.745** (2018); r = −0.827**(2019), n = 72].
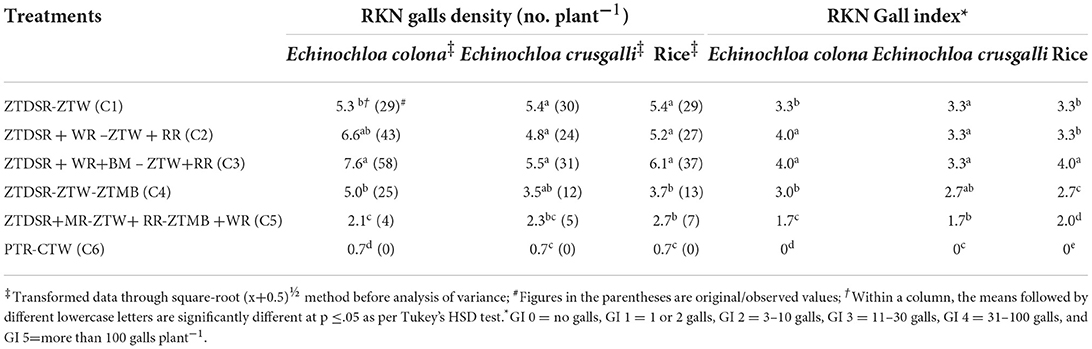
Table 7. Root-knot nematode (RKN) galls (no. plant−1) and gall index (GI) in weeds Echinochloa colona, Echinochloa crusgalli, and rice crop under unweeded control at 60 DAS/DAT across the conservation agriculture practices (mean of 2 years).
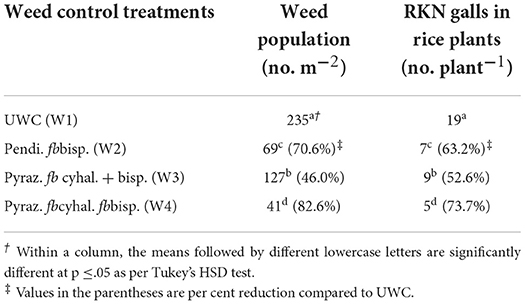
Table 8. Reduction in weed population (no. m−2) and root-knot nematode (RKN) galls in rice plants (no. plant−1) across the weed control/herbicides treatments at 60 DAS/DAT (Pooled mean of 2 years).
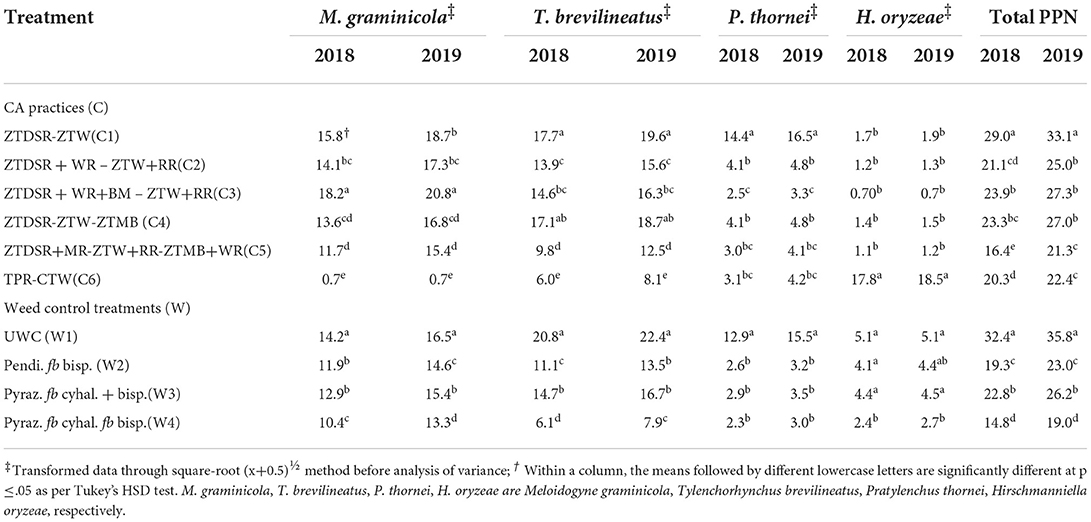
Table 9. Plant parasitic nematodes (PPN) population (no. 200 cc soil−1) across CA and weed control treatments in rice at 60 DAS/DAT during 2018 and 2019.
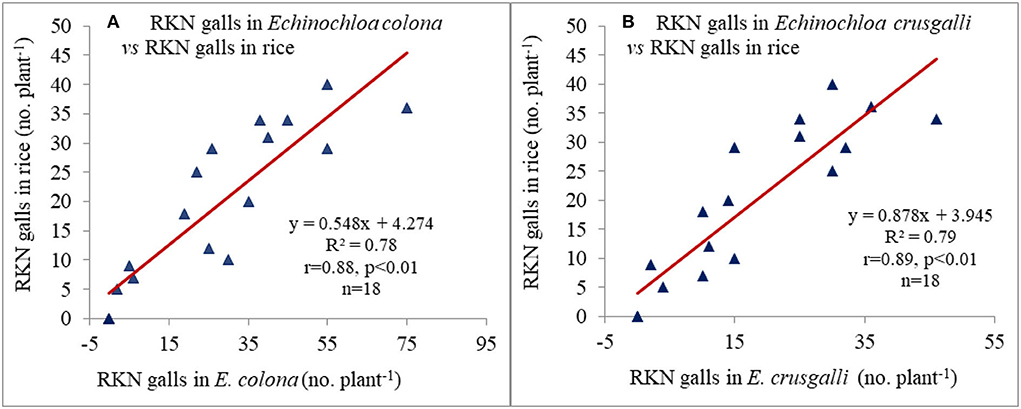
Figure 2. Relationship between root-knot nematode (RKN) galls in (A) Echinochloa colona and (B) Echinochloa crusgalli with RKN galls in rice (mean of 2 years).
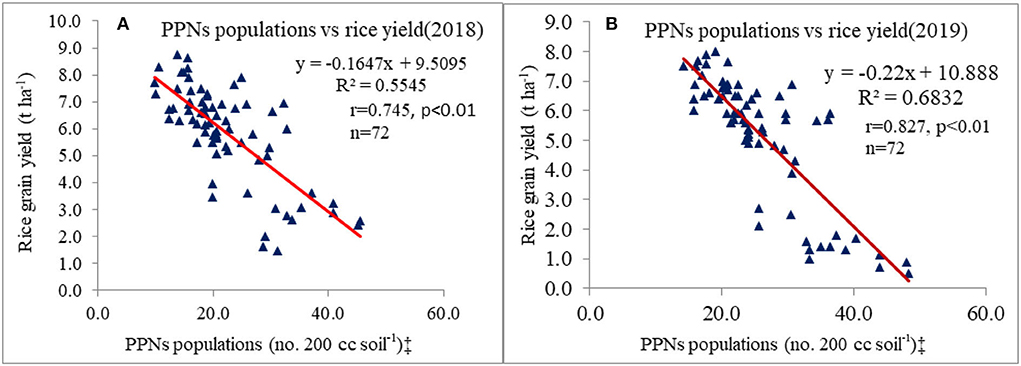
Figure 3. Relationship between plant parasitic nematodes (PPNs) populations and rice grain yield (A) during 2018 and (B) during 2019. ‡PPNs data were transformed through square-root (x + 0.5)½ method before correlation analysis.
Effects on rice grain yield and economics
Pooled ANOVA revealed that the mean effects of year, CA, and weed control practices, and their interactions (namely, Y × W; C × W) on rice grain yield were significant (Table 3). Rice grain yield was significantly higher in the 1st year (2018) than in the 2nd year (2019) (Table 10). Conventional PTR (C6) resulted in significantly higher rice yield than any DSRs (C1-C5) (Table 10). Among DSRs, the triple ZT system with three crops residue (C5) was most superior with 6.3, 22.4, 22.0, and 21.0% higher yield in 2018, and 13.1, 17.8, 22.1, and 22.7% higher yield in 2019 than C1, C2, C3, and C4, respectively. Among the weed control practices, the application of pyrazosulfuron fb cyhalofop fb bispyribac led to a significant increase in the yield than W1, W2, and W3 during both years (Table 10). This treatment increased 2 years' mean grain yield by 176.1, 19.6, and 7.7% than UWC, pyrazosulfuron fb tank-mix cyhalofop+bispyribac, and pendimethalin fb bispyribac, respectively. This herbicide treatment had significant interaction with CA, leading to comparable rice yields in C5 and C6, which were significantly higher than in other DSRs combined with these herbicides treatment (Table 10). The conventional farmers' practice (PTR-CTW; C6) incurred a higher cost of production (Figure 4) than all DSRs (C1–C5). The C1, C2, C3, C4, and C5 led to a reduction in the cost of production by US$ 235, 201, 174, 235, and 229 ha−1, respectively, compared to C6. The triple ZT system with three crops residue (C5) fetched comparable net returns with that of PTR-CTW and resulted in significantly higher net returns than those in the rest of the DSRs. The net benefit:cost (NB:C) was significantly higher due to C5 (1.80) than C6 (1.31) and other DSRs. Among herbicides/weed control treatments, the pyrazosulfuron fb cyhalofop fb bispyribac (W4) resulted in significantly higher net returns and NB:C than UWC and other herbicide treatments (Figure 4). This treatment (W4) obtained 10.2% higher net returns compared to pendimethalin fb bispyribac (W2), which is the farmers' herbicides/weed control practice adopted for rice in India.
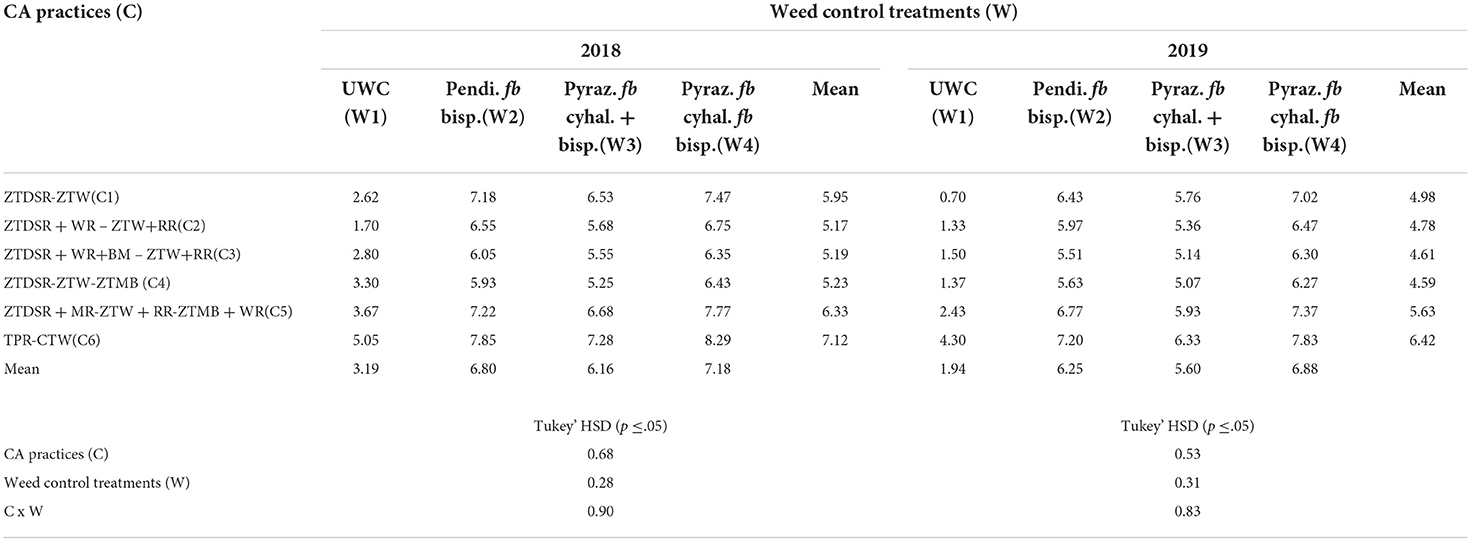
Table 10. Interaction between CA and weed control/herbicides treatments on grain yield of rice (t ha−1) in 2018 and 2019.
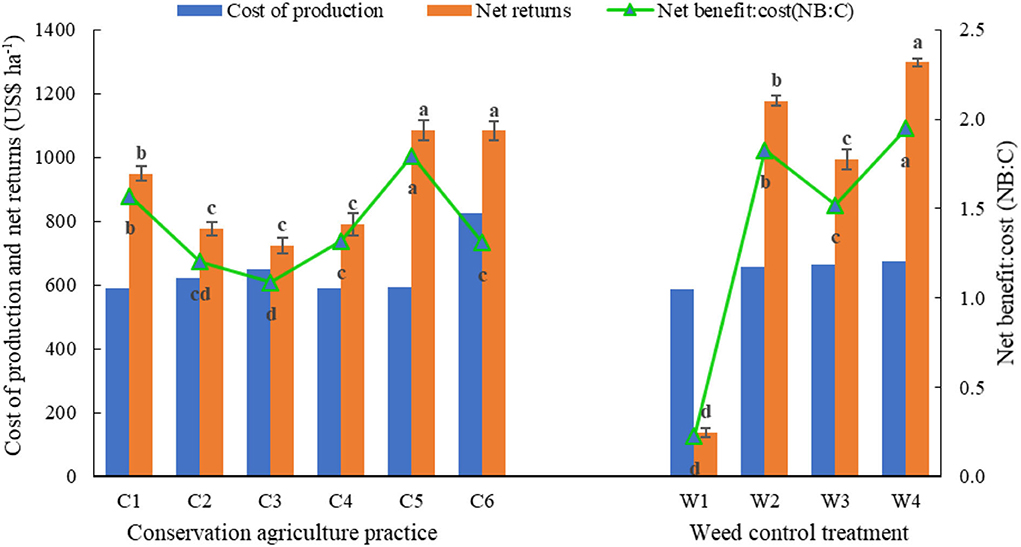
Figure 4. Benefit–cost economics of rice cultivation as influenced by CA and weed control practices (mean of two years). Mean having different lowercase letters on the vertical bars are significantly different at p ≤ 0.05 as per Tukey's HSD test. Vertical bars represent mean ± standard error. C1–C6 and W1–W4 are treatments mentioned in Table 1.
Herbicides residue in soil and rice grains and straw
Residues of all four herbicides (i.e., bispyribac, cyhalofop, pendimethalin, pyrazosulfuron) in rice grains and straw, and at 0–15 cm and 15–30 cm depth of soil were below the detectable level (BDL), except the negligible residue of pendimethalin varying from 0.013 to 0.018 mg kg−1 observed in upper 0–15 cm soil (Table 11). Pendimethalin residue was slightly higher in ZT residue-retained DSRs than PTR.
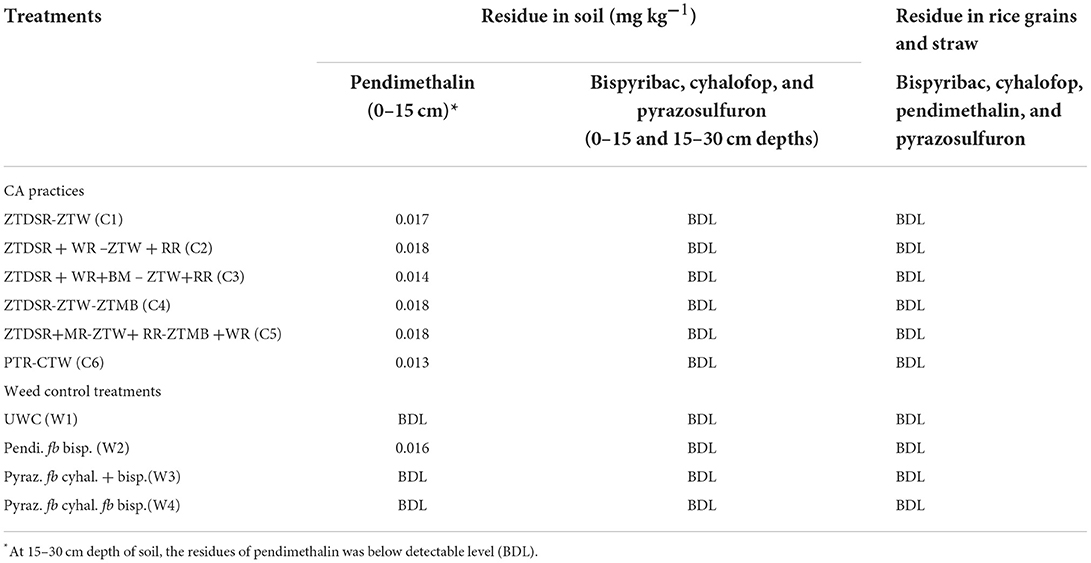
Table 11. Herbicides residue (mg kg−1) in soil (0–15 and 15–30 cm depths), and in rice grains and straw across the CA and weed control practices at harvest of rice crop.
Discussion
Weed dynamics and interference
Contrasting tillage and crop establishment practices followed for 8 years led to weed dynamics/diversity in rice. Under PTR, intensive tillage/puddling resulting in the deeper placement of weed seeds, and continuous standing water preventing weed germination, particularly of photoblastic weeds could reduce almost all weeds except E. crusgalli, which was higher in PTR due to its ecological preference for growing under stagnant water. On the contrary, ZTDSR (mean of five DSRs) had 83 and 56% higher weed density in 2018 and 2019, respectively, and higher densities of grassy weeds E. colona, D. aegyptium, D. retroflexa, and L. chinensis than PTR. Under negligible or no soil disturbance, the ZT plots had more weed seeds on the soil surface, particularly of small-seeded grassy weeds. Ample sunlight on the soil surface led to higher weed emergence (Chauhan and Opena, 2012), and their seed bank build-up (Mishra and Singh, 2012). A similar thing happened under the triple ZT system without residue (C4). Besides ZT, mungbean crop grown during summer provided a favorable microclimate through adequate moisture and lower/buffered soil temperature, promoting germination of annual broad-leaved weeds E. alba, P. niruri, and T. portulacastrum (Table 5), and sedges C. esculentus and C. rotundus (Table 6).
During summer (May and June), the C4 plot had a lower temperature at 0–5 and 5–15 cm soils (~30–34°C & 29–32°C, resp.) due to mungbean crop than the C1–C3 and C6 plots (44–52°C and 38–42°C, resp.) (Field experience). Higher 44–52°C temperature at 0–5 cm soil in latter plots (kept fallow during summer) led to little solarization and might prove lethal to many annual weed seeds and tubers of C. esculentus and C. rotundus. Webster (2003) reported that soil temperature of more than 45°C considerably reduced tuber viability of C. esculentus and C. rotundus, and C. esculentus tubers were more sensitive to heat than C. rotundus tubers. Crops residue also reduced weeds. Three crops (rice, wheat, and mungbean) residues under the mungbean-inclusive triple ZT system (C5) led to a considerable reduction in C. esculentus and C. rotundus (which were highly dominant in C4). The residue acts as a physical barrier to sunlight reducing weed germination and releasing allelo-chemicals into the soil (Jabran and Chauhan, 2015). Kumar et al. (2013) found that wheat residue suppressed E. crusgalli, E. colona, D. aegyptium, and E. alba in ZTDSR. Residue can also encourage weed seed foraging and predation actions by ants, insects, and birds and reduce surface seed bank.
Repeated weed flushes, crop stage-specific emergence of certain weeds, and new weed insurgence call for sound weed management in DSR (Jabran and Chauhan, 2015). In this study, ZT, residues (rice, wheat, mungbean, and brown manure crop Sesbania), and herbicides were adopted to pursue integrated weed management in DSR. The application of pyrazosulfuron fb cyhalofop fb bispyribac was most effective causing a significant reduction in densities of grassy, broad-leaved, and sedge weeds by 72, 60, and 43%, respectively (2-year mean). Pyrazosulfuron led to balanced control of early-emerging grassy, broad-leaved, and sedge weeds right from germination. Bispyribac controlled E. colona, E. crusgalli (grassy weeds); E. alba, P. niruri, A. philoxeroides, T. portulacastrum (broad-leaved weeds) effectively; and had little effect on C. esculentus, C. rotundus, C. difformis, and C. iria (sedges). It was not effective against newly emerged grassy weeds D. aegyptium, D. retroflexa, and L. chinensis. Cyhalofop applied in a sequence-controlled E. colona, E. crusgalli, D. retroflexa, L. chinensis, and E. indica effectively and D. aegyptium moderately. Thus, the sequential application of pyrazosulfuron fb cyhalofop fb bispyribac led to better weed control in DSR. The tank-mix of cyhalofop+bispyribac was inferior in controlling grassy weeds than their sequential application, probably, due to antagonism. Ottis et al. (2005) also reported antagonistic effects of cyhalofop with halosulfuron, triclopyr, and propanil in rice.
Nematodes dynamics and management
In this study, besides rice, five weeds (E. colona, E. crusgalli, D. retroflexa, E. indica, and E. alba) had RKN galls and were alternate hosts. These weeds are present in the global list of 24 weed hosts of RKN (Rich et al., 2009) except D. retroflexa, which might be a new host not reported earlier. Higher the weed density, the higher the population of RKN and total PPNs and vice-versa. The associations of these nematodes with weeds led to a reduction in rice yield. There was a direct relationship between RKN galls of these weeds and RKN galls in rice. Puddling and continuous submergence leading to the absence of some weeds or poor weed growth were responsible for the absence or negligible infestation of RKN, T. brevilineatus, and P. thornei in PTR (Table 9). In contrast, non-flooded aerobic soil conditions and intermittent irrigations were responsible for the higher infestation of PPNs under DSR (Jain et al., 2012; Kyndt et al., 2014). However, the triple ZT cropping system with residue (C5) led to reductions in RKN by 27, 15, 43, and 12% and T. brevilineatus by 66, 31, 38, and 60%, respectively, in soil compared to C1, C2, C3, and C4 (mean of 2 years) (Table 9). This CA practice also led to reducing RKN galls significantly in E. colona, E. crusgalli, and rice (Section 3.2). Rotation with non-host crop mungbean could be more useful to control RKN in this treatment. Besides, higher organic matter accumulation through rice, wheat, and mungbean residues and improved soil conditions led to the suppression of soil pathogens including PPNs (Kandel et al., 2017; Liu et al., 2019). Widmer et al. (2002) reported that adding organic matter through cover/rotational crop residue, green manure, compost, or organic amendment could influence PPNs and free-living nematodes. Rotating non-host crops such as mustard, sesame, and millet can also reduce RKN. Sesbania brown manuring was useful in maize (Das et al., 2020a), but Sesbania brown manuring (C3) invited more PPNs in DSR. Sesbania is a host of rice RKN and its decomposition releases some biocides in the rhizosphere stimulating nematodes might be the reason. The application of pyrazosulfuron fb cyhalofop fb bispyribac through better weed control led to 23, 66, 81, and 49% reduction in RKN, T. brevilineatus, P. thornei, and H. oryzeae, respectively, compared to UWC (mean of 2 years). Herbicides affect nematodes indirectly by altering the composition and density of weeds (Yeates et al., 1999), albeit sometimes they may have direct toxicity to nematodes. Das et al. (2010) reported the effect of atrazine and pendimethalin on PPNs. Herbicides causing mortality of weeds/host plants lead to non-availability of weeds/alternate host plants, which can bring down PPNs. Our results showed significant positive correlations between RKNs galls of weeds and rice (Figures 2A,B) and indicated the indirect effect of herbicides on RKNs. This conforms with Noling and Gilreath (2002) and Kutywayo and Been (2006).
Rice yield, economics, and herbicides residue
The variation over the years in rice yield was due to prevailing weather conditions, mainly rainfall, temperature, and sunshine (Supplementary Figures 1A,B). In 2018, higher rainfall (922.6 mm against 546.7 mm in 2019) from June to November (Supplementary Figure 1B), lower fluctuation in maximum and minimum temperatures (Supplementary Figure 1A) during the growth period, and greater availability of bright sunshine hours during the reproductive phase of rice led to better partitioning of photosynthates to grains and gave higher yield in both DSR and TPR. The DSRs, experiencing more biotic (weed and nematode) and abiotic (Fe deficiency and moisture) stresses had lower rice yield than PTR (Table 10). However, the triple ZTDSR with three crops residue (C5) gave a 9.4–22.0% higher yield than other ZTDSRs and was closer to PTR. This CA-based DSR, besides having better weed/nematode control, had better soil physical (aggregation, porosity, water content, soil strength) (Mondal et al., 2019) chemical (C and N accumulation) (Bhattacharyya et al., 2015) and biological (microbial biomass carbon, phosphatase, dehydrogenase, and β-glucosidase activities) conditions (Jat et al., 2020), which could help to achieve higher yield. Similarly, better weed control and consequently, a lower infestation of PPNs led to higher rice yield in the pyrazosulfuron fb cyhalofop fb bispyribac treatment. Relatively higher yield and lower cost of production made the triple ZT system with three crops residue (C5) superior to other DSRs (Figure 4), and comparable with PTR in terms of net returns, despite PTR having higher grain yield than C5. This amply highlighted that the CA-based DSR (C5) could be an equally remunerative alternative to PTR (Gathala et al., 2013; Baghel et al., 2020) and a more climate-resilient practice through a considerable reduction in methane emission (not reported here). The pyrazosulfuron fb cyhalofop fb bispyribac treatment required a slightly higher cost (Figure 4), mainly, due to the extra cost incurred on herbicides but a higher yield (176.1% higher than UWC) obtained in this treatment through better weed and nematode control led to higher net returns than other weed control treatments.
Our study indicates how weed control using herbicides indispensable for harnessing higher yield and income in DSR. Injudicious use of herbicides may have adverse effects on the environment and human health. We studied the herbicides at their recommended doses, which could hardly inflict any observable effect. For example, the residues of pendimethalin, pyrazosulfuron, bispyribac, and cyhalofop in rice grains and straw obtained from this study were below detectable levels, and rice grains and straw were safe for consumption by humans and animals, respectively. The FSSAI (2017) has already fixed the maximum residue limit (MRL) of pendimethalin, pyrazosulfuron, and bispyribac in rice grains as 0.05, 0.01, and 0.05 mg kg−1, respectively. In soil, a negligible amount of pendimethalin was detected at harvest but was safe for rotational crops like wheat (data not shown). All four herbicides were applied within 25 DAS, and rice was harvested after 115–120 DAS. A long time (~90–95 days) had elapsed, and herbicides were degraded in rice plants and soil through physical, chemical, and microbiological means, leading to below detectable levels of residues. Of course, a lower dose of application of these herbicides (< 100 g ha−1) except pendimethalin also played a role.
Conclusion
This study revealed that a CA-based triple ZT system, involving ZT direct-seeded rice (DSR) with mungbean residue - ZT wheat with rice residue - ZT mungbean with wheat residue combined with the application of pyrazosulfuron fb cyhalofop fb bispyribac could provide comparable rice yield through better weed and nematode control and would be an alternative to puddled transplanted rice (PTR). Among 14 weeds observed in rice, 5 (Echinochloa colona, Echinochloa crusgalli, Dinebra retroflexa, Eleusine indica, and Eclipta alba) were alternate hosts of root-knot nematodes (RKNs). Herbicides pyrazosulfuron fb cyhalofop fb bispyribac led to effective weed control in DSR. But, weed dynamics takes place over time in crop field ecosystem, and, therefore, dynamic herbicide recommendations through herbicide rotation may be resorted for better weed control in DSR in the future. Herbicide, crop residue, ZT, and non-host summer crops/mungbean would break the cycle of RKNs and reduce the infestations of RKNs and other plant parasitic nematodes (PPNs) in DSR. The direct effect of herbicides (pendimethalin, pyrazosulfuron, cyhalofop, or bispyribac) on these nematodes should be studied, which could not be studied in this study. Greater yield variability in ZTDSR should be addressed through focussed future research on developing newer varieties tolerant to various biotic (weeds, nematodes) and abiotic stresses (Fe and Zn deficiency and moisture shortage). This study would help to design integrated pest management modules involving interactions among weeds, nematodes, insect pests, and diseases, which might lead to a more productive and profitable CA-based DSR across diverse rice ecologies.
Data availability statement
The original contributions presented in the study are included in the article/Supplementary material, further inquiries can be directed to the corresponding author.
Author contributions
RR: conceptualization, investigation, and writing-original draft preparation. TD: conceptualization, methodology, and writing–reviewing and editing. P: methodology. TB: methodology and investigation. AG and RB: reviewing and editing. DC and SP: editing. SB: writing, review, and editing. VK and SS: original draft preparation. SG: methodology. All authors contributed to the article and approved the submitted version.
Acknowledgments
The authors gratefully acknowledge the necessary services and supplies provided by the Division of Agronomy, the Division of Nematology, and the Division of Agricultural Chemicals, ICAR–Indian Agricultural Research Institute, New Delhi during the investigation.
Conflict of interest
The authors declare that the research was conducted in the absence of any commercial or financial relationships that could be construed as a potential conflict of interest.
Publisher's note
All claims expressed in this article are solely those of the authors and do not necessarily represent those of their affiliated organizations, or those of the publisher, the editors and the reviewers. Any product that may be evaluated in this article, or claim that may be made by its manufacturer, is not guaranteed or endorsed by the publisher.
Supplementary material
The Supplementary Material for this article can be found online at: https://www.frontiersin.org/articles/10.3389/fsufs.2022.1017013/full#supplementary-material
References
Abbas, A., Khaliq, A., Saqib, M., Majeed, M. Z., Ullah, S., and Haroon, M. (2019). Influence of tillage systems and selective herbicides on weed management and productivity of direct-seeded rice (Oryza sativa). Planta Daninha. 37, e019186252 doi: 10.1590/s0100-83582019370100083
Awan, T. H., Cruz, P. C. S., and Chauhan, B. S. (2015). Efficacy and economics of different herbicides, their weed species selectivity, and the productivity of mechanized dry-seeded rice. Crop Prot. 78, 239–246.. doi: 10.1016/j.cropro.2015.09.016
Baghel, J. K., Das, T. K., Pankaj, M.ukherjee, I., Nath, C. P., Bhattacharyya, R., Ghosh, S., et al. (2020). Impacts of conservation agriculture and herbicides on weeds, nematodes, herbicide residue and productivity in direct-seeded rice. Soil Till. Res. 201, 104634. doi: 10.1016/j.still.2020.104634
Behera, B., and Das, T. K. (2019). Brown manure species, weeds and maize in a co-culture in the field: who stands more competitive? Pestic. Res. J. 31, 129–134. doi: 10.5958/2249-524X.2019.00001.3
Behera, B., Das, T. K., Ghosh, S., Kaur, R., and Singh, R. (2018). Brown manuring in maize (Zea mays): Effects on weed interference and crop productivity. Indian J. Agron. 63, 524–527.
Bhattacharyya, R., Das, T. K., Sudhishri, S., Dudwal, B., Sharma, A. R., Bhatia, A., et al. (2015). Conservation agriculture effects on soil organic carbon accumulation and crop productivity under a rice–wheat cropping system in the western Indo-Gangetic Plains. Eur. J. Agron. 70, 11–21. doi: 10.1016/j.eja.2015.06.006
Bridge, J., Plowright, R. A., and Peng, D. (2005). “Nematode parasites of rice,” in Plant Parasitic Nematodes in Subtropical and Tropical Agriculture Luc, M., Sikora, R.A., Bridge, J. (Eds.). Wallingford, UK: CABI. p. 87–12 doi: 10.1079/9780851997278.0087
Chandel, S. T., Gaur, H. S., and Alam, M. M. (2002). Population dynamics of the root knot nematode Meloidogyne tricicoryzae under five rice-based cropping system. Arch. Phytopathol. Plant Prot. 35, 43–51. doi: 10.1080/0323540021000009588
Chauhan, B. S., Awan, T. H., Abugho, S. B., Evengelista, G., and Yadav, S. (2015). Effect of crop establishment methods and weed control treatments on weed management, and rice yield. Field Crop Res. 172, 72–84. doi: 10.1016/j.fcr.2014.12.011
Chauhan, B. S., and Opena, J. (2012). Effect of tillage systems and herbicides on weed emergence, weed growth, and grain yield in dry-seeded rice systems. Field Crop Res. 137, 56–69. doi: 10.1016/j.fcr.2012.08.016
Coyne, D. L., Nicol, J. M., and Claudius-Cole, B. (2007). Practical Plant Nematology: A Field and Laboratory Guide. Cotonou, Benin: SP-IPM Secretariat, International Institute of Tropical Agriculture (IITA).
Das, T. K., and Das, D. K. (2018). Using chemical seed dormancy breakers with herbicides for weed management in soybean and wheat. Weed Res. 58, 188–199. doi: 10.1111/wre.12295
Das, T. K., Ghosh, S., and Nath, C. P. (2020a). Brown manuring optimization in maize: impacts on weeds, crop productivity and profitability. J. Agric. Sci. (Cambridge). 157, 599–610. doi: 10.1017/S0021859620000064
Das, T. K., Nath, C. P., Das, S., Biswas, S., Bhattacharyya, R., Sudhishri, S., et al. (2020b). Conservation Agriculture in rice-mustard cropping system for five years: impacts on crop productivity, profitability, water-use efficiency, and soil properties. Field Crops Res. 250, 107781. doi: 10.1016/j.fcr.2020.107781
Das, T. K., Saharawat, Y. S., Bhattacharyya, R., Sudhishri, S., Bandyopadhyay, K. K., Sharma, A. R., et al. (2018). Conservation agriculture effects on crop and water productivity, profitability and soil organic carbon accumulation under a maize-wheat cropping system in the North-western Indo-Gangetic Plains. Field Crops Res. 215, 222–231. doi: 10.1016/j.fcr.2017.10.021
Das, T. K., Sakhuja, P. K., and Zelleke, H. (2010). Herbicide efficacy and non-target toxicity in highland rainfed maize of Eastern Ethiopia. Int. J. Pest Manag. 56, 315–325. doi: 10.1080/09670874.2010.497872
Devaraja, K. P., and Pankaj, Sirohi, A. (2018). The effect of root-knot nematode, Meloidogyne graminicolaon the growth of direct-seeded rice cultivars. J. Entomol. Zool. Stud. 6, 2929–2933.
Elling, A. A. (2013). Major emerging problems with minor Meloidogyne species. Phytopathol. 103, 1092–1102. doi: 10.1094/PHYTO-01-13-0019-RVW
FAO (2020). What is Conservation Agriculture? Available online at: http://www.fao.org/conservation-agriculture/overview/what-is-conservation-agriculture/en/. (accessed April 24, 2020).
Farooq, M., Siddique, K. H. M., Rehman, R., Aziz, T., Lee, D., and Wahid, A. (2011). Rice direct seeding: experiences, challenges and opportunities. Soil Tillage Res. 111, 87–98 doi: 10.1016/j.still.2010.10.008
FSSAI (2017). Food Safety and Standards (Contaminants, Toxins, and Residues) Amendment Regulations (Gazette notification 27 December, 2017). New Delhi: Food Safety and Standards Authority of India (FSSAI), Ministry of Health and Family Welfare, Govt. of India.
Gathala, M. K., Kumar, K., Sharma, P. C., Saharawat, Y. S., Jat, J. S., Singh, M., et al. (2013). Optimizing intensive cereal-based cropping systems addressing current and future drivers of agricultural change in the northwestern Indo-Gangetic Plains of India. Agric. Ecosys. Environ. 177, 85–97. doi: 10.1016/j.agee.2013.06.002
Gaur, H. S., and Singh, A. K. (1993). Effect of puddling practices and nitrogen levels on the root-knot nematode infecting wheat and rice. Indian J. Nematol. 23, 4.
Gharde, Y., Singh, P. K., Dubey, R. P., and Gupta, P. K. (2018). Assessment of yield and economic losses in agriculture due to weeds in India. Crop Prot. 107, 12–18. doi: 10.1016/j.cropro.2018.01.007
Ghosh, S., Das, T. K., Sharma, D. K., and Gupta, K. (2019). Potential of conservation agriculture for ecosystem services: a review. Indian J. Agric. Sci. 89, 1572–1579.
Gupta, D. K., Bhatia, A., Kumar, A., Das, T. K., Jain, N., Tomer, R., et al. (2016). Mitigation of greenhouse gas emission from rice–wheat system of the Indo-Gangetic plains: Through tillage, irrigation and fertilizer management. Agric. Ecosyst. Environ. 230, 1–9. doi: 10.1016/j.agee.2016.05.023
Jabran, K., and Chauhan, B. S. (2015). Weed management in aerobic rice systems. Crop Prot. 78, 151–163. doi: 10.1016/j.cropro.2015.09.005
Jain, R. K., Khan, M. R., and Kumar, V. (2012). Rice root-knot nematode (Meloidogyne graminicola) infestation in rice. Arch. Phytopathol. Plant Prot. 45, 635–645. doi: 10.1080/03235408.2011.588059
Jat, H. S., Choudhary, M., Datta, A., Yadav, A. K., Meena, M. D., Devi, R., et al. (2020). Temporal changes in soil microbial properties and nutrient dynamics under climate smart agriculture practices. Soil Tillage Res. 199, 104595. doi: 10.1016/j.still.2020.104595
Jones, J., Haegeman, A., Danchin, E. G. J., Gaur, G. S., Helder, J., Jones, M. G. K., et al. (2013). Top 10 plant-parasitic nematodes in molecular plant pathology. Mol. Plant Pathol. 14, 946–961. doi: 10.1111/mpp.12057
Kandel, S. L., Smiley, R. W., Campbell, K. G., Elling, A. A., Huggins, D., and Paulitz, T. C. (2017). Spatial distribution of root lesion nematodes (Pratylenchus spp) in a long-term no-till cropping system and their relationship with soil and landscape properties. Eur. J. Plant Pathol. DOI 10.1007/s10658-017-1341-3. doi: 10.1007/s10658-017-1341-3
Kassam, A., Friedrich, T., and Derpsch, R. (2018). Global spread of conservation agriculture. Int. J. Environ. Stud. doi: 10.1080/00207233.2018.1494927
Khan, M. R. (2015). “Nematode diseases of crops in India,” in Recent Advances in the Diagnosis and Management of Plant Diseases. Awasthi, L. P. (ed). New Delhi: Springer. p 183–224. doi: 10.1007/978-81-322-2571-3_16
Kumar, V., Khan, M. R., and Walia, R. K. (2020). Crop loss estimations due to plant-parasitic nematodes in major crops in India. Natl. Acad. Sci. Lett. 43, 409–412. doi: 10.1007/s40009-020-00895-2
Kumar, V., and Ladha, J. K. (2011). Direct seeding of rice: recent developments and future research needs. Adv. Agron. 111, 296–413. doi: 10.1016/B978-0-12-387689-8.00001-1
Kumar, V., Singh, S., Chhokar, R. S., Malik, R. K., Brainard, D. C., and Ladha, J. K. (2013). Weed management strategies to reduce herbicide use in zero-till rice-wheat cropping systems of the Indo-Gangetic Plains. Weed Technol. 27, 241–254. doi: 10.1614/WT-D-12-00069.1
Kutywayo, V., and Been, T. H. (2006). Host status of six major weeds to Meloidogyne chitwoodi and Pratylenchus penetrans, including a preliminary field survey concerning other weeds. Nematol. 8, 647–657. doi: 10.1163/156854106778877839
Kyndt, T., Fernandez, D., and Gheysen, G. (2014). Plant-parasitic nematode infections in rice: Molecular and cellular insights. Annu. Rev. Phytopathol. 52, 135–153. doi: 10.1146/annurev-phyto-102313-050111
Ladha, J. K., Kumar, V., Alam, M. M., Sharma, S., Gathala, M. K., Chandna, P., et al. (2009). “Integrating crop and resource management technologies for enhanced productivity, profitability and sustainability of the rice—wheat system in South Asia,” in Integrated Crop and Resource Management in the Rice–Wheat System of South Asia Ladha, J. K. (Ed.). Los Baños, the Philippines: IRRI. p. 69–108.
Liu, T., Feng Hu, F., and Li, H. (2019). Spatial ecology of soil nematodes: Perspectives from global to micro scales. Soil Biol. Biochem. 137, 107565. doi: 10.1016/j.soilbio.2019.107565
Mantelin, S., BellafIore, S., and Kyndt, T. (2017). Meloidogyne graminicola: a major threat to rice agriculture. Mol. Plant Pathol. 18, 3–15. doi: 10.1111/mpp.12394
Mishra, J. S., and Singh, V. P. (2012). Tillage and weed control effects on productivity of a dry seeded rice–wheat system on a Vertisol in Central India. Soil Tillage Res. 123, 11–20. doi: 10.1016/j.still.2012.02.003
Mohammad, A., Sudhishri, S., Das, T. K., Singh, M., Bhattacharyya, R., Dass, A., et al. (2018). Water balance in direct-seeded rice under conservation agriculture in north-western Indo-Gangetic plains of India. Irrigation Sci. 36, 381–393.doi: 10.1007/s00271-018-0590-z
Mondal, S., Chakraborty, D., Das, T. K., Shrivastava, M., Mishra, A. K., Bandyopadhyay, K. K., et al. (2019). Conservation agriculture had a strong impact on the sub-surface soil strength and root growth in wheat after a 7-year transition period. Soil Tillage Res. 195, 104385 doi: 10.1016/j.still.2019.104385
Nawaz, A., Farooq, M., Lal, R., Rehman, A., Hussain, T., and Nadeem, A. (2017a). Influence of sesbania brown manuring and rice residue mulch on soil health, weeds and system productivity of conservation rice–wheat systems. Land Degrad. Develop. 28, 1078–1090. doi: 10.1002/ldr.2578
Nawaz, A., Farooq, M., Lal, R., Rehman, A., and Rehman, H. (2017b). Comparison of conventional and conservation rice-wheat systems in Punjab, Pakistan. Soil Tillage Res.169, 35–43. doi: 10.1016/j.still.2017.01.012
Noling, J. W., and Gilreath, J. P. (2002). “Weed and nematode management: simultaneous considerations,” in MBAO. Ann. Int. Res. Conf. on Methyl Bromide Alternatives and Emissions Reductions 6. Orlando, FL; Fresno, CA: Methyl Bromide Alternatives Outreach. p. 1.
Oerke, E. C. (2006). Crop losses to pests: centenary review. J. Agric. Sci. 144, 31–43. doi: 10.1017/S0021859605005708
Ornat, C., and Sorribas, F. J. (2008). “Integrated management of root-knot nematodes in Mediterranean horticultural crops,” in Integrated Management and Biocontrol of Vegetable and Grain Crops Nematodes. Netherlands: Springer. p. 295–319. doi: 10.1007/978-1-4020-6063-2_14
Ottis, B. V., Mattice, J. D., and Talbert, R. E. (2005). Determination of antagonism between cyhalofop-butyl and other rice (Oryza sativa) herbicides in barnyard grass (Echinochloa crus-galli). J. Agric. Food Chem. 53, 4064–4068. doi: 10.1021/jf050006d
Pandey, B. P., and Kandel, T. P. (2020). Response of rice to tillage, wheat residue and weed management in a rice-wheat cropping system. Agronomy. 10, 1734. doi: 10.3390/agronomy10111734
Pankaj, S. H. K., Gaur, H. S., and Singh, A.K. (2006). Effect of zero tillage on the nematode fauna in a rice-wheat cropping system. Nematol. Medit. 34, 175–178.
Pankaj, S. H. K., Singh, K., and Lal, J. (2015). Management of rice root knot nematode, Meloidogyne graminicolain rice (Oryza sativa). Indian J. Agric. Sci. 85, 701–704. Available online at: https://epubs.icar.org.in/index.php/IJAgS/article/view/48511
Pederson, G. A., and Windham, G. L. (1989). Resistance to Meloidogynae incognita in Trifolium interspecific hybrids and species related to white clover. Plant Disease 73, 567–569. doi: 10.1094/PD-73-0567
Pokharel, R. R., Abawi, G. S., Zhang, N., Duxbury, J. M., and Smart, C. D. (2007). Characterization of isolates of Meloidogyne from rice-wheat production fields in Nepal. J. Nematol. 39, 221.
Raj, R., Kumar, A., Kumar, V., Singh, C. B., and Pandey, U. C. (2016). Herbicide options for controlling weeds in transplanted rice (Oryza sativa) under North Eastern Plains Zone. Indian J. Agron. 61, 197–203.
Raj, R., Kumar, A., Solanki, I. S., Dhar, S., Dass, A., Gupta, A. K., et al. (2017). Influence of crop establishment methods on yield, economics and water productivity of rice cultivars under upland and lowland production ecologies of Eastern Indo-Gangetic Plains. Paddy Water Environ. 15, 861–877. doi: 10.1007/s10333-017-0598-7
Rao, A. N., Johnson, D. E., Sivaprasad, B., Ladha, J. K., and Mortimer, A. M. (2007). Weed management in direct seeded rice. Adv. Agron. 93, 153–255. doi: 10.1016/S0065-2113(06)93004-1
Rich, J. R., Brito, J. A., Kaur, R., and Ferrell, J. A. (2009). Weed species as hosts of Meloidogyne: a review. Nematropica 39, 157–185.
Schenck, F. J., and Hobbs, J. E. (2004). Evaluation of the Quick, Easy, Cheap, Effective, Rugged, and Safe (QuEChERS) approach to pesticide residue analysis. Bull. Environ. Contam.Toxicol. 73, 24–30. doi: 10.1007/s00128-004-0388-y
Sen, S., Kaur, R., Das, T. K., Raj, R., and Shivay, Y. S. (2021). Impacts of herbicides on weeds, water productivity, and nutrient-use efficiency in dry direct-seeded rice. Paddy Water Environ. 19, 227–238. doi: 10.1007/s10333-020-00834-3
Southey, J. F. (1986). Laboratory methods for work with plant and soil nematodes. Reference Book 402. London, UK: Her Majesty's Stationery Office (HMSO). Ministry of Agriculture, Fisheries and Food.
Suong, M., Chapuis, E., Leng, V., Tivet, F., Waele, D. D., Thi, H. N., et al. (2019). Impact of a conservation agriculture system on soil characteristics, rice yield, and root-parasitic nematodes in a Cambodian lowland rice field. J. Nematol. 51, e2019-85. doi: 10.21307/jofnem-2019-085
Webster,T.M. (2003). High temperatures and durations of exposure reduce nutsedge (Cyperus spp) tuber viability. Weed Sci. 51, 1010–1015. doi: 10.1614/WS-03-018R
Widmer, T. L., Mitkowski, N. A., and Abawi, G. S. (2002). Soil organic matter and management of plant-parasitic nematodes. J. Nematol. 34, 289–295.
X-rates (2017). Exchange Rates.to=USD. Available online at: http://www.x-rates.com/average/from=INR& (accessed May 3, 2022).
Yadav, D. B., Yadav, A., Vats, A. K., Gill, G., and Malik, R. K. (2021). Direct seeded rice in sequence with zero-tillage wheat in north-western India: addressing system-based sustainability issues. SN Appl. Sci. 3, 844. doi: 10.1007/s42452-021-04827-7
Yeates, G. W., Wardle, D. A., and Watson, R. N. (1999). Responses of soil nematodes populations, community structure, diversity and temporal variability to agricultural intensification over a seven-year period. Soil Biol. Biochem. 31, 1721–1733. doi: 10.1016/S0038-0717(99)00091-7
Keywords: bispyribac-Na, cyhalofop-butyl, LC-MS/MS, plant parasitic nematodes, QuEChERS, root-knot nematode, Sesbania brown manuring, grain yield
Citation: Raj R, Das TK, Pankaj, Banerjee T, Ghosh A, Bhattacharyya R, Chakraborty D, Prasad S, Babu S, Kumar V, Sen S and Ghosh S (2022) Co-implementation of conservation tillage and herbicides reduces weed and nematode infestation and enhances the productivity of direct-seeded rice in North-western Indo-Gangetic Plains. Front. Sustain. Food Syst. 6:1017013. doi: 10.3389/fsufs.2022.1017013
Received: 11 August 2022; Accepted: 23 September 2022;
Published: 21 October 2022.
Edited by:
Gopal Shukla, Uttar Banga Krishi Viswavidyalaya, IndiaReviewed by:
Md Abdur Rouf Sarkar, Bangladesh Rice Research Institute, BangladeshNarendra Kumar, Indian Institute of Pulses Research (ICAR), India
Copyright © 2022 Raj, Das, Pankaj, Banerjee, Ghosh, Bhattacharyya, Chakraborty, Prasad, Babu, Kumar, Sen and Ghosh. This is an open-access article distributed under the terms of the Creative Commons Attribution License (CC BY). The use, distribution or reproduction in other forums is permitted, provided the original author(s) and the copyright owner(s) are credited and that the original publication in this journal is cited, in accordance with accepted academic practice. No use, distribution or reproduction is permitted which does not comply with these terms.
*Correspondence: T. K. Das, dGtkYXM2NEBnbWFpbC5jb20=