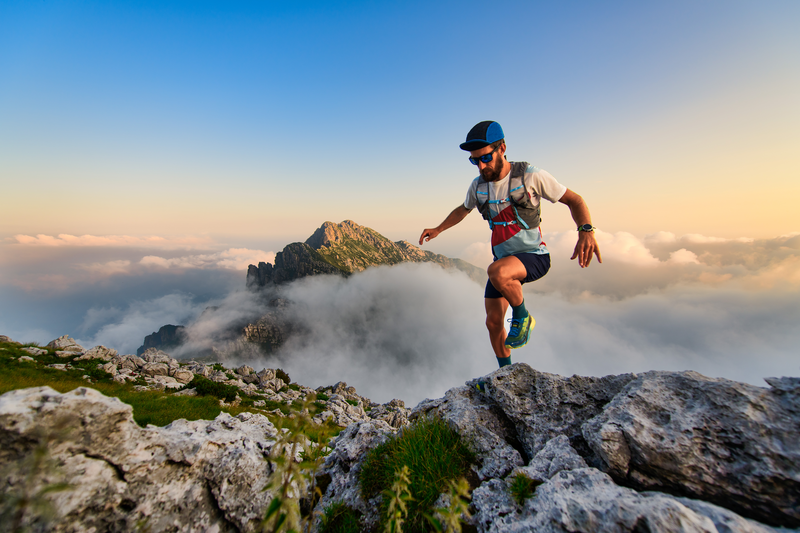
94% of researchers rate our articles as excellent or good
Learn more about the work of our research integrity team to safeguard the quality of each article we publish.
Find out more
REVIEW article
Front. Sustain. Food Syst. , 04 November 2022
Sec. Nutrition and Sustainable Diets
Volume 6 - 2022 | https://doi.org/10.3389/fsufs.2022.1005058
This article is part of the Research Topic Microbial Biomass as a Sustainable Source of Nutritional and Environmental Benefits View all 4 articles
Honey bees are essential agricultural pollinators that are threatened by various interacting stressors, posing risks to beekeeping industries and human food security. Malnutrition is a major factor underlying managed bee colony losses that can be countered by feeding artificial diets, which aim to deliver essential macro- and micronutrients. Current bee nutritional supplements show room for improvement and require resources that compete with human food production. Algae and microalgae in particular have been gaining traction in the literature as alternative feed sources and nutritional supplements for livestock, including honey bees. Herein, we review the current literature and categorize the effects of algae supplementation on honey bee colony productivity as well as effects on individual bee physiology and health. In general, we conclude that algae biomass appears to be suitable for use as a bee feed additive and as a source of health-stimulating natural products. Additionally, we suggest research areas that could improve the development of sustainable algae-based nutrition supplements for honey bees.
Honey bees (Apis mellifera) play a major economic role in the modern world and are a vital aspect of human food security (Khalifa et al., 2021). Managed bee colonies contribute to 35% of global food production, while their services and products are a multibillion-dollar global industry, providing economic benefits to agricultural, food production, and medicinal sectors (Klein et al., 2006; van der Sluijs and Vaage, 2016). However, honey bees are currently facing high colony mortality rates, habitat loss, and climate change, which threaten their populations and the services they provide to humans (Potts et al., 2010). As such, modern beekeeping is challenged to overcome factors such as changing weather patterns, lack of forage, reduced forage nutritional diversity, parasites, pathogens, and pesticides (Potts et al., 2010; Vaudo et al., 2015; Dolezal and Toth, 2018). Managed bee colonies require constant human inputs in the form of disease control and supplemental nutrition. Artificial diets and feed supplements from a variety of plant and animal products show mixed results in their capacity to support colony growth and productivity (Noordyke and Ellis, 2021; Paray et al., 2021; Tsuruda et al., 2021). The current research focused on artificial diets indicates that there are opportunities for improving bee feed to serve the growing demands of beekeepers who have become increasingly reliant on supplemental nutrition (Ricigliano et al., 2022b). Importantly, given the challenges of feeding the world's human population, sustainable ingredients that do not compete with human food production are excellent candidates to address this crucial need of modern beekeeping. Algae biomass shows promise as an alternative feed source as well as a source of health-modulating natural products for honey bees (Ricigliano and Simone-Finstrom, 2020). Due to their nutrition content, sustainability, and amenability to trait manipulation, algae could potentially be developed into novel nutritional supplements that can bolster bee populations in the face of current stressors (Oswald et al., 1967; Stengel et al., 2011; Vigani et al., 2015; Piwowar and Harasym, 2020; Ricigliano, 2020; Xing et al., 2021). This review first provides an overview of honey bee nutrition and nutritional supplementation, then examines current literature concerning the effects of algae and associated products on honey bee colony productivity and bee physiology.
Nutritional needs for honey bees are provided primarily by floral nectar and pollen. Nectar acts as the main carbohydrate source for bees (Wright et al., 2018; Tsuruda et al., 2021). Pollen is the primary source of macronutrients for honey bees, providing protein, essential amino acids, and lipids (Brodschneider and Crailsheim, 2010; Wright et al., 2018; Tsuruda et al., 2021). At the colony level, pollen consumption is dependent on the size of the colony, with some estimates of pollen consumption approaching 57 kg per year to provide sufficient protein for a colony of 20,000 individuals (Tsuruda et al., 2021). Honey bee colonies function as a “common stomach” in which nutritional deficiencies stimulate foraging workers to gather nectar and pollen (Schmickl and Karsai, 2017; Wright et al., 2018; Tsuruda et al., 2021). Once stored in the hive, pollen is consumed by nurse bees who digest and assimilate nutrition, producing a protein- and lipid-rich glandular secretions (i.e., jelly proteins) that are used to rear larvae and feed other bees (Crailsheim et al., 1992). Since honey bees cannot synthesize arginine, histidine, lysine, tryptophan, phenylalanine, methionine, threonine, leucine, isoleucine, and valine, these amino acids must be present in the diet (De Groot, 1953). Amino acids supplied in free form appear to shorten bee lifespan and are therefore best delivered in the form of crude protein from pollen or feed with an amino acid profile that recapitulates jelly proteins (Wright et al., 2018). Lipids can comprise as little as 2% to as much as 60% of the weight of the pollen (Roulston and Cane, 2000; Roulston et al., 2000). Essential fatty acids, such as linoleic acid, γ-linoleic α-linoleic acid, and palmitic acid, are important lipid constituents that can comprise up to 80% of the lipid content (Wright et al., 2018). Phytosterols from pollen are also essential for physiological functions in bees (Chakrabarti et al., 2020). The health benefits of dietary lipids are impacted by the ratios in which they occur relative to other nutrients. While typically balanced in pollen, a 10:1 protein to lipid content ratio and a low linoleic acid: α-linoleic acid ratio is optimal for bee health, with higher ratios and concentrations of certain fatty acids causing increased mortality (Ma et al., 2015; Wright et al., 2018; Tsuruda et al., 2021). Honey bees must maintain proper nutrition due to interacting stressors, notably parasites and pathogens (Dolezal and Toth, 2018). This complex of nutritional and pathogenic stressors can impair immune function, retard development, and reduce bee lifespans, which can quickly destroy entire colonies (Dolezal and Toth, 2018; Ramsey et al., 2019). Nutritional stresses can also lower disease resistance by impacting the diversity and function of essential gut microbiota (Castelli et al., 2020). Five species clusters of bacteria consisting of Snodgrassella alvi, Gilliamella apicola, two species of Lactobacillus sp., and Bifidobacterium sp. are considered core to the honey bee gut microbiota (Raymann and Moran, 2018). These bacteria influence honey bee nutrient utilization, immunity, development, hormone signaling, and behavior (Engel et al., 2012; Moran, 2015; Raymann and Moran, 2018). While some physiological effects in correlation with microbiota populations have been reported, the complex interplay among other environmental factors necessitates further studies to understand the full impact of nutrition on bee gut microbiota (Engel et al., 2016; Raymann and Moran, 2018).
Beekeepers feed managed colonies artificial “pollen substitute” diets to compensate for periods of inadequate pollen forage and to increase the size of colonies before their use in pollination services (Johansson and Johansson, 1977; Ricigliano et al., 2022b). A variety of commercial feed products and diet formulations employ different components as partial or full replacement for natural pollen. Artificial diets incorporate protein-rich ingredients such as soy, yeast, pea, milk proteins, or eggs (Paray et al., 2021). Some diets may also include a fraction of bee-collected pollen, which can increase consumption and brood rearing (Ricigliano et al., 2022a). Generally, bees have a strong preference for natural pollen from diverse sources and will consume pollen before consuming artificial diets (Herbert et al., 1980; Requier et al., 2015; Wright et al., 2018). Therefore, feed is typically given during dearth periods such as during winter (Manning, 2018; Tsuruda et al., 2021). Soy tends to be used the most often due to the availability and cheap cost of the product, however, soy contains anti-nutritional factors such as protease inhibitors and toxic sugars (Ricigliano, 2020; Noordyke and Ellis, 2021; Paray et al., 2021). Additionally, soy-based products may be unpalatable having been reported as being consumed at lower levels than soy-free substitutes and accumulating at higher levels among hive debris compared to natural pollen diets (Ellis and Hayes, 2009; Saffari et al., 2010; Noordyke and Ellis, 2021). Common animal products such as egg and whey proteins have shown some benefits as a protein substitute; however, their efficiency varies and there are environmental concerns with these protein sources (Eshel et al., 2014; Paray et al., 2021). The impact of feed ingredients varies due to factors such as environment, season, laboratory vs. field conditions, and formulation (Manning, 2018; Tsuruda et al., 2021). As such, an all-encompassing artificial diet that meets the nutritional requirements of bees under diverse management conditions has yet to be developed (Manning, 2018; Wright et al., 2018; Noordyke and Ellis, 2021).
Algae are a rich source of carbohydrates, proteins, lipids, vitamins, minerals, and amino acids (Yaakob et al., 2014; Singh et al., 2017; Khan et al., 2018). Macroalgae can be seen with the naked eye, whereas microalgae require the use of an optical device to see individual organisms (Suganya et al., 2016; Pereira, 2021). There are over 40,000 algae species from two domains that are broadly divided into prokaryotic unicellular cyanobacteria microalgae (blue-green algae), and eukaryotic unicellular/multicellular algae (green algae, red algae, and brown algae) (Yaakob et al., 2014; Suganya et al., 2016; Khan et al., 2018; Pereira, 2021; Ullmann and Grimm, 2021). Microalgae are particularly efficient at producing large quantities of complete proteins, polyunsaturated fatty acids (PUFAS), and other important nutritional biomolecules (Yaakob et al., 2014; Singh et al., 2017; Ullmann and Grimm, 2021). Delivered either as a whole cell powder or as extracts, algae and algae products are welldocumented to have multiple beneficial effects on livestock, which are evident in mammals, birds, and fish (Yaakob et al., 2014; Suganya et al., 2016; Andrade et al., 2018; Khan et al., 2018; Araújo et al., 2021). Nutrition is also important to the ability of an organism to resist pathogens and their associated diseases. A wide variety of biomolecules occurring in algae have shown promise for supporting disease resistance in animals (Yaakob et al., 2014; Suganya et al., 2016; Singh et al., 2017; Andrade et al., 2018; Khan et al., 2018). Algae are a rich source of carotenoids such as astaxanthin as well as polyunsaturated fatty acids such as docosahexaenoic acid, both of which modulate animal stress responses (Yaakob et al., 2014; Suganya et al., 2016; Singh et al., 2017; Khan et al., 2018). In general, research indicates that algal biomass and extracts can bolster immune function, general stress resistance, and survival in livestock and aquaculture stocks (Madeira et al., 2017; Saadaoui et al., 2021; Valente et al., 2021). Though the benefits of microalgae to insects are understudied compared to other livestock, the available literature indicates benefits to insect health, production, growth, and reproduction (Truzzi et al., 2020). In recent decades, microalgae production techniques have been developed to allow for the efficient production of different species (Singh and Sharma, 2012; Araújo et al., 2021; Ullmann and Grimm, 2021). Today the most commonly produced microalgae are the green algae in the division Chlorophyta, and blue-green cyanobacteria in the genus Arthrospira, commonly called spirulina (Andrade et al., 2018; Araújo et al., 2021). Modern technologies enable the mass culturing of microalgae under a variety of conditions for biomass production and wastewater treatment, thus enabling different industries to use algae as a sustainable resource (Singh and Sharma, 2012; Suganya et al., 2016; Khan et al., 2018; Wollmann et al., 2019; Piwowar and Harasym, 2020; Araújo et al., 2021).
Literature related to the effects of algae on honey bees was obtained via database searches between May 2019 and August 2022. Databases used included Google Scholar, EBSCOhost, Scopus, and the University of Nebraska Lincoln's database search engine. Key terms used in the searches included “algae”, “bees”, “honey bees”, “apis”, “chlorella”, and “spirulina”. Search results were characterized based on bee colony productivity and health, individual bee physiology, and disease resistance, algae species used, and delivery methods (Supplementary Table 1). A total of 35 pieces of literature were identified, which comprised journal articles, agricultural publications, university publications, patents, and consortiums. A total of 24 literature pieces were used in this review. The earliest publications relating to algae and honey bees were published in 1976 and 1977. There were no publications in the 1980s, one publication in the 1990s, and three publications in the 2000s. There were sixteen publications in the 2010s and 13 publications between January 2020 and August 2022. Most of the literature was published in Eastern Europe with eleven publications from Moldova, four from Russia, three from the Czech Republic, and two from Ukraine. Five publications were from the United States, two from China, and one each from France, India, Turkey, Saudi Arabia, Egypt, Sweden, Germany, and Italy. A total of 24 different algae varieties were reported. Algae species varied across microalgae and macroalgae; however, most of the literature is focused on microalgae. The algae spanned different genera with the majority belonging to Arthrospira (spirulina) and Chlorella. The green microalgae Oocystis borgei Snow and Scenedesmus quadricauda each were reported twice in the literature. All other named species were reported only once. The remaining literature did not provide species names and only provided the genus (Supplementary Table 1). The cyanobacterium Arthrospira platensis (spirulina) was used in most publications followed by green microalgae belonging to the genus Chlorella. Algae delivery methods varied in the reviewed literature and were administered to colonies or caged bees as whole-cell powders or extracts. Formulations included pastes, powders, patties, candies, or liquid supplements (Supplementary Table 1).
The available literature indicates that when fed with algae products, honey bees appear to benefit at the colony level in their fecundity, brood rearing, colony population size, and production of honey and wax. This was reflected across multiple countries and environments.
The addition of microalgae biomass or supplements in nutritional sources increased egg-laying rates in honey bee queens. The egg laying rate increased in hives supplemented with Oocystis borgei Snow when compared to controls fed sugar paste. Queens from hives supplemented with Oocystis borgei Snow experienced an 8.3% increase in 24-h egg laying rate compared to controls, and a 6.6% increase relative to hives fed sugar paste with added spirulina (Cebatori et al., 2017). Queens from hives fed sugar paste containing Scenedesmus quadricauda exhibited a 7.8% increase in egg laying compared to controls fed sugar paste, and a 6.2% increase relative to hives fed spirulina paste (Cebatori et al., 2016). Hives fed sugar syrup supplemented with Chlorella vulgaris had an increase in queen egg laying rates when compared to controls fed honey or sugar syrup. Experimental hives showed a 6.4% increase in 24-h egg laying rate relative to unfed controls, and a 12.7% increase compared to controls fed sugar syrup (Eremia et al., 2013). Queens from hives fed sugar syrup with spirulina extracts had a 44.7% higher 24-h egg laying rate relative to hives fed only sugar (Toderaş et al., 2014). Queens from hives fed algae pastes containing Scenedesmus quadricauda, Scenedesmus apiculatus, and Oocystis borgei Snow expressed an increased 24-h egg laying rate by 8.8% compared to controls fed honey and powdered sugar and an increase of 7.1% relative to hives fed pastes containing spirulina (Toderaş et al., 2017). The reported increases in queen fecundity are in agreement with reports of increased capped brood and adult bee populations (Brood production and viability and Colony population sizes sections). Queens from algae-fed hives fed algae-produced mated queens that were 7.3% heavier than control hives fed honey. Interestingly, bees-fed algae suspensions produced significantly larger queen cells compared to controls (Eremia et al., 2012).
Algae supplementation has been shown to increase honey bee colony brood production. The five articles reporting increased queen fecundity in hives supplemented with algae (Queen fecundity section) reported correlations in increased capped brood numbers and brood viability. Hives fed with sugar paste containing Oocystis borgei Snow exhibited increased capped brood and brood viability compared to hives fed sugar paste or sugar paste supplemented with spirulina. Experimental hives showed a significant increase of 8% in capped brood and an increase of 1.5% in brood viability relative to controls. Experimental hives also experienced an in an increase of 6.5% in capped brood compared to hives-fed paste containing spirulina (Cebatori et al., 2017). Colonies fed a paste of Scenedesmus quadricauda experienced a significant increase in capped brood and brood viability. Scenedesmus-fed hives exhibited an increase of 7.7% in capped brood and 1.1% in brood viability compared to controls (Cebatori et al., 2016). Hives fed sugar syrup containing Chlorella vulgaris showed a significant increase in capped brood compared to unfed hives and controls fed sugar syrup (Eremia et al., 2013). Hives fed sugar syrup containing spirulina extracts were observed to have 44.9% more brood than control hives fed sugar, and brood was on average 14% more viable than brood from control hives (Toderaş et al., 2014). Hives fed sugar pastes supplemented with Scenedesmus quadricauda, Scenedesmus apiculatus, and Oocystis borgei Snow had significantly more brood, averaging at 8.3% more than control and averaging 6.8% more brood than control hives fed sugar paste with spirulina. The same study reported that hives supplemented with Spirulina platensis, Scenedesmus quadricauda, and Scenedesmus apiculatus had a significant increase in brood viability, averaging 1.4% higher than control hives (Toderaş et al., 2017). This increase in total brood and brood viability correlated with increased colony sizes in hives-fed algae supplements (Colony population sizes section). Similar increases in brood production in algae-fed hives were reported in other studies. Hives fed a paste of Chlorella sorokiniana showed a significant increase in spring brood rearing when compared to controls fed sugar. Algae-supplemented hives experienced a two-fold increase in capped brood area when compared to control hives. Chlorella spp. applied directly as a paste led to a 44.4% increase in brood area compared to hives fed with Chlorella-supplemented candies (Jehlík et al., 2019). Colonies fed during dearth periods with patties or honey-containing spirulina were observed to have a 54.2% increase in total brood area relative to unfed controls (Kumar et al., 2013). Bee colonies infested with Varroa destructor mites and treated with algae filtrates had higher levels of capped brood compared to controls. Colonies treated with filtrates of Chroococcus minutus, Calothrix parietina, and Gloeocapsa giganteus featured an average increase of 45.3% more brood compared to untreated controls. Colonies treated with filtrates of Chroococcus minutus and Gloeocapsa giganteus had an 8.6% and 3.8% respective increase in brood area compared to controls treated with oxalic acid (Hassan and Mahmoud, 2006).
In agreement with increased queen fecundity (Queen fecundity section) and brood production (Brood production and viability section), honey bee colonies treated with algae-based supplements had increased colony population sizes. Colonies fed paste of Oocystis borgei Snow exhibited a 7.1 and 8.1% increase in population size relative to controls fed sugar paste or paste supplemented with spirulina, respectively (Cebatori et al., 2017). Colonies fed paste containing Scenedesmus quadricauda had a 9.3 and 8.3% increase in colony population size compared to hives fed sugar paste or supplemented with spirulina, respectively (Cebatori et al., 2016). Hives supplemented with Chlorella vulgaris exhibited a 10.7% increase in population size relative to unfed controls and hives fed sugar syrup (Eremia et al., 2013). Hives fed spirulina extracts featured on average 17.5% larger populations than control hives (Toderaş et al., 2014). Hives-fed paste containing Scenedesmus quadricauda, Scenedesmus apiculatus, and Oocystis borgei Snow featured an average population increase of 8.2% compared to controls, and a 7.2% increase compared to hives fed spirulina paste (Toderaş et al., 2017).
Overall, the reviewed literature reported increased honey production in hives supplemented with algae products. Hives-fed paste containing Oocystis borgei Snow featured a 38.7% increase in honey production compared to controls (Cebatori et al., 2017). Similarly, hives fed extracts of Scenedesmus quadricauda showed a 27.6% increase in honey production relative to controls (Cebatori et al., 2016). Hives fed pastes of Spirulina plantensis, Scenedesmus quadricauda, Scenedesmus apiculatus, and Oocystis borgei Snow increased in honey production by up to 28.8% compared to controls fed honey and powdered sugar (Toderaş et al., 2017). Hives supplemented with Chlorella vulgaris exhibited a 17.2% increase in honey production relative to control hives (Eremia et al., 2013). Honey production increased by up to 69.1% in hives supplemented with suspensions of Chroococcus minutus, Calothrix parietina, and Gloeocapsa giganteus relative to controls (Hassan and Mahmoud, 2006). Honey production increased by 12.1% in hives fed Chlorella in sugar syrup suspension (Eremia et al., 2021). Hives fed a 10% spirulina solution featured increased antioxidant activities and fatty acid content in the honey produced compared to honey from control hives (Guldas et al., 2022). While this is a promising finding with implications for human nutrition, further studies are necessary to ensure that honey produced under these conditions is suitable for human consumption. Nevertheless, spirulina is typically produced for use in human food products and supplements. Wax production was also observed to increase when hives were supplemented with algae biomass. Hives-fed sugar syrup containing spirulina extracts featured 39.7% higher wax production compared to controls fed sugar (Toderaş et al., 2014). Total wax production increased on average by 20.8% when hives were fed algae pastes of Spirulina plantensis, Scenedesmus quadricauda, Scenedesmus apiculatus, and Oocystis borgei Snow compared to controls (Toderaş et al., 2017). Colonies fed pastes of Oocystis borgei Snow exhibited a 27.9% increase in wax production compared to controls (Cebatori et al., 2017). Experimental hives supplemented with sugar paste containing Scenedesmus quadricauda increased wax production by 11.5% compared to control hives (Cebatori et al., 2016).
The literature indicates that algae-based feed supplements and extracts applied either directly to colonies or to pathogens and pests have the potential to inhibit disease growth or infestations when compared to different controls. Experimental hives supplemented with Oocystis borgei Snow exhibited increased hygienic behavior, an indicator of disease resistance potential, when compared to the control hives (Cebatori et al., 2017). Similarly, hives fed extracts of Scenedesmus quadricauda showed increased hygienic behavior when compared to controls (Cebatori et al., 2016). Experimental hives supplemented with Spirulina platensis, Scenedesmus quadricauda, Scenedesmus apiculatus, and Oocystis borgei Snow had a 2.7% increase in hygienic behavior to the control hives fed with honey and powdered sugar (Toderaş et al., 2017). Four studies reported similar observations of disease resistance when pathogens or their honey bee hosts were directly or indirectly exposed to algae products. Hives infested with Varroa destructor mites and fed algae had lower infestation rates compared to untreated control hives and hives treated with oxalic acid. The average mite infestation rate was 2.7% when fed isolates of Chroococcus minutus, Calothrix parietina, and Gloeocapsa giganteus compared to untreated control hives with an infestation rate of 26.26% and oxalic acid-treated hives with an infestation rate of 2.9% (Hassan and Mahmoud, 2006). Honey bees infected with Nosema ceranae and fed Porphyridium marinum and Porphyridium purpureum extracts had lower parasite loads compared to controls. Experimental bees expressed up to a 30% decrease in Nosema loads relative to unfed controls and at half the Nosema load of the individuals treated with fumagillin (Roussel et al., 2015). Extracts of Chlorella sorokiniana had anti-microbial effects on plate cultures of Paeniebacillius larvae bacteria (Vránová, 2017), and similar antimicrobial effects were observed when applying Chlorella vulgaris extracts to spores and vegetative state cultures of Paenibacillus larvae bacteria (Dostálková et al., 2021).
The literature reports a number of different physiological impacts on individual bees fed algae-based nutritional supplements. Thorax and fat body masses of bees fed spirulina paste matched that of pollen-fed bees (Ricigliano and Simone-Finstrom, 2020). Similarly, bees fed spirulina had head protein concentrations that matched pollen-fed bees but were lower than bees fed a commercial pollen substitute (Ricigliano and Simone-Finstrom, 2020). In a different study, spirulina-fed workers met or exceed the body masses of pollen-fed workers across two different genetic lines of honey bees. Furthermore, bees supplemented with spirulina had a significant increase in mRNA expression levels of the nutritional storage lipoprotein Vitellogenin (Ricigliano et al., 2021). In another study, bees fed sugar pastes of Chlorella vulgaris or spirulina exhibited similar growth characteristics as bees fed natural pollen (Ricigliano et al., 2022a). Bees fed algae expressed higher mRNA levels of Vitellogenin compared to sugar-fed controls, whereas Chlorella fed-bees featured Vitellogenin mRNA levels trending higher, but not statistically different than pollen-fed bees. Furthermore, bees fed both algae types featured higher levels of antioxidant gene transcripts and spirulina-fed bees expressed higher transcript levels of shock protein 90 (Ricigliano et al., 2022a). Increased body and tissue masses and nutrient levels were observed in bees fed Chlorella sorokiniana relative to bees from control hives fed sugar. Average midgut mass increased 1.5-fold in bees from algae-fed hives relative to control hives and bees had larger hypopharyngeal glands when fed Chlorella sorokiniana. In the same study adult bees fed Chlorella sorokiniana featured a 1.7-fold higher fat body lipid content and a 5.6-fold increase in larval fat body lipids. Furthermore, bees fed Chlorella sorokiniana showed increased concentrations of lineic acid relative to control bees whereas fat body glycogen concentrations decreased 3.8- and 1.5-fold in workers and larvae, respectively. Finally, bees fed Chlorella sorokiniana had significantly higher levels of Vitellogenin mRNA transcripts and lower levels of TOR and InR2 mRNA transcripts relative to controls (Jehlík et al., 2019). This study is in agreement with a similar study that tested the impacts of Chlorella sorokiniana. Bees fed diets supplemented with 0.5–10% Chlorella sorokiniana exhibited increased hypopharyngeal gland development, higher amino acid content, and higher levels of Vitellogenin mRNA expression (Jang et al., 2022).
Gut microbiota changes were observed in honey bee colonies sprayed with a commercial supplement containing seaweed extracts. Bees sprayed with a mixture of sugar syrup and seaweed extracts showed a 13% increase in gut abundance of Bartonella spp. after 1 month. Furthermore, the product containing seaweed extracts also led to a 6.8% decrease in gut Bombilactobacillus spp. which was correlated with a significant decrease in the species Bombilactobacillus mellis. In the same study, bees supplemented with a commercial probiotic exhibited a significantly higher abundance of total Lactobacillaceae spp. when compared with bees sprayed with the seaweed supplement (Alberoni et al., 2021). Bees fed spirulina were observed to have comparable levels of beneficial gut bacteria to bees fed natural pollen diets. Spirulina-fed bees had similar abundances of Lactobacillus as bees fed natural pollen and had higher abundances of Lactobacillus relative to bees fed commercial pollen substitute or sugar. Furthermore, bees fed spirulina had higher abundances of Bifidobacterium than bees fed pollen substitute or sugar. However, average Bifidobacterium abundance was higher in bees fed natural pollen relative to bees fed spirulina. Bees fed spirulina also had on average higher abundances of Snodgrassella relative to bees fed sugar, pollen substitute, or pollen (Ricigliano and Simone-Finstrom, 2020). Interestingly, Cyanobacteria spp. were observed to compromise 1.83% of the total gut microbiome of foraging bees collected during the beginning of the overwintering period (Liu et al., 2021). While not core constituents of the gut microbiome, cyanobacteria are present in “dirty” or turbid water sources that are attractive to foraging honey bees (Butler, 1940). Population changes in gut microbiota and other associated variables are still a relatively new field of study (Zheng et al., 2018). More research is necessary to understand the impacts of algae products on honey bee gut microbiota.
The literature contains mixed reports pertaining to the effects of different algae species on bee mortality. Bees fed with water containing Anabaena flos-aquae were observed to have no difference in mortality rates compared to the controls provided distilled water (Barker, 1977). Mortality rates of bees fed Chlorella sorokiniana was observed to be no different from mortality rates of controls fed sugar (Jehlík et al., 2019). Bees fed spirulina were observed to have negligible mortality rates compared to pollen- and sugar-fed controls after 10 days of feeding (Ricigliano et al., 2021). In another study, bees fed spirulina had a negligible difference in mortality rates compared to controls fed sugar, pollen, or pollen substitute (Ricigliano and Simone-Finstrom, 2020). In some instances, algae supplementation increased survival. Honey bees infected with the gut pathogen Nosema ceranae had increased survival rates when fed polysaccharides extracted from the algae Porphyridium marinum relative to untreated and fumagillin-treated controls (Roussel et al., 2015). Colonies fed sugar syrup containing Chlorella in the fall had a 20.8% increase in overwinter survival relative to controls, and a 5.7% increase in survival compared relative to controls fed a commercial feed additive (Eremia et al., 2021). Caged bees fed sugar syrup with different concentrations of Chlorella vulgaris suspension had significantly lower mortality rates than bees fed only sugar syrup (Yefimenko and Odnosum, 2020). Bees fed pollen patties containing 2 or 5% Chlorella had the lowest mortality rate compared to other tested diets (Jang et al., 2022). Some literature indicates that algae consumption increases mortality rates and could cause detrimental physiological effects. Bees fed pollen, pollen substitute, and date paste were observed to have higher mortality rates when different concentrations of spirulina were added to the diets (Khan and Ghramh, 2022). Honey bee larvae reared under laboratory conditions and directly fed a diet containing Chlorella vulgaris extracts showed a significant increase in mortality relative to controls fed a standard larval diet. In the study, larvae fed Chlorella vulgaris extracts had an average mortality rate that was 31.3% higher than controls. Here, the authors argue that this may be due to the direct feeding of the algae to larvae instead of prior digestion from nurse bees (Dostálková et al., 2021). Bees fed sugar water containing the cyanobacterial toxin, beta-N-methylamino-L-alanine (BMAA) showed a significant increase in mortality relative to controls. In the same study, bees exposed to BMAA retained the toxins 72 h after ingestion and spread toxins via trophallaxis. Furthermore, the brains of bees exposed to BMAA showed a 1.8-fold increase in reactive oxygen species, had significantly higher levels of intracellular Ca2+ ions within the neuron cells, and had higher levels of spontaneous activity. Finally, bees exposed to BMAA also expressed a significant decrease in operant conditioning learning to odor stimuli after 5 days (Okle et al., 2013).
Overall, current research indicates that algae biomass and extracts can provide numerous benefits to honey bees. Incorporating algae feeds and supplements into existing beekeeping practices have the potential to sustainably improve honey bee colony productivity and health. Additional studies and reproduction of previous results are necessary to build a complete profile of the ideal parameters for feed composition and delivery to bee colonies. Future research should focus on large-scale colony-feeding experiments using feed compositions that showed promise in laboratory and or small-scale field experiments. Characterization of diverse algae species for their nutritional value may help identify species candidates for honey bee diet development. Fractionization and metabolomic characterization of algae extracts combined with laboratory bioassays may identify natural products and structural leads for bee therapeutic development. Genetic engineering techniques may enable algae strain development that could address the specific health requirements of honey bees in a changing global climate. Among the literature reviewed, all but one study focused on Apis mellifera. Further research could be expanded to the development of nutrition supplements for other bee species of commercial importance such as Apis cerana and the genus Bombus.
All authors listed have made a substantial, direct, and intellectual contribution to the work and approved it for publication.
Thank you to the following individuals whose help made this review possible: James Bray, Erin Bauer, Emma Groom, Kasper Brandt, Ethan Goddard, Dan Nichols, and Maureen Lucasey-Nichols. Thank you to everyone who provided advice and encouragement during the writing of this review.
The authors declare that the research was conducted in the absence of any commercial or financial relationships that could be construed as a potential conflict of interest.
All claims expressed in this article are solely those of the authors and do not necessarily represent those of their affiliated organizations, or those of the publisher, the editors and the reviewers. Any product that may be evaluated in this article, or claim that may be made by its manufacturer, is not guaranteed or endorsed by the publisher.
The Supplementary Material for this article can be found online at: https://www.frontiersin.org/articles/10.3389/fsufs.2022.1005058/full#supplementary-material
Alberoni, D., Baffoni, L., Braglia, C., Gaggìa, F., and di Gioia, D. (2021). Honeybees exposure to natural feed additives: How is the gut microbiota affected? Microorganisms 9, 1009. doi: 10.3390/microorganisms9051009
Andrade, L. M., Andrade, C. J., Nascimento, C. A. O., and Dias, M. (2018). Chlorella and Spirulina microalgae as sources of functional foods, nutraceuticals, and food supplements; an overview. MOJ Food Process. Technol. 6, 45–58. doi: 10.15406/mojfpt.2018.06.00144
Araújo, R., Vázquez Calderón, F., Sánchez López, J., Azevedo, I. C., Bruhn, A., Fluch, S., et al. (2021). Current status of the algae production industry in europe: an emerging sector of the blue bioeconomy. Front. Marine Sci. 7. doi: 10.3389/fmars.2020.626389
Barker, R. J. (1977). Are algae toxic to honey bees? J. Arizona Acad. Sci. 12, 84–85. doi: 10.2307/40022219
Brodschneider, R., and Crailsheim, K. (2010). Nutrition and health in honey bees. Apidologie 41, 278–294. doi: 10.1051/apido/2010012
Butler, G. C. (1940). The choice of drinking water by the honey bee. J. Evol. Biol. 27, 253–261. doi: 10.1242/jeb.17.3.253
Castelli, L., Branchiccela, B., Garrido, M., Invernizzi, C., Porrini, M., and Romero, H. Impact of nutritional stress on honeybee gut microbiota, immunity, Nosema ceranae infection. Microb. Ecol. 80, 908–919. (2020). doi: 10.1007/s00248-020-01538-1
Cebatori, V., Buzu, I., Gliga, O., and Postolachi, O. (2017). New nutritional supplements for bees during deficient harvesting periods. Lucrǎri Ştiinţific—Universitatea de Ştiinţe Agricole Şi Medicinǎ Veterinarǎ, Seria Zootehnie 67, 73–80. Available online at: https://www.cabdirect.org/cabdirect/abstract/20173233302 (accessed October 19, 2022).
Cebatori, V., Buzu, I., Postolachi, O, and Gliga, O. (2016). Testing of the nutrient supplement enriched with biomass of aquatic algae in the bee's feed. Sci. Pap. Ser. D. Anim. Sci. 59, 85–90. Available online at: http://animalsciencejournal.usamv.ro/pdf/2016/Art16.pdf (accessed October 19, 2022).
Chakrabarti, P., Lucas, H. M., and Sagili, R. R. (2020). Novel insights into dietary phytosterol utilization and its fate in honey bees (Apis mellifera L.). Molecules 25, 571. doi: 10.3390/molecules25030571
Crailsheim, K., Schneider, L., Hrassnigg, N., Bühlmann, G., Brosch, U., Gmeinbauer, R., et al. (1992). Pollen consumption and utilization in worker honeybees (Apis mellifera carnica): dependence on individual age and function. J. Insect Physiol. 38, 409–419. doi: 10.1016/0022-191090117-V
De Groot A. P. (1953) Protein and amino acid requirements of the honeybee (Apis mellifica L.). Physiol. Comp. Oecol. 3, 1–83..
Dolezal, A. G., and Toth, A. L. (2018). Feedbacks between nutrition and disease in honey bee health. Curr. Opin. Insect Sci. 26, 114–119. doi: 10.1016/j.cois.2018.02.006
Dostálková, S., Urajová, P., CInčárová, D., Vránová, T., Hrouzek, P., Petrivalský, M., et al. (2021). Fatty acids and their derivatives from Chlorella vulgaris extracts exhibit in vitro antimicrobial activity against the honey bee pathogen Paenibacillus larvae. J. Apicult. Res. 1–13. doi: 10.1080/00218839.2021.1994264
Ellis, A. M., and Hayes, G. W. (2009). An evaluation of fresh versus fermented diets for honey bees (Apis mellifera). J. Apicult. Res. 48, 215–216. doi: 10.3896/IBRA.1.48.3.11
Engel, P., Kwong, W. K., McFrederick, Q., Anderson, K. E., Barribeau, S. M., Chandler, J. A., et al. (2016). The bee microbiome: impact on bee health and model for evolution and ecology of host-microbe interactions. MBio 7. doi: 10.1128/mBio.02164-15
Engel, P., Martinson, V. G., and Moran, N. A. (2012). Functional diversity within the simple gut microbiota of the honey bee. Proceed. Natl. Acad. Sci. 109, 11002–11007. doi: 10.1073/pnas.1202970109
Eremia, N., Bahcivanji, M., and Zagareanu, A. (2012). The effect of algal suspension “Chlorella vulgaris” using in artificial raising of queens. Sci. Pap. Ser. D Anim. Sci. 55, 158–161. Available online at: http://dspace.uasm.md:8080/xmlui/handle/123456789/4705 (accessed October 19, 2022).
Eremia, N., Bahcivanji, M., and Zagareanu, A. (2013). Study of influence of algal “Chlorella Vulgaris” suspension on growth and productivity of bees families. Lucrări Stiintifice-Seria Zootehnie 59, 148–152. Available online at: http://dspace.uasm.md:8080/xmlui/handle/123456789/5792 (accessed October 19, 2022).
Eremia, N., Neykovchena, J., and Kataraga, I. (2021). “ВЛИЯНИЕ СУСПЕНЗИИ ХЛОPЕЛЛЫ И КОPMОВОЙ ДОБAВКИ НA ЗИMОСТОЙКОСТЬ ПЧЕЛИНЫХ СЕMЕЙ (Influence of Chlorella suspension and feed additive on winter resistance of bee families),” in International Scientific and Practical Conference “Science, Education, culture”, Dedicated to the 30th Anniversary Comrat State University. Available online at: http://dspace.uasm.md:8080/handle/123456789/5778 (accessed October 19, 2022).
Eshel, G., Shepon, A., Makov, T., and Milo, R. (2014). Land, irrigation water, greenhouse gas, and reactive nitrogen burdens of meat, eggs, and dairy production in the United States. Proc. Natl. Acad. Sci. 111, 11996–12001. doi: 10.1073/pnas.1402183111
Guldas, M., Gurbuz, O., Cakmak, I., Yildiz, E., and Sen, H. (2022). Effects of honey enrichment with Spirulina platensis on phenolics, bioaccessibility, antioxidant capacity and fatty acids. LWT 153, 112461. doi: 10.1016/j.lwt.2021.112461
Hassan, R. E., and Mahmoud, N. A. (2006). Effect of some algal metabolites producedes for controlling varroa mite infesting honeybee colonies. Assiut J. Agric. Sci. 37, 233–244.
Herbert, E. W., Shimanuki, H., and Shasha, B. S. (1980). Brood rearing and food consumption by honeybee colonies fed pollen substitutes supplemented with starch-encapsulated pollen extracts. J. Apicult. Res. 19, 115–118. doi: 10.1080/00218839.1980.11100008
Jang, H., Ghosh, S., Sun, S., Cheon, K. J., Mohamadzade Namin, S., and Jung, C. (2022). Chlorella-supplemented diet improves the health of honey bee (Apis mellifera). Front. Ecol. Evol. 10. doi: 10.3389/fevo.2022.922741
Jehlík, T., Kodrík, D., Krištufek, V., Koubová, J., Sábová, M., Danihlík, J., et al. (2019). Effects of Chlorella sp. on biological characteristics of the honey bee Apis mellifera. Apidologie 50, 564–577. doi: 10.1007/s13592-019-00670-3
Johansson, T. S. K., and Johansson, M. P. (1977). Feeding honeybees pollen and pollen substitutes. Bee World 58, 105–118. doi: 10.1080/0005772X.1977.11097658
Khalifa, S. A. M., Elshafiey, E. H., Shetaia, A. A., El-Wahed, A. A. A., Algethami, A. F., Musharraf, S. G., et al. (2021). Overview of bee pollination and its economic value for crop production. Insects 12, 688. doi: 10.3390/insects12080688
Khan, K. A., and Ghramh, H. A. (2022). Nutritional efficacy of different diets supplemented with microalga Arthrospira platensis (spirulina) in honey bees (Apis mellifera). J. King Saud Univ. Sci. 34, 101819. doi: 10.1016/j.jksus.2021.101819
Khan, M. I., Shin, J. H., and Kim, J. D. (2018). The promising future of microalgae: current status, challenges, and optimization of a sustainable and renewable industry for biofuels, feed, and other products. Microb. Cell Fact. 17. doi: 10.1186/s12934-018-0879-x
Klein, A. M., Vaissière, B. E., Cane, J. H., Steffan-Dewenter, I., Cunningham, S. A., Kremen, C., et al. (2006). Importance of pollinators in changing landscapes for world crops. Proc. Royal Soc. B Biol. Sci. 274, 303–313. doi: 10.1098/rspb.2006.3721
Kumar, R., Mishra, R. C., and Agrawal, O. P. (2013). Effect of feeding artificial diets to honey bees during dearth period under Panchkula (Haryana) conditions. J. Entomol. Res. 37, 41–46.
Liu, P., Zhu, Y., Ye, L., Shi, T., Li, L., Cao, H., et al. (2021). Overwintering honeybees maintained dynamic and stable intestinal bacteria. Sci. Rep. 11. doi: 10.1038/s41598-021-01204-7
Ma, L., Wang, Y., Hang, X., Wang, H., Yang, W., and Xu, B. (2015). Nutritional effect of alpha-linolenic acid on honey bee colony development (Apis Mellifera L.). J. Apicult. Sci. 59, 63–72. doi: 10.1515/jas-2015-0023
Madeira, M. S., Cardoso, C., Lopes, P. A., Coelho, D., Afonso, C., Bandarra, N. M., et al. (2017). Microalgae as feed ingredients for livestock production and meat quality: a review. Livestock Sci. 205, 111–121. doi: 10.1016/j.livsci.2017.09.020
Manning, R. (2018). Artificial feeding of honey bees based on an understanding of nutritional principles. Anim. Prod. Sci. 58, 689. doi: 10.1071/AN15814
Moran, N.A. (2015). Genomics of the honey bee microbiome. Curr. Opin. Insect Sci. 10, 22–28. doi: 10.1016/j.cois.2015.04.003
Noordyke, E. R., and Ellis, J. D. (2021). Reviewing the efficacy of pollen substitutes as a management tool for improving the health and productivity of western honey bee (Apis mellifera) colonies. Front. Sustain. Food Syst. 5. doi: 10.3389/fsufs.2021.772897
Okle, O., Rath, L., Galizia, C. G., and Dietrich, D. R. (2013). The cyanobacterial neurotoxin beta-N-methylamino-l-alanine (BMAA) induces neuronal and behavioral changes in honeybees. Toxicol. Appl. Pharmacol. 270, 9–15. doi: 10.1016/j.taap.2013.04.003
Oswald, W. J., Goleuke, C. G., and and University of California Berkeley (1967). “Large-scale production of algae,” in U.S. Department of Energy Office of Scientific and Technical Information. Available online at: https://www.osti.gov/biblio/5398149 (accessed October 19, 2022).
Paray, B. A., Kumari, I., Hajam, Y. A., Sharma, B., Kumar, R., Albeshr, M. F., et al. (2021). Honeybee nutrition and pollen substitutes: a review. Saudi J. Biol. Sci. 28, 1167–1176. doi: 10.1016/j.sjbs.2020.11.053
Piwowar, A., and Harasym, J. (2020). The importance and prospects of the use of algae in agribusiness. Sustainability 12, 5669. doi: 10.3390/su12145669
Potts, S. G., Biesmeijer, J. C., Kremen, C., Neumann, P., Schweiger, O., and Kunin, W. E. (2010). Global pollinator declines: trends, impacts and drivers. Trends Ecol. Evol. 25, 345–353. doi: 10.1016/j.tree.2010.01.007
Ramsey, S. D., Ochoa, R., Bauchan, G., Gulbronson, C., Mowery, J. D., Cohen, A., et al. (2019). Varroa destructor feeds primarily on honey bee fat body tissue and not hemolymph. Proc. Natl. Acad Sci. 116, 1792–1801. doi: 10.1073/pnas.1818371116
Raymann, K., and Moran, N. A. (2018). The role of the gut microbiome in health and disease of adult honey bee workers. Curr. Opin. Insect Sci. 26, 97–104. doi: 10.1016/j.cois.2018.02.012
Requier, F., Odoux, J. F., Tamic, T., Moreau, N., Henry, M., Decourtye, A., et al. (2015). Honey bee diet in intensive farmland habitats reveals an unexpectedly high flower richness and a major role of weeds. Ecol. Appl. 25, 881–890. doi: 10.1890/14-1011.1
Ricigliano, V. A. (2020). Microalgae as a promising and sustainable nutrition source for managed honey bees. Arch. Insect Biochem. Physiol. 104. doi: 10.1002/arch.21658
Ricigliano, V. A., Cank, K. B., Todd, D. A., Knowles, S. L., and Oberlies, N. H. (2022a). Metabolomics-guided comparison of pollen and microalgae-based artificial diets in honey bees. J. Agric. Food Chem. 70, 9790–9801. doi: 10.1021/acs.jafc.2c02583
Ricigliano, V. A., Ihle, K. E., and Williams, S. T. (2021). Nutrigenetic comparison of two varroa-resistant honey bee stocks fed pollen and spirulina microalgae. Apidologie 52, 873–886. doi: 10.1007/s13592-021-00877-3
Ricigliano, V. A., and Simone-Finstrom, M. (2020). Nutritional and prebiotic efficacy of the microalga Arthrospira platensis (spirulina) in honey bees. Apidologie 51, 898–910. doi: 10.1007/s13592-020-00770-5
Ricigliano, V. A., Williams, S. T., and Oliver, R. (2022b). Effects of different artificial diets on commercial honey bee colony performance, health biomarkers, and gut microbiota. BMC Veter. Res. 18. doi: 10.1186/s12917-022-03151-5
Roulston, T. H., and Cane, J. H. (2000). Pollen nutritional content and digestibility for animals. Plant Syst. Evol. 222, 187–209. doi: 10.1007/BF00984102
Roulston, T. H., Cane, J. H., and Buchmann, S. L. (2000). What governs protein content of pollen: pollinator preferences, pollen-pistil interactions, or phylogeny? Ecol. Monogr. 70, 617–643. doi: 10.1890/0012-9615(2000)070[0617:WGPCOP]2.0.CO;2
Roussel, M., Villay, A., Delbac, F., Michaud, P., Laroche, C., Roriz, D., et al. (2015). Antimicrosporidian activity of sulphated polysaccharides from algae and their potential to control honeybee nosemosis. Carbohydr. Polym. 133, 213–220. doi: 10.1016/j.carbpol.2015.07.022
Saadaoui, I., Rasheed, R., Aguilar, A., Cherif, M., Jabri, H., Sayadi, S., et al. (2021). Microalgal-based feed: promising alternative feedstocks for livestock and poultry production. J. Anim. Sci. Biotechnol. 12. doi: 10.1186/s40104-021-00593-z
Saffari, A., Kevan, P. G., and Atkinson, J. L. (2010). Palatability and consumption of patty-formulated pollen and pollen substitutes and their effects on honey bee colony performance. J. Apic. Sci. 54, 63–71. Available online at: https://www.apiservices.biz/documents/articles-en/patty_formulated_pollen_and_substitutes.pdf (accessed October 19, 2022).
Schmickl, T., and Karsai, I. (2017). Resilience of honeybee colonies via common stomach: a model of self-regulation of foraging. PLoS ONE 12, e0188004. doi: 10.1371/journal.pone.0188004
Singh, R., Parihar, P., Singh, M., Bajguz, A., Kumar, J., Singh, S., et al. (2017). Uncovering potential applications of cyanobacteria and algal metabolites in biology, agriculture and medicine: current status and future prospects. Front. Microbiol. 8. doi: 10.3389/fmicb.2017.00515
Singh, R., and Sharma, S. (2012). Development of suitable photobioreactor for algae production—a review. Renew. Sustain. Energy Rev. 16, 2347–2353. doi: 10.1016/j.rser.2012.01.026
Stengel, D. B., Connan, S., and Popper, Z. A. (2011). Algal chemodiversity and bioactivity: sources of natural variability and implications for commercial application. Biotechnol. Adv. 29, 483–501. doi: 10.1016/j.biotechadv.2011.05.016
Suganya, T., Varman, M., Masjuki, H., and Renganathan, S. (2016). Macroalgae and microalgae as a potential source for commercial applications along with biofuels production: a biorefinery approach. Renew. Sustain. Energy Rev. 55, 909–941. doi: 10.1016/j.rser.2015.11.026
Toderaş, I., Cebatori, V., Ungureanu, L., Buzu, I., Gheorghită, C., Floquet, S., et al. (2017). New nutritive supplements for feeding mellifera bees in the deficit periods of collection in nature. Buletinul Academiei de Stiinte a Moldovei. Stiintele Vietii, 2, 26–43. Available online at: https://ibn.idsi.md/sites/default/files/imag_file/New%20nutritive%20supplements%20for%20feeding%20mellifera%20bees%20in%20the%20deficit%20periods%20of%20collection%20in%20nature.pdf (accessed October 19, 2022).
Toderaş, I., Rudic, V., Gulea, A., Cebatori, V., and Buzu, I. (2014). The influence of organic remedies next-generation bioactive agents on the vital activity of bee families Apis mellifera. Buletinul Academiei de Stiinte a Moldovei. Stiintele Vietii 3, 4–15. Available online at: https://ibn.idsi.md/ro/vizualizare_articol/34167 (accessed October 19, 2022).
Truzzi, C., Giorgini, E., Annibaldi, A., Antonucci, M., Illuminati, S., Scarponi, G., et al. (2020). Fatty acids profile of black soldier fly (Hermetia illucens): Influence of feeding substrate based on coffee-waste silverskin enriched with microalgae. Anim. Feed Sci. Technol. 259, 114309. doi: 10.1016/j.anifeedsci.2019.114309
Tsuruda, J. M., Chakrabarti, P., and Sagili, R. R. (2021). Honey bee nutrition. Veter. Clin. North Am. Food Anim. Pract. 37, 505–519. doi: 10.1016/j.cvfa.2021.06.006
Ullmann, J., and Grimm, D. (2021). Algae and their potential for a future bioeconomy, landless food production, and the socio-economic impact of an algae industry. Organ. Agric. 11, 261–267. doi: 10.1007/s13165-020-00337-9
Valente, L. M., Cabrita, A. R., Maia, M. R., Valente, I. M., Engrola, S., Fonseca, A. J., et al. (2021). Microalgae as feed ingredients for livestock production and aquaculture. Microalgae 239–312. doi: 10.1016/B978-0-12-821218-9.00009-8
van der Sluijs, J. P., and Vaage, N. S. (2016). Pollinators and global food security: the need for holistic global stewardship. Food Ethics 1, 75–91. doi: 10.1007/s41055-016-0003-z
Vaudo, A. D., Tooker, J. F., Grozinger, C. M., and Patch, H. M. (2015). Bee nutrition and floral resource restoration. Curr. Opin. Insect Sci. 10, 133–141. doi: 10.1016/j.cois.2015.05.008
Vigani, M., Parisi, C., Rodríguez-Cerezo, E., Barbosa, M. J., Sijtsma, L., Ploeg, M., et al. (2015). Food and feed products from micro-algae: market opportunities and challenges for the EU. Trends Food Sci. Technol., 42, 81–92. doi: 10.1016/j.tifs.2014.12.004
Vránová, T. (2017). Antibakteriální účinky extraktu z rasy Chlorella sorokiniana vuči bakterii Paenibacillus larvae. Palacky University. Available online at: https://https://theses.cz/id/bf6beq/tereza.vranova.2017.pdf?lang=en (accessed October 19, 2022).
Wollmann, F., Dietze, S., Ackermann, J., Bley, T., Walther, T., Steingroewer, J., et al. (2019). Microalgae wastewater treatment: Biological and technological approaches. Eng. Life Sci. 19, 860–871. doi: 10.1002/elsc.201900071
Wright, G. A., Nicolson, S. W., and Shafir, S. (2018). Nutritional physiology and ecology of honey bees. Ann. Rev. Entomol. 63, 327–344. doi: 10.1146/annurev-ento-020117-043423
Xing, W., Zhang, R., Shao, Q., Meng, C., Wang, X., Wei, Z., et al. (2021). Effects of laser mutagenesis on microalgae production and lipid accumulation in two economically important fresh Chlorella strains under heterotrophic conditions. Agronomy 11, 961. doi: 10.3390/agronomy11050961
Yaakob, Z., Ali, E., Zainal, A., Mohamad, M., and Takriff, M. S. (2014). An overview: biomolecules from microalgae for animal feed and aquaculture. J. Biol. Res. Thessaloniki 21. doi: 10.1186/2241-5793-21-6
Yefimenko, T., and Odnosum, H. (2020). the effect of feeding a suspension of Chlorella vulgaris (strain IFR No C-111) on the life span of bees. Sci. Prod. J. Beekeep. Ukraine 1, 13–18. doi: 10.46913/beekeepingjournal.2020.4.02
Zagareanu, A. (2013). The use of algal suspension of Chlorella vulgaris in beekeeping. Stiinta Agricola 115, 78–82.
Keywords: microalgae, Apis mellifera, spirulina, Chlorella, pollen substitute, biomass, sustainable diet, pathogen resistance
Citation: Nichols BJ and Ricigliano VA (2022) Uses and benefits of algae as a nutritional supplement for honey bees. Front. Sustain. Food Syst. 6:1005058. doi: 10.3389/fsufs.2022.1005058
Received: 27 July 2022; Accepted: 06 October 2022;
Published: 04 November 2022.
Edited by:
José Carlos Pires, University of Porto, PortugalReviewed by:
Jevrosima Stevanovic, University of Belgrade, SerbiaCopyright © 2022 Nichols and Ricigliano. This is an open-access article distributed under the terms of the Creative Commons Attribution License (CC BY). The use, distribution or reproduction in other forums is permitted, provided the original author(s) and the copyright owner(s) are credited and that the original publication in this journal is cited, in accordance with accepted academic practice. No use, distribution or reproduction is permitted which does not comply with these terms.
*Correspondence: Benjamin J. Nichols, YmVuamFtaW4ubmljaG9sc0BibGFja2J1cm4uZWR1; Vincent A. Ricigliano, VmluY2VudC5yaWNpZ2xpYW5vQFVTREEuR09W
Disclaimer: All claims expressed in this article are solely those of the authors and do not necessarily represent those of their affiliated organizations, or those of the publisher, the editors and the reviewers. Any product that may be evaluated in this article or claim that may be made by its manufacturer is not guaranteed or endorsed by the publisher.
Research integrity at Frontiers
Learn more about the work of our research integrity team to safeguard the quality of each article we publish.