- Agricultural Science and Engineering School, Liaocheng University, Liaocheng, China
Mung bean is rich in bioactive components, but the main compound and pharmacological mechanism in reducing oxidative and free radical damage are unclear. Network pharmacology and 2,2′-azino-bis-3-ethylbenzthiazoline-6-sulfonic acid (ABTS) radical scavenging activities were employed to uncover the antioxidant mechanism of potentially active compounds, considering the interactions between mung bean targets and oxidative and free radical damage. These key targets were analyzed by protein–protein interactions (PPIs), and key genes were used to find the biological pathway and therapeutic mechanism by Gene Ontology (GO) and Kyoto Encyclopedia of Genes and Genomes (KEGG) analyses. The results showed that five antioxidant components and 18 mung bean targets were screened. β-carotene and vitexin both played a crucial role in mung bean against oxidative and free radical damage, and the ABTS radical scavenging activities of β-carotene and vitexin were 94.84 and 87.79%, which were equivalent to those of vitamin C. Key targets may be AR, HSP90AA1, MYC, and CASP3 for mung bean to exert antioxidant activity. GO and KEGG indicated that mung bean may mainly act on thyroid hormone signaling pathway, estrogen signaling pathway, p53 signaling pathway, etc. In vitro antioxidant activity tests showed that the bioactive ingredients of mung beans had great antioxidant activity. Network pharmacology analysis also revealed the underlying molecular mechanisms of oxidative and free radical damage. This study provides new insights and evidence to explore the bioactive compounds and biological functions of food cereals and legumes, as well as a reference for the functional evaluation of food ingredients and the development of functional foods.
Introduction
Oxidative stress is caused by an imbalance between reactive oxygen species and antioxidants in the body's metabolic process, resulting in damage to tissue cells and biological macromolecules, which is an important risk factor in the development of many diseases. Therefore, improving the antioxidant capacity of the body is conducive to the maintenance of human health (Gomez-Cabrera et al., 2021). In the normal or abnormal (hypoxic) respiratory metabolism of biological organisms, reactive oxygen species are produced, among which free radicals are the main reactive oxygen substances (Koufen and Stark, 2000). Oxidative and free radical damage is closely related to many diseases and aging in human beings. When the number of free radicals in the body exceeds the normal range, there will be a chain reaction, free radicals snatch electrons from other substances, originally stable substances become unstable, triggering functional disorders in the body. Almost all biomolecules, such as DNA, proteins, lipids, sugars, and organic acids in cells may be attacked and subjected to extreme damage (Battino et al., 1999). These changes cause cell denaturation and necrosis, leading to the occurrence of aging of the whole organism (Harman, 2003). A large number of studies have revealed the mechanism of reactive oxygen species, such as free radicals and oxidative stress state, leading to chronic inflammation, while chronic inflammation is the main risk factor causing metabolic diseases, such as cancer, diabetes, cardiovascular diseases, neurological diseases, and lung diseases (Reuter et al., 2010). Free radical scavengers, also known as antioxidants, are substances that eliminate the damage caused by oxidative and free radical damage and can combine with free radicals to ensure their electrical balance before they can grab the electron from others (Haider et al., 2020). Oxidative and free radical damage led to body aging and the occurrence of chronic diseases, so the use of natural free radical scavengers and the development of new functional foods for antioxidants to maintain health have become important research topics.
Legumes are the main food source of protein, carbohydrates, fiber, and vitamins, and have been consumed by the Chinese for thousands of years (Yang et al., 2014). Clinical evidence showed that legumes have potential health benefits, and the benefits of legumes are phytochemicals that have antioxidant properties (Milenkovic et al., 2017). These antioxidants that are derived from food legumes are positively correlated with the lower occurrence of degenerative diseases, such as diabetes, cancer, arthritis, and Alzheimer's disease (Makiuchi et al., 2017; Singh et al., 2017; Xu et al., 2021). More interest in the potential consumption of food legumes has focused on the development of increasing functional ingredients with healthy and healing potential (Miceli et al., 2016; Ganesan and Xu, 2018). Mung bean (Vigna radiate L.) is an important short-season and summer-growing dietary grain legume (Ganesan and Xu, 2018). Mung bean is rich in bioactive components, which have different health benefits, such as detoxification, alleviating summer heatstroke, antioxidant, antidiabetic, and anticancer effects (Heim et al., 2002; Liu et al., 2013; Yang et al., 2020). Importantly, mung bean extracts possessed significantly higher antioxidants and levels of polyphenols than soybean extracts, which showed that they might be superior functional foods (Tang et al., 2014). In mung bean, 19 kinds of major phenolic compounds, including vitexin, ferulic, and chlorogenic acid, were quantified (Xu et al., 2021). In addition, the content of β-carotene, phytosterol, vitamin E, and tryptophan of mung bean in 100 g of the edible portion from previous studies were 68 μg, 23 mg, 0.51 mg, and 0.26 g, respectively (Ganesan and Xu, 2018). However, the underlying molecular mechanisms through specific compounds in legumes, such as mung bean, that exert antioxidant benefits on human health remain largely unexplored.
With the rapid progress of bioinformatics, systems biology, and pharmacology, network-based profiling is considered a cost-effective strategy to guide the action mechanism study of bioactive compounds, in which novel targets of known compounds can be identified in a highly efficient manner (Zhang F. X. et al., 2021). The proteins to which active compounds bind form identifiable neighborhoods in the human interactome, so the interaction between the targets of the active compounds and the proteins associated with specific diseases are predictive of the known therapeutic effects of the active compounds (Valle et al., 2021). Network pharmacology is a new in silico target profile to guide the study of the disease treatment mechanism of bioactive compounds in traditional Chinese medicine (Zhao et al., 2015). The interaction between the targets of the active compounds and the proteins associated with oxidative and free radical damage might predict the known therapeutic effects of the active compounds. Therefore, network pharmacology was employed to predict mung bean processed and related biological pathways related to oxidation resistance of human diseases in this study. And, the prediction of the active compound on the potential antioxidant effect verified by 2,2′-azino-bis-3-ethylbenzthiazoline-6-sulfonic acid (ABTS) radical scavenging activity. This research is expected to have the reference for mechanism interpretation of health effects underlying food-related compounds.
Materials and methods
Materials
Sitosterol, vitexin, and Boc-D-Trp-OH were purchased from Shanghai Macklin Biochemical Co., Ltd. (Shanghai, China). Vitamins C and E were purchased from Beijing Biotopped Science and Technology Co., Ltd. (Beijing, China). β-carotene was purchased from Shanghai Yuanye Bio-Technology Co., Ltd. (Shanghai, China). All other chemicals used in these experiments were of analytical grade.
Acquisition of active compounds and targets of mung bean
The active components of mung bean were retrieved from the Traditional Chinese Medicine Database and Analysis Platform (TCMSP) database (http://www.tcmspw.com/tcmsp.php) (Ru et al., 2014), then screened according to oral availability (OB) ≥30% and drug-like (DL) ≥0.18 (Lv et al., 2020), and their structure was obtained from molecule information in the TCMSP database. To find the targets related to diabetes in the category of protein coding, we searched the GeneCards database (https://www.genecards.org/) with “oxidative and free radical damage” as the keyword (Song et al., 2020).
Compound-target network construction
The mung bean compound–target network was established using Cytoscape 3.8.2 software (Bethesda, MD, USA), which performed network, visualization, and topological analyses (Pan et al., 2020).
ABTS radical scavenging activity
Antioxidant activities of sitosterol, β-carotene, vitamin E, vitexin, and Boc-D-Trp-OH were measured according to the method described by Dudonné et al. (2009) with modifications. Approximately 2.7 ml of ABTS working solution and 0.3-ml sample solutions [dissolved in dimethyl sulfoxide (DMSO)] were mixed and incubated for 30 min in the dark. Vitamin C was used as positive control.
Construction of a protein–protein interaction network
A protein–protein interaction (PPI) network was obtained using STRING (http://string-db.org/cgi/input.pl). The species was set to “Homo sapiens,” minimum interaction threshold was selected as “medium confidence >0.4,” and the other parameters were kept in the default setting (Wang et al., 2020). In the network, each node represented a target protein, and each edge represented the PPI (Yi et al., 2021).
Gene ontology and Kyoto encyclopedia of genes and genomes pathway enrichment analyses
To elucidate the function of metabolites and carry out the enrichment analysis of the biological pathway, Gene Ontology (GO) and Kyoto Encyclopedia of Genes and Genomes (KEGG) pathway enrichment analyses were performed. The intersection targets were input into R (R X64 4.0.2) for enrichment analysis of GO and KEGG pathways, the first 20 channels were selected (Luo et al., 2021). A value of p < 0.05 was considered statistically significant.
Statistical analysis
Experimental data were processed by one-way analysis of variance (ANOVA) using IBM SPSS Statistics 20 (IBM, NY, USA) with Duncan's multiple range test (p < 0.05). The results were reported as mean ± standard deviation (SD).
Results and discussion
Collection and screening of active compounds and construction of compound–target network
A total of six active compounds of mung bean were collected with oral bioavailability ≥30% and DL ≥0.18 from the TCMSP database (Xia et al., 2020). Specific information of the active compounds is listed in Table 1. Compound-related targets obtained from the TCMSP database were verified using the UniProt protein database and converted into their corresponding gene names, and the targets related to oxidative and free radical damage were acquired by retrieving GeneCards (Wang et al., 2021).
After removing the compounds without targets, the compound–target network was developed, involving 23 nodes (including five compounds and 18 genes) and 20 edges (Figure 1), the top compounds were β-carotene, vitexin_qt, vitamin E, Boc-D-Trp-OH, and PQH. The genes, NCOA2, BCL2, CASP9, CASP3, CASP8, HMOX1, CYP3A4, CAV1, CTNNB1, MYC, CASP7, F10, PTGS1, AR, DPP4, HSP90AA1, PRKACA, and CALM1, might be the main targets for mung bean to exert antioxidant activity. This result suggested that β-carotene might play a crucial role and had more biological functions in mung bean against oxidative and free radical damage (Song et al., 2020). It has been previously confirmed that consumption of foods rich in carotenoids, consisting of β-carotene, lycopene, and so on, can reduce the incidence of certain diseases, such as cardiovascular diseases, cancers, and other degenerative diseases due to their antioxidizing effects (Perera and Yen, 2007; Eggersdorfer and Wyss, 2018; Li et al., 2021). The antioxidant activity of β-carotene was determined using the reducing power assay, which is discussed in the following experiments.
ABTS radical scavenging activity of potential bioactive compounds
Radical scavenging activity of 2,2′-azino-bis-3-ethylbenzthiazoline-6-sulfonic acid was performed to evaluate the antioxidant action of sitosterol, β-carotene, vitamin E, vitexin, Boc-D-Trp-OH, the predicted active ingredients in mung bean, providing an experimental evidence for the food application of mung bean in the treatment of oxidative and free radical damage. Vitamin C was selected as a positive control, and vitamin C concentration and the predicted active ingredients tested were 1.0 mg/ml to evaluate antioxidant properties. The result is shown in Figure 2, and vitamin C, β-carotene, and vitexin showed great antioxidant activity. The ABTS radical scavenging activity of β-carotene, vitexin, and Boc-D-Trp-OH was equivalent to that of vitamin C (p > 0.05). ABTS radical scavenging activity of vitamin E and sitosterol at the same concentration was significantly lower than that of vitamin C (p < 0.05). In previous studies, the singlet oxygen quenching ability of β-carotene was obviously higher than that of vitamins C and E (Zhao et al., 1997). And, β-carotene inhibited peroxidation, its antioxidant effect was higher than that of vitamin E (Matsuno and Miki, 1990). Epidemiological studies reveal that β-carotene had a strong antioxidant capability due to the availability of free radicals and oxygen scavenging characteristics, which contributes to the body's protection against the effects of free radicals, which are related to specific diseases, including cardiovascular diseases and cancer (Siems et al., 2005; Di Martino et al., 2018). β-carotene has several applications in the food and feed industries, due to its unique yellow-orange color (Kaur et al., 2019). Isovitexin and L-tryptophan exhibited significant ABTS radical scavenging activity, which were more potent than that of L-ascorbic acid (Bai et al., 2016). Vitexin, a major antioxidant compound, could be isolated from mung bean and found to exert its effect at various levels of antioxidant action (Li et al., 2012; Khole et al., 2014; Bai et al., 2016).
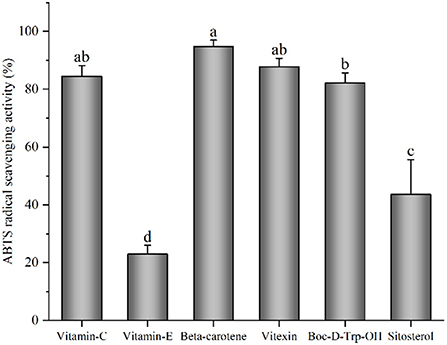
Figure 2. The 2,2′-azino-bis-3-ethylbenzthiazoline-6-sulfonic acid (ABTS) radical scavenging activity of vitamin C and potential bioactive compounds (means that do not share a letter were significantly different, p < 0.05).
PPI network of the antioxidant targets of mung bean
To further explore the importance of the selected targets (shown in Figure 1), the PPI of target proteins was established based on the string database, as shown in Figure 3 (Zhang M. et al., 2021). This PPI network consisted of 17 nodes and 56 edges, and more numbers of edges, the stronger the protein interaction. Based on the median degree centrality, closeness centrality, and betweenness centrality, the top 17 target genes are shown in Figure 4. Among them, AR, HSP90AA1, MYC, and CASP3 showed stronger interactions with other factors, which were considered to play a relatively important role in the PPI network and might be the main targets of mung bean in the treatment of oxidative and free radical damage (Wang et al., 2021).
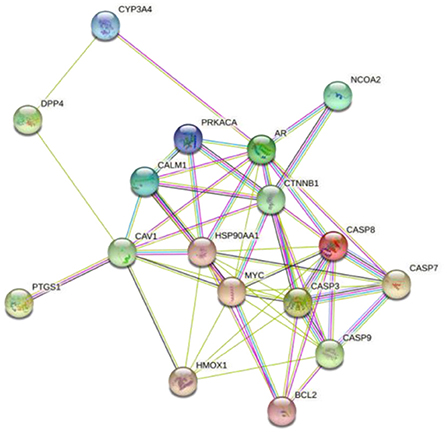
Figure 3. The protein–protein interaction (PPI) network of mung bean putative and oxidative and free radical damage-related target genes.
GO and KEGG enrichment analyses
Gene Ontology annotation analysis is shown in Figure 5. Biological processes and molecular function mainly included cysteine-type endopeptidase, peptidase activity, endopeptidase activity, DNA-binding transcription factor binding, and ubiquitin and ubiquitin-like protein ligase binding.
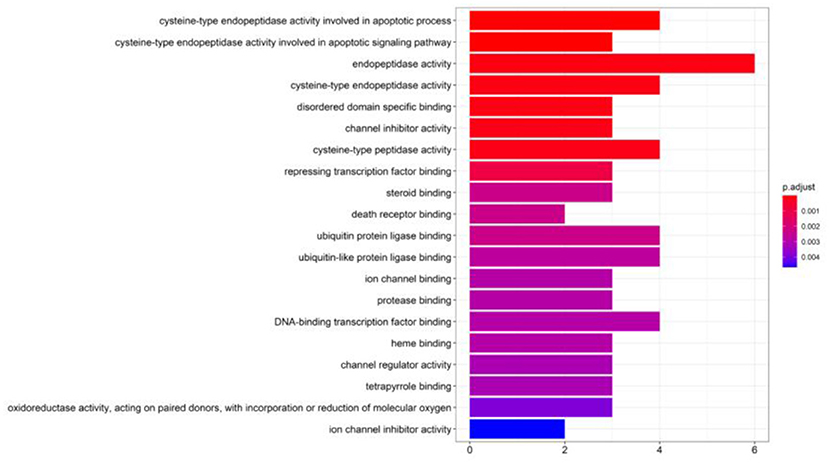
Figure 5. Gene ontology (GO) terms of the top 20 GO functional terms (p < 0.05). The color of terms turned from blue to red, the redder the bar, the smaller the adjusted p-value.
Kyoto Encyclopedia of Genes and Genomes enrichment analysis was performed to cluster major pathways associated with mung bean, and the top 20 KEGG pathways are listed in Figure 6. The results of the KEGG pathway enrichment analysis showed that antioxidant targets of mung bean were significantly enriched in the thyroid hormone signaling pathway, estrogen signaling pathway, and p53 signaling pathway.
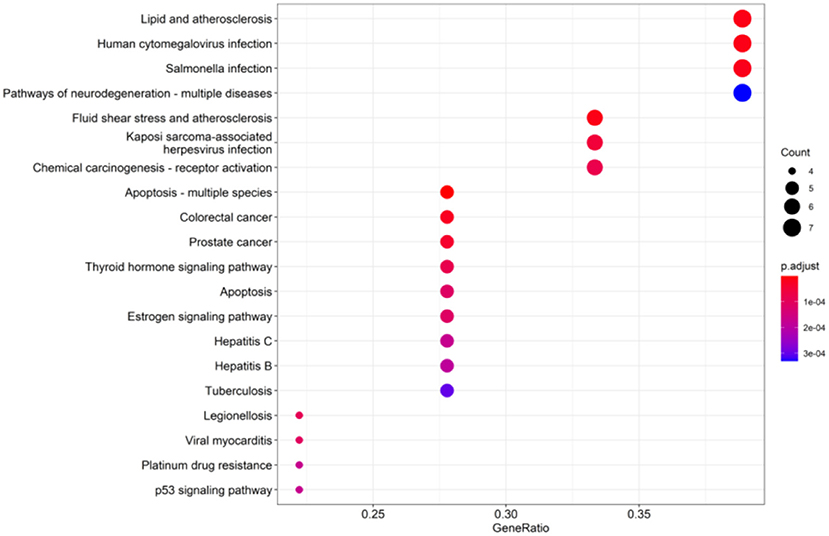
Figure 6. Kyoto Encyclopedia of Genes and Genomes (KEGG) pathway enrichment analysis of the top 20 pathways. Color represented p-value and size of the spot represented the count of genes.
Conclusion
Bioactive compounds in food materials have attracted scientists across the world in search of new natural antioxidant substances. These antioxidants can be derived from legumes and cereals, which provide the energies required for human activities and also have a diverse multitude and magnitude of biological activities. Network pharmacology and reduced power effect were used to uncover the mechanism of the effect of the potential active compounds on oxidation resistance by considering the interactions between mung bean targets and oxidative and free radical damage. β-carotene played a crucial role and had the most biological functions in mung bean against oxidative and free radical damage. The ABTS radical scavenging activity of β-carotene and vitexin was equivalent to that of vitamin C. Key targets associated with oxidative and free radical damage included AR, HSP90AA1, MYC, and CASP3. GO and KEGG indicated that mung bean mainly acted on the thyroid hormone signaling pathway, estrogen signaling pathway, p53 signaling pathway, etc. In this study, potential bioactive compounds and antioxidant mechanisms against oxidative and free radical damage in mung bean were preliminarily elucidated, to provide a reference for the functional evaluation of food ingredients and the development of functional foods. In future studies, the antioxidant efficacy and biological mechanisms of β-carotene and vitexin from mung bean will be investigated with more in vivo/in vitro experimental verification.
Data availability statement
The original contributions presented in the study are included in the article/supplementary material, further inquiries can be directed to the corresponding author.
Author contributions
Conceptualization, software, formal analysis, data curation, writing—original draft preparation, writing—review and editing, project administration, and funding acquisition: FK. Methodology and validation: FK, YL, and QZ. Investigation: QZ, FK, YZ, and YL. Resources and supervision: FK and XG. Visualization: QZ and FK. All authors have read and agreed to the published version of the manuscript.
Funding
This study was financially supported by the Doctoral Research Startup Foundation of Liaocheng University (No. 318052122), the Natural Science Foundation of Shandong Province (No. ZR2020QC061), the Research and Development of Key Technology of Nutrition Enhancement of Bone-derived Pet Food (No. K22LD04).
Conflict of interest
The authors declare that the research was conducted in the absence of any commercial or financial relationships that could be construed as a potential conflict of interest.
Publisher's note
All claims expressed in this article are solely those of the authors and do not necessarily represent those of their affiliated organizations, or those of the publisher, the editors and the reviewers. Any product that may be evaluated in this article, or claim that may be made by its manufacturer, is not guaranteed or endorsed by the publisher.
References
Bai, Y., Chang, J., Xu, Y., Cheng, D., Liu, H., Zhao, Y., et al. (2016). Antioxidant and myocardial preservation activities of natural phytochemicals from mung bean (Vigna radiata L) seeds. J. Agric. Food Chem. 64, 4648–4655. doi: 10.1021/acs.jafc.6b01538
Battino, M., Bullon, P., Wilson, M., and Newman, H. (1999). Oxidative injury and inflammatory periodontal diseases: the challenge of anti-oxidants to free radicals and reactive oxygen species. Crit. Rev. Oral Biol. Med. 10, 458–476. doi: 10.1177/10454411990100040301
Di Martino, A., Trusova, M. E., Postnikov, P. S., and Sedlarik, V. (2018). Enhancement of the antioxidant activity and stability of β-carotene using amphiphilic chitosan/nucleic acid polyplexes. Int. J. Biol. Macromol. 117, 773–780. doi: 10.1016/j.ijbiomac.2018.06.006
Dudonné, S., Vitrac, X., Coutière, P., Woillez, M., and Mérillon, J.-M. (2009). Comparative study of antioxidant properties and total phenolic content of 30 plant extracts of industrial interest using DPPH, ABTS, FRAP, SOD, and ORAC assays. J. Agric. Food Chem. 57, 1768–1774. doi: 10.1021/jf803011r
Eggersdorfer, M., and Wyss, A. (2018). Carotenoids in human nutrition and health. Arch. Biochem. Biophys. 652, 18–26. doi: 10.1016/j.abb.06, 001.
Ganesan, K., and Xu, B. A. (2018). Critical review on phytochemical profile and health promoting effects of mung bean (Vigna radiata). Food Sci. Hum. Wellness 7, 11–33. doi: 10.1016/j.fshw.2017.11.002
Gomez-Cabrera, M. C., Carretero, A., Millan-Domingo, F., Garcia-Dominguez, E., Correas, A. G., Olaso-Gonzalez, G., et al. (2021). Redox-related biomarkers in physical exercise. Redox Biol. 42, 101956. doi: 10.1016/j.redox.2021.101956
Haider, K., Haider, M. R., Neha, K., and Yar, M. S. (2020). Free radical scavengers: an overview on heterocyclic advances and medicinal prospects. Eur. J. Med. Chem. 204, 112607. doi: 10.1016/j.ejmech.2020.112607
Harman, D. (2003). The free radical theory of aging. Antioxid. Redox Signal. 5, 557–561. doi: 10.1089/152308603770310202
Heim, K. E., Tagliaferro, A. R., and Bobilya, D. J. (2002). Flavonoid antioxidants: chemistry, metabolism and structure-activity relationships. J. Nutr. Biochem. 13, 572–584. doi: 10.1016/S0955-2863(02)00208-5
Kaur, P., Ghoshal, G., and Jain, A. (2019). Bio-utilization of fruits and vegetables waste to produce β-carotene in solid-state fermentation: characterization and antioxidant activity. Process Biochem. 76, 155–164. doi: 10.1016/j.procbio.2018.10.007
Khole, S., Chatterjee, S., Variyar, P., Sharma, A., Devasagayam, T. P. A., Ghaskadbi, S., et al. (2014). Bioactive constituents of germinated fenugreek seeds with strong antioxidant potential. J. Funct. Foods. 6, 270–279. doi: 10.1016/j.jff.2013.10.016
Koufen, P., and Stark, G. (2000). Free radical induced inactivation of creatine kinase: sites of interaction, protection, and recovery. Biochim. Biophys. Acta. Mol. Basis Dis. 1501, 44–50. doi: 10.1016/S0925-4439(00)00005-3
Li, H., Cao, D., Yi, J., Cao, J., and Jiang, W. (2012). Identification of the flavonoids in mungbean (Phaseolus radiatus L) soup and their antioxidant activities. Food Chem. 135, 2942–2946. doi: 10.1016/j.foodchem.2012.07.048
Li, J., Lu, Y., Chen, H., Wang, L., Wang, S., Guo, X., et al. (2021). Effect of photoperiod on vitamin e and carotenoid biosynthesis in mung bean (Vigna radiata) sprouts. Food Chem. 358, 129915. doi: 10.1016/j.foodchem.2021.129915
Liu, H.-K., Cao, Y., Huang, W.-N., Guo, Y.-D., and Kang, Y.-F. (2013). Effect of ethylene on total phenolics, antioxidant activity, and the activity of metabolic enzymes in mung bean sprouts. Eur. Food Res. Technol. 237, 755–764. doi: 10.1007/s00217-013-2048-0
Luo, Z., Fu, C., Li, T., Gao, Q., Miao, D., Xu, J., et al. (2021). Hypoglycemic effects of licochalcone a on the streptozotocin-induced diabetic mice and its mechanism study. J. Agric. Food Chem. 69, 2444–2456. doi: 10.1021/acs.jafc.0c07630
Lv, X., Xu, Z., Xu, G., Li, H., Wang, C., Chen, J., et al. (2020). Investigation of the active components and mechanisms of schisandra chinensis in the treatment of asthma based on a network pharmacology approach and experimental validation. Food Funct. 11, 3032–3042. doi: 10.1039/D0FO00087F
Makiuchi, T., Sobue, T., Kitamura, T., Ishihara, J., Sawada, N., Iwasaki, M., et al. (2017). The relationship between vegetable/fruit consumption and gallbladder/bile duct cancer: a population-based cohort study in Japan. Int. J. Cancer. 140, 1009–1019. doi: 10.1002/ijc.30492
Matsuno, T., and Miki, W. (1990). Biological functions and activities of animal carotenoids. Kagaku Seibutsu 28, 219–227. doi: 10.1271/kagakutoseibutsu1962.28.219
Miceli, N., Buongiorno, L. P., Celi, M. G., Cacciola, F., Dugo, P., Donato, P., et al. (2016). Role of the flavonoid-rich fraction in the antioxidant and cytotoxic activities of Bauhinia forficata Link (Fabaceae) leaves extract. Nat. Prod. Res. 30, 1229–1239. doi: 10.1080/14786419.2015.1050671
Milenkovic, D., Morand, C., Cassidy, A., Konic-Ristic, A., Tomás-Barberán, F., Ordovas, J. M., et al. (2017). Interindividual variability in biomarkers of cardiometabolic health after consumption of major plant-food bioactive compounds and the determinants involved. Adv. Nutr. 8, 558–570. doi: 10.3945/an.116.013623
Pan, L., Li, Z., Wang, Y., Zhang, B., Liu, G., Liu, J., et al. (2020). Network pharmacology and metabolomics study on the intervention of traditional Chinese medicine Huanglian Decoction in rats with type 2 diabetes mellitus. J. Ethnopharmacol. 258, 112842. doi: 10.1016/j.jep.2020.112842
Perera, C. O., and Yen, G. M. (2007). Functional properties of carotenoids in human health. Int. J. Food Prop. 10, 201–230. doi: 10.1080/10942910601045271
Reuter, S., Gupta, S. C., Chaturvedi, M. M., and Aggarwal, B. B. (2010). Oxidative stress, inflammation, and cancer: how are they linked? Free Radic. Biol. Med. 49, 1603–1616. doi: 10.1016/j.freeradbiomed.2010.09.006
Ru, J., Li, P., Wang, J., Zhou, W., Li, B., Huang, C., et al. (2014). TCMSP: a database of systems pharmacology for drug discovery from herbal medicines. J. Cheminform. 6, 13. doi: 10.1186/1758-2946-6-13
Siems, W., Wiswedel, I., Salerno, C., Crif,ò, C., Augustin, W., Schild, L., et al. (2005). β-Carotene breakdown products may impair mitochondrial functions - potential side effects of high-dose β-carotene supplementation. J. Nutr. Biochem. 16:385–397. doi: 10.1016/j.jnutbio.2005.01.009
Singh, B., Singh, J. P., Kaur, A., and Singh, N. (2017). Phenolic composition and antioxidant potential of grain legume seeds: a review. Food Res. Int. 101, 1–16. doi: 10.1016/j.foodres.2017.09.026
Song, Y., Yang, J., Jing, W., Wang, Q., Liu, Y., Cheng, X., et al. (2020). Systemic elucidation on the potential bioactive compounds and hypoglycemic mechanism of Polygonum Multiflorum based on network pharmacology. Chin. Med. 15, 121. doi: 10.1186/s13020-020-00401-2
Tang, D., Dong, Y., Ren, H., Li, L., and He, C. A. (2014). review of phytochemistry, metabolite changes, and medicinal uses of the common food mung bean and its sprouts (Vigna radiata). Chem. Cent. J. 8, 4. doi: 10.1186/1752-153X-8-4
Valle, I. F., Roweth, H. G., Malloy, M. W., Moco, S., Barron, D., Battinelli, E., et al. (2021). Network medicine framework shows that proximity of polyphenol targets and disease proteins predicts therapeutic effects of polyphenols. Nat. Food 2, 143–155. doi: 10.1038/s43016-021-00243-7
Wang, J., Ge, W., Peng, X., Yuan, L., He, S., Fu, X., et al. (2021). Investigating the active compounds and mechanism of huashi xuanfei formula for prevention and treatment of covid-19 based on network pharmacology and molecular docking analysis. Mol. Divers. 26, 1175–1190. doi: 10.1007/s11030-021-10244-0
Wang, S., Gao, J., Li, Q., Ming, W., Fu, Y., Song, L., et al. (2020). Study on the regulatory mechanism and experimental verification of icariin for the treatment of ovarian cancer based on network pharmacology. J. Ethnopharmacol. 262, 113189. doi: 10.1016/j.jep.2020.113189
Xia, Q. D., Xun, Y., Lu, J. L., Lu, Y. C., Yang, Y. Y., Zhou, P., et al. (2020). Network pharmacology and molecular docking analyses on Lianhua Qingwen capsule indicate akt1 is a potential target to treat and prevent covid-19. Cell Prolif. 53:e12949. doi: 10.1111/cpr.12949
Xu, H., Zhou, Q., Liu, B., Cheng, K.-W., Chen, F., and Wang, M. (2021). Neuroprotective potential of mung bean (Vigna radiata L) Polyphenols in Alzheimer's disease: a review. J. Agric. Food Chem. 69, 11554–11571. doi: 10.1021/acs.jafc.1c04049
Yang, H.-W., Hsu, C.- K., and Yang, Y.-F. (2014). Effect of thermal treatments on anti-nutritional factors and antioxidant capabilities in yellow soybeans and green-cotyledon small black soybeans. J. Sci. Food Agric. 94, 1794–1801. doi: 10.1002/jsfa.6494
Yang, Q.-Q., Ge, Y.-Y., Gunaratne, A., Kong, K.-W., Li, H.-B., Gul, K., et al. (2020). Phenolic profiles, antioxidant activities, and antiproliferative activities of different mung bean (Vigna radiata) varieties from Sri Lanka. Food Biosci. 37, 100705. doi: 10.1016/j.fbio.2020.100705
Yi, J., Tian, M., Hu, L., Kang, N., Ma, W., Zhi, J., et al. (2021). The mechanisms of celastrol in treating papillary thyroid carcinoma based on network pharmacology and experiment verification. Ann. Transl. Med. 9, 866. doi: 10.21037/atm-21-1854
Zhang, F. X., Tang, Z. L., and Qiu, Z. C. (2021). A novel strategy for exploring food originated anti-adipogenesis substances and mechanism by structural similarity evaluation, adme prediction, network pharmacology and experimental validation. Food Funct. 12, 7081–7091. doi: 10.1039./D1FO01124C
Zhang, M., Li, P., Zhang, S., Zhang, X., Wang, L., Zhang, Y., et al. (2021). Study on the mechanism of the danggui-chuanxiong herb pair on treating thrombus through network pharmacology and zebrafish models. ACS Omega 6, 14677–14691. doi: 10.1021/acsomega.1c01847
Zhao, F., Guochun, L., Yang, Y., Shi, L., Xu, L., Yin, L., et al. (2015). network pharmacology approach to determine active ingredients and rationality of herb combinations of modified-simiaowan for treatment of gout. J. Ethnopharmacol. 168:1–16. doi: 10.1016/j.jep.2015.03.035
Keywords: antioxidant, network pharmacology, mung bean, bioactive compound, ABTS radical scavenging activity+
Citation: Kong F, Li Y, Zhang Y, Zeng Q and Guo X (2022) Elucidation of the potential antioxidant compound and mechanism of mung bean using network pharmacology and in vitro anti-oxidative activity. Front. Sustain. Food Syst. 6:1000916. doi: 10.3389/fsufs.2022.1000916
Received: 22 July 2022; Accepted: 12 August 2022;
Published: 11 October 2022.
Edited by:
Rana Muhammad Aadil, University of Agriculture Faisalabad, PakistanReviewed by:
Anees Ahmed Khalil, University of Lahore, PakistanTabussam Tufail, University of Lahore, Pakistan
Sidra Jabeen, University of Agriculture, Faisalabad, Pakistan
Copyright © 2022 Kong, Li, Zhang, Zeng and Guo. This is an open-access article distributed under the terms of the Creative Commons Attribution License (CC BY). The use, distribution or reproduction in other forums is permitted, provided the original author(s) and the copyright owner(s) are credited and that the original publication in this journal is cited, in accordance with accepted academic practice. No use, distribution or reproduction is permitted which does not comply with these terms.
*Correspondence: Feng Kong, d3lrZjIwMTJAMTI2LmNvbQ==