- 1Rodale Institute, Kutztown, PA, United States
- 2Iowa State University Extension and Outreach, Ames, IA, United States
- 3Department of Agricultural and Environmental Sciences, Tennessee State University, Nashville, TN, United States
Reducing tillage and supporting continuous living cover (CLC) can improve agroecosystem sustainability under both organic and conventional field crop production. What is less clear, however, is how reducing tillage affects the economic sustainability of organic field crop systems with CLC as compared to conventional field crop systems. To address this knowledge gap, we conducted a comprehensive economic analysis based on field records and crop yields from the long-term Farming Systems Trial (FST) at Rodale Institute in Kutztown, Pennsylvania. The FST (established in 1981) comprises three farming systems (conventional, low-input organic, and manure-based organic) which were split into tilled and reduced-till treatments in 2008. FST field activities, inputs, and crop yields from 2008 to 2020 were used to construct enterprise budgets to assess cumulative labor, costs, returns, and economic risk of six replicated theoretical farms. Reducing tillage on the conventional farms led to lower gross revenues (−10%), but lower annual costs (−5%) helped maintain similar net returns but increased economic risk as compared to tilled conventional farms. Reducing tillage on the low-input organic farms also led to lower gross revenues (−13%) and lower annual costs (−6%), which maintained net returns and increased risk relative to the tilled, low-input organic farms. For the more diverse manure-based organic farms that include periods of mixed perennial cover, reducing tillage had a smaller effect on overall costs (−2%) and no effect on gross revenues, net returns, or economic risk. Overall, reducing tillage did not affect the long-term profitability of any of the three FST farming systems. Regardless of tillage practices or organic price premiums, the manure-based organic system supported higher net returns than the conventional system. This finding suggests that continuous living cover and manure inputs may have a greater influence on system profitability than tillage practices.
1. Introduction
Innovative agricultural practices developed during the 20th century helped double grain yields since the 1960's (Ramankutty et al., 2018), but the widespread adoption of synthetic pesticides, inorganic fertilizers, expansive monocultures, and intensive tillage has come at great human health and environmental costs (Tilman et al., 2002; LaCanne and Lundgren, 2018; Sanaullah et al., 2020). To continue feeding, fueling, and clothing a growing population, these once-innovative, now conventional agricultural practices may need to be phased out in favor of alternative, conservation-based practices. Conservation-based practices can improve soil health and environmental quality, and include strategies such as reducing pesticide use, diversifying crop rotations, aiming for continuous living cover (CLC), and applying organic fertilizers (Palm et al., 2014). It is no coincidence that these practices are fundamental to organic crop production, which overall has been shown to reduce the negative effects of agriculture on environmental health (Gomiero et al., 2011). In addition to these environmental benefits, organic production is often more profitable because of the price premiums that consumers are willing to pay for organic products (Reganold and Wachter, 2016).
Although organic farmers have outpaced conventional farmers in the overall adoption of conservation-based practices (Gomiero et al., 2011), one specific practice–reducing tillage–has been more readily adopted under conventional management (Mirsky et al., 2012; Claassen et al., 2018). Reduced-till management has been adopted by over 65% of conventional farmers in the United States in part because most have access to low-cost herbicides that provide an effective way to manage weeds without regular tillage (Pittelkow et al., 2015; Benbrook, 2016; NASS, 2019; White et al., 2019). Conventional reduced-till strategies can significantly lower operating costs and, depending on climate and other cropping conditions, can support high crop yields (Archer and Reicosky, 2009; Chavas et al., 2009; Toliver, 2010; Deines et al., 2019). Lower crop yields in response to reducing tillage are often attributed to high soil compaction, nutrient deficiencies, and/or high weed pressure (Pittelkow et al., 2015)—all factors that could be mitigated by supporting continuous living cover. While many conventional farmers have adopted reduced-till strategies, far fewer have adopted practices that support continuous living cover (e.g., only 7.5% of farmers plant cover crops; NASS, 2019).
In contrast, one of the most popular strategies to reduce tillage in organic systems is cover crop-based, rotational no-till, where cover crops are mechanically terminated to form a weed-barrier mulch (Ashford and Reeves, 2003; Wallace et al., 2017; Frasconi et al., 2019). Under this strategy, mechanical termination of cover crops usually occurs at the same time as planting, eliminating any period of bare soil between cover crop termination and planting cash crops. Despite the potential cost savings and soil conservation benefits of reducing tillage and maintaining continuous living cover, uncertainty regarding yields and profitability could limit the adoption of reduced-till adoption in organic systems.
Reducing tillage under organic production has been hypothesized to have similar economic benefits as under conventional production (Peigné et al., 2007; Mirsky et al., 2012), but few studies have tested this hypothesis (Delate et al., 2012; Wittwer et al., 2021). Moreover, existing studies were either based on short-term trials (Delate et al., 2012), which may not capture year-to-year variability in crop yields (Delbridge et al., 2011) or excluded genetically modified crops and pesticide seed coatings (Wittwer et al., 2021) that currently dominate U.S. field crop production (Douglas and Tooker, 2015; Donley, 2019) and significantly affect management costs and profitability of conventional systems (Finger et al., 2011; Alford and Krupke, 2018). Although these existing studies support the hypothesis that reduced-till organic production is profitable, there is a clear knowledge gap regarding the long-term economic impacts of reducing tillage in organic farming systems compared to conventional systems. A long-term economic comparison of such systems could help address this knowledge gap and provide critical information for farmers and policymakers interested in organic reduced-till production.
In this paper, we conducted a comprehensive economic analysis of the long-term Farming Systems Trial (FST) at the Rodale Institute in Kutztown, Pennsylvania, which provided a unique opportunity to perform a side-by-side economic comparison of reducing tillage in organic and conventional farming systems with different fertility inputs and different degrees of continuous living cover. We hypothesized that reducing tillage would lower crop yields and gross revenue in both organic and conventional field crop systems, but net returns would be higher due to the lower production costs associated with reducing tillage. The results of this economic analysis will serve as a valuable resource for extension agents, farmers, and policymakers to assess the economic advantages and disadvantages of reducing tillage in organic and conventional farming systems.
2. Materials and methods
2.1. Design of the farming systems trial
This economic analysis was based on field operation and input records of the Farming Systems Trial (FST) at the Rodale Institute in Kutztown, Pennsylvania (Berks County, 40° 33′ 5″-75° 43′ 47″). The FST was originally established in 1981 to study soil health, agronomy, and economics during a transition to organic grain production. The FST initially comprised three conventionally-tilled cropping systems: (1) a conventional system with inorganic fertilizer inputs (CNV); (2) a low-input organic system that relies on leguminous cover crops to supply nitrogen inputs (LEG); and (3) an organic system with cover crops, periodic manure inputs, and a perennial hay phase of 2–3 years during each crop rotation (MNR). Cropping systems were replicated eight times (18 × 92-m plots), with each replicate divided into three subplots (6 × 92-m) planted in different phases of the crop rotations. In 2008, reduced-till treatments were introduced to the study by reducing tillage in half of the system replicates (RT-CNV, RT-LEG, and RT-MNR) while conventional, full tillage (FT) continued in the other four replicates (FT-CNV, FT-LEG, and FT-MNR). Herbicide application helped to achieve complete no-till production in the RT-CNV treatment, lowering average Soil Tillage Intensity Ratings (STIR) ratings to 4.5 ± 0.4 compared to 142.7 ± 8.4 in the FT-CNV treatment (Pearsons et al., 2023). Rotational no-till was achieved in the organic systems by no-till planting organic maize (Zea mays L.) and soybeans (Glycine max L.) into cover crops that were mechanically terminated by use of a roller-crimper (Ashford and Reeves, 2003; Moyer, 2021). Depending on the sub-plot, no-till organic maize and soybeans accounted for 15–24% of planting events in the RT-MNR treatment and 15–30% of planting events in the RT-LEG treatment (Supplementary Figures S1, S2). Moldboard plowing and disking preceded all other crops and cover crops in the organic treatments while chisel plowing and disking preceded all crops and cover crops in the FT-CNV treatment. Average STIR ratings for the FT-LEG and FT-MNR treatments were 263.7 ± 29.2 and 196.2 ± 37.9 respectively, while STIR ratings for the RT-LEG and RT-MNR treatments were 178.5 ± 15.5 and 126.3 ± 18.8 respectively (Pearsons et al., 2023).
Between 2008 and 2020, crop rotations differed among the three systems (Supplementary Figures S1, S2). The CNV system followed 2- and 3-year rotations of maize, soybean and occasionally wheat (Triticum aestivum L.). In some years, hairy vetch (Vicia villosa) or cereal rye (Secale cereale) cover crops were planted in CNV sub-plots. The LEG system followed a 4-year maize–oats (Avena sativa L.)–soybeans–wheat rotation, with hairy vetch preceding maize and rye cover preceding oats and soybeans. As an additional source of nitrogen, clover (Trifolium spp.) was planted concurrently with oats. From 2008–2014, a barley crop (Hordeum vulgare L.) was grown in the FT-LEG plots prior to soybeans and in place of the rye cover crop. The MNR system followed the LEG rotation but with the addition of 2–3 years of alfalfa-orchardgrass hay (7:4 w:w Medicago sativa L. and Dactylis glomerata L.), 1 year of maize silage, and one additional year of wheat (Supplementary Figures S1, S2). In an average year, all four organic treatments had living cover for over 10 months (FT-LEG = 10.3 months, RT-LEG = 10.6 months, FT-MNR = 10.4 months, RT-MNR = 10.2 months). Even with occasional cover crops, the CNV treatments had living cover for fewer months (FT-CNV = 6.3 months; RT-CNV = 8.4 months) compared to the organic treatments.
Typical field operations for each crop within each treatment are summarized in Table 1. For each year of the study, fertility inputs in the CNV system were based on soil tests and recommendations from the Penn State Agricultural Analytical Services Laboratory (University Park, PA, U.S.A.) and herbicide applications (timing, mixtures, and rates) were based on recommendations from Weed Extension Specialists from the Pennsylvania State University. Between 2008 and 2020, the only external fertility input to the LEG system was potassium sulfate, applied at a rate of 170 kg K ha−1 in 2008 and 2012. For the MNR system, composted manure was applied at a target rate of 89.7 kg N ha−1 prior to planting corn silage and oats. Potassium sulfate was also applied to the MNR system in 2008 and 2012, at the same 170 kg K ha−1 rate as in the LEG system. No pest management strategies were deployed in the MNR system, but parasitoid wasps (Trichogramma ostriniae; IPM Labs, Locke, NY, USA) were deployed in 2012 to help control European corn borer (Ostrinia nubilalis) in the LEG system based on recommendations from Pennsylvania State University Extension.
2.2. Relative crop yields
Relative yields (YR) were analyzed to account for the different frequencies for which specific crops were grown within each treatment. YR was calculated as the ratio of the experiment yield compared to county average yields:
Where YR = relative yield (a unitless value), YE = experimental yield at the subplot level, and YA = county average yield for a given crop in a given year. Average county yields were obtained from the USDA NASS – Quick Stats database (NASS, 2021). In years where average crop yields were unavailable for Berks County, values from nearby Lehigh County (maize: 2009, 2010, 2013; oats: 2010, 2013, 2019) or all of Pennsylvania (wheat: all years; barley: 2009, 2010; hay: 2016, 2017, 2019, 2020; silage corn: 2013, 2018) were substituted. Student's t-tests were used to test if average crop yields were significantly different than county averages (H0: YR = 1) for each crop in each treatment. Raw yield data from the FST for this period (2008–2020) were analyzed and discussed as part of an assessment of grain quality from the FST (Pearsons et al., 2022).
2.3. Enterprise budgets
Records of field activities, inputs, and crop yields from the FST were used to construct enterprise budgets (Chase et al., 2019; Chase, 2020) for each subplot for each year from 2008 through 2013, as well as from 2016 through 2020 (780 budgets). The typical FST crop rotations were interrupted in 2014, so 2014 and 2015 were excluded from this analysis.
For each year of the study, input costs were estimated using management records, recent prices from vendors, government databases, agricultural extension documents, and communication with extension specialists. Estimated costs of crop production were not available for Pennsylvania after 2016, so machinery operation costs, per hour labor costs, fertilizer costs, cash rent equivalent of land, and most conventional seed costs (maize, soybeans, oats, alfalfa, and orchardgrass) were derived from annual Estimated Costs of Crop Production in Iowa documents (Duffy and Smith, 2008a,b; Duffy, 2009, 2011, 2012, 2013; Plastina, 2016, 2017, 2018, 2019, 2020). Hourly labor requirements were derived from Estimating the Field Capacity of Farm Machines (Hanna, 2016). Other seed costs were based on actual purchase prices for seeds planted in the FST or were estimated based on the relative price of seeds purchased for the FST in 2021. Based on these relative prices, organic maize and soybean seeds were priced as 80% the cost of conventional maize and soybean seeds; organic oat, orchardgrass, and alfalfa seeds were priced as 120% conventional seeds; rye seeds were priced the same as oat seeds; wheat and barley seeds were priced at 2 × oats; hairy vetch was priced as 40% the cost of alfalfa seeds; and clover was priced as 70% the cost of alfalfa seeds. All seed costs and estimates used in this study are listed in Supplementary Table S1.
Herbicide cost estimates were based on annual average prices set by dealers throughout Pennsylvania; these estimates were compiled and confidentially provided to the authors by Penn State Extension. The cost of deploying parasitoid wasps in 2012 was provided by IPM Labs (Locke, NY, USA), with labor costs estimates derived from Gagnon et al. (2016). Year-adjusted costs to apply composted manure were based on scaled-up labor, fuel, and machinery inputs required to produce, haul, and spread compost at the Rodale Institute in 2008. Annual cost estimates for labor, fertilizers, manure, parasitoid wasps, and seeds are included in the Supplementary Table S1 (confidential herbicide costs not included).
Average annual market crop prices for Pennsylvania were obtained from USDA NASS Quick stats database (NASS, 2021). For years where organic market prices for specific crops were unavailable for Pennsylvania (noted in Supplementary Table S2), the organic price premium was either estimated based on the organic price premium for grains in Iowa (AMS, 2021; NASS, 2021) or interpolated based on averaging the price premium in adjacent years. Missing organic maize silage prices were estimated as 0.33 × the value of organic hay based on the method used by Oregon State University (Downing et al., 2013), while all straw prices were estimated as 0.75 × the average value of conventional hay (Chase et al., 2019). Market prices are listed in Supplementary Table S2, with annotations to note estimated or adjusted values.
2.4. Modeling representative farms
To better reflect how the management practices applied in the FST would affect labor, costs, returns, and risk for a representative farm, we used enterprise budgets to model six replicated theoretical farms, each based on one FST plot (3 systems × 2 tillage treatments, each replicated 4 times). Each farm comprised three, 18-ha fields which corresponded to the three subplots within each FST plot. Field size was chosen based on the average farm size in Pennsylvania during 2008–2020 (54-ha; NASS, 2019). Statistical analyses were performed on the labor, costs, and returns from these 24 theoretical farms.
2.5. Risk and sensitivity analyses
We assessed system risk using (1) a simple assessment of year-to-year variability of net returns for each farm (standard deviation) and (2) a safety-first model which additionally accounts for average net returns (Musser et al., 1981; Hanson et al., 1990; White et al., 2019). The output of the safety-first model (lower confidence limit for net returns, L) can give farmers an idea of how often they can expect net returns to exceed a certain value. The most common lower confidence limit for net returns is L75, which represents the lowest net returns a farmer can expect in 3 out of every 4 years, where:
with E equal to average annual net returns and S equal to the standard deviation of average annual net returns. Larger L75 values indicate less risky systems, as they are expected to produce higher net returns in most years (3 out of 4 years). Additionally, we used linear interpolation to assess the sensitivity of the two organic systems to variation in price premiums (White et al., 2019).
2.6. Statistical analyses
All statistical analyses were performed in R (v.4.0.3). Relative crop yields and returns (with and without organic premiums) were compared across systems and tillage treatments using linear mixed effect models (LMER from the “lme4” package), with system and tillage as fixed effects and year, farm replicate, and the interaction of year and farm replicate as random effects (Bates et al., 2015). As the goal of this analysis was to test the effect of reducing tillage within each system, tillage treatment was nested within system. For all models, model residuals were tested for homogenous variance and normality. Pairwise mean comparisons were generated using the EMMEANS function from the “emmeans” package, with “mvt” P-value adjustments to account for multiple comparisons (Lenth, 2021). Raw yield data from the same period (2008–2020) for this experiment have been previously analyzed and discussed as part of an assessment of grain quality from the FST (Pearsons et al., 2022).
3. Results
3.1. Relative crop yields
Depending on the crop, relative yields differed across farming systems and between tillage treatments (Figure 1, crop-specific results are presented and discussed in the Supplementary material). Contrary to our hypothesis, overall relative yields (averaged across all crops) did not significantly differ between tillage treatments [Tillage (System): = 3.4, P = 0.34]. Overall relative yields significantly differed across farming systems (System: = 118.9, P < 0.0001), with higher yields in the MNR system compared to county averages and significantly lower yields in the LEG system compared to county averages (MNR relative = 1.23 ± 0.06, LEG relative = 0.78 ± 0.07).
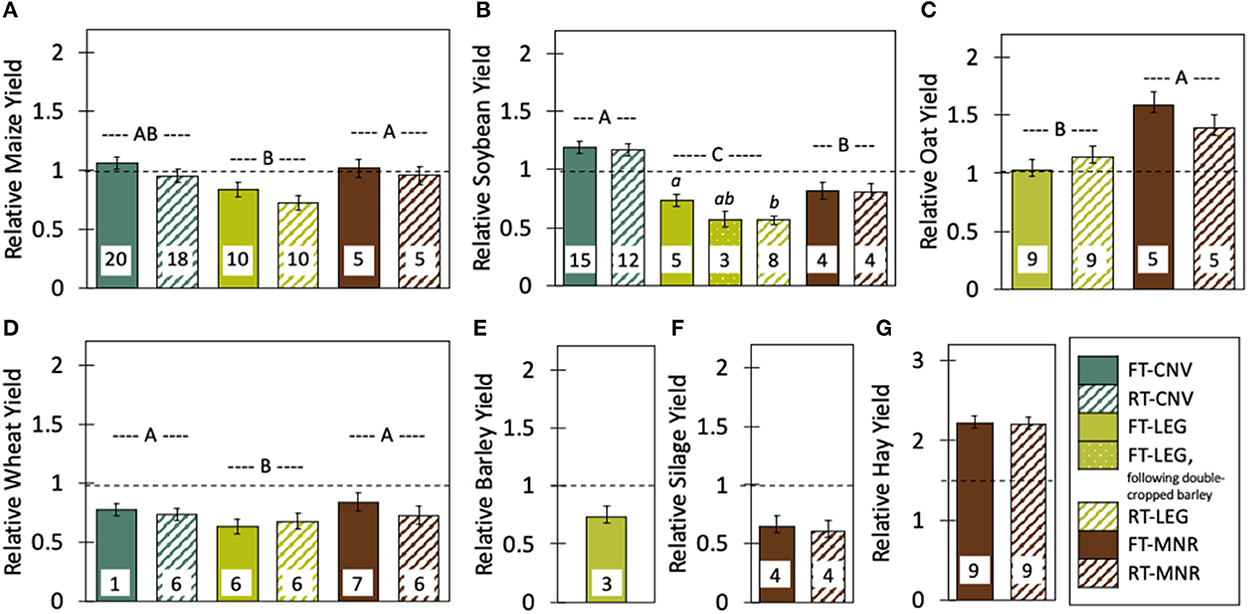
Figure 1. Relative yields across all tillage × system treatments in the FST from 2008–2020 for (A) maize [System: = 31.8, P < 0.0001, Tillage (System): = 4.7, P = 0.20], (B) soybeans [System: = 279.2, P < 0.0001, Tillage (System): = 4.9, P = 0.18], (C) oats [System: = 29.3, P < 0.0001, Tillage (System): = 6.2, P = 0.05], (D) wheat [System: = 18.5, P < 0.0001, Tillage (System): = 11.2, P = 0.01], (E) barley, (F) maize silage (Tillage: = 0.7, P = 0.42), and (G) hay (Tillage: = 0.02, P = 0.90). Means and error bars are estimated marginal means (EMM) and standard errors from mixed models. Different uppercase letters above bars indicate significant differences across systems (Tukey HSD, P < 0.05). Different lowercase letters above bars in (B) indicate significant differences in soybean yields across tillage treatments, with FT yields split between soybeans that were planted following rye cover crops (solid green bar) or double-cropped following barley (green bar with white dots).
3.2. Costs
3.2.1. Field operations and labor costs
Annual costs associated with field operation were lowest under conventional management (Figure 2; Table 2), averaging 21% higher in the LEG system and 34% higher in the MNR system. Reducing tillage reduced field operation costs across all three systems by an average of 16%. Low field operation costs in the CNV system corresponded with the lowest labor costs, which were over twice as high in both organic systems. Reducing tillage reduced labor costs across all three systems by an average of 20%.
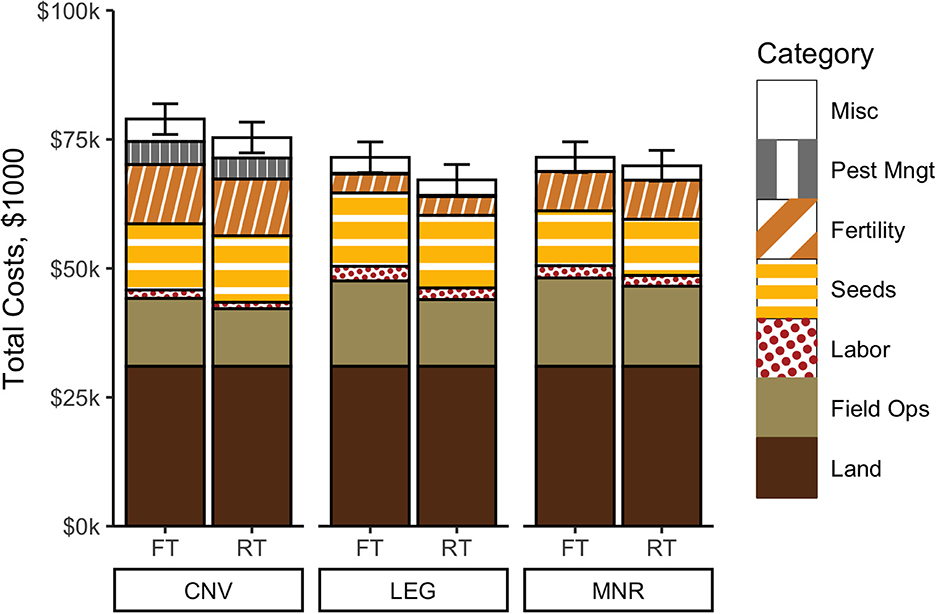
Figure 2. Average annual costs for each representative farm with different tillage × system treatments. Total costs include land (brown), field operations (variable and fixed preharvest and harvest actives; tan), labor (dotted red), seeds (horizontal yellow lines), fertility inputs (diagonal gold lines), pest management inputs (vertical gray lines), and all other costs (interest, insurance, and miscellaneous expenses; white). Error bars are standard errors for total costs within each tillage × system treatment.

Table 2. Means and standard deviations (sd) of annual costs for representative farms with different tillage × system treatments.
3.2.2. Input costs (seeds, fertility, pest management)
Fertility, seed, and pest management costs all differed across farming systems, but these inputs did not vary much between tillage treatments (Figure 2, Table 2). Although manure applications were more expensive than mineral fertilizer application (an average of $452 ha−1 for manure, $318 ha−1 for mineral fertilizers applied to conventional maize), more frequent fertilizer applications in the CNV rotation meant average annual fertility costs were nearly 50% higher for the CNV system compared to the MNR system. Regional differences in manure costs could further increase or decrease the difference in fertility costs between the CNV and MNR systems (Delate et al., 2003; Carr et al., 2020). Minimal fertility inputs in the low-input LEG rotation led to notably lower fertility costs in the LEG system compared to the CNV (−67%) and MNR systems (−52%).
Seed costs were the lowest in the MNR system (Table 2). Despite the higher cost of GM maize and soybean seeds, limited cover crop use meant seed costs were 10% lower in the CNV system compared to the LEG system. The long hay phase of the MNR system kept seed costs 16% lower than in the CNV system.
Input costs associated with pest management averaged around $4,272 in the CNV treatments, largely as herbicide inputs for weed control. Pest management inputs were negligible in the two organic systems. Including the labor and field operations associated with weed management (cultivation or herbicide applications), overall weed management costs were nearly four times higher in the CNV system (FT-CNV = $5,332, RT-CNV = $5,256) compared to the FT organic treatments (FT-LEG = $1,465, FT-MNR = $1,195) and over eleven times higher than the RT organic treatments (RT-LEG = $455, RT-MNR = $478). Reducing tillage did decrease weed management costs in the LEG system by 18% but did not appreciably affect weed management costs in the CNV or MNR systems.
3.2.3. Total costs
Excluding land (rent) costs (average of $567 ha−1 year−1), annual field operations and seed costs were the most substantial cost categories. Overall, total annual costs (sum of land, labor, field operations, inputs [seeds, fertility, pest management], and other costs [insurance, interest, and miscellaneous costs]) were notably different across the three farming systems and between tillage treatments within each system (Figure 2, Table 2). Even when accounting for very different organic-management strategies (i.e., different tillage practices, crop rotations, and fertility sources), total costs were comparable between the two organic systems and only 9% lower compared to conventional management. Although no-till management reduced total costs for the representative NT-CNV farms compared to FT-CNV (−5%), annual costs were still around 7% higher under NT-CNV management compared to organic management (Figure 2). Reducing tillage also lowered total costs under LEG management (−6%) but only decreased total costs under MNR management by 2%.
3.3. Gross revenues and net returns
Both with and without organic premiums, economic returns differed across the representative farms that employed different management and tillage strategies (Figure 3; Table 3). When organic price premiums were applied to the grain and forage produced in the organic systems, both organic farms had higher gross and net returns than the CNV farm (Figures 3A, C; Table 3; gross returns: CNV = $84,807, LEG = $102,228, MNR = $124,716; net returns: CNV = $7,651, LEG = $32,882, MNR = $53,994); on average, LEG and MNR management supported 21 and 47% greater gross revenues than CNV management, respectively. Organic premiums increased gross revenue in the LEG and MNR systems by 81 and 47%, respectively (Figures 3A, B), and with lower costs under organic management, cumulative net returns under MNR management were over 7 times higher than under CNV management. If the organic grain and forage were sold at conventional prices without organic premiums, the LEG system would have generated net losses (–$12,924). As overall relative yields were higher in the MNR system compared to the CNV system (largely driven by high-value hay) the MNR system would have generated 45% higher net returns than the CNV system even if the organic grains and forages were sold at conventional prices (Figure 3D; MNR = $13,859, CNV = $7,651; t-ratio = −10.4; P = 0.07). Reducing tillage led to lower gross revenue under both CNV and LEG management but did not significantly affect gross revenue under MNR management (Figure 3, Table 3; −10% in CNV, −13% in LEG). Net returns, however, did not significantly differ between tillage treatments (Figures 3C, D).
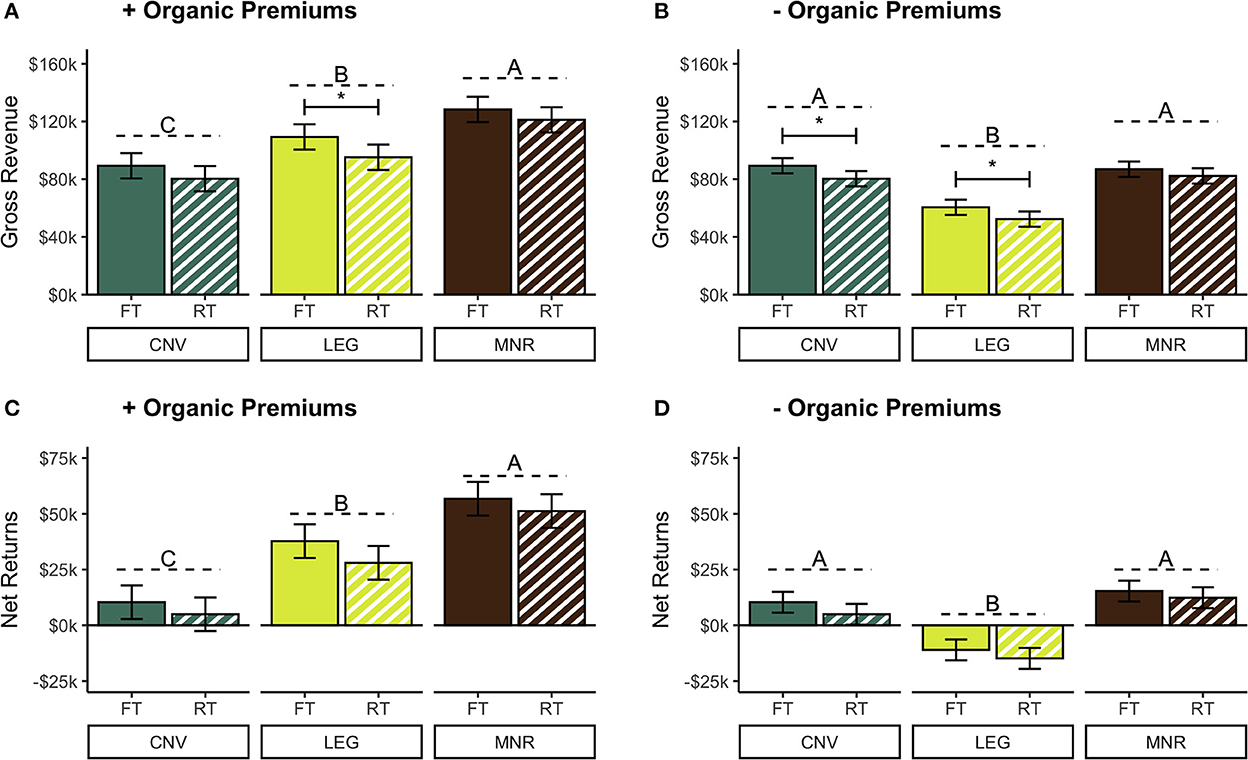
Figure 3. Average annual gross revenue and net returns for each representative farm with different tillage × system treatments (from 2008 to 2013, 2016 to 2020). Gross revenue (A) with and (B) without organic premiums, net returns (gross revenue – total costs) (C) with and (D) without organic premiums. Error bars are standard errors, different letters indicate significant differences across systems (Tukey HSD, P < 0.05), while asterisks indicate significant differences between tillage treatments within each system (at P < 0.05).
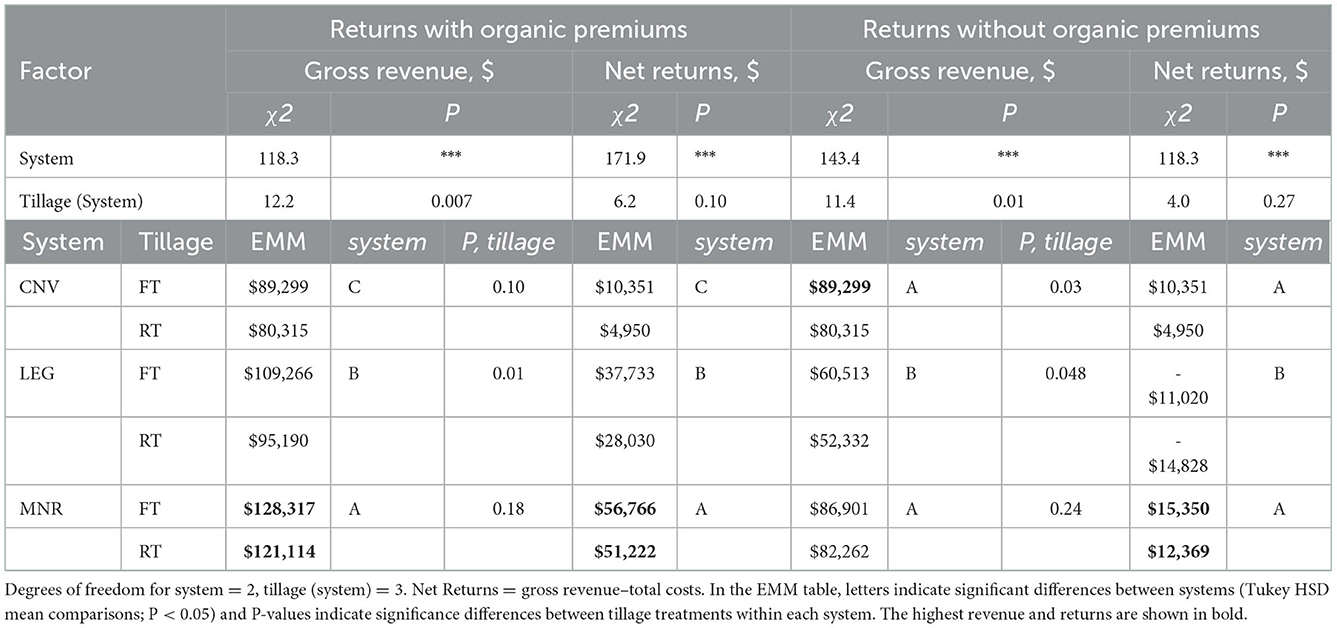
Table 3. ANOVA tables and estimated marginal mean values (EMM) for differences in annual gross revenue and net returns for representative farms with different tillage × system treatments, with tillage nested in system and denoted as tillage (system).
3.4. Risk and sensitivity analysis
Year-to-year variability (SD) and risk (L) differed for the three farming systems, but reducing tillage had a much smaller effect on year-to-year variability of net returns. Regardless of tillage practices, CNV management was the most stable, as year-to-year variability in net returns was 41–55% lower than that of the organically managed farms (Table 4). Despite high year-to-year variability in net returns, however, organic farms were lower-risk options. All four organic farms had positive values for 75% lower risk limits while the two conventional farms had negative values (Table 4). Lower risk limits were higher under organic management because high cumulative net returns compensated for high year-to-year variability. Values for 75% lower risk limits were consistently lower where tillage was reduced in all three systems, so reducing tillage may increase system risk.
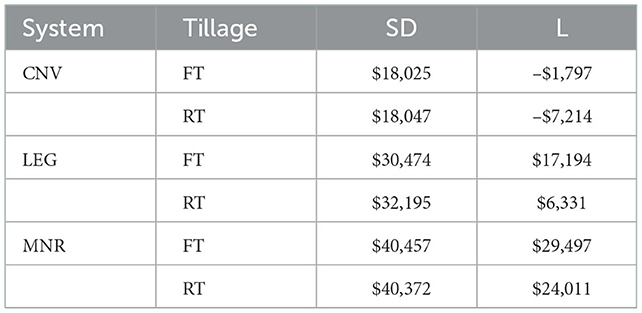
Table 4. Year-to-year variability (SD, standard deviation) and 75% lower confidence limits (L) for net returns in each tillage × system treatments.
The four organic systems demonstrated different sensitivities to organic price premiums (Figure 4). Regardless of organic price premiums, the FT-MNR and RT-MNR treatments generated higher net returns than the FT-CNV and RT-CNV treatments. For the FT-LEG and RT-LEG treatments, organic price premiums would have to drop over 56 or 41%, respectively, for net returns to drop below the returns of the FT-CNV treatment.
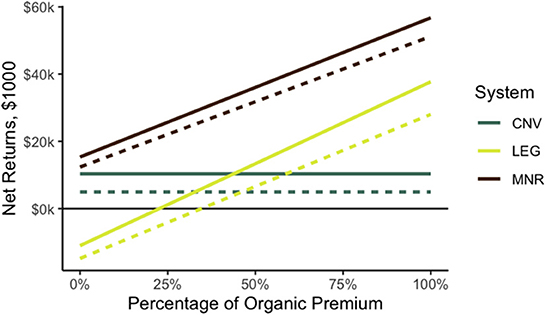
Figure 4. Sensitivity of net returns (NR) to changes in organic price premiums from 0 to 100% (0% = conventional prices, 100% = organic prices). Trends for each treatment: NRFT−CNV = $10,351; NRRT−CNV = $4,950; NRFT−LEG = –$11,020 + $48,753[%]; NRRT−LEG = –$14,828 + $42,858[%]; NRFT−MNR = $15,350 + $41,416[%]; NRRT−MNR = $12,369 + $38,853[%].
4. Discussion
We expected lower crop yields under reduced-till management but the corresponding reduction in management costs would more-than-compensate for decreased revenues on the representative farms. Although field operation and labor costs were lower under reduced-till management, these cost savings did not over-compensate for decreased revenue and did not lead to significantly higher net returns. The MNR system supported the most days of continuous living cover and was the overall most profitable system, but reducing tillage did not appreciably affect management costs, gross revenues, or net returns for the representative RT-MNR farms. For the representative CNV and LEG farms, reducing tillage did negatively affect overall gross revenues (−10% in CNV, −13% in LEG), which drove down gross revenues compared to the FT-CNV and FT-LEG treatments.
For the CNV system, reducing tillage marginally reduced maize yields. RT systems often require co-adoption of high residue retention (Pittelkow et al., 2015) and/or continuous living cover provided by winter cover crops (Marcillo and Miguez, 2017) to maintain high maize yields. As noted in the methods section, the RT-CNV treatment only had living cover for an average of 8.4 months of the year. Compared to the RT organic systems, this left an additional 2 months without living cover–a higher risk for erosion, nutrient loss, and missed opportunities to build soil health (Dabney et al., 2001). The LEG system, despite supporting continuous living cover for over 10 months of the year, did not support robust crop growth and high yields (Pearsons et al., 2022). The low-input design of the LEG system did help minimize fertility costs, but such low inputs significantly limited crop productivity. In past assessments of the FST, the LEG system was able to support comparable yields to the CNV system (Hanson et al., 1997), but decades of relying on biological N fixation may have limited long-term N, P, and K availability in this low-input system (Reimer et al., 2020).
Like with gross revenues, overall costs were notably lower in the RT-CNV and RT-LEG treatments compared to their FT counterparts. These cost savings, however, were smaller than hypothesized, sitting around 5%. In the CNV system, input costs accounted for a greater proportion of total costs (37%) than field operations and labor (18% of total costs). Eliminating tillage operations in the RT-CNV treatment reduced field operation and labor costs by 16.3% but did not have a substantial effect on herbicide use, seed inputs, or inorganic fertilizer inputs. Reducing tillage may have even small effects on conventional management costs in future years, as herbicide-resistant weeds (Reddy and Norsworthy, 2010) and emerging nutrient deficiencies (Elkin et al., 2016) drive up input costs relative to field operations and labor, but only if these costs outpace rising labor and fuel costs (White et al., 2019).
For the LEG system, input costs accounted for a smaller proportion of total costs (23%) than field operations and labor (25%), which presented a greater opportunity for reducing tillage to affect total management costs. Indeed, despite fewer opportunities to reduce tillage compared to the CNV system (15–30% of planting events), the associated field operation and labor cost savings of reducing tillage (−22%) had a slightly greater effect on total costs (−6%) under LEG management.
Due to the multiyear hay phase of the MNR crop rotation, tillage intensity in the FT-MNR treatment was more similar to the RT-LEG treatment than the FT-LEG treatment. This meant there were fewer opportunities to further reduce tillage in the RT-MNR treatment, and therefore fewer opportunities for costs to differ between the MNR treatments.
Despite lower overall costs for the two organic systems, field operation and labor costs were notably higher compared to the conventional system. Most of these additional field operation and labor costs were associated with cover crop establishment. Although cover crops can provide numerous agronomic and environmental benefits, the added costs to establish cover crops may be a barrier to adoption (Roesch-McNally et al., 2018). Unlike cover crops, adding perennial crops to a cropping system (e.g., hay in the MNR system) can increase continuous living cover and inherently reduces tillage activity, without the added cost of cover crop seeds or no-till specific equipment (e.g., no-till planters, roller-crimpers, and high residue cultivators). Perennial hay production also reduced labor costs and crop seed costs across the MNR rotation. High hay yields and value helped drive the MNR system's profitability, and years of continuous living cover likely supported the high maize, soybean, and small grain yields in the MNR system. As long as farmers have the equipment, time, and interest in hay production (Olmstead and Brummer, 2008), perennial hay production can be a valuable addition to organic and conventional farming systems.
This perennial hay production, however, meant the MNR crop rotation presented fewer opportunities to further reduce tillage compared to the rotations used in the CNV and LEG systems. Overall, net returns in the MNR treatments were more reflective of overall lower costs associated with organic production, manure fertilization, high organic premiums, and high hay yields rather than tillage practices. Strategies to reduce tillage may be best applied to crop rotations which present more opportunities to reduce tillage, such as simple maize-soybean rotations. Efforts to reduce tillage in more diverse organic rotations (i.e., no-till planting cover crops, small grains, and hay) would help to further reduce costs and increase benefits of reducing tillage under organic management.
Regardless of tillage practices, the effect of organic management on costs and net returns was largely consistent with past economic analyses of the FST (Hanson et al., 1990, 1997), other long-term trials (Delate et al., 2003; Chavas et al., 2009; White et al., 2019), and meta-analyses (Crowder and Reganold, 2015; McBride et al., 2015). Generally, field operations and labor costs are higher under organic management, but lower inputs and high organic premiums offset these added costs.
From 1982 to 1984, seed costs, field operation, and labor costs were higher for the LEG system compared to the CNV system (by 23, 4, and 20%, respectively; Hanson et al., 1997), while fertilizer and pest management inputs were only a factor for the CNV system (The MNR system was not included in this initial analysis; Hanson et al., 1997). While the magnitude of these differences changed over four decades, seed costs, field operation, and labor costs were still higher for the LEG system (by 10, 21, and 75%, respectively) while fertilizer and pest management inputs remained higher for the CNV system (by 67 and 96%, respectively). At the start of the FST overall costs were about 18% lower for the LEG system compared to the CNV system (Hanson et al., 1997). Decades later, overall costs were still lower for the LEG system, but this cost advantage had dropped to just over 10%. This decrease can partly be attributed to the fertility and pest management inputs in the LEG system but is mostly reflective of how rising fuel and labor costs have a stronger impact on organic systems. Other long-term trials have similarly observed how fertility inputs and pest management drive up overall costs under conventional management, despite higher field operation and labor costs under organic management (Delate et al., 2003; Crowder and Reganold, 2015; White et al., 2019).
Also consistent with the first economic assessment of the FST (covering the first 9 years after establishment, 1981–1989; Hanson et al., 1990; Roberts and Swinton, 1995), the low-input LEG system continued to be a less risky option than CNV management. In the initial analysis, the LEG system was considered less risky because annual net returns were more stable in the LEG system compared to the CNV system (Hanson et al., 1990; Roberts and Swinton, 1995). After three decades, net returns destabilized in the LEG system (greater year-to-year variability), but higher prices for organic grain meant the more variable, low-input, and lower-yielding LEG system was still a lower-risk option compared to the CNV system. With the highest annual net returns and highest lower limit of net returns, the system with the most diverse crop rotation–the MNR system–would be considered the least risky option based on the safety-first method (Musser et al., 1981). Other economic analyses have similarly concluded that organic systems with long, diverse crop rotations can be lower risk than conventionally managed systems (White et al., 2019). Although high crop diversity will usually increase the year-to-year variability of costs and gross revenues, high diversity can help buffer against fluctuating crop prices and crop failures.
The most notable difference between the first economic assessment of the FST and this current one is how price premiums affect organic system returns. In the first economic assessment of the FST, the LEG system generated similar net profits as the CNV system, without organic price premiums (Hanson et al., 1997). With the decreased cost advantage (from 18 to 10%) and lower yields compared to the CNV system, the LEG system became reliant on organic price premiums. As demonstrated by the sensitivity analysis, however, both the FT-LEG and RT-LEG treatments would remain competitive unless price premiums were reduced by over 56 or 41%, respectively. Additionally, the MNR system generated similar or higher net returns as the CNV system, regardless of price premiums. Similar studies and meta-analyses have found that organic systems can be profitable at lower price premiums as long as crop yields are supported by sufficient fertility inputs and timely pest management (Delate et al., 2003; Crowder and Reganold, 2015; White et al., 2019).
4.1. Implications for farmer decision-making
For reducing tillage to positively affect net returns, reduced-till strategies must substantially decrease input costs or increase gross revenue. High input costs (seeds, fertility, and pest management) can overshadow the economic benefits of reducing tillage in conventional systems, whereas limited opportunities to reduce tillage can overshadow the economic benefits of reducing tillage in diverse organic systems. With no significant negative effect on overall crop yields, net returns, nor overall economic risk, the decision to reduce tillage will likely depend on farmers' capital, labor availability, or non-economic factors such as soil conservation.
Grain farmers are often limited by capital (Baumgart-Getz et al., 2012; Roesch-McNally et al., 2018), which can incentivize practices that reduce overall input and operation costs, even if those practices have limited effects on yields or profits. As reducing tillage did not affect net returns or overall yields, the lower operation and labor costs of the RT-CNV and RT-LEG treatments could be an incentive for both conventional and organic producers to reduce tillage. Other studies have found similar cost-related benefits of reducing tillage in conventional systems, especially as rising fuel costs have led tillage and cultivation operations to account for a growing proportion of overall production costs (Chavas et al., 2009; White et al., 2019). Limited capital, however, could also be a significant barrier for farmers to lease or purchase the equipment needed to reduce tillage (e.g., no-till planter, roller crimper, and high reside cultivator).
Although labor accounted for <5% of total costs, labor can play a disproportionate role for farmers that rely on off-farm income (Lee and McCann, 2019). For farms of similar size to the theoretical farms in this economic analysis (54 ha), most labor is provided by farmers who have primary occupations beyond farming (for farms between 40 and 56 ha, <25% used hired labor and 61% of operators spent more than half of their time earning off-farm income; NASS, 2019). Farmers that rely on off-farm income may be inclined to adopt practices that reduce labor inputs, such as reduced-till production. Reduced-till organic management could provide a less labor-intensive option for conventional farmers interested in transitioning to organic production, which is consistently shown to have higher labor requirements compared to conventional production (Hanson et al., 1990, 1997; Delate et al., 2003; Crowder and Reganold, 2015; White et al., 2019). Additionally, conventional farmers that are interested in organic production but do not want to move away from RT practices (Smith et al., 2011) may be encouraged by the overall lower costs and similar net returns between the RT and FT organic systems. The high profitability (net returns) of the MNR system especially indicates that manure inputs in combination with continuous living cover (perennial hay) into long and diverse crop rotations may be the most profitable production strategy from the FST. The similarity between yields in the CNV system and county averages (CNVrelative = 1.04 ± 0.06) indicate that the conditions of the FST experiment are representative of how these systems would perform at a larger scale in the mid-Atlantic region, so it follows that similar yields could be achieved on farms modeled after the RT-MNR system.
4.2. Conclusion
Overall, this updated economic analysis of the FST reinforces findings that organic field crop production can be economically favorable compared to conventional field crop production. Although reducing tillage does not appear to affect the profitability of organic field crop production, there are clear long-term economic benefits for grain farmers to transition to organic production. The decision to also reduce tillage, however, will depend on the specific goals and resources of an individual farmer and may be driven more by prospects of improved soil health rather than economics (Zikeli and Gruber, 2017). As price premiums were not the only driver of high profits in the MNR system, conventional farmers and organic farmers alike may be encouraged to adopt some of the conservation-based practices employed in this organic system–long and diverse crop rotations, continuous living cover, and organic fertility inputs–in pursuit of more environmentally and economically sustainable agriculture.
Data availability statement
The raw data supporting the conclusions of this article will be made available by the authors, without undue reservation.
Author contributions
AS, EO, and YR: conceptualization and project administration. CC and KP: data curation. KP: formal analysis and visualization. AS, EO, GZ, and YR: funding acquisition. EO, GZ, KP, and YR: investigation. AS, CC, KP, and YR: writing–original draft. AS, EO, CC, GZ, KP, and YR: writing–review and editing. All authors have read and agreed to the published version of the manuscript.
Funding
This material is based upon work supported by the William Penn Foundation under Grant Award Number 188-17. Additional funding was provided by the Rockefeller Foundation, Towards Sustainability Foundation, and the Blooming Prairie Foundation.
Acknowledgments
We would like to acknowledge Dr. Mark Hanna and Dr. D. D. Lingenfelter for providing reliable cost estimates for labor and herbicide inputs. Additionally, we would like to thank the farm operations team, researchers, technicians, and interns that have kept FST running for the past 40 years, especially farm managers: Ross Duffield, Daniel Kemper, Rick Carr, and Jeff Moyer; research staff: Dr. Paul Hepperly, Rita Seidel, Dr. David Schmeisser, Christine Ziegler, Alex Dutt, Jessica Lang, and Anne Benson. We thank Dr. Reza Afshar for his insights and feedback while drafting this manuscript.
Conflict of interest
The authors declare that the research was conducted in the absence of any commercial or financial relationships that could be construed as a potential conflict of interest.
Publisher's note
All claims expressed in this article are solely those of the authors and do not necessarily represent those of their affiliated organizations, or those of the publisher, the editors and the reviewers. Any product that may be evaluated in this article, or claim that may be made by its manufacturer, is not guaranteed or endorsed by the publisher.
Author disclaimer
The opinions expressed in this publication are those of the author(s) and do not necessarily reflect the views of the William Penn Foundation, Rockefeller Foundation, Towards Sustainability Foundation, or Blooming Prairie Foundation.
Supplementary material
The Supplementary Material for this article can be found online at: https://www.frontiersin.org/articles/10.3389/fsufs.2022.1004256/full#supplementary-material
References
Alford, A. M., and Krupke, C. H. (2018). A meta-analysis and economic evaluation of neonicotinoid seed treatments and other prophylactic insecticides in indiana maize from 2000–2015 with IPM recommendations. J. Econ. Entomol. 111, 689–699. doi: 10.1093/jee/tox379
AMS (2021). Market News: Custom Reports Portal. Available online at: https://marketnews.usda.gov/mnp/ls-report-config (accessed August 6, 2021).
Archer, D. W., and Reicosky, D. C. (2009). Economic performance of alternative tillage systems in the northern corn belt. Agron. J. 101, 296–304. doi: 10.2134/agronj2008.0090x
Ashford, D. L., and Reeves, D. W. (2003). Use of a mechanical roller-crimper as an alternative kill method for cover crops. Am. J. Altern. Agric. 18, 37–45. doi: 10.1079/AJAA2003037
Bates, D., Mächler, M., Bolker, B., and Walker, S. (2015). Fitting linear mixed-effects models using lme4. J. Stat. Softw. 67, 1–48. doi: 10.18637/jss.v067.i01
Baumgart-Getz, A., Prokopy, L. S., and Floress, K. (2012). Why farmers adopt best management practice in the United States: A meta-analysis of the adoption literature. J. Environ. Manage. 96, 17–25. doi: 10.1016/j.jenvman.2011.10.006
Benbrook, C. M. (2016). Trends in glyphosate herbicide use in the United States and globally. Environ. Sci. Eur. 28, 3. doi: 10.1186/s12302-016-0070-0
Carr, P. M., Cavigelli, M. A., Darby, H., Delate, K., Eberly, J. O., Fryer, H. K., et al. (2020). Green and animal manure use in organic field crop systems. Agron.j. 112, 648–674. doi: 10.1002/agj2.20082
Chase, C. (2020). Using whole farm and enterprise records to make decisions. IOWA State Univ. Exten. Outreach. FFED33, 1–6.
Chase, C., Delate, K., Hanlon, O., and Topaloff, A. (2019). Organic crop production enterprise budgets. IOWA State Univ. Exten. Outreach FFED0027, 1–7.
Chavas, J. P., Posner, J. L., and Hedtcke, J. L. (2009). Organic and conventional production systems in the Wisconsin Integrated Cropping Systems Trial: II. economic and risk analysis 1993–2006. Agron. J. 101, 288–295. doi: 10.2134/agronj2008.0055x
Claassen, R., Bowman, M., McFadden, J., Smith, D., and Wallander, S. (2018). Tillage Intensity and Conservation Cropping in the United States. USDA, Economic Research Service Economic Information Bulletin Number 197.
Crowder, D. W., and Reganold, J. P. (2015). Financial competitiveness of organic agriculture on a global scale. Proc. Natl. Acad. Sci. U. S. A. 112, 7611–7616. doi: 10.1073/pnas.1423674112
Dabney, S. M., Delgado, J. A., and Reeves, D. W. (2001). Using winter cover crops to improve soil and water quality. Commun. Soil Sci. Plant Anal. 32, 1221–1250. doi: 10.1081/CSS-100104110
Deines, J. M., Wang, S., and Lobell, D. B. (2019). Satellites reveal a small positive yield effect from conservation tillage across the US Corn Belt. Environ. Res. Lett. 14, 124038. doi: 10.1088/1748-9326/ab503b
Delate, K., Cwach, D., and Chase, C. (2012). Organic no-tillage system effects on soybean, corn and irrigated tomato production and economic performance in Iowa, USA. Renew. Agric. Food Syst. 27, 49–59. doi: 10.1017/S1742170511000524
Delate, K., Duffy, M., Chase, C., Holste, A., Friedrich, H., and Wantate, N. (2003). An economic comparison of organic and conventional grain crops in a long-term agroecological research (LTAR) site in Iowa. Am. J. Altern. Agric. 18, 59–69. doi: 10.1079/AJAA200235
Delbridge, T. A., Coulter, J. A., King, R. P., Sheaffer, C. C., and Wyse, D. L. (2011). Economic performance of long-term organic and conventional cropping systems in minnesota. Agron. J. 103, 1372–1382. doi: 10.2134/agronj2011.0371
Donley, N. (2019). The USA lags behind other agricultural nations in banning harmful pesticides. Environ. Health 18, 44. doi: 10.1186/s12940-019-0488-0
Douglas, M. R., and Tooker, J. F. (2015). Large-scale deployment of seed treatments has driven rapid increase in use of neonicotinoid insecticides and preemptive pest management in U.S. field crops. Environ. Sci. Technol. 49, 5088–5097. doi: 10.1021/es506141g
Downing, T., Chamberlain, A.-M., Gamroth, M., and Peters, A. (2013). What Are Your Forages Worth? Oregon State University. PNW 259, 1–4.
Duffy, M. (2009). Estimated Costs of Crop Production in Iowa - 2010. Iowa State University Extension and Outreach. Ag Decision Maker. File A1-20, 1–12.
Duffy, M. (2011). Estimated Costs of Crop Production in Iowa - 2011. Iowa State University Extension and Outreach. Ag Decision Maker, File A1-20, 1–13.
Duffy, M. (2012). Estimated Costs of Crop Production in Iowa - 2012. Iowa State University Extension and Outreach. Ag Decision Maker, File A1-20, 1–13.
Duffy, M. (2013). Estimated Costs of Crop Production in Iowa - 2013. Iowa State University Extension and Outreach. Ag Decision Maker, File A1-20, 1–13.
Duffy, M., and Smith, D. (2008a). Estimated Costs of Crop Production in Iowa - 2008. Iowa State University Extension and Outreach. Ag Decision Maker, File A1-20, 1–12.
Duffy, M., and Smith, D. (2008b). Estimated Costs of Crop Production in Iowa - 2009. Iowa State University Extension and Outreach. Ag Decision Maker, File A1-20, 1–12.
Elkin, K. R., Veith, T. L., Lu, H., Goslee, S. C., Buda, A. R., Collick, A. S., et al. (2016). Declining atmospheric sulfate deposition in an agricultural watershed in central Pennsylvania, USA. Agric. Environ. Lett. 1, 160039. doi: 10.2134/ael2016.09.0039
Finger, R., El Benni, N., Kaphengst, T., Evans, C., Herbert, S., Lehmann, B., et al. (2011). A Meta analysis on farm-level costs and benefits of GM Crops. Sustainability 3, 743–762. doi: 10.3390/su3050743
Frasconi, C., Martelloni, L., Antichi, D., Raffaelli, M., Fontanelli, M., Peruzzi, A., et al. (2019). Combining roller crimpers and flaming for the termination of cover crops in herbicide-free no-till cropping systems. PLoS ONE 14, e0211573. doi: 10.1371/journal.pone.0211573
Gagnon, A.-È., Audette, C., Duval, B., and Boisclair, J. (2016). Can the use of trichogramma ostriniae (hymenoptera: trichogrammatidae) to control ostrinia nubilalis (lepidoptera: crambidae) be economically sustainable for processing sweet corn? J. Econ. Entomol. 110, 59–66. doi: 10.1093/jee/tow293
Gomiero, T., Pimentel, D., and Paoletti, M. G. (2011). Environmental impact of different agricultural management practices: conventional vs. organic agriculture. CRC. Crit. Rev. Plant Sci. 30, 95–124. doi: 10.1080/07352689.2011.554355
Hanna, M. (2016). Estimating the Field Capacity of Farm Machines. Iowa State University Extension and Outreach. Ag Decision Maker, File A3-24, 1–5.
Hanson, J. C., Johnson, D. M., Peters, S. E., and Janke, R. R. (1990). The profitability of sustainable agriculture on a representative grain farm in the Mid-Atlantic Region, 1981–89. Northeast. J. Agric. Resour. Econ. 19, 90–98. doi: 10.1017/S0899367X00002154
Hanson, J. C., Lichtenberg, E., and Peters, S. E. (1997). Organic vs. conventional grain production in the mid-Atlantic: An economic and farming system overview. Am. J. Altern. Agric. 12, 2–9. doi: 10.1017/S0889189300007104
LaCanne, C. E., and Lundgren, J. G. (2018). Regenerative agriculture: merging farming and natural resource conservation profitably. PeerJ. 6, e4428. doi: 10.7717/peerj.4428
Lee, S., and McCann, L. (2019). Adoption of cover crops by U.S. Soybean Producers. J. Agric. Appl. Econ. 51, 527–544. doi: 10.1017/aae.2019.20
Lenth, R. V. (2021). emmeans: Estimated Marginal Means, aka Least-Squares Means. Available online at: https://CRAN.R-project.org/package=emmeans (accessed October 06, 2021).
Marcillo, G. S., and Miguez, F. E. (2017). Corn yield response to winter cover crops: an updated meta-analysis. J. Soil Water Conserv. 72, 226–239. doi: 10.2489/jswc.72.3.226
McBride, W. D., Greene, C., Foreman, L., and Ali, M. (2015). The Profit Potential of Certified Organic Field Crop Production. USDA, Economic Research Service Economic Research Report Number 188. doi: 10.2139/ssrn.2981672
Mirsky, S. B., Ryan, M. R., Curran, W. S., Teasdale, J. R., Maul, J., Spargo, J. T., et al. (2012). Conservation tillage issues: Cover crop-based organic rotational no-till grain production in the mid-Atlantic region, USA. Renew. Agric. Food Syst. 27, 31–40. doi: 10.1017/S1742170511000457
Moyer, J. (2021). Roller/Crimper No-Till: Advancing No-Till Agriculture – Crops, Soil, Equipment. Greenly, CO: Acres U.S.A.
Musser, W. N., Ohannesian, J., and Benson, F. J. (1981). A safety first model of risk management for use in extension programs. North Cent. J. Agric. Econ. 3, 41. doi: 10.2307/1349407
NASS (2019). 2017 Census of Agriculture: Pennsylvania State and County Data. United States Department of Agriculture, National Agricultural Statistics Service.
NASS (2021). Quick stats Database. Available online at: https://quickstats.nass.usda.gov/ (accessed August 6, 2021).
Olmstead, J., and Brummer, E. C. (2008). Benefits and barriers to perennial forage crops in Iowa corn and soybean rotations. Renew. Agric. Food Syst. 23, 97–107. doi: 10.1017/S1742170507001937
Palm, C., Blanco-Canqui, H., DeClerck, F., Gatere, L., and Grace, P. (2014). Conservation agriculture and ecosystem services: an overview. Agric. Ecosyst. Environ. 187, 87–105. doi: 10.1016/j.agee.2013.10.010
Pearsons, K. A., Omondi, E. C., Heins, B. J., Zinati, G., Smith, A., and Rui, Y. (2022). Reducing tillage affects long-term yields but not grain quality of maize, soybeans, oats, and wheat produced in three contrasting farming systems. Sustainability 14, 631. doi: 10.3390/su14020631
Pearsons, K. A., Omondi, E. C., Zinati, G., Smith, A., and Rui, Y. (2023). A tale of two systems: Does reducing tillage affect soil health differently in long-term, side-by-side conventional and organic agricultural systems? Soil Tillage Res. 226, 105562. doi: 10.1016/j.still.2022.105562
Peigné, J., Ball, B. C., Roger-Estrade, J., and David, C. (2007). Is conservation tillage suitable for organic farming? A review. Soil Use Manag. 23, 129–144. doi: 10.1111/j.1475-2743.2006.00082.x
Pittelkow, C. M., Liang, X., Linquist, B. A., van Groenigen, K. J., Lee, J., Lundy, M. E., et al. (2015). Productivity limits and potentials of the principles of conservation agriculture. Nature 517, 365–368. doi: 10.1038/nature13809
Plastina, A. (2016). Estimated Costs of Crop Production in Iowa - 2016. Iowa State University Extension and Outreach. Ag Decision Maker, File A1-20, 1–13.
Plastina, A. (2017). Estimated Costs of Crop Production in Iowa - 2017. Iowa State University Extension and Outreach. Ag Decision Maker, File A1-20, 1–13.
Plastina, A. (2018). Estimated Costs of Crop Production in Iowa - 2018. Iowa State University Extension and Outreach. Ag Decision Maker, File A1-20, 1–13.
Plastina, A. (2019). Estimated Costs of Crop Production in Iowa - 2019. Iowa State University Extension and Outreach. Ag Decision Maker, File A1-20, 1–13.
Plastina, A. (2020). Estimated Costs of Crop Production in Iowa - 2020. Iowa State University Extension and Outreach. Ag Decision Maker, File A1-20, 1–13.
Ramankutty, N., Mehrabi, Z., Waha, K., Jarvis, L., Kremen, C., Herrero, M., et al. (2018). Trends in global agricultural land use: Implications for environmental health and food security. Annu. Rev. Plant Biol. 69, 789–815. doi: 10.1146/annurev-arplant-042817-040256
Reddy, K. N., and Norsworthy, J. K. (2010). “Managing glyphosate-resistant weeds and population shifts in midwestern US cropping systems,” in: Glyphosate-resistant Crop Production Systems: Impact on Weed Species Shifts. eds. Nandula, VK. (Hoboken, United States: Wiley).
Reganold, J. P., and Wachter, J. M. (2016). Organic agriculture in the twenty-first century. Nat. Plants 2, 15221. doi: 10.1038/nplants.2015.221
Reimer, M., Hartmann, T. E., Oelofse, M., Magid, J., Bünemann, E. K., and Möller, K. (2020). Reliance on biological nitrogen fixation depletes soil phosphorus and potassium reserves. Nutr. Cycl. Agroecosyst. 118, 273–291. doi: 10.1007/s10705-020-10101-w
Roberts, W. S., and Swinton, S. M. (1995). The profitability of sustainable agriculture on a representative grain farm in the Mid-Atlantic Region, 1981–89: comment. Agric. Resour. Econ. Rev. 24, 136–137. doi: 10.1017/S1068280500003695
Roesch-McNally, G. E., Basche, A. D., Arbuckle, J. G., Tyndall, J. C., Miguez, F. E., Bowman, T., et al. (2018). The trouble with cover crops: Farmers' experiences with overcoming barriers to adoption. Renew. Agric. Food Syst. 33, 322–333. doi: 10.1017/S1742170517000096
Sanaullah, M., Usman, M., Wakeel, A., Cheema, S. A., Ashraf, I., and Farooq, M. (2020). Terrestrial ecosystem functioning affected by agricultural management systems: a review. Soil Tillage Res. 196, 104464. doi: 10.1016/j.still.2019.104464
Smith, R. G., Barbercheck, M. E., Mortensen, D. A., Hyde, J., and Hulting, A. G. (2011). Yield and net returns during the transition to organic feed grain production. Agron.J. 103, 51–59. doi: 10.2134/agronj2010.0290
Tilman, D., Cassman, K. G., Matson, P. A., Naylor, R., and Polasky, S. (2002). Agricultural sustainability and intensive production practices. Nature 418, 671–677. doi: 10.1038/nature01014
Toliver, D. K. (2010). Effects of no-tillage on crop yields and net returns across the United States. (Master's thesis). University of Tennessee, Knoxville, TN, United States.
Wallace, J., Williams, A., Liebert, J., Ackroyd, V., Vann, R., Curran, W., et al. (2017). Cover crop-based, organic rotational no-till corn and soybean production systems in the mid-Atlantic United States. Agriculture 7, 34. doi: 10.3390/agriculture7040034
White, K. E., Cavigelli, M. A., Conklin, A. E., and Rasmann, C. (2019). Economic performance of long-term organic and conventional crop rotations in the Mid-Atlantic. Agron. J. 111, 1358–1370. doi: 10.2134/agronj2018.09.0604
Wittwer, R. A., Bender, S. F., Hartman, K., Hydbom, S., Lima, R. A. A., Loaiza, V., et al. (2021). Organic and conservation agriculture promote ecosystem multifunctionality. Sci. Adv. 7, eabg6995. doi: 10.1126/sciadv.abg6995
Keywords: conservation tillage, no-till, continuous living cover, organic agriculture, economics, profitability
Citation: Pearsons KA, Chase C, Omondi EC, Zinati G, Smith A and Rui Y (2023) Reducing tillage does not affect the long-term profitability of organic or conventional field crop systems. Front. Sustain. Food Syst. 6:1004256. doi: 10.3389/fsufs.2022.1004256
Received: 27 July 2022; Accepted: 22 December 2022;
Published: 10 January 2023.
Edited by:
Carol Williams, University of Wisconsin–Madison, United StatesReviewed by:
Raghavendra Singh, Indian Institute of Pulses Research (ICAR), IndiaTim Delbridge, Oregon State University, United States
Copyright © 2023 Pearsons, Chase, Omondi, Zinati, Smith and Rui. This is an open-access article distributed under the terms of the Creative Commons Attribution License (CC BY). The use, distribution or reproduction in other forums is permitted, provided the original author(s) and the copyright owner(s) are credited and that the original publication in this journal is cited, in accordance with accepted academic practice. No use, distribution or reproduction is permitted which does not comply with these terms.
*Correspondence: Andrew Smith, YW5kcmV3LnNtaXRoQHJvZGFsZWluc3RpdHV0ZS5vcmc=; Yichao Rui,
cnVpeUBwdXJkdWUuZWR1
†Present addresses: Kirsten A. Pearsons, University of California Cooperative Extension, Salinas, CA, United States
Yichao Rui, Department of Agronomy, Purdue University, West Lafayette, IN, United States