- 1Department of Soil, Crop, and Climate Sciences, University of the Free State, Bloemfontein, South Africa
- 2Agricultural Research Council – Natural Resources & Engineering (NRE), Pretoria, South Africa
Maize is a major food crop in Sub-Saharan Africa (SSA), and its productivity is affected by climate change-adaptive sustainable management practices. A 2-year field study (2019/20 and 2020/21 growing seasons) was carried out to evaluate the effect of sunn hemp (Crotalaria juncea L.) living mulch management on maize (Zea mays L.) production. Three sunn hemp planting periods were simultaneous with maize planting (P1), V15 maize growth stage (P2), and R1 maize growth stage (P3) and three densities 16.1 plants m−2 (D1-low), 32.1 plants m−2 (D2-medium), and 48.1 plants m−2 (D3-high). The intercrop components were planted in a split-plot treatment arrangement as an additive series with three replications and laid out in a randomized complete block design under the in-field rainwater harvesting (IRWH) technique. The growing season conditions revealed significant differences in rainfall distribution. Therefore, the planting period had highly significant (p < 0.001) effects on sunn hemp biomass, with an LSD value of 610.2 kg ha−1, showing that the highest was obtained in P2 (2636.7 kg ha−1) compared to P3 (811.3 kg ha−1). However, the P3 treatment resulted in maize grain yield penalty, with yields as follows: P3 (2775.2 kg ha−1), sole maize (3263.8 kg ha−1), P2 (3281.9 kg ha−1), and P1 (3287.8 kg ha−1). P2 yielded a significantly (p < 0.05) high-income equivalent ratio of 2.09, indicating a 109% advantage for increasing farmers' income by integrating sunn hemp under the no-till area of IRWH. P1 and P2 sunn hemp planting periods are viable options for smallholder farmers in summer rainfall regions to improve economic benefits.
Highlights
- Sunn hemp was used as a living mulch in a rainwater harvesting maize-based system.
- Identification of optimum planting and density for sunn hemp can improve performance.
- Biomass and grain improved with simultaneous and V15 maize growth stage planting.
- The highest income equivalent ratio and net benefits were obtained at medium density.
Introduction
Maize (Zea mays L.) is a versatile multi-purpose crop that is primarily used as a feed crop worldwide, but it is also an important food crop for many developing countries, particularly those in Sub-Saharan Africa (SSA), Latin America and Asia. It is grown on 90 million hectares, accounting for over 300 million MT of production [Food and Agriculture Organization of the United Nations (FAOSTAT), 2018]. However, rainfed cultivation practiced by ~90% of farmers in rural SSA and resource-constrained smallholder systems yields <1 t ha−1 of maize grain (Nyakudya and Stroosnijder, 2011; Van Ittersum et al., 2016; Njoroge et al., 2018). Rainfed production is unreliable in semiarid regions worldwide due to water scarcity caused by low rainfall and uneven distribution throughout the season. The yield varies significantly from year to year due to high precipitation variations. SSA country average yields for dryland maize ranged from 1.68 to 1.99 t ha−1 over a 9-year (2007–2016) (Food and Agriculture Organization of the United Nations (FAOSTAT), 2018). In-field rainwater harvesting techniques for drylands crop production have been implemented in many arid and semiarid regions worldwide to reduce future water scarcity.
In-field rainwater harvesting techniques describe the collection, storage, and use of rainwater runoff for crop production (Oweis and Taimeh, 1996). “In-situ” rainwater harvesting systems, also known as soil and water conservation systems, can increase the amount of water stored in the soil profile by holding rainwater where it falls (Salem et al., 2015). Deep tillage, contour farming, and ridging are common in-situ techniques that eliminate the separation of rainwater collection and storage areas. Micro-catchment techniques involve the construction of small structures across land slopes which collects surface runoff from the untilled-overland flow on short catchment lengths within the field and store in plant zone for subsequent plant use (Hensley et al., 2000). The ridge and furrow micro catchment water harvesting increased soil water content and grain yields (Liu et al., 2018; Wang et al., 2018). Several studies have investigated the benefits of micro-catchment techniques in crop production (Tabor, 1995; Zougmoré et al., 2003; Hensley et al., 2011). The in-field rainwater harvesting (IRWH) technique consists of a 2 m runoff strip and 1 m collection into the basin (Hensley et al., 2000; Botha et al., 2003, 2015). However, runoff strip requirement is associated with a loss of land area, which could be used for crop production compared to conventional tillage practices (Dzvene et al., 2021).
The suitability of the IRWH technique is thus argued to be related to local rainfall and soil profile characteristics, but management practices such as mulching and cropping system diversification can significantly improve its efficiency (Botha et al., 2003; Mzezewa et al., 2011; Tesfuhuney et al., 2015). With the IRWH technique, maize yields are affected by the amount and distribution of rainfall, with low precipitation (or severe drought years) resulting in low yields because there may be no runoff to collect (Botha et al., 2015; Dzvene et al., 2021). However, complementary management practices with varying mulching materials (Botha, 2006) or mulching levels (Tesfuhuney et al., 2015) improved maize productivity. Thus, the technique can be more effective in semiarid, drought-prone regions. According to the recommended application of the technology, Hensley et al. (2000) argued that the potential for yield enhancement is limited to clayey soils with a shallow profile. However, improvements in maize production were realized on fine sandy soil with high silt content and a B horizon profile that limits water movement (Tesfuhuney et al., 2015).
In contrast to conventional full area tillage, the technique provides yield benefits in different soils during dry years due to improved soil water storage (SWS) and reduced plant population because crops are only established in tramlines. The IRWH technique has been shown to increase maize, sunflower (Helianthus annuus), and dry bean (Phaseolus vulgaris) yields by 40, 30, and 90%, respectively (Botha et al., 2003). As evidenced by high evaporation losses in the runoff strip (Botha et al., 2003; Tesfuhuney et al., 2015), the improved water conditions are not limited to the basin area. In the Mzezewa et al. (2011) study to improve sunflower yield under the IRWH technique, cowpea (Vigna unguiculata) was incorporated as a living mulch using a row replacement intercropping series. Intercropping sunflower with cowpea resulted in grain yield reduction by 39.5–82.8% compared to the sole sunflower (Mzezewa et al., 2011). However, additional research using additive intercropping series is essential for integrating cover crops as living mulches. This can promote the utilization of the no-till strip to compensate for the associated land loss with IRWH and thus necessitates the careful selection of a multipurpose living mulch species, such as sunn hemp.
Sunn hemp (Crotalaria juncea L.) is native to India and grown for fiber, forage, and cover crop (Balkcom and Reeves, 2005; Mosjidis and Wehtje, 2011; Parenti et al., 2021). Sunn hemp is a drought-tolerant legume crop, requiring low rainfall (≈200 mm) to grow successfully (de Oliveira Miranda et al., 2020; Subrahmaniyan et al., 2021). The crop grows well in well-drained alluvial soils with sandy loam or loamy texture (Baligar and Fageria, 2007; Ashworth et al., 2015). In terms of biomass productivity, sunn hemp produces in the range of 2,500–4,000 kg ha−1 in 60–75 days, improving soil organic carbon and nitrogen, and can outperform cowpea, pigeon pea (Cajanus cajan), and other tropical legume cover crops (Akanvou et al., 2001; Baligar and Fageria, 2007; Baraibar et al., 2018). It is an economical crop under dryland because of its enhanced water and nutrient conservation in the soil (Sharma et al., 2010) and its ability to fix biological nitrogen in the soil (150–165 kg ha−1). In SA, C. juncea is usually cultivated as green manure, fodder, or blast fiber crop (Cook et al., 1998). Maize grain yield was not affected by the relay intercropping of sunn hemp in a warm temperate region of SA (Murungu et al., 2011). Sunn hemp cover crop increased maize grain yield by 8–27% in the absence of nitrogen (N) fertilization (Jeranyama et al., 2000). Therefore, sunn hemp has been used as a cover crop before or simultaneously with maize cropping systems.
Identifying a suitable planting date is one of the efficient strategies in rainfed agriculture to avoid living mulch competition with the main crop (Lawson et al., 2015). Dry spells caused by erratic rainfall distribution and occurrences of a short growing season, on the other hand, can jeopardize the growth of living mulches if they are planted late in the season. Intercropping velvet bean [Mucuna pruriens (L) DC], fish bean (Tephrosia vogelii Hook.f.), blue lupin [Lupinas angustifolius var. angustifolius (L)], hairy vetch (Vicia villosa Roth.), common oat (Avena sativa L.), and rhodes grass cv Katambora (Chloris gayana Kunth) as late as 8 weeks after planting maize compromised their biomass productivity (Mhlanga et al., 2016). Changing a cover crop's planting date in other climates was shown to affect its growth, biomass yield, and main crop yield (Mirsky et al., 2011; Lawson et al., 2015). Therefore, appropriate economically viable living mulch planting dates may allow smallholder farmers to diversify cropping systems and increase economic efficiency. Crop diversification among smallholder farmers has been linked to relative income stability, but may have a negative impact on farm economic efficiency due to intra-seasonal climate variability (Ponce, 2020). The current study hypothesized that optimum planting dates and densities of sunn hemp living mulch in a no-tillage maize under IRWH will increase the biomass and grain yield productivity and enhance the economic returns of smallholders in semiarid areas of SA. Therefore, this study, investigated how planting periods (P) and densities (D) of sunn hemp living mulch affected growth parameters, biomass, maize grain yields, and economic returns in a maize-based system under the IRWH technique.
Materials and methods
Experimental site and design
This study was conducted during 2019/2020 and 2020/2021 growing seasons at the Kenilworth Experimental Research Farm, University of the Free State (UFS) near Bloemfontein (Latitude 29°01′S, Longitude 26°09′E, Altitude 1,354 m above sea level), SA. The soil at the study site was classified as a Bainsvlei form according to the South African System (Soil Classification Working Group, 1991), equivalent to Chromic Stagnic Plinthic Cambisols (World Reference Base for Soil Resources (WRB), 2006). The soil was deep (>2,000 mm) with a fine sandy loam texture (Chimungu, 2009) and a clay, sand, and silt fraction of 8.5, 85, and 7%, respectively, at the start of the experiment (Table 1). The soil of the experimental site was moderately acidic with an average pH of 5.2, NH4-N concentrations of 10.3 mg kg−1, NO3-N concentrations of 11.2 mg kg−1, and available phosphorous concentrations of 7 mg kg−1 in the upper 300 mm horizons. The mean exchangeable base values for sodium, potassium, calcium, and magnesium were 22, 142, 336, and 100 mg kg−1, respectively. The climate of the study area was categorized as semiarid with relatively low and erratic annual rainfall of 528 mm and mean annual minimum and maximum temperatures of 11.0 and 25.5°C, associated with high annual evaporative demand of 2,294 mm.
Meteorological variables were measured from an automatic weather station installed at the experimental farm. The automatic weather station consisted of a tipping bucket rain gauge, cup anemometer, wind vane, a pyrometer and combined temperature and humidity sensor. All meteorological data (rainfall, minimum and maximum temperatures, minimum and maximum relative humidity, wind speed and direction, and solar radiation) were recorded on a CR10X data logger (Campbell Scientific, USA) every 5 min, averaged over 1 h for storage and daily data were calculated by averaging the hourly data.
The land was prepared conventionally with a ripper, mold plow and disc. A ridge maker was used to make furrows and the basins were constructed using a basin maker against the N-S slope for the IRWH plots in December 2017 in an E-W direction. The runoff strips in the plots were raked to smooth the topsoil. Toward the end of January 2018, a smooth runoff slope was formed by hand in the runoff section. The IRWH plots were established with a 2:1 basin to runoff strip width ratio, which was based on previous field experience with crops in semiarid environments. Maize crops were planted in tramlines (1.1 m wide), which were adopted from the previous IRWH technique in the eastern Free State, SA (Botha et al., 2003). The no-till runoff zone was used in this experiment to integrate living mulch and to assess economic benefits.
Maize was planted at a constant population of 18,000 plants ha−1. As a result, the experimental design for sunn hemp management was set up as a split-plot arranged in a randomized complete block design with three replications. To identify growth stages, the standard maize developmental stage system was used (from seedling emergence VE, to physiological maturity PM) (Ritchie et al., 1993). The experimental treatments (main plots) were sunn hemp at planting periods simultaneous with maize planting (P1), V15 maize growth stage (P2), and R1 maize growth stage (P3) assigned to the main plot. Sunn hemp was planted at three density levels of 16.1 plants m−2 (D1-low), 32.1 plants m−2 (D2-medium), and 48.1 plants m−2 (D3-high) in order to determine the optimum for intercropping. The sunn hemp subplots were planted in five rows, 90° to the runoff direction, with a 30 cm row spacing on the runoff strip of the IRWH technique. The intercrop components were sown in an additive series in both seasons (Connolly et al., 2001). Sole maize and sole sunn hemp were included, where sole sunn hemp was also planted at the respective three plant densities only at P1 treatment. The main plots were 180 m2 (12 m width 15 m length), and the subplots were 60 m2 (12 m width 5 m length). The schematic illustrations of the maize-based IRWH technique with sunn hemp management are depicted in Figure 1.
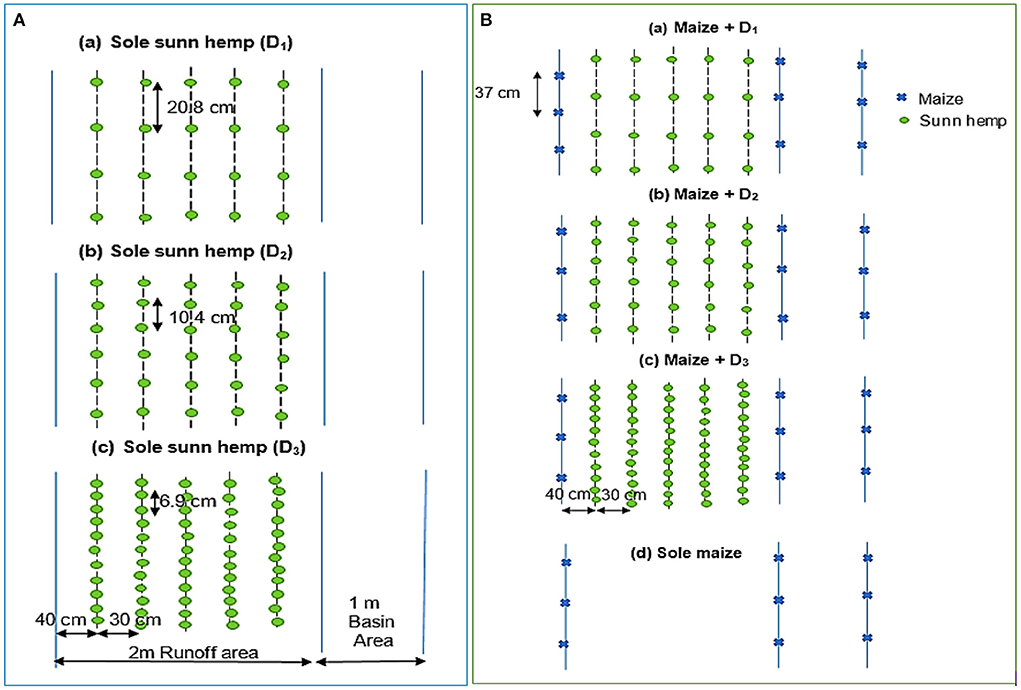
Figure 1. The spatial arrangement for living mulch cover crop planting density. (A) (a) Sole sunn hemp at D1 (16.1 plants m−2); (b) sole sunn hemp at D2 (32.1 plants m−2); (c) sole sunn hemp at D3 (48.1 plants m−2). (B) (a) Maize + sunn hemp at D1 (16.1 plants m−2); (b) maize + sunn hemp at D2 (32.1 plants m−2); (c) maize + sunn hemp at D3 (48.1 plants m−2); (d) sole maize.
Cropping system management
Planting of sole maize, sole sunn hemp, and P1 occurred on 3 December, 2019, and 23 November, 2020. The P2 sunn hemp was planted on 16 January, 2020 and 8 January, 2021, for each cropping season. P3 sunn hemp planting was done on 7 and 1 February for first and second growing seasons, respectively. Experimental crops were planted at relatively high densities and thinned to treatment planting densities 2 weeks after emergence. The growing season was sufficient for P1 and sole sunn hemp treatments to grow to maturity and produce seeds for sustainability in subsistence farming systems. However, growth measurements in all sunn hemp treatments were taken at 50% flowering and sunn hemp planted at P2 was terminated on 16 April, 2020 and 7 April, 2021. The sunn hemp planted at P3 grew slowly and did not reach flowering stage. Rainfed maize fertilizer applications were based on a potential yield of 5,000 kg ha−1 as determined by the Fertilizer Society of South Africa (FSSA) (2007). Maize (cv. Pioneer P2432R) and sunn hemp (cv. local) was fertilized with 200 kg ha−1 2:3:2 (22) NPK equivalent to 13 kg N ha−1, 19 kg P ha−1, and 13 kg K ha−1. No topdressing was applied on the sunn hemp. To meet the N requirements, a top dressing of 250 kg ha−1 LAN (28% N) (i.e., 70 kg ha−1 N) was applied as a split application to maize plots at 4 and 7 weeks after emergence. Weeds were manually controlled throughout the season, and spotted beetles were controlled with Dursban 480 EC as required. Crop harvesting was done by hand, and maize and sunn hemp stover was left in the field.
Data collection and calculations
Sunn hemp plant height and biomass
The plant height of sunn hemp during the flowering stage was determined by the average of 10 representative plants chosen from a 1 m−2 quadrant randomly placed in the plot. To calculate the biomass, all the plant samples harvested in the quadrant were oven-dried to a constant weight at 60°C for 72 h.
Growing degree days and leaf area index
The growing degree days for sunn hemp growth for each day from day after emergence (DAE) to flowering were calculated by averaging the maximum and minimum temperatures (°C) and subtracting 9.9°C as the base temperature (Qi et al., 1999). A linear AccuPAR LP-80 ceptometer was used to measure leaf area index (LAI) (Decagon Devices, Pullman, Washington, USA). The ceptometer collected data from 80 sensors embedded along with an 84 cm probe. At midday (12h00–14h00), LAI measurements were taken under clear skies. The LAI values were measured only at flowering of sunn hemp. At the soil surface, the line sensor was positioned perpendicular to the crop row.
Maize yield and harvest index
In this experiment, an area of 5 m2 maize plants were manually harvested at maturity from the middle of each plot. Maize biomass was weighed soon after harvesting. The maize cobs were threshed using a hand-powered thresher. Maize grains were weighed and the mass was calculated at a water content of 12%. Harvest index (HI) was calculated as the ratio of grain to aboveground biomass.
Approach and estimation of economic performance
The economic performance of the sunn hemp was evaluated using net benefits, income equivalent ratio, replacement value of sunn hemp, and relative net return index. Non-labor costs such as fertilizers, seeds, and insecticides were used to calculate production costs. This was because household members in subsistence farming performed manual labor for field operations like planting, weeding, harvesting, and threshing (Botha et al., 2003). Revenues were calculated by multiplying the harvestable plot outputs with the local market price to obtain the amount of money earned from the sale of plot output (grain from maize and fodder from sunn hemp).
The net benefits (or profits) ha−1 were calculated as the difference between seasonal revenues and seasonal costs for each cropping system for maize-sunn hemp system (NBmsh in Equation 1). The net benefits for sunn hemp were calculated based on fodder yield at flowering, as seen in Equation (1) (Midega et al., 2014):
where seasonal maize yields from sunn hemp, sole maize, sunn hemp fodder yield, and sunn hemp seed yield are denoted by Ymsh, Yms, Yshf, and Yshs, respectively. Pm, Pshf, and Pshs are prices for maize grain, sunn hemp fodder, and sunn hemp seed (as the seed has different prices), respectively. Cmsh and Cms are the costs of producing sunn hemp living mulch and sole maize crop.
The income equivalent ratio is defined as the area required under pure stand to produce the same gross income under the same management level as that required under a sunn hemp system (Devasenapathy, 2008), as shown in Equations (2–4).
Where IER is the income equivalent ratio, IERm and IERsh represent the partial IER of maize and sunn hemp, respectively. Ymi and Yms represent the yields of maize in sunn hemp and pure stand (sole), respectively. Yshi and Yshs are sunn hemp yields in intercropping and pure stand, respectively. Pm and Psh are the prices for maize grain and sunn hemp (or Lucerne) fodder or seed, respectively.
The replacement value of a sunn hemp living mulch system proposed by Moseley (1994) and Singh et al. (2015) was used to account for the variable costs associated with the sunn hemp enterprise in relation to the economic value of the cultivated crop (Equation 5).
where Cms is the variable cost of maize (the main crop) in a pure stand. The relative net return index was calculated following a formula (Equation 6) suggested by Mead and Riley (1981).
Where RNRI is the relative net return index, Dmsh is the difference in the cost of cultivation (variable cost) between maize-sunn hemp system and that of pure maize stand. A RNRI value >1 is preferred because it indicates that sunn hemp gives higher returns compared to pure stand.
Statistical analysis
The JMP Pro 14 statistical software for Windows was used for all analyses (SAS Institute, Inc., North Carolina, USA, 2010). Treatments were considered as maize plots with varying planting periods and densities of sunn hemp for one-way analysis of variance (ANOVA) to test the statistical significance of treatments in comparison with the sole maize between growing seasons. However, for testing the planting period and density effects, as well as their two way interactions on sunn hemp growth and biomass, the variables were fitted as fixed factors. When the significance of the treatment on the F-statistic is mentioned, it refers to a comparison using the least significant differences (LSD) at the 0.05 probability level.
Results
Weather conditions and growing degree days
Treatment differences were attributed to varying weather patterns across the experimental seasons. Rainfall distribution, average temperature and timing of sunn hemp planting for the 2019/20 and 2020/21 growing seasons are presented in Figure 2. The primary weather variable, rainfall, influenced sunn hemp living mulch management practices in both seasons. Therefore, because of the rainfall variations (Figure 2A), the maize and sunn hemp planting dates differed between the two experimental years, with early planting occurring in the first growing season (2019/20). The total amount of rainfall during the growing season was 673.7 mm, evenly distributed throughout the season, with 16.1, 24.2, 18.9, 20.1, and 18.4% rainfall in December, January, February, March, and April, respectively. Plant emergence was slow and poor in all treatments because of the small amount (2.3%) of rainfall received at P1 planting. The continuous rainfall distribution throughout the following months was favorable for P2 and P3 treatments during 2019/20, as shown in Figure 2A.
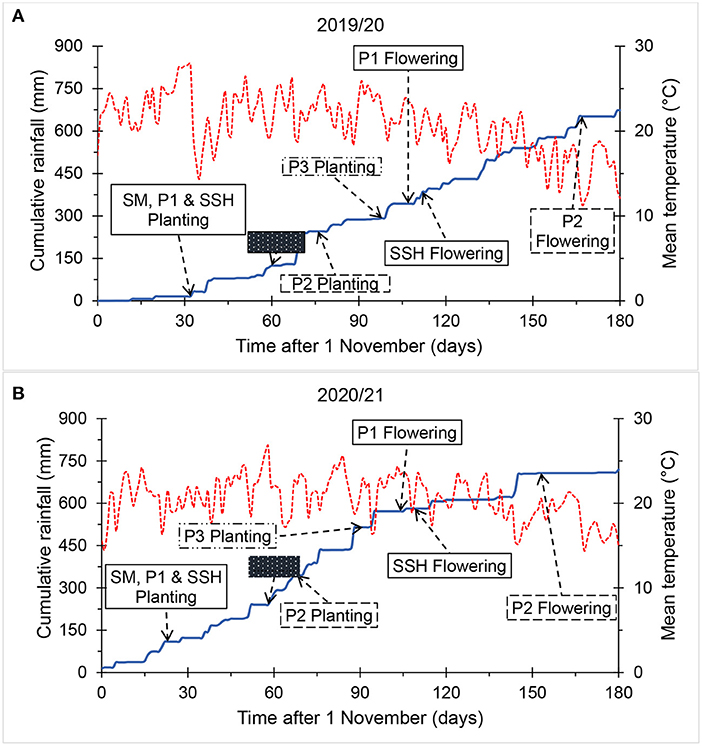
Figure 2. Cumulative daily rainfall, average daily temperature, and agronomic management practices during (A) 2019/20 and (B) 2020/2021 growing seasons. Red dotted line is the average daily air temperature, blue line is the cumulative rainfall since 1 November, shaded block is when in-field rainwater harvesting basins were modified. SM, sole maize; SSH, sole sunn hemp; P1, simultaneous sunn hemp intercropping; P2, intercropping sunn hemp at the early maize vegetative growth stage; P3, intercropping sunn hemp at the late maize vegetative growth stage.
Due to the good early rainfall distribution during the 2020/21 season, sole maize, sole sunn hemp and P1 treatments were planted in late November 2020, as 17% of total seasonal rainfall had already been received (Figure 2B). However, it was a disadvantage for establishing and growing P1 and sole sunn hemp treatments because sunn hemp is prone to fungal and bacterial diseases under wet conditions. Rainfall peaked in January (32.5% of total seasonal rainfall) but declined from February to April 2021. April received only 2% of total seasonal rainfall, causing poor and delayed germination in the P3 treatment. Overall, the second cropping season (2020/21) was the wettest, with 718.8 mm of seasonal rainfall received.
Sunn hemp flowering occurred at different days after emergence (DAE) during the respective 2019/20 and 2020/21 growing seasons as follows: sole sunn hemp (76 and 82 DAE), P1 (71 and 77 DAE), and P2 (88 and 82 DAE) (Figures 2A,B). The growing degree days (°Cd) to reach flowering varied depending on the sunn hemp planting period, cropping system and season (Figures 3A,B). During the 2019/20 and 2020/21 growing seasons, the sole sunn hemp treatment required 988 and 995°Cd to grow and reach the flowering stage, respectively. During the 2019/20 and 2020/21 growing seasons, P1 treatments required 934 and 936°Cd, while P2 treatments required 933 and 941°Cd to grow and reach flowering stage, respectively. This demonstrated that intercropping sunn hemp reduced the growing degree days required for flowering, resulting in earlier flowering when compared to sole sunn hemp control treatments. Sunn hemp living mulch planted at P3 did not reach flowering stage because it is a photoperiod sensitive crop.
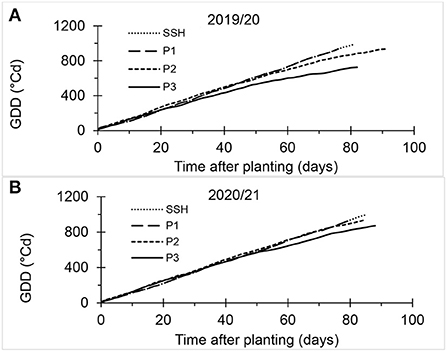
Figure 3. The growing degree days (GDD) (°Cd) for sunn hemp living mulch management practices during (A) 2019/2020 and (B) 2020/2021 growing seasons (SSH, sole sunn hemp; P1, simultaneous sunn hemp and maize planting; P2, sunn hemp planted at the V15 maize growth stage; P3, sunn hemp planted at R1 maize growth stage).
Management effects on sunn hemp growth and yield
Plant height
The sunn hemp plant height of 200.6 cm obtained with the P2 treatment during the second growing season (2020/21) was significantly different from the 163 cm obtained with the same treatment during the first growing season (2019/20) (Table 2). Sunn hemp growth in the 2019/20 growing season, on the other hand, achieved significantly higher plant heights in sole sunn hemp and P3 when compared to the 2020/21 growing season. The highest sunn hemp plant height values obtained with P2 in both growing seasons demonstrated the competitive response of sunn hemp establishment into standing maize, which is an important factor for farmers to consider in adoption decisions.
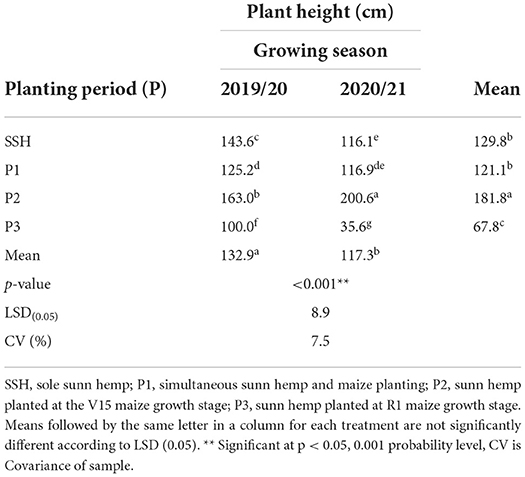
Table 2. Sunn hemp plant height as influenced by planting period (P) and growing season (S) under the in-field rainwater harvesting technique in the central semiarid area of the Free State Province, South Africa.
Leaf area index
There was consistent significant (p < 0.05) increases in LAI values with plant density in the sole sunn hemp (D1, 2.19 m−2m−2; < D2, 5.18 m−2m−2; < D3, 6.20 m−2m−2) and P1 (D1, 1.86 m−2m−2; < D2, 4.07 m−2m−2; < D3, 5.66 m−2m−2) treatments during the 2019/20 cropping season (Figure 4A). Management at P1 with D3 planting density had comparable significant (p < 0.05) LAI values to D2 and D3 values in sole sunn hemp in the same cropping season. The overall results revealed a linear increase in sunn hemp leaf area index with increasing plant density per area (Figure 5B). However, comparable LAI values were obtained during the 2020/21 growing season with P2 and P3 management at D2 and D3 planting densities (Figure 4B). The LAI is an important indicator of sunn hemp morphological plasticity, which can be exploited by managing plant density. The interplay of planting period and density is one practice that farmers can use to improve the benefits of sunn hemp living mulch, as reflected in LAI, which represents canopy shading.
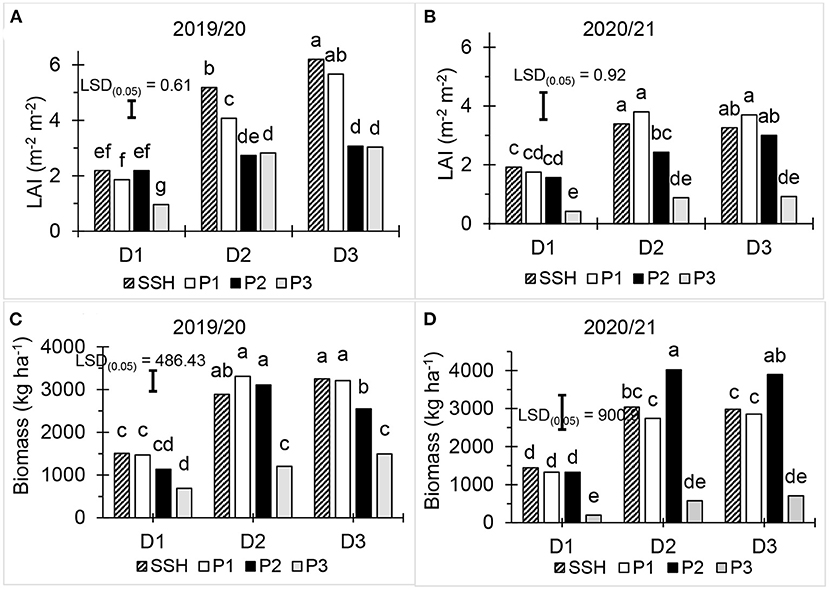
Figure 4. Planting period and density effects on sunn hemp under the in-field rainwater harvesting technique in the central semiarid area of the Free State Province, South Africa: (A) leaf area index (m−2m−2) during 2019/2020 growing season; (B) leaf area index (m−2m−2) during 2020/21 growing season; (C) biomass (kg ha−1) during 2019/20 growing season; (D) biomass (kg ha−1) during 2020/21 growing season (SSH, sole sunn hemp; P1, simultaneous sunn hemp and maize planting; P2, sunn hemp planted at the V15 maize growth stage; P3, sunn hemp planted at R1 maize growth stage; D1-low, 16.1 plants m−2; D2-medium, 32.1 plants m−2; D3-high, 48.1 plants m−2). Different letters on the bars indicate statistical significance.
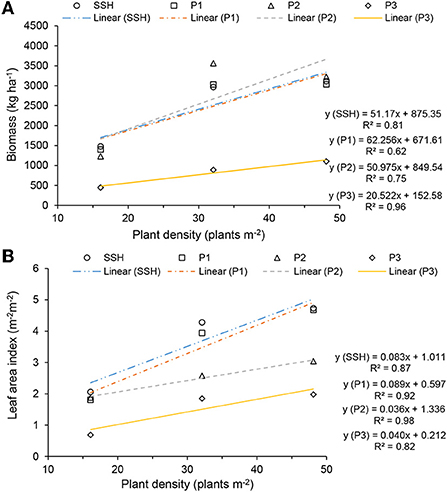
Figure 5. Sunn hemp biomass (kg ha−1) (A) and leaf area index (m−2m−2) (B) as a function of plant density per area (SSH, sole sunn hemp; P1, simultaneous sunn hemp and maize planting; P2, sunn hemp planted at the V15 maize growth stage; P3, sunn hemp planted at R1 maize growth stage).
Yield
The biomass yield differed significantly (p < 0.05) between planting period treatments when planted at D3 during the 2019/20 growing season (Figure 4C). The differences were: P1D3, 3,209 kg ha−1, < P2D3, 2,546 kg ha−1, and < P3D3, 1495.3 kg ha−1, in that order. The P3 treatment produced significantly less biomass than the sole sunn hemp and other treatments at all plant densities. However, the biomass yield obtained with P3 at D2 and D3 did not differ statistically from that obtained with sole sunn hemp, P1, and P2 at D1. The P3 effect was the same during 2020/21 growing season (Figure 4D). Sunn hemp planting with P2 at D2 and D3 during the 2020/21 growing season performed significantly (p < 0.05) better when compared to sole sunn hemp and other planting period treatments. The study's findings revealed a linear increase in sunn hemp biomass (Figure 5A) with increasing plant density per area. The interplay of sunn hemp living mulch planting period and density management with the growing season emphasizes the importance of adjusting them based on the forecasted weather conditions for the growing season. Allowing the sole sunn hemp and P1 treatments growth to maturity (see Appendix A1) showed that highest biomass yields were obtained at D2 (sole sunn hemp: 10234.7 kg ha−1 and P1: 9355.5 kg ha−1). The sole sunn hemp treatment at D2 (1029.7 kg ha−1), D3 (917.8 kg ha−1) and for P1 at D2 (911.3 kg ha−1) had the highest seed yield.
Effects of sunn hemp management on maize yield
Planting period
Sunn hemp planting period had significant (p < 0.001) effects on maize biomass showing that P1 and P2 treatments improved maize biomass productivity in both growing seasons (Table 3a). During the 2019/2020 growing season, P1 (6292.8 kg ha−1), and P2 (6096.9 kg ha−1) treatments were the highest compared to P3 (4996.4 kg ha−1) and sole maize (5411.7 kg ha−1). Sunn hemp planting at P3 resulted in significant (p < 0.014) maize grain yield reduction. The maize grain yields were P3 (2775.2 kg ha−1), sole maize (3263.8 kg ha−1), P2 (3281.9 kg ha−1), and P1 (3287.8 kg ha−1). Planting sunn hemp with P1 is an important management factor to for farmers to consider when aiming for higher biomass and grain yields. The 2019/20 growing season resulted in the highest grain yield of 3395.6 kg ha−1 compared to 2908.7 kg ha−1 in 2020/21 growing season. The harvest index significantly differed between the two growing seasons with the highest (0.60) obtained in 2019/20 (Table 3a).
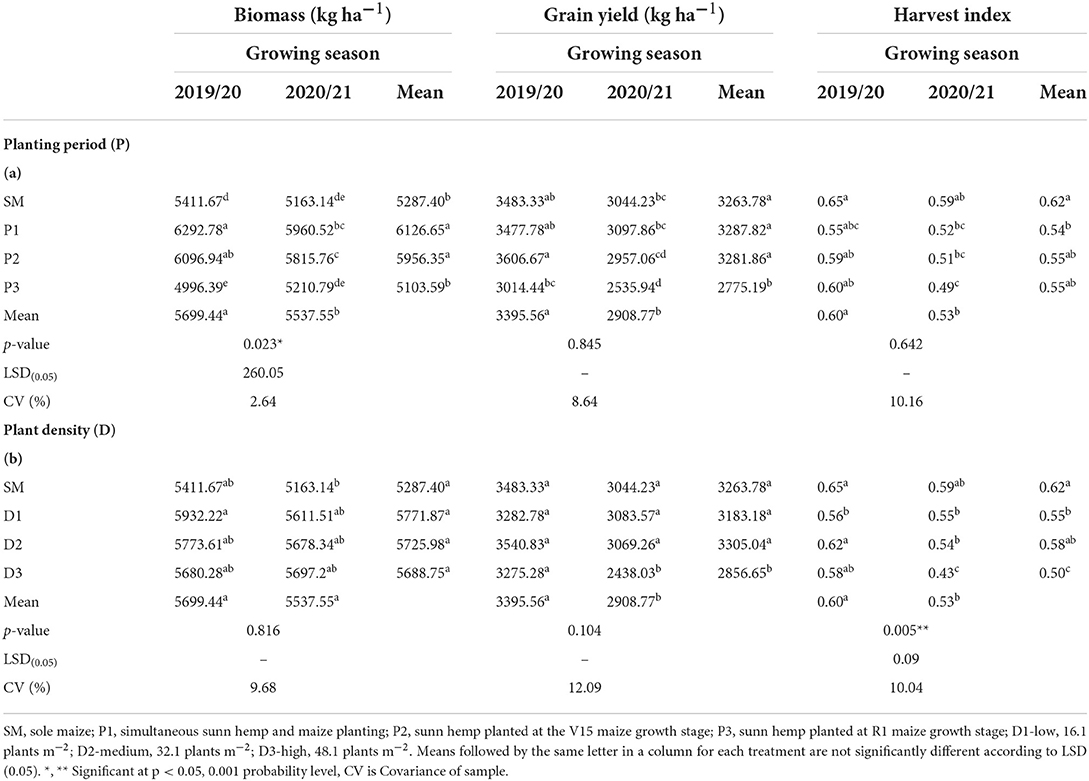
Table 3. Effects of sunn hemp living mulch planting period (P) and density (D) on maize biomass, grain yield, and harvest index under the in-field rainwater harvesting technique in the central semiarid area of the Free State Province, South Africa.
Plant density
Sunn hemp plant density resulted in significant (p < 0.005) effects on maize grain yield where at D2, the highest grain yield of 3305.0 kg ha−1 was not different from sole maize (3263.8 kg ha−1) and D1 (3183.2 kg ha−1) (Table 3b). The lowest grain yield was obtained with D3 (2856.7 kg ha−1). Sunn hemp plant density had significant (p < 0.005) effects on harvest index showing that 2019/20 growing season resulted in high values of 0.65 and 0.62 obtained in sole maize and D2, respectively, and were not significantly different in D3 (0.58) (Table 3b). Therefore, D2 was the optimum sunn hemp plant density to consider as maize grain yield was higher compared to sole maize control. This is an advantage for obtaining living mulch benefits with less sunn hemp seed and without reducing maize grain yield. The overall results showed a linear decrease in biomass and grain yield with increase in sunn hemp plant density per area (Figures 6A,B).
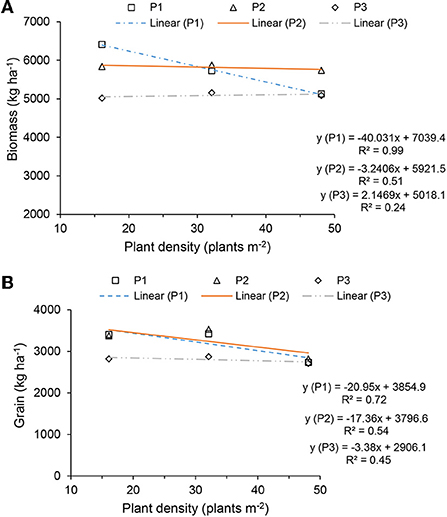
Figure 6. Maize biomass (A) and grain yield (kg ha−1) (B) as a function of plant density per area (P1, simultaneous sunn hemp and maize planting; P2, sunn hemp planted at the V15 maize growth stage; P3, sunn hemp planted at R1 maize growth stage).
Interactive management effects on economic benefits
Sunn hemp planting period yielded high net benefits for P1 (USD 404.07) and P2 (USD 408.31) (Table 4). P3 had the lowest profits due to the low biomass of sunn hemp and the yield reduction effect. However, D2 was the best sunn hemp planting density, with a net benefit advantage of USD 407.96, indicating that farmers should consider seed saving. Furthermore, when mature P1 sunn hemp seeds were harvested, D2 (see Appendix A2) provided the highest profits. Their impact exhibited a similar pattern to net benefits for the replacement value of sunn hemp (RVsh) and relative net return index. The sunn hemp planting period effect for RVsh was P1 (1.09) and P2 (1.12), and for relative net return index P1 (1.20) and P2 (1.21). At P1 and P2 planting periods, the RVsh was more significant than one, indicating that using sunn hemp as living mulch was a highly beneficial management practice for farmers using IRWH technique. However, farmers should avoid using the P3 planting period because a relative net return index value <1 indicated that sunn hemp in sole stand yielded higher returns. Although the planting density effect was increased in both RVsh and RNRI at D2, an RVsh value of 0.95 at D3 indicated that intercropping that sole stand resulted in a loss. When P1 was harvested at maturity, the RVsh and RNRI values obtained with D1 (see Appendix A2) were more significant than one, but they were the lowest compared to D2 and D3.
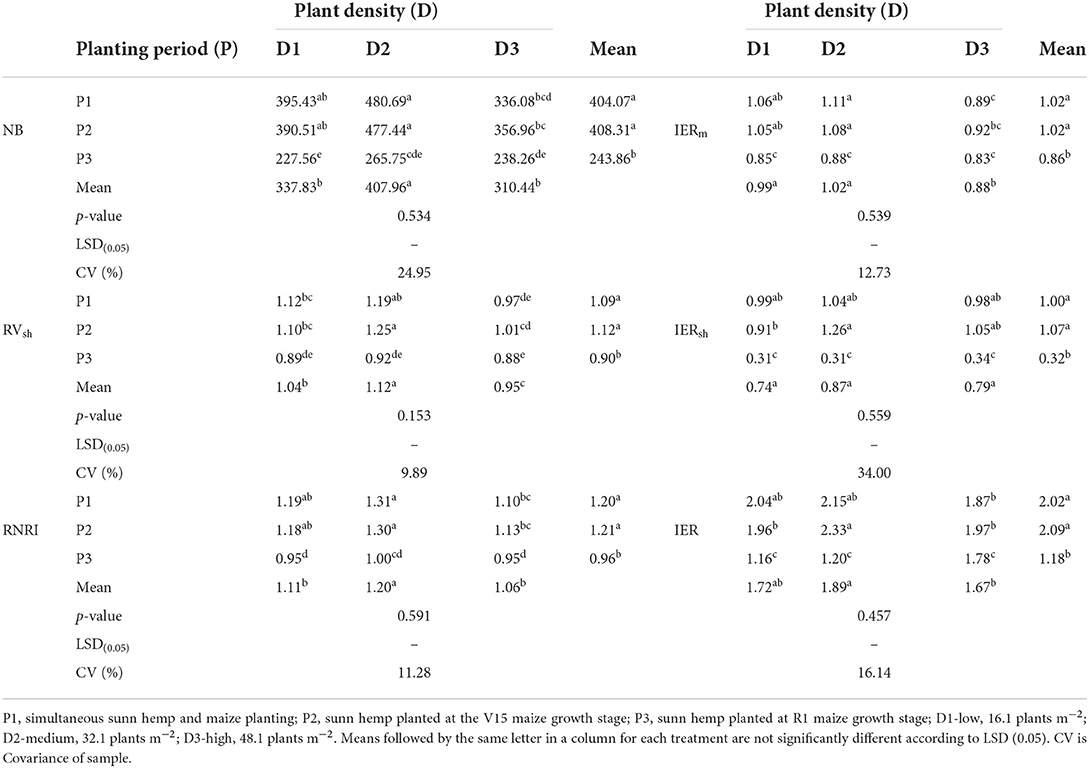
Table 4. Effects on sunn hemp management on net benefits (NB), replacement value of sun hemp (RVsh), relative net return index (RNRI), maize income equivalent ratio (IERm), sunn hemp income equivalent ratio (IERsh), and income equivalent ratio (IER) under in-field rainwater harvesting technique in the central semiarid area of the Free State Province, South Africa.
Sunn hemp planting period and plant density had a significant (p < 0.05) effect on the maize income equivalent ratio (IERm) (Table 4). The planting period resulted in a significantly lower IERm (0.86) at P3 than at P1 and P2 (1.02). Plant density also reduced IERm at D3 (0.88) vs. D1 (0.99) and D2 (1.02). The values obtained at P3 and D3 were >0.5, indicating that the benefits of intercropping in improving economic returns were realized. However, planting period at P3 (0.32) resulted in a value <0.5 for sunn hemp IERsh. The total income equivalent ratio for all planting period treatments P1 (2.02), P2 (2.09), and P3 (1.18) was greater than unity, but the P3 value was significantly lower.
Discussion
The goal of this study was to determine how planting periods (P) and densities (D) of sunn hemp living mulch affected growth parameters, biomass, maize grain yields, and economic returns in an IRWH maize-based system. Comprehensive living mulch performance and impact on main crop is required for providing recommendations for smallholder farmers in rainfed semiarid areas to sustainably integrate living mulches in maize monocropping systems.
Sunn hemp performance
The impacts of living mulch planting period on growth and biomass are well-known. Generally, earlier planting of living mulch into the main crop will result in early ground cover and higher biomass relative to late planting. However, improved growth of early planted living mulches is attributed to the long growing season exposure of temperature and rainfall distribution (Wilson et al., 2013; Curran et al., 2018). This notion, however, is dependent on the living mulch species. In this study, 17% of rainfall was received at the start of the 2020/21 growing season (Figure 2B) but early sunn hemp establishment and growth at P1 was slow and some leaf necrosis was observed (data not shown). However, the plants recovered and densities were not compromised because treatments were planted at relatively high densities. High rainfall limits the growth of sunn hemp, which is susceptible to waterlogging soil conditions and becomes vulnerable to fungal and bacterial diseases (Baligar and Fageria, 2007). In relation to this study, establishment of sunn hemp was particularly challenging under the IRWH technique especially when planting with high rainfall amounts and subsequent intolerance to water logging conditions (Subrahmaniyan et al., 2021). Other research has shown that late planting of living mulch into an established main maize crop is a recommended management practice for allowing the living mulch to utilize late season rainfall and soil moisture (Mirsky et al., 2011; Belfry and Van Eerd, 2016; Mhlanga et al., 2016).
In this study, the late planting of sunn hemp into standing maize at the R1 maize growth stage (P3) resulted in significantly low biomass (Figures 4C,D). Although rainfall was fairly distributed during 2019/20 compared to 2020/21 growing season there were no significant differences observed in biomass with P3. This contradicts the notion of less rainfall amount and distribution availability associated with late planting of living mulches (Wilson et al., 2013). Therefore, there are other factors involved in determining the biomass of sunn hemp. Simultaneously planting with maize (P1) and V15 maize growth stage (P2) were the best planting periods for obtaining high biomass. This observation was likely due to well-distributed rainfall, less maize competition for water or sunlight, and enough number of growing degree days required for sufficient biomass production (Lawson et al., 2015). Therefore, in this study competition for sunlight with main crop and limited number of growing degree days could have been the main factors that limited P3 biomass production. Figures 2A,B showed that the mean temperatures dropped in both growing seasons when the P3 living mulches were planted. Sunn hemp development and biomass production is influenced by the interaction of photoperiod and temperature, as well as planting period (de Oliveira Miranda et al., 2020; Subrahmaniyan et al., 2021). Dropping temperatures observed at late planting in the growing season and the association with shortening day lengths could have been the main causes of a decrease in biomass production in P3. As a result, seeding sunn hemp early in the summer wet season yielded significantly more than later sowing. In addition, the growing season had a direct effect on the vegetative growth and development of sunn hemp.
Information on how sunn hemp plant density influences its growth and biomass is vital for optimizing living mulch benefits (de Oliveira Miranda et al., 2020). In this study, the evaluation of sunn hemp at three plant densities showed that D2 (32.1 plants m−2) and D3 (48.1 plants m−2) resulted in the highest biomass accumulation when compared to D1 (16.1 plants m−2) (Figures 4C,D). Sunn hemp biomass production is plant density responsive, meaning that it increases when planted at higher plant densities relative to low plant densities (de Oliveira Miranda et al., 2020). This study found that biomass attained with P1 (simultaneous planting sunn hemp with maize) and P2 (planting at the V15 maize growth stage) at D2 (32.1 plants m−2) and D3 (48.1 plants m−2) were higher than those in D1 (16.1 plants m−2). It was evident that low density planting of sunn hemp resulted in low biomass regardless of the planting time. Thus, when considering increasing biomass production by increasing plant density, it is critical to consider seed availability and cost. Farmers may be discouraged from using higher sunn hemp plant densities in smallholder and subsistence farming due to the low biomass return compared to the higher seed cost when increasing plant density at D2 and D3. As a result, farmers must produce their own seed in order to continue to practice sustainable agriculture. However, the LAI values were similar for the various planting periods and densities between both growing seasons (Figures 4A,B). These results show that sunn hemp can compensate for low planting densities through intraspecific mechanisms of competition, such as branching and higher morphological plasticity, i.e., modified growth habits to occupy more space (Morris et al., 2015). According to Morris et al. (2015), planting sunn hemp at low density resulted in six branches, more than the three produced by high-density planting. Although increasing plant populations have traditionally been proposed to increase cover cropping benefits (Mosjidis and Wehtje, 2011), this explains the similarities in LAI values in this study. Such information is particularly relevant to the organic farming system, where weed management is expensive and the farmer has limited options because of the non-inclusivity of chemical management. When sunn hemp was planted at low densities ranging from 20 to 50 plants m−2, Mosjidis and Wehtje (2011) discovered no significant differences in biomass yields and substantial reductions in weed biomass. Sunn hemp living mulch could greatly benefit from maize production systems under IRWH technique because weed management is a pressing issue, especially for financially limited subsistence farmers.
Maize performance
The fundamental principle of a living mulch is to provide vegetative cover during the main crop growing season on soil surfaces that would otherwise be bare (Mzezewa et al., 2011). However, competition for resources with main crop is one of the biggest and most obvious concerns and limitations of living mulches, which may result in main crop yield reductions (Jeranyama et al., 2000). This is a particular problem in areas where water is a limiting resource, or where production is dependent on rainfall, as living mulches will utilize water that could otherwise have been utilized by the main crop (Batista De Morais et al., 2020). This study (Table 3a) showed that planting the sunn hemp living mulch earlier at P1 (simultaneous planting sunn hemp with maize) and P2 (planting at the V15 maize growth stage) could boost maize production with the IRWH technique. Sunn hemp has a vigorous early growth that is associated with high water use, so introducing it earlier in the maize growing season is a subtle management approach, which allows it to use water when the maize requires less water. Therefore, the earlier establishment of the sunn hemp living mulch resulted in a complementary use of water resources, which could have had an effect on soil moisture conservation through canopy shading later in the growing season when maize water demand was at a peak.
Maize water demands are critical at the flowering and grain filling stages and water competition during those periods can result in maize grain yield penalty (Batista De Morais et al., 2020). This is important in explaining the grain yield reductions observed in this study when the sunn hemp living mulch was planted at the R1 maize growth stage (P3). In 2019/20, the maize grain yield obtained with P3 was not significantly different from P1 (simultaneous planting of sunn hemp and maize) or sole maize (Table 3). Furthermore, P3 maize grain yield did not differ significantly from P2 maize grain yield during the 2020/21 growing season (planting at the V15 maize growth stage). Excessive nutrient competition during critical growth stages for component crops with P3 (R1 maize growth stage), particularly for soil mineral N, may have resulted in maize grain yield reduction, as N is a critical macronutrient that determines yield potential. However, if there had been a dry season, water competition effects on maize grain yield reduction could have been observed with P1 (simultaneous planting of sunn hemp and maize) and P2 (planting at the V15 maize growth stage). Similar findings on main crop grain yield reduction were observed in Brazil when maize was intercropped with living mulches including palisade grass (Urochloa brizantha cv. Marandu), pigeon pea (Cajanus cajan cv. Iapar 43), and sunn hemp (Batista De Morais et al., 2020). However, in another study, cover crops did not affect maize biomass or grain yields in SA's warm temperate zone when they were relay planted at the maize vegetative growth stage under conventional tillage (Murungu et al., 2011). Relay planting of cover crops at the maize establishment stage on sandy, loamy soil in Zimbabwe did not reduce maize grain yields, whether fertilized with 60 kg N−1 or not (Jeranyama et al., 2000). However, due to differences in growing conditions, planting time, and species grown, there can be several positive, neutral, negative or mixed responses of living mulch on the main crop (Mohammadi and Ghobadi, 2010; Belfry and Van Eerd, 2016). Ruffatti et al. (2019), for example, found a 7–22% reduction in maize yield when using a rye and radish blend as a living mulch. In contrast, Belfry and Van Eerd (2016) observed no yield reduction when intercropping 17 different cover crop species along with varying mixes into maize at the V4–V6 stage. Balkcom and Reeves (2005) obtained maize grain yield improvement of 22% in a maize cropping sequence following sunn hemp. The findings indicated that growing maize with living mulch is a management tool to provide ecosystem benefits, but its success and impact on the main crop depends on the growing environment and specific management activities.
Economic performance
The effect of sunn hemp living mulch on the economic status in a maize-based cropping system was investigated using economic benefit analysis. Cover cropping is rarely evaluated in terms of the purported economic impacts of its use in a cropping system. However, studies report negative effects, such as crop yield reductions caused by cover crop use (Ruffatti et al., 2019). Claims of massive economic gain are common with cover crops in cropping systems as well (Schomberg et al., 2014). The importance of cover crops is to increase productivity by improving soil fertility, to reduce the build-up of endemic diseases and pests associated with monoculture, and to reduce production costs by reducing or avoiding the use of external inputs, particularly fertilizers and pesticides (Baraibar et al., 2018). Cover crops have, however, costs that may limit their use. These include the cost of additional seed, the time required to manage an additional crop, which may necessitate changes to current cropping plans, and the cost of an additional herbicide or increased tillage to kill the cover crop in order to plant the cash crop (Cai et al., 2019).
The lack of immediate economic return and the area that must be occupied by living mulches are among the main causes for the lack of adoption. As a result, selecting living mulch species with immediate economic returns, such as the sale of forage or seeds (Schomberg et al., 2014), is the first appropriate management to improve the economic returns of crop-livestock smallholder farmers. A comparison of net benefits between sunn hemp planting period and density treatments was made based on the value of the harvested sunn hemp forage at flowering or seed harvest at maturity. We assumed that sunn hemp seed harvest was an opportunity for smallholder farmers to ensure continued adoption. In this scenario, P1 (simultaneous planting sunn hemp with maize) offers an economic advantage over termination of sunn hemp at flowering, since the seed value of sunn hemp would be greater and forage cost of sunn hemp would be less. Therefore, at forage stage, sunn hemp planted at P1 yielded higher net benefits than when planted at P2 (planting at the V15 maize growth stage) and P3 (planting at the R1 maize growth stage) (Table 4). At maturity, sunn hemp planted at P1 yielded higher net benefits than when planted at D2 (medium density: 32.1 plants m−2) and D3 (high density: 48.1 plants m−2) (Appendix A2). However, the high net benefits of P1 (simultaneous planting sunn hemp with maize) both at flowering and maturity were greater at D2 (32.1 plants m−2) than at D3 (48.1 plants m−2), and this was associated with higher seed yields at medium density. The effect on net benefits was also similar for replacement value, relative net return index, and income equivalent ratio.
The income equivalent ratio (IER) may vary yearly due to fluctuating market prices for inputs and crop outputs. Because it affects market demand and supply dynamics, climate change directly impacts price volatility. The IER is conceptually similar to the LER, with the difference that yield is measured in terms of net income rather than plant productivity. The IER (Devasenapathy, 2008), simply states that a (i) value of one indicates that the income of the sole cropping system and the intercropping system is equivalent, (ii) a value >1 indicates that the intercropping system provides a positive income benefit, and (iii) a value <1 indicates that the sole cropping system provides a higher income than the intercropping system. In this regard, results of partial IER for maize (IERm) obtained for P3, which was <1 but above 0.5, means that there was an economic advantage for both component crops in gross income (Table 4). However, partial IERsh obtained with P3 of <0.5 showed an income loss with sunn hemp integration. This is because crop yield, price, and inputs determine gross income even when agronomic responses are consistent.
Conclusion
The results show that late planting of sunn hemp living mulch during R1 maize growth stage had a negative effect on maize grain yield. However, planting of sunn hemp during the V15 maize growth stage was appropriate for incorporation with maize production in semiarid areas. This was due to the good amount of sunn hemp biomass produced without reducing maize yield relative to maize monocrop. The economic analyses indicated that the highest economic productivity in terms of net benefits, replacement value, and relative net return index was achieved with simultaneous sunn hemp planting with maize (P1) at the planting densities of 32.1 plants m−2 (D2) and 48.1 plants m−2 (D3). Therefore, we recommended an earlier sunn hemp planting at a medium density to improve biomass, maize grain yield and economic benefits in the semiarid areas.
Data availability statement
The datasets presented in this article are not readily available because the experiments are still ongoing. Requests to access the datasets should be directed to dzvenea@gmail.com.
Author contributions
AD, WT, SW, and GC conceptualized the research idea, designed the research, monitored data collection, analyzed the data, draft, and write the final manuscript. All authors contributed to the article and approved the submitted version.
Acknowledgments
The authors are grateful to Mr. Elias Yokwane, Department of Soil, Crop, and Climate Sciences, University of the Free State, Bloemfontein, for technical assistance during field trials. The Water Research Commission (WRC), the National Research Fund (NRF), and the Food and Beverages Manufacturing Sector Education and Training Authority (FoodBev SETA) are acknowledged.
Conflict of interest
The authors declare that the research was conducted in the absence of any commercial or financial relationships that could be construed as a potential conflict of interest.
Publisher's note
All claims expressed in this article are solely those of the authors and do not necessarily represent those of their affiliated organizations, or those of the publisher, the editors and the reviewers. Any product that may be evaluated in this article, or claim that may be made by its manufacturer, is not guaranteed or endorsed by the publisher.
References
Akanvou, R., Bastiaans, L., Kropff, M. J., Goudriaan, J., and Becker, M. (2001). Characterization of growth, nitrogen accumulation and competitive ability of six tropical legumes for potential use in intercropping systems. J. Agron. Crop Sci. 187, 111–120. doi: 10.1046/j.1439-037X.2001.00503.x
Ashworth, A. J., West, C. P., Allen, F. L., Keyser, P. D., Weiss, S. A., Tyler, D. D., et al. (2015). Biologically fixed nitrogen in legume intercropped systems: comparison of nitrogen-difference and nitrogen-15 enrichment techniques. Agron. J. 107, 2419–2430. doi: 10.2134/agronj14.0639
Baligar, V. C., and Fageria, N. K. (2007). Agronomy and physiology of tropical cover crops. J. Plant Nutr. 30, 1287–1339. doi: 10.1080/01904160701554997
Balkcom, K. S., and Reeves, D. W. (2005). Sunn-hemp utilized as a legume cover crop for corn production. Agron. J. 97, 26–31. doi: 10.2134/agronj2005.0026
Baraibar, B., Hunter, M. C., Schipanski, M. E., Hamilton, A., and Mortensen, D. A. (2018). Weed suppression in cover crop monocultures and mixtures. Weed Sci. 66, 121–133. doi: 10.1017/wsc.2017.59
Batista De Morais, D., Felippe Ratke, R., Bortolon, L., Junio De Jesus Lacerda, J., Loiola Edvan, R., Mario Zuffo, A., et al. (2020). Maize intercropping systems improve nutrient for the cowpea crop in sandy soils. Commun. Soil Sci. Plant Anal. 51, 491–502. doi: 10.1080/00103624.2020.1718688
Belfry, K. D., and Van Eerd, L. L. (2016). Establishment and impact of cover crops intersown into corn. Crop Sci. 56, 1245–1256. doi: 10.2135/cropsci2015.06.0351
Botha, J. J. (2006). Evaluation of maize and sunflower production in semiarid area using in field rainwater harvesting (Ph.D. thesis in Soil Science). University of the Free State, Bloemfontein, South Africa.
Botha, J. J., Anderson, J. J., and Van Staden, P. P. (2015). Rainwater harvesting and conservation tillage increase maize yields in South Africa. Water Resources Rural Dev. 6, 66–77. doi: 10.1016/j.wrr.2015.04.001
Botha, J. J., van Rensburg, L. D., Anderson, J. J., Hensley, M., Macheli, M. S., Van Staden, P. P., et al. (2003). Water Conservation Techniques on Small Plots in Semi-Arid Areas to Enhance Rainfall Use Efficiency, Food Security, and Sustainable Crop Production. Water Research Commission Report 1176/1/03, Pretoria, South Africa.
Cai, Z., Udawatta, R. P., Gantzer, C. J., Jose, S., Godsey, L., and Cartwright, L. (2019). Economic impacts of cover crops for a Missouri wheat-corn-soybean rotation. Agriculture 9, 83. doi: 10.3390/agriculture9040083
Chimungu, J. G. (2009). Comparison of field and laboratory measured hydraulic properties of selected diagnostic soil horizons (M.Sc. Thesis in Soil Science). University of the Free State, Bloemfontein, South Africa.
Connolly, J., Goma, H. C., and Rahim, K. (2001). The information content of indicators in intercropping research. Agric. Ecosyst. Environ. 87, 191–207. doi: 10.1016/S0167-8809(01)00278-X
Cook, C. G., Scott, A. W. Jr, and Chow, P. (1998). Planting date and cultivar effects on growth and stalk yield of sunn hemp. Indust. Crop. Prod. 8, 89–95. doi: 10.1016/S0926-6690(97)10013-9
Curran, W. S., Hoover, R. J., Mirsky, S. B., Roth, G. W., Ryan, M. R., Ackroyd, V. J., et al. (2018). Evaluation of cover crops drill interseeded into corn across the Mid-Atlantic region. Agron. J. 110, 435–443. doi: 10.2134/agronj2017.07.0395
de Oliveira Miranda, N., Morais de Sousa Vanomark, G. M., Costa e Melo, I. G., and Bezerra de Góes, G. (2020). Biomass of Crotalaria juncea as a function of plant densities in the semiarid region of North eastern Brazil. Agronomía Colombiana 38, 148–155. doi: 10.15446/agron.colomb.v38n1.78957
Devasenapathy, P. (2008). Efficiency Indices for Agriculture Management Research. New Delhi: New India Publishing. p. 162.
Dzvene, A. R., Tesfuhuney, W., Walker, S., Fourie, A., Botha, J. J., and Ceronio, G. (2021). Farmers' knowledge, attitudes, and perceptions for the adoption of in-field rainwater harvesting (IRWH) technique in Thaba Nchu, South Africa. Afr. J. Sci. Technol. Innov. Dev. 1–18. doi: 10.1080/20421338.2021.1960542
Fertilizer Society of South Africa (FSSA) (2007). FSSA Fertilizer Handbook. Fertilizer Society of South Africa, Pretoria.
Food Agriculture Organization of the United Nations (FAOSTAT) (2018). National Yield Statistics. Available online at: http://www.fao.org/faostat/en/#data/QC
Hensley, M., Bennie, A. T. P., Van Rensburg, L. D., and Botha, J. J. (2011). Review of 'plant available water' aspects of water use efficiency under irrigated and dryland conditions. Water SA 37, 771–780. doi: 10.4314/wsa.v37i5.14
Hensley, M., Botha, J. J., Anderson, J. J., van Staden, P. P., and du Toit, A. (2000). Optimising Rainfall Use Efficiency for Developing Farmers With Limited Access to Irrigation Water. Water Research Commission Report 878/1/00, Pretoria.
Jeranyama, P., Hesterman, O. B., Waddington, S. R., and Harwood, R. R. (2000). Relay-intercropping of sunn hemp and cowpea into a smallholder maize system in Zimbabwe. Agron. J. 92, 239–244. doi: 10.2134/agronj2000.922239x
Lawson, A., Cogger, C., Bary, A., and Fortuna, A. M. (2015). Influence of seeding ratio, planting date, and termination date on rye-hairy vetch cover crop mixture performance under organic management. PLoS ONE 10, 0129597. doi: 10.1371/journal.pone.0129597
Liu, G., Zuo, Y., Zhang, Q., Yang, L., Zhao, E., Liang, L., et al. (2018). Ridge-furrow with plastic film and straw mulch increases water availability and wheat production on the Loess Plateau. Sci. Rep. 8, 1–12. doi: 10.1038/s41598-018-24864-4
Mead, R., and Riley, J. (1981). A review of statistical ideas relevant to intercropping research. J. R. Stat. Soc. Ser. A 144, 462–487. doi: 10.2307/2981827
Mhlanga, B., Cheesman, S., Maasdorp, B., Mupangwa, W., Munyoro, C., Sithole, C., et al. (2016). Effects of relay cover crop planting date on their biomass and maize productivity in a sub-humid region of Zimbabwe under conservation agriculture. Netherlands J. Agric. Sci. Wageningen 78, 93–101. doi: 10.1016/j.njas.2016.05.001
Midega, C. A., Salifu, D., Bruce, T. J., Pittchar, J., Pickett, J. A., and Khan, Z. R. (2014). Cumulative effects and economic benefits of intercropping maize with food legumes on Striga hermonthica infestation. Field Crops Res. 155, 144–152. doi: 10.1016/j.fcr.2013.09.012
Mirsky, S. B., Curran, W. S., Mortenseny, D. M., Ryany, M. R., and Shumway, D. L. (2011). Timing of cover-crop management effects on weed suppression in no-till planted soybean using a roller-crimper. Weed Sci. 59, 380–389. doi: 10.1614/WS-D-10-00101.1
Mohammadi, G. R., and Ghobadi, M. E. (2010). The effects of different autumn-seeded cover crops on subsequent irrigated corn response to nitrogen fertilizer. Agric. Sci. 1, 148. doi: 10.4236/as.2010.13018
Morris, J. B., Chase, C., Treadwell, D., Koenig, R., Cho, A., Morales-Payan, J. P., et al. (2015). Effect of sunn hemp (Crotalaria juncea L.) cutting date and planting density on weed suppression in Georgia, USA. J. Environ. Sci. Health Part B 50, 614–621. doi: 10.1080/03601234.2015.1028855
Moseley, W. G. (1994). An equation for the replacement value of agroforestry. Agroforestry Syst. 26, 47–52. doi: 10.1007/BF00705151
Mosjidis, J. A., and Wehtje, G. (2011). Weed control in sunn hemp and its ability to suppress weed growth. Crop Prot. 30, 70–73. doi: 10.1016/j.cropro.2010.08.021
Murungu, F. S., Chiduza, C., and Muchaonyerwa, P. (2011). Effects of relay inter-cropping summer cover crops with maize on cover crop biomass and maize yields in a warm-temperate region of South Africa. South Afr. J. Plant Soil 28, 147–150. doi: 10.1080/02571862.2011.10640027
Mzezewa, J., Gwata, E. T., and Van Rensburg, L. D. (2011). Yield and seasonal water productivity of sunflower as affected by tillage and cropping systems under dryland conditions in the Limpopo Province of South Africa. Agric. Water Manage. 98, 1641–1648. doi: 10.1016/j.agwat.2011.06.003
Njoroge, R., Otinga, A. N., Okalebo, J. R., Pepela, M., and Merckx, R. (2018). Maize (Zea mays L.) response to secondary and micronutrients for profitable N, P and K fertilizer use in poorly responsive soils. Agronomy 8, 49. doi: 10.3390/agronomy8040049
Nyakudya, I. W., and Stroosnijder, L. (2011). Water management options based on rainfall analysis for rainfed maize (Zea mays L.) production in Rushinga district, Zimbabwe. Agric. Water Manage. 98, 1649–1659. doi: 10.1016/j.agwat.2011.06.002
Oweis, T. Y., and Taimeh, A. Y. (1996). Evaluation of a small basin water-harvesting system in the arid region of Jordan. Water Resources Manage. 10, 21–34. doi: 10.1007/BF00698809
Parenti, A., Cappelli, G., Zegada-Lizarazu, W., Sastre, C. M., Christou, M., Monti, A., et al. (2021). SunnGro: a new crop model for the simulation of sunn hemp (Crotalaria juncea L.) grown under alternative management practices. Biomass Bioenergy 146, 105975. doi: 10.1016/j.biombioe.2021.105975
Ponce, C. (2020). Intra-seasonal climate variability and crop diversification strategies in the Peruvian Andes: a word of caution on the sustainability of adaptation to climate change. World Dev. 127, 104740. doi: 10.1016/j.worlddev.2019.104740
Qi, A., Wheeler, T. R., Keatinge, J. D. H., Ellis, R. H., Summerfield, R. J., and Craufurd, P. Q. (1999). Modelling the effects of temperature on the rates of seedling emergence and leaf appearance in legume cover crops. Exp. Agric. 35, 327–344. doi: 10.1017/S0014479799003099
Ritchie, S. W., Hanway, J. J., Benson, G. O., and Herman, J. C. (1993). How A Corn Plant Develops, Special Report No. 48, Iowa State University. Ames, IW: Science and Technology Cooperative Extension Service.
Ruffatti, M. D., Roth, R. T., Lacey, C. G., and Armstrong, S. D. (2019). Impacts of nitrogen application timing and cover crop inclusion on subsurface drainage water quality. Agric. Water Manage. 211, 81–88. doi: 10.1016/j.agwat.2018.09.016
Salem, H. M., Valero, C., Muñoz, M. Á., and Gil-Rodríguez, M. (2015). Effect of integrated reservoir tillage for in-situ rainwater harvesting and other tillage practices on soil physical properties. Soil Tillage Res. 151, 50–60. doi: 10.1016/j.still.2015.02.009
Schomberg, H. H., Fisher, D. S., Reeves, D. W., Endale, D. M., Raper, R. L., Jayaratne, K. S. U., et al. (2014). Grazing winter rye cover crop in a cotton no-till system: yield and economics. Agron. J. 106, 1041–1050. doi: 10.2134/agronj13.0434
Sharma, A. R., Singh, R., Dhyani, S. K., and Dube, R. K. (2010). Moisture conservation and nitrogen recycling through legume mulching in maize (Zea mays)-wheat (Triticum aestivum) cropping system. Nutrient Cycling Agroecosyst. 87, 187–197. doi: 10.1007/s10705-009-9327-y
Singh, R. J., Ahlawat, I. P. S., and Sharma, N. K. (2015). Resource use efficiency of transgenic cotton and peanut intercropping system using modified fertilization technique. Int. J. Plant Prod. 9, 523–540. doi: 10.22069/IJPP.2015.2461
Soil Classification Working Group. (1991). Soil Classification - A Taxonomic System for South Africa. Soil and Irrigation Research Institute, Department of Agricultural Development, Pretoria, South Africa
Subrahmaniyan, K., Veeramani, P., and Zhou, W. J. (2021). Does heat accumulation alter crop phenology, fibre yield and fibre properties of sunnhemp (Crotalaria juncea L.) genotypes with changing seasons? J. Integr. Agric. 20, 2395–2409. doi: 10.1016/S2095-3119(20)63357-3
Tabor, J. A. (1995). Improving crop yields in the Sahel by means of water-harvesting. J. Arid Environ. 30, 83–106. doi: 10.1016/S0140-1963(95)80041-7
Tesfuhuney, W. A., Van Rensburg, L. D., Walker, S., and Allemann, J. (2015). Quantifying and predicting soil water evaporation as influenced by runoff strip lengths and mulch cover. Agric. Water Manage. 152, 7–16. doi: 10.1016/j.agwat.2014.11.018
Van Ittersum, M. K., Van Bussel, L. G., Wolf, J., Grassini, P., Van Wart, J., Guilpart, N., et al. (2016). Can sub-Saharan Africa feed itself? Proc. Nat. Acad. Sci. U.S.A. 113, 14964–14969. doi: 10.1073/pnas.1610359113
Wang, H., Zhang, X., Yu, X., Hou, H., Fang, Y., and Ma, Y. (2018). Maize-fababean rotation under double ridge and furrows with plastic mulching alleviates soil water depletion. Agric. Water Manage. 207, 59–66. doi: 10.1016/j.agwat.2018.04.033
Wilson, M. L., Baker, J. M., and Allan, D. L. (2013). Factors affecting successful establishment of aerially seeded winter rye. Agron. J. 105, 1868–1877. doi: 10.2134/agronj2013.0133
World Reference Base for Soil Resources (WRB) (2006). A Framework for International Classification, Correlation and Communication. World Soil Resources Report 103. IUSS/ISRIC/FAO. Available online at: http://www.fao.org/3/a0510e/A0510E.pdf
Zougmoré, R., Zida, Z., and Kambou, N. F. (2003). Role of nutrient amendments in the success of half-moon soil and water conservation practice in semiarid Burkina Faso. Soil Tillage Res. 71, 143–149. doi: 10.1016/S0167-1987(03)00050-3
Appendix
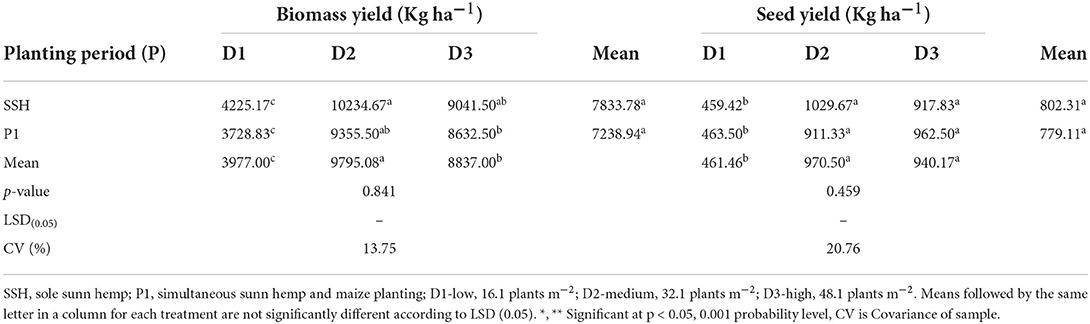
Appendix A1. Effects of planting density on biomass and seed yield of sunn hemp at maturity harvest under the in-field rainwater harvesting technique in the central semiarid area of the Free State Province, South Africa.
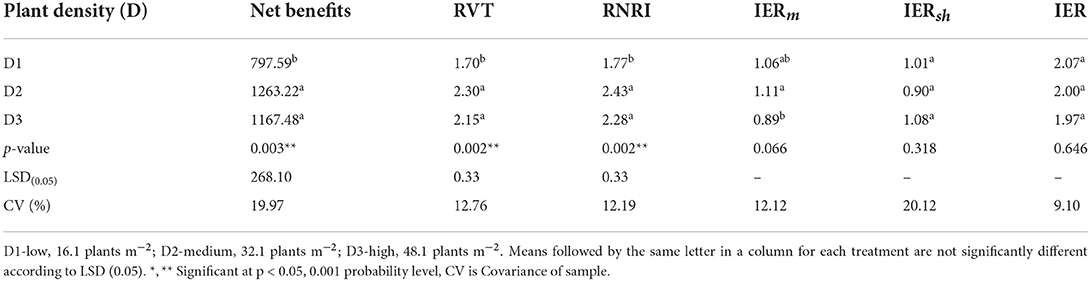
Appendix A2. Effects of planting density on net benefits (NB), replacement value of sun hemp (RVsh), relative net return index (RNRI), maize income equivalent ratio (IERm), sunn hemp income equivalent ratio (IERsh), and income equivalent ratio (IER) at sunn hemp maturity harvest under the in-field rainwater harvesting technique in the central semiarid area of the Free State Province, South Africa.
Keywords: maize grain yield, biomass, planting period, plant density, income equivalent ratio, net benefits, living mulch
Citation: Dzvene AR, Tesfuhuney W, Walker S and Ceronio G (2022) Effects of intercropping sunn hemp into maize at different times and densities on productivity under rainwater harvesting technique. Front. Sustain. Food Syst. 6:1009443. doi: 10.3389/fsufs.2022.1009443
Received: 01 August 2022; Accepted: 12 September 2022;
Published: 04 October 2022.
Edited by:
Cosmas Parwada, Zimbabwe Open University, ZimbabweReviewed by:
Shimelis Raji, Hawassa University, EthiopiaMisheck Musokwa, University of KwaZulu-Natal, South Africa
Copyright © 2022 Dzvene, Tesfuhuney, Walker and Ceronio. This is an open-access article distributed under the terms of the Creative Commons Attribution License (CC BY). The use, distribution or reproduction in other forums is permitted, provided the original author(s) and the copyright owner(s) are credited and that the original publication in this journal is cited, in accordance with accepted academic practice. No use, distribution or reproduction is permitted which does not comply with these terms.
*Correspondence: Admire R. Dzvene, dzvenea@gmail.com