- Department of Entomology, California Academy of Sciences, San Francisco, CA, United States
Global socioeconomic systems exacerbate disparities that leave a disturbing proportion of the human population malnourished, making conventional food sources such as animal livestock unsustainable at global scales. Edible insects have the potential to solve both malnourishment and the unsustainability of conventional livestock agriculture. The sustainability and accessibility of farmed, edible insects, however, depends on their feed source. Future expansion of farming rests on developing locally available and affordable insect feeds. This article presents a literature review of studies which evaluate the performance of edible crickets (Orthoptera) in response to alternative feed sources such as weeds and the byproducts of agricultural and food industries. For each insect species evaluated, we analyze measurements of feed and insect performance, feed composition, effects of diet on cricket growth and survivorship, as well as other sources of performance differences. The aim of this review is to assess the current state of alternative feed research for farmed crickets and to provide guidelines for future studies on alternative feeds.
Introduction
As of 2020, 690 million people, 8.9 percent of the world's population, are hungry (FAO, 2020) and the world's demand for animal protein is increasing at an alarming rate (Delgado et al., 2001; Steinfeld et al., 2006). The realization that systems producing conventional livestock provide a poor solution to the problems of malnourished communities harks back to a 1975 publication by Meyer-Rochow, who advocated the use of insects as food and feed and suggested FAO and WHO take up that idea (Meyer-Rochow, 1975). Current systems producing conventional livestock provide a poor solution to the problems of malnourished communities. Not only do conventionally farmed livestock demand environmentally and economically expensive feed crops, but the land degradation caused by livestock rearing and low conversion efficiencies render them an unrealistic resource for impoverished communities (Gerber and FAO, 2013).
The dominant discourse of “food security” results in conventional technocratic solutions which rely on increasing conventional agricultural production and the short-term distribution of fortified foods (Patel, 2009; Sampson et al., 2021). These interventions by governments and NGOs present malnutrition in terms of access to nutritional, physical, and economic wellbeing, but avoid discussion of the social control of food systems and divert attention from structural inequities of power that reproduce malnutrition (Patel, 2009; Misra, 2018).
An enormous shift in global food infrastructure is warranted to address these concerns. By establishing food systems which are thoroughly sustainable (culturally, environmentally, politically, economically, etc.), malnourished communities can feed themselves while subverting structural inequities of power that produce malnourishment. There is now growing interest in entomophagy, or insect-eating, as an alternative food source for animal and humans. This is because insects can convert feed to protein and micronutrients with impressive efficiency.
Entomophagy presents an opportunity to substitute conventional livestock with insect minilivestock (van Huis et al., 2013; Magara et al., 2021). Studies examining the substitution of animal protein with insect protein are promising. Insects have been shown to be good sources of vital nutrients, including proteins, lipids, fatty acids, iron, and vitamin B12 (van Huis et al., 2013; Makkar et al., 2014; Sánchez-Muros et al., 2014; Akhtar and Isman, 2018; Magara et al., 2021). Dehydrated crickets have been shown to have 55–69% protein, 6–27% fat, and 8–12% fiber, and 3–9% ash composition, and contain at least 18 amino acids, including 8–9 essential amino acids (Chakravorty et al., 2014; Ghosh et al., 2017; Escamilla-Rosales et al., 2019). Insect production has also been shown to be more sustainable than conventional livestock in terms of greenhouse gas emissions (Oonincx et al., 2010; Oonincx and Boer, 2012; van Zanten et al., 2015), energy (Oonincx and Boer, 2012), land (Oonincx and Boer, 2012; Sánchez-Muros et al., 2014; Salomone et al., 2017), water (Sánchez-Muros et al., 2014; Salomone et al., 2017), feed conversion efficiency (van Huis et al., 2013), carbon footprint (Sánchez-Muros et al., 2014) and overall global warming potential (Vauterin et al., 2021).
Perhaps the most exciting potential of insects as food and feed is their ability to convert weeds as well as agricultural and food industry byproducts into high value substances (Van Peer et al., 2021). Insects provide an opportunity to support malnourished communities with inexpensive, locally sourced waste resources and reduce the growing issues of food waste (Parfitt et al., 2010; Mbow et al., 2019). Thus, minilivestock could provide a sustainable, immediate, short-term opportunity to address malnutrition outside of current, unsustainable structures of food production. Edible insects also provide an opportunity for a more sustainable food system in the global north where ecological devastation caused by feed crop production and an overabundance of biological waste and greenhouse gas emissions is widespread (Caro et al., 2014).
One challenge to the adoption of edible insect farming as a means of food and nutrition security is sourcing sustainable, low cost feed, especially in countries where hunger is most prevalent (Halloran et al., 2018). Edible insects have been shown to possess the potential to be reared successfully on organic side streams, especially compound side streams that respect their dietary requirements (Van Peer et al., 2021). However, a comprehensive, specific review of the effects of sustainable side-stream feeds on the growth and survival performance of crickets has yet to be undertaken. Research on feed can ensure that insect farming is accessible across all socioeconomic situations and scales of production. Here we review 21 research studies that focus on sustainable alternatives to commercial feeds for insects with a specific interest in Orthopterans. Alternative feeds include byproducts of agricultural and food industries, as well as locally available weeds and post-consumer food sources. We summarize the methods in this body of research, including the commonly measured insect species, treatment diets, feed measurements, and insect measurements. We also summarize the effects of feed source, composition, and environmental factors on insect growth and survival performances. We synthesize general characteristics of higher performing feeds and discuss the potential and requirements of sustainability for rearing edible insects.
Aim of the Study
This paper carries out an in-depth study of the literature on edible Orthoptera performance when raised on weeds, agricultural and food industry byproducts. The study consolidates current knowledge of the field and identifies common practices and requirements for rearing edible insects on alternative feeds.
The purpose of this study is to focus future insect feed practices toward weeds and agricultural and food industry byproducts, and to provide guidance enabling future in-depth studies can more specifically identify the feed requirements for sustainably reared edible insects.
Methods
A literature search updated to June 2021 was performed on Google Scholar. The search terms used consisted of the following: edible insects, edible orthoptera, edible cricket, feeds, alternative feed, organic waste, side-stream, byproducts, weeds, agriculture, food industry, insect feed. Publications conducted in English from 1962 through 2021 were considered. Articles listed in the references of selected publications were also considered for inclusion. The criteria for inclusion were studies which investigated: the effect of organic side-stream, byproduct, and/or wastes-sourced diets on Orthoptera growth, survival, conversion efficiency, and/or nutritional composition; the effects of organic side-stream diets varying in nutritional composition on Orthoptera performance; time-based variations in feeding regimes on Orthoptera performance; the effects of organic side-stream supplementation of conventional diets on Orthoptera performance; the optimization of Orthoptera feed composition; and the impacts of feed on the sustainability of Orthoptera farming operations. Studies were also considered for addition that were suggested through the contribution of reviewers.
Figure 1 demonstrates a flowchart for the search and inclusion process. The initial search yielded 79 papers, for which the titles and abstracts were gathered, and the full papers read when relevant. Out of these, 48 papers were excluded as they did not meet the inclusion criteria. The remaining 31 papers were read through and an additional 10 were excluded because they did not evaluate a species of Orthoptera or did not collect data on cricket performance. A total of 21 papers were included in this review.
While many of the studies included heterogeneous parameters and data, enough were shared that the following data were extracted and compiled for the purpose of this review: insect species studied, treatment diets, feed plan, feed source, feed category, feed composition, feed measurements, crude protein, carbohydrate, and crude fat compositions of feeding regimes, insect measurements, insect life stage/duration of study, mean final weight (g), and final survival. A list of all the raw data for each study is presented in Supplementary Material 2.
Summary
Insect Species
Of the insect species of Orthoptera examined (Supplementary Table 1), the most common species by far is Acheta domesticus Linnaeus, 1758, followed by Gryllus bimaculatus De Geer, 1773, and Teleogryllus mitratus Burmeister, 1838, referred to by its synonyms Teleogryllus testaceus Walker, 1869, and Gryllus mitratus Burmeister, 1838, and Gryllus testaceus Walker, 1869 (Figure 2). There was one study for each of the following species: Valanga nigricornus Burmeister, 1838, Xenocatantops humulis Serville, 1838, and Scapsipedus icipe Hugel and Tanga, 2018 (Figure 2).
Treatment Diets
The number of treatment diets varied from 2 to 18 (Supplementary Table 1). The composition of control diets consisted either of commercial chicken feed, commercially available cricket feed, or dog food (Supplementary Table 1). The alternative feed diets were determined by local vegetable and animal side-stream resources and varied widely in composition. The level of processing of alternative diets also varied from freshly harvested vegetables to processed grains and grocery store waste filtrate (Supplementary Table 1).
The feeding model for alternative feed treatments can be divided into two plans: commercial feed supplemented simultaneously with weeds and/or agricultural and food industry byproducts, and feed consisting completely of weeds and/or agricultural and food industry byproducts. The frequency of implementation of these two feeding plans was nearly equal (Supplementary Table 1).
The feed sources can be divided into five categories, in order of frequency: agricultural product, agricultural byproduct, animal byproduct, commercial feed, weeds, food waste (Supplementary Table 2; Figure 3). Food waste is defined as post-consumer vegetables and vegetable byproducts of food such as heterogeneous post-consumer waste and vegetable and fruit scraps such as waste from open markets, supermarkets, food service providers, food distributors, and wholesale food vendors. The availability of waste from this source is proportionally smallest within the food production chain; however, the high quality of marketable products makes is a valuable feed source (Van Peer et al., 2021). Weeds refer to uncultivated plants which grow in undesired locations, such as roadsides, fallow land, fields, and wastelands. Agricultural byproducts are pre-consumer vegetable products produced in the process of farming or food processing, as well as plant parts not sold to consumers, such as rice/wheat bran, barley mash, and mung bean sprout residue. Most organic residues are produced at this primary production level and thus present a promising resource for cricket feed, however they may need to be processed from their raw form to present suitable feed (Van Peer et al., 2021). Agricultural products are the primary vegetable products, both processed and unprocessed such as vegetables, fruits, roots, grains, flours. Animal byproducts are the byproducts of animal agriculture and meat production such as blood meal, fish offal, liver powder, and chicken manure. These have all been shown to provide excellent sources of protein, vitamins, minerals, and lipids (Van Peer et al., 2021). Feed sources varied both within individual studies, with multiple experimental diets composed from a variety of feed sources, and within single diets, with many individual experimental feeding regimes composed from multiple sources (Supplementary Table 2; Figure 3).
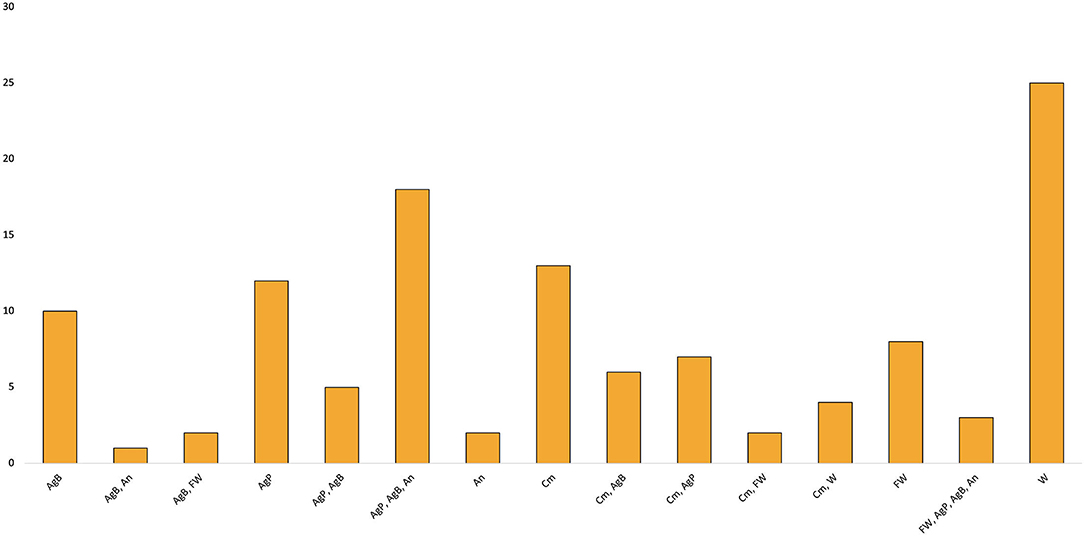
Figure 3. Frequency of feed sources across all diets. AgB, agricultural byproduct; AgP, agricultural product; An, animal byproduct; Cm, commercial feed; FW, food waste; W, weed.
The nutritional composition of the feeds varied widely. The composition (as percent of dry matter) across all diets ranged from 5.2 to 95% crude protein, 1.3 to 69.1% carbohydrate, and 0.1 to 19.3% crude fat, with respective averages of 22.8, 46.3, and 5.1% (Supplementary Table 2).
Feed Measurements
The most common feed macronutrient parameters considered were crude protein (CP), crude fat (CF), ash, and carbohydrate, in that order (Figure 4). Crude fat content was also found under the synonym of ether extract, which refers to the extraction method for determining crude fat content (Saha et al., 2010), as well as crude lipid or total oil content, although these designations may use alternative extraction methods producing a different composition of substances. Carbohydrate content is also referred to as starch content (Vaga et al., 2019, 2020), considered an underestimate of carbohydrate content as it encompasses a smaller array of carbohydrate fractions (Saha et al., 2010). Other frequently recorded parameters included dry matter, crude fiber, phosphorus, calcium, potassium, nitrogen, acid detergent fiber (ADF), neutral detergent fiber (NDF), manganese, copper, iron, gross energy, vitamin C, vitamin A, sodium, magnesium, and zinc. The following were only recorded in one study: total organic content, cholesterol, phytosterol, ergosterol, total sterols, vitamins B-carotene, E, K, B1-9, niacin, pantothenic acid, riboflavin, thiamin, choline, total fatty acids, fatty acid composition, nitrogen free extracts, starch, soluble nitrogen, ammonia, pH, and soluble CP.
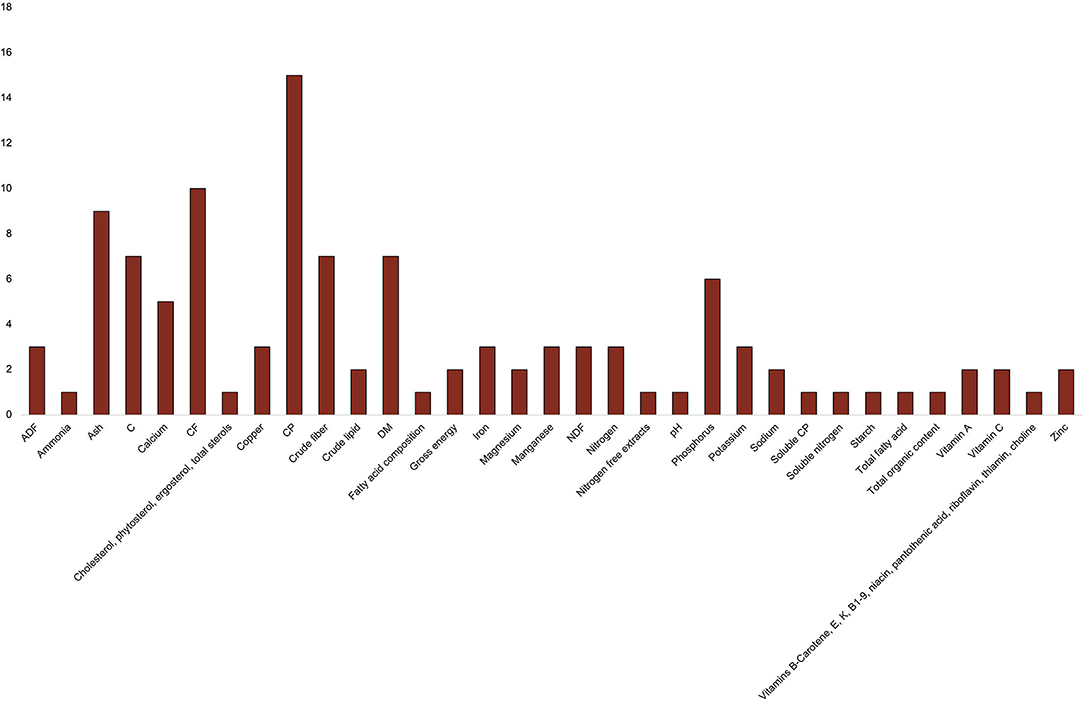
Figure 4. Frequency of feed nutrients measured across studies. ADF, acid detergent fiber; C, carbohydrate; CF, crude fiber; CP, crude protein; DM, dry matter; NDF, neutral detergent fiber.
Insect Measurements
All studies included parameters with which to measure insect growth and development, with most studies recording mortality or survival rates as a function of experimental feeding regime (Figure 5). Insect final survival ranged from 0 to 100% across studies (Supplementary Table 3) with an average of 53%. Mean weight and weight gain were also recorded frequently (Figure 5). The mean final weight across all studies ranged from 1.39 to 0.03 g (Supplementary Table 3), with an average of 0.45 g for all insect species. Less commonly observed were growth index/rate, CP, efficiency conversion of ingested food (ECI), biomass accumulation, feed conversion ratio (FCR), final weight, development time, feed intake, dry matter (DM), crude fat (CF), ash, fiber, weight yield, and water consumption (Figure 5).
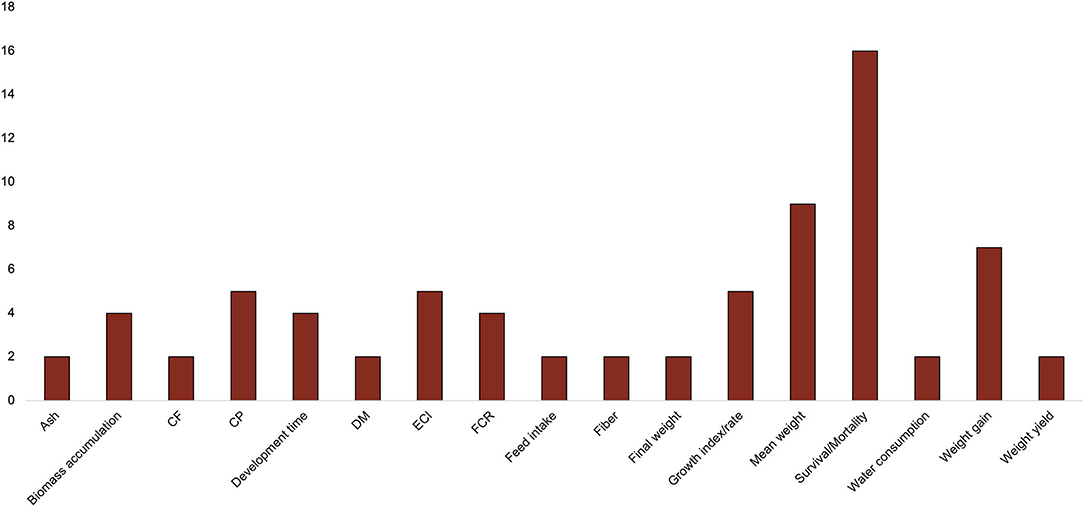
Figure 5. Frequency of insect measurements across studies. CF, crude fiber; CP, crude protein, DM, dry matter; ECI, efficiency conversion of ingested food; FCR, feed conversion ratio.
The following were only recorded once: longevity, mortality between sexes, duration of preoviposition, oviposition, and postoviposition, developmental duration, body length, daily egg load, lifetime fecundity, egg eclosion period, egg hatchability (Magara et al., 2019), quantity of feed consumed, mean body length, cost of feeding, carbohydrate (Bawa et al., 2020), P-FCR (protein feed conversion ratio), individual size (Dobermann et al., 2019), feed conversion (mg/eggs), egg production (daily) (Fuah et al., 2015), mean consumption of individual food ingredients ratios and nutrient intake, dry weight food consumption, percent food assimilation, ending biomass, ending percentage adults, live weight, dry weight (Morales-Ramos et al., 2020), energy (Harsányi et al., 2020), protein conversion efficiency (Lundy and Parrella, 2015), initial weight (Megido et al., 2016), feed consumption (Miech et al., 2016), microbial counts and relative abundance (Ng'ang'a et al., 2020), maturity period (Oloo et al., 2020), nitrogen efficiency, phosphorus content, total fatty acids (Oonincx et al., 2015), developmental rate, glycoalkaloid concentrations, fatty acid content and composition (Sorjonen et al., 2019), daily DM intake, and periodic FCR (Vaga et al., 2019).
Life Stage and Duration
The timing of insect developmental stages varied widely, ranging from the onset of experimental feeds at <24 h old, to starting experimental diets at 4 weeks of age (Supplementary Table 1). The duration of experimental feeding regimes also varied widely from 28 days (Megido et al., 2016) to 168 days (Oonincx et al., 2015).
Discussion
Feed Category Effect on Insect Performance
Food Waste Diets
Pure food waste diets had an average crude protein content of 17% DM and an average final survival of 6%. Diets which were supplemented in part with food waste had an average crude protein content of 23% DM and a 77% average final survival (Supplementary Tables 2, 3).
The following food waste diets resulted in the highest final survival (75% or greater) and greatest mean final weight for both A. domesticus and G. bimaculatus in order from greatest to least final survival: turnip rape (high protein), turnip rape (medium protein), and turnip rape (low protein) (Supplementary Table 3). All 3 of these diets are supplemented and consist of Patton (1967) diet 16 in which the soybean meal (major protein source) was replaced by turnip rape (Brassica rapa). These three diets have between 15 and 30.5% crude protein, and are mixed diets composed of commercially available processed animal and plant products and food waste (Patton, 1967).
Of the A. domesticus reared on pure food waste diets, processed food waste clearly yielded higher mean final weight than unprocessed food waste. Post-processed grocery store food waste filtrate processed through proprietary, aerobic enzymatic digestion performed significantly better regarding both mean final weight and survival than minimally processed post-consumer food waste (Lundy and Parrella, 2015). This is most likely due to the increase in accessible protein content due to enzymatic digestion. These results imply that high quality, processed food waste provided as supplementation to a conventional commercial diet can yield high survival and weight outcomes. However, lower quality, unprocessed, food waste diets may not provide adequate nutrition to use independently.
Weed Diets
Pure weed diets had an 18% DM average crude protein content and 18% average final survival (Supplementary Tables 2, 3). The single recording of commercial feeds supplemented with weeds had 85% final survival and 1.39 g mean final weight for G. bimaculatus. The overall survival on weed diets was low, with only the chicken feed supplemented with Commelina sinensis (Ng'ang'a et al., 2020), and pure Ipomoea cairica (Choo et al., 2017) diets resulting in survival > 44.4%, which yielded final survivals of 85 and 60%, respectively (Supplementary Table 3). Unfortunately, neither of the macronutrient compositions were disclosed in either of these studies.
Final mean A. domesticus weight for weed-based diets were highest only for pure Synedrela nodiflora and final mean T. testaceus weights (>0.65 g) were highest for pure Cleome rutidosperma, Cleome viscosa, and Commelina benghalensis (Miech et al., 2016) (Supplementary Table 3). These four weeds contain relatively high crude protein (16.7–22.9%) content and were all presented to the insects fresh and unprocessed (Supplementary Table 2).
The overall performance of Orthoptera raised on weed-based diets suggests that a weed-based feed yields the best performance when used as a supplementation of conventional diets. However, weeds containing sufficiently high protein can be used as a pure diet with satisfactory results.
Agricultural Byproduct Diets
Diets consisting solely of agricultural byproducts had an average protein of 24% DM and an average survival of 34% (Supplementary Tables 2, 3). Diets which included agricultural byproducts supplemented by conventional feeds had average crude protein content of 23% DM and an average survival of 60% (Supplementary Tables 2, 3). Of the 14 diets containing agricultural byproducts that resulted in greater that 80% survival, only two were pure agricultural byproduct feeds. These two were wheat bran and pumpkin leaves and had crude protein levels of 16.18 and 24.42%, respectively (Supplementary Table 2). Both successful feeds were agricultural byproducts that had been processed beforehand. Wheat bran is removed from a dried wheat seed during the milling process, resulting in a dry, pulverized substrate. The pumpkin leaves were also dried and pulverized into a powder, rather than being presented as fresh leaves (Magara et al., 2019). The remaining 12 high survival agricultural byproduct diets were all some version of Patton's diets 16, 15, or 13 (Patton, 1967), which again consist of a mix of high quality, processed, animal and plant products Supplementary Table 3).
The highest mean final weights for S. icipe were recorded for wheat bran and pumpkin leaves (Supplementary Table 3). The highest mean final weights for T. testaceus were recorded in the cassava top and mung bean sprout residue diets, which had 28.5 and 19.4% crude protein contents, respectively (Supplementary Table 3). Both diets consisted solely of agricultural byproducts. The highest G. bimaculatus and A. domesticus weights were recorded for supplemented Patton's 3, 13, 15, and 16 diets with 20.4–30.5% crude protein content (Supplementary Table 3).
The level of processing does not seem to always correlate with high performance. Cassava tops were fed to T. testaceus fresh and unprocessed with great success (Miech et al., 2016), and the lowest recorded survival for agricultural byproduct diets was the poultry manure + wheat straw + rice silage diet which resulted in no A. domesticus surviving to a harvestable size (Lundy and Parrella, 2015). Although fermented rice silage may free up accessible nitrogen for the crickets, the relatively low crude protein (8.8%), and high acid detergent fiber (50%) and ash (33%) composition of this agricultural byproduct feed was evidently not ideal. All other agricultural byproduct diets resulted in final survival of 65% or lower (Supplementary Tables 2, 3).
Agricultural Product Diets
Pure agricultural product diets had an average crude protein content of 27% DM and 50% average final survival (Supplementary Tables 2, 3). Diets supplemented with agricultural products had an average crude protein content of 24% DM and an average survival of 64% (Supplementary Tables 2, 3). The diets resulting in 90% or greater final survival were as follows: Brassica rapa + dog food, Pure Pride cricket feed + pumpkin powder, Pure Pride cricket feed + fresh pumpkin pulp, barley mash (medium protein), young cassava leaf flour + brown rice flour + banana, barley mash (high protein), soybean meal, taro aerial part flour, barley feed (medium protein). The average of crude protein concentration for these diets was 23.8% DM with all values falling between 15.0 and 41.1% DM (Supplementary Table 2). Of these 9 diets, only the cassava and brown rice flour + banana, soybean, and taro aerial part flour consist wholly of direct agricultural products, with the remaining 6 relying on supplementation either dog food, cricket feed, or Patton's 16 diet mix. The 3 high performing pure agricultural product diets were all processed into meals or flours before fed to the insects.
All A. domesticus mean final weights > 0.25 g were recorded for conventional feeds supplemented with agricultural products (Supplementary Tables 2, 3). The highest final weights were all supplemented with cricket feed, Patton's Diets 3, 13, 15, or 16, or dog food (Supplementary Table 3). The highest G. bimaculatus mean final weights were all recorded for 22.5–30.5% crude protein variations on Patton's 16 diet (Sorjonen et al., 2019) (Supplementary Table 3). The highest T. testaceus mean final weights were recorded for pure agricultural products consisting of young cassava leaf flour with or without brown rice flour and banana (Supplementary Table 3).
These results imply that conventional feeds supplemented with processed or unprocessed agricultural products yield high survival and weight gain. Agricultural products without supplementation also have potential as feeds, but only when they have been processed into flours, meals, etc. Feeds consisting only of unprocessed agricultural products such as fresh vegetables and silage result in generally low survival and weight gain.
Crude Protein
Overall, higher crude protein content yielded higher performance until reaching saturation. Survival and weight gain increased, and development time decreased, with an increase in protein content in A. domesticus, S. icipe, and R. differens (Lundy and Parrella, 2015; Magara et al., 2019; Harsányi et al., 2020). Sixty-eight percentage of variation in A. domesticus population viability was attributed to crude protein content (Harsányi et al., 2020). The positive effects of high feed protein content appear to reach saturation at protein contents higher than 20–30%, above which result in greater mortality, lighter weight, and longer development times (Orinda et al., 2017; Magara et al., 2019; Oloo et al., 2020). This saturation of the positive effects of protein was also found to be true in one study which investigated Ruspolia differens (Serville, 1838, Orthoptera:Tettigoniidae), implying that this effect may extend to other Orthoptera as well (Sorjonen et al., 2020).
A high-protein diet was also found to be vital to egg production and fecundity (Fuah et al., 2015; Magara et al., 2019). Higher feed protein levels (16.2–56%) correlated with shorter preoviposition periods, longer oviposition durations, longer postoviposition durations, higher fecundity, higher egg hatchability, and shorter egg eclosion periods in S. icipe due to the high demand for protein compounds in ovipositing females, as eggs are composed mostly of protein and lipids (Magara et al., 2019). Magara et al. (2019) also found that diet protein content caused a strong sex ratio bias in offspring, with a female-biased sex ratio observed in S. icipe populations reared on higher protein (16–41%) diets of wheat bran, soybean, and/or pumpkin leaf. Higher-protein diets were also correlated with higher-protein content in A. domesticus (Bawa et al., 2020; Oloo et al., 2020).
While FCR generally remained the same across diet treatments (Oonincx et al., 2015; Miech et al., 2016; Veenenbos and Oonincx, 2017), a few studies found FCR to be dependent on diet treatment (Lundy and Parrella, 2015; Sorjonen et al., 2019) with higher efficiency correlated with higher protein content in the feed (Sorjonen et al., 2019; Bawa et al., 2020). Interestingly, fermenting, or ensiling, Phleum pratense, a process which increases soluble nitrogen, had no effect on FCR of A. domesticus (Vaga et al., 2019, 2020).
Low-protein diets were shown to lengthen development time compared to higher protein feeding regimes (Oonincx et al., 2015; Magara et al., 2019; Sorjonen et al., 2019, 2020; Oloo et al., 2020), with 68% of the effect on development rate being attributed to crude protein content (Harsányi et al., 2020). Low protein diets were shown to result in higher mortality (Lundy and Parrella, 2015; Magara et al., 2019; Sorjonen et al., 2019; Harsányi et al., 2020) and cause a significant male bias (Magara et al., 2019).
In R. differens, lower feed protein content was shown to correspond to high fatty acid content. This suggests that lower feed protein levels necessitates an increase in volume of feed consumed, resulting in increased carbohydrate consumption and thus an increase in conversion of carbohydrates into fatty acids stored in the fat body (Sorjonen et al., 2020).
Inaccessibility of protein resulted in lower survival. Lundy and Parrella (2015) determined that the protein present in minimally processed post-consumer food waste was inaccessible to A. domesticus, causing high mortality. An imbalanced amino acid profile in blood meal was also shown to result in lower growth rates for A. domesticus and G. bimaculatus (Orinda et al., 2017). Megido et al. (2016) found that while cashew leaves contained an adequate nutritional composition, a cashew leaf diet yielded extremely high mortality (94.44%) for T. testaceus due to a negative nitrogen balance.
Sorjonen et al. (2019, 2020) recorded the highest survival weight gain, and development time outcomes for A. domesticus, G. bimaculatus, and R. differens for mid-to-high protein diet levels (17.9–30.5%) and the lowest for low protein diets (15%), however, not all mid-to-high protein diets resulted in comparable performance. Similarly, while the highest survival and weight gain were found in A. domesticus fed diets containing 20–30% protein by Patton (1967), not all diets within that range yielded high performance. Megido et al. (2016) found that protein levels in diets had no significant impact on protein levels in T. testaceus. These examples of ambiguity in the correlation between feed protein levels and insect performance may be attributed to differences in protein accessibility and amino acid profile.
Carbohydrate
Greater feed carbohydrate content was shown to be associated with an increase in A. domesticus survival (Vaga et al., 2019, 2020), T. mitratus and A. domesticus weight gain (Megido et al., 2016; Vaga et al., 2019), and faster development in A. domesticus and G. bimaculatus (Orinda et al., 2017). Higher A. domesticus body length was recorded for a vegetable-supplemented chicken feed diet compared to a pure control diet, which may be attributed to increased carbohydrate levels (Bawa et al., 2020).
The studies that did not have direct correlations between carbohydrate content and performance nevertheless had the highest weights recorded for high carbohydrate levels (Patton, 1967; Sorjonen et al., 2019).
Crude Fat
Crude fat contents ranging from 4 to 20% were associated with higher S. icipe survival (Magara et al., 2019) and T. mitratus and A. domesticus weight gain (Lundy and Parrella, 2015; Megido et al., 2016; Vaga et al., 2020). High fatty acid contents (6.4–9.5%) were also associated with higher weight in R. differens (Sorjonen et al., 2020).
Low concentrations of fatty acids, specifically oleic acid (C18:1n9) and linoleic acid (C18:2n6) may have caused higher mortality and prolonged development in A. domesticus, suggesting that at least one of these is required in a successful diet (Oonincx et al., 2015). These results align with the consensus that certain lipids, specifically cholesterol, linoleic acid, and linolenic acid are considered as essential for insect diets (Van Peer et al., 2021).
Fiber
Overall, high indigestible fiber content in feeds was correlated to low survival and growth in insects (Lundy and Parrella, 2015; Orinda et al., 2017; Vaga et al., 2020). Negative associations between crude fiber and growth were observed for crude fiber concentrations above 20% for A. domesticus), and positive correlations for crude fiber concentrations below 10% (Vaga et al., 2020). Magara et al. (2019) found that higher survival rates were associated with a slightly larger range of crude fiber content, between 12.4 and 38.0% in the diets of S. icipe.
The ratio of crude protein to acid detergent fiber explained 28% of the variability in A. domesticus performance (Lundy and Parrella, 2015). However, there was no correlation found between fiber levels and T. mitratus weight gain (Miech et al., 2016). These variations in response to fiber content may be due to the degree of digestibility of the fiber, as well as variation in the ability to digest fiber across species.
Supplementation
The effect of supplementing conventional diets with alternative feeds was positive overall. Higher survival rates were documented for A. domesticus reared on diets supplemented with alternative feeds when compared to a control (Sorjonen et al., 2019; Harsányi et al., 2020; Quek et al., 2020). Higher weight gain was recorded in supplemented diets compared to control diets for G. bimaculatus (Ng'ang'a et al., 2020) and A. domesticus (Quek et al., 2020). Ng'ang'a et al. (2020) found that bacterial endospore counts were lower for G. bimaculatus that had been fed chicken feed supplemented with the local weed Commelina sinensis than on a pure chicken feed diet. This implies that supplementation with fresh vegetables may reduce risks from bacterial endospores.
Most cases where feed substrate had no significant impact on weight gain were in supplemented diets (Veenenbos and Oonincx, 2017; Morales-Ramos et al., 2020; Vaga et al., 2020). Veenenbos and Oonincx (2017) and Ng'ang'a et al. (2020) discovered similar survival and development time across supplemented and pure conventional diets.
Other Sources of Growth and Development Performance Differences
In the small group experiment of Morales-Ramos et al. (2020), the authors found that although the control diets yielded significantly higher survival rates compared to the experimental diets, the macronutrient contents of all diets contained similarly high values for crude protein, fat, and carbohydrate content. In another study, no correlations were observed between weight gain and feed macronutrient composition, although all experimental diets resulted in significantly lower weight gain than the chicken feed control (Miech et al., 2016). These ambiguities of the relationship between feed macronutrient content and insect performance may imply that cricket performance cannot be explained solely by major macronutrient composition of the diets (Oonincx et al., 2015; Miech et al., 2016; Orinda et al., 2017; Vaga et al., 2020).
An initial explanation for such a broad range of insect performances may be because this body of study examines a range of Orthoptera species. Different Orthoptera, specifically A. domesticus and G. bimaculatus, have been shown to perform differently given the same diet (Orinda et al., 2017; Sorjonen et al., 2019).
Sex has also been shown to have a significant effect on a number of growth and survival parameters, with female adult fresh weight higher than male (Kulma et al., 2019; Ng'ang'a et al., 2020). Male A. domesticus have also been shown to have higher crude protein, and lower lipid content than females. No difference in fatty acid or amino acid content across sexes (Kulma et al., 2019).
The water content of feed substrates, as well as the humidity of the environment, have been shown to impact the growth and development of multiple Orthoptera species. Increased water availability from high water content vegetables was shown to increase A. domesticus body weight and length (Bawa et al., 2020), and the supplementation of fresh vegetables was shown to decrease water requirements for G. bimaculatus (Ng'ang'a et al., 2020) and A. domesticus (Vaga et al., 2019). Higher than ideal humidity levels have been shown to cause high mortality in T. testaceus (Miech et al., 2016). Meanwhile, lower environmental humidity levels have been attributed to behavioral changes in feed consumption, in which the smaller G. mitratus species increased consumption of fresh leaves to cope with the threat of desiccation (Fuah et al., 2015).
Micronutrients in feed have also been shown to impact the growth, development, weight gain, and survival. A. domesticus fed animal product-based feeds that contained Vitamin B12 developed and grew faster, perhaps due to increased food utilization efficiency (Morales-Ramos et al., 2020). Sterol, manganese, and Vitamin C had strongly positive effects on end biomass, and excessive consumption of vitamins B1 and B5 interacted negatively with other micronutrients to reduce biomass accumulation in A. domesticus (Morales-Ramos et al., 2020). These results align with the fact that Vitamins B1, B2, B3, and B5, as well as calcium, chlorine, copper, iron, magnesium, manganese, phosphorus, potassium, sodium, sulfur, and zinc are generally considered beneficial or essential for insects (Van Peer et al., 2021).
The presence of other molecules in feed may reduce growth and survival in A. domesticus. Such substances include D-ribose, D-xylose, D- and L-arabinose and L-sorbose (Neville and Luckey, 1962), as well as trypsin inhibitors, saponin, lectin, and anti-herbivore compounds such as glucosinolates (Quek et al., 2020). However, the presence of lectin in broad beans was not shown to limit the growth of the Gryllidae species R. differens (Sorjonen et al., 2020). In the same study, potato glycoalkaloids likely present in the feed were not shown to decrease R. differens performance. Further study of the impact of these molecules and compounds on Orthoptera growth and development is vital to understanding the specific requirements for successful alternative feeds.
Feed particles that are too large and lack uniformity have been shown to reduce feed intake and negatively impact growth parameters (Patton, 1967; Fuah et al., 2015). A behavioral preference was observed for soft-textured cassava leaves over tougher papaya leaves (Fuah et al., 2015), and for softer leaves and fruit peels rather than drier grains (Kinyuru and Kipkoech, 2018). The source and microbial contamination of feed is also potentially a major factor in growth and survival, as roadside collection of forage may introduce parasites or contamination, causing reduced survival (Miech et al., 2016).
Environmental factors dictate survival and growth parameters. For example, A. domesticus fed identical diets were shown to perform differently depending on group size (Morales-Ramos et al., 2020), large-scale environments were shown to incur greater metabolic costs (Lundy and Parrella, 2015), and temperature variation may affect mortality (Miech et al., 2016).
Nutritional variation by developmental stage has also been shown to significantly impact growth and survival parameters. A number of studies have shown that there is no observed difference in weight gain across experimental and control diets within the first 1–5 weeks of development, after which there is a sharp decline in weight gain observed for less nutritious alternative feeds (Patton, 1967; Ng'ang'a et al., 2020; Oloo et al., 2020; Vaga et al., 2020). Delaying the onset of lower-nutrient experimental diet regimes until 1–2 weeks after hatching ensures that the groups have equal metabolic capacity during later stages of significant growth (Lundy and Parrella, 2015; Dobermann et al., 2019). This phenomenon is demonstrated by the fact that S. icipe nymphs fed protein-deficient diets yield smaller adults (Magara et al., 2019). However, the same study also provides evidence that S. icipe adults are more susceptible to protein deficiencies than nymphs. The protein and fat content in crickets have also been shown to correspond with insect age (Kinyuru and Kipkoech, 2018). The protein content of A. domesticus has been shown to increase with age (Finke, 2002). These results imply overall that supplementing the diet based on developmental stage may be a viable option for alternative feeds but may result in smaller mean body weights regardless of what stage is exposed to more protein-deficient diets.
Sustainability
Farming insects as food and feed has great sustainability potential, but little research has been done to investigate the parameters by which sustainability should be measured, or the components necessary to produce insects sustainably. Research into the use of weeds, agricultural, and food industry byproducts as feed allows for an opportunity to answer these questions, as the ability of Orthoptera species to convert waste streams into accessible sources of nutrients is the focus of farming sustainable insects. Sustainability concerning human food resources encompasses an extremely broad range of definitions, and an even broader range of methods of possible interventions. For this paper, the sustainability of insects as a food source will be defined by the environmental impact of feed sources and the insect's efficiency of feed conversion.
The feed conversion ratio (FCR) of A. domesticus is lower than that of conventional livestock animals. The best performing (lowest) FCR in live weight for A. domesticus range from 1.1 to 2.3 (Lundy and Parrella, 2015; Oonincx et al., 2015; Miech et al., 2016; Veenenbos and Oonincx, 2017; Bawa et al., 2020; Vaga et al., 2020). In regards to Gryllidae, R. differens live weight FCR was demonstrated as low as 0.63 (Sorjonen et al., 2020). These are significantly lower than live weight FCR values of beef (10–12) and pork (5–6), and comparable to that of chicken (1.7–2.5) (Feddes et al., 2002; Smil, 2002a; Lundy and Parrella, 2015). However, the proportion of edible weight of crickets (80%) is significantly higher than that of beef (40%), chicken, and pork (55%) (Nakagaki and Defoliart, 1991; Flachowsky, 2002; Smil, 2002a). Thus, the edible weight FCR of A. domesticus (1.7) is less than half of that of chicken (4.2), and a fraction of pork (10.7) and beef (31.7) (Smil, 2002b; Lundy and Parrella, 2015). Similarly, the dry matter edible efficiency conversion of ingested food (ECI) is much more efficient for A. domesticus (21.1%), compared to that of poultry (9.9%), pork (8.5%) and beef (3.6%) (Nakagaki and Defoliart, 1991).
An increase in feed protein content has been shown to decrease FCR and increase ECI (Oonincx et al., 2015; Sorjonen et al., 2019). High-protein weed and byproduct diets resulted in high-performing live weight FCR values of 0.63–1.5 for various edible Orthoptera (Miech et al., 2016; Sorjonen et al., 2019, 2020; Bawa et al., 2020). These results imply that the metabolic efficiency of Orthoptera species may be improved when reared on sufficiently nutritious waste-sourced feeds. In two cases, however, the live weight FCR (Miech et al., 2016) and dry weight ECI (Morales-Ramos et al., 2020) did not vary between diet treatments with variations in macronutrient nutrient compositions. While this implies the influence of parameters besides macronutrient composition on food conversion efficiency, it also demonstrates that alternative feed sources can produce comparable FCR and ECI outcomes to conventional feeds.
If the successful growth and survival of crickets depends on the quality of the feed substrate, identifying scalable, high-quality feed sources is key to successfully integrating crickets as a protein source. Feed sources are the major contributor of emissions when raising animals for protein (Fiala, 2008; Lehner and Rosenberg, 2017); therefore, the ability of crickets to be raised on weeds and agricultural and food industry byproducts may allow them to be both less resource-intensive, and more efficient, than conventional meat livestock (Lundy and Parrella, 2015). With the edible weight FCR performance of Orthoptera fed low-quality weeds and agricultural and food industry byproducts surpassing that of poultry, taking advantage of resources that would otherwise be disposed of would render Orthoptera even more sustainable. Thus, waste can be reintroduced to the consumption cycle via the metabolic capabilities of insects and introduced an entirely new source of human nutrition with little to no loss of additional resources.
Another challenge to the sustainability of edible cricket operation is establishing continuous local supply of a nutritionally viable feed source (Van Peer et al., 2021). While identifying waste sources with high nutritional composition may seem difficult in terms of economic feasibility, such feeds produced greater weight gain, length, and protein content, which may be vital to making economical decisions on feed composition. Of the sustainable feed sources examined, several present viable options as resources for mass producing Orthoptera as a human food source. Based on the distribution of feed source types within successful experimental diets, the most successful sources for alternative feed are supplemented food waste, weed, or agricultural byproducts. One challenge is that these sources may also provide potential food resources for humans. An example is the cassava plant (Manihot esculenta), where the raw tops, leaves, or dried plant material has been ground into flour and used as a feed resource. Several studies have used cassava plant parts to great success, either as a supplement to commercial diets, or as a pure alternative feed source (Fuah et al., 2015; Megido et al., 2016; Miech et al., 2016). However, cassava plants are also commonly consumed by humans, making their use as insect feed counterproductive if the plant parts could be used to improve the diets of malnourished humans.
Shortcomings and Future Directions
There are several gaps in research and promising directions for future study. One major problem is the lack of study standardization. For example, the onset of experimental diets varies from <1 day old to 57 days old (Supplementary Table 1). This makes comparing performances in response to diet across studies difficult, as a variation in nutritional quality throughout the life cycle has been shown to cause differences in growth and survival across the entire lifetime (Patton, 1967; Ng'ang'a et al., 2020; Oloo et al., 2020; Vaga et al., 2020).
Feed measurements similarly lack standardization. Macronutrient extraction methods are not reliably disclosed, making it difficult to determine whether crude or pure protein contents are presented in nutrient composition tables. This is especially true in the case of fiber contents. Different studies may report crude fiber, acid detergent fiber, and neutral detergent fiber contents. Each of these fiber measurements varies in extraction methodology and thus content, making them impossible to compare across studies. The same problem applies to carbohydrates, which are vaguely listed under starch or nitrogen free extracts. Furthermore, the macro- and micronutrients disclosed vary widely between studies. While most studies include crude protein, crude fat, ash, and carbohydrate content of the feed, the significance of other parameters should not be overlooked.
While several interesting and useful feed parameters were described, they appeared in very few studies. These include fatty acid, vitamin, and amino acid contents, as well as microbial diversity and abundance. The ratio of collagen to total protein content of animal product-based feeds may be vital to establishing appropriate amino acid composition and content of a feed (Van Peer et al., 2021). Crickets have been shown to contain antinutrients, specifically tannins and phytic acid (Adeduntan, 2005). Antinutrient content of crickets is thought to be associated with allelochemicals present in plants that they feed on, but information on this subject is limited and warrants further investigation (Meyer-Rochow et al., 2021). Strong evidence for the importance of these micronutrient contents to determining growth and survival performance of Orthoptera demonstrates that a greater number of studies should provide and disclose such analysis of their feeds.
A lack of insect measurement standardization represents a further barrier to successful synthesis. While most studies examine mortality and weight, weight is listed under many different labels such as weight gain, mean weight, individual weight, weight yield, initial and final weight, live weight, and dry weight (Supplementary Table 1). Even less reliably disclosed are growth, ECI, FCR, crude protein content, and development rates. Furthermore, investigation into bacterial endospore presence in regards to diets and rearing environment presents an important and little-explored aspect to cricket rearing (Vandeweyer et al., 2017).
Misidentification of both insect and feed plant species is another issue that arises within this body of study. Given the evidence that species show differences in growth, survival, and feed conversion ratio performance, correct identification of insect species is vital to designing feed parameters for a rearing candidate species. Studies should follow current classification including taxonomic synonyms (Fuah et al., 2015) and provide rigorous methods for the identifications, including the deposition of vouchers.
Overall standardization and clearer disclosure of experimental diet onset, nutrient extraction methods, feed measurements, insect measurements, and rigorous species identification would make a synthesis of performances across studies of edible Orthoptera fed weeds and agricultural and food industry byproducts much more rigorous.
Conclusion
This article reviews the literature on the performance of edible Orthoptera raised on diets of weeds, agricultural and food industry byproducts. In doing so, we provide an overview of the common methods and practices of such studies and clarify requirements for future directions in the field. This review focused on 22 studies conducted between 1967 and 2020, which represented the most relevant articles surrounding the growth, survival, and other performance outcomes of edible Orthoptera fed diets composed partly or wholly of alternative feeds.
The focus of the discussion was on the effects of feed substrate and macronutrient composition on survival, weight, development, efficiency, and nutritional outcomes of the insects. Overall, positive trends in performance were identified with higher crude protein, carbohydrate, and fat compositions. However, there exists some nuance across studies as to whether macronutrient content alone can account for differences in insect performance. Thus, attention should be paid to other aspects of feed composition such as vitamins, minerals, amino acids, and fatty acids to confidently prescribe an ideal feed. Additionally, differences in performances were observed across Orthoptera species fed the same diet, implying that species-specific metabolic capacities warrant consideration.
The overall performance of edible Orthoptera raised on weeds, agricultural, and food industry byproducts is promising. There is much evidence to suggest that organic waste resources of sufficient quality can produce Orthoptera as successfully as conventional commercial insect feeds. This suggests that Orthoptera may be reared as a human food source both sustainably and inexpensively, as feed resources could be sourced from locally available resources. This breakthrough in sustainable protein production for human consumption could not only provide vital food sources to malnourished and impoverished communities otherwise unable to feed themselves, but also a revolution in the sustainability of conventional livestock agriculture in developed nations.
Author Contributions
CK: data curation, methodology, formal analysis, visualization, writing – original draft, and writing – reviewing and editing. BF: conceptualization, funding acquisition, resources, methodology, supervision, and writing – reviewing and editing. Both authors contributed to the article and approved the submitted version.
Funding
The research was supported in part by National Science Foundation, USA DEB-1856400.
Conflict of Interest
The authors declare that the research was conducted in the absence of any commercial or financial relationships that could be construed as a potential conflict of interest.
Publisher's Note
All claims expressed in this article are solely those of the authors and do not necessarily represent those of their affiliated organizations, or those of the publisher, the editors and the reviewers. Any product that may be evaluated in this article, or claim that may be made by its manufacturer, is not guaranteed or endorsed by the publisher.
Supplementary Material
The Supplementary Material for this article can be found online at: https://www.frontiersin.org/articles/10.3389/fsufs.2021.810421/full#supplementary-material
References
Adeduntan, S. A. (2005). Nutritonal and antinutritional characteristics of some insects foragaing in akure forest reserve Ondo State, Nigeria. J. Food Technol. 3, 563–567.
Akhtar, Y., and Isman, M. B. (2018). “10 - Insects as an alternative protein source,” in Proteins in Food Processing, 2nd Edn. Series in Food Science, Technology and Nutrition, eds R. Y. Yada (Cambridge: Woodhead Publishing), 263–288.
Bawa, M., Songsermpong, S., Kaewtapee, C., and Chanput, W. (2020). Effect of diet on the growth performance, feed conversion, and nutrient content of the house cricket. J. Insect Sci. 20, 2. doi: 10.1093/jisesa/ieaa014
Caro, D., Davis, S. J., Bastianoni, S., and Caldeira, K. (2014). Global and regional trends in greenhouse gas emissions from livestock. Clim. Change 126, 203–216. doi: 10.1007/s10584-014-1197-x
Chakravorty, J., Ghosh, S., Jung, C., and Meyer-Rochow, V. B. (2014). Nutritional composition of Chondacris rosea and Brachytrupes orientalis: two common insects used as food by tribes of Arunachal Pradesh, India. J. Asia Pac. Entomol. 17, 407–415. doi: 10.1016/j.aspen.2014.03.007
Choo, B. Y. R., Yan, A. T., Loh, R. H. J., Chin, Z. Y., Jo, C. S., Lee, K. E. M., et al. (2017). Can waste vegetables and weeds be used to farm crickets and grasshoppers in Singapore. Utar Agric. Sci. J. 3, 18–22.
Delgado, C., Rosegrant, M., Steinfeld, H., Ehui, S., and Courbois, C. (2001). Livestock to 2020: the next food revolution. Outlook Agric. 30, 27–29. doi: 10.5367/000000001101293427
Dobermann, D., Michaelson, L., and Field, L. m. (2019). The effect of an initial high-quality feeding regime on the survival of Gryllus bimaculatus (black cricket) on bio-waste. J. Insects Food Feed. 5, 117–123. doi: 10.3920/JIFF2018.0024
Escamilla-Rosales, M. F., Ariza-Ortega, J. A., Ramos-Cassellis, M. E., Castañeda-Antonio, D., Romo-Gómez, C., Díaz-Reyes, J., et al. (2019). Comparison of the proximal chemical and fatty acid composition of the fried grasshopper's (Orthoptera) dish. Eur. Food Res. Technol. 245, 1629–1640. doi: 10.1007/s00217-019-03272-6
FAO (2020). The State of Food Security and Nutrition in the World 2020: Transforming Food Systems for Affordable Healthy Diets. Rome, Italy: FAO, IFAD, UNICEF, WFP and WHO.
Feddes, J., Emmanuel, E., and Zuidhoft, M. (2002). Broiler performance, body weight variance, feed and water intake, and carcass quality at different stocking densities. Poult. Sci. 81, 774–779. doi: 10.1093/ps/81.6.774
Fiala, N. (2008). Meeting the demand: An estimation of potential future greenhouse gas emissions from meat production. Ecol. Econ. 67, 412–419. doi: 10.1016/j.ecolecon.2007.12.021
Finke, M. D. (2002). Complete nutrient composition of commercially raised invertebrates used as food for insectivores. Zoo Biol. 21, 269–285. doi: 10.1002/zoo.10031
Flachowsky, G. (2002). Efficiency of energy and nutrient use in the production of edible protein of animal origin. J. Appl. Anim. Res. 22, 1–24. doi: 10.1080/09712119.2002.9706374
Fuah, A. M., Siregar, H. C. H., and Endrawati, Y. C. (2015). Cricket farming for animal protein as profitable business for small farmers in Indonesia. J. Agric. Sci. Technol. 5, 4. doi: 10.17265/2161-6256/2015.04.008
Gerber, P. J., and FAO (2013). Tackling Climate Change Through Livestock: A Global Assessment of Emissions and Mitigation Opportunities. Rome: Food and Agriculture Organization of the United Nations.
Ghosh, S., Lee, S.-M., Jung, C., and Meyer-Rochow, V. (2017). Nutritional composition of five commercial edible insects in South Korea. J. Asia Pac. Entomol. 20, 2. doi: 10.1016/j.aspen.2017.04.003
Halloran, A., Megido, R. C., Oloo, J., Weigel, T., Nsevolo, P., and Francis, F. (2018). Comparative aspects of cricket farming in Thailand, Cambodia, Lao People's Democratic Republic, Democratic Republic of the Congo and Kenya. J. Insects Food Feed 4, 101–114. doi: 10.3920/JIFF2017.0016
Harsányi, E., Juhász, C., Kovács, E., Huzsvai, L., Pintér, R., Fekete, G., et al. (2020). Evaluation of organic wastes as substrates for rearing zophobas morio, tenebrio molitor, and acheta domesticus larvae as alternative feed supplements. Insects 11, 1–18. doi: 10.3390/insects11090604
Kinyuru, J. N., and Kipkoech, C. (2018). Production and growth parameters of edible crickets: experiences from a farm in a high altitude, cooler region of Kenya. J. Insects Food Feed 4, 247–251. doi: 10.3920/JIFF2017.0081
Kulma, M., Kourimsk,á, L., Plach,ý, V., BoŽik, M., Adámkov,á, A., and Vrabec, V. (2019). Effect of sex on the nutritional value of house cricket, Acheta domestica L. Food Chem. 272, 267–272. doi: 10.1016/j.foodchem.2018.08.049
Lehner, P., and Rosenberg, N. (2017). Legal Pathways to Carbon-Neutral Agriculture. Rochester, NY: Social Science Research Network. Environmental Law Institute, 32p.
Lundy, M. E., and Parrella, M. P. (2015). Crickets are not a free lunch: protein capture from scalable organic side-streams via high-density populations of acheta domesticus. PLoS ONE 10, e0118785. doi: 10.1371/journal.pone.0118785
Magara, H. J. O., Niassy, S., Ayieko, M. A., Mukundamago, M., Egonyu, J. P., Tanga, C. M., et al. (2021). Edible crickets (Orthoptera) around the world: distribution, nutritional value, and other benefits—a review. Front. Nutr. 7, 257. doi: 10.3389/fnut.2020.537915
Magara, H. J. O., Tanga, C. M., Ayieko, M. A., Hugel, S., Mohamed, S. A., Khamis, F. M., et al. (2019). Performance of newly described native edible cricket Scapsipedus icipe (Orthoptera: Gryllidae) on various diets of relevance for farming. J. Econ. Entomol. 112, 653–664. doi: 10.1093/jee/toy397
Makkar, H., Tran, G., Heuz,é, V., and Ankers, P. (2014). State-of-the-art on use of insects as animal feed. Anim. Feed Sci. Technol. 197, 1–33. doi: 10.1016/j.anifeedsci.2014.07.008
Mbow, C., Rosenzweig, C., Barioni, L. G., Benton, T. G., Herrero, M., Krishnapillai, M., et al. (2019). “Food security,” in Climate Change and Land: An IPCC Special Report on Climate Change, Desertification, Land Degradation, Sustainable Land Management, Food Security, and Greenhouse Gas Fluxes in Terrestrial Ecosystems. IPCC. Available online at: https://www.ipcc.ch/srccl/chapter/chapter-5/ (accessed September 9, 2021).
Megido, R. C., Alabi, T., Nieus, C., Blecker, C., Danthine, S., Bogaert, J., et al. (2016). Optimisation of a cheap and residential small-scale production of edible crickets with local by-products as an alternative protein-rich human food source in Ratanakiri Province, Cambodia. J. Sci. Food Agric. 96, 627–632. doi: 10.1002/jsfa.7133
Meyer-Rochow, V. (1975). Can insects help to ease the problem of world food shortage? Search 6, 261–262.
Meyer-Rochow, V. B., Gahukar, R. T., Ghosh, S., and Jung, C. (2021). Chemical Composition, nutrient quality and acceptability of edible insects are affected by species, developmental stage, gender, diet, and processing method. Foods 10, 1036. doi: 10.3390/foods10051036
Miech, P., Berggren, Å., Lindberg, J. e, Chhay, T., Khieu, B., and Jansson, A. (2016). Growth and survival of reared Cambodian field crickets (Teleogryllus testaceus) fed weeds, agricultural and food industry by-products. J. Insects Food Feed 2, 285–292. doi: 10.3920/JIFF2016.0028
Misra, M. (2018). Moving away from technocratic framing: agroecology and food sovereignty as possible alternatives to alleviate rural malnutrition in Bangladesh. Agric. Human Values. 35, 473–487. doi: 10.1007/s10460-017-9843-3
Morales-Ramos, J. A., Rojas, M. G., Dossey, A. T., and Berhow, M. (2020). Self-selection of food ingredients and agricultural by-products by the house cricket, Acheta domesticus (Orthoptera: Gryllidae): a holistic approach to develop optimized diets. PLoS ONE 15, e0227400. doi: 10.1371/journal.pone.0227400
Nakagaki, B. J., and Defoliart, G. R. (1991). Comparison of diets for mass-rearing Acheta domesticus (Orthoptera: Gryllidae) as a novelty food, and comparison of food conversion efficiency with values reported for livestock. J. Econ. Entomol. 84, 891–896. doi: 10.1093/jee/84.3.891
Neville, P. F., and Luckey, T. D. (1962). Carbohydrate and roughage requirement of the cricket, Acheta domesticus. J. Nutr. 78, 139–146. doi: 10.1093/jn/78.2.139
Ng'ang'a, J., Imathiu, S., Fombong, F., Borremans, A., Van Campenhout, L., Broeck, J. V., et al. (2020). Can farm weeds improve the growth and microbiological quality of crickets (Gryllus bimaculatus)? J. Insects Food Feed 6, 199–209. doi: 10.3920/JIFF2019.0051
Oji, U. I., Etim, H. E., and Okoye, F. C. (2007). Effects of urea and aqueous ammonia treatment on the composition and nutritive value of maize residues. Small Ruminant Res. 69, 232–236. doi: 10.1016/j.smallrumres.2006.01.015
Oloo, J. A., Ayieko, M., and Nyongesah, J. M. (2020). Acheta domesticus (Cricket) feed resources among smallholder farmers in Lake Victoria region of Kenya. Food Sci. Nutr. 8, 69–78. doi: 10.1002/fsn3.1242
Oonincx, D. G. A. B., and Boer, I. J. M. (2012). Environmental impact of the production of mealworms as a protein source for humans – a life cycle assessment. PLoS ONE 7, e51145. doi: 10.1371/journal.pone.0051145
Oonincx, D. G. A. B., Itterbeeck, J., van Heetkamp, M. J. W., Brand, H., van, den, Loon, J. J. A., van, Huis, A., and van (2010). An exploration on greenhouse gas and ammonia production by insect species suitable for animal or human consumption. PLoS ONE 5, e14445. doi: 10.1371/journal.pone.0014445
Oonincx, D. G. A. B., Van Broekhoven, S., Van Huis, A., and Van Loon, J. J. A. (2015). Feed conversion, survival and development, and composition of four insect species on diets composed of food by-products. PLoS ONE 14, 10. doi: 10.1371/journal.pone.0222043
Orinda, M., Mosi, R., Ayieko, M., and Amimo, F. (2017). Growth performance of Common House cricket and Field Cricket fed on agro byproducts. J. Entomol. Zool. Stud. 5, 1664–1668.
Parfitt, J., Barthel, M., and Macnaughton, S. (2010). Food waste within food supply chains: quantification and potential for change to 2050. Philos. Trans. R. Soc. B Biol. Sci. 365, 3065–3081. doi: 10.1098/rstb.2010.0126
Patton, R. L. (1967). Oligidic diets for Acheta domesticus (Orthoptera: Gryllidae). Ann. Entomol. Soc. Am. 60, 1238–1242. doi: 10.1093/aesa/60.6.1238
Quek, X. T., Liang, L., Tham, H. H., Yeo, H., Tan, M. K., and Tan, H. T. W. (2020). Are the growth and survival of Acheta domesticus comparable when reared on okara, waste vegetables and premium animal feed? J. Insects Food Feed 6, 161–168. doi: 10.3920/JIFF2019.0039
Saha, U. K., Sonon, L. S., Hancock, D. W., Hill, N. S., Stewart, L., Heusner, G. L., et al. (2010). Common Terms Used in Animal Feeding and Nutrition. Athens: The University of Georgia Cooperative Extension, College of Agricultural and Environmental Sciences and Family and Consumer Sciences.
Salomone, R., Saija, G., Mondello, G., Giannetto, A., Fasulo, S., and Savastano, D. (2017). Environmental impact of food waste bioconversion by insects: application of life cycle assessment to process using Hermetia illucens. J. Clean. Prod. 140, 890–905. doi: 10.1016/j.jclepro.2016.06.154
Sampson, D., Cely-Santos, M., Gemmill-Herren, B., Babin, N., Bernhart, A., Bezner Kerr, R., et al. (2021). Food sovereignty and rights-based approaches strengthen food security and nutrition across the globe: a systematic review. Front. Sustain. Food Syst. 5, 288. doi: 10.3389/fsufs.2021.686492
Sánchez-Muros, M.-J., Barroso, F. G., and Manzano-Agugliaro, F. (2014). Insect meal as renewable source of food for animal feeding: a review. J. Clean. Prod. 65, 16–27. doi: 10.1016/j.jclepro.2013.11.068
Smil, V. (2002a). Eating meat: evolution, patterns, and consequences. Popul. Dev. Rev. 28, 599–639. doi: 10.1111/j.1728-4457.2002.00599.x
Smil, V. (2002b). Nitrogen and food production: proteins for human diets. Ambio 31, 126–131. doi: 10.1579/0044-7447-31.2.126
Sorjonen, J. M., Lehtovaara, V. J., Immonen, J., Karhap,ää, M., Valtonen, A., and Roininen, H. (2020). Growth performance and feed conversion of Ruspolia differens on plant-based by-product diets. Entomol. Exp. Appl. 168, 460–471. doi: 10.1111/eea.12915
Sorjonen, J. M., Valtonen, A., Hirvisalo, E., Karhapää, M., Lehtovaara, V. J., Lindgren, J., et al. (2019). The plant-based by-product diets for the mass-rearing of Acheta domesticus and Gryllus bimaculatus. PLoS ONE 14, e0218830. doi: 10.1371/journal.pone.0218830
Steinfeld, H., Gerber, P., Wassenaar, T. D., Nations F. A. O. of the U., Castel, V., and Rosales, M. et al. (2006). Livestock's Long Shadow: Environmental Issues and Options. Rome: Food & Agriculture Organization of the United Nations.
Vaga, M., Berggren, Å., and Jansson, A. (2020). Growth, survival and development of house crickets (Acheta domesticus) fed flowering plants. J. Insects Food Feed 7, 151–161. doi: 10.3920/JIFF2020.0048
Vaga, M., Berggren, Å., Pauly, T., and Jansson, A. (2019). Effect of red clover-only diets on house crickets (Acheta domesticus) growth and survival. J. Insects Food Feed 6, 179–189. doi: 10.3920/JIFF2019.0038
van Huis, A., Van Itterbeeck, J., Klunder, H., Mertens, E., Halloran, A., Muir, G., et al. (2013). Edible Insects Future Prospects for Food and Feed Security. Rome: Food and Agriculture Organization of the United Nations.
Van Peer, M., Frooninckx, L., Coudron, C., Berrens, S., Álvarez, C., Deruytter, D., et al. (2021). Valorisation potential of using organic side streams as feed for Tenebrio molitor, Acheta domesticus and Locusta migratoria. Insects 12, 796. doi: 10.3390/insects12090796
van Zanten, H. H. E., Mollenhorst, H., Oonincx, D. G. A. B., Bikker, P., Meerburg, B. G., and de Boer, I. J. M. (2015). From environmental nuisance to environmental opportunity: housefly larvae convert waste to livestock feed. J. Clean. Prod. 102, 362–369. doi: 10.1016/j.jclepro.2015.04.106
Vandeweyer, D., Crauwels, S., Lievens, B., and Van Campenhout, L. (2017). Metagenetic analysis of the bacterial communities of edible insects from diverse production cycles at industrial rearing companies. Int. J. Food Microbiol. 261, 11–18. doi: 10.1016/j.ijfoodmicro.2017.08.018
Vauterin, A., Steiner, B., Sillman, J., and Kahiluoto, H. (2021). The potential of insect protein to reduce food-based carbon footprints in Europe: the case of broiler meat production. J. Clean. Prod. 320, 128799. doi: 10.1016/j.jclepro.2021.128799
Keywords: edible insects, food waste, sustainable farming, nutrition, regenerative agriculture, protein
Citation: Kuo C and Fisher BL (2022) A Literature Review of the Use of Weeds and Agricultural and Food Industry By-Products to Feed Farmed Crickets (Insecta; Orthoptera; Gryllidae). Front. Sustain. Food Syst. 5:810421. doi: 10.3389/fsufs.2021.810421
Received: 06 November 2021; Accepted: 27 December 2021;
Published: 31 January 2022.
Edited by:
Uma Tiwari, Technological University Dublin, IrelandReviewed by:
Victor Benno Meyer-Rochow, University of Oulu, FinlandCaswell Munyai, University of KwaZulu-Natal, South Africa
Copyright © 2022 Kuo and Fisher. This is an open-access article distributed under the terms of the Creative Commons Attribution License (CC BY). The use, distribution or reproduction in other forums is permitted, provided the original author(s) and the copyright owner(s) are credited and that the original publication in this journal is cited, in accordance with accepted academic practice. No use, distribution or reproduction is permitted which does not comply with these terms.
*Correspondence: Brian L. Fisher, bfisher@calacademy.org