- 1Institute for Crop Science and Resource Conservation, Crop Science, University of Bonn, Bonn, Germany
- 2Faculty of Agriculture, Plant Production and Genetics Department, Shahid Chamran University of Ahvaz, Ahvaz, Iran
Introduction: Soil organic carbon (SOC) dynamic is one of the important factors that directly influence soil properties and quality. In agro-ecosystems, the SOC dynamics are strongly linked to agricultural management practices.
Methods: In this study, we investigated the response of SOC and its fractions to various combination of agricultural management practices based on measurements obtained from an experiment conducted over four growing seasons from 2018 to 2020 in Ahvaz, Iran. The experimental treatments involved three agricultural strategies combined with four crop rotation systems. The agricultural strategies comprised conventional (CON: mineral fertilizer, removal of all crop residues), organic (ORG: organic fertilizer, 30% return of crop residues to the soil), and integrated (INT: mineral/organic fertilizer, 15% return of crop residues) strategies. The crop rotation systems were: fallow-wheat (F-W), corn-wheat (C-W), sesame-wheat (S-W), and mung bean-wheat (B-W). Soil samples were collected from all treatments and SOC, labile-C, and non-labile-C were measured.
Results and discussion: After two years of experiment, no significant improvement was found in SOC of CON strategy (p ≤ 0.05). The ORG and INT strategies contained on average 1.1 and 1.06 times more SOC than the CON strategy, respectively. The value of labile-C was decreased during summer cultivations and increased in the soil samples collected after winter cultivations. However, although the quantity of labile-C in ORG (2 g kg−1) was higher than INT (1.83 g kg−1) and CON (1.87 g kg−1) overall during the experiment time, after the second summer cultivation despite the gradual accumulation of organic matter due to high levels of temperature and humidity, the content in ORG reduced to1.47 g kg−1. In all three agricultural management strategies, the SOC content in the four rotation systems was according to the following descending order B-W (5.7 g kg−1) > C-W (5.29 g kg−1) > S-W (5.23 g kg−1) > F-W (4.52 g kg−1). Therefore, for this region M-W and S-W crop rotation systems are recommended in addition to C-W (which is the most common rotation system). However, crop rotation systems were more beneficial for C-sequestration when combined with organic and inorganic fertilization and crop residue incorporation. This study gives promising results for implementing INT and ORG strategies under long-term cropping systems containing various summer crops in rotation with wheat for improving SOC dynamics in semi-arid regions in Iran.
1. Introduction
Agroecosystems are the largest carbon (C) pools in terrestrial ecosystems, with global storage of over 1,550 GT (Batjes, 1996). In fact, cropland soils alone account for 590–1,180 million tons of C that are stored as soil organic matter originating from crop residues and manure (FAO., 2002). SOC sequestration has been recognized as one of the main agents in improving ecosystem services including soil quality upgrades by improving soil organic matter and total nitrogen (Khormali et al., 2009), retention of water and nutrients (Tisdall and Oades, 1982), increasing agricultural productivity (Plaza-Bonilla et al., 2016), improving soil aggregate stability and soil structure (Ayoubi et al., 2012; Zeraatpisheh et al., 2021), control of erosion (Rahimi et al., 2013; Li et al., 2016), and enhancing surface and subsurface water quality (Laird, 2008). Sequestration of C in soils is also a natural process to mitigation of global warming through reduction in atmospheric CO2 levels (Afshar et al., 2010; Brandão et al., 2013). Soil C pools, which are an important energy source for the soil food web (Chan et al., 2001) and improving nutrient cycling, are primarily the labile organic carbon pools (Luo et al., 2019). They comprise fractions of SOC pools with most rapid turnover times from days to months (Li et al., 2018). These fractions affect soil formation and its functions in multiple ways and are recognized as indicators for representing soil quality (Benbi et al., 2015). Potassium permanganate oxidizable organic C (KMnO4-C, here after labile-C) is one of the labile-C fractions which is estimated by the chemical oxidation method using KMnO4 (Blair et al., 1995). It has been suggested as the most sensitive indicator to investigate the effect of agricultural management strategies e.g., organic and inorganic fertilizer application, returning crop residues to the soil, crop rotation systems, and tillage regimes on dynamics of soil organic labile-C (Plaza-Bonilla et al., 2014; Blanco-Moure et al., 2016). The non-labile-C fraction, which has remarkably low degrees of stabilization decomposition over time, and had high recalcitrant to conversion in response to agricultural practices can be calculated based on the difference between SOC and labile-C (Majumder et al., 2008).
Many agricultural practices have been adopted as restorative management strategies to improve soil fertility by storing more C in soils. These include: green manure incorporation into the soil (Maltas et al., 2018), reduced tillage (Calegari et al., 2008), crop rotation (Gregorich et al., 2001), crop residues managements (Chalise et al., 2018; Stella et al., 2019; Radicetti et al., 2020), and nutrient management (Havlin, 2020), all which influence the amount of C retained in the soil. For instance, integrating organic manure with mineral fertilizers (Diekow et al., 2005; Liu et al., 2014; Maltas et al., 2018), returning crop residues to the soil instead of removing them (Liu et al., 2013), improvement in root and shoot biomass as a result of using mineral fertilizers (Cambardella and Elliott, 1992; Bhattacharyya et al., 2012) are among practices that have been introduced to improve the amount of SOC and labile-C levels. The technical potential of these management practices on SOC improvement varies in the range of 100–1,000 kgC ha−1 year−1 based on the soil properties and climate conditions across different regions globally (Lal, 2010). Moreover, along with organic and inorganic resources management, appropriate rotation of crops is an important factor in SOC dynamics. For example, the inclusion of legumes in different crop rotation systems considerably promoted SOC pools compared to cereal-cereal cropping system and sole cereal cropping (Liu et al., 2020).
Despite the abundance of reseaches revealing the positive effects of different combinations of crop rotation and agricultural strategies on C-sequestration (Diekow et al., 2005; Ding et al., 2012; Yadav et al., 2019), so far few studies have explored the variations of SOC and labile-C dynamics in semi-arid regions (Maia et al., 2007) comprising summer crops cultivation in rotation with winter crops (like wheat) i.e., two growing seasons in 1 year. Understanding these dynamics under such particular cropping systems is crucial for two reasons. First, since the weather during two growing seasons of these regions vary between dry in summer and wet in winter periods, it is still not clear if the dynamics remain similar under both conditions. Second, considering the fact that arable lands in semi-arid regions have small SOC pools, it is vital to optimize fertilization, crop rotation systems, and crop residues management in order to alleviate the environmental impacts and ameliorate SOC pools (Samal et al., 2017; Chen et al., 2018; Li et al., 2018).
With this study, we aim to contribute to the literature on this topic by investigating the role of various crops planted in rotation with wheat in combination with residue management and organic fertilizer application in semi-arid regions. Khuzestan province, located in the southwestern part of Iran, has been selected as a case study in semi-arid area. The region is the main center to produce high quality and quantity grain wheat with the 1.37 million Mg ha−1 year−1 productivity (Iran Wheat Flour Exported to Oman, Italy, Iraq, 2021) in the country. Continuous monocropping and wheat-maize cultivation as two conventional cropping systems (Hajabbasi and Hemmat, 2000; Kabiri et al., 2015) together with high temperature (Cooper et al., 2011) in the most parts of Khuzestan province have led to depletion of soil organic carbon (SOC) stocks. In addition, the values of SOC in semi-arid regions are fundamentally low owing to high levels of oxidation (Maia et al., 2019). Due to intensive farming, about 50%−67% of the original SOC pools in cultivated soils can be lost in semi-arid regions (cumulative amount about 30–40 t C ha−1) (Lal, 2018). Continuation of these practices will probably result in further degradation of soil quality and fertility through reducing C pools (Yu et al., 2006) which directly led to a decline in crop productivity (Chauhan et al., 2012). Therefore, in this study, we look at the contribution of various agricultural practices for SOC sequestration.
More specifically, the objectives of this study are to: (i) determine the changes in lability of C in summer crop cultivation (under high temperatures) and winter wheat crop (under wet conditions), (ii) find out the response of SOC dynamics and its fractions to various strategies of organic/inorganic fertilizers application and crop residues management in semi-arid regions, and (iii) assess and explain the effect of combinations of various fertilizing/residue management strategies with different crop rotation systems on the dynamics of SOC and its fractions.
2. Materials and methods
2.1. Study area
Khuzestan province (48°41E and 31°20N, altitude: 22.5 m), located in the southwestern part of Iran (Figure 1A), is one of several regions in the country known as a capital of wheat production (1.7 million Mg year−1). The average temperature in the region is 25°C with a maximum and minimum temperature of 48 and 4°C in summer and winter, respectively. The average annual precipitation is 213 mm yr−1 with the highest intensity in January (IRIMO, 2020; Figure 1B). The dominant soil texture in the topsoil in this experimental site was sandy-loam with 67% sand, 21% silt, 12% clay (FAO, 2021), 0.039% total nitrogen, and total 0.45% C. Additional soil characteristics include 26 kg ha−1 total phosphorus (organic and inorganic forms, Self-Davis et al., 2000) and 318 kg ha−1 available potassium content (Dandwate, 2020). Saturated soil suspension with a ratio of 1 unit of soil: 2 units of water had an electrical conductivity of 3.4 dS m−1 and pH of 7.8. According to the USDA, the soil of the experimental field was classified as aridisol (Baillie, 2006).
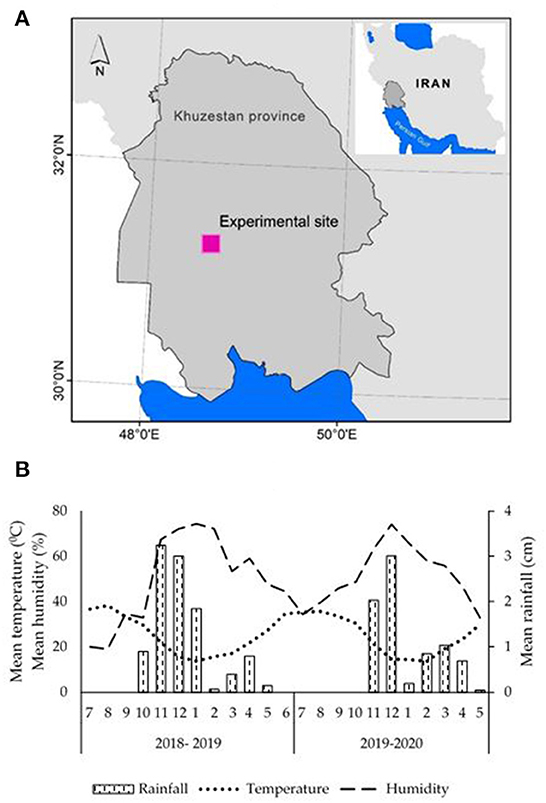
Figure 1. (A) Location of the experimental site in Iran, (B) Monthly average of rainfall, mean temperature, and humidity at the experimental site during 2018–2020.
The total agricultural lands in the province are comprised of 700,000 ha irrigated and 500,000 ha rainfed arable. The staple crops cultivated in Khuzestan province are wheat, sugarcane, corn, alfalfa, and rice. The province is known for a good quality of wheat that contains 12%−13% protein and 28%−30% wet gluten (Iran Wheat Flour Exported to Oman, Italy, Iraq, 2021). The dominant cropping system in most regions is a conventionally grown double-cropping rotation of winter wheat together with summer maize/rice. However, some regions grow winter wheat as monocropping.
Khuzestan province is an emitter of CO2 emissions due to the presence of oil and gas industry. About 1,529.4 Mg day−1 of CO2 is emitted in the region, which is 34% of the total national CO2 emission (Kamali et al., 2009; CO2 Country Profile., 2021). Although increased CO2 levels are beneficial for crops in terms of the raw material required for photosynthesis, the benefit remains only as long as increasing temperatures do not reduce the growth period of the plant. Therefore, agricultural management practices (e.g., crop rotation systems, methods of fertilization, crop residue management, and tillage types) are crucial for mitigating the amount of produced CO2.
2.2. Experimental design
A two-factorial randomized complete block design with a plot size of 12 m2 (3 × 4 m; Hoshmand, 2018) was applied. Treatments were comprised of three different fertilizer and crop residue management strategies as: conventional (CON), organic (ORG), and integrated management strategy (INT) which are combined with four types of crop rotation system (Supplementary Figure S1). In total, 12 treatments were applied. To reduce experimental error, each factor combination was replicated three times.
The three distinct fertilizer and crop residue management (agricultural strategies) for the experimental plots were:
1. Conventional (CON): All fertilizer inputs used for all crops were chemical. The amount of nitrogen, phosphorus, and potassium applied for different crops was: 110, 100, and 100 kg ha−1 for wheat; 200, 100, and 100 kg ha−1, for corn; 75, 50, and 50 kg ha−1 for sesame; and 30, 50, and 50 kg ha−1 for mung bean, respectively. The seeds were sprayed with fungicide (difenoconazole), which is common practice in the region. Weed invasion (the dominant weed was Cynodon dactylon L.) was controlled with Roundup (41% active substance glyphosate) herbicide. Crop residues were completely removed from the soil.
2. Organic (ORG): All inputs were organic and biological. No chemical fertilizer was used. The total amounts of applied compost and vermicompost (Table 1) were 15 and 10 t ha−1 of fresh matter, respectively, for wheat, mung bean, and sesame and 20 and 10 t ha−1 of fresh matter, respectively, for corn. Table 1 presents the detailed chemical properties of the two types of manure used. For all ORG treatment plots, a combination of two-thirds compost and one-third vermicompost was applied. Before planting the crops, the manure was evenly spread on the surface of the experimental plots and then mixed with the soil. Wheat seeds were soaked in biological phosphate fertilizer (Pseudomonas putida and Pantoea agglomerans: 107 bacteria gr−1) 1 h before planting. Weed control was conducted manually by mechanical means. In addition, 30% of crop residues (Table 1) from the preceding year were returned to the soil, which was equivalent to 90, 288, 393, and 129 g m−2 for wheat, mung bean, sesame, and corn, respectively. Humic acid was sprayed at the wheat pollination stage at 5 ml L −1 m−2.
3. Integrated management strategy (INT): A combination of chemical, organic, and biological fertilizers was used. The amounts of compost and vermicompost (the same organic fertilizers that were used in ORG) were 7.5 and 5 t ha−1, respectively, for wheat, mung bean, and sesame, and 10 and 5 t ha−1, respectively, for corn. As in ORG, a combination of two-thirds compost and one-third vermicompost was used for all INT plots. In addition, we applied 50% of the total amount of fertilizers used in CON. Weed was controlled using a combination of chemical (Roundup herbicide with a concentration equal to half of that used in CON) and mechanical methods. Furthermore, only 15% of the crop residues from the preceding year were returned to the soil, which was equivalent to 45, 144, 196.5, and 64.5 g m−2 for wheat, mung bean, sesame, and corn, respectively. Seeds were soaked in biological phosphate fertilizer (3R-BioPhosphate with 35% of P), and humic acid containing 6.5% of nitrogen was sprayed per m−2 at half the amount of that of ORG.
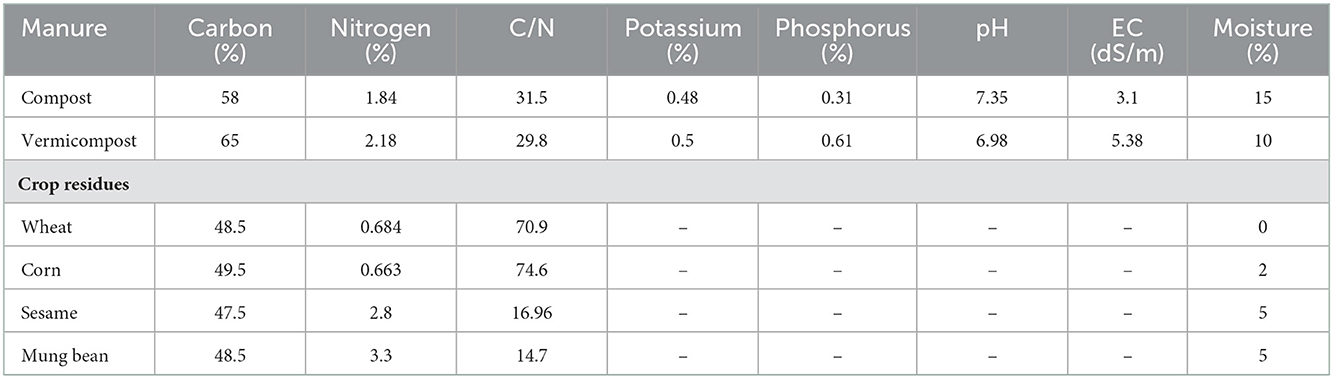
Table 1. Content of carbon, nitrogen, C/N, potassium, phosphorus, pH, EC, and moisture for compost and vermicompost used in the experiment.
The effects of natural fallow, cereal, oil seed, and legume crops as summer crops in rotation with wheat cultivation as winter crop were tested. Accordingly, we designed four crop rotation systems: (1) fallow–wheat (F-W); (2) corn–wheat (C-W); (3) sesame–wheat (S-W); and (4) mung bean–wheat (B-W; Supplementary Figure S1). The experiment began in July 2018 and ended in May 2020 (Supplementary Figure S1). The last crop cultivated on the field before the start of experiment was winter wheat under a combination of chemical and organic fertilizer applications.
The cultivation method for summer crops was furrow planting. After harvesting the summer crops, the furrows were destroyed and the soil in each experimental plot was mixed. The wheat crop was then planted in rows with inter- and intra-row distances of 7 and 2 cm, respectively (Table 2). Table 2 presents detailed information regarding the cultivar, plant density, inter- and intra-row distance, planting and harvest dates of different crops used in the experiment, and the date of soil sampling. Furrows were initially prepared using a moldboard plow. Subsequently, to prevent mixing of the experimental plots, shoveles and rakes were used to prepare furrows for the summer crops and rows for the planting of wheat. Irrigation was applied on the basis of water requirements and soil moisture conditions. The water requirement for each plant was calculated using CROPWAT version 8.0 on the basis of the crop coefficient and evapotranspiration (Steduto et al., 2012). Accordingly, the irrigation requirements for one growth period were 369, 347, 337, and 315 mm for wheat, corn, mung bean, and sesame, respectively.
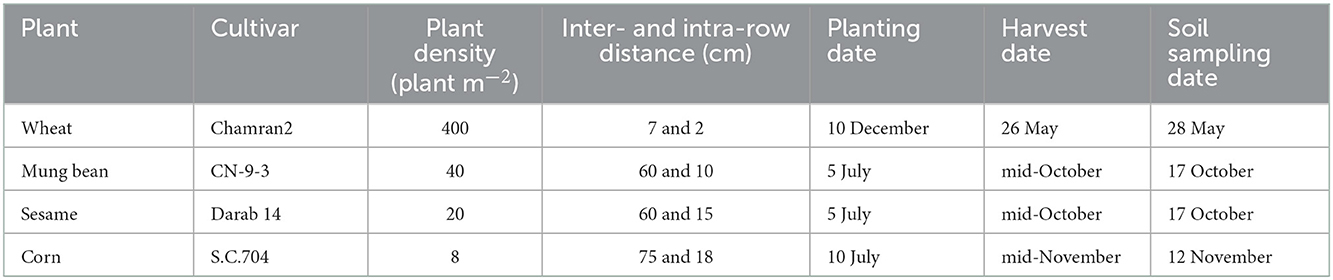
Table 2. Cultivar, plant density, inter- and intra-row distance, planting, harvesting, and soil sampling dates (2018–2020) for different winter and summer crops used in the experiment.
2.3. Soil sampling and analyses
After harvesting each crop, soil samples were obtained from the top 20 cm (Amini and Asoodar, 2015; He et al., 2020) of the soil (Table 2). Soil sampling was performed at three locations of each experimental plot using an auger. The collected samples were then mixed to obtain one sample. To estimate the total SOC and the fractions of labile-C and non-labile-C, the soil samples were first air-dried and then passed through a sieve with a mesh size of 2 mm. After sieving, the substrate exhibited a uniform composition of soil aggregates <2 mm. The amount of SOC was determined using a vitriol acid-potassium dichromate wet oxidation method (Walkley and Black, 1934) and on the basis of the proposed formula by Gelman et al. (2012). For each soil sample, the amount of SOC was measured three times and the average was used as the representative value.
Labile-C was measured using the method suggested by Blair et al. (1995) in which soil samples are exposed to oxidation with potassium permanganate (KMnO4) to oxidize a proportion of total C sensitive to reaction. The absorbance of the filtered samples was read using a spectrophotometer at 550 nm. The labile-C was finally calculated according to Weil et al. (2003) as:
where a, b, Wt, and L are the intercept of the standard curve, slope of the standard curve, weight of air-dried soil sample in kg, and volume of the stock solution reacted, respectively. The initial solution (KMnO4) concentration is 0.02 mol L−1. Abs is absorbance (at 550 nm) of filtered samples and 9,000 = mg of C oxidized by 1 mole of MnO4 changing from Mn7+ to Mn2+. Accordingly, we estimated non-labile-C [%] as the difference between SOC and labile-C (Blair et al., 1995).
The following formula was used to calculate the annual change in SOC sequestration:
where BD is the value of soil bulk density in kg m−3. Sd has intercepted soil sampling depth in meters. Twenty-four months represents the entire experiment period.
2.4. Statistical analyses
The effects of crop rotation systems, agricultural strategies, and their interactions on SOC, labile-C, and non-labile-C changes were tested. The analysis of variance (ANOVA) was done using the mixed method of SAS, version 9.4 (SAS Institute., 2015), based on the mixed split-plot design and considering the random effect of seasonal weather variation (summer and winter). To compare and explain the results, the means of double and triple interactions of the experimental factors were considered. Statistical results of mean comparisons were obtained by Duncan method at a 0.05 significant probability level through SAS, version 9.4 (p) (Onofri, 2007).
3. Results
3.1. Effect of agricultural strategy on the SOC fractions
The results of ANOVA revealed a significant effect of seasonal weather variation, agricultural strategy, and crop rotation system and also the interactions of these factors on SOC, labile-C, and non-labile-C at the level of p ≤ 0.01 and p ≤ 0.05 (Table 3). Our results demonstrate that the value of labile-C was notably decreased in summer both in 2018 and 2019 and increased during winter of 2019 and 2020. In most cases, ORG and INT (compared with CON) had a considerable positive effect on improving the labile-C amount over time with the exception of summer 2019, in which the ORG samples exhibited significantly lower labile-C than those in INT and CON (Figure 2B). The highest value of SOC (5.6 g kg−1) was observed in ORG after winter 2020 (Figure 2B). Unlike for the INT and ORG agricultural strategies, the content of SOC in CON did not change significantly over time (p ≤ 0.05; Figure 2A). The results indicate that non-labile-C remarkably influenced by different agricultural strategies. The non-labile-C content followed the order of ORG > INT > CON, and were based on the change in SOC and labile-C, which were decreased during the winter and increased during summer cultivations (Figure 2C).
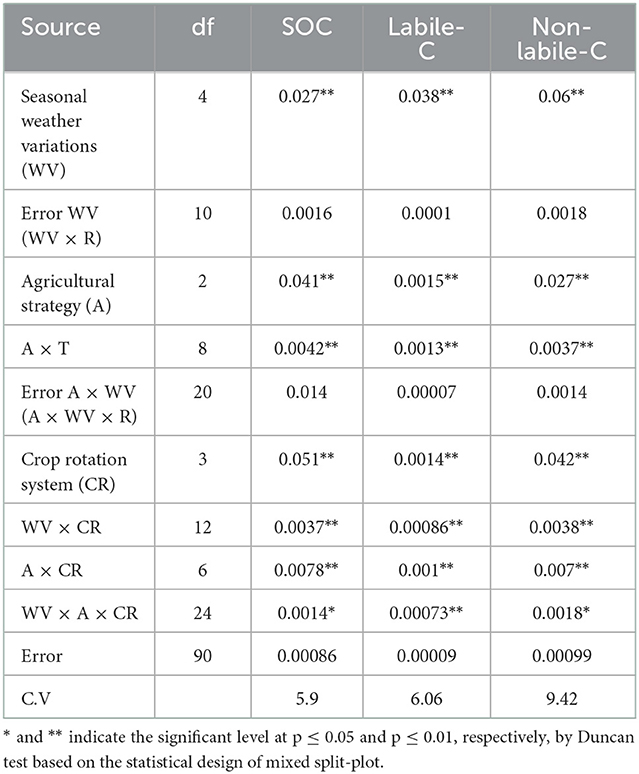
Table 3. Results of analysis of variance (ANOVA) and levels of significance for the effect of seasonal weather variations (WV), agricultural strategy (A), crop rotation system (CR) and their interactions on the SOC, labile-C, and non-labile-C during 2018–2020 at the experimental plots near Ahvaz city in Khuzestan province in Iran.
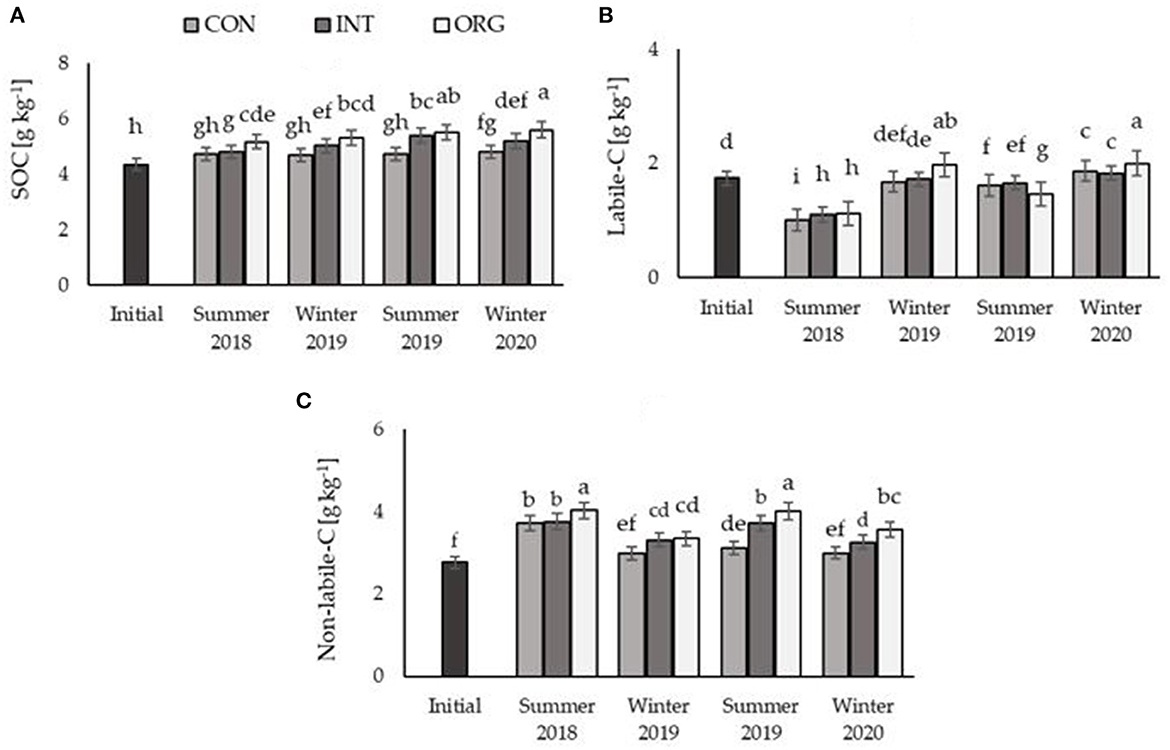
Figure 2. Effects of four seasonal weather variations and three agricultural strategies (conventional, CON; organic, ORG; and integrated management strategy, INT) on (A) soil organic carbon (SOC), (B) labile-C, and (C) non-labile-C content. The initial bar indicates the measured values at the start of the experiment. The x-axis depict the time of sampling after the harvest of the respective crops. Letters indicate significant difference at p ≤ 0.05 according to Duncan's multiple range test. Identical letters indicate no significant difference between the treatments.
3.2. Effect of crop rotation systems on SOC fractions
Our results reveal that over time, the B-W crop rotation system with the value of 5.7 g kg−1 resulted in the highest SOC. In addition, statistically no significant (p ≤ 0.05) differences observed in SOC under F-W over time compared to all crop rotation systems that comprised summer crops in rotation with wheat (fallow-wheat rotation). The variation of SOC dynamics was similar for C-W and S-W crop rotation systems over time (Figure 3A). Regarding labile-C, the four crop rotation systems exhibited substantial differences between summer and winter. The S-W rotation in winter 2019 with 1.9 g kg−1 and the B-W crop rotation in winter 2019 and 2020 with 1.97 and 2 g kg−1, respectively, yielded the highest amounts of labile-C. By contrast, in summer 2018, the amount of labile-C did not exhibit notable differences among the crop rotation systems, whereas it was significantly lower than that of similar crop rotation systems over time (Figure 3B). Similar to SOC and labile-C, the non-labile-C was the highest (significant level of p ≤ 0.01) in the B-W crop rotation system. In contrast to the amount of labile-C, the amount of non-labile-C was low during winter and high during summer. However, the lowest values of non-labile-C were observed for F-W in the winter of both 2019 and 2020 with 2.8 and 2.6 g kg−1, values that were significantly lower than those of other crop rotation systems (Figure 3C).
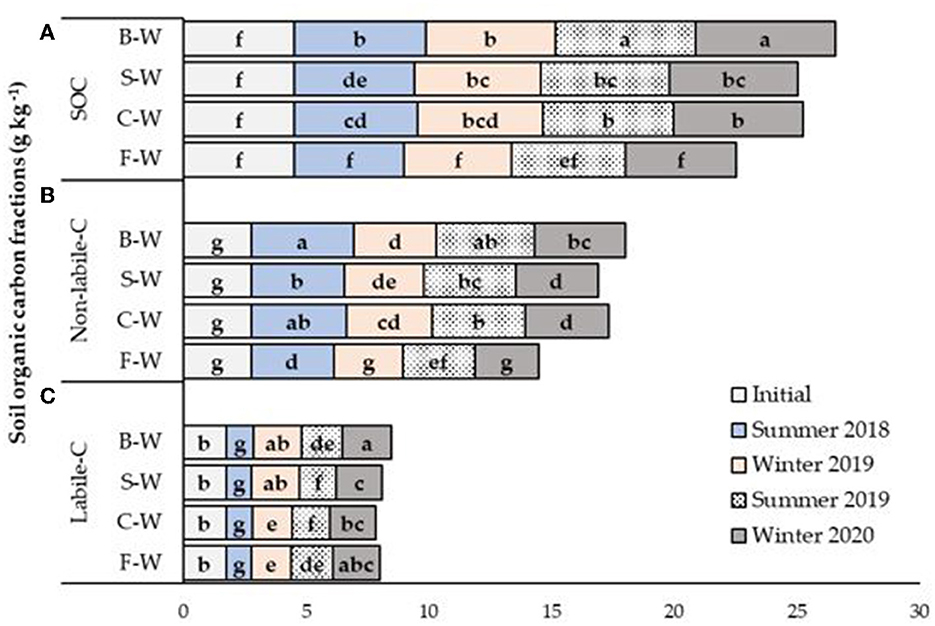
Figure 3. Effects of four seasonal weather variations: summer 2018, 2019 and winter2019, 2020, and four crop rotation systems: F-W, fallow-wheat; C-W, corn-wheat; S-W, sesame-wheat, and B-W, mung bean-wheat on (A) soil organic carbon (SOC), (B) labile-C and (C) non-labile-C content. The initial bar indicates the measured values at the start of the experiment. Letters indicate significant difference at p ≤ 0.05 according to Duncan's multiple range test. Identical letters indicate no significant difference between the treatments.
3.3. Interaction of weather variation, agricultural strategy, and crop rotation system on SOC fractions
A mean comparison of the triple interaction of the experimental treatments revealed an increasing trend in the content of SOC over time (relative to the initial SOC) in ORG and INT under all crop rotation systems except F-W (Figures 4A,D). In CON under all crop rotation systems, no significant differences in terms of SOC content compared to the initial value (p ≤ 0.05) were observed, except for the B-W crop rotation system, in which a 0.057% increase in SOC was observed after the summer 2019 and winter 2020 (Figure 4D). For the F-W crop rotation system (among all other agricultural strategies) no significant difference was observed, except for INT in summer 2019, in which the amount of SOC (5 g kg−1) was significantly higher than in the other agricultural treatments. In addition, the highest amount of SOC was observed in ORG (6.6, 5.7, and 5.7 g kg−1 for B-W, C-W, and S-W, respectively), followed by INT (5.5, 5.5, and 5.3 g kg−1, for B-W, C-W, and S-W, respectively), and CON (5.1, 4.7, and 5 g kg−1 for B-W, C-W, and S-W, respectively; Figures 4B–D). Overall, the highest and lowest SOC values were observed for B-W (6.6 g kg−1) and F-W (4.2 g kg−1), respectively (Figures 4A,D).
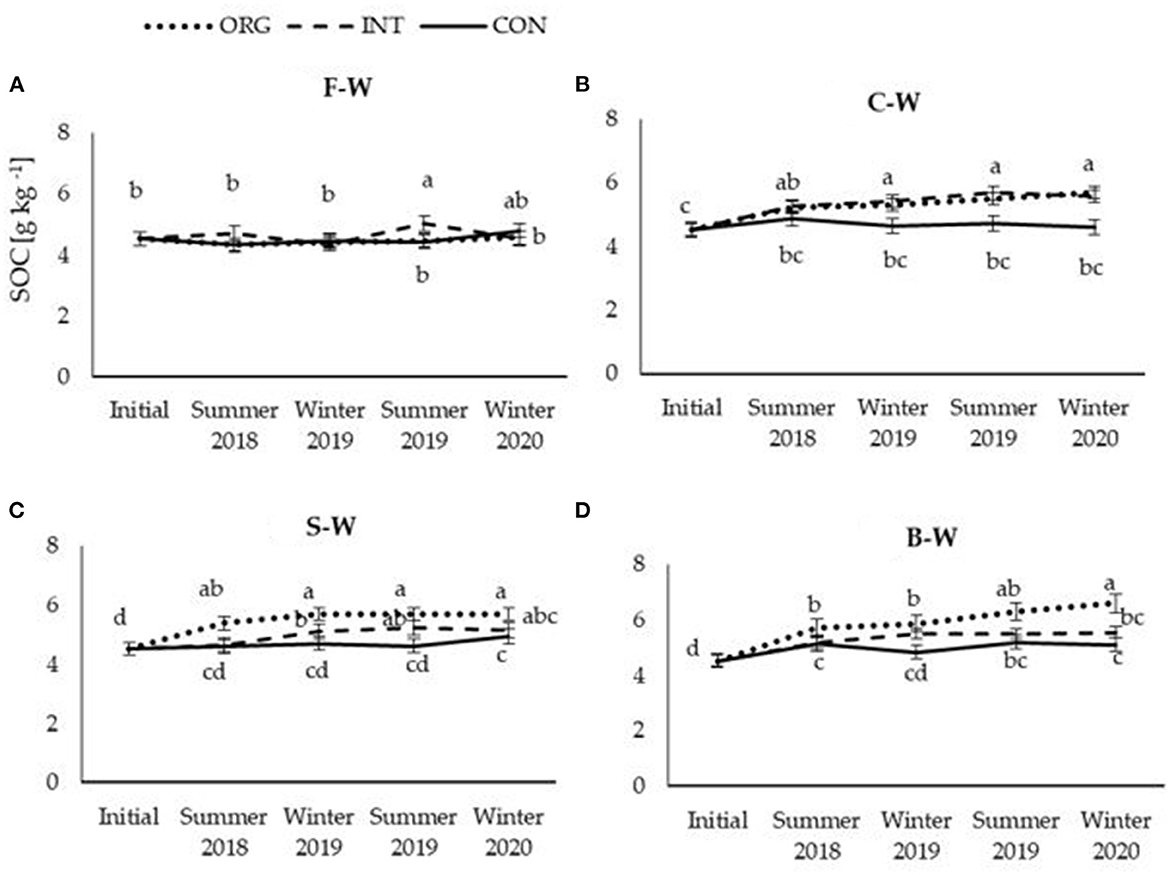
Figure 4. Interaction effects of four seasonal weather variations, three agricultural strategies (conventional, CON; organic, ORG; and integrated management strategy, INT), and four crop rotation systems: (A) F-W, fallow-wheat; (B) C-W, corn-wheat; (C) S-W, sesame-wheat, and (D) B-W, mung bean-wheat, on SOC change. The initial point indicates the measured values at the start of the experiment. The years depict the harvest time for winter and summer crops. Letters indicate a significant (different letters) and no significant (Identical letters) difference at p ≤ 0.05 according to Duncan's multiple range test.
The annual changes of SOC sequestration revealed that the F-W crop rotation system in ORG exhibited the lowest SOC content with a value of 0.05 t C ha−1 year−1 (Table 4). However, C-W in INT exhibited a relative increase of 0.15% and was as effective as the B-W crop rotation in annual SOC sequestration in the soil with a value of 1.49 t C ha−1 year−1 (Table 4).
For both years and all agricultural strategies and crop rotation systems, a non-significant increase in the amount of labile-C was observed (relative to the baseline value), especially after harvesting winter wheat (Supplementary Figures S2A,D). However, in most cases, the amount of labile-C declined after the summer harvest. Although no significant difference was observed between the winters of 2019 and 2020 in terms of labile-C (p ≤ 0.05), the values measured in the second year indicate an increase over time. Similar to SOC, the labile-C content exhibited the highest value (2.3 g kg−1) in ORG under the B-W crop rotation system (Supplementary Figure S2D), whereas the lowest value was observed in summer 2018 in the S-W and C-W crop rotation systems in CON and ORG (0.9 g kg−1; Supplementary Figures S2B,C). For most of the crop rotation systems, a higher amounts of non-labile-C were observed in ORG and INT. By contrast, in the S-W crop rotation system in INT, non-labile-C decreased over time compared to that in CON. Similar as in SOC and labile-C, the highest value of non-labile-C (4.5 g kg−1) was observed in ORG in the B-W crop rotation system (Supplementary Figure S3D). Overall, our analysis of the experimental treatments demonstrates that the amount of non-labile-C increased over time (Supplementary Figures S3A,D), except in the F-W rotation system under INT, in which the lowest amount of non-labile-C (2.2 g kg−1) was observed (Supplementary Figure S3A).
4. Discussion
4.1. Impact of weather variation on the SOC fractions
The weather condition in Khuzestan provided us this opportunity to monitor the change in soil labile-C under two different growing seasons in 1 year. Our results revealed that the amounts of labile-C under all crop rotation systems and agricultural strategies were reduced during high temperatures in summer cultivation (Supplementary Figure S2). Similar findings were reported by Lal (2015) and Naresh et al. (2018). The negative correlation between labile-C and high temperatures may strongly linked with the decomposition rate of organic matter and the increase in soil respiration (Fang et al., 2005; Luo et al., 2017). In summer, high temperature led to increased water evaporation from the irrigated soil under crop cultivation. Therefore, in low soil moisture the activity of microorganisms and organic matter decomposition decreased (Van Veen and Kuikman, 1990; Sierra et al., 2015). Remoistening the soil through crop irrigation has probably resulted in opportunistic using of organic matter by soil microorganisms and acceleration of organic matter decomposition. Consequently, the content of labile-C reduced due to its release from the soil in the form of CO2. A study conduced by Thakur et al. (2018) delineated that high temperature (more than 43°C) and low precipitation led to depletion of SOC pools due to increased soil respiration. This situation was more apparent under ORG in summer 2019 in which a high level of decomposing crop residues, compost and vermicompost accumulated over time. In contrast with summer, temperate weather and distribution of precipitation during growing winter wheat provided the conditions required for sequestering C released by the decomposition of organic matter (Carvalhais et al., 2014).
4.2. Impact of agricultural strategy on the SOC fractions
Our findings revealed that the different agricultural strategies in combination with crop rotation systems played a crucial role in building up SOC, dynamics of C fractions, and significantly contributed to C-sequestration in the soil. The effects clearly differed among agricultural strategies and crop rotation systems. In all agricultural strategies and crop rotation systems, a positive relationship between SOC and labile-C was observed. Improving SOC in the soil surface (0–30 cm) in ORG and INT illustrated that these strategies are potentially effective approaches for sequestering C in soil in such a semi-arid agricultural region. Increasing the presence and decomposition rate of organic matter over time through adding bio and organic (compost/vermicompost) fertilizers as well as returning 30% of crop residues in the soil in ORG and applying half doses of them in INT could be the main reasons for this improvement. According to Abdelhafez et al. (2018), organic matter derived from manure had stable organic compounds during decomposition and remained safe from respiration. Therefore, they significantly improved C sequestration and boosted C pools in farmlands in the semi-arid regions. The application of inorganic fertilizer in combination with organic inputs in INT improved root and shoot biomass and enhanced SOC and its fractions through higher return of above and below ground residues in the soil (Cambardella and Elliott, 1992; Six et al., 2002; Bhattacharyya et al., 2012). CON also exhibited a positive effect over time through producing abundant plant biomass in shoots and root organs during plant growth and retaining root and additional plant residues in the soil. This is consistent with the results of Rudrappa et al. (2006) and Liu et al. (2013). The differences in labile-C among various agricultural strategies can be associated with various factors. One of these might be the close link of labile-C with the amount and types of crop residue returned to the soil. In fact, the decomposition rate of crop residues (root and shoot) is one of the key factor explaining differences among labile-C values. For example leguminous residues with low C/N ratios aid in alleviating nitrogen limitations and are a substrate of accessible C for an extend range of microbial taxa leading to increased rates of labile-C fraction (Fortuna et al., 2003; Culman et al., 2013).
4.3. Impact of weather variation and crop rotation system on SOC fractions
Continuous crop rotation systems always resulted in high SOC content compared with F-W. This confirms the positive role of crops for C-sequestration. Maintenance and accumulation of crop roots and their incompletely decomposed residues near the soil surface is one of the most beneficial effects of wheat crop rotation systems with summer crops on C sequestration in agricultural lands (Bremer and Ellert, 2008). Differences in SOC and labile-C changes between crop rotation systems can be owing to the different quantity and quality of returned crop residues. For example, high levels of root biomass production in the cereal-dominated rotation systems (here corn-wheat crop rotation system) with the above content of less-decomposable cellulose in their residues and high C/N ratio may contribute to a remarkable quantity of SOC over time (Ghosh et al., 2016; Islam et al., 2022). In addition, improvement of SOC and labile-C through the inclusion of legumes (B-W; in this study mung bean) in the rotation with wheat might be attributable to an increase in the rate of residue decomposition (i.e., the C/N ratios of involved crops). Similar findings have been reported in the literature (Drury and Tan, 1995; Gregorich et al., 2001; Parihar et al., 2018). Legumes also exert a positive effect on the rhizosphere through their root traits, which can improve the process of C-sequestration and also the turnover of labile-C in the topsoil (Fu and Cheng, 2002; Wu et al., 2017). Yet, because of the limited growth period of legumes, the interaction of plant roots with the soil leading to decreased soil hardness, amelioration of root extension, and improvement of soil bioactivity in the various crop rotation systems, returning their residues to the soil might be more effective in promoting SOC and labile-C than merely their growth (Whitbread et al., 2000; Blanco-Moure et al., 2016; Bongiorno et al., 2019).
4.4. Interaction effect of weather variation, agricultural strategy, and crop rotation system on SOC fractions
The C-W crop rotation system in combination with organic and inorganic fertilizers was as effective as B-W on the annual SOC sequestration in the soil. As was reported by Gong et al. (2009) under C-W cultivation, the incorporation of inorganic fertilizer with manure through an increase in crop root biomass and crop stubble exerted a considerable positive influence on SOC improvement. In general, low SOC observed in F-W could be related to both agricultural management strategy and crop rotation system treatments. Bare soil in the summer (high temperature), as well as reduced entry of root and shoot crop residues (Maia et al., 2007) in combination with organic matter (ORG and INT) into the soil could be two important reasons that caused a slight change in C-sequestration during the experimental times in F-W under all agricultural strategies. The negative effect of high temperature was also apparent in the relatively lower improvement of SOC in F-W for ORG. High temperature and summer fallow caused probably an increased C emission from the soil due to the increased decomposition rate of organic matter (Pei et al., 2017). It seems that temperature sensitivity to SOC decomposition was decreased by placing the summer crops in different rotation systems.
Furthermore, the capacity of plants for C-sequestration under distinct agricultural strategies depends on the growth and expansion of crop canopy (Aquino et al., 2017), which affects the relative change of non-labile-C to labile-C. In C-W, for example, we observed lower values of labile-C of summer corn in ORG compared with that in CON or INT, which might be due to the low initial growth of corn in this treatment as well as low decomposition of its residues due to high level of C/N ratio. This resulted in a high portion of non-labile-C in the soil. Finally, the duration for which plants were present on the farm is crucial and must be considered. A shorter growing season in summer compared to that in winter could be a reason for the variation of SOC and its fractions (Diekow et al., 2005; Majumder et al., 2008).
5. Conclusion
Our results demonstrated that although, the agricultural strategies based on crop residue management and organic fertilizer were more effective for C-sequestration, at high temperature and zero precipitation, accumulation of organic matter over time similar to ORG condition led to a greater reduction in labile-C pools. The comparison of various oil seeds, legumes, and cereal crops in rotation with wheat revealed a positive effect of B-W followed by C-W and S-W on SOC and C-fractions and exhibited superior performance compared with leaving the plots fallow. To have more details of the positive role of crops in C-sequestration, it is also important to consider the amount of C derived from roots and shoots (Huang et al., 2021). Our experiment did not specifically tackle this point (this was beyond the scope experiment). We recommend that additional researches should investigate the effect of temperature and different levels of nutrient management on soil C dynamics in the semi-arid regions. It can help to find an effective boundary between agricultural strategies and high temperature on C pools retention. More detailed results can be generated by conducting longer experiments and using agroecosystem modeling that involves climate-change scenarios at broader spatial scales. Increasing the time span of experiments would aid in exploring the role of climatic changes on the effectiveness of various treatments.
Data availability statement
The original contributions presented in the study are included in the article/Supplementary material, further inquiries can be directed to the corresponding author.
Author contributions
AG: writing, conceptualization, methodology, data collecting, and data processing. BK and TG: writing, review and editing, and data processing. AA and AR: methodology and data collecting. All authors listed contributed to the article and approved it for submission.
Funding
The study was supported by the Shahid Chamran University of Ahvaz and the University of Bonn. The authors are also grateful to Ms. Sara Rahdarian for supporting the projects regarding lab analysis.
Conflict of interest
The authors declare that the research was conducted in the absence of any commercial or financial relationships that could be construed as a potential conflict of interest.
Publisher's note
All claims expressed in this article are solely those of the authors and do not necessarily represent those of their affiliated organizations, or those of the publisher, the editors and the reviewers. Any product that may be evaluated in this article, or claim that may be made by its manufacturer, is not guaranteed or endorsed by the publisher.
Supplementary material
The Supplementary Material for this article can be found online at: https://www.frontiersin.org/articles/10.3389/fsufs.2022.1016000/full#supplementary-material
Abbreviations
CON, conventional agricultural strategy; ORG, organic agricultural strategy; INT, integrated agricultural strategy; C, carbon; SOC, soil organic carbon, F-W, rotation of fallow-wheat; C-W, rotation of corn-wheat; S-W, rotation of sesame-wheat; B-W, rotation of mung bean-wheat.
References
Abdelhafez, A. A., Abbas, M. H. H., Attia, T. M. S., El Bably, W., and Mahrous, S. E. (2018). Mineralization of organic carbon and nitrogen in semi-arid soils under organic and inorganic fertilization. Environ. Technol. Innov. 9, 243–253. doi: 10.1016/j.eti.2017.12.011
Afshar, F. A., Ayoubi, S., and Jalalian, A. (2010). Soil redistribution rate and its relationship with soil organic carbon and total nitrogen using 137Cs technique in a cultivated complex hillslope in western Iran. J. Environ. Radioact. 101, 606–614. doi: 10.1016/j.jenvrad.2010.03.008
Amini, S., and Asoodar, M. A. (2015). Investigation the effect of conservation tillage on soil organic matter (SOM) and soil organic carbon (SOC) (the review). N. Y. Sci. J. 8, 16–24. doi: 10.7537/marsnys080315.03
Aquino, A. L., Sta Cruz, P. C., Zamora, O. B., Aguilar, E. A., and Lasco, R. D. (2017). Carbon sequestration in organic and conventional corn production system. Philipp. J. Crop. Sci. 42, 11–18.
Ayoubi, S., Mokhtari Karchegani, P., Mosaddeghi, M. R., and Honarjoo, N. (2012). Soil aggregation and organic carbon as affected by topography and land use change in western Iran. Soil Tillage Res. 121, 18–26. doi: 10.1016/j.still.2012.01.011
Baillie, I. C. (2006). Soil survey staff 1999, soil taxonomy. Soil Use Manag. 17, 57–60. doi: 10.1111/j.1475-2743.2001.tb00008.x
Batjes, N. H. (1996). Total carbon and nitrogen in the soils of the world. Euro. J. Soil Sci. 47, 151–163. doi: 10.1111/j.1365-2389.1996.tb01386.x
Benbi, D. K., Brar, K., Toor, A. S., and Sharma, S. (2015). Sensitivity of labile soil organic carbon pools to long-term fertilizer, straw and manure management in rice-wheat system. Pedosphere 25, 534–545. doi: 10.1016/S1002-0160(15)30034-5
Bhattacharyya, P., Roy, K. S., Neogi, S., Adhya, T. K., Rao, K. S., Manna, M. C., et al. (2012). Effects of rice straw and nitrogen fertilization on greenhouse gas emissions and carbon storage in tropical flooded soil planted with rice. Soil Tillage Res. 124, 119–130. doi: 10.1016/j.still.2012.05.015
Blair, G. J., Lefroy, R. D., and Lisle, L. (1995). Soil carbon fractions based on their degree of oxidation, and the development of a carbon management index for agricultural systems. Aust. J. Agric. Res. 46, 1459–1466. doi: 10.1071/AR9951459
Blanco-Moure, N., Gracia, R., Bielsa, A. C., and López, M. V. (2016). Soil organic matter fractions as affected by tillage and soil texture under semiarid Mediterranean conditions. Soil Tillage Res. 155, 381–389. doi: 10.1016/j.still.2015.08.011
Bongiorno, G., Bünemann, E. K., Oguejiofor, C. U., Meier, J., Gort, G., Comans, R. R., et al. (2019). Sensitivity of labile carbon fractions to tillage and organic matter management and their potential as comprehensive soil quality indicators across pedoclimatic conditions in Europe. Ecol. Indic. 99, 38–50. doi: 10.1016/j.ecolind.2018.12.008
Brandão, M., Levasseur, A., Kirschbaum, M. U. F., Weidema, B. P., Cowie, A. L., Jørgensen, S. V., et al. (2013). Key issues and options in accounting for carbon sequestration and temporary storage in life cycle assessment and carbon footprinting. Int. J. Life Cycle Assess. 8, 230–240. doi: 10.1007/s11367-012-0451-6
Bremer, E., and Ellert, B. H. (2008). Soil organic carbon after twelve years of various crop rotations in an aridic boroll. Soil Sci. Soc. Am. J. 72, 4–8. doi: 10.2136/sssaj2007.0327
Calegari, A., Hargrove, W. L., Dos, D., Rheinheimer, S., Ralisch, R., Tessier, D., et al. (2008). Impact of long-term no-tillage and cropping system management on soil organic carbon in an oxisol: a model for sustainability. J. Agron. 1013–1019. doi: 10.2134/agronj2007.0121
Cambardella, C. A., and Elliott, E. T. (1992). Particulate soil organic-matter changes across a grassland cultivation sequence. Soil Sci. Soc. Am. J. 56, 777–783. doi: 10.2136/sssaj1992.03615995005600030017x
Carvalhais, N., Forkel, M., Khomik, M., Bellarby, J., Jung, M., Migliavacca, M., et al. (2014). Global covariation of carbon turnover times with climate in terrestrial ecosystems. Nature 514, 213–217. doi: 10.1038/nature13731
Chalise, K. S., Singh, S., Wegner, B. R., Kumar, S., Pérez-gutiérrez, J. D., Osborne, S. L., Nleya, T., Guzman, J., and Rohila, J. S. (2018). Cover crops and returning residue impact on soil organic carbon, bulk density, penetration resistance, water retention, infiltration, and soybean yield. Agron. J. 111, 99–108. doi: 10.2134/agronj2018.03.0213
Chan, K. Y., Bowman, A., and Oates, A. (2001). Oxidizible organic carbon fractions and soil quality changes in an oxic paleustalf under different pasture leys. Soil Sci. 166, 61–6. doi: 10.1097/00010694-200101000-00009
Chauhan, B. S., Mahajan, G., Sardana, V., Timsina, J., and Jat, M. L. (2012). Productivity and sustainability of the rice–wheat cropping system in the Indo-Gangetic Plains of the Indian subcontinent: problems, opportunities, and strategies. Adv. Agron. 117, 315–369. doi: 10.1016/B978-0-12-394278-4.00006-4
Chen, Z., Wang, Q., Wang, H., Bao, L., and Zhou, J. (2018). Crop yields and soil organic carbon fractions as influenced by straw incorporation in a rice–wheat cropping system in southeastern China. Nutr. Cycling Agroecosyst. 112, 61–73. doi: 10.1007/s10705-018-9929-3
CO2 Country Profile. (2021). Avalable online at: https://link.springer.com/ article/10.1007/s10705-018-9929-3 (accessed November 30, 2022).
Cooper, J. M., Burton, D., Daniell, T. J., Griffiths, B. S., and Zebarth, B. J. (2011). Carbon mineralization kinetics and soil biological characteristics as influenced by manure addition in soil incubated at a range of temperatures. Eur. J. Soil Biol. 47, 392–399. doi: 10.1016/j.ejsobi.2011.07.010
Culman, S. W., Snapp, S. S., Green, J. M., and Gentry, L. E. (2013). Short- and long-term labile soil carbon and nitrogen dynamics reflect management and predict corn agronomic performance. Agron. J. 105, 493–502. doi: 10.2134/agronj2012.0382
Dandwate, S. C. (2020). Analysis of soil samples for its physicochemical parameters from Sangamner city. GSC Biol. Pharm. Sci. 12, 123–128. doi: 10.30574/gscbps.2020.12.2.0243
Diekow, J., Mielniczuk, J., Knicker, H., Bayer, C., Dick, D. P., Kögel-Knabner, I., et al. (2005). Carbon and nitrogen stocks in physical fractions of a subtropical acrisol as influenced by long-term no-till cropping systems and N fertilisation. Plant Soil 268, 319–328. doi: 10.1007/s11104-004-0330-4
Ding, X., Han, X., Liang, Y., Qiao, Y., Li, L., Li, N., et al. (2012). Changes in soil organic carbon pools after 10 years of continuous manuring combined with chemical fertilizer in a Mollisol in China. Soil Tillage Res. 122, 36–41. doi: 10.1016/j.still.2012.02.002
Drury, C., and Tan, C. S. (1995). Long-term (35 years) effects of fertilization, rotation and weather on corn yields. Can. J. Plant Sci. 75, 355–362. doi: 10.4141/cjps95-060
Fang, C., Smith, P., Moncrieff, J. B., and Smith, J. U. (2005). Similar response of labile and resistant soil organic matter pools to changes in temperature. Nature 433, 57–59. doi: 10.1038/nature03138
FAO. (2002). World Agriculture: Towards 2015/2030, Vol. 20. Rome: FAO.doi: 10.1016/S0264-8377(03)00047-4
FAO. (2021). Soil Texture. FAO.Available online at: https://www.fao.org/fishery/docs/CDrom/FAO_Training/FAO_Training/General/x6706e/x6706e06.htm
Fortuna, A., Harwood, R., Kizilkaya, K., and Paul, E. A. (2003). Optimizing nutrient availability and potential carbon sequestration in an agroecosystem. Soil Biol. Biochem. 35, 1005–1013. doi: 10.1016/S0038-0717(03)00084-1
Fu, S., and Cheng, W. (2002). Rhizosphere priming effects on the decomposition of soil organic matter in C 4 and C 3 grassland soils. Plant Soil 1, 289–294. doi: 10.1023/A:1014488128054
Gelman, F., Binstock, R., and Halicz, L. (2012). Application of the Walkley-Black titration for the organic carbon quantification in organic rich sedimentary rocks. Fuel 96, 608–610. doi: 10.1016/j.fuel.2011.12.053
Ghosh, B. N., Meena, V. S., Alam, N. M., Dogra, P., Bhattacharyya, R., Sharma, N. K., et al. (2016). Impact of conservation practices on soil aggregation and the carbon management index after seven years of maize-wheat cropping system in the Indian Himalayas. Agric. Ecosyst. Environ. 216, 247–257. doi: 10.1016/j.agee.2015.09.038
Gong, W., Yan, X., and Wang, J. (2009). Long-term manuring and fertilization effects on soil organic carbon pools under a wheat – maize cropping system in North China Plain. Plant Soil 67–76. doi: 10.1007/s11104-008-9705-2
Gregorich, E. G., Drury, C. F., and Baldock, J. A. (2001). Changes in soil carbon under long-term maize in monoculture and legume-based rotation. Can. J. Soil Sci. 81, 21–31. doi: 10.4141/S00-041
Hajabbasi, M. A., and Hemmat, A. (2000). Tillage impacts on aggregate stability and crop productivity in a clay-loam soil in central Iran. Soil Tillage Res. 56, 205–212. doi: 10.1016/S0167-1987(00)00140-9
Havlin, J. L. (2020). “Soil: Fertility and nutrient management”, In Landscape and Land Capacity (CRC Press), 251–265. doi: 10.1201/9780429445552
He, G., Zhang, Z., Zhang, J., and Huang, X. (2020). Soil organic carbon dynamics and driving factors in typical cultivated land on the Karst Plateau. Int. J. Environ. Res. Public Health 17, 1–17. doi: 10.3390/ijerph17165697
Hoshmand, R. (2018). Design of Experiments for Agriculture and the Natural Sciences (Chapman and Hall/CRC), 40–46. doi: 10.1201/9781315276021
Huang, J., Weixing, L., Sen, Y., Lu, Y., Ziyang, P., Meifeng, D., Shan, X., Beibei, Z., Jitendra, A., and Lingli, L. (2021). Plant carbon inputs through shoot, root, and mycorrhizal pathways affect soil organic carbon turnover differently. Soil Biol. Biochem. 160, 108322. doi: 10.1016/j.soilbio.2021.108322
Iran Wheat Flour Exported to Oman Italy, Iraq. (2021). Available online at: https://www.en.eghtesadonline.com/Section-economy-4/23410-iran-wheat-flour-exported-to-oman-italy-iraq (accessed March 31, 2021).
IRIMO (2020). 2017–2020 Statistical Yearbook of Meteorology. Iran: Islamic Republic of Iran Meteorological Organization.
Islam, M. A., Bell, R. W., Johansen, C., Jahiruddin, M., Haque, M. E., Vance, W., et al. (2022). Conservation agriculture practice influences soil organic carbon pools in intensive rice-based systems of the Eastern Indo-Gangetic Plain. Soil Use Manag. 38, 1217–1236. doi: 10.1111/sum.12798
Kabiri, V., Raiesi, F., and Ghazavi, M. A. (2015). Six years of different tillage systems affected aggregate-associated SOM in a semi-arid loam soil from Central Iran. Soil Tillage Res. 154, 114–125. doi: 10.1016/j.still.2015.06.019
Kamali, F., Talebian, M., Khosravi, M., Vahidi, A., and Roayaei, E. (2009). Recovery based ranking oil reservoir for CO2 miscible injection. In Shiraz 2009-1st EAGE International Petroleum Conference and Exhibition. p. cp-125. doi: 10.3997/2214-4609.20145854
Khormali, F., Ajami, M., Ayoubi, S., Srinivasarao, C., and Wani, S. P. (2009). Role of deforestation and hillslope position on soil quality attributes of loess-derived soils in Golestan province, Iran. Agric. Ecosyst. Environ. 134, 178–189. doi: 10.1016/j.agee.2009.06.017
Laird, D. A. (2008). The charcoal vision: a win–win–win scenario for simultaneously producing bioenergy, permanently sequestering carbon, while improving soil and water quality. Agron. J. 100, 178–181. doi: 10.2134/agrojnl2007.0161
Lal, R. (2010). Beyond Copenhagen: mitigating climate change and achieving food security through soil carbon sequestration. Food Secur. 2, 169–177. doi: 10.1007/s12571-010-0060-9
Lal, R. (2015). Sequestering carbon and increasing productivity by conservation agriculture. J. Soil Water Conserv. 70, 55A−62A. doi: 10.2489/jswc.70.3.55A
Lal, R. (2018). Digging deeper: a holistic perspective of factors affecting soil organic carbon sequestration in agroecosystems. Glob. Chang. Biol. 24, 3285–3301. doi: 10.1111/gcb.14054
Li, J., Wen, Y., Li, X., Li, Y., Yang, X., Lin, Z., et al. (2018). Soil labile organic carbon fractions and soil organic carbon stocks as affected by long-term organic and mineral fertilization regimes in the North China Plain. Soil Tillage Res. 175, 281–290. doi: 10.1016/j.still.2017.08.008
Li, Y., Jiao, J., Wang, Z., Cao, B., Wei, Y., Hu, S., et al. (2016). Effects of revegetation on soil organic carbon storage and erosion-induced carbon loss under extreme rainstorms in the hill and gully region of the loess plateau. Int. J. Environ. Res. Public Health, 13, p.456. doi: 10.3390/ijerph13050456
Liu, E., Teclemariam, S. G., Yan, C., Yu, J., Gu, R., Liu, S., et al. (2014). Long-term effects of no-tillage management practice on soil organic carbon and its fractions in the northern China. Geoderma 213, 379–384. doi: 10.1016/j.geoderma.2013.08.021
Liu, E., Yan, C., Mei, X., Zhang, Y., and Fan, T. (2013). Long-term effect of manure and fertilizer on soil organic carbon pools in dryland farming in Northwest China. PLoS ONE, 8, e56536. doi: 10.1371/journal.pone.0056536
Liu, K., Bandara, M., Hamel, C., Knight, J. D., and Gan, Y. (2020). Intensifying crop rotations with pulse crops enhances system productivity and soil organic carbon in semi-arid environments. Field Crops Res. 248, 107657. doi: 10.1016/j.fcr.2019.107657
Luo, Y., Li, Q., Shen, J., Wang, C., Li, B., Yuan, S., et al. (2019). Effects of agricultural land use change on organic carbon and its labile fractions in the soil profile in an urban agricultural area. Land Degradation. Dev. 30, 1875–1885. doi: 10.1002/ldr.3388
Luo, Z., Feng, W., Luo, Y., Baldock, J., and Wang, E. (2017). Soil organic carbon dynamics jointly controlled by climate, carbon inputs, soil properties and soil carbon fractions. Global Change Biol. 23, 4430–4439. doi: 10.1111/gcb.13767
Maia, S. M. F., Gonzaga, G. B. M., Silva, L. K., dos, S., Lyra, G. B., Gomes, T. C., and de, A. (2019). Soil organic carbon temperature sensitivity of different soil types and land use systems in the Brazilian semi-arid region. Soil Use Manag. 35, 433–442. doi: 10.1111/sum.12508
Maia, S. M. F., Xavier, F. A. S., Oliveira, T. S., Mendonça, E. S., and Araújo Filho, J. A. (2007). Organic carbon pools in a luvisol under agroforestry and conventional farming systems in the semi-arid region of Ceará, Brazil. Agrofor. Syst. 71, 127–138. doi: 10.1007/s10457-007-9063-8
Majumder, B., Mandal, B., and Bandyopadhyay, P. K. (2008). Soil organic carbon pools and productivity in relation to nutrient management in a 20-year-old rice-berseem agroecosystem. Biol. Fertil. Soils, 44, 451–461. doi: 10.1007/s00374-007-0226-6
Maltas, A., Kebli, H., Oberholzer, H. R., Weisskopf, P., and Sinaj, S. (2018). The effects of organic and mineral fertilizers on carbon sequestration, soil properties, and crop yields from a long-term field experiment under a Swiss conventional farming system. Land Degrad. Dev. 29, 926–938. doi: 10.1002/ldr.2913
Naresh, R. K., Gupta, R. K., and Panwar, A. S. (2018). Spatial and temporal variations of soil organic carbon fractions and soil carbon storage in rice- wheat cropping system under varying tillage, straw and fertilizer management : a review. Farm. Syst. Res. 7, 1667–1690.
Onofri, A. (2007). “Routine statistical analyses of field experiments by using an Excel extension,” in Proceedings 6th National Conference Italian Biometric Society: “La statistica nelle scienze della vita e dell'ambiente.” Pisa, 93–96.
Parihar, C. M., Jat, S. L., Singh, A. K., Datta, A., Parihar, M. D., Varghese, E., et al. (2018). Changes in carbon pools and biological activities of a sandy loam soil under medium-term conservation agriculture and diversified cropping systems. Eur. J. Soil Sci. 69, 902–912. doi: 10.1111/ejss.12680
Pei, J., Zhuang, S., Cui, J., Li, J., Li, B., Wu, J., et al. (2017). Biochar decreased the temperature sensitivity of soil carbon decomposition in a paddy field. Agric. Ecosyst. Environ. 249, 156–164. doi: 10.1016/j.agee.2017.08.029
Plaza-Bonilla, D., Álvaro-Fuentes, J., and Cantero-Martínez, C. (2014). Identifying soil organic carbon fractions sensitive to agricultural management practices. Soil Tillage Res. 139, 19–22. doi: 10.1016/j.still.2014.01.006
Plaza-Bonilla, D., Nolot, J. M., Passot, S., Raffaillac, D., and Justes, E. (2016). Grain legume-based rotations managed under conventional tillage need cover crops to mitigate soil organic matter losses. Soil Tillage Res. 156, 33–43. doi: 10.1016/j.still.2015.09.021
Radicetti, E., Campiglia, E., Langeroodi, A. S., Zsembeli, J., Mendler-Drienyovszki, N., Mancinelli, R., et al. (2020). Soil carbon dioxide emissions in eggplants based on cover crop residue management. Nutr. Cycling Agroecosyst. 118, 39–55. doi: 10.1007/s10705-020-10081-x
Rahimi, M. R., Ayoubi, S., and Abdi, M. R. (2013). Magnetic susceptibility and Cs-137 inventory variability as influenced by land use change and slope positions in a hilly, semiarid region of west-central Iran. J. Appl. Geophys. 89, 68–75. doi: 10.1016/j.jappgeo.2012.11.009
Rudrappa, L., Purakayastha, T. J., Singh, D., and Bhadraray, S. (2006). Long-term manuring and fertilization effects on soil organic carbon pools in a Typic Haplustept of semi-arid sub-tropical India. Soil Tillage Res. 88, 180–192. doi: 10.1016/j.still.2005.05.008
Samal, S. K., Rao, K. K., Poonia, S. P., Kumar, R., Mishra, J. S., Prakash, V., et al. (2017). Evaluation of long-term conservation agriculture and crop intensification in rice-wheat rotation of Indo-Gangetic Plains of South Asia: carbon dynamics and productivity. Eur. J. Agron. 90, 198–208. doi: 10.1016/j.eja.2017.08.006
SAS Institute. (2015). Base SAS 9, 4. Procedures Guide. Available online at: https://books.google.com/books?hl=nlandlr=andid=rChZCwAAQBAJandoi= fndandpg=PR3anddq=SAS+Institute.+Base+SAS+9.4+procedures+guide.+SAS+I nstitute,+2015.andots=ITyeRRSM1handsig=SQS3HAktsSK1XCuyCepevUPvTRo (accessed December 15, 2022).
Self-Davis, M. L., Moore, P. A. Jr., and Joern, B. C. (2000). Determination of water-and/or dilute salt-extractable phosphorus. Methods of phosphorus analysis for soils, sediments, residuals, and waters. Southern Coop. Ser. Bull, 396, 24–26.
Sierra, C. A., Trumbore, S. E., Davidson, E. A., Vicca, S., and Janssens, I. (2015). Sensitivity of decomposition rates of soil organic matter with respect to simultaneous changes in temperature and moisture. J. Adv. Model. Earth Syst. 7, 335–356. doi: 10.1002/2014MS000358
Six, J., Conant, R. T., Paul, E. A., and Paustian, K. (2002). Stabilization of organic matter by soil minerals: implications for C-saturation of soils. Plant Soil 241, 155–176. doi: 10.1023/A:1016125726789
Steduto, P., Hsiao, C., Fereres, T. E., and Raes, D. (2012). FAO Irrigation and Drainage paper nr. 66. climasouth.eu. Available online at: http://www.climasouth.eu/sites/default/files/FAO~56.pdf (accessed December 15, 2022).
Stella, T., Mouratiadou, I., Gaiser, T., Berg-Mohnicke, M., Wallor, E., Ewert, F., et al. (2019). Estimating the contribution of crop residues to soil organic carbon conservation. Environ. Res. Lett. 14. doi: 10.1088/1748-9326/ab395c
Thakur, M. P., Reich, P. B., Hobbie, S. E., Stefanski, A., Rich, R., Rice, K. E., et al. (2018). Reduced feeding activity of soil detritivores under warmer and drier conditions. Nat. Clim. Chang. 8, 75–78. doi: 10.1038/s41558-017-0032-6
Tisdall, J. M., and Oades, J. M (1982). Organic matter and water-stable aggregates in soils. Eur. J. Soil Sci. 33, 141–163. doi: 10.1111/j.1365-2389.1982.tb01755.x
Van Veen, J. A., and Kuikman, P. J. (1990). Soil structural aspects of decomposition of organic matter by micro-organisms. Biogeochemistry 11, 213–233. doi: 10.1007/BF00004497
Walkley, A., and Black, I. A. (1934). An examination of the degtjareff method for determining soil organic matter, and a proposed modification of the chromic acid titration method. Soil Sci. 37, 29–38. doi: 10.1097/00010694-193401000-00003
Weil, R. R., Islam, K. R., Stine, M. A., Gruver, J. B., and Samson-Liebig, S. E. (2003). Estimating active carbon for soil quality assessment: a simplified method for laboratory and field use. Am. J. Altern. Agric. 18, 3–17. doi: 10.1079/AJAA2003003
Whitbread, A. M., Blair, G. J., and Lefroy, R. D. B. (2000). Managing legume leys, residues and fertilisers to enhance the sustainability of wheat cropping systems in Australia 1. The effects on wheat yields and nutrient balances. Soil Tillage Res. 54, 63–75. doi: 10.1016/S0167-1987(99)00112-9
Wu, G. L., Liu, Y., Tian, F. P., and Shi, Z. H. (2017). Legumes functional group promotes soil organic carbon and nitrogen storage by increasing plant diversity. Land Degrad. Dev. 28, 1336–1344. doi: 10.1002/ldr.2570
Yadav, G. S., Lal, R., Meena, R. S., Babu, S., Das, A., Bhowmik, S. N., et al. (2019). Conservation tillage and nutrient management effects on productivity and soil carbon sequestration under double cropping of rice in north eastern region of India. Ecol. Indic. 105, 303–315. doi: 10.1016/j.ecolind.2017.08.071
Yu, G., Fang, H., Gao, L., and Zhang, W. (2006). Soil organic carbon budget and fertility variation of black soils in Northeast China. Ecol. Res. 21, 855–867. doi: 10.1007/s11284-006-0033-9
Zeraatpisheh, M., Ayoubi, S., Mirbagheri, Z., Mosaddeghi, M. R., and Xu, M. (2021). Spatial prediction of soil aggregate stability and soil organic carbon in aggregate fractions using machine learning algorithms and environmental variables. Geoderma Reg. 27, e00440. doi: 10.1016/j.geodrs.2021.e00440
Keywords: carbon sequestration, crop rotation, crop residue, labile-C, weather variation
Citation: Gorooei A, Aynehband A, Rahnama A, Gaiser T and Kamali B (2023) Cropping systems and agricultural management strategies affect soil organic carbon dynamics in semi-arid regions. Front. Sustain. Food Syst. 6:1016000. doi: 10.3389/fsufs.2022.1016000
Received: 10 August 2022; Accepted: 08 December 2022;
Published: 04 January 2023.
Edited by:
Nishant K. Sinha, Indian Institute of Soil Science (ICAR), IndiaReviewed by:
Nirmalendu Basak, Central Soil Salinity Research Institute (ICAR), IndiaShamsollah Ayoubi, Isfahan University of Technology, Iran
Copyright © 2023 Gorooei, Aynehband, Rahnama, Gaiser and Kamali. This is an open-access article distributed under the terms of the Creative Commons Attribution License (CC BY). The use, distribution or reproduction in other forums is permitted, provided the original author(s) and the copyright owner(s) are credited and that the original publication in this journal is cited, in accordance with accepted academic practice. No use, distribution or reproduction is permitted which does not comply with these terms.
*Correspondence: Aram Gorooei, Z29yb29laUB1bmktYm9ubi5kZQ==