- 1Department of Food Studies, New York University, New York City, NY, United States
- 2Union of Concerned Scientists, Oakland, CA, United States
- 3Center for Data Sciences, New York University, New York City, NY, United States
- 4Department of Environmental Studies, New York University, New York City, NY, United States
- 5Sustainable Food and Farming Program, Earth Justice, New York, NY, United States
Recent calls for a global food transformation have centered on simultaneously improving human and environmental health, recognizing that food and nutrient diversity have declined over time while food systems have exacted a heavy climate and ecological toll. Grain legumes and coarse grain crops provide important human nutrition and environmental benefits, but the production and consumption of many of these crops remains relatively low compared to major commodities, such as maize, wheat, rice, and soy. Outstanding hurdles to scaling up these “minor commodity” crops include (among other things) their relatively lower yields, and lower farmer adoption, based partly on actual or perceived profitability and marketability. We hypothesize that these limitations are attributable in part to unequal funding for these crops' research and development (R&D) both on a national and global scale. In the United States, we show that investment patterns for a snapshot of USDA-funded research grants from 2008 to 2019 consistently favor major commodity crops, which received 3 to 4.5 times more funding and 3 to 5 times as many grants than the minor commodity crop groups. This current USDA funding allocation poses a barrier to food system transformations. Achieving nutritious diets for planetary health requires more public agricultural investment toward minor commodity crops and increased collaboration between public health, nutrition, agriculture, and environmental sectors.
Twentieth Century Agricultural Development and Current Trends
Global agricultural production was transformed by twentieth century technologies. In the United States, research and development (R&D) funding was structured to boost productivity of a select few cereal crops, and these trends carried over to impact production in other countries as well (due in part to globalization/global politics) (Cullather, 2013). Notable developments during this period included high-yielding seed varieties and the industrial production of synthetic, nitrogenous fertilizers and other inputs—technologies that catalyzed unprecedented calorie production per acre (Evenson and Gollin, 2003). These technological changes nearly tripled crop yields (Pingali, 2012; Gordon et al., 2017), and as adoption increased, native seed and crop varieties were increasingly phased out from crop rotations (DeFries et al., 2015).
Shifting agricultural focus toward higher yields, net farm returns, and access to global markets has encouraged more homogenous cropping systems (Welch and Graham, 2000) centered around a select few major commodity crops, namely maize, wheat, rice and later soybean (Eiselen and Webb, 2009). The percentage of global cereal area occupied by maize, wheat, and rice rose from 66 to 79% between 1961 and 2013, while land area devoted to barley, oats, rye, millet, and sorghum (all minor commodity grains) declined from 33 to 19% (DeFries et al., 2015). A diverse variety of minor commodity legumes and vegetables were also supplanted by shifts in the production landscape. India's post-colonial agricultural development, for example, was primarily characterized by the wide expansion of rice and wheat production, thereby decreasing the prevalence and diversity of coarse grains and pulses (Davis et al., 2019). Within the Philippines, farmers' adoption of intensive rice cultivation displaced a variety of nutrient-dense, leafy green vegetables (Cagauan, 1995). In the United States, major commodities were already planted over large areas by the 1960's, but their production increased over the next five decades (Figure 1A), far exceeding that of minor commodities, such as grain legumes and coarse grains (Figure 1A, see Table 1 for included crop species).
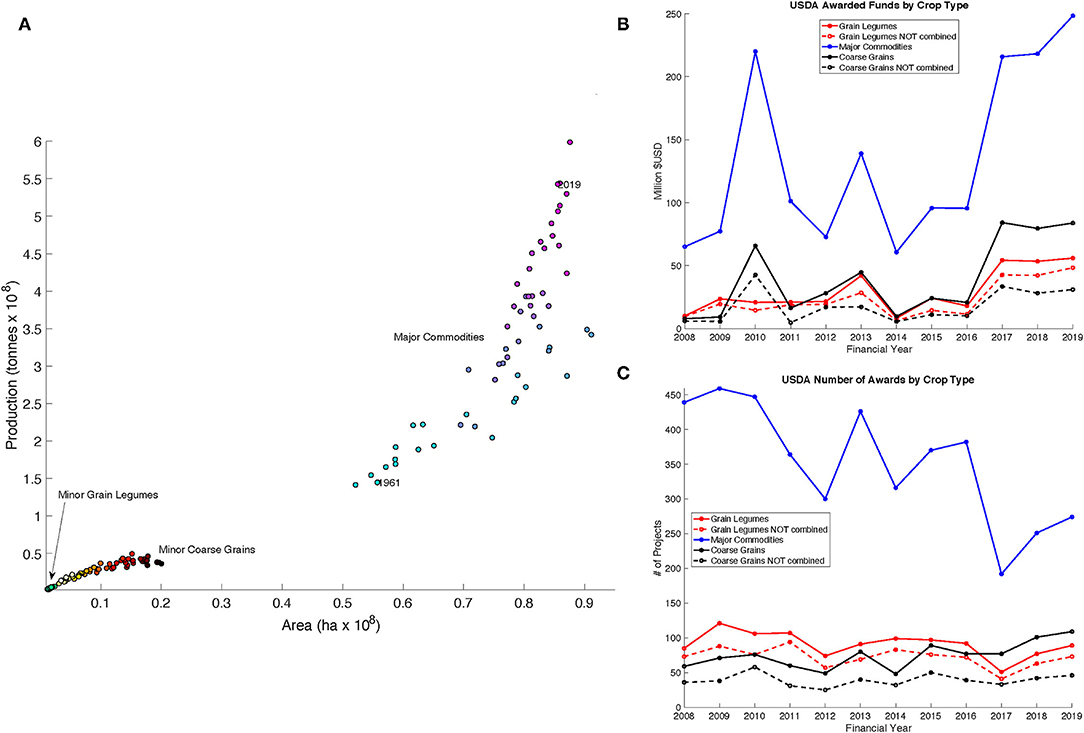
Figure 1. (A) Production vs. Area Planted per crop group (Table 1) in the United States from 1961 to 2019. In panel A, three different color gradients (one for each crop group) are used to represent the temporal dimension. For major commodities, light blue indicates earlier years while bright pink represents later years. For minor coarse grains, whose area is declining, darker reds represent earlier years while white/yellow represents later years. Minor grain legumes are shown in green (no gradient), and there is little change over time in both production and area planted. Data is obtained from FAOSTAT. (B) Total USDA awarded funds made annually from 2008 to 2019 to grain legumes, coarse grains, and major commodities (Table 1) and (C) Number of USDA awards made annually by crop group. Grain legumes NOT combined and Coarse Grains NOT combined (dashed lines) excludes maize, rice, wheat, and soy. Grant data was obtained from the Federal RePORTER database (https://federalreporter.nih.gov) using a regular expression approach with the Advanced Search function based on the crops listed in Table 1. These searches yielded 4,220 funded projects on major commodity crops, 896 funded projects on minor coarse grains, and 1,089 funded projects on minor grain legumes. We note that not all US government agencies reported consistently to Federal RePORTER from 2008 to 2019. As such, it is possible that funding levels for all crop species may be higher than what is shown here.
These developments have led to a substantial decrease in total crop diversity in the global food supply (Khoury et al., 2014). Despite the 7000+ plant species that have been cultivated for food production over the course of human history, 66% of total crop production in 2018 was accounted for by just nine crop species (FAO, 2019). In this analysis, we explore how these trends are underscored in part by systematic disparities in research funding for major and minor crop groups.
Impact of Current Agricultural Systems on Human Health and the Environment
Despite successfully increasing yields, global agriculture's increased crop homogeneity, both in species and variety, has nevertheless impacted the health of both humans and the environment (Weis, 2007; Pingali, 2012; Gordon et al., 2017). While production and consumption patterns vary among countries, the current agricultural landscape has serviced higher intakes of refined grains, starches, and carbohydrates; vegetable oils; and meat (Kearney, 2010; Popkin et al., 2012; Tilman and Clark, 2014). Twentieth century gains in public health due to increased calorie availability have thus been partly offset by shifts toward unhealthy diets that lack nutritional diversity [Remans et al., 2014; Research Institute (IFPRI), 2017]. Furthermore, rates of chronic non-communicable diet-related diseases, including coronary heart disease, stroke, and type 2 diabetes, continue to rise (Hu, 2011; Zhou et al., 2016; Willett et al., 2019).
Mounting evidence shows that modern agriculture is also a major driver of global environmental change (Stevenson et al., 2013; Campbell et al., 2017). Agriculture and related land use, combined with post-production food system activities, are responsible for 21–37% or more of total net anthropogenic GHG emissions (IPCC, 2019; Clark et al., 2020; Tubiello et al., 2021). Additionally, agriculturally-driven land use and land cover change, resulting partly from expanding a few major commodities, is a leading driver of nutrient pollution, habitat loss and degradation, and biodiversity loss (Campbell et al., 2017; Tilman et al., 2017; Henry et al., 2019).
Needs for a “Great Food Transformation”
Addressing human and environmental health consequences of industrial agriculture demands agroecosystems that prioritize multiple goals inclusive of food and nutrition security, environmental and biodiversity protection, and improved ecosystem services (Cassidy et al., 2013; IPCC, 2019; Willett et al., 2019). In early 2019, the EAT-Lancet Commission, a coalition of 37 scientists studying the intersection of nutrition and agriculture, called for a global food transformation that supports human health and the environment simultaneously and sustainably into the future: a “diet for planetary health” (Willett et al., 2019).
Key to achieving these goals is the increased diversification of cropping systems as well as the consumption of a greater variety of plant-based foods in nutritious diets (Willett et al., 2019). Minor commodity legumes and coarse grains (e.g., Table 1) are generally much more nutrient dense than the dominant major commodity varieties, particularly after processing by industrial methods (Meng et al., 2005; Ortiz-Monasterio et al., 2007). Legumes (e.g., lentil, peas and cowpeas) contain approximately three times more zinc, five times more iron and magnesium, and six times more calcium than maize, wheat, and rice (Longvah et al., 2017). Other coarse grains (e.g., sorghum, millet, and barley) also have similar, higher micro-nutrient values (Longvah et al., 2017). There also exist thousands of varieties (traditional and wild) of maize, rice, and wheat, with potential to provide both higher nutrient content and adaptation to local growing conditions (Mammadov et al., 2018). However, cultivation of these varieties has also declined with the advent of higher-yielding crops (Hellin et al., 2014; Eliazer-Nelson et al., 2019; Rathna Priya et al., 2019).
Expanding minor commodity grain legume and coarse grain production also aligns with climate change adaptation and mitigation goals (Foyer et al., 2016; Tamburini et al., 2020). Many species of grain legumes and coarse grains display natural propensities for drought and heat tolerance, conditions that occur frequently in sub-tropical and semi-arid regions, where some nations are experiencing disproportionate food insecurity and climate change impacts (Cagauan, 1995; Foyer et al., 2016; Cullis and Kunert, 2017). Furthermore, these crops' relatively low water demand also facilitates conserving increasingly limited water resources (Cagauan, 1995; Davis et al., 2018; Clark et al., 2019). While their efficacy can vary regionally (Palm et al., 2001), grain legumes (e.g., see Table 1) in particular may also reduce nitrous oxide emissions and nutrient runoff (Piotrowska-Dlugosz and Wilczewski, 2012; Xie et al., 2016). Moreover, these same characteristics, particularly if further improved, can also contribute to climate change mitigation (Foyer et al., 2016). Integrating grain legumes into conventional rotations of a single commodity crop (e.g., maize) may significantly increase soil carbon sequestration potential (Lal, 2010; Minasny et al., 2017; Stagnari et al., 2017) and, as a fresh food source, they also exhibit relatively low global warming potential (0.51 kg CO2 eq kg−1) compared to more commonly produced cereals (1.10 kg CO2 eq kg-1) across the lifecycle of their production (Clune et al., 2017).
However, while these minor commodity crop groups have many valuable characteristics, they are generally less productive than major commodity grains (Ramakrishna et al., 2000; Joshi and Rao, 2017), and farmers may lack effective management options, particularly related to pests, diseases, and weed pressure, specific to these crops (Siddique et al., 2012; Rubiales et al., 2015; Farooq et al., 2017). These production and adoption gaps persist partly because these minor commodity crops have not been a focus of twentieth century R&D, and therefore have not received the same systematic support to boost productivity that major commodities have received. Indeed, some institutions are committed to the breeding and improvement of such crops, e.g., the International Crops Research Institute for the Semi-Arid Tropics (ICRISAT), the International Center for Agricultural Research in the Dry Areas (ICARDA), and the Indian Institute of Pulses Research. However, particularly in industrialized countries like the United States, there remains a large gap in the current scale and coordination of R&D directed at these minor commodity crops compared to what major commodity crops receive (Tadele, 2019). To fully harness the potential of these minor commodity crops for improved planetary health, greater public investment into research dedicated to improving these crops, and their application to diversified cropping systems, is needed (Foyer et al., 2016; Stagnari et al., 2017).
Crop Research and Development Investment Gaps in the United States
Agricultural R&D investment is a key means of improving crops and agricultural systems across a range of measures, including productivity, climate change resilience, and environmental impact (Pingali, 2012; Pardey et al., 2014). R&D investments, alongside other mechanisms such as subsidies and crop insurance, also play an important role in determining not only which crops and agricultural systems prevail, but also how those crops and systems change over time (Alston et al., 2011; DeLonge et al., 2016; Pimbert and Moeller, 2018; Biovision Foundation for Ecological Development IPES-Food, 2020). Public R&D funding, as opposed to private sector-driven research, is especially important because such investments can be directed to serve planetary health goals and the public good more broadly (Lehner and Rosenberg, 2018).
It has been well-established that agricultural R&D investments are economically beneficial by way of enhancing crop production via improved varieties, multifactor productivity, and pest/disease/weed resistance (Steensland, 2019; International Food Policy Research Institute, 2020; Baldos and Blaustein-Reito, 2021; Ortiz-Bobea et al., 2021). A large body of work exists to understand and quantify the role of (public) agricultural R&D in twentieth century crop improvements (Alston et al., 2000; Evenson, 2002; Baldos et al., 2018; Steensland, 2019), and it is now widely accepted that such investments were a primary driver of these gains (Alston et al., 2000, 2009; Baldos et al., 2018; Steensland, 2019). Due to the close relationship between public R&D investment and crop productivity, there are calls to increase such investments across the world to improve food security outcomes (Andersen and Song, 2013; Baldos and Blaustein-Reito, 2021).
Despite proven payoffs, public agricultural research funding within the United States has declined in recent decades, and there is concern that these declining investments will reduce domestic productivity (Pardey et al., 2006, 2012; Clancy et al., 2016). Furthermore, critical areas needed for food system transformation are lacking in existing public investments. In particular, an analysis of United States Department of Agriculture (USDA) research investments and projects totaling nearly $300 million discovered that <15% of total funding was distributed to projects that incorporated any element of agroecology, while the largest portion of funds was allocated toward projects aiming to boost crop productivity (DeLonge et al., 2016). A similar analysis showed how investments in “sustainable nutrition science”—research and education at the intersection of food production, climate and environment, and nutrition—reached only $15.7 million between FY2016 and FY2019 (Reinhardt, 2021). Important areas of agricultural research, like diversified cropping systems, have not had an equal opportunity to reap the benefits that transpire from consistent governmental funding and support (Miles et al., 2017).
In our review of research funding between 2008 and 2019, minor commodity grain legumes and coarse grains (see Table 1 for included species) systematically received far less research funding from the USDA, both in the total dollars spent and in the number of grants funded, than the major commodity crop group (Figures 1B,C). Averaged over this decade, the major commodities received nearly quadruple the number of grants and 4.5 times more funding than the minor commodity grain legumes group and nearly 5 times as many grants and 3 times more funding than the minor commodity coarse grains group. While the number of major commodity crop awards has actually declined (459 grants in 2009 down to 274 grants in 2019, Figure 1C), the awarded funds have increased since 2016 (Figure 1B). The amount of funding issued per grant for major commodities has risen from a mean of $148,000 in 2008 to $906,000 in 2019 (sd = $321,500—relative to 2019 mean). Minor commodity coarse grains and grain legumes similarly saw increased funding since 2016, although their overall funding levels remain low in comparison to the major commodities. The amount of funding issued per grant for grain legumes has risen from a mean of $118,000 in 2008 to $629,000 in 2019 (sd = $281,000—relative to 2019 mean) and for coarse grains it has gone from a mean of $133,000 to $768,000 (sd = $311,500—relative to 2019 mean). Despite per-project funding increases for minor commodity crop groups, they are still underrepresented in terms of area planted, production (Figure 1A) and overall funding in US agriculture, and thus require additional investment in order to maximize their contributions to planetary health.
Discussion: Toward a “Global Food Transformation”
Given the crop and agricultural system advancements that result from dedicated R&D investment, the current USDA funding distribution, which favors major commodity crops, is an important barrier to the improvement of minor commodity crops and their utility to planetary health. Overcoming this barrier is crucial, particularly in the context of declining agricultural investments (Clancy et al., 2016) and the growing call for food production that better supports both human health and the environment. Diverse agricultural landscapes and diets with a variety of minor commodity crops could help fulfill the multi-fold goals of providing food and nutrition security, environmental restoration, and climate mitigation and adaptation. However, this shift requires (1) a major re-orientation of public agricultural investment toward minor commodities and (2) increased collaboration across public programs designed to promote food security and meet environment, climate, and conservation goals.
Reorienting Public Agricultural Investment
Public research investment into minor commodity crops must increase in order to meet the full potential of these crops to serve human and planetary health. This repurposing of agricultural production support is essential so that measures that are typically unsustainable can be replaced with those that lead to diversification toward more nutritious foods (FAO et al., 2021). A portion of this research funding should target crop-specific improvements to advance these crops' roles in improving agroecosystem resilience to climate shocks, profitability, and delivering human nutrition. Research is also needed to develop these minor commodities' roles in climate mitigation inclusive of improved total factor productivity and overall yield, which can lead to potential beneficial reductions in their required land area (when combined with deliberate, conservation-oriented land use policy and planning) (Lobell et al., 2013). As seen in Figure 1A, an exponential increase in production for major commodities aligns with high levels of investment in R&D, thus suggesting that minor commodities could undergo a similar transformation if invested in more heavily. Furthermore, public research spending should also expand beyond crop improvements alone to investigate the potential for these crops to serve various ecosystem services and nutritional benefits—e.g., enhanced nutrient cycling, soil health, biodiversity, and increased micronutrient content—as part of more diverse cropping systems (DeLonge et al., 2016; Foyer et al., 2016; Tamburini et al., 2020).
Achieving these multiple, convergent research aims falls within the purview of the National Institute of Food and Agriculture's Agriculture and Food Research Initiative (AFRI). For example, the AFRI Sustainable Agricultural Systems (SAS) program “promote[s] the sustainable supply of abundant, affordable, safe, nutritious, and accessible food, while enhancing and improving the long-term health and well-being of all Americans” (NIFA, 2021). In its third year, this program has seen its budget rise from an initial $99 million in 2019 to ~$150 million in 2021. However, only one out of the 17 research projects funded during the program's first 2 years focused on minor commodity crops (e.g., Kernza grain) or approaches for improved human nutrient consumption (NIFA, 2019, 2020). Research funding, such as in SAS and other AFRI programs, should prioritize projects that serve both human and planetary health (Ingram, 2011; Carlisle and Miles, 2013; Bilali et al., 2019; Rosenzweig et al., 2020). This should be done alongside a consistent annual increase in funding from Congress for both this program and NIFA's Sustainable Agriculture Research and Education (SARE) program, the only USDA-competitive grants program that focuses exclusively on sustainable agriculture (Lehner and Rosenberg, 2018).
Furthermore, US R&D programs would benefit from knowledge exchanges with international research institutions. This could take the form of programmatically-funded field work and modeling on minor commodities performed in partnership between the USDA, the US Agency for International Development and institutions such as the Consultative Group's ICRISAT, ICARDA, and the International Center for Tropical Agriculture; the African Center for Crop Improvement; and other agricultural universities and national breeding organizations (e.g., Indian Institute of Pulses Research). Recommendations advanced by the 2016 International Year of Pulses (Calles et al., 2019) provide a model for the kinds of exchanges that would help to kickstart R&D on minor commodities and sustainable cropping systems for planetary health. These include: formal and structured consultation with international producers, trade organizations, and decisionmakers; the development of awareness-raising campaigns; and the creation of publicly-accessible databases.
Beyond research, there exists a mismatch between the intended goals of the Farm Bill conservation programs and the USDA's operationalization of these programs. For example, while federal crop insurance premiums are technically coupled to conservation compliance as of the 2014 Farm Bill, improvements are needed to ensure positive outcomes, including avoiding planting in environmentally sensitive areas (Ristino and Steier, 2019; NIFA, 2021). Further, incorporating soil data into the Federal Crop Insurance Program could strengthen the program and improve outcomes (Woodard, 2016). Crop insurance premiums have also largely benefitted producers of major commodity crops, the production of which may incur environmental damages that the same Farm Bill's conservation provisions aim to mitigate (Ristino and Steier, 2019).
Similarly, payments made by key federal agricultural conservation programs are also subject to mis-alignment between conservation goals and actual implementation. A review of disbursed funds by the National Resources Conservation Service's Environmental Quality Incentives Program (EQIP) from 2009 to 2018 found that only 2–27% of EQIP funding has been used to support practices conveying the highest levels of environmental benefits, such as diversified farming systems (NSAC, 2015) that move beyond the prime focus of major commodity crops. In 2015, for example, over $88M in obligated EQIP payments was used to support activities that may not be additively contributing to enhanced conservation goals as much as remediating environmental pollution (Basche et al., 2020), such as waste storage, animal mortality, and manure management in concentrated feedlot operations (NSAC, 2015). The USDA must provide additional incentives to expand the use and improvement of diverse minor commodity crops, as well as improve the execution, monitoring, and enforcement (where applicable) of existing conservation programs to achieve combined human and environmental goals.
Increasing Collaboration Among the Agricultural, Nutrition, and Environmental Sectors
Research and decision-making on food and nutrition security, agriculture, and the environment should not be conducted in isolation from each other (Reinhardt, 2021). Until recently, the discourse on food system sustainability and agriculture-related policies was largely siloed from discussions centered around food and nutrition security (Bilali et al., 2019). However, research at the intersection of human and environmental health will be integral for increasingly convergent agriculture and environmental policies. For example, a recent executive order mandates “. federal programs to encourage adoption of climate-smart agricultural practices that produce verifiable carbon reductions and sequestrations and create new sources of income and jobs for rural Americans” which demonstrates convergent thinking around agriculture and the environment (Exec Order No. 14008. 86 FR 7619, 2021). Missing from this Order is that any resulting programs should also benefit public health and food security. This broader approach could enable increased development and integration of minor commodities into whole farming-system approaches.
An example entry point to link public health to agricultural and environmental outcomes are initiatives like the Interagency Committee on Human Nutrition Research (ICHNR), charged with improving coordination across federal agencies engaged in funding and conducting nutrition research over 2016–2021 (Fleischhacker et al., 2017). Among their research priorities were the “[development of monitoring systems and data systems]. to evaluate change in nutritional and health status, as well as in the food supply, composition, and consumption” and “. interdisciplinary research [to] identify effective approaches to enhance the environmental sustainability of healthy eating patterns” (Fleischhacker et al., 2017). To the extent that this committee can facilitate inter-agency coordination, their work should continue into the foreseeable future and also consider how current federal agricultural spending, research and otherwise, may be redistributed to enhance these lines of research and build new emphases on crops and cropping systems for planetary health.
Ultimately, public agricultural R&D will continue to play a critical role in the trajectory of our food systems. There is large potential for the USDA to implement principles of planetary health—intersecting human and environmental security—by increasing funding support to the integration and improvement of minor commodity legumes and coarse grains into a more diverse agricultural landscape. A global food transformation can start through changes in priorities of currently funded programs.
Data Availability Statement
The original contributions presented in the study are included in the article/supplementary material, further inquiries can be directed to the corresponding author/s.
Author Contributions
AB and SM conceived the manuscript ideas and concept, undertook data analyses, and wrote the manuscript. MH and MS reviewed the manuscript and contributed edits, comments, and ideas. DM undertook data analyses. MD contributed to manuscript conceptualization and ideas and manuscript writing/editing. All authors contributed to the article and approved the submitted version.
Funding
This work was supported by Center for Environment and Animal Protection, New York University.
Conflict of Interest
The authors declare that the research was conducted in the absence of any commercial or financial relationships that could be construed as a potential conflict of interest.
Publisher's Note
All claims expressed in this article are solely those of the authors and do not necessarily represent those of their affiliated organizations, or those of the publisher, the editors and the reviewers. Any product that may be evaluated in this article, or claim that may be made by its manufacturer, is not guaranteed or endorsed by the publisher.
Acknowledgments
We would like to thank Peter Lehner and Carrie Apfel from Earthjustice for their insightful comments and contributions to this piece. Additionally, we would like to thank the Center for Environmental and Animal Protection (CEAP) for providing financial support for this project.
References
Alston, J. M., Andersen, M., James, J., and Pardey, P. G. (2011). The economic returns to U.S. public agricultural research. Am. J. Agric. Econ. 93, 1257–1277. doi: 10.1093/ajae/aar044
Alston, J. M., Beddow, J. M., and Pardey, P. G. (2009). Agricultural research, productivity, and food prices in the long run. Science 325, 1209–1210. doi: 10.1126/science.1170451
Alston, J. M., Marra, M. C., Pardey, P. G., and Wyatt, T. J. (2000). A meta analysis of rates of return to agricultural RandD: ex pede herculem? Res. Rep. Washington, DC: International Food Policy Research Institute (IFPRI), 113:150.
Andersen, M. A., and Song, W. (2013). The economic impact of public agricultural research and development in the united states. Agric. Econ. 44, 287–295. doi: 10.1111/agec.12011
Baldos, U., and Blaustein-Reito, D. (2021). Investing in Public RandD for a Competitive and Sustainable US Agriculture. The Breakthrough Institute.
Baldos, U. L. C., Viens, F. G., Hertel, T. W., and Fuglie, K. O. (2018). RandD spending, knowledge capital, and agricultural productivity growth: a bayesian approach. Am. J. Agric. Econ. 101, 291–310. doi: 10.1093/ajae/aay039
Basche, A., Tully, K., Álvarez-Berríos, N. L., Reyes, J., Lengnick, L., Brown, T., et al. (2020). Evaluating the untapped potential of U.S. conservation investments to improve soil and environmental health. Front. Sustain. Food Syst. 4:547876. doi: 10.3389/fsufs.2020.547876
Bilali, H., Callenius, C., Strassner, C., and Probst, L. (2019). Food and nutrition security and sustainability transitions in food systems. Food Energy Secur. 8:e00154. doi: 10.1002/fes3.154
Biovision Foundation for Ecological Development and IPES-Food (2020). Money Flows: What Is Holding Back Investment in Agroecological Research for Africa? Biovision Foundation for Ecological Development and International Panel of Experts on Sustainable Food Systems.
Cagauan, A. G. (1995). “The impact of pesticides on ricefield vertebrates with emphasis on fish,” in Impact of Pesticides on Farmer Health and the Rice Environment, eds P. L. Pingali and P. A. Roger (Dordrecht: Springer), 203–248. doi: 10.1007/978-94-011-0647-4_8
Calles, T., Xipsiti, M., and del Castello, R. (2019). Legacy of the international year of pulses. Environ. Earth Sci. 78:124. doi: 10.1007/s12665-019-8106-6
Campbell, B. M., Beare, D. J., Bennett, E. M., Hall-Spencer, J. M., Ingram, J. S. I., Jaramillo, F., et al. (2017). Agriculture production as a major driver of the earth system exceeding planetary boundaries. Ecol. Soc. 22:8. doi: 10.5751/ES-09595-220408
Carlisle, L., and Miles, A. (2013). Closing the knowledge gap: how the USDA could tap the potential of biologically diversified farming systems. J. Agric. Food Syst. Commun. Dev. 4, 219–225. doi: 10.5304/jafscd.2013.034.025
Cassidy, E. S., West, P. C., Gerber, J. S., and Foley, J. A. (2013). Redefining agricultural yields: from tonnes to people nourished per hectare. Environ. Res. Lett. 8:034015. doi: 10.1088/1748-9326/8/3/034015
Clancy, M., Fuglie, K., and Heisey, P. (2016). US agricultural RandD in an era of falling public funding. Amber Waves, 10. doi: 10.22004/ag.econ.249840
Clark, M. A., Domingo, N. G., Colgan, K., Thakrar, S. K., Tilman, D., Lynch, J., et al. (2020). Global food system emissions could preclude achieving the 1.5° and 2° C climate change targets. Science 370, 705–708. doi: 10.1126/science.aba7357
Clark, M. A., Springmann, M., Hill, J., and Tilman, D. (2019). Multiple health and environmental impacts of foods. Proc. Nat.l Acad. Sci. 116, 23357–23362. doi: 10.1073/pnas.1906908116
Clune, S., Crossin, E., and Verghese, K. (2017). Systematic review of greenhouse gas emissions for different fresh food categories. J. Clean. Prod. 140, 766–783. doi: 10.1016/j.jclepro.2016.04.082
Cullather, N. (2013). The Hungry World: America's Cold War Battle Against Poverty in Asia. Cambridge, MA: Harvard University Press.
Cullis, C., and Kunert, K. J. (2017). Unlocking the potential of orphan legumes. J. Exp. Bot. 68, 1895–1903. doi: 10.1093/jxb/erw437
Davis, K. F., Chhatre, A., Rao, N. D., Singh, D., Ghosh-Jerath, S., Mridul, A., et al. (2019). Assessing the sustainability of post-Green Revolution cereals in India. Proc. Nat. Acad. Sci. 116, 25034–25041. doi: 10.1073/pnas.1910935116
Davis, K. F., Chiarelli, D. D., Rulli, M. C., Chhatre, A., Richter, B., Singh, D., et al. (2018). Alternative cereals can improve water use and nutrient supply in India. Sci. Adv. 4:eaao1108. doi: 10.1126/sciadv.aao1108
DeFries, R., Fanzo, J., Remans, R., Palm, C., Wood, S., and Anderman, T. L. (2015). Metrics for land-scarce agriculture. Science 349, 238–240. doi: 10.1126/science.aaa5766
DeLonge, M. S., Miles, A., and Carlisle, L. (2016). Investing in the transition to sustainable agriculture. Environ. Sci. Policy 55, 266–273. doi: 10.1016/j.envsci.2015.09.013
Eiselen, H., and Webb, P. J. R. (2009). For a World Without Hunger. Stuttgart: Hampp Media/Balance Publications, Fiat Panis, 410–434.
Eliazer-Nelson, A. R. L., Ravichandran, K., and Antony, U. (2019). The impact of the Green Revolution on indigenous crops of India. J. Ethn. Food 6:8. doi: 10.1186/s42779-019-0011-9
Evenson, R. E. (2002). “Private and public research and extension,” in Handjournal of Agricultural Economics, eds B. L. Gardner and G. C. Rausser (New York, NY: Elsevier).
Evenson, R. E., and Gollin, D. (2003). Assessing the impact of the green revolution, 1960 to 2000. Science 300, 758–762. doi: 10.1126/science.1078710
FAO (2019). The State of the World's Biodiversity for Food and Agriculture. FAOCommission on Genetic Resources for Food and Agriculture Assessments. Available online at: http://www.fao.org/3/CA3129EN/CA3129EN.pdf.
FAO UNDP and UNEP. (2021). A Multi-Billion-Dollar Opportunity – Repurposing Agricultural Support to Transform Food Systems. Rome: FAO.
Farooq, M., Gogoi, N., Barthakur, S., Baroowa, B., Bharadwaj, N., Alghamdi, S. S., et al. (2017). Drought stress in grain legumes during reproduction and grain filling. J. Agron. Crop Sci. 203, 81–102. doi: 10.1111/jac.12169
Fleischhacker, S. E., Ballard, R. M., Starke-Reed, P. E., Galuska, D. A., and Neuhouser, M. L. (2017). Developmental process and early phases of implementation for the US interagency committee on human nutrition research national nutrition research roadmap 2016-2021. J. Nutr. 147, 1833–1838. doi: 10.3945/jn.117.255943
Foyer, C. H., Lam, H. M., Nguyen, H. T., Siddique, K. H. M., Varshney, R. K., Colmer, T. D., et al. (2016). Neglecting legumes has compromised human health and sustainable food production. Nat. Plants 2, 1–10. doi: 10.1038/nplants.2016.112
Gordon, L. J., Bignet, V., Crona, B., Henriksson, P. J. G., Van Holt, T., Jonell, M., et al. (2017). Rewiring food systems to enhance human health and biosphere stewardship. Environ. Res. Lett. 12:100201. doi: 10.1088/1748-9326/aa81dc
Hellin, J., Bellon, M. R., and Hearne, S. J. (2014). Maize landraces and adaptation to climate change in mexico. J. Crop Improve. 28, 484–501. doi: 10.1080/15427528.2014.921800
Henry, R. C., Alexander, P., Rabin, S., Anthoni, P., Rounsevell, M. D., and Arneth, A. (2019). The role of global dietary transitions for safeguarding biodiversity. Glob. Environ. Change 58:101956. doi: 10.1016/j.gloenvcha.2019.101956
Hu, F. B. (2011). Globalization of diabetes: the role of diet, lifestyle, and genes. Diabetes Care 34, 1249–1257. doi: 10.2337/dc11-0442
Ingram, J. (2011). A food systems approach to researching food security and its interactions with global environmental change. Food Secur. 3, 417–431. doi: 10.1007/s12571-011-0149-9
International Food Policy Research Institute (2020). 2020 Global Food Policy Report: Building Inclusive Food Systems. Washington, DC: International Food Policy Research Institute.
IPCC (2019). Climate Change and Land: An IPCC Special Report on Climate Change, Desertification, Land Degradation, Sustainable Land Management, Food Security, and Greenhouse Gas Fluxes In Terrestrial Ecosystems, eds P. R. Shukla, J. Skea, E. Calvo Buendia, V. Masson-Delmotte, H.-O. Pörtner, D. C. Roberts, et al.
Joshi, P. K., and Rao, P. P. (2017). Global pulses scenario: status and outlook. Ann. N. Y. Acad. Sci. 1392, 6–17. doi: 10.1111/nyas.13298
Kearney, J. (2010). Food consumption trends and drivers. Philos. Trans. R. Soc. Lond. B. Biol. Sci. 365, 2793–2807. doi: 10.1098/rstb.2010.0149
Khoury, C. K., Bjorkman, A. D., Dempewolf, H., Ramirez-Villegas, J., Guarino, L., Jarvis, A., et al. (2014). Increasing homogeneity in global food supplies and the implications for food security. Proc. Nat. Acad. Sci. 111, 4001–4006. doi: 10.1073/pnas.1313490111
Lal, R. (2010). Enhancing eco-efficiency in agro-ecosystems through soil carbon sequestration. Crop Sci. 50, S-120-S-131. doi: 10.2135/cropsci2010.01.0012
Lehner, P., and Rosenberg, N. (2018). Promoting climate-friendly agriculture for the benefit of farmers, rural communities, and the environment. Nat. Resour. Environ. 33:2018.
Lobell, D. B., Hammer, G. L., McLean, G., Messina, C., Roberts, M. J., and Schlenker, W. (2013). The critical role of extreme heat for maize production in the United States. Nat. Clim. Chang. 3, 497–501. doi: 10.1038/nclimate1832
Longvah, T., Ananthan, R., Bhaskar, K., and Venkaiah, K. (2017). Indian Food Composition Tables. National Institute of Nutrition, Indian Council of Medical Research.
Mammadov, J., Buyyarapu, R., Guttikonda, S., Parliament, K., Abdurakhmonov, I. Y., and Kumpatla, S. P. (2018). Wild relatives of maize, rice, cotton, and soybean: treasure troves for tolerance to biotic and abiotic stresses. Front. Plant Sci. 9:886. doi: 10.3389/fpls.2018.00886
Meng, F., Wei, Y., and Yang, X. (2005). Iron content and bioavailability in rice. J. Trace Elements Med. Biol. 18, 333–338. doi: 10.1016/j.jtemb.2005.02.008
Miles, A., DeLonge, M. S., and Carlisle, L. (2017). Triggering a positive research and policy feedback cycle to support a transition to agroecology and sustainable food systems. Agroecol. Sustain. Food Syst. 41, 855–879. doi: 10.1080/21683565.2017.1331179
Minasny, B., Malone, B. P., McBratney, A. B., Angers, D. A., Arrouays, D., Chambers, A., et al. (2017). Soil carbon 4 per mille. Geoderma 292, 59–86. doi: 10.1016/j.geoderma.2017.01.002
NIFA (2019). New USDA Scientific Research Program Promotes Sustainable Agricultural Practices. Available online at: https://nifa.usda.gov/press-release/nifa-invests-778-million-research-sustaining-food
NIFA (2020). USDA Scientific Research Program Funds Sustainable Agricultural Systems Projects. Available online at: https://nifa.usda.gov/press-release/nifa-sustainable-ag-systems-projects
NIFA (2021). AFRI Sustainable Agricultural Systems. Available online at: https://nifa.usda.gov/program/afri-sas
NSAC (2015). Cafos and Cover Crops: A Closer Look at 2015 Eqip Dollars. Available online at: https://sustainableagriculture.net/blog/fy15-general-eqip-update/
Ortiz-Bobea, A., Ault, T. R., Carrillo, C. M., Chambers, R. G., and Lobell, D. B. (2021). Anthropogenic climate change has slowed global agricultural productivity growth. Nat. Clim. Chang. 11, 306–312. doi: 10.1038/s41558-021-01000-1
Ortiz-Monasterio, J. I., Palacios-Rojas, N., Meng, E., Pixley, K., Trethowan, R., and Peña, R. J. (2007). Enhancing the mineral and vitamin content of wheat and maize through plant breeding. J. Cereal Sci. 46, 293–307. doi: 10.1016/j.jcs.2007.06.005
Palm, C. A., Gachengo, C. N., Delve, R. J., Cadisch, G., and Giller, K. E. (2001). Organic inputs for soil fertility management in tropical agroecosystems: application of an organic resource database. Agric. Ecosyst. Environ. 83, 27–42. doi: 10.1016/S0167-8809(00)00267-X
Pardey, P. G., Alston, J. M., and Chan-Kang, C. (2012). “Agricultural production, productivity and RandD over the past half century: an emerging new world order,” in 2012 Conference, August 18-24. 2012 (Foz do Iguacu, International Association of Agricultural Economists).
Pardey, P. G., Beintema, N., Dehmer, S., and Wood, S. (2006). Agricultural Research: A Growing Global Divide? International Food Policy Research Institute.
Pardey, P. G., Chan-Kang, C., Dehmer, S., Beddow, J. M., Hurley, T. M., Rao, X., et al. (2014). Investments in and the economic returns to agricultural and food RandD worldwide. Encycl. Agric. Food Syst. 78–97. doi: 10.1016/B978-0-444-52512-3.00045-0
Pimbert, M., and Moeller, N. (2018). Absent agroecology aid: on UK agricultural development assistance since 2010. Sustainability 10:505. doi: 10.3390/su10020505
Pingali, P. L. (2012). Green revolution: impacts, limits, and the path ahead. Proc. Nat. Acad. Sci. 109, 12302–12308. doi: 10.1073/pnas.0912953109
Piotrowska-Dlugosz, A., and Wilczewski, E. (2012). Effect of catch crops cultivated for green manure and mineral nitrogen fertilization on soil enzyme activities and chemical properties. Geoderma 189, 72–80. doi: 10.1016/j.geoderma.2012.04.018
Popkin, B. M., Adair, L. S., and Ng, S. W. (2012). Global nutrition transition and the pandemic of obesity in developing countries. Nutr. Rev. 70, 3–21. doi: 10.1111/j.1753-4887.2011.00456.x
Ramakrishna, A., Gowda, C. L. L., and Johansen, C. (2000). “Management factors affecting legumes production in the indo-gangetic plains,” in Legumes in Rice and Wheat Cropping Systems of the Indo-Gangetic Plain: Constraints and Opportunities, eds C. Johansen, J. M. Duxbury, S. M. Virmani, C. L. L. Gowda, S. Pande, and P. K. Joshi (Patancheru; New York, NY:ICRISAT; Cornell University), 156–165.
Rathna Priya, T., Eliazer Nelson, A. R. L., and Ravichandran, K. (2019). Nutritional and functional properties of coloured rice varieties of South India: a review. J. Ethn. Food 6:11. doi: 10.1186/s42779-019-0017-3
Reinhardt, S. (2021). From Silos to Systems: Investing in Sustainable Nutrition Science for a Healthy Future. Cambridge, MA: Union of Concerned Scientists.
Remans, R., Wood, S. A., Saha, N., Anderman, T. L., and DeFries, R. S. (2014). Measuring nutritional diversity of national food supplies. Glob. Food Secur. 3, 174–182. doi: 10.1016/j.gfs.2014.07.001
Ristino, L., and Steier, G. (2019). Losing ground: a clarion call for farm bill reform to ensure a food secure future. Columbia J. Environ. Law. 42. doi: 10.7916/cjel.v42i1.3732
Rosenzweig, C., Mbow, C., Barioni, L. G., Benton, T. G., Herrero, M., Krishnapillai, M., et al. (2020). Climate change responses benefit from a global food system approach. Nat. Food 1, 94–97. doi: 10.1038/s43016-020-0031-z
Rubiales, D., Fondevilla, S., Chen, W., Gentzbittel, L., Higgins, T. J., Castillejo, M. A., et al. (2015). Achievements and challenges in legume breeding for pest and disease resistance. CRC. Crit. Rev. Plant Sci. 34, 195–236. doi: 10.1080/07352689.2014.898445
Siddique, K. H. M., Johansen, C., Turner, N. C., Jeuffroy, M. H., Hashem, A., Sakar, D., et al. (2012). Innovations in agronomy for food legumes. A review. Agron. Sustain. Dev. 32, 45–64. doi: 10.1007/s13593-011-0021-5
Stagnari, F., Maggio, A., Galieni, A., and Pisante, M. (2017). Multiple benefits of legumes for agriculture sustainability: an overview. Chem. Biol. Technol. Agric. 4:2. doi: 10.1186/s40538-016-0085-1
Steensland, A. (2019). 2019 Global Agricultural Productivity Report: Productivity Growth for Sustainable Diets and More. Virginia Tech College of Agriculture and Life Sciences.
Stevenson, J. R., Villoria, N., Byerlee, D., Kelley, T., and Maredia, M. (2013). Green revolution research saved an estimated 18 to 27 million hectares from being brought into agricultural production. Proc. Nat. Acad. Sci. 110, 8363–8368. doi: 10.1073/pnas.1208065110
Tadele, Z. (2019). Orphan crops: their importance and the urgency of improvement. Planta 250, 677–694. doi: 10.1007/s00425-019-03210-6
Tamburini, G., Bommarco, R., Wanger, T. C., Kremen, C., van der Heijden, M. G., Liebman, M., et al. (2020). Agricultural diversification promotes multiple ecosystem services without compromising yield. Sci. Adv. 6:eaba1715. doi: 10.1126/sciadv.aba1715
Tilman, D., and Clark, M. (2014). Global diets link environmental sustainability and human health. Nature 515, 518–522. doi: 10.1038/nature13959
Tilman, D., Clark, M., Williams, D. R., Kimmel, K., Polasky, S., and Packer, C. (2017). Future threats to biodiversity and pathways to their prevention. Nature 546, 73–81. doi: 10.1038/nature22900
Tubiello, F. N., m Conchedda, G., Wanner, N., Federici, S., Rossi, S., and Grassi, G. (2021). Carbon emissions and removals from forests: new estimates. 1990-2020. Earth Syst. Sci. Data 13, 1681–1691. doi: 10.5194/essd-13-1681-2021
Weis, A. J. (2007). The Global Food Economy: The Battle for the Future of Farming. New York, NY; London: Zed Journals.
Welch, R. M., and Graham, R. D. (2000). A new paradigm for world agriculture: productive, sustainable, nutritious, healthful food systems. Food Nutr. Bull. 21, 361–366. doi: 10.1177/156482650002100404
Willett, W., Rockström, J., Loken, B., Springmann, M., Lang, T., Vermeulen, S., et al. (2019). Food in the anthropocene: the EAT–Lancet commission on healthy diets from sustainable food systems. Lancet Comm. 393, 447–492. doi: 10.1016/S0140-6736(18)31788-4
Woodard, J. (2016). Integrating high resolution soil data into federal crop insurance policy: implications for policy and conservation. Environ. Sci. Policy 66, 93–100. doi: 10.1016/j.envsci.2016.08.011
Xie, Z., Tu, S., Shah, F., Xu, C., Chen, J., Han, D., et al. (2016). Substitution of fertilizer-N by green manure improves the sustainability of yield in double-rice cropping system in south China. Field Crops Res. 188, 142–149. doi: 10.1016/j.fcr.2016.01.006
Keywords: investment, research, orphan crops, commodities, legumes, coarse grains, USDA
Citation: Bollington A, DeLonge M, Mungra D, Hayek M, Saifuddin M and McDermid SS (2021) Closing Research Investment Gaps for a Global Food Transformation. Front. Sustain. Food Syst. 5:794594. doi: 10.3389/fsufs.2021.794594
Received: 13 October 2021; Accepted: 02 November 2021;
Published: 30 November 2021.
Edited by:
Kurt A. Rosentrater, Iowa State University, United StatesReviewed by:
Shankar Lal Jat, Indian Council of Agricultural Research (ICAR), IndiaChristophe David, Institut Supérieur d'Agriculture Rhône-Alpes, France
Copyright © 2021 Bollington, DeLonge, Mungra, Hayek, Saifuddin and McDermid. This is an open-access article distributed under the terms of the Creative Commons Attribution License (CC BY). The use, distribution or reproduction in other forums is permitted, provided the original author(s) and the copyright owner(s) are credited and that the original publication in this journal is cited, in accordance with accepted academic practice. No use, distribution or reproduction is permitted which does not comply with these terms.
*Correspondence: Sonali Shukla McDermid, c3BzMjQ2QG55dS5lZHU=