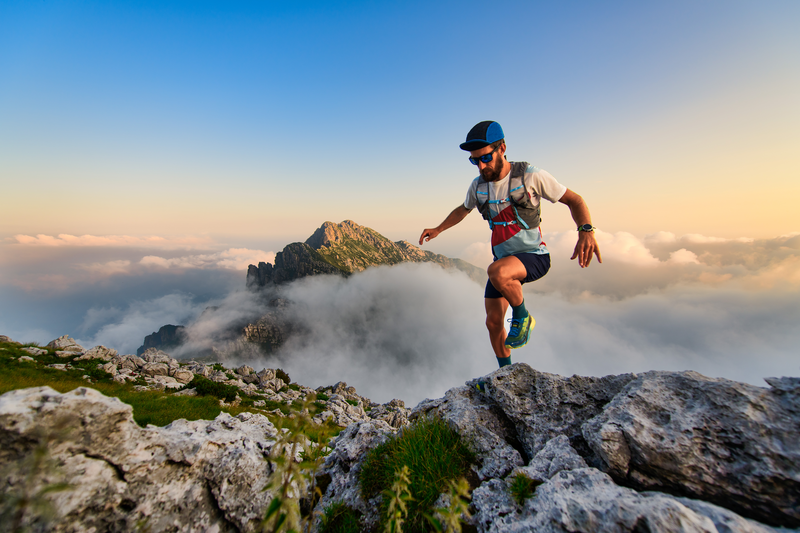
94% of researchers rate our articles as excellent or good
Learn more about the work of our research integrity team to safeguard the quality of each article we publish.
Find out more
ORIGINAL RESEARCH article
Front. Sustain. Food Syst. , 01 February 2022
Sec. Agroecology and Ecosystem Services
Volume 5 - 2021 | https://doi.org/10.3389/fsufs.2021.758724
This article is part of the Research Topic Nitrogen Use To Improve Sustainable Yields in Agricultural Systems View all 11 articles
The Abuja Fertilizer Declaration in 2006 recommended the increase of fertilizer use from the current practice for Sub-Saharan Africa (SSA) to achieve food sufficiency and improve soil fertility status. However, the current recommended rates of fertilizer have not been evaluated for specific crops on their potential to reduce the yield gap and optimize nitrogen use efficiency (NUE). In this study, with nitrogen (N) being a significant yield-determinant nutrient, four N use scenarios were drawn from existing recommendations and were evaluated under field conditions for maize crops in two catchments of the Lake Victoria basin. The scenarios included Business as Usual (BAU, 0 kg N ha−1), 25% of the Abuja declaration (ADS 12.5 kg N ha−1), 50% of the Abuja declaration (ADS 25 kg N ha−1), and Abuja declaration–Abuja scenario (ADS, 50 kg N ha−1). The results revealed that increasing N input levels significantly influenced the growth and yield of maize crops. The ADS scenario recorded the highest grain yield increase (167.39%) in Nyando and 103.25% in Rangwe catchments compared to the BAU scenario. N deficits were observed in all the N use scenarios with a range of −66.6 to −125.7 kg N ha−1 in Nyando and −62.5 to −105.4 kg N ha−1 in Rangwe catchments with the 50% ADS scenario having the highest deficits. The deficits imply that the added N input is insufficient to create an N balance for optimal NUE with consequent high risks of soil N mining. In both catchments, all N use scenarios were within the recommended agro-physiological N efficiency (APEN) level of between 40 and 60 kg kg−1 N. The partial N balance obtained at Nyando (1.56–3.11) and Rangwe (1.10–4.64) was higher than the optimal values, a sign of insufficiency of N inputs and possible risk of soil N depletion in all the scenarios. Our findings conclude that the proposed N rates in the region are still very low for food sufficiency and optimized NUE. Therefore, there is a need to explore other sources of N such as biological N fixation and organic manure and inform policy- and decision-makers to recommend higher rates beyond the “Abuja declaration” with the prospect of reaching target yield and optimizing NUE values based on specific crop recommendations.
Low soil fertility in Sub-Saharan Africa (SSA) is a significant challenge limiting the realization of higher crop productivity among small-scale farmers (Vanlauwe and Giller, 2006; Ten Berge et al., 2019). In most farms, negative nutrient balances have been reported where the use of nutrients has been below 10 kg ha−1, and in most cases, no mineral fertilizer is applied (Chianu et al., 2012; FAO, 2017; Gram et al., 2020) compared to over 100 kg ha−1 in Europe, North America, and China (Rurinda et al., 2020). Nitrogen (N) is a critical element for increased crop production and is a significant yield-determining nutrient in farming systems (Noor et al., 2020; Quemada et al., 2020). Ninety percent of fertilizers applied in Africa are used to supply N, although the current application rates are far below the recommended rates (Thar et al., 2021). Although N is required in adequate quantities to sustain yields, caution should be exercised to avoid excessive application. According to the African Union (2006), agricultural ministers pointed out that increasing fertilizer application rates to 50 kg ha−1 in SSA could be the main solution to lift the low productivity levels of maize. Against this background, with ~90% of the fertilizer used being of N form, assessing its efficiency on soil fertility is critical. According to Richards et al. (2016) and a report by the African Union (2014), the recommended rate of 50 kg ha−1 by the Abuja Declaration on Fertilizer for the African Green Revolution remains a nightmare for many farmers. The low inputs have contributed to the depletion of soil stocks, characterized as “soil mining,” leading to soil fertility losses (Ten Berge et al., 2019; Leitner et al., 2020), implying that the amount of nutrients absorbed/removed by crops is higher than fertilizers applied (Chianu et al., 2012). N depletion rates in SSA have been reported to be more than 100 kg N ha−1 (Akintoye et al., 1999; Nyamangara et al., 2003; Oikeh et al., 2003; Pasley et al., 2020).
Maize remains one of the most cultivated crops due to its essence in the food and livelihoods of the population in SSA (Badu-Apraku and Fakorede, 2017; Ten Berge et al., 2019). However, maize yields in this region are low due mainly to insufficient fertilizer inputs and accessibility of input (Jama et al., 2017; Beesigamukama et al., 2020; Gweyi-Onyango et al., 2021). Existing evidence shows that maize yield is as low as 1.4 t ha−1 against a potential of 4–13 t ha−1 in SSA when proper nutrition and improved varieties are used (Mueller et al., 2012; Tamene et al., 2016). According to Mueller et al. (2012), closing yield gaps in SSA to ~50% of the attainable yields requires addressing the existing nutrient deficiencies, which remains a challenge for many smallholder farmers. As reported by Dzanku et al. (2015), smallholder agriculture in Africa experiences large food crop yield gaps under rainfed conditions. With the anticipated population increase in SSA, approaches are required to reduce the yield gap particularly for cereal crop that forms the most basic meal for every household (Van Ittersum et al., 2016). Small-scale agrarian livelihood is the most dominant production system, contributing to most national-level food production, with a significant farm size being <2 ha (Leitner et al., 2020). Low use N input and small crop land for most rural farmers contribute significantly to large yield gaps. However, increasing N input is linked to increment of yield and minimizes the current gaps and food insecurities. This can be attested from Malawi's experience where maize yield doubled with fertilizers' subsidies that allowed farmers access to N fertilizers and use of improved maize varieties (Folberth et al., 2013; Masso et al., 2017; Katengeza, 2020).
Nitrogen use efficiency (NUE) is defined as a ratio between the amount of N removed with harvest and N applied in the cropping system. It is an established metric used to benchmark the management of N in defined systems (Congreves et al., 2021; Ntinyari et al., 2021). NUE provides information on the relative utilization of applied N to an agricultural production system to either specific plots or farms (Brentrup and Pallière, 2010). The components of NUE that are critical in the analysis of N management are as follows: the partial N budget (PNB) that shows the nutrient recovery efficiency; the agronomic efficiency of N (AEN) shows the measure of crop yield with the amount of N added (Dobermann, 2005); the agro-physiological N efficiency (APEN) shows the economic yield per unit N accumulated from the N fertilizer applied (Dobermann, 2005); and N surplus/deficit that shows a balance between N input and output from the system with positive values indicating surplus and negative values showing N deficits.
Insufficient N inputs in most SSA countries have been linked to NUE values above 100% (Edmonds et al., 2009), compared to 70% in regions with good use of N in cropping systems (Sutton et al., 2013; Masso et al., 2020). The European Union Nitrogen Expert Panel (EUNEP, 2016) described the desired NUE to range between 50 and 90%. NUE levels higher than 90% represent chances of extreme risks of mining soil N stocks (Quemada et al., 2020). Comparatively, global NUE averages approximately 45–50%, indicating that a few countries have achieved a desirable NUE.
According to Elrys et al. (2020), the failure of African countries to achieve a six-fold increase in fertilizer input, as suggested by the Alliance for a Green Revolution in Africa (Trade and Africa, (2018), has led to poor NUE values posing a severe threat toward achieving food sufficiency and environmental sustainability. Therefore, there is a need to adopt better practices to optimize NUE and minimize the risks of excess soil nutrient mining in scenarios with low N inputs (Kuyah et al., 2021). According to Hirel et al. (2011), N fertilizer's in-season application is an essential facet toward improving NUE. Proper timing of N fertilizer application improves synchronization of available N to plants and maximizes uptake and utilization (Yadav et al., 2017; Ullah et al., 2019; Ishfaq et al., 2021). To optimize NUE, farmers are encouraged to apply the 4 R stewardship of nutrient management (i.e., right rate, right source, right timing, and right placement) to increase NUE while minimizing losses and environmental impacts (Davidson et al., 2016; Masso et al., 2017; Ladha et al., 2020; Ntinyari and Gweyi-Onyango, 2021).
Although recommendations by the Abuja declaration have been made to increase fertilizer inputs, their influence on NUE has not been evaluated at the plot level. Besides, most of the studies have relied on model projections in estimating the change in yield over time (Mueller et al., 2012; Leitner et al., 2020). Still, they have neglected key indicators for NUE for major crops within the region. Based on this, scenarios for N use were simulated at field conditions to give insights into possibilities of reducing the yield gap, optimizing NUE, and contributing to farmers' knowledge on improving N management. The scenario's choices were based on the Comprehensive Africa Agriculture Development Programme (CAADP), Abuja declaration of 2006, and Malabo declaration of 2014 aligned with global agriculture and Sustainable Development Goals. The objective of this study was to evaluate the Abuja 2006 declaration by using gradual increases of N as a main inorganic fertilizer in SSA for maize for its effectiveness in reducing yield gaps and enhance achievement of optimal nitrogen use efficiencies. The scenarios described in this study show a projected transition of N inputs uses by the farmers from the current practices. This is one of the first studies to assess the effect of the Abuja fertilizer declaration (50 kg ha−1) on optimization of NUE in yield, agronomic, and environmental sustainability, assuming that farmers will transit gradually from current practices. The results from this study can be a basis for the formulation of new policies and priorities for sustainable N management within the region.
Two field experiments were carried out in two distinct catchments of the Lake Victoria basin, namely, Nyando and Rangwe. Nyando is located in Kisumu County 34.912190°S, −0.148550°E at an elevation of 1,154 m above sea level. The average monthly temperatures are between 24.0 and 25°C. The soils in the study site are Vertisols black cotton soils with shallow depths, high organic matter, and moderate pH levels (Gachene and Kimaru, 2003). The catchment receives cumulative rainfall of 1,350 mm annually. Rangwe is located in Homabay county at 34.573104°S, 0.623583°E with an elevation of 1,166 m above sea level. The average monthly temperatures range between 22.1 and 23.9°C. The soils are Eutric Fluvisol with low organic matter and moderate pH levels. The cumulative rainfall for the catchment is 1,646 mm annually. The analysis of the selected physical–chemical characteristics were done according to Okalebo et al. (2002) (Table 1).
The change in N fertilizer use was assumed to be influenced by implementing various recommendations and policy interventions set aside for the Africa's Green revolution and livelihood transformation through Agriculture (Trade and Africa, 2018).
The first scenario evaluated maize simulation (BAU) representing zero N input (0 kg N ha−1), reflecting the actual farmers' practices in the two catchments. This scenario was guided by the fact that 60% of the farmers in the region do not use N fertilizer input in their maize fields. Therefore, this scenario assumes that the farmers in this category would continue to grow crops without any N inputs over time.
The second scenario represented 25% of the recommended rate by the Abuja declaration of 2006 on the set target of 50 kg ha−1 and recommended by Africa.fertilizer.org. In this scenario, N input was set to 12.5 kg N ha−1, which was the average nutrient application rate by 2015 from Abuja fertilizer declaration in 2006.
Scenario 3 represented 50% of the recommended rate by the Abuja declaration of 2006 on the set target of 50 kg ha−1 of nutrients. The N input for this scenario was 25 kg N ha−1 and represented a double increment and a transition from the 25% ADS scenario that was the base value in the fertilizer declaration summit. This scenario also reflects the current N input rates by some farmers in selected countries in SSA (Sheahan et al., 2014).
This scenario used 50 kg N ha−1, which was adopted as the set target for Abuja fertilizer declaration. Nevertheless, many farmers are still far short of the Abuja fertilizer summit. The scenario represented 100% transition by the farmers to the “summit adopted” rate of N application. This scenario assumes that there will be changes in favor of fertilizer accessibility; hence, farmers will purchase/access and apply the recommended N inputs in maize cropping systems.
Maize (Zea mays L.) seeds of Sc Duma-43 from the Seed Co. (hybrid variety and recommended for the Lake Victoria catchment) were sown in the fields during the cropping season of September 2020–January 2021. A total of 16 plots measuring 5 × 5 m with border widths of 0.5 and 1 m for plots and blocks, respectively, were adopted and arranged in a randomized complete block design (RCBD) with four replications for each scenario. The spacing for the maize plants was 75 × 25 cm. Three plants per hill were planted and thinning was done to 2 seedlings per hill after 2 weeks of germination. Urea, a commonly available source of nitrogen fertilizer in the region, was applied into fields except for control (BAU) and other treats as 12.5 kg N ha−1, 25 kg N ha−1, and 50 kg N ha−1. The N fertilizer was applied in two splits; the first split was at planting, while the second application was during the vegetative stage, which corresponded to 30 days after planting (DAP). During the experiment, the standard agronomic practices of maize crop production, including weeding and pest control, were carried out.
Data on biomass and other growth parameters were collected at three critical stages of maize production: vegetative, which was 30 DAP, tasseling (60 DAP), and physiological maturity (90 DAP). Five plant samples were collected from the experimental plots at vegetative (V6), reproductive (R1), and physiological maturity (R6) harvesting stages. The samples were thoroughly washed in running water to free them from soil and any other surface impurities. The samples were separated into leaves, stems, roots, and grain at harvesting and taken to the laboratory for drying at a temperature of 70°C for 48 h (after achieving constant weight). The dried samples were then ground using a mechanical grinder.
At maturity, yield data were collected from each plot after all the ears had reached physiological maturity. Plants were harvested by cutting at ground level, and ears were threshed. Both grain and stover were air-dried and then oven-dried in the laboratory until a constant moisture content of 12.5% was reached. The yield obtained from the net plot of each N use scenario was determined and extrapolated into tons per hectare (t ha−1).
Soil samples were collected from the two experimental fields at the start of the experiment to analyze the selected chemical and physical compositions of the soils The soils were sampled from depths (0–20 and 20–40 cm) for analysis of total organic N, available N (), and ), organic carbon, available P, pH, bulk density electrical conductivity (EC) soil texture, and exchangeable cation (K and Ca). Electrical conductivity (EC) and pH were determined using extracts 1:2.5 [weight/volume (w/v)] for soil to distilled water. The pH and EC were then read directly using a pH (AD1000, Adwa, Romania) and EC meter (AVI, Labtech, India), respectively (Okalebo et al., 2002). The available N (NH4-N and NO3-N) was extracted from soil using 0.5 M potassium sulfate at a ratio of 1:10 (w/v). The potassium sulfate mixture was shaken for 1 h using an orbital and linear shaker (KOS-3333/KCS-3333, MRC, UK). Filtration of the solution was done using Whatman No. 1 filter paper, and the filtrate was used for further analysis using the colorimetric method at 655 and 419 nm as described by Okalebo et al. (2002). Total N in soil was determined using the Kjeldahl digestion and distillation method. Exchangeable Ca and Mg were determined using Atomic Absorption Spectrometry at 422.7 and 285.2 nm, respectively (iCE 3300 AA system, Thermo Scientific, Shanghai, China), and K was determined using flame photometry. Available phosphorus was analyzed using Bray 2 method as described by Okalebo et al. (2002). Air-dried samples were ground using an analytical mill for N concentration in grain and plant tissues per N use scenario. The N content in the plant tissue (grain and stover) will be determined by Kjeldahl digestion procedure (Baker and Thompson, 1992). A sample of 0.3 g of milled plant material was put in a digestion tube and digestion mixture, 1% NaOH was added, and total N was determined through distillation. To determine nitrogen partitioned to roots, stem, leaves, and grain, the N content obtained was divided by the total amount of N in the whole plant and later converted to a percentage by multiplying by 100.
The calculated N use efficiency indicators, according to Fixen et al. (2015), were as follows:
a. Partial nutrient balance (PNB) was determined to show nutrient recovery efficiency, usually expressed as nutrient output per unit of nutrient input (a ratio of “removal to use”) (Equation 1).
b. Agro-physiological N efficiency (APEN kg grain kg−1) was calculated to determine the economic yield per unit N accumulated from each fertilizer treatment (Equation 2).
c. Agronomic efficiency of N (AEN kg grain (kg N)−1) was calculated to show the increase in yield per the unit of N increase applied (Equation 3).
d. N surplus/deficit was calculated to show the balance between the applied N and the crop N removal (Equation 4).
The collected data were checked for normality using the Shapiro–Wilk test. Plant biomass, N grain yield, NUE, and total nitrogen crop production were analyzed using a one-way analysis of variance test using R software version 4.1.0. Computation of least squares means was done using “lsmeans” package, followed by mean separation using adjusted Tukey's method implemented using “cld” function from the “multicompView” package. Distribution of means for grain and stover yield was done using the ggplot command from the ggpubr package (R Core Team, 2019).
Biomass accumulation varied significantly (p ≤ 0.05) among the N use scenarios during vegetative (V6), reproductive (R1), and physiological maturity (R6) stages of maize production (Table 2). There was a significant increase in biomass accumulation from vegetative (V6), reproductive (R1), and maturity (R6) in the two catchment areas (Table 2), with ADS (50 kg N ha−1) having the highest biomass accumulation of 0.72–10.42 t ha−1 in Nyando and 0.42–8.62 t ha−1 in Rangwe across all the three phenological stages.
Table 2. Biomass accumulation of maize as influenced by various N use scenarios during maize phenological stages.
The different N use scenarios showed significant differences (p < 0.001) in maize grain yield in the two catchments (Figure 1). A positive trend on grain yield increase with increasing N application rates was observed. In Nyando, a significant (p < 0.001) difference was observed across the scenarios, with ADS 50 kg N ha−1 having the highest yield of 6.15 t ha−1, which was 167.39% higher compared with the BAU (0 kg N ha−1) (Figure 1A).
Figure 1. Box plots of maize grain yield (A for Nyando and B for Rangwe) influenced by N use scenarios. Boxes and whiskers show 5 and 95% percentiles, boxes denote 25% (Q1) and 75% (Q3) quartiles, the line in the middle is the median, while single dots indicate outlying values.
In Rangwe, a similar trend of maize yield was observed with ADS at 50 kg N ha−1, recording the yield of 5.00 t ha−1, which was 103.25% higher than the BAU (0 kg N ha−1). However, the yield differences were not significantly different between AS and the other two N scenarios (i.e., 25 and 50% ADS) in Rangwe. The regression analyses (Figure 2A) showed that grain yield increased with N rates, and the response assumed a polynomial function, with R2 values of 0.93 and 0.98 for Nyando and Rangwe, respectively. However, in Rangwe (Figure 2B), the curve seems to plateau, which could result from other factors, including calcium and potassium, which were relatively low in the site, hence affecting N in the test variety. In all the N use scenarios, in both catchments, the highest N concentration was partitioned to the grain, representing 39.01–42.07 and 38.62–41.09% of the entire plant uptake in both Nyando and Rangwe catchment areas (Figures 3, 4).
Figure 2. Maize grain yield fertilizer as a polynomial function to N use scenarios in two catchments (A) Nyando and (B) Rangwe.
Figure 3. Nitrogen partitioning as influenced by nitrogen use scenario (A) for BAU, (B) for 25% ADS, (C) for 50% ADS, and (D) for Abuja declaration scenario (ADS) in the Rangwe catchment.
Figure 4. Nitrogen partitioning as influenced by nitrogen use scenario (A) for BAU, (B) for 25% ADS, (C) for 50% ADS, and (D) for Abuja declaration scenario (ADS) in the Nyando catchment.
The results indicate that ~40% of N uptake was removed from the field with the grain harvested for all nitrogen use scenarios. The lowest partitioning of N concentration was observed in the roots with mean percentage ranges of 28.19–31.19 and 28.41–29.85% in Rangwe and Nyando, respectively (Figure 4).
Agro-physiological nitrogen efficiency (APEN) was not significantly (p < 0.05) different among the N use scenarios in the two catchments. However, there was a clear trend with the highest N inputs (ADS 50 kg N ha−1) having higher APEN values. The lack of differences could be influenced by the N scenario adopted in this study being too low to affect maize grain significantly. Moreover, degraded soils could have contributed to the lack of differences and N inputs being too low. The ADS scenario at 50 kg N ha−1 recorded the highest APEN, with a mean value of 57.60 kg grain kg−1 N in Rangwe and 51.7 kg grain kg−1 in Nyando catchment. In both catchments, all N use scenarios achieved the recommended APEN level of between 40 and 60 kg grain kg−1 N (Table 3).
Table 3. Effects of N use scenario fertilizers on nitrogen use efficiencies and partial N balance maize.
N surplus/deficit of maize varied significantly (p < 0.001) across all the scenarios in the two catchments evaluated (Table 3). The 50% ADS (25 kg N ha−1) had the largest N deficit of −125 and −105 kg N ha−1 in Nyando and Rangwe catchments. This indicates that the addition of more N input could optimize N and reduce the negative imbalances in the soils; however, the 50 kg N ha−1 suggested at the Abuja fertilizer summit is still very low for maize crop to contribute to the optimization of overall NUE.
The PNB of maize varied significantly (p < 0.001) across all the N use scenarios evaluated in the two catchments (Table 3). The 25% ADS (12.5 kg N ha−1) had the highest values of PNB of 3.11 kg grain N (kg N)−1 in Nyando, while 50% ADS (25 kg N ha−1) and AS (50 kg N ha−1) had 2.39 and 1.56 kg grain N (kg N)−1 PNB, respectively.
A similar reducing trend of PNB with an increased N was also observed at Rangwe. The declining trend of PNB with N was linear (Figure 5, Table 3). Moreover, ADS (50 kg N ha−1) fertilizer application resulted in a near-optimal PNB of 1.10 kg grain N (kg N)−1 (Figure 5). The higher N application rate resulted in values closer to optimal PNB, while the low N rates had higher values, far from the optimal range. Figure 6 confirmed a strong association between nitrogen scenarios and partial N balance for both Nyando and Rangwe study sites. The increase of N application rates as defined by the N use scenarios resulted in lowering partial N balance with R2 = 0.86 and 0.97 for Nyando and Rangwe, respectively (Figure 5).
Figure 5. Relationship between nitrogen use scenarios and partial N balance for two study catchments (A) for Nyando and (B) for Rangwe.
Figure 6. Graphical representation of partial N balance across the N use scenarios and the target values (A) for Nyando catchment and (B) for the Rangwe catchment.
AEN had significant differences across the N use scenarios in the two catchments. AEN depicts the efficiency of N recovery from the applied fertilizers in grain yield. In all the N use scenarios, the AEN obtained was greater than the common values of 10–30 kg grain (kg N)−1 (Table 4).
The values obtained were between 81.7 and 118.7 kg grain (kg N)−1 in Nyando and between 45.4 and 154.3 kg grain (kg N)−1 in the Rangwe catchment, which are higher values than 30 kg grain (kg N)−1, implying that the amount of N fertilizer supplied was very low to optimize AEN.
PNB that expressed the nutrient output per unit of nutrient input varied significantly across the scenarios. The values observed in this study were above the typically optimal range that indicates insufficient or low N level of the applied fertilizer. The PNB of 1.56–3.11 and 1.10–4.64 kg grain (kg N)−1 (Table 3) showed that the amount of N used was not sufficient to supply the required quantity of N to optimize NUE, hence a need for an increased application. Increasing the fertilizer rate from the current ADS scenario could lead to more sustainable PNB in the region, a fact that is supported by the positive regression in Figure 5. With no plateaus observed in the regression lines, it may be an indication that increasing more N for the two regions would result in desirable PNB values (lower than <1) (Figure 6). Besides, the high PNB values >1 imply that more nutrients were being removed from the plot than what was applied; a situation of soil N mining. Therefore, there is lack of a mass balance between N used and N removed in the crop, with precise/optimal ranges shown in Figure 6.
Negative values of N balance observed in all the scenarios in the two catchments (Table 3) implied higher crop removal of N compared to N input, a process that is likely to contribute to extreme depletion of the soil N status. The N deficits indicate that even with the implementation of the Abuja recommendation, the soil fertility will still decline due to continuous mining. The current findings agree with those of Snyder and Bruulsema (2007), who suggested that a PNB value close to 1 is an indicator of the nutrient's mass balance and higher than 1 shows extreme deficits in the systems. However, this was not achieved in our case, and this could probably mean more N input needs to be added to correct the existing deficiencies of mineral N inputs. Similar results of higher PNB >1 were also reported by Fixen et al. (2015) in the global PNB analysis, with SSA having the highest values. APEN of maize across the scenarios varied in the two catchments expressing the plants' ability to transform nutrients applied into economic yield (Table 3). The increasing trend in APEN values obtained in this study could suggest that an additional supply of more N inputs into the soils would lead to a positive contribution of the maize crop to transform the acquired N into economic yield. Therefore, achieving an optimal NUE for maize crops requires increment of N inputs as confirmed in this study and also agrees with the projection's analysis for Elrys et al. (2020) on Africa achieving food sufficiency in 2050. The APEN values for ADS (50 kg N ha−1) results in the Rangwe catchment agreed with Fixen et al. (2015), who reported APEN optimal values of 40–60 kg grain kg−1. The results are also supported by the findings of Snyder and Bruulsema (2007), who suggested that APEN values >50 kg/kg could be obtained in low N use in some of the properly managed systems.
Our results on AEN showed that by increasing the fertilization rate to 50 kg ha−1 as the recommendation in the Abuja Fertilizer summit, there is a possibility of optimizing AEN (Table 4), particularly for the Rangwe site though the optimal range was not achieved, and there was a significant milestone toward enhanced insights into the sustainability of the agricultural system. The decreasing trend of AEN with increase in N fertilizer application rates indicates that with more N, there are possibilities of achieving an optimal value of AEN for cereal crops between 10 and 30 kg grain (kg N)−1. The AEN values >30 kg grain (kg N)−1 as reported by Vanlauwe et al. (2011) demonstrated that lower N rates resulted in higher AEN in the African context, which strongly agrees with the findings of our current study. In addition to increasing N fertilizer input, hybrid maize varieties are also recommended to optimize AEN for the region. Narrowing the agronomic use efficiency gap for the region can be achieved by increasing addition of N input coupled with improved management practices (Kuyah et al., 2021). According to Ahrens et al. (2010), higher values of AEN result from fields with depleted soil N pools and are due to less fertilization, which is an implication that the current study did not achieve adequate N input to obtain optimized AEN values. In addition, there is a need to understand other factors contributing to high AEN beyond the optimal values at the farm level as a way of addressing the existing gap for example by including improving fertilization (Ahrens et al., 2010).
The results indicate that more biomass could be achieved at higher N application. The findings also imply that increasing N fertilization reflected in the scenarios adopted in this study could result in more grain yield. Thus, this confirms that farmers need to change their current practices of little (or lack of) fertilizer application to more improved practices for higher yields to achieve food sufficiency and reduce the yield gap. Although the ADS of 50 kg ha−1 results in incremental grain yield, exploring more alternative sources like animal manure and biological nitrogen fixation may be critical for the region for more sustainable production. These results are in concurrence with findings of others (Abbasi et al., 2013; Chen et al., 2017; Hammad et al., 2017; Srivastava et al., 2018) who reported an increasing trend on yield and growth under influence of N application. The lower biomass accumulation at the vegetative stage reported in our study agrees with Mueller and Vyn (2018), who associated the low dry weight to reduced N uptake at vegetative as compared to silking and tasseling stages. Sen et al. (2016) also reported reduced biomass accumulation in maize crops grown in low N status compared to those supplied with N fertilizers. The higher biomass accumulation at the tasseling stage could be due to an increase in soil N status upon the second split application of the slow-release urea at the vegetative stage and the increased demand of N by the plants stage. Moreover, Wang et al. (2017) and Nasielski and Deen (2019) also reported a higher biomass accumulation of maize at reproductive stages under different N applications.
The increase in yield without any plateaus in the regression lines signifies the need for more N to achieve food sufficiency. However, in Rangwe (Figure 2B), the curve seems to plateau, which could result from other factors, including calcium (Ca) and potassium (K) that were relatively low in the site, hence affecting N in the test variety. The significant increase in grain yield with N rates confirmed the potential of meeting the food needs and reducing the yield gap if more N fertilizers were used (Pasley et al., 2019). In addition, the lack of a plateau in the yield response model indicates that increasing N fertilizers to 50 kg N ha−1 could not be optimum, specifically for soils reported to have negative N balances like the case of SSA (Figure 2A). The variety (Sc Duma-43) used could also be more effective in scavenging for the available nutrients to promote growth and consequent positive increment in yield. Besides, the variety could be more effectively utilizing N use effectively although the rates used are lower to match the need for the crop. This may be a pointer that the rates used by the farmers are well below the plants' demands, which partially agrees with the low NUE in these sites (Table 3).
With optimal N management through proper application timing, specifically for slow-release fertilizers, there is a higher chance of obtaining a significant increase in yield (Grant et al., 2012; Davies et al., 2020). Although increasing application of N fertilizers may have a positive increase in both grain and stover yields, it may be difficult for some farmers in SSA due to financial constraints and therefore need for exploring alternative sources of N (IFDC, 2003; Pasley et al., 2019; Elrys et al., 2020). However, this can be made possible by increasing availability and subsidizing the cost of fertilizers, and exploring more organic sources of N. Therefore, policy instruments, including extension services, are critical for the region to offer technical efficiency in reducing the yield gap. This can be achieved by addressing N inputs management, including the timing for application for maximum as knowledge is limited (van Dijk et al., 2020).
The partitioning of about 50% of nitrogen into the root and the straw is an indicator practice of N recycling specifically for farms where plowing is done to incorporate both the straw and the roots in the soils after harvest (Figures 3, 4). These findings can be used to guide policy and decision-making on straw management as a way of improving N management sustainability. Enlightening farmers through local extension services on the benefits of incorporating straws into the soils other than burning or feeding to livestock would be a milestone toward increasing nitrogen recycling into the cropping systems for the region.
The higher concentration of N in the grain is an indicator of N recovery from the cropping systems and the proportion of N that is exported from the field. The findings agree with Ning et al. (2017), who reported a significant decrease in N concentration in the stover and a higher increase of N concentration in the grain of maize both under low and high nitrogen inputs.
This is the first study to assess the Abuja 2006 fertilizer summit declaration from an African perspective on increasing fertilizer input, NUE optimization, and agronomic performance at a plot level and for specific crops. We conclude that the suggested fertilizer increment to 50 kg ha−1 as spelt out in the Abuja declaration will slightly improve the growth and yield of maize, but is not sufficient to overcome the soil fertility decline, compared to other regions with plausible nitrogen management strategies coupled with strong policies. Negative N balances were also evident from this analysis, an indicator of higher N removal than the N input, indicating the presence of low N status that leads to soil N mining and degradation of the overall fertility and quality of the soil. In addition, the PNB showed increased soil N deficits in systems with values >1, an implication that more balanced N inputs are essential. Hence, policies should target higher N fertilizer levels to reduce the yield gap, optimizing the current NUE to a sustainable range. With the unlikelihood of most farmers achieving the Abuja recommendation rate, we recommend to explore other complementary sources of N such as animal manure and biological nitrogen fixation to improve the present scenario of low inputs into cropping systems within the region. In addition, we also recommended development of specific crop optimum rates as a basket of options and also take into consideration the socio-economic context of the smallholder farmers. Therefore, the findings of this study can be helpful in decision-making and policymaking to formulate new targets for fertilizers, particularly N input above 50 kg N ha−1, to optimize NUE and reduce the yield gap for sustainability, and also focus more on integrated soil fertility management as a package for nutrient management in systems.
The original contributions presented in the study are included in the article/Supplementary Material, further inquiries can be directed to the corresponding author.
CM was the source of idea. MG, JG-O, JM, and GN reviewed the methodologies. NW collected the data, performed statistical analysis, and wrote up the first manuscript. All authors reviewed the manuscript, provided input and suggestions in the text, and approved the submitted version of the manuscript.
The authors wish to acknowledge toward an International Nitrogen Management System (INMS) project through the International Institute of Tropical Agriculture (IITA) for providing financial support for this study.
The authors declare that the research was conducted in the absence of any commercial or financial relationships that could be construed as a potential conflict of interest.
All claims expressed in this article are solely those of the authors and do not necessarily represent those of their affiliated organizations, or those of the publisher, the editors and the reviewers. Any product that may be evaluated in this article, or claim that may be made by its manufacturer, is not guaranteed or endorsed by the publisher.
We would like to thank the International Institute of Tropical Agriculture (IITA) for facilitating this study and the Department of Agricultural Science and Technology in Kenyatta University for providing laboratory facilities.
The Supplementary Material for this article can be found online at: https://www.frontiersin.org/articles/10.3389/fsufs.2021.758724/full#supplementary-material
Abbasi, M. K., Tahir, M. M., and Rahim, N. (2013). Effect of N fertilizer source and timing on yield and N use efficiency of rainfed maize (Zea mays L.) in Kashmir–Pakistan. Geoderma 195, 87–93. doi: 10.1016/j.geoderma.2012.11.013
African Union (2006). Abuja Declaration on Fertilizer for the African Green Revolution. Declaration of the African Union Special Summit of the Heads of State and Government. Abuja: African Union.
African Union (2014). Implementation Strategy and Roadmap to Achieve the 2025 Vision on CAADP. Addis Ababa: African Union.
Ahrens, T. D., Lobell, D. B., Ortiz-Monasterio, J. I., Li, Y., and Matson, P. A. (2010). Narrowing the agronomic yield gap with improved nitrogen use efficiency: a modeling approach. Ecol. Appl. 20, 91–100. doi: 10.1890/08-0611.1
Akintoye, H. A., Kling, J. G., and Lucas, E. O. (1999). N-use efficiency of single, double, and synthetic maize lines grown at four N levels in three ecological zones of West Africa. Field Crops Res. 60, 189–199. doi: 10.1016/S0378-4290(98)00122-1
Badu-Apraku, B., and Fakorede, M. A. B. (2017). “Maize in Sub-Saharan Africa: importance and production constraints,” in Advances in Genetic Enhancement of Early and Extra-Early Maize for Sub-Saharan Africa (Cham: Springer), 3–10. doi: 10.1007/978-3-319-64852-1_20
Baker, W. H., and Thompson, T. L. (1992). “Determination of total nitrogen in plant samples by Kjeldahl,” in Plant Analysis Reference Procedures for the Southern Region of the United States, Vol. 368, ed O. Plank (Athens, GA: University of Georgia), 13–16.
Beesigamukama, D., Mochoge, B., Korir, N., Musyoka, M. W., Fiaboe, K. K., Nakimbugwe, D., et al. (2020). Nitrogen fertilizer equivalence of black soldier fly frass fertilizer and synchrony of nitrogen mineralization for maize production. Agronomy 10:1395. doi: 10.3390/agronomy10091395
Brentrup, F., and Pallière, C. (2010). “Nitrogen use efficiency as an agro-environmental indicator,” in Proceedings of the OECD Workshop on Agrienvironmental Indicators. (Leysin). 23–26.
Chen, P., Du, Q., Liu, X., Zhou, L. I., Hussain, S., Lei, L. U., et al. (2017). Effects of reduced nitrogen inputs on crop yield and nitrogen use efficiency in a long-term maize-soybean relay strip intercropping system. PLoS ONE 12:e0184503. doi: 10.1371/journal.pone.0184503
Chianu, J. N., Chianu, J. N., and Mairura, F. (2012). Mineral fertilizers in the farming systems of sub-Saharan Africa. A review. Agron. Sustainable Dev. 32, 545–566. doi: 10.1007/s13593-011-0050-0
Congreves, K. A., Otchere, O., Ferland, D., Farzadfar, S., Williams, S., and Arcand, M. M. (2021). Nitrogen use efficiency definitions of today and tomorrow. Front. Plant Sci. 12:637108. doi: 10.3389/fpls.2021.637108
Davidson, E. A., Nifong, R. L., Ferguson, R. B., Palm, C., Osmond, D. L., and Baron, J. S. (2016). Nutrients in the nexus. J. Environ. Stud. Sci. 6, 25–38. doi: 10.1007/s13412-016-0364-y
Davies, B., Coulter, J. A., and Pagliari, P. H. (2020). Timing and rate of nitrogen fertilization influence maize yield and nitrogen use efficiency. PLoS ONE 15:e0233674. doi: 10.1371/journal.pone.0233674
Dobermann, A. R. (2005). Nitrogen Use Efficiency-State of the Art. Lincoln, NE: Agronomy and Horticulture, Faculty Publications. 316.
Dzanku, F. M., Jirström, M., and Marstorp, H. (2015). Yield gap-based poverty gaps in rural Sub-Saharan Africa. World Dev. 67, 336–362. doi: 10.1016/j.worlddev.2014.10.030
Edmonds, D. E., Abreu, S. L., West, A., Caasi, D. R., Conley, T. O., Daft, M. C., et al. (2009). Cereal nitrogen use efficiency in sub–Saharan Africa. J. Plant Nutr. 32, 2107–2122. doi: 10.1080/01904160903308184
Elrys, A. S., Metwally, M. S., Raza, S., Alnaimy, M. A., Shaheen, S. M., Chen, Z., et al. (2020). How much nitrogen does Africa need to feed itself by 2050? J. Environ. Manag. 268, 110488. doi: 10.1016/j.jenvman.2020.110488
EUNEP (2016). EUNEP, Nitrogen Use Efficiency (NUE) - Guidance Document for Assessing NUE at Farm Level. Available online at: http://www.eunep.com/wp-content/uploads/2019/09/NUE-Guidance-Document.pdf (accessed December 11, 2021).
FAO (2017). The State of Food and Agriculture. Leveraging Food Systems for Inclusive Rural Transformation. Rome: FAO Publishing Group.
Fixen, P., Brentrup, F., Bruulsema, T., Garcia, F., Norton, R., and Zingore, S. (2015). “Nutrient/fertilizer use efficiency: measurement, current situation, and trends,” in Managing Water and Fertilizer for Sustainable Agricultural Intensification, eds P. Drechsel, P. Heffer, H. Magen, R. Mikkelsen, D. Wichelns (Paris: International Fertilizer Industry Association (IFA); International Water Management Institute (IWMI); International Plant Nutrition Institute (IPNI), and International Potash Institute (IPI)), 270.
Folberth, C., Yang, H., Gaiser, T., Abbaspour, K. C., and Schulin, R. (2013). Modeling maize yield responses to improvement in nutrient, water, and cultivar inputs in sub-Saharan Africa. Agricu. Syst. 119, 22–34. doi: 10.1016/j.agsy.2013.04.002
Gachene, C. K., and Kimaru, G., . (2003). Soil Fertility and Land Productivity: A Guide for Extension Workers in the Eastern Africa Region (No. 30). Nairobi: Regional Land Management Unit.
Gram, G., Roobroeck, D., Pypers, P., Six, J., Merckx, R., and Vanlauwe, B. (2020). Combining organic and mineral fertilizers as a climate-smart integrated soil fertility management practice in sub-Saharan Africa: a meta-analysis. PLoS ONE 15:e0239552. doi: 10.1371/journal.pone.0239552
Grant, C. A., Wu, R., Selles, F., Harker, K. N., Clayton, G. W., Bittman, S., et al. (2012). Crop yield and nitrogen concentration with controlled release urea and split applications of nitrogen as compared to non-coated urea applied at seeding. Field Crops Res. 127, 170–180. doi: 10.1016/j.fcr.2011.11.002
Gweyi-Onyango, J. P., Ntinyari, W., Egesa, A. O., Mose, R., Njinju, S., Giweta, M., et al. (2021). Differences in seasons and rice varieties provide opportunities for improving Nitrogen Use Efficiency and management in irrigated rice in Kenya. Environ. Res. Lett. 16:ac03dd. doi: 10.1088/1748-9326/ac03dd
Hammad, H. M., Farhad, W., Abbas, F., Fahad, S., Saeed, S., Nasim, W., et al. (2017). Maize plant nitrogen uptake dynamics at limited irrigation water and nitrogen. Environ. Sci. Pollut. Res. 24, 2549–2557. doi: 10.1007/s11356-016-8031-0
Hirel, B., Tétu, T., Lea, P. J., and Dubois, F. (2011). Improving nitrogen use efficiency in crops for sustainable agriculture. Sustainability 3, 1452–1485. doi: 10.3390/su3091452
IFDC (2003). Input Subsidies and Agricultural Development: Issues and Options for Developing and Transitional Economies, Paper Series P-29. IFDC, Muscle Shoals, Alabama, United States. p. 35. Available online at: https://www.inter-reseaux.org/wp-content/uploads/15_Bumb_et_al-Input_Subsidies_and_Agric_Dev.pdf
Ishfaq, M., Akbar, N., Zulfiqar, U., Ali, N., Jabran, K., Nawaz, M., et al. (2021). Influence of nitrogen fertilization pattern on productivity, nitrogen use efficiencies, and profitability in different rice production systems. J. Soil Sci. Plant Nutr. 21, 145–161. doi: 10.1007/s42729-020-00349-0
Jama, B., Kimani, D., Harawa, R., Mavuthu, A. K., and Sileshi, G. W. (2017). Maize yield response, nitrogen use efficiency and financial returns to fertilizer on smallholder farms in southern Africa. Food Secur. 9, 577–593. doi: 10.1007/s12571-017-0674-2
Katengeza, S. P. (2020). “Impact of farm input subsidies vis-à-vis climate-smart technologies on maize productivity: a tale of smallholder farmers in Malawi,” in Climate Impacts on Agricultural and Natural Resource Sustainability in Africa, eds B. R. Singh, A. Safalaoh, N. Y. Amuri, L. O. Eik, B. K. Sitaula, and R. Lal (Cham: Springer), 549–567. doi: 10.1007/978-3-030-37537-9_31
Kuyah, S., Sileshi, G. W., Nkurunziza, L., Chirinda, N., Ndayisaba, P. C., Dimobe, K., et al. (2021). Innovative agronomic practices for sustainable intensification in sub-Saharan Africa. A review. Agron. Sustain. Dev. 41, 1–21. doi: 10.1007/s13593-021-00673-4
Ladha, J. K., Jat, M. L., Stirling, C. M., Chakraborty, D., Pradhan, P., Krupnik, T. J., et al. (2020). Achieving the sustainable development goals in agriculture: the crucial role of nitrogen in cereal-based systems. Adva. Agron. 163, 39–116. doi: 10.1016/bs.agron.2020.05.006
Leitner, S., Pelster, D. E., Werner, C., Merbold, L., Baggs, E. M., Mapanda, F., et al. (2020). Closing maize yield gaps in sub-Saharan Africa will boost soil N2O emissions. Curr. Opin. Environ. Sustain. 47, 95–105. doi: 10.1016/j.cosust.2020.08.018
Masso, C., Baijukya, F., Ebanyat, P., Bouaziz, S., Wendt, J., Bekunda, M., et al. (2017). Dilemma of nitrogen management for future food security in sub-Saharan Africa–a review. Soil Res. 55, 425–434. doi: 10.1071/SR16332
Masso, C., Bekunda, M., and Mngube, F. M. (2020). “The INI African regional nitrogen centre: challenges and opportunities in Africa,” in Just Enough Nitrogen (Cham: Springer), 457–466. doi: 10.1007/978-3-030-58065-0_31
Mueller, N. D., Gerber, J. S., Johnston, M., Ray, D. K., Ramankutty, N., and Foley, J. A. (2012). Closing yield gaps through nutrient and water management. Nature 490, 254–257. doi: 10.1038/nature11420
Mueller, S. M., and Vyn, T. J. (2018). Physiological constraints to realizing maize grain yield recovery with silking-stage nitrogen fertilizer applications. Field Crops Res. 228, 102–109. doi: 10.1016/j.fcr.2018.08.025
Nasielski, J., and Deen, B. (2019). Nitrogen applications made close to silking: Implications for yield formation in maize. Field Crops Res. 243:107621. doi: 10.1016/j.fcr.2019.107621
Ning, P., Fritschi, F. B., and Li, C. (2017). Temporal dynamics of post-silking nitrogen fluxes and their effects on grain yield in maize under low to high nitrogen inputs. Field Crops Res. 204, 249–259. doi: 10.1016/j.fcr.2017.01.022
Noor, M. A., Nawaz, M. M., ul Hassan, M., Sher, A., Shah, T., Abrar, M. M., et al. (2020). “Small farmers and sustainable N and P management: Implications and potential under changing climate,” in Carbon and Nitrogen Cycling in Soil (Singapore: Springer), 185–219. doi: 10.1007/978-981-13-7264-3_6
Ntinyari, W., and Gweyi-Onyango, J. P. (2021). “Greenhouse gases emissions in agricultural systems and climate change effects in Sub-Saharan Africa,” in African Handbook of Climate Change Adaptation (Cham: Springer), 1081–1105. doi: 10.1007/978-3-030-45106-6_43
Ntinyari, W., Gweyi-Onyango, J. P., Giweta, M., Mochoge, B., Mutegi, J., Nziguheba, G., et al. (2021). Nitrogen use efficiency trends for sustainable crop productivity in Lake Victoria basin: smallholder farmers' perspectives on nitrogen cycling. Environ. Res. Commun. (in press). doi: 10.1088/2515-7620/ac40f2
Nyamangara, J., Piha, M. I., and Giller, K. E. (2003). Effect of combined cattle manure and mineral nitrogen on maize N uptake and grain yield. Afr. Crop Sci. J. 11, 389–300. doi: 10.4314/acsj.v11i4.27579
Oikeh, S. O., Carsky, R. J., Kling, J. G., Chude, V. O., and Horst, W. J. (2003). Differential N uptake by maize cultivars and soil nitrate dynamics under N fertilization in West Africa. Agric. Ecosyst. Environ. 100, 181–191. doi: 10.1016/S0167-8809(03)00194-4
Okalebo, J. R., Gathua, K. W., and Woomer, P. L. (2002). Laboratory Methods of Soil and Water Analysis: A Working Manual. Nairobi: TSBF-CIAT and SACRED Africa.
Pasley, H. R., Cairns, J. E., Camberato, J. J., and Vyn, T. J. (2019). Nitrogen fertilizer rate increases plant uptake and soil availability of essential nutrients in continuous maize production in Kenya and Zimbabwe. Nutr. Cycl. Agroecosyst. 115, 373–389. doi: 10.1007/s10705-019-10016-1
Pasley, H. R., Camberato, J. J., Cairns, J. E., Zaman-Allah, M., Das, B., and Vyn, T. J. (2020). Nitrogen rate impacts on tropical maize nitrogen use efficiency and soil nitrogen depletion in eastern and southern Africa. Nutr. Cycl. Agroecosyst. 116, 397–408. doi: 10.1007/s10705-020-10049-x
Quemada, M., Lassaletta, L., Jensen, L. S., Godinot, O., Brentrup, F., Buckley, C., et al. (2020). Exploring nitrogen indicators of farm performance among farm types across several European case studies. Agric. Syst. 177:102689. doi: 10.1016/j.agsy.2019.102689
R Core Team (2019). R: A Language and Environment for Statistical Computing. Vienna: R Foundation for Statistical Computing.
Richards, M. B., Ittersum, M. K. V., Mamo, T., Stirling, C. M., Vanlauwe, B., and Zougmoré, R. B. (2016). Fertilizers and Low Emission Development in Sub-Saharan Africa. Copenhagen: CGIAR Research Program on Climate Change, Agriculture and Food Security.
Rurinda, J., Zingore, S., Jibrin, J. M., Balemi, T., Masuki, K., Andersson, J. A., et al. (2020). Science-based decision support for formulating crop fertilizer recommendations in sub-Saharan Africa. Agric. Syst. 180:102790. doi: 10.1016/j.agsy.2020.102790
Sen, S., Smith, M. E., and Setter, T. (2016). Effects of low nitrogen on chlorophyll content and dry matter accumulation in maize. Afr. J. Agric. Res. 11, 1001–1007. doi: 10.5897/AJAR2015.9673
Sheahan, M., Barrett, C. B., and Sheahan, M. B. (2014). Understanding the Agricultural Input Landscape in Sub-Saharan Africa: Recent Plot, Household, and Community-Level Evidence. World Bank Policy Research Working Paper 7014. Abidjan: World Bank Group. doi: 10.1596/1813-9450-7014
Snyder, C. S., and Bruulsema, T. W. (2007). Nutrient Use Efficiency and Effectiveness in North America. International Plant Nutrition Institute. (Peachtree).
Srivastava, R. K., Panda, R. K., Chakraborty, A., and Halder, D. (2018). Enhancing grain yield, biomass and nitrogen use efficiency of maize by varying sowing dates and nitrogen rate under rainfed and irrigated conditions. Field Crops Res. 221, 339–349. doi: 10.1016/j.fcr.2017.06.019
Sutton, M. A., Bleeker, A., Howard, C. M., Erisman, J. W., Abrol, Y. P., Bekunda, M., et al. (2013). Our Nutrient World. The Challenge to Produce More Food and Energy With Less Pollution. Edinburgh: Centre for Ecology and Hydrology.
Tamene, L., Mponela, P., Ndengu, G., and Kihara, J. (2016). Assessment of maize yield gap and major determinant factors between smallholder farmers in the Dedza district of Malawi. Nutr. Cycl. Agroecosyst. 105, 291–308. doi: 10.1007/s10705-015-9692-7
Ten Berge, H. F., Hijbeek, R., van Loon, M. P., Rurinda, J., Tesfaye, K., Zingore, S., et al. (2019). Maize crop nutrient input requirements for food security in sub-Saharan Africa. Glob. Food Secur. 23, 9–21. doi: 10.1016/j.gfs.2019.02.001
Thar, S. P., Farquharson, R. J., Ramilan, T., Coggins, S., and Chen, D. (2021). Recommended vs. practice: smallholder fertilizer decisions in central Myanmar. Agriculture 11:65. doi: 10.3390/agriculture11010065
Ullah, H., Santiago-Arenas, R., Ferdous, Z., Attia, A., and Datta, A. (2019). Improving water use efficiency, nitrogen use efficiency, and radiation use efficiency in field crops under drought stress: A review. Advances in agronomy 156, 109–157. doi: 10.1016/bs.agron.2019.02.002
van Dijk, M., Morley, T., van Loon, M., Reidsma, P., Tesfaye, K., and van Ittersum, M. K. (2020). Reducing the maize yield gap in Ethiopia: decomposition and policy simulation. Agric. Syst. 183:102828 doi: 10.1016/j.agsy.2020.102828
Van Ittersum, M. K., Van Bussel, L. G., Wolf, J., Grassini, P., Van Wart, J., Guilpart, N., et al. (2016). Can sub-Saharan Africa feed itself? Proc. Natl. Acad. Sci. 113, 14964–14969. doi: 10.1073/pnas.1610359113
Vanlauwe, B., and Giller, K. E. (2006). Popular myths around soil fertility management in sub-Saharan Africa. Agric. Ecosyst. Environ. 116, 34–46. doi: 10.1016/j.agee.2006.03.016
Vanlauwe, B., Kihara, J., Chivenge, P., Pypers, P., Coe, R., and Six, J. (2011). Agronomic use efficiency of N fertilizer in maize-based systems in sub-Saharan Africa within the context of integrated soil fertility management. Plant Soil 339, 35–50. doi: 10.1007/s11104-010-0462-7
Wang, M., Wang, L., Cui, Z., Chen, X., Xie, J., and Hou, Y. (2017). Closing the yield gap and achieving high N use efficiency and low apparent N losses. Field Crops Res. 209, 39–46. doi: 10.1016/j.fcr.2017.03.016
Keywords: nitrogen use efficiency, low yield, soil mining, nitrogen deficit, partial N balance, soil fertility, maize
Citation: Winnie N, Giweta M, Gweyi-Onyango J, Mochoge B, Mutegi J, Nziguheba G and Masso C (2022) Assessment of the 2006 Abuja Fertilizer Declaration With Emphasis on Nitrogen Use Efficiency to Reduce Yield Gaps in Maize Production. Front. Sustain. Food Syst. 5:758724. doi: 10.3389/fsufs.2021.758724
Received: 03 November 2021; Accepted: 23 December 2021;
Published: 01 February 2022.
Edited by:
Andrews Opoku, Kwame Nkrumah University of Science and Technology, GhanaReviewed by:
Libère Nkurunziza, Swedish University of Agricultural Sciences, SwedenCopyright © 2022 Winnie, Giweta, Gweyi-Onyango, Mochoge, Mutegi, Nziguheba and Masso. This is an open-access article distributed under the terms of the Creative Commons Attribution License (CC BY). The use, distribution or reproduction in other forums is permitted, provided the original author(s) and the copyright owner(s) are credited and that the original publication in this journal is cited, in accordance with accepted academic practice. No use, distribution or reproduction is permitted which does not comply with these terms.
*Correspondence: Mekonnen Giweta, SC5NZWtvbm5lbkBjZ2lhci5vcmc=
Disclaimer: All claims expressed in this article are solely those of the authors and do not necessarily represent those of their affiliated organizations, or those of the publisher, the editors and the reviewers. Any product that may be evaluated in this article or claim that may be made by its manufacturer is not guaranteed or endorsed by the publisher.
Research integrity at Frontiers
Learn more about the work of our research integrity team to safeguard the quality of each article we publish.