- Honey Bee Research and Extension Laboratory, Entomology and Nematology Department, University of Florida, Gainesville, FL, United States
Western honey bees (Apis mellifera L.) collect pollen from flowers as their source of protein, fat, vitamins, and minerals. Beekeepers feed pollen substitutes to their honey bee colonies to mitigate a lack of natural pollen resources in the environment. Despite their widespread use, it is unclear if pollen substitutes are beneficial to colony health and productivity. Herein, we review the literature regarding pollen substitute efficacy in four major categories: (1) consumption/palatability of pollen substitutes, (2) colony productivity, (3) pest and disease response, and (4) physiological response. Collectively, the literature shows a mix of positive, neutral, and negative impacts of pollen substitutes on honey bee colony health. Additionally, we recommend areas for improvement in pollen substitute research. We hope this review will lead to more research on pollen substitutes given nutrition is a key factor impacting the health of managed honey bees globally.
Introduction
Western honey bees (Apis mellifera L.; hereafter “honey bee”) require nectar and pollen from flowers for a complete diet. They convert nectar into honey, which provides carbohydrates for energy (Brodschneider and Crailsheim, 2010). They convert pollen to bee bread which provides the protein, fat, vitamins, and minerals that immature bees need to grow and develop (Brodschneider and Crailsheim, 2010). Pollen needs to be available both in adequate quantity and quality to enhance honey bee colony health (Brodschneider and Crailsheim, 2010). For example, quality pollen improves the ability of bees to combat stressors such as pests and pathogens (Rinderer et al., 1974; Alaux et al., 2010; Di Pasquale et al., 2013; Dolezal and Toth, 2018; Dolezal et al., 2019) and pesticides (Schmehl et al., 2014).
It can be difficult for beekeepers to locate good pollen resources for their colonies. There are a few key reasons for this. First, land-use change is a major driver of lack of pollen forage for bees (Otto et al., 2016). Increases in commodity crop cultivation, notably corn and soybean, have been linked to nutritional stress in managed honey bees because these crops are poor sources of pollen and nectar (Dolezal et al., 2016; Smart et al., 2019). Second, beekeepers often place commercial colonies on monocultures to provide pollination services for the target crop (Smart et al., 2019; Topitzhofer et al., 2019). However, these crops often do not provide a high-quality pollen and may impact pollen diversity in the area overall if the crop is grown across many hectares (Decourtye et al., 2010; Topitzhofer et al., 2019). Additionally, beekeepers regularly manage colonies in the face of seasonal pollen dearth, when pollen is not available to bees in adequate quantity or quality. Colonies also may experience adverse weather conditions, like extended periods of cold or rain, that may prevent their bees from foraging for the pollen that they need (Mattila and Otis, 2006a). Consequently, beekeepers must find solutions that can be used to address pollen deficiencies in their colonies.
Beekeepers often use artificial diets to replace the protein, fat, vitamins, and minerals lost when natural pollen is not available or of sufficient quality (Somerville, 2005; Mortensen et al., 2019). These diets are called pollen substitutes when they contain no natural pollen, or pollen supplements when they contain some natural pollen (Standifer et al., 1977; Saffari et al., 2010a). Herein, we use the term pollen substitute because the majority of the diets used by beekeepers do not contain natural pollen, given pollen is expensive and can spread disease (Saffari et al., 2010a). Beekeepers can purchase commercially available diets or formulate their own (Mortensen et al., 2019). Pollen substitutes do not truly replace natural pollen because they lack many of the nutrients that natural pollen provides (Manning, 2018; Wright et al., 2018).
The use of pollen substitutes began in the early 1900s in the U.S. and Canada (Manning, 2018). Mykola H. Haydak and Elton W. Herbert Jr. were pioneers of honey bee nutrition and pollen substitute research, dedicating their careers to it (Manning, 2018). In many of their early studies, they used various soy flours and brewer's yeast as bases for diets. These remain a common, cheap, and accessible source of protein in pollen substitutes today. Other common ingredients of pollen substitutes include pea protein, potato protein, corn gluten, egg products, milk products, and blood meal, among many other ingredients (Brodschneider and Crailsheim, 2010; Saffari et al., 2010a; Mortensen et al., 2019). Early pollen substitute research set the stage for modern feeding practices.
Today, beekeepers use a multitude of different diets and feeding practices. Beekeepers typically place pollen substitutes directly above the brood nest in patty form (Standifer et al., 1977; Somerville, 2005; Figure 1). They may also place them outside of the hives in feeders in dry powder form (Standifer et al., 1977; Somerville, 2005; Saffari et al., 2010b). Less commonly, beekeepers may feed pollen substitutes in liquid form in feeders connected to the hive body (DeGrandi-Hoffman et al., 2008). Nearly all published research thus far has been dedicated to the use of pollen substitute or supplement patties, with the exception of those mentioned for dry (Saffari et al., 2010b) and liquid (DeGrandi-Hoffman et al., 2008) substitutes.
Modern beekeeping practices require that pollen substitutes be economical, have a long shelf life, and be easy to feed to colonies (Saffari et al., 2010a). At the same time, a good pollen substitute should be palatable to the bees and have positive impacts on their health. The most economical solution is not always the best for bee health. As an example, natural pollen is difficult to source cheaply in large quantities and carries the risk of pathogen transmission, even if it would be ideal for pollen substitutes to include it (Sihag and Gupta, 2011; Manning, 2018). Unfortunately, no substitute diet has been formulated that can replace the nutrition provided by pollen completely (Manning, 2018; Wright et al., 2018). Beekeepers spend a lot of time and money managing for pollen deficiencies in their colonies. Despite this, there is no robust body of research/literature that universally supports their use.
The purpose of this review is to synthesize the previous research conducted on pollen substitute diets and their impacts on honey bee colony health, evaluate methods used to test pollen substitutes, recommend areas for improvement in pollen substitute research and suggest future research needs. This review is comprised of four categories of honey bee health parameters that pollen substitute research typically addresses: (1) consumption/palatability of pollen substitutes, (2) colony productivity, (3) pest and pathogen response, and (4) physiological response. Following that, we provide a critique of methods used for pollen substitute research and make suggestions for improvement and future study. We include summary tables of all the substitute diets tested to date, their health impacts on colonies, and corresponding references as Supplementary Tables 1–3. By developing this review, we hope to improve the direction, quantity and quality of pollen substitute research, with a goal of helping beekeepers make better management decisions for colony nutrition.
Consumption/Palatability of Pollen Substitutes
Until recently (Noordyke et al., 2021), no one had shown that honey bees even ingest pollen substitute patties. It was only inferred that they do because the substitute patties disappear from hives and a number of measurable effects from substitute patties have been documented in the literature (Supplementary Tables 1–3). Now, we can more confidently review the literature on pollen substitute consumption/palatability knowing that honey bees are consuming, and not ejecting, at least some pollen substitute patties when they disappear from the hive (Noordyke et al., 2021).
The attractiveness of a diet is usually determined by measuring the consumption rate, typically defined as the change in diet mass over time (Herbert and Shimanuki, 1978; Alqarni, 2006; DeGrandi-Hoffman et al., 2008; Pankiw et al., 2008; Ellis and Hayes, 2009; Saffari et al., 2010a; Li et al., 2012, 2014; Sagili and Breece, 2012; Morais et al., 2013; Amro et al., 2016; Almeida-Dias et al., 2018; Manning, 2018; Lamontagne-Drolet et al., 2019). If a diet disappears more quickly than does another, it seems natural to conclude that bees prefer it to the one that disappeared at a slower rate (Ellis and Hayes, 2009; Almeida-Dias et al., 2018;). However, the loss of diet mass is not necessarily consumption as diets can lose weight to evaporation or simply due to bee removal from a hive. It may also seem that bee “preference” for a diet (again, determined by the loss of diet mass) is a possible sign of superior nutritional value of a diet, under the assumption that bees elect to consume a diet that is most valuable to them nutritionally. However, this is also not a safe assumption, neither has it been shown to be true.
Many reasons could be driving honey bees to consume more or less of a particular diet than another. Generally, honey bees consume more bee bread or natural pollen than they consume pollen substitutes (Herbert et al., 1980a; Pernal and Currie, 2000; Li et al., 2012; Ricigliano and Simone-Finstrom, 2020). Thus, the availability of incoming pollen or stored bee bread may affect honey bee use of any substitutes provided by the beekeeper. Both variables, which we discuss later in the manuscript, could be measured prior to and during a study to confirm their possible impact on consumption.
It is, of course, logical that honey bees would prefer to consume more of their natural diet than a substitute. However, some investigators found that bees consumed as much or more substitute than natural diets provided to them (Alqarni, 2006; DeGrandi-Hoffman et al., 2008; Saffari et al., 2010a). There are a few possible reasons a “preference” for the substitute diets was found. First, the bees may have determined the substitute diets to be of high quality, hence preferring to feed on these diets. In contrast, it is also possible that the bees determined the natural diets to be of inferior quality, thus causing the bees to prefer the substitutes (DeGrandi-Hoffman et al., 2008). Third, bees may have preferred the substitute diet simply because it contained more sugar than did the natural diet. Many commercially available pollen substitutes are reconstituted with some sort of sugar syrup in an attempt to make it palatable to the bees (Somerville, 2005). This certainly leads to a skewed preference. Finally, it is possible that the bees needed to consume more pollen substitute than natural pollen to fulfill their nutritional needs, giving the appearance of “preference” for the substitute diet when it is just a need to consume more to receive the same level of nutrition as they would if they consumed bee bread. Some insects are known to overeat to compensate for insufficient nutrients (Simpson et al., 1995). For example, Haydak (1949) found that honey bees ingested more total nitrogen than usual when pollen substitutes were deficient in certain vitamins. Similarly, Li et al. (2014) found that honey bees consumed significantly more pollen substitute with 15 and 25% crude protein than pollen substitute with 35% crude protein, possibly to compensate for lower protein availability. Regardless, insects are not always demonstrating preference for a diet when they consume more of it, neither does consumption mean that a given diet is qualitatively better than another (Paiva et al., 2019). In fact, diets that are consumed less than others can have better impact on health (Li et al., 2014; Amro et al., 2016; Ricigliano and Simone-Finstrom, 2020), which could indicate superior nutritional value. It is important that a pollen substitute is attractive to the bees; otherwise, it is of little use to them. However, researchers should be cautious to draw conclusions about diet quality based on consumption alone, especially if consumption is based solely on the disappearance of a diet from a hive, which it usually is.
Choice tests conducted outside the hive, with no sugar added to the diet, are another way to evaluate the attractiveness of a diet. A choice test can indicate that honey bees prefer a dry diet if they consume more of it than they do other dry diets offered simultaneously. Saffari et al. (2010b) gave honey bee colonies a choice of three dry pollen substitutes offered in feeders in the apiary and also performed non-choice tests in which each diet was offered alone. They found similar patterns in consumption rate in both tests. By pairing choice and non-choice tests, and allowing bees to forage for dry diets to which no sugar was added, Saffari et al. (2010b) were able to make a stronger case for the attractiveness of a particular pollen substitute diet to the bees.
Notably, some common pollen substitute ingredients are unpalatable to bees. Diets with lactose are unattractive to colonies, possibly because lactose is toxic to bees (Herbert and Shimanuki, 1978). Saffari et al. (2010a) hypothesized that soy-based diets are less palatable than others because bees largely ignored soy-based diets in their study; however, other investigators have found soy-based diets to be attractive to bees (Sihag and Gupta, 2011; Manning, 2018). Ellis and Hayes (2009) found that colonies lost more soy-based pollen substitute patties than natural pollen patties through screened bottom boards as debris, suggesting that bees may have removed the soy-based diets as debris. Many diets are soy-based because soy is a cheap and accessible source of protein; therefore, finding ways to increase the palatability of soy could be of importance to beekeepers.
Researchers have attempted to improve consumption of pollen substitutes experimentally by: (1) adding bee-collected pollen pellets (Alqarni, 2006; Schmidt and Hanna, 2006; Sihag and Gupta, 2011; Watkins de Jong et al., 2019), (2) adding lipid extracts from pollen (Herbert et al., 1980a), (3) adding non-nutritive phagostimulant extracts from pollen (Schmidt and Hanna, 2006), (4) fermenting the diet (Ellis and Hayes, 2009; Almeida-Dias et al., 2018; Paiva et al., 2019), (5) adding synthetic brood pheromone in the brood nest (Pankiw et al., 2008; Sagili and Breece, 2012), (6) offering the diet in a semi-liquid form directly in the comb (Sihag and Gupta, 2011), and (7) increasing the surface area of pollen substitute patties (Avni et al., 2009). Honey bees also prefer fresh pollen to older pollen (Herbert and Shimanuki, 1982; Pernal and Currie, 2000; Carroll et al., 2017), which could be important to consider when adding natural pollen to enhance the consumption of supplemental diets. It could be useful to apply some of these methods to increase palatability of commercial pollen substitutes.
It is important to pair consumption studies in the laboratory with those of full colonies in the field to get a more accurate picture of the palatability/attractiveness of a pollen substitute to bees. Manning (2018) showed that full colonies consumed pollen substitutes heavily, but caged bees in the laboratory did not consume them at similar rates and experienced decreased longevity compared to bees fed control diets in the laboratory. They suggest that there may have been a problem with an ingredient used in their laboratory assay, but it also highlights the potential for discrepancies in the way bees treat diets depending on their environment. For example, it is possible that the lack of brood in cage studies might affect how workers consume protein diets. Ultimately, consumption in controlled laboratory settings alone may not imply bees will accept or consume the diets in the field.
Evaporative controls are important to include in consumption studies because some diets lose mass to water evaporation much faster than do others. Researchers could incorrectly conclude that a diet is being consumed more than another when it is actually losing more mass to evaporation. Evaporative controls are widely lacking in field-based pollen substitute studies, but a few investigators have tested them in laboratory settings (Pernal and Currie, 2000; Schmidt and Hanna, 2006; Williams et al., 2013; Omar et al., 2017; Ricigliano and Simone-Finstrom, 2020). Investigators should use evaporative controls when measuring consumption at the colony level. This can be done by placing the test diets above the brood nest in a screened container (Noordyke et al., 2021). The screen excludes bee access to the diet (thus no consumption) while allowing the diet to experience the internal conditions of the hive. Empty packages used to ship bees work well for this purpose (Figure 2A).
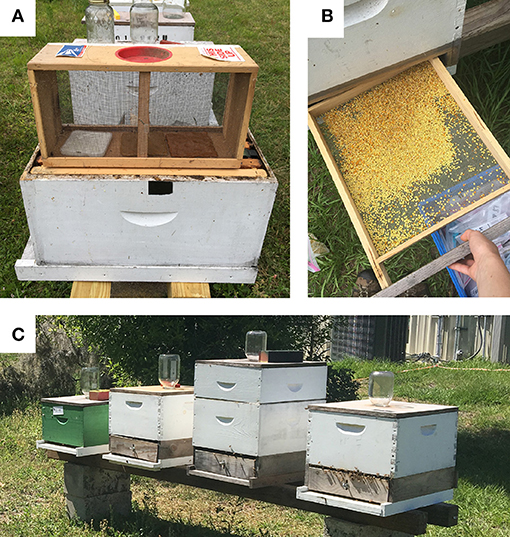
Figure 2. (A) An empty package used to exclude bees from evaporative control diets. (B) A pollen sample from a sentinel colony fitted with a pollen trap. (C) Multiple sentinel colonies fitted with pollen traps used to monitor pollen availability during a pollen substitute study.
Colony Productivity
Beekeepers feed pollen substitutes to their colonies because they believe the diets benefit colony strength and/or productivity in some way. They feed pollen substitutes to colonies with a production goal in mind. In this section, we review research on pollen substitute impacts on colony strength (i.e., adult bee population, brood production) and other measures of productivity (i.e., honey production, queen quality, etc.).
Adult Bee Population
One of the main goals of pollen substitute feeding is to increase the number of adult bees in the hive, usually referred to as colony “strength.” Of nine published studies, six concluded that pollen substitutes increased adult bee populations. Another showed no change in adult populations with feeding. Two others were inconclusive (Supplementary Table 1). Several of these studies showed that adult bee populations increased after feeding colonies with certain pollen substitutes compared to colonies that received no diet (Abbas et al., 1995; DeGrandi-Hoffman et al., 2008; Saffari et al., 2010a; Sihag and Gupta, 2011, 2013; Kumar et al., 2013). Most of these studies were conducted during seasons with adverse weather conditions or extreme pollen dearth. For example, Abbas et al. (1995) conducted the study during the rainy season in summer in Pakistan. Sihag and Gupta (2011) found an increase in adult bee populations with pollen substitute feeding during winter pollen dearth in India, and again later during a “harsh summer dearth period” (Sihag and Gupta, 2013). Pollen traps were not used in these studies to simulate pollen dearth. On the other hand, some studies have shown no change in adult bee populations after feeding colonies certain pollen substitutes (DeGrandi-Hoffman et al., 2008; Saffari et al., 2010a; Mortensen et al., 2019). In the case of Saffari et al. (2010a), the lack of change with feeding BeePro (Mann Lake Ltd., Hackensack, MN, USA) patties was likely due to diet palatability or quality, because the study was conducted during periods of pollen dearth in late fall and early spring and another diet, Feedbee (Feedbee, Toronto, Canada), performed better. DeGrandi-Hoffman et al. (2008) found no difference in adult bee populations between treatments during summer in Arizona and attributed it to the availability of natural pollen in the area the study was conducted. Pollen substitutes likely are less effective when bees have access to their natural diet. Interestingly, Mortensen et al. (2019) conducted a study during a summer pollen dearth in Florida and found no impact of feeding on colony strength. Perhaps region also plays a role, in which warmer climates show less of a marked impact of pollen substitutes than regions that have harsher winters and shorter colony growth periods.
It is important that investigators measure natural pollen availability during pollen substitute studies. Natural pollen availability can change suddenly, making it impossible to say that a study was conducted during pollen dearth unless it was confirmed that foragers collected little to no pollen during the study. One solution is to include sentinel colonies fitted with pollen traps to measure pollen collection during the study (Noordyke et al., 2021), (Figures 2B,C). Pollen collection could also be measured by performing regular pollen forager counts at the entrances of hives or by measuring the area of bee bread stores regularly. The latter two methods are more time consuming than using sentinel colonies. Future field studies on pollen substitutes should measure natural pollen availability to identify the conditions under which pollen substitutes are effective.
Overall, pollen substitutes can be beneficial for adult bee populations when few natural pollen resources are available, but they might not be economically justifiable when pollen is available in any quality/quantity. More research focused on the specific situations in which pollen substitute feeding impacts colony strength (i.e., season, climate, weather, pollen flow, pollen quality) would be useful for beekeepers who want to optimize the timing of their feeding practices.
Brood Production
Beekeepers also hope that the application of pollen substitutes will stimulate colonies to produce more brood. Despite this, studies on the impact of pollen substitute feeding on brood production have yielded mixed results. Five of 22 studies demonstrated an increase in brood production with pollen substitute feeding. Additionally, three showed no impact, two showed an overall increase but no differences between diet formulations, and 12 were inconclusive (Supplementary Table 1). Nevertheless, as seen for adult bee populations, we can identify some patterns based on the availability of natural pollen, weather conditions, the season during which the studies were conducted, and the quality of the diets tested.
It is almost always during pollen dearth or adverse weather conditions when colonies exhibit an increase in brood production with pollen substitute feeding compared to those not receiving any diet (DeGrandi-Hoffman et al., 2008; Avni et al., 2009; Saffari et al., 2010a; Sihag and Gupta, 2011, 2013; Kumar et al., 2013; Morais et al., 2013). This indicates that feeding pollen substitutes was better for colony brood production than doing nothing at all under these environmental circumstances. Interestingly, Mortensen et al. (2019) did not see an increase in brood production when feeding pollen substitutes compared to nothing at all during a summer pollen dearth in Florida, highlighting that they do not universally lead to more brood production. However, it is possible that some natural pollen was available to the colonies in this study, as the investigators did not measure the amount of pollen coming into the hives. In two studies, investigators showed a significant difference in brood production between colonies fed and not fed pollen substitutes during pollen dearth, but the differences between the two groups vanished when a natural pollen flow began (DeGrandi-Hoffman et al., 2008; Avni et al., 2009). Similarly, Mattila and Otis (2006a) only found a long-term benefit in brood production when they fed pollen substitutes while the weather was unusually cold and rainy in the spring, conditions that prevented foraging. Collectively, these data suggest that pollen substitutes increase the amount of brood produced most when natural pollen is unavailable.
Pollen substitutes likely have negligible impacts on brood when pollen is available naturally. Lamontagne-Drolet et al. (2019) found no difference in brood production between colonies fed commercial pollen substitutes (either pollen-limited or not) and colonies with access to natural pollen. At face value, this suggests that feeding enhanced brood production during pollen limitation in the pollen-limited group. However, a pollen-limited negative control group was not included in the study, making it difficult to know the true impact in this instance. Sometimes, beekeepers feed pollen substitutes regardless of pollen availability, believing that even if natural pollen is available environmentally, supplementing it will lead to more brood. However, the studies cited above highlight that this is not a reasonable assumption.
As for natural pollen availability, the season when substitutes are fed seems to determine if brood production is enhanced. Brood production can be enhanced with spring feeding of pollen substitutes (Mattila and Otis, 2006a; Saffari et al., 2010a). Summer feeding, on the other hand, has not been shown to increase brood production (DeGrandi-Hoffman et al., 2008; Mortensen et al., 2019). Colonies supplemented in the fall may experience brood rearing burnout (colonies had expended their energetic reserves and produced less brood than controls) in the spring (Mattila and Otis, 2006a). Thus, the benefits and drawbacks of fall feedings should be studied further given commercial beekeepers in the U.S. often feed in the fall/winter in attempt to strengthen colonies before using colonies to pollinate early season crops such as almonds. DeGrandi-Hoffman et al. (2008) showed that brood rearing could be stimulated with pollen substitute feeding during an Arizona winter, when control colonies would not produce any brood. Brood rearing increased with added synthetic brood pheromone and pollen substitute in both a subtropical winter (Pankiw et al., 2008) and a temperate fall climate (Sagili and Breece, 2012), but neither study had a control with brood pheromone and no pollen substitute to show how much influence the pollen substitute alone had. The influence of season on the efficacy of feeding is relatively unclear.
Unsurprisingly, diet quality also has an impact on brood rearing. A diet is considered high quality if it provides similar health benefits to honey bees that natural pollen would. Soy flour provides a good example of a diet ingredient that can be enhanced to increase colony productivity. In one study, colonies fed a soybean diet did not produce any brood to the sealed stage (Amro et al., 2016). In another study, colonies fed soy flour alone stopped rearing brood after the first cycle (Hagedorn and Moeller, 1968). Colonies that consumed an unfortified soybean flour diet stopped rearing brood to maturity while soybean flour fortified with riboflavin and niacin supported better brood rearing (Haydak, 1949). Nevertheless, Haydak (1949) suggests that there are other limiting nutrients in soy flour than those two vitamins, because a soy flour/brewer's yeast diet outperformed the fortified soy diet. Soy is typically mixed with many other ingredients to improve diet quality (Sihag and Gupta, 2011, 2013; Kumar et al., 2013), but it is important to consider its potential limitations as an ingredient when formulating new recipes (Saffari et al., 2010a). The same is likely true for other diet components.
Researchers have improved brood production by fortifying pollen substitute diets with various ingredients. The addition of natural pollen to supplemental diets has been shown to improve brood rearing (Sihag and Gupta, 2011); however, older pollen is less suitable for brood production (Hagedorn and Moeller, 1968; Herbert and Shimanuki, 1982). This, of course, raises the possibility that all of the extra brood production that results from feeding a colony a pollen-fortified diet came as a result of the addition of the pollen and not from the supplemental diet. Brood rearing can also be enhanced when pollen substitutes include 2 or 4% lipid extracts from natural pollen (Herbert et al., 1980a). Colonies reared the most brood when cholesterol or 24-methylenecholesterol were added to pollen substitutes (Herbert et al., 1980b). In a later study, the addition of 24-methylenecholesterol to pollen substitutes increased worker survival, abdominal lipid content, and head protein content in the laboratory (Chakrabarti et al., 2020), reinforcing its importance in the honey bee diet.
Some investigators only measured brood production as a short-term response to pollen substitute feeding, but knowing the long-term response also would be useful for beekeepers. Hagedorn and Moeller (1968) measured brood rearing for three consecutive brood cycles. They could differentiate between diets that allowed colonies to rear more brood from diets that allowed colonies to rear brood for longer. It could be helpful for more modern studies to measure the impact of pollen substitutes on brood rearing over multiple brood cycles. Knowing this for more diets would allow beekeepers to choose diets depending on the desired impact on brood rearing.
Also, studies often are designed to address the amount of brood produced, but the quality of brood produced is equally important. Li et al. (2012) looked at the effect of pollen substitute quality on brood development. Egg hatch and pupation success were best when diets had 30–35% crude protein (Li et al., 2012). Brood mortality (Winston et al., 1983) and brood physiology (see “Body Protein Content” and “Morphology/Bee Body Weight”) are also indicators of brood quality. Some investigators have included basic brood quality measures in their studies (Li et al., 2012, 2014), but most, if they include any quality measure at all, tend to focus on adult worker quality (see “Worker Bee Longevity” and “Physiological Response”). Bees use pollen to foster brood growth and development; therefore, focusing on both brood quality, and not just quantity, could be helpful when determining the value of pollen substitutes to brood production.
Honey Production
Stronger colonies (i.e., more adult bees and brood) produce more honey (Bhusal and Thapa, 2006); thus, pollen substitutes often are expected to enhance honey production. Multiple studies have shown that honey production increases with pollen substitute feeding (Doull, 1980; Abbas et al., 1995; Saffari et al., 2010a; Sihag and Gupta, 2011, 2013; Morais et al., 2013). However, all of these studies had a sample size of five or fewer colonies, except for the one conducted by Saffari et al. (2010a). Also, Doull (1980) measured honey production by extrapolating from the total colony weight; therefore, adult bee population, brood, and equipment weight could skew the results. Nevertheless, the other investigators found an increase in honey production in colonies fed pollen substitutes, sometimes as much as 3.8 to 4.6 times the honey produced compared to that produced by colonies in the negative control groups (Abbas et al., 1995).
In other cases, honey production was unaffected by pollen substitutes (Goodwin et al., 1994; Mattila and Otis, 2006a; Avni et al., 2009; Saffari et al., 2010a). A long-term study showed a spike in honey production with spring feeding that leveled out later in the summer (Mattila and Otis, 2006a). They also showed that honey production and other colony strength parameters only increased in the subsequent fall when poor spring weather conditions prevented foraging. This provides additional evidence that pollen substitute feeding might only be effective during times of nutrition stress, regardless of what led to the stress (unavailable pollen, bad weather, etc.).
Haydak and Tanquary (1943) looked at the effects of pollen substitute feeding on package establishment in early April in Minnesota over seven years. They assessed package establishment by measuring brood and honey production over the summer after bees were hived. They found no apparent advantage of feeding pollen substitutes on establishment; however, their sample size was small each year and the authors admit that more research is needed in this area.
Royal Jelly Quality
Nurse bees consume most of the pollen in the colony and assimilate the proteins, fats, vitamins, and minerals into royal jelly and brood food (Wright et al., 2018). Therefore, royal jelly/brood food is directly connected to the pollen nutrition of the colony (Wright et al., 2018). Haydak (1960) looked at vitamin composition of royal jelly from caged colonies fed bee bread or a pollen substitute. The vitamin quality of royal jelly was not especially different between feeding groups in the laboratory. Sereia and de Toledo (2013) found that royal jelly physiochemical and microbiological quality remained relatively constant across colonies fed several different protein diets and sucrose only controls. Feeding pollen substitutes might play a subtle role in royal jelly quality, but more work is needed on this topic.
Queen Quality/Rearing
Two studies have looked at the impact of pollen substitutes on queen weight as an indicator of queen quality (Haydak and Tanquary, 1943; Manning, 2018). Neither included negative controls; so, the conclusions that can be drawn from the studies are limited. Haydak and Tanquary (1943) had limited statistical analysis and discussion on queen weight in their study. Manning (2018) found that queens from colonies fed pollen or soy protein isolate with 10% added oil (equal proportions of almond, linseed, and evening primrose oil) produced the heaviest queens, colonies fed Feedbee produced queens of an intermediate weight, and colonies fed a diet of defatted soybean flour or soy protein isolate with 5% added oil produced the lightest queens. Queen weight might be one indicator of quality, but it does not necessarily tell a beekeeper that a queen will have high reproductive potential.
Pollen substitutes may also influence queen longevity. Queen losses were higher in colonies fed Bee-Pro patties than colonies fed MegaBee patties or pollen over winter, although there was no negative control for comparison (DeGrandi-Hoffman et al., 2016). No other published results on this topic exist.
Worker Bee Longevity
The effect of pollen substitute feeding on honey bee longevity is primarily measured by caging newly emerged workers, feeding them different protein diets, and comparing bee longevity in the various treatment groups. Studies have found that workers live longer when given a pollen substitute than when fed sucrose solution alone (Alqarni, 2006; Manning et al., 2007; Omar et al., 2017; Almeida-Dias et al., 2018). However, worker bees are usually longer lived on a pollen diet than a substitute (Alqarni, 2006; Manning et al., 2007; Manning, 2018), though sometimes bee longevity is the same with either a substitute believed to be of high quality or pollen (Omar et al., 2017; Almeida-Dias et al., 2018).
Although caging and feeding workers in a laboratory is the common way of determining the impact of pollen substitutes on bee longevity, some investigators have allowed colonies to rear brood on substitute diets and caged the resulting newly emerged workers to compare longevity between treatment groups (Mattila and Otis, 2006b; Li et al., 2014; Lamontagne-Drolet et al., 2019). This method could reveal more about how pollen substitutes impact bee longevity at the colony level than could laboratory-based studies. Colonies that were fed diets with 25 or 35% crude protein produced workers that lived longer than those reared on diets with 15% crude protein (Li et al., 2014). In contrast, Lamontagne-Drolet et al. (2019) found that feeding two commercial pollen substitutes to colonies actually shortened worker bee lifespan. They saw no other negative impact of the substitutes on colony health (except maybe increased Varroa destructor infestations in one instance; see “Varroa destructor Infestation”), but decreased longevity could indicate adverse physiological effects. Mattila and Otis (2006b) found that workers reared in colonies fed pollen supplements or substitutes lived longer than workers from pollen-limited colonies during the first year of a study, while living shorter periods the next year. They attributed this difference to environmental factors changing between years, such as adverse weather and natural pollen availability. These mixed results are concerning, especially if workers reared on pollen substitutes die sooner than if they were given no substitute at all.
Less studied is the effect of pollen substitute feeding on brood survival. Winston et al. (1983) found decreased brood mortality in colonies fed pollen substitutes compared to those in the control group. The reverse was true for adult bees in the study. The sample size was small, but this study indicates that artificial diets may impact immature and adult survival differently.
Certain diet ingredients can negatively affect bee survival. Raffinose and stachyose, which comprise about 40% of the sugars found in soybeans, are toxic to honey bees (Barker, 1977). Fortunately, these sugars can be diluted to safe levels with sucrose (Barker, 1977). Manning et al. (2007) found that caged bees on soy flour alone did not live as long as caged bees with soy flour mixed with other ingredients. They also found increased mortality risk with levels above 2% oleic acid and decreased risk with 6% linoleic acid. Lactose also may be toxic to honey bees (Herbert and Shimanuki, 1978) so beekeepers should carefully check diets that include milk products to assess the amount of lactose present before using them. Moving forward, it is important to consider possible toxicity of diet ingredients and how diet formulations may impact worker bee survival.
Pest and Pathogen Response
Honey bees are typically more resistant to pests and pathogens when they are well-nourished than when not (Rinderer et al., 1974; Alaux et al., 2010; Di Pasquale et al., 2013; Dolezal and Toth, 2018; Dolezal et al., 2019; Hristov et al., 2020), although variation in seasonal nutritional requirements may influence honey bee response to infections (DeGrandi-Hoffman et al., 2018). DeGrandi-Hoffman and Chen (2015) suggested that investigators who develop new pollen substitutes should pay closer attention to the seasonal needs of honey bees. For example, fall honey bees could benefit from diets that boost immunity before winter (DeGrandi-Hoffman and Chen, 2015). Previous studies have looked at viral loads (DeGrandi-Hoffman et al., 2010, 2016), Vairimorpha (Nosema) spp. [new genus name Vairimorpha (Tokarev et al., 2020)] loads (Fleming et al., 2015; DeGrandi-Hoffman et al., 2016; Lamontagne-Drolet et al., 2019; Mortensen et al., 2019), and Varroa destructor infestation in individual bees or colonies fed pollen substitutes (Lamontagne-Drolet et al., 2019). One study explored how pollen substitutes affect small hive beetle (Aethina tumida) health in the laboratory (Stuhl, 2017). Both American (Paenibacillus larvae) and European (Melissococcus plutonius) foulbrood have been overlooked in pollen substitute research.
Viral Infection
In three studies, investigators measured the influence of pollen substitute feeding on viruses that commonly infect honey bees. Workers from colonies fed exclusively MegaBee patties or Bee-Pro patties over winter had higher rates of Black queen cell virus (BQCV) compared to workers from colonies allowed to forage on high quality pollen (DeGrandi-Hoffman et al., 2016). It is possible that a lack of essential nutrients or a harmful ingredient in the pollen substitutes caused higher susceptibility to BQCV. However, it is important to note that the pollen substitute-fed colonies in this study were fitted with pollen traps, which can induce stress in colonies. In the same study, there were no clear patterns in Deformed wing virus (DWV), Israeli acute paralysis virus (IAPV), Kashmir bee virus (KBV), or Sacbrood titers in bees from colonies fed pollen substitutes versus those fed pollen (DeGrandi-Hoffman et al., 2016). Workers from colonies fed natural pollen patties had significantly lower DWV titers than did workers from colonies fed Bee-Pro patties (Watkins de Jong et al., 2019). On the other hand, DeGrandi-Hoffman et al. (2010) found that Deformed wing virus titers were significantly lower in caged bees fed pollen or MegaBee than those fed high fructose corn syrup. This suggests that pollen substitutes can help prevent disease, though caution should be used when interpreting these results given this was a laboratory-based study.
Vairimorpha (Nosema) ceranae Infection
Vairimorpha (Nosema) cerana is a microsporidian pathogen that competes for nutrition in the guts of infected bees (Mayack and Naug, 2009). Consequently, some investigators have conducted research on the relationship between V. ceranae loads and pollen substitute feeding. As with the viral load research, the results are generally mixed.
Worker bees fed Bee-Pro in cages in the laboratory had increased V. ceranae counts during fall compared to those fed wildflower pollen and other commercial diets; however, there was no increase in V. ceranae in bees fed during spring (Fleming et al., 2015). When this study was scaled to the colony level during summer, there was no difference in V. ceranae spore counts between workers from colonies fed wildflower pollen patties or those from colonies fed pollen substitutes (Mortensen et al., 2019). Similarly, workers fed Bee-Pro in the laboratory had significantly higher V. ceranae counts than did workers fed wildflower pollen, although the season during which the study was conducted was not mentioned (Watkins de Jong et al., 2019). Workers from colonies given natural pollen or Bee-Pro with 10% added natural pollen had decreased V. ceranae loads compared to those of workers from colonies fed Bee-Pro alone, suggesting that adding a small amount of natural pollen to artificial diets could be beneficial to colony health (Watkins de Jong et al., 2019). Lamontagne-Drolet et al. (2019) found no difference in V. ceranae in workers from full colonies fed various protein diets in the spring. On the other hand, DeGrandi-Hoffman et al. (2016) found more V. ceranae in workers from colonies fed MegaBee and Bee-Pro over winter than in workers from colonies that foraged naturally. However, all test colonies were fitted with pollen traps which may have been an additional source of stress promoting Vairimorpha (Nosema) spore counts. It is difficult to determine if pollen substitutes impact V. ceranae colonization in the honey bee midgut given the conflicting accounts. Seasonal variation in V. ceranae prevalence and sensitivity to different diets could be driving the differences in the literature.
Small Hive Beetle
Small hive beetles (SHBs) are common secondary pests of honey bee colonies that are attracted to pollen and nectar stores (Ellis and Hepburn, 2006). Pollen substitutes are anecdotally believed to attract SHBs in colonies. Stuhl (2017) reared SHBs on several commercial pollen substitutes in the laboratory, a common practice when rearing SHBs in vitro (Neumann et al., 2013). Bee-Pro supported the best SHB longevity but did not support the best reproduction. Global Patties (Airdrie, AB, Canada) yielded the most larvae but did not facilitate the best survival (likely because the diet was overrun by larvae). The author concluded that many pollen substitute diets could support SHB survival and reproduction. In another study, investigators found that SHB infestation and damage were worse in colonies given vegetable shortening patties than colonies without patties (Elzen et al., 2002). Although vegetable shortening patties are not pollen substitutes, this suggests that pollen substitutes should be used and monitored carefully in regions where SHBs are abundant. Diets should also be highly palatable to honey bees so they are consumed quickly before SHBs can establish (Stuhl, 2017). This can also be addressed by providing less diet to colonies at a single feeding, with more frequent feedings, to allow bees to consume the diet quickly.
Varroa destructor Infestation
The relationship between V. destructor infestation and pollen substitute feeding is understudied. Investigators from one study found that colonies fed Global Patties, a pollen supplement, had more V. destructor than did colonies fed UltraBee (Mann Lake Ltd., Hackensack, MN, USA) or pollen (Lamontagne-Drolet et al., 2019). The patterns in the other health parameters the investigators measured could not explain this difference. Pollen substitute feeding also shortened worker bee lifespan in this study, but more so in colonies fed UltraBee than Global Patties. Thus, it is not clear how pollen substitute feeding can influence V. destructor infestation.
Physiological Response
This section focuses on physiological response of individual bees to pollen substitutes. It is important to take a closer look at individual bee development, behavior, and function in response to pollen substitutes. Ideally, improvements in individual bee health due to pollen substitute feeding would have upstream effects on colony productivity.
Antioxidant Activity
Workers from colonies that consumed pollen substitutes with higher dietary protein had more activity of the superoxide dismutase enzyme (an antioxidizing agent) than did workers from colonies that consumed substitutes with lower protein (Li et al., 2012). Diets with 35% protein also supported the best brood development (Li et al., 2012). Antioxidant enzyme activity is not well-studied in honey bees and the authors suggest further research in this area.
Behavior
Colonies utilize pollen substitute patties differently than they do natural pollen. Investigators found that a proportion of workers ingested pollen substitute patties, workers did not store patty as bee bread, and workers did not feed patty directly to developing larvae (Noordyke et al., 2021). Workers only minimally lost patty as colony debris (Noordyke et al., 2021).
Digestibility
Honey bee ingestion of a diet does not imply the diet is digestible. Artificial diets contain many protein sources that are not natural to the honey bee diet and may not be easily digested (DeGrandi-Hoffman et al., 2016). DeGrandi-Hoffman et al. (2016) found that nurse bees digested about 35% of the soluble protein in two artificial diets compared to 70% of the soluble protein in pollen. It is a waste of materials, and money for the beekeeper, if the majority of the protein in artificial diets is indigestible and expelled as frass.
Another measure of digestibility, other than measuring soluble protein content throughout the gut, is the number of digestive enzymes present in the gut. Li et al. (2012) found increased proteolytic enzyme activity in the midguts of workers fed diets with higher protein contents. This is evidence that bees do digest pollen substitutes but it does not indicate to what extent they digest them.
Fat Body Size
Fat bodies are responsible for endocrine regulation, immunity, vitellogenesis, the production of antimicrobial molecules, and nutrient storage and regulation in insects (Arrese and Soulages, 2010). Because fat bodies are linked to nutrition, it is believed that a quality diet will result in larger fat bodies (Ricigliano and Simone-Finstrom, 2020). Fat bodies were larger in bees given pollen substitutes fortified with essential amino acids than in bees provided pollen substitutes without fortified ingredients (Rogala and Szymaś, 2004). Also, fat bodies were the same size in bees fed pure dry spirulina (an ingredient being tested for its suitability in pollen substitutes) and in bees fed pollen in the laboratory (Ricigliano and Simone-Finstrom, 2020). Both pure dry spirulina and pollen resulted in larger fat bodies in bees than did sugar alone (Ricigliano and Simone-Finstrom, 2020). These results suggest that high quality pollen substitutes can increase honey bee fat body size when natural pollen is lacking, but more research is necessary to determine this.
Effects on the Expression of Immune-Related Genes
Gene expression can indicate how an organism responds to nutrition at the molecular level. There is a general trend that pollen substitutes upregulate genes related to immune function (Danihlík et al., 2018), vitellogenin synthesis (Ricigliano and Simone-Finstrom, 2020), and antioxidant enzymes (Li et al., 2014). Danihlík et al. (2018) demonstrated that an upregulation of immune-related genes resulted in significantly more antimicrobial peptide apidaecin 1 in bees fed Feedbee or pollen versus bees fed sucrose solution only.
Effects on Hemocyte Count and Cell-Mediated Immunity
Few investigators have used hemocyte count and cellular structure of the hemolymph to measure honey bee health with pollen substitute feeding. Hemocyte count can be problematic as an indicator of bee health on its own. Rogala and Szymaś (2004) found that hemocyte count in caged workers increased with increasing quality of pollen substitutes. Interestingly, bees that consumed natural pollen had lower hemocyte counts than did bees that consumed pollen substitutes, but natural pollen performed better in the other physiological parameters that they tested. This suggests that increased hemocyte counts do not necessarily indicate better physiological health.
Measuring the numbers of different types of hemocytes and their overall metabolic activity could indicate more about honey bee nutrition than measuring hemocyte count alone. The function and structure of the hemolymph cellular system was impaired when bees did not receive protein (Szymaś and Jedruszuk, 2003). A sugar only diet resulted in bees with higher counts of older hemocytes with lower metabolic activity than in bees fed pollen substitutes or natural pollen (Szymaś and Jedruszuk, 2003). In this case, hemocyte counts were similar between bees in the pollen and pollen substitute groups, but the metabolic activity of hemocytes was slightly lower in pollen substitute groups.
Hemolymph Protein Titer
Hemolymph transports digested nutrients throughout the insect body for use or storage (Simpson et al., 1995). Researchers have regarded high soluble protein levels in the hemolymph as an indicator of a good pollen substitute. In fact, most studies on hemolymph protein titers showed that bees provided pollen substitutes had higher soluble protein in their hemolymph than did bees provided only sucrose (Cremonez et al., 1998; De Jong et al., 2009; Morais et al., 2013; Almeida-Dias et al., 2018; Paiva et al., 2019). This makes sense because a bee without access to any protein would not suddenly have large amounts in the hemolymph. As a next step, it is important to know if pollen substitutes increase hemolymph protein content as much as does natural pollen. Some researchers found that hemolymph protein titers of pollen substitute-fed bees were lower than in pollen-or bee bread-fed bees (Cremonez et al., 1998; Amro et al., 2016; Almeida-Dias et al., 2018; Paiva et al., 2019). Other researchers found that certain pollen substitutes caused bees to have the same or higher hemolymph protein titers than pollen-or bee bread-fed bees (Cremonez et al., 1998; De Jong et al., 2009; Morais et al., 2013; DeGrandi-Hoffman et al., 2016). These results suggest that pollen substitutes provide some benefit over nothing at all.
Bee bread is a fermented pollen and honey product. Thus, some researchers have tried to ferment pollen substitutes to make the diets more palatable/useful to the bees. Bees that consumed fermented diets had higher hemolymph protein titers than did bees consuming unfermented diets (Almeida-Dias et al., 2018; Paiva et al., 2019) possibly because they consumed more fermented diet or because fermentation increased digestibility (Paiva et al., 2019).
Investigators of only two studies on hemolymph protein and pollen substitute feeding looked at bees fed in full-size colonies (Amro et al., 2016; DeGrandi-Hoffman et al., 2016). Amro et al. (2016) found that bees from free flying colonies given bee bread had more hemolymph protein than did bees from colonies given substitutes in flight cages. DeGrandi-Hoffman et al. (2016) found no difference in hemolymph protein between bees from free flying and substitute-fed colonies whose hives were fitted with pollen traps. Neither study included controls for flight cages or pollen traps. Both induce stress that could influence bee physiology.
Hypopharyngeal Gland Development
Different protein sources are known to influence the development of honey bee worker glands. The hypopharyngeal glands of nurse bees secrete the proteinaceous portion of brood food (Wright et al., 2018). Better hypopharyngeal gland development with feeding could indicate better results downstream for brood production. Hypopharyngeal gland development is determined by measuring the acini size or the protein content of the glands (Corby-Harris and Snyder, 2018). Many studies conducted on this topic in the laboratory did not include negative controls; so, it is hard to know the true impact of the test diets on the measured parameters. However, the studies that did include negative controls showed that bees that consumed pollen substitutes had better hypopharyngeal gland development than bees that did not (Pernal and Currie, 2000; Alqarni, 2006; DeGrandi-Hoffman et al., 2010; Omar et al., 2017). One study showed that hypopharyngeal gland development was the same for bees fed pollen and a commercial pollen substitute, although the size of acini was only correlated with the amount of pollen consumed and not pollen substitute (DeGrandi-Hoffman et al., 2010). On the other hand, multiple studies found that high quality, fresh, and/or mixed pollens promoted hypopharyngeal gland development more than did pollen substitutes (Hagedorn and Moeller, 1968; Pernal and Currie, 2000; Rogala and Szymaś, 2004; Omar et al., 2017). Furthermore, artificial supplements with added pollen resulted in better hypopharyngeal gland development in bees than did diets without pollen (Alqarni, 2006; Watkins de Jong et al., 2019). Like many other health parameters, it seems that pollen substitutes are better than no protein source at all but could be improved to support better hypopharyngeal gland development.
Pankiw et al. (2008) measured hypopharyngeal gland development in bees fed pollen substitutes in the presence of brood pheromone in full colonies. Interestingly, the addition of synthetic brood pheromone resulted in greater patty consumption and hypopharyngeal gland development than did the absence of synthetic brood pheromone. They did not include a control in which they gave pheromone and no pollen substitute; therefore, we cannot be certain that the brood pheromone/pollen substitute combination was the driver. Brood pheromone may have exerted more of an effect on the nurse bees than the addition of pollen substitute.
There are a few gaps in the literature that focus on hypopharyngeal gland development with pollen substitute feeding. In general, there is a lack of studies that measure hypopharyngeal gland development and pollen substitute feeding at the colony level. Additionally, it would be helpful to measure hypopharyngeal gland size alongside the quality and quantity of brood food produced by nurse bees fed pollen substitutes. This would allow one to determine if the increased gland size actually led to the production of more/better brood food.
Influence on Gut Microbiome Diversity
The composition of the honey bee gut microbiome is linked to nutrition (Raymann and Moran, 2018) and could have interesting implications for pollen substitute research. However, only one study has included an analysis of the honey bee gut microbiome with pollen substitute feeding. Ricigliano and Simone-Finstrom (2020) measured the relative abundance of Lactobacillus Firm 5, Bifidobacterium, and Snodgrassella in the entire honey bee gut. A diet of spirulina supported the most Lactobacillus Firm 5 than any other diet type, and generally performed the best in all three microbial species. Bees fed a more traditional pollen substitute or a negative control had lower bacterial abundance in comparison. Certainly, more work is needed on this subject.
Morphology/Bee Body Weight
Some investigators have studied the impact of artificial pollen diets on the morphology and/or body weight of bees. However, only a few included negative controls for comparison. Nevertheless, some patterns can still be distinguished. For example, the weight of newly emerged workers increased linearly with increasing protein content in pollen substitutes, but the weight of pupae was not different (Li et al., 2012). Worker bee head weight was highest in bees fed pollen, followed by Feedbee, and then other soy-based diets (Manning, 2018). It took workers longer to reach maximum thorax weight when they consumed soy-based diets mixed with pollen that was stored frozen or dried over multiple years (Hagedorn and Moeller, 1968). Thorax weight was highest in bees fed dry spirulina compared to those fed pollen, a commercial pollen substitute, and a sugar control (Ricigliano and Simone-Finstrom, 2020). Both studies showed that inferior nutrition could affect thorax development. In another study, the dry weight of workers was not affected by diet, but it was possible that incoming natural pollen diminished detectable effects of supplementation (Mattila and Otis, 2006b).
In terms of morphology, Szymaś et al. (2012) evaluated the epithelial structure of the midgut of bees fed bee bread, a pollen substitute, or pollen substitutes fortified with probiotics in cages. They concluded that pollen substitutes fortified with probiotics were beneficial for the epithelial tissues in the honey bee midgut. Despite this, the methods the investigators used to evaluate the epithelium of the midgut were rather subjective and not well-described; thus, it is difficult to draw conclusions from their results. It seems that bee body weight can be an indicator of the nutritional value of a diet, but the impact of diet on gut morphology is less clear.
Body Protein Content
Body protein content may be an indication of how well a honey bee is able to store or assimilate dietary proteins. Soluble protein of newly emerged adults and larvae was higher in colonies that had access to natural pollen than in colonies that were pollen-limited and fed substitutes (Amro et al., 2016). On the other hand, nurse bees from colonies that had access to natural pollen had lower soluble body protein than nurse bees from colonies provided pollen substitutes (Lamontagne-Drolet et al., 2019). In another study, newly emerged workers and larvae had higher soluble body protein with increasing dietary protein (Li et al., 2012, 2014). Interestingly, this also corresponded to increased body weight in the resulting adults (Li et al., 2012).
The protein content of individual bee body parts has also been measured after pollen substitute feeding. The protein of the head capsule was higher in caged worker bees fed pollen substitutes than those fed sucrose alone (DeGrandi-Hoffman et al., 2010; Ricigliano and Simone-Finstrom, 2020). Protein of the head capsule can also indicate hypopharyngeal gland development (DeGrandi-Hoffman et al., 2010). Although the results for body protein content are not entirely consistent, it seems that pollen substitutes can have a positive impact.
Vitellogenin
A few studies that measured soluble protein titers in hemolymph went a step further and measured vitellogenin levels in the hemolymph. Vitellogenin is a glycolipoprotein which plays roles in nutrient storage, nursing and foraging behavior, lifespan, and overwintering physiology, among others (Amdam et al., 2012). Vitellogenin patterns were generally similar to soluble hemolymph protein. Like soluble protein, vitellogenin titers increased with pollen substitute feeding compared to negative controls (Cremonez et al., 1998; Paiva et al., 2019). Also, fermented diets resulted in higher vitellogenin levels in bees than did unfermented diets (Almeida-Dias et al., 2018; Paiva et al., 2019). Although the research on this subject is limited, these studies suggest that vitellogenin levels can be improved with pollen substitute feeding.
Discussion
The collective literature suggests mixed results from feeding colonies pollen substitutes, likely due to the inherent challenges encountered when conducting this type of research. This is due to a few primary reasons: (1) there are a multitude of diets to test, (2) the diets can have different formulations which change frequently, (3) there is great variability in the environments in which tests are conducted, (4) results from laboratory studies do not necessarily reflect what would occur in the field, and (5) many of the diet studies are missing key design elements.
First, it is difficult to test all of the pollen substitute or supplement recipes because there are so many of them in use. In addition to the commercial recipes, beekeepers may also add their own ingredients to commercial formulations or make a unique recipe. It is hard to get a clear picture of the efficacy of pollen substitutes in general when each recipe has a potentially different impact on honey bee colony health. It would be beneficial to beekeepers if each pollen substitute recipe was thoroughly tested before use; yet, this is not realistic given the sheer number of recipe variations. Although the peer reviewed literature covers a large number of commercial and homemade pollen substitute recipes (Supplementary Tables 1–3), only a small fraction of what beekeepers use in their operations has been tested.
Additionally, some of the diets have different formulations, making it necessary to determine the efficacy of all the test formulations. The manufacturer can change these frequently, even before adequate research can be conducted on the older formulation. A commercial pollen substitute diet that was tested years ago could have a different formulation today. As an interesting aside, many of the diets available commercially were created by industry, with hardly any literature available to evaluate the diet before it reaches the market. In fact, many of the diets have little peer reviewed research to support their efficacy.
Variability in the environments in which diets are tested is another factor influencing pollen substitute efficacy (region, climate, weather, season, pollen availability). It is impossible to design experiments for every single situation. Thus, beekeepers must do a lot of work to determine which situations necessitate feeding pollen substitutes and which do not. If pollen substitutes only work under specific environmental circumstances as suggested by the literature, then beekeepers are likely wasting effort and money on feeding pollen substitutes to their colonies.
Another challenge is that results from laboratory studies do not always agree with those derived from colony-level studies (Fleming et al., 2015; Manning, 2018; Mortensen et al., 2019). When possible, it would be helpful to pair laboratory and field studies, doing both simultaneously. Results are more reliable when laboratory and field studies are consistent. In instances when the results do not agree, the differences between the two can reveal environmental variables that influence pollen substitute efficacy. Although laboratory studies are useful for controlling the environmental variables inherent to field studies, it is important to remember that the results might not be relevant to beekeepers.
Another potential reason for inconsistencies in the literature is a general lack of key design elements in many of the diet studies. There are four main ways this has occurred in the literature: (1) lack of controls, (2) low sample size, (3) laboratory studies, and (4) lack of pollen monitoring.
For example, many studies on pollen substitutes lack proper controls (Supplementary Tables 1–3). It is difficult to draw conclusions and make solid comparisons between studies when negative controls (colonies not receiving any type of diet) are frequently omitted from study designs. ~37% of studies covered in this literature review lacked negative controls (Supplementary Tables 1–3). Negative controls, both at laboratory and field levels, should consist of bees/colonies that are pollen-limited and receive only sugar diets. If a pollen substitute does not promote honey bee health more than does a negative control diet, then the diet is not a useful investment for beekeepers. It is understandably risky to deprive colonies of protein for long periods of time (Lamontagne-Drolet et al., 2019). Researchers who partner with beekeepers must be transparent about the use of negative controls.
Likewise, much of the research conducted on pollen substitutes is plagued by low sample size. Admittedly, honey bee colonies are complex and variable, making it difficult to use enough colonies in a study. However, it is easy to lose statistical power if sample size is too low (i.e., n < 10 colonies). Sample size is most often an issue with studies conducted on pollen substitutes prior to 1980. It could be beneficial to revisit and revitalize older studies because they laid the groundwork for the diet formulations beekeepers use today, but many doing so with a very low sample size.
As mentioned previously, laboratory studies designed to test pollen substitute efficacy are limited. Although it is easier to increase the sample size when studying caged bees in the laboratory than when studying full honey bee colonies in the field, full colonies may respond differently to feeding.
Another issue is that investigators do not usually measure the amount of natural pollen coming into colonies during field studies. Many investigators claim that their study was conducted during a natural pollen dearth; however, it is impossible to know this if colonies are not being monitored for the presence of pollen. This can be resolved in three ways. Study designs can include (1) sentinel colonies with pollen traps to monitor pollen flow, (2) strategies to monitor incoming pollen foragers, or (3) frequent monitoring of combs within a hive for bee bread stores. Of these, using sentinel colonies is the least time-consuming and invasive method. Additionally, sentinel colonies provide pollen samples that can be frozen and saved for later analysis (nutrient profile, pesticide load, etc.). Natural pollen availability likely influences the efficacy of pollen substitutes (DeGrandi-Hoffman et al., 2008). Thus, it is important to measure the amount of natural pollen available to colonies during pollen substitute studies.
Despite some of the challenges in pollen substitute research, we can conclude that pollen substitutes are usually better for colony health than a complete lack of protein, but less so when any pollen is available at all in the environment. Currently, the literature suggests that pollen substitutes are most useful during times of extreme pollen dearth or environmental conditions that prevent foraging. Beekeepers might be able to use this knowledge to maximize colony health for the effort put into feeding pollen substitutes.
However, we cannot ignore the fact that pollen substitutes are generally inferior to natural pollen (Manning, 2018; Wright et al., 2018). Planting diverse natural forage for bees is still the best long-term solution to honey bee nutritional stress (DeGrandi-Hoffman et al., 2016). Until we find better ways to provide natural pollen for honey bees, pollen substitutes are a convenient short-term fix. The highly migratory nature of commercial beekeeping also necessitates portable and available artificial diets. The goal of future pollen substitute research should be to improve the efficacy of diets.
As suggested throughout the body of this literature review, there are plenty of gaps in pollen substitute research that could be filled to benefit beekeepers. A major cause for concern is the potential for certain diets to increase pest and pathogen loads or decrease bee longevity (Supplementary Tables 1–3). Pollen substitutes should not put the health of colonies at risk; thus, there is an immediate need for more research in this area. Also, more research is needed on diet impacts on brood quality and physiology, queen health, and diet digestibility. Even if a pollen substitute has the ideal protein formulation, it can still be inadequate if it is limited in other essential nutrients for honey bee growth and development. Additionally, we do not have a complete understanding of the fat, vitamin, and mineral requirements of honey bees (Wright et al., 2018). Basic honey bee nutrition needs continued attention to improve feeding regimens and honey bee health.
Another need is to conduct pollen substitute studies with commercial colonies managed by beekeepers rather than simply in the laboratory or with research colonies maintained by academic or other investigative institutions (Mattila and Otis, 2006a; Lamontagne-Drolet et al., 2019). Commercial colonies undergo significant stress that research colonies might not undergo (Mattila and Otis, 2006a). Also, commercial colonies often experience many different climates, regions, and weather patterns in which we do not fully understand the dynamics of pollen substitute feeding.
The following, though not exhaustive, is a list of critical research gaps:
- Pollen substitute impact on pest and pathogen intensity (viruses, Varroa, Vairimorpha (Nosema) spp., small hive beetle, American and European foulbrood)
- Pollen substitute impact on brood quality and physiology
- Pollen substitute impact on queen health
- Pollen substitute digestibility to adult worker bees
- Pollen substitute fat, vitamin, and mineral nutrients impact on individual worker and colony health
- Pollen substitute impact on commercial honey bee colony health, especially during migration and various pollination contracts
- Pollen substitute efficacy under various environmental circumstances relevant to beekeepers such as different climates, regions, weather patterns, and seasons
- Pollen substitute efficacy when different quality and quantity of natural pollen is available to colonies
Pollen substitutes are an important part of modern colony management. Researchers can significantly improve feeding management best practices by focusing on the gaps in the current literature. Refined practices will result in better use of research funds toward the improvement of honey bee colony health.
Author Contributions
EN and JE wrote the manuscript following an initial draft by EN. Both authors contributed to the article and approved the submitted version.
Conflict of Interest
The authors declare that the research was conducted in the absence of any commercial or financial relationships that could be construed as a potential conflict of interest.
Publisher's Note
All claims expressed in this article are solely those of the authors and do not necessarily represent those of their affiliated organizations, or those of the publisher, the editors and the reviewers. Any product that may be evaluated in this article, or claim that may be made by its manufacturer, is not guaranteed or endorsed by the publisher.
Acknowledgments
We thank Dr. Bryony Bonning and Dr. William Kern for providing valuable comments on earlier drafts. A previous draft of this review appears in a Master's thesis in the University of Florida's online thesis and dissertation repository (Noordyke, 2020).
Supplementary Material
The Supplementary Material for this article can be found online at: https://www.frontiersin.org/articles/10.3389/fsufs.2021.772897/full#supplementary-material
References
Abbas, T., Hasnain, A., and Ali, R. (1995). Black gram as a pollen substitute for honey bees. Anim. Feed Sci. Technol. 54, 357–359. doi: 10.1016/0377-8401(95)00772-F
Alaux, C., Ducloz, F., Crauser, D., and Le Conte, Y. (2010). Diet effects on honeybee immunocompetence. Biol. Lett. 6, 562–565. doi: 10.1098/rsbl.2009.0986
Almeida-Dias, J. M., Morais, M. M., Francoy, T. M., Pereira, R. A., Turcatto, A. P., and De Jong, D. (2018). Fermentation of a pollen substitute diet with beebread microorganisms increases diet consumption and hemolymph protein levels of honey bees (Hymenoptera, Apidae). Sociobiology 65, 760–765. doi: 10.13102/sociobiology.v65i4.3293
Alqarni, A. S. (2006). Influence of some protein diets on the longevity and some physiological conditions of honeybee Apis mellifera L. workers. J. Biol. Sci. 6, 734–737. doi: 10.3923/jbs.2006.734.737
Amdam, G. V., Fennern, E., and Havukainen, H. (2012). “Vitellogenin in honey bee behavior and lifespan,” in Honeybee Neurobiology and Behavior, eds C. Galizia, D. Eisenhardt, and M. Giurfa (Dordrecht: Springer), 17–29. doi: 10.1007/978-94-007-2099-2_2
Amro, A., Omar, M., and Al-Ghamdi, A. (2016). Influence of different proteinaceous diets on consumption, brood rearing, and honey bee quality parameters under isolation conditions. Turk. J. Vet. Anim. Sci. 40, 468–475. doi: 10.3906/vet-1507-28
Arrese, E. L., and Soulages, J. L. (2010). Insect fat body: energy, metabolism, and regulation. Annu. Rev. Entomol. 55, 207–225. doi: 10.1146/annurev-ento-112408-085356
Avni, D., Dag, A., and Shafir, S. (2009). The effect of surface area of pollen patties fed to honey bee (Apis mellifera) colonies on their consumption, brood production and honey yields. J. Apic. Res. 48, 23–28. doi: 10.3896/IBRA.1.48.1.06
Barker, R. J. (1977). Some carbohydrates found in pollen and pollen substitutes are toxic to honey bees. J. Nutr. 107, 1859–1862. doi: 10.1093/jn/107.10.1859
Bhusal, S. J., and Thapa, R. B. (2006). Response of colony strength to honey production: regression and correlation analysis. J. Inst. Agric. Anim. Sci. 27, 133–137. doi: 10.3126/jiaas.v27i0.706
Brodschneider, R., and Crailsheim, K. (2010). Nutrition and health in honey bees. Apidologie 41, 278–294. doi: 10.1051/apido/2010012
Carroll, M. J., Brown, N., Goodall, C., Downs, A. M., Sheenan, T. H., and Anderson, K. E. (2017). Honey bees preferentially consume freshly-stored pollen. PLoS ONE. 12:e0175933. doi: 10.1371/journal.pone.0175933
Chakrabarti, P., Lucas, H. M., and Sagili, R. R. (2020). evaluating effects of a critical micronutrient (24-methylenecholesterol) on honey bee physiology. Ann. Entomol. Soc. 113, 176–182. doi: 10.1093/aesa/saz067
Corby-Harris, V., and Snyder, L. A. (2018). Measuring hypopharyngeal gland acinus size in honey bee (Apis mellifera) workers. J. Vis. Exp. 139:58261. doi: 10.3791/58261
Cremonez, T. M., De Jong, D., and Bitondi, M. M. G. (1998). Quantification of hemolymph proteins as a fast method for testing protein diets for honey bees (Hymenoptera: Apidae). J. Econ. Entomol. 91, 1284–1289. doi: 10.1093/jee/91.6.1284
Danihlík, J., Škrabišová, M., Lenobel, R., Šebela, M., Omar, E., Petˇrivalský, M., et al. (2018). Does the pollen diet influence the production and expression of antimicrobial peptides in individual honey bees? Insects 9:79. doi: 10.3390/insects9030079
De Jong, D., da Silva, E. J., Kevan, P. G., and Atkinson, J. L. (2009). Pollen substitutes increase honey bee haemolymph protein levels as much as or more than does pollen. J. Apic. Res. 48, 34–37. doi: 10.3896/IBRA.1.48.1.08
Decourtye, A., Mader, E., and Desneux, N. (2010). Landscape enhancement of floral resources for honey bees in agro-ecosystems. Apidologie 41, 264–277. doi: 10.1051/apido/2010024
DeGrandi-Hoffman, G., and Chen, Y. (2015). Nutrition, immunity, and viral infections in honey bees. Curr. Opin. Insect Sci. 10, 170–176. doi: 10.1016/j.cois.2015.05.007
DeGrandi-Hoffman, G., Chen, Y., Huang, E., and Huang, M. H. (2010). The effect of diet on protein concentration, hypopharyngeal gland development and virus load in worker honey bees (Apis mellifera L.). J. Insect Physiol. 56, 1184–1191. doi: 10.1016/j.jinsphys.2010.03.017
DeGrandi-Hoffman, G., Chen, Y., Rivera, R., Carroll, M., Chambers, M., Hidalgo, G., et al. (2016). Honey bee colonies provided with natural forage have lower pathogen loads and higher overwinter survival than those fed protein supplements. Apidologie 47, 186–196. doi: 10.1007/s13592-015-0386-6
DeGrandi-Hoffman, G., Gage, S. L., Corby-Harris, V., Carroll, M., Chambers, M., Graham, H., et al. (2018). Connecting the nutrient composition of seasonal pollens with changing nutritional needs of honey bee (Apis mellifera L.) colonies. J. Insect Physiol. 109, 114–124. doi: 10.1016/j.jinsphys.2018.07.002
DeGrandi-Hoffman, G., Wardell, G., Ahumada-Segura, F., Rinderer, T., Danka, R., and Pettis, J. (2008). Comparisons of pollen substitute diets for honey bees: consumption rates by colonies and effects on brood and adult populations. J. Apic. Res. 47, 265–270. doi: 10.1080/00218839.2008.11101473
Di Pasquale, G., Salignon, M., Le Conte, Y., Belzunces, L. P., Decourtye, A., Kretzschmar, A., et al. (2013). Influence of pollen nutrition on honey bee health: do pollen quality and diversity matter? PLoS ONE. 8:e72016. doi: 10.1371/journal.pone.0072016
Dolezal, A. G., Carrillo-Tripp, J., Judd, T. M., Miller, W. A., Bonning, B. C., and Toth, A. L. (2019). Interacting stressors matter: diet quality and virus infection in honeybee health. Royal Soc. Open Sci. 6:181803. doi: 10.1098/rsos.181803
Dolezal, A. G., Carrillo-Tripp, J., Miller, W. A., Bonning, B. C., and Toth, A. L. (2016). Intensively cultivated landscape and Varroa mite infestation are associated with reduced honey bee nutritional state. PLoS ONE 11:e0153531. doi: 10.1371/journal.pone.0153531
Dolezal, A. G., and Toth, A. L. (2018). Feedbacks between nutrition and disease in honey bee health. Curr. Opin. Insect Sci. 26, 114–119. doi: 10.1016/j.cois.2018.02.006
Doull, M. K. (1980). Relationships between consumption of a pollen supplement, honey production, and brood rearing in colonies of honeybees Apis mellifera L. I. Apidologie 11, 361–365. doi: 10.1051/apido:19800404
Ellis, A. M., and Hayes, G. W. (2009). An evaluation of fresh vs. fermented diets for honey bees (Apis mellifera). J. Apic. Res. 48, 215–216. doi: 10.3896/IBRA.1.48.3.11
Ellis, J. D., and Hepburn, H. R. (2006). An ecological digest of the small hive beetle (Aethina tumida) in honey bee colonies (Apis mellifera). Insect. Soc. 53, 8–19. doi: 10.1007/s00040-005-0851-8
Elzen, P. J., Westervelt, D., Causey, D., Ellis, J., Hepburn, H. R., and Neumann, P. (2002). Method of application of tylosin, an antibiotic for American foulbrood control, with effects on small hive beetle (Coleoptera: Nitidulidae) populations. J. Econ. Ent. 95, 1119–1122. doi: 10.1603/0022-0493-95.6.1119
Fleming, J. C., Schmehl, D. R., and Ellis, J. D. (2015). Characterizing the impact of commercial pollen substitute diets on the level of Nosema spp. in honey bees (Apis mellifera L.). PLoS ONE 10:e0132014. doi: 10.1371/journal.pone.0132014
Goodwin, R. M., Houten, A. T., and Perry, J. H. (1994). Effect of feeding pollen substitutes to honey bee colonies used for kiwifruit pollination and honey production. N. Z. J. Crop Hortic. Sci. 22, 459–462. doi: 10.1080/01140671.1994.9513858
Hagedorn, H. H., and Moeller, F. E. (1968). Effect of the age of pollen used in pollen supplements on their nutritive value for the honeybee. I. effect on thoracic weight, development of hypopharyngeal glands, and brood rearing. J. Apic. Res. 7, 89–95. doi: 10.1080/00218839.1968.11100195
Haydak, M. H. (1949). Causes of deficiency of soybean flour as a pollen substitute for honeybees. J. Econ. Entomol. 42, 573–579. doi: 10.1093/jee/42.4.573
Haydak, M. H. (1960). Vitamin content of royal jelly from honey bee colonies fed normal diet and from those fed pollen substitutes. Ann. Entomol. Soc. Am. 53, 695–695. doi: 10.1093/aesa/53.5.695
Haydak, M. H., and Tanquary, M. C. (1943). Pollen and pollen substitutes in the nutrition of the honeybee. Min. Tech. Bull. 160, 1–23.
Herbert, E. W., and Shimanuki, H. (1978). Chemical composition and nutritive value of bee-collected and bee-stored pollen. Apidologie 9, 33–40. doi: 10.1051/apido:19780103
Herbert, E. W., Shimanuki, H., and Shasha, B. S. (1980a). Brood rearing and food consumption by honeybee colonies fed pollen substitutes supplemented with starch-encapsulated pollen extracts. J. Apic. Res. 19, 115–118. doi: 10.1080/00218839.1980.11100008
Herbert, E. W., Svoboda, J. A., Thompson, M. J., and Shimanuki, H. (1980b). Sterol utilization in honey bees fed a synthetic diet: effects on brood rearing. J. Insect Physiol. 26, 287–289. doi: 10.1016/0022-1910(80)90135-3
Herbert, E. W. J., and Shimanuki, H. (1982). Effect of population density and available diet on the rate of brood rearing by honey bees offered a pollen substitute. Apidologie 13, 21–28. doi: 10.1051/apido:19820103
Hristov, P., Shumkova, R., Palova, N., and Neov, B. (2020). Factors associated with honey bee colony losses: a mini-review. Vet. Sci. 7:166. doi: 10.3390/vetsci7040166
Kumar, R., Mishra, R. C., and Agrawal, O. P. (2013). Effect of feeding artificial diets on honey bees during dearth period under Panchkula (Haryana) conditions. J. Entomol. Res. 37, 41–45.
Lamontagne-Drolet, M., Samson-Robert, O., Giovenazzo, P., and Fournier, V. (2019). The impacts of two protein supplements on commercial honey bee (Apis mellifera L.) colonies. J. Apic. Res. 58, 800-813. doi: 10.1080/00218839.2019.1644938
Li, C., Xu, B., Wang, Y., Feng, Q., and Yang, W. (2012). Effects of dietary crude protein levels on development, antioxidant status, and total midgut protease activity of honey bee (Apis mellifera ligustica). Apidologie 43, 576–586. doi: 10.1007/s13592-012-0126-0
Li, C., Xu, B., Wang, Y., Yang, Z., and Yang, W. (2014). Protein content in larval diet affects adult longevity and antioxidant gene expression in honey bee workers. Entomol. Exp. Appl. 151, 19–26. doi: 10.1111/eea.12167
Manning, R. (2018). Artificial feeding of honeybees based on an understanding of nutritional principles. Anim. Prod. Sci. 58, 689–703. doi: 10.1071/AN15814
Manning, R., Rutkay, A., Eaton, L., and Dell, B. (2007). Lipid-enhanced pollen and lipid-reduced flour diets and their effect on the longevity of honey bees (Apis mellifera L.). Aust. J. Entomol. 46, 251–257. doi: 10.1111/j.1440-6055.2007.00598.x
Mattila, H. R., and Otis, G. W. (2006a). Influence of pollen diet in spring on development of honey bee (Hymenoptera: Apidae) colonies. J. Econ. Entomol. 99, 604–613. doi: 10.1093/jee/99.3.604
Mattila, H. R., and Otis, G. W. (2006b). The effects of pollen availability during larval development on the behaviour and physiology of spring-reared honey bee workers. Apidologie 37, 533–546. doi: 10.1051/apido:2006037
Mayack, C., and Naug, D. (2009). Energetic stress in the honeybee Apis mellifera from Nosema ceranae infection. J. Invertebr. Pathol. 100, 185–188. doi: 10.1016/j.jip.2008.12.001
Morais, M. M., Turcatto, A. P., Pereira, R. A., Francoy, T. M., Guidugli-Lazzarini, K. R., Goncalves, L. S., et al. (2013). Protein levels and colony development of Africanized and European honey bees fed natural and artificial diets. Genet. Mol. Res. 12, 6915–6922. doi: 10.4238/2013.December.19.10
Mortensen, A. N., Jack, C. J., Bustamante, T. A., Schmehl, D. R., and Ellis, J. D. (2019). Effects of supplemental pollen feeding on honey bee (Hymenoptera: Apidae) colony strength and Nosema spp. infection. J. Econ. Entomol. 112, 60-66. doi: 10.1093/jee/toy341
Neumann, P., Evans, J. D., Pettis, J. S., Pirk, C. W. W., Schäfer, M. O., Tanner, G., et al. (2013). Standard methods for small hive beetle research. J. Apic. Res. 52, 1-32. doi: 10.3896/IBRA.1.52.4.19
Noordyke, E. R. (2020). Determining the efficacy of pollen substitutes as a management tool for western honey bee (Apis mellifera L.) colonies. (dissertation/Master's thesis), University of Florida, Gainesville, FL, United States.
Noordyke, E. R., van Santen, E., and Ellis, J. D. (2021). Tracing the fate of pollen substitute patties in Western honey bee (Hymenoptera: Apidae) colonies. J. Econ. Entomol. 114, 1421-1430. doi: 10.1093/jee/toab083
Omar, E., Abd-Ella, A. A., Khodairy, M. M., Moosbeckhofer, R., Crailsheim, K., and Brodschneider, R. (2017). Influence of different pollen diets on the development of hypopharyngeal glands and size of acid gland sacs in caged honey bees (Apis mellifera). Apidologie 48, 425–436. doi: 10.1007/s13592-016-0487-x
Otto, C. R. V., Roth, C. L., Carlson, B. L., and Smart, M. D. (2016). Land-use change reduces habitat suitability for supporting managed honey bee colonies in the Northern Great Plains. PNAS 113, 10430–10435. doi: 10.1073/pnas.1603481113
Paiva, J. P. L. M., Esposito, E., Morais Honorato de Souza, G. I., Francoy, T. M., and Morais, M. M. (2019). Effect of ensiling on the quality of protein supplements for honey bees Apis mellifera. Apidologie 50, 414-424. doi: 10.1007/s13592-019-00661-4
Pankiw, T., Sagili, R. R., and Metz, B. N. (2008). Brood pheromone effects on colony protein supplement consumption and growth in the honey bee (Hymenoptera: Apidae) in a subtropical winter climate. J. Econ. Entomol. 101, 1749–1755. doi: 10.1603/0022-0493-101.6.1749
Pernal, S. F., and Currie, R. W. (2000). Pollen quality of fresh and 1-year-old single pollen diets for worker honey bees (Apis mellifera L.). Apidologie 31, 387–409. doi: 10.1051/apido:2000130
Raymann, K., and Moran, N. A. (2018). The role of the gut microbiome in health and disease of adult honey bee workers. Curr. Opin. Insect Sci. 26, 97–104. doi: 10.1016/j.cois.2018.02.012
Ricigliano, V. A., and Simone-Finstrom, M. (2020). Nutritional and prebiotic efficacy of the microalga Arthrospira platensis (spirulina) in honey bees. Apidologie 51, 898-910. doi: 10.1007/s13592-020-00770-5
Rinderer, T. E., Rothenbuhler, W. C., and Gochnauer, T. A. (1974). The influence of pollen on the susceptilbility of honey bee larvae to Bacillus larvae. J. Invertebr. Pathol. 23, 347–350. doi: 10.1016/0022-2011(74)90100-1
Rogala, R., and Szymaś, B. (2004). Nutritional value for bees of pollen substitute enriched with synthetic amino acids part II. Biological methods. J. Apic. Sci. 48, 29–36.
Saffari, A., Kevan, P. G., and Atkinson, J. L. (2010a). Palatability and consumption of patty-formulated pollen and pollen substitutes and their effects on honeybee colony performance. J. Apic. Sci. 54, 63–71.
Saffari, A., Kevan, P. G., and Atkinson, J. L. (2010b). Consumption of three dry pollen substitutes in commercial apiaries. J. Apic. Sci. 54, 13–20.
Sagili, R. R., and Breece, C. R. (2012). Effects of brood pheromone (SuperBoost) on consumption of protein supplement and growth of honey bee (Hymenoptera: Apidae) colonies during fall in a northern temperate climate. J. Econ. Entomol. 105, 1134–1138. doi: 10.1603/EC11437
Schmehl, D. R., Teal, P. E. A., Frazier, J. L., and Grozinger, C. M. (2014). Genomic analysis of the interaction between pesticide exposure and nutrition in honey bees (Apis Mellifera). J. Insect Physiol. 71, 170–190. doi: 10.1016/j.jinsphys.2014.10.002
Schmidt, J. O., and Hanna, A. (2006). Chemical nature of phagostimulants in pollen attractive to honeybees. J. Insect Behav. 19, 521–532. doi: 10.1007/s10905-006-9039-y
Sereia, M. J., and de Toledo, V. A. A. (2013). Quality of royal jelly produced by Africanized honeybees fed a supplemented diet. Food Sci. Technol. 33, 304–309. doi: 10.1590/S0101-20612013005000039
Sihag, R. C., and Gupta, M. (2013). Testing the effects of some pollen substitute diets on colony build up and economics of beekeeping with Apis mellifera L. J. Entomol. 10, 120–135. doi: 10.3923/je.2013.120.135
Sihag, R. C., and Gupta, M. (2011). Development of an artificial pollen substitute/supplement diet to help tide the colonies of honeybee (Apis mellifera L.) over the dearth season. J. Apic. Sci. 55, 15–29.
Simpson, S. J., Raubenheimer, D., and Chambers, P. G. (1995). “The mechanisms of nutritional homeostasis” in Regulatory Mechanisms in Insect Feeding, eds R. F. Chapman, and G. de Boer (Boston, MA: Springer), 251–278. doi: 10.1007/978-1-4615-1775-7_9
Smart, M. D., Otto, C. R. V., and Lundgren, J. G. (2019). Nutritional status of honey bee (Apis mellifera L.) workers across an agricultural land-use gradient. Sci. Rep. 9:16252. doi: 10.1038/s41598-019-52485-y
Somerville, D. (2005). Fat Bee Skinny Bees–A Manual on Honey Bee Nutrition for Beekeepers. Rural Industries Research and Development Corporation.
Standifer, L. N., Moeller, F. E., Kauffeld, N. M., Herbert, E. W. Jr., and Shimanuki, H. (1977). Supplemental Feeding of Honey Bee Colonies. United States Department of Agriculture.
Stuhl, C. J. (2017). Survival and reproduction of small hive beetle (Coleoptera: Nitidulidae) on commercial pollen substitutes. Fla. Entomol. 100, 693–697. doi: 10.1653/024.100.0414
Szymaś, B., and Jedruszuk, A. (2003). The influence of different diets on haemocytes of adult worker honey bees, Apis mellifera. Apidologie 34, 97–102. doi: 10.1051/apido:2003012
Szymaś, B., Langowska, A., and Kazimierczak-Baryczko, M. (2012). Histological structure of the Midgut of honey bees (Apis Mellifera L.) fed pollen substitutes fortified with probiotics. J. Apic. Sci. 56, 5–12. doi: 10.2478/v10289-012-0001-2
Tokarev, Y. S., Huang, W., Solter, L. F., Malysh, J. M., Becnel, J. J., and Vossbrinck, C. R. (2020). A formal redefinition of the genera Nosema and Vairimorpha (Microsporidia: Nosematidae) and reassignment of species based on molecular phylogenetics. J. Invertebr. Pathol. 169:107279. doi: 10.1016/j.jip.2019.107279
Topitzhofer, E., Lucas, H., Chakrabarti, P. C., Breece, Bryant, V., and Sagili, R. (2019). Assessment of pollen diversity available to honey bees (Hymenoptera: Apidae) in major cropping systems during pollination in the western United States. J. Econ. Entomol. 112, 2040–2048. doi: 10.1093/jee/toz168
Watkins de Jong, E., DeGrandi-Hoffman, G., Chen, Y., Graham, H., and Ziolkowski, N. (2019). Effects of diets containing different concentrations of pollen and pollen substitutes on physiology, Nosema burden, and virus titers in the honey bee (Apis mellifera L.). Apidologie 50, 845–858. doi: 10.1007/s13592-019-00695-8
Williams, G. R., Alaux, C., Costa, C., Csaki, T., Doublet, V., Eisenhardt, D., et al. (2013). Standard methods for maintaining adult Apis mellifera in cages under in vitro laboratory conditions. J. Apic. Res. 52, 1-36. doi: 10.3896/IBRA.1.52.1.04
Winston, M. L., Chalmers, W. T., and Lee, P. C. (1983). Effects of two pollen substitutes on brood mortality and length of adult life in the honeybee. J. Apic. Res. 22, 49–52.
Keywords: pollen substitute, pollen supplement, pollen patty, nutrition, health, productivity, honey bee, Apis mellifera
Citation: Noordyke ER and Ellis JD (2021) Reviewing the Efficacy of Pollen Substitutes as a Management Tool for Improving the Health and Productivity of Western Honey Bee (Apis mellifera) Colonies. Front. Sustain. Food Syst. 5:772897. doi: 10.3389/fsufs.2021.772897
Received: 08 September 2021; Accepted: 18 October 2021;
Published: 10 November 2021.
Edited by:
Kimberly Ann Stoner, Connecticut Agricultural Experiment Station, United StatesReviewed by:
Petar Hristov, Bulgarian Academy of Sciences, BulgariaDavid Tarpy, North Carolina State University, United States
Ramesh Sagili, Oregon State University, United States
Copyright © 2021 Noordyke and Ellis. This is an open-access article distributed under the terms of the Creative Commons Attribution License (CC BY). The use, distribution or reproduction in other forums is permitted, provided the original author(s) and the copyright owner(s) are credited and that the original publication in this journal is cited, in accordance with accepted academic practice. No use, distribution or reproduction is permitted which does not comply with these terms.
*Correspondence: Emily R. Noordyke, emilynoordyke@gmail.com