- 1Department of Forage Plants and Agrometeorology, Faculty of Agronomy, Federal University of Rio Grande do Sul (UFRGS), Porto Alegre, Brazil
- 2Department Environment and Agronomy, Institut National de Recherche pour l'Agriculture, l'Alimentation et l'Environnement (INRAE), Lusignan, France
Closely integrated crop and livestock production systems used to be the rule in agriculture before the industrial revolution. However, agricultural landscapes have undergone a massive intensification process in recent decades. This trajectory has led to uniform landscapes of specialized cropping systems or consolidated zones of intensive livestock production. Loss of diversity is at the core of increasing side effects on the environment from agriculture. The unintended consequences of specialization demand the reconciliation of food production with environmental quality. We argue that the reconnection of grazing livestock to specialized crop landscapes can restore decoupled biogeochemical cycles and reintroduce the necessary complexity to restore ecosystem functioning. Besides, the reconnection of crops and livestock promotes several ecosystem services underlying multifunctionality. We focus on the capacity of integrated crop-livestock systems to create biophysical and socioeconomic resilience that cope with weather and market oscillations. We present examples of redesigned landscapes that leverage grazing animals to optimize food production per unit of land while mitigating the externalities of specialized agriculture. We also debate mindset barriers to the shift of current specialization trends toward the design of multifunctional landscapes.
Introduction
Multifunctional landscapes are those providing multiple ecosystem services (ES) simultaneously (Lovell and Johnston, 2009; Butterfield et al., 2016). By balancing the delivery of provisioning, regulating, supporting and cultural ES, the promotion of landscape multifunctionality is critical to ensure the sustainability of “working lands” (sensu Kremen and Merenlender, 2018) and human well-being (Millennium Ecosystem Assessment, 2005; Butterfield et al., 2016; Wood et al., 2018; Fagerholm et al., 2020). However, agricultural landscapes have undergone the opposite trend in the last decades. The introduction of high yielding crop cultivars, the growing use of chemicals (inorganic fertilizers, herbicides and pesticides) and the development of high-tech machinery have resulted in an intensive, specialized farming model that has been successful in maximizing single ES (e.g., food production) but often at the expense of other fundamental ES, such as biodiversity and climate regulation (Tilman et al., 2001; Foley et al., 2005; Kremen and Merenlender, 2018).
The reduced diversity and increased uniformity perceived nowadays in agricultural landscapes are often a result of the spatial decoupling between crop and livestock production (Lemaire et al., 2015). For millennia, the fluxes connecting them—such crop residues being used to feed livestock and animal manure serving as the main nutrient source for crop production—were fundamental to sustain food production in ancient agricultural societies (Russelle et al., 2007; Bogaard et al., 2013). Since the “Green Revolution,” however, specialized cropping systems have increasingly encroached upon natural ecosystems worldwide, as reported in the Rio de la Plata grasslands region of South America (Modernel et al., 2016; de Faccio Carvalho et al., 2021). Ruminant livestock production, in turn, has moved from extensive grazing systems to feedlots, or concentrated in pasture areas under increased stocking rates, frequently resulting in overgrazing (de Faccio Carvalho and Batello, 2009; Modernel et al., 2016). The creation of these consolidated zones of specialized production has resulted in loss of diversity and agroecosystems multifunctionality, besides environmental issues such as water contamination and atmospheric pollution (Verhoeven et al., 2006; Gerber et al., 2013).
The reconnection of crop and livestock production in integrated crop-livestock systems (ICLS) has been proposed as an alternative to the kind of specialized agricultural production that results in landscape uniformization, simplification of ecological processes and heavy reliance on external inputs (Russelle et al., 2007; FAO, 2010; Lemaire et al., 2015). In his framework inspired by the classic paper “The Strategy of Ecosystem Development” (Odum, 1969), Tracy (2007) identified modern cropping systems based on monocultures as an example of a young, developing ecosystem: “highly productive and biologically simple, but generally unstable and leaky from a mineral cycling perspective.” In contrast, mature ecosystems are “more stable and retentive of soil nutrients” and “although less productive, [they] provide many valuable ecosystem services we depend on to maintain a high quality of life” (Tracy, 2007). In this sense, although modern agriculture has been successful in maximizing food production, mankind also depends on several other ES to thrive, and those are usually provided by mature ecosystems. Thus, reconciling these demands is a challenge. For containing elements of both, ICLS represent a good compromise between developing ecosystems and mature ecosystems: high productivity, conferred by the production of crops and livestock, and a wide range of ES emerging from the complex interactions and synergies between system components (Tracy, 2007).
In this manuscript, we present the benefits from reconnecting grazing livestock to previously uniform crop landscapes as an alternative to restore the multifunctionality lost over decades of agricultural specialization. We present this framework by exploring the effects of grazing livestock integration across different spatio-temporal scales and with different levels of planned biodiversity as pivotal to the design of future multipurpose foodscapes (i.e., the landscapes providing humans and herbivores with nourishment). We also address barriers and levers in socialscapes (i.e., communities and cultures in close relationship with foodscapes) that are important to take into account in the design of agricultural systems where grazing livestock share space with crops in valuable cropping areas.
Restoring Landscape Multifunctionality: Planned Biodiversity Reconnecting Grazing Animals to Crop Landscapes
Rethinking agriculture to shift the focus from the production of single ES back to a multifunctional perspective where key ES are integrated (e.g., provision of quality food and water, carbon sequestration, and promotion of biodiversity above and below ground) is critical to ensure that food production systems will be able to feed a growing human population. Besides, climate change and its associated uncertainties exacerbate the need for resilient food systems (Foley et al., 2011; Kremen and Merenlender, 2018; Wood et al., 2018). Therefore, considering that biodiversity loss is a major driver of reduced multifunctionality across all terrestrial ecosystems (Cardinale et al., 2012; Fanin et al., 2018), biodiversity-based, resilience-oriented farming practices must be targeted if the aim is to restore landscape multifunctionality (Foley et al., 2005, 2011; Kremen and Merenlender, 2018). Alternatives for the re-diversification of agricultural systems with associated gains in ES delivery include the use of diversified crop rotations or polycultures (Kremen and Merenlender, 2018; Bowles et al., 2020; Guzman et al., 2021), cover crops (Pinto et al., 2017; Sekaran et al., 2021; Villarino et al., 2021) and the recoupling of crop and livestock production (Soussana and Lemaire, 2014; de Faccio Carvalho et al., 2021; Sekaran et al., 2021).
Increased crop rotation diversity (or “planned biodiversity,” defined as the biodiversity chosen by the farmer; Brustel et al., 2018) has been recognized for improving crop yields (Bowles et al., 2020) and stability in the face of climatic oscillations (Gaudin et al., 2015; Bowles et al., 2020). These characteristics are critical for food security in the context of climate change. Also, polycultures improve the richness and diversity of soil arbuscular mycorrhizal fungal communities in areas previously managed under intensive monoculture farming, with applications for landscape multifunctionality restoration (Guzman et al., 2021). As part of the “associated biodiversity” (i.e., the component of agrobiodiversity that emerges from farming practices; Duprat et al., 2018), these organisms play an important role in nutrient acquisition, soil structure formation and drought tolerance.
The inclusion of cover crops in agricultural rotations has also been recognized for providing multiple ES, in such a way that these plants have been called “service crops” (Piñeiro et al., 2014; Pinto et al., 2017). Benefits of cover cropping include reduced soil erosion and compaction, weed suppression, improved pollination, nitrogen fixation and reduced leaching, carbon sequestration and accumulation in soils, and improved soil aggregation, water retention and drought tolerance (Tonitto et al., 2006; Piñeiro et al., 2014; Pinto et al., 2017; Reicosky et al., 2021). Examples of cover crops typically used in Latin America include grass species such as oats (Avena spp.), Italian ryegrass (Lolium multiflorum) and signal grass (Urochloa spp.), legumes such as vetches (Vicia spp.) and clovers (Trifolium spp.), and others. In general, these forage species are chosen because of their biomass production potential and feasibility to no-till systems, which are widely used in many countries.
Despite all those benefits, cover crops lack human-edible food production (de Faccio Carvalho et al., 2018a) and direct economic returns (Wittwer et al., 2017). Furthermore, because crop growers are usually concerned about soil compaction, millions of hectares are cultivated with non-grazed cover crops just in the same period that livestock experience feed shortages in disconnected grazing systems. ICLS, in contrast, can encompass all these alternatives by using diverse cover crops as high-quality forage for grazing. When combined with soil conservation practices such as no-tillage, ICLS can fully exploit the synergisms and emergent properties of the system (de Faccio Carvalho et al., 2010).
Grazing of cover crops in annual or perennial cropping systems is one of the many possible models of crop-livestock integration (Brewer and Gaudin, 2020). Examples based on cover crop grazing range from simpler designs where the same crop rotation takes place repeatedly every year (e.g., soybean production followed by temperate grass cover crops grazed by cattle; de Albuquerque Nunes et al., 2021), to more complex crop rotations with a greater diversity of crop species [e.g., Sudan grass (Sorghum sudanense), soybean, maize and rice in the same area in subsequent summers, followed by a mixture of Italian ryegrass + white clover (Trifolium repens) grazed in winter; Carlos et al., 2020]. Cover crop grazing in perennial systems is well-represented by grazing of the understory vegetation in orchards (Ramos et al., 2011) and vineyards (Ryschawy et al., 2021). Other ICLS models include stubble grazing (Rakkar et al., 2017), sod-based crop rotations (Katsvairo et al., 2006) and production of dual-purpose crops (Kirkegaard et al., 2012). The possible designs of diverse cropping systems connected with livestock are almost unlimited, expanding across various spatiotemporal scales, ranging from plot to farm and to the territorial scale (Moraine et al., 2017).
In this context, reconnecting livestock to specialized crop landscapes represents the addition of a trophic level in the planned biodiversity of agricultural systems (de Albuquerque Nunes et al., 2021). The grazing animal restores routes of nutrient cycling through forage ingestion and digestion, releasing, in the form of dung and urine, nutrients with greater lability (Arnuti et al., 2020) that impact soil stoichiometry. For example, P bioavailability in the soil increased 32% in grain crop areas where grazing was reintegrated (Deiss et al., 2016). By acting as “catalysts” of system processes (de Faccio Carvalho et al., 2009), grazing animals can both amplify ES provisioning with the application of best management practices or accelerate land degradation through the overexploitation of resources (e.g., overgrazing; Figure 1).
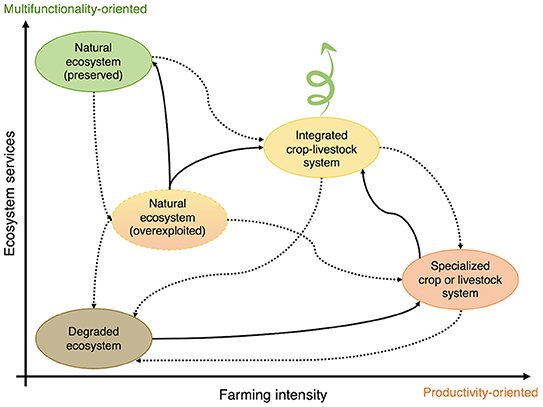
Figure 1. Conceptual model of states and transitions in agroecosystems as a function of farming specialization and ecosystem services (ES) delivery. Solid and dotted lines denote positive and negative effects of the transition on ES delivery, respectively. Negative transitions could be caused by overexploitation of resources (e.g., overgrazing) or adoption of aggressive, non-conservationist agricultural practices (e.g., multi-pass tillage) or industrial livestock production (e.g., feedlots). Positive transitions could be promoted through sound grazing management practices (e.g., moderate grazing intensity), conservation agriculture practices (e.g., no-till and crop rotation diversity) and adoption of crop-livestock integration. Green arrow indicates that ES provided by integrated crop-livestock systems are potentially higher depending on synergisms levels (see explanation in the text). For clarity, only some possible states and transitions are shown.
While grazers are the dynamic agents driving changes in the landscape, plants react to grazing management (e.g., grazing intensity) by signaling the direction (+ or –) of these changes (e.g., herbage growth rates). The soil environment captures these impacts by acting as the “memory” of the system (de Faccio Carvalho et al., 2009). Thus, the reconnection of livestock in crop landscapes should be planned to explore the synergistic relationships between these components, rather than simply producing crops and animal products in the same unit area to improve farm income; “the whole should be greater than the sum of the parts” (FAO, 2010; de Faccio Carvalho et al., 2014). Then, those redesigned landscapes would approach their potential to enhance the provisioning of ES and rehabilitate landscape multifunctionality.
Reconnecting Livestock and Crops Across Multiple Spatio-Temporal Scales
Landscape multifunctionality depends not only on the reconnection of crop and livestock production but also on the distribution of these components over space and time. Ecosystem services are supplied by ecological functions associated with individuals, populations, and communities, and their spatio-temporal distribution is determined by the inherent scales in which these organisms operate (Laca, 2021). Because ES involve flows of matter, energy or information (Cadenasso et al., 2003), their production varies as a function of how these ES provisioning agents differ in mobility and perception of the environment, which is ultimately related to the organism size (Ritchie and Olff, 1999). As a consequence, ES responses vary with the spatio-temporal design on which the ICLS are implemented (Lindborg et al., 2017). Thus, a more complete and practical understanding of ICLS multifunctionality requires consideration of the spatio-temporal relations among the crop and livestock components.
Although some ICLS studies have predominantly focused on the succession of crops and pastures in the same paddock (Franzluebbers and Stuedemann, 2008; Acosta-Martínez et al., 2010; Assmann et al., 2014; Martins et al., 2017; de Albuquerque Nunes et al., 2021), crop-livestock integration can expand across various spatio-temporal scales. Importantly, though, only those designed to explore the synergistic relationships between the plant-animal-soil components of the system can be considered effectively integrated (FAO, 2010; de Faccio Carvalho et al., 2014). Examples of scenarios at different scales range from systems such as cover crop grazing in the understory of orchards (Ramos et al., 2011) and vineyards (Niles et al., 2017), where crop and livestock production are simultaneous in the same plot (i.e., closely integrated in space and time), to integration at the landscape or territorial scale (territorial crop-livestock integration; Moraine et al., 2017), where specialized crop and livestock farms coordinate exchanges of products or byproducts such as hay and manure (Figure 2). Certain models of integration can even involve more than one spatio-temporal scale simultaneously, such as happens in sheep-vineyard systems of California, where shepherds are contracted for temporary grazing to reduce weed competition and fuel load in the understory, among other benefits (Ryschawy et al., 2021). Although sheep and vineyards are closely integrated in space and time during the grazing period, a coordination of actions at the territorial scale is also required.
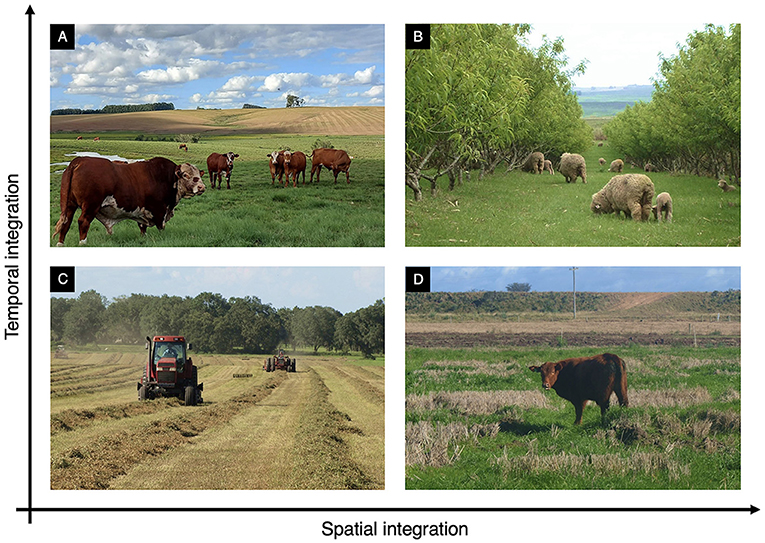
Figure 2. Examples of integrated systems in multiple spatio-temporal designs. The axes denote the degree of interspersion in which crops and livestock are integrated along a spatio–temporal continuum. For a purpose of simplicity, only four discrete examples are shown, but multiple spatio-temporal designs are possible (see Bell and Moore, 2012). (A) Soybean cropping and native grasslands simultaneously occurring in neighboring fields. Pollination services for soybeans are enhanced because native grassland ecosystems provide habitat for a diversity of pollinators. Photo credit: Méia Albuquerque. (B) Sheep grazing the understory vegetation of a peach orchard. Grazing reduces the competitiveness of weed species and redistributes nutrients over the orchard. Photo credit: Thomaz Mercio. (C) Farmers harvesting hay. Integration at the landscape level involves coordinate exchanges of products or byproducts such as hay and manure between specialized crop and livestock farms. Photo credit: Marcelo Wallau. (D) Succession of crops and livestock in the same paddock, where stubble and cover crops are grazed by domestic herbivores, usually such as cattle and/or sheep. The picture shows a steer grazing in an Italian ryegrass pasture in winter following rice cultivation. Photo credit: Fernanda Moojen.
Benefits and Trade-Offs of Reconnecting Livestock and Crops at Different Spatio-Temporal Scales
The reconnection between grazing livestock and croplands has a fundamental role to play in system stoichiometry (Soussana and Lemaire, 2014). On the one hand, ruminants decouple C and N cycles, releasing digestible C through enteric emissions (3–5%), and returning mostly indigestible C via dung (60%) and high concentrations of digestible N in urine (70%) (IPCC, 2006). On the other hand, C and N cycles are recoupled by photosynthesis and plant growth processes until decomposition or grazing decouples them once again. Because the balance between C–N coupling by vegetation and C–N decoupling by animals determines the benefits and environmental impacts of ICLS, the distribution of livestock and crops over space and time can influence system dynamics in a positive or negative manner (Soussana and Lemaire, 2014). For example, when proper grazing management is applied (e.g., moderate grazing intensity), nutrient cycling is boosted by grazing of cover crops at the paddock scale without reducing the nutrient budgets.
For example, Alves et al. (2019) reported that P and K exportation from an ICLS area in sheep meat was 0.7 and 1.3 kg ha−1 yr−1 on average, respectively, over 14 years of annual soybean/maize - grazed Italian ryegrass succession, which represented only 6 and 5% of total exportations from that area (~95% was exported in grain crops). Instead of being exported, the greatest share of nutrients is redistributed in the paddock during livestock foraging processes, affecting the spatial pattern of soil attributes and creating a mosaic of nutrient-rich zones near fences, supplement troughs and watering points, and nutrient-poor zones away from these attractants (Dubeux et al., 2006; da Silva et al., 2014, 2020). Alternatives to remedy the uneven nutrient distribution caused by grazing animals management practices such as variable location of supplement troughs and spatial patterns of seeding of preferred species. However, da Silva et al. (2014) and de Albuquerque Nunes et al. (2021) reported no differences in succeeding soybean yields (kg ha−1) regardless of the spatio-temporal patterns of dung deposition caused by different grazing intensities in the preceding winter of a soybean-beef cattle system.
When the integration occurs at the landscape level, forage exportation (e.g., hay) from a hypothetical farm A to farm B will decrease nutrient availability in farm A if the same amount of nutrients is not replenished via, for example, manure application (which would ideally come from farm B). Also, nutrient excesses in farm B can drive leakage from the system if those nutrients are not managed properly or returned to farm A. Not returning nutrients to farm A characterizes a typical specialization trend, such as in intensive landless livestock farms in peri-urban regions of high-density population. This pattern of concentrating landless, specialized farms near urban areas to provide fresh feed by importing nutrients from outside is reported to result in concentration of nutrients and pollution when nutrients are not recycled properly (Chadwick et al., 2021).
Decreasing input dependence is a key factor in promoting sustainability (Bonaudo et al., 2014). There are numerous benefits of reconnecting grazing ruminants to croplands in these aspects. For example, the weed suppressing effect promoted by grazing best management practices in crop-pasture rotations combined with no-till management reduces herbicide dependence and associated costs of weed control at paddock scale (Schuster et al., 2016; Dominschek et al., 2021). There is also a reduction in the incidence of crop and livestock diseases in some cases. Roese et al. (2020) observed reduced incidence of diseases in the aerial parts of grain crops in ICLS with trees (eucalypts) due to the microclimate effect created in the understory. Portugal et al. (2018) reported a reduction in livestock diseases caused by ectoparasites (e.g., ticks) due to the break in the organisms' life cycle resulting from crop rotations. In both cases, there was a reduction in the use of chemicals as a consequence.
Through well-managed pastures there is an increase in the opportunity for visits by pollinators and other flying animals, due to the heterogeneity promoted by grazing that creates new food webs (Orford et al., 2016; Van Rijn and Wäckers, 2016; Enri et al., 2017; Jacoboski et al., 2017). Pollination increases the production of seeds of forage species, decreasing the need to purchase seeds due to natural reseeding (Rao and Stephen, 2009; Boelt et al., 2015; Rundlöf et al., 2018). In addition, forest or pasture components create opportunities for shelter to predators of agricultural pests such as spiders and birds (Bretagnolle et al., 2011; Prevedello et al., 2018; Freiberg et al., 2020).
Reconnecting grazing ruminants to croplands promotes changes in the physico-chemical and biological control mechanisms in the soil and allows for an improvement in the efficiency of the use of nutrients by plants, resulting in a system less dependent on external inputs (Denardin et al., 2020). Resource-use efficiency per unit energy production was higher in soybean-Italian ryegrass rotations grazed by sheep compared to non-grazed Italian ryegrass cover crop at paddock scale (Farias et al., 2020).
Bell and Moore (2012) analyzed the multi-dimensional features of integrated livestock and crop enterprises according to space-time dimensions. Inspired by smallholder systems in western Africa and large-sized commercial farms in Australia, they proposed that benefits are higher when activities are closer to one another. The latter scenario is typical of Latin American integrated systems (de Faccio Carvalho et al., 2021), so we can use these closely integrated systems to explore the synergisms at their theoretical maximum level. Moreover, as mentioned earlier, the dichotomy of ungrazed vs. grazed cover crops being studied in Latin America provides a unique opportunity to examine the specific effects of the reintroduction of grazing animals in specialized crop landscapes in isolation from the plant effect (ungrazed pasture as a cover crop) and at smaller spatial scales (Table 1).
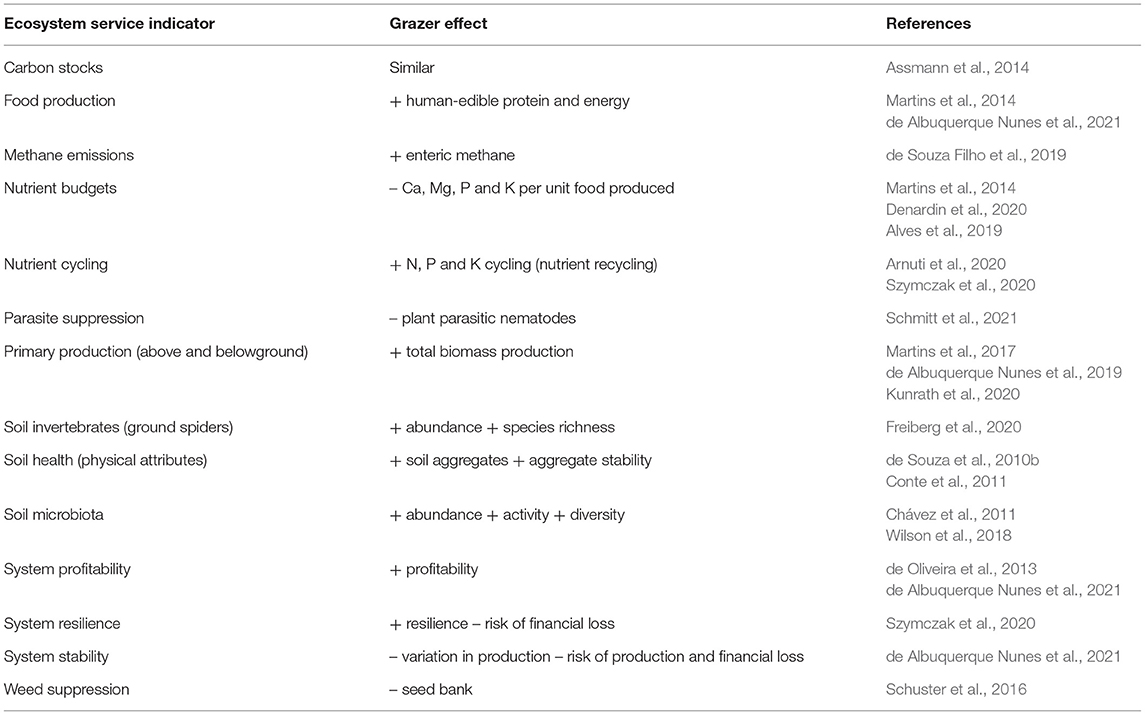
Table 1. Grazer effect at the paddock level and at moderate grazing intensity on ecosystem service indicators.
Assuming that the on-farm paddock scale represents the highest potential benefit to systems that reconnect livestock and crop production, it is worth noting how grazing animals are pivotal to restoring multifunctionality in specialized crop landscapes. By coupling and decoupling nutrients in the same area among different compartments, reconnected livestock-crop landscapes reach more complex organizational structures and increased hierarchical exchanges among the different living organisms. As presented in Table 1, multiple benefits cascade among different compartments affecting the whole system, whereupon emergent properties may arise (de Faccio Carvalho et al., 2018b).
At this point we surmise that system resilience is a key feature enhanced by reconnected crop-livestock landscapes that best represents the overall restoration of ecosystem functioning. So, we can focus specifically on resilience-oriented integrated systems.
Resilience: Harnessing Functional Diversity by Reconnecting Livestock to Crop Landscapes
Biodiversity is essential to building resilience (Ulanowicz et al., 2009) and can be characterized at two hierarchical levels of functionality that are important in regulating the structure and functioning of ecosystem services (Hooper et al., 2005; Duffy et al., 2007; Moonen and Bàrberi, 2008). The vertical dimension represents the trophic levels in the system, while the horizontal dimension is related to the number of species within each trophic level (Duffy et al., 2007). The vertical dimension of ICLS comprises plants and herbivores. On the other hand, the horizontal dimension is composed of functional genetic diversity, functional types of plants and ruminant and monogastric herbivores (Bell and Moore, 2012; Garrett et al., 2017). The design of reconnected crop-livestock systems provides a way of planning both dimensions of diversity in space and time, aiming to benefit from synergies between system components and achieve a higher overall system performance compared to the sum of individual performances (de Moraes et al., 2014; de Faccio Carvalho et al., 2018a).
ICLS are based on the philosophy of building resilience by increasing systems' capacity to adapt and self-organize in response to external disturbances, such as environmental changes (Bonaudo et al., 2014; de Moraes et al., 2014). This is largely due to the adoption of agricultural practices that increase soil organic matter, water and nutrient use efficiency, nutrient recycling, biodiversity, and spatio-temporal heterogeneity (Wezel et al., 2014; Altieri et al., 2015; Lemaire et al., 2015; Garrett et al., 2017; Van Oijen et al., 2020). To be resilient, an ecosystem must exhibit capacity to maintain its integrity over time and must have a reserve of flexible pathways, through a diversity of flows, to adapt to uncertainties (Ulanowicz et al., 2009; Altieri et al., 2015; Stark et al., 2018). From this perspective, Szymczak et al. (2020) observed that the greater diversity of nitrogen and phosphorus flows created by the reconnection between grazing animals and crop production in a commercial ICLS enhanced its resilience in comparison to a specialized soybean production system.
The vertical diversification represented by the addition of a trophic level when grazing herbivores are reconnected to crop landscapes increases the functional diversity and complexity of these systems (Duffy et al., 2007; Mori et al., 2013; Sanderson et al., 2013). It provides matter processing by digestion and nutrient cycling, as a small amount of the nutrients ingested by grazing animals is exported from the system. As a result of the improved nutrient flows and their ecological interactions (e.g., nutrient recycling via defecation; da Silva et al., 2014, 2020; Arnuti et al., 2020), improvements in soil chemical, physical and biological attributes are observed at field scale (e.g., de Souza et al., 2010a,b; Chávez et al., 2011; Assmann et al., 2014; Martins et al., 2014; Deiss et al., 2016; Damian et al., 2021). Ultimately, it enhances the long-term stability of crop production without compromising grain yields (de Albuquerque Nunes et al., 2021). This rationale can also be applied on-farm (among paddocks) or at landscape scale (among farms). Animals would be moved at different periods and act as connecting agents between system components, improving agricultural resilience (de Faccio Carvalho et al., 2018a; Peterson et al., 2018; Stark et al., 2018; Paramesh et al., 2020; Tittonell, 2020).
In addition to the resilience of nutrient flows, the reconnection of grazing livestock to crop landscapes provides a means to enhance economic resilience by diversifying income, which buffers market and climate oscillations by smoothing farm incomes in poor crop production years (de Albuquerque Nunes et al., 2021). Therefore, when one production activity faces disturbances, the other activity may not be affected. Also, pasture-based livestock systems present greater adaptive capacity to deal with climatic oscillations, making ICLS less vulnerable than pure cropping systems. Using two differing approaches to study system responses to stress, both Szymczak et al. (2020) and de Albuquerque Nunes et al. (2021) observed a reduced risk of financial loss when crop and livestock production were reconnected (ICLS) relative to specialized soybean systems. Maximum profitability potential was increased by up to ~30% in ICLS compared to the pure soybean system in the best environmental conditions (de Albuquerque Nunes et al., 2021).
The challenge of feeding a growing human population in a world of increasing uncertainties has been extensively debated by scientists. Therefore, to ensure food security, the foodscapes we plan today and into the future should consider the effects of climate change (e.g., increased weather variability and anomalies) and focus not only on increasing food production, but also approaches to deal with uncertainty. To this end, a study with historical data of a long-term experiment in southern Brazil (2001–2016) showed that reconnecting beef cattle to soybean systems increased the overall food production and long-term production stability in terms of human-edible protein. Moreover, it reduced the chance of failure in less favorable environments due to the production surplus provided by grazing cattle (de Albuquerque Nunes et al., 2021). Using the same historical ICLS dataset, Peterson et al. (2020) simulated climate conditions and the productivity and resilience of that ICLS for the next 40 years (2020–2060) using APSIM model, observing that ICLS gross margins exceeded that of the specialized system in 95% of years, while resilience to precipitation anomalies (more frequent in simulated climate scenario) depended on disturbance type and timing.
Socialscapes: Mindset Transition From Specialized Toward Integrated Systems
Here we address part of the social dimension driving agricultural landscapes beyond the technical outcomes exposed throughout this paper. Reconnecting grazing animals to crop landscapes is not simply a technical decision. Because people ultimately manage landscapes, there are specific processes involving human behavior that are required to sensitize people and build the necessary conditions to manage more complex food production systems. First, there is an awareness step to prepare the mindset shift from current specialized production (thinking crop and livestock goals, planning and management separately) to a long-term, integrated systems thinking (Moojen, 2021). Second, the inherent complexity of ICLS implies the requirement of more complicated farm planning (i.e., spatio-temporal land use, financial planning, short- and long-term objectives). In this regard, proper advising is imperative to assist farmers in a desirable co-design processes (Moojen, 2021). Therefore, a distinct capacity building is crucial to both farmers and advisors, making them aware of the potential interactions between crops and livestock and capable of redesigning the farming system with ICLS principles by developing specific skills to manage multifunctional systems (Bonaudo et al., 2014).
It is worth mentioning that the specialization trend of crop and livestock production systems has side-effects beyond environmental boundaries. Academia, research and extension centers have been oriented toward segregated crop and livestock production. Consequently, teaching, research and extension initiatives focus on separate specialized outputs from each activity, and lack in the technical capabilities and knowledge adapted to ICLS (Garrett et al., 2020). Holistic approaches have been replaced by simple technical schedules. This state of affairs is a barrier to ICLS adoption (Bonaudo et al., 2014). To face it, the adaptation of agricultural courses to include systems thinking, didactic learning tools, and interdisciplinarity could help train future professionals to reacquire holistic perceptions and enable a path forward for sustainable ICLS implementation (Jouan et al., 2020).
Participatory methods for designing ICLS at the farm and landscape level have been reported as a promising way to connect farmers, advisors, and researchers to exchange knowledge and analyze scenarios (Ryschawy et al., 2014; Moraine et al., 2017). This is the case with “serious games,” i.e., games aiming at specific learning outcomes (Wouters et al., 2009). Serious games can have goals such as (i) supporting negotiation of silvopastoral management (Etienne, 2003), (ii) assessing impacts of farming practices on sustainability (Jouan et al., 2020), (iii) designing technical and organizational scenarios among farmers (Ryschawy et al., 2018), (iv) exploring consequences of land-use decisions (Salvini et al., 2016) and co-designing spatio-temporal ICLS scenarios (Moojen, 2021). Overall, some tools and methodologies to assist people involved in the transitions are available, so it is necessary that their use be encouraged and customized to each context to remove barriers to multifunctional landscape design.
At the institutional scale, robust extension systems are needed to transition toward ICLS. Governmental projects must consider the greater complexity of ICLS in relation to specialized systems, thus providing flexibility for adapting tools and approaches for each farming context and skills like leadership and systemic vision to those involved in the project (Price et al., 2009).
Equally as important as the sensitization and empowerment of the people in the system is the economic viability of ICLS, which is crucial for adoption at the farm and landscape level. Credit availability for ICLS projects, supply chain infrastructure, and farmers' willingness to diversify production are some of the factors determining ICLS adoption (Gil et al., 2016). As mentioned in previous sections, studies have shown the importance of livestock production as an effective way for diversifying revenue sources in specialized agricultural systems, increasing overall system productivity, profitability, and stability to external stressors, and consequently reducing economic risks (Bell and Moore, 2012; de Oliveira et al., 2013; Szymczak et al., 2020; de Albuquerque Nunes et al., 2021). However, each ICLS has its own idiosyncrasies. For example, when a forest component is involved, economic benefits need to be analyzed on a larger temporal scale given the time needed for woodcuts, harvesting of fruit, or even shade for livestock comfort. The challenge, therefore, is to quantify and plan economic flows both in the short- and in the long-term according to each ICLS farm design.
Conclusions
In this manuscript we present evidence that the reconnection of grazing livestock to specialized crop landscapes can restore biogeochemical cycles decoupled by uniform landscapes lacking the diversity necessary for proper ecosystem functioning. Our approach enables disentangling the effects of forage plants from the grazing animal to underline ecosystem service indicators promoted specifically by the grazing process. This perspective highlights the capacity of grazing animals to recover landscape multifunctionality. Spatio-temporal designs of crop-livestock integration will affect the level of ecosystem services delivered. Multiple benefits can cascade over the whole system if moderate grazing is adopted, and resilience is a key feature that arises. This path of mixing crops and livestock embraces complexity against current specialization trends, requiring capacity building and mindset shifting. To conclude, grazing animals have an important role in the design of future foodscapes. Grazing herbivores are part of natural ecosystems, and commercial landscapes should aspire to mimic the beneficial functions of natural ecosystems.
Author Contributions
PF and GL conceived the initial idea. PF, PA, and AP-P wrote the first draft. PF, PA, AP-P, LS, WS, and FM provided input to the original draft. PF, PA, AP-P, LS, WS, FM, and GL revised the final version. All authors contributed to the article and approved the submitted version.
Funding
The authors are grateful to the Coordination for Improvement of Higher Education Personnel (CAPES), to the National Council for the Development of Scientific and Technological Development (CNPq), and to Fundação Agrisus for grants and research funding.
Conflict of Interest
The authors declare that the research was conducted in the absence of any commercial or financial relationships that could be construed as a potential conflict of interest.
Publisher's Note
All claims expressed in this article are solely those of the authors and do not necessarily represent those of their affiliated organizations, or those of the publisher, the editors and the reviewers. Any product that may be evaluated in this article, or claim that may be made by its manufacturer, is not guaranteed or endorsed by the publisher.
Acknowledgments
We thank Dr. Caitlin Peterson for grammar corrections in the final version of the manuscript. The support of GPSIPA research group and Aliança SIPA regarding the maintenance of ICLS long-term research is also greatly acknowledged.
References
Acosta-Martínez, V., Bell, C. W., Morris, B. E. L., Zak, J., and Allen, V. G. (2010). Long-term soil microbial community and enzyme activity responses to an integrated cropping-livestock system in a semi-arid region. Agric. Ecosyst. Environ. 137, 231–240. doi: 10.1016/j.agee.2010.02.008
Altieri, M. A., Nicholls, C. I., Henao, A., and Lana, M. A. (2015). Agroecology and the design of climate change-resilient farming systems. Agron. Sustain. Dev. 35, 869–890. doi: 10.1007/s13593-015-0285-2
Alves, L. A., Denardin, L. G. O., Martins, A. P., Anghinoni, I., de Faccio Carvalho, P. C., and Tiecher, T. (2019). Soil acidification and P, K, Ca and Mg budget as affected by sheep grazing and crop rotation in a long-term integrated crop-livestock system in southern Brazil. Geoderma 351, 197–208 doi: 10.1016/j.geoderma.2019.04.036
Arnuti, F., Denardin, L. G. O., de Albuquerque Nunes, P. A., Alves, L. A., Cecagno, D., de Assis, J., et al. (2020). Sheep dung composition and phosphorus and potassium release affected by grazing intensity and pasture development stage in an integrated crop-livestock system. Agronomy 10:1162. doi: 10.3390/agronomy10081162
Assmann, J. M., Anghinoni, I., Martins, A. P., Costa, S. E. V. G.A., Cecagno, D., Carlos, F. S., et al. (2014). Soil carbon and nitrogen stocks and fractions in a long-term integrated crop–livestock system under no-tillage in southern Brazil. Agric. Ecosyst. Environ. 190, 52–59. doi: 10.1016/j.agee.2013.12.003
Bell, L. W., and Moore, A. D. (2012). Integrated crop–livestock systems in Australian agriculture: trends, drivers and implications. Agric. Syst. 111, 1–12 doi: 10.1016/j.agsy.2012.04.003
Boelt, B., Julier, B., Karagic, D., and Hampton, J. (2015). Legume seed production meeting market requirements and economic impacts. Crit. Rev. Plant Sci. 34, 412–427. doi: 10.1080/07352689.2014.898477
Bogaard, A., Fraser, R., Heaton, T. H., Wallace, M., Vaiglova, P., Charles, M., et al. (2013). Crop manuring and intensive land management by Europe's first farmers. PNAS 110, 12589–12594. doi: 10.1073/pnas.1305918110
Bonaudo, T., Bendahan, A. B., Sabatier, R., Ryschawy, J., Bellon, S., Leger, F., et al. (2014). Agroecological principles for the redesign of integrated crop–livestock systems. Eur. J. Agron. 57, 43–51. doi: 10.1016/j.eja.2013.09.010
Bowles, T. M., Mooshammer, M., Socolar, Y., Calderón, F., Cavigelli, M. A., Culman, S. W., et al. (2020). Long-term evidence shows that crop-rotation diversification increases agricultural resilience to adverse growing conditions in North America. One Earth 2, 284–293. doi: 10.1016/j.oneear.2020.02.007
Bretagnolle, V., Gauffre, B., Meiss, H., and Badenhausser, I. (2011). “The role of grassland areas within arable cropping systems for the conservation of biodiversity at the regional level,” in Grassland Productivity and Ecosystem Services, eds G. Lemaire, J. Hodgson, and A. Chabbi (Wallingford, England: CAB International), 251–260.
Brewer, K. M., and Gaudin, A. C. (2020). Potential of crop-livestock integration to enhance carbon sequestration and agroecosystem functioning in semi-arid croplands. Soil Biol. Bioc. 149:107936. doi: 10.1016/j.soilbio.2020.107936
Brustel, L., Seyvet, M., and Sarthou, J.-P. (2018). Planned biodiversity: Definition. Dictionnaire d'Agroecologie. Available online at: https://dicoagroecologie.fr/en/encyclopedia/planned-biodiversity/ (accessed April 01, 2021).
Butterfield, B. J., Camhi, A. L., Rubin, R. L., and Schwalm, C. R. (2016). Tradeoffs and compatibilities among ecosystem services: biological, physical and economic drivers of multifunctionality. Adv. Ecol. Res. 54, 207–243. doi: 10.1016/bs.aecr.2015.09.002
Cadenasso, M. L., Pickett, S. T., Weathers, K. C., and Jones, C. G. (2003). A framework for a theory of ecological boundaries. BioScience 53, 750–758. doi: 10.1641/0006-3568(2003)053[0750:AFFATO]2.0.CO;2
Cardinale, B. J., Duffy, J. E., Gonzalez, A., Hooper, D. U., Perrings, C., Venail, P., et al. (2012). Biodiversity loss and its impact on humanity. Nature 486, 59–67. doi: 10.1038/nature11148
Carlos, F. S., Denardin, L. G. O., Martins, A. P., Anghinoni, I., de Faccio Carvalho, P. C., Rossi, I., et al. (2020). Integrated crop–livestock systems in lowlands increase the availability of nutrients to irrigated rice. Land Deg. Dev. 31, 2962–2972. doi: 10.1002/ldr.3653
Chadwick, D. R., Williams, J. R., Lu, Y., Ma, L., Bai, Z., Hou, Y., et al. (2021). Strategies to reduce nutrient pollution from manure management in China. Front. Agr. Sci. Eng. 7, 45–55. doi: 10.15302/J-FASE-2019293
Chávez, L. F., Escobar, L. F., Anghinoni, I., de Faccio Carvalho, P. C., and Meurer, E. J. (2011). Metabolic diversity and microbial activity in the soil in an integrated crop-livestock system under grazing intensities. Pesq. Agropecu. Bras. 46, 1254–1261. doi: 10.1590/S0100-204X2011001000020
Conte, O., Flores, J. P. C., Cassol, L. C., Anghinoni, I., de Faccio Carvalho, P. C., Levien, R., et al. (2011). Evolução de atributos físicos de solo em sistema de integração lavoura-pecuária. Pesqui. Agropecu. Bras. 46, 1301–1309. doi: 10.1590/S0100-204X2011001000026
da Silva, F. D., Amado, T. J. C., Bredemeier, C., Bremm, C., Anghinoni, I., and de Faccio Carvalho, P. C. (2014). Pasture grazing intensity and presence or absence of cattle dung input and its relationships to soybean nutrition and yield in integrated crop–livestock systems under no-till. Eur. J. Agron. 57, 84–91. doi: 10.1016/j.eja.2013.10.009
da Silva, F. D., de Albuquerque Nunes, P. A., Bredemeier, C., Cadenazzi, M., Amaral, L., et al. (2020). Spatiotemporal distribution of cattle dung patches in a subtropical soybean-beef system under different grazing intensities in winter. Agronomy 10:1423. doi: 10.3390/agronomy10091423
Damian, J. M., Matos, E. S., Pedreira, B. C., de Faccio Carvalho, P. C., de Souza, A. J., Andreote, F. D., et al. (2021). Pastureland intensification and diversification in Brazil mediate soil bacterial community structure changes and soil C accumulation. App. Soil Ecol. 160:103858. doi: 10.1016/j.apsoil.2020.103858
de Albuquerque Nunes, P. A., Bredemeier, C., Bremm, C., Caetano, L. A. M., de Almeida, G. M., de Souza Filho, W., et al. (2019). Grazing intensity determines pasture spatial heterogeneity and productivity in an integrated crop-livestock system. Grassl. Sci. 65, 49–59. doi: 10.1111/grs.12209
de Albuquerque Nunes, P. A., Laca, E. A., de Faccio Carvalho, P. C., Li, M., de Souza Filho, W., Kunrath, T. R., et al. (2021). Livestock integration into soybean systems improves long-term system stability and profits without compromising crop yields. Sci. Rep. 11:1649. doi: 10.1038/s41598-021-81270-z
de Faccio Carvalho, P. C., Anghinoni, I., de Moraes, A., de Souza, E. D., Sulc, R. M., Lang, C. R., et al. (2010). Managing grazing animals to achieve nutrient cycling and soil improvement in no-till integrated systems. Nut. Cyc. Agroec. 88, 259–273. doi: 10.1007/s10705-010-9360-x
de Faccio Carvalho, P. C., Anghinoni, I., de Moraes, A., Lang, C. R., Sulc, R. M., de Souza, E. D., et al. (2009). “Indicadores para avaliar sistemas de integração lavoura e pecuária de corte,” in Workshop Integração Lavoura-Pecuária-Floresta no Bioma Pampa, eds J. L. S. Silva, et al. (Pelotas, RS: Embrapa) 1–9.
de Faccio Carvalho, P. C., and Batello, C. (2009). Access to land, livestock production and ecosystem conservation in the Brazilian Campos biome: the natural grasslands dilemma. Liv. Sci. 120, 158–162. doi: 10.1016/j.livsci.2008.04.012
de Faccio Carvalho, P. C., de Albuquerque Nunes, P. A., and Anghinoni, I. (2018b). “O Processo de Pastejo como Gerador de Propriedades Emergentes em Sistemas Integrados de Produção Agropecuária,” in Sistemas Integrados de Produção Agropecuária no Brasil, eds E. D. de Souza, F. D. da Silva, T. S. Assmann, M. A. C. Carneiro, P. C. de Faccio Carvalho, and H. B. Paulino (Rondonópolis, MT: Copiart).
de Faccio Carvalho, P. C., de Moraes, A., Pontes, L. D. S., Anghinoni, I., Sulc, R. M., and Batello, C. (2014). Definitions and terminologies for integrated crop-livestock system. Rev. Cienc. Agron. 45, 1040–1046. doi: 10.1590/S1806-66902014000500020
de Faccio Carvalho, P. C., Peterson, C. A., de Albuquerque Nunes, P. A., Martins, A. P., de Souza Filho, W., Bertolazi, V. T., et al. (2018a). Animal production and soil characteristics from integrated crop-livestock systems: toward sustainable intensification. J. Ani. Sci. 96, 3513–3525. doi: 10.1093/jas/sky085
de Faccio Carvalho, P. C., Savian, J. V., Della Chiesa, T., de Souza Filho, W., Terra, J. A., Pinto, P., et al. (2021). Land-use intensification trends in the Rio de la Plata region of South America: toward specialization or recoupling crop and livestock production. Front. Agric. Sci. Eng. 8, 97–110. doi: 10.15302/J-FASE-2020380
de Moraes, A., de Faccio Carvalho, P. C. F., Anghinoni, I., Lustosa, S. B. C., Costa, S. E. V. G.A., and Kunrath, T. R. (2014). Integrated crop- livestock systems in the Brazilian subtropics. Eur. J. Agron. 57, 4–9. doi: 10.1016/j.eja.2013.10.004
de Oliveira, C. A. O., Bremm, C., Anghinoni, I., de Moraes, A., Kunrath, T. R., and de Faccio Carvalho, P. C. (2013). Comparison of an integrated crop–livestock system with soybean only: economic and production responses in southern Brazil. Renewable Agric. Food Syst. 29, 230–238. doi: 10.1017/S1742170513000410
de Souza Filho, W., de Albuquerque Nunes, P. A., Barro, R. S., Kunrath, T. R., de Almeida, G. M., Genro, T. C. M., et al. (2019). Mitigation of enteric methane emissions through pasture management in integrated crop-livestock systems: trade-offs between animal performance and environmental impacts. J. Clean. Prod. 213, 968–975. doi: 10.1016/j.jclepro.2018.12.245
de Souza, E. D., Costa, S. E. V. G.A., Anghinoni, I., de Faccio Carvalho, P. C., de Oliveira, E. V. F., Martins, A. P., et al. (2010b). Soil agregation in a crop-livestock integration system under no-tillage. Rev. Bras. Cienc. Solo 34, 1365–1374. doi: 10.1590/S0100-06832010000400033
de Souza, E. D., Costa, S. E. V. G.A., Anghinoni, I., de Lima, C. V. S., de Faccio Carvalho, P. C., and Martins, A. P. (2010a). Biomassa microbiana do solo em sistema de integração lavoura-pecuária em plantio direto, submetido a intensidades de pastejo. Rev. Bras. Cienc. Solo 34, 79–88 doi: 10.1590/S0100-06832010000100008
Deiss, L., Moraes, A., Dieckow, J., Franzluebbers, A. J., Gatiboni, L. C., Sassaki, G. I., et al. (2016). Soil phosphorus compounds in integrated crop-livestock systems of subtropical Brazil. Geoderma 274, 88–96. doi: 10.1016/j.geoderma.2016.03.028
Denardin, L. G. O., Martins, A. P., Carmona, F. C., Veloso, M. G., Carmona, G. I., de, F., et al. (2020). Integrated crop–livestock systems in paddy fields: new strategies for flooded rice nutrition. Agron. J. 112, 2219–2229. doi: 10.1002/agj2.20148
Dominschek, R., Barroso, A. A. M., Lang, C. R., Moraes, A., Sulc, R. M., and Schuster, M. Z. (2021). Crop rotations with temporary grassland shifts weed patterns and allows herbicide-free management without crop yield loss. J. Clean Prod. 306:127140. doi: 10.1016/j.jclepro.2021.127140
Dubeux, J. C. B. Jr., Stewart, R. L. Jr., Sollenberger, L. E., Vendramini, J. M. B., and Interrante, S. M. (2006). Spatial heterogeneity of herbage response to management intensity in continuously stocked Pensacola bahiagrass pastures. Agron. J. 98, 1453–1459. doi: 10.2134/agronj2005.0246
Duffy, J. E., Cardinale, B. J., France, K. E., McIntyre, P. B., Thébault, E., and Loreau, M. (2007). The functional role of biodiversity in ecosystems: incorporating trophic complexity. Ecol. Lett. 10, 522–538. doi: 10.1111/j.1461-0248.2007.01037.x
Duprat, P., Thiechart, M., and Sarthou, J.-P. (2018). Associated biodiversity: Definition. Dictionnaire d'Agroecologie. Available online at: https://dicoagroecologie.fr/en/encyclopedia/associated-biodiversity/ (accessed April 01, 2021).
Enri, S. R., Probo, M., Farruggia, A., Lanore, L., Blanchetete, A., and Dumont, B. A. (2017). A biodiversity-friendly rotational grazing system enhancing flower-visiting insect assemblages while maintaining animal and grassland productivity. Agric. Ecosyst. Environ. 241, 1–10. doi: 10.1016/j.agee.2017.02.030
Etienne, M. (2003). SYLVOPAST: a multiple target role-playing game to assess negotiation processes in sylvopastoral management planning. J. Artif. Soc. Soc. Simul. 6:5.
Fagerholm, N., Martín-López, B., Torralba, M., Oteros-Rozas, E., Lechner, A. M., Bieling, C., et al. (2020). Perceived contributions of multifunctional landscapes to human well-being: evidence from 13 European sites. People Nat. 2, 217–234. doi: 10.1002/pan3.10067
Fanin, N., Gundale, M. J., Farrell, M., Ciobanu, M., Baldock, J. A., Nilsson, M. C., et al. (2018). Consistent effects of biodiversity loss on multifunctionality across contrasting ecosystems. Nat. Ecol. Evol. 2, 269–278. doi: 10.1038/s41559-017-0415-0
FAO (2010). An International Consultation on Integrated Crop-Livestock Systems for Development: The Way Forward for Sustainable Production Intensification. Integrated Crop Management, Vol. 13. Rome: FAO. 64p.
Farias, G. D., Dubeux, J. C. B. Jr, Savian, J. V., Duarte, L. P., Martins, A. P., Tiecher, T., et al. (2020). Integrated crop-livestock system with system fertilization approach improves food production and resource-use efficiency in agricultural lands. Agron. Sustain. Dev. 40:39. doi: 10.1007/s13593-020-00643-2
Foley, J. A., DeFries, R., Asner, G. P., Barford, C., Bonan, G., Carpenter, S. R., et al. (2005). Global consequences of land use. Science 309, 570–574. doi: 10.1126/science.1111772
Foley, J. A., Ramankutty, N., Brauman, K. A., Cassidy, E. S., Gerber, J. S., Johnston, M., et al. (2011). Solutions for a cultivated planet. Nature 478, 337–342. doi: 10.1038/nature10452
Franzluebbers, A. J., and Stuedemann, J. A. (2008). Early response of soil organic fractions to tillage and integrated crop–livestock production. Soil Sci. Soc. Am. J. 72, 613–625. doi: 10.2136/sssaj2007.0121
Freiberg, J. A., Dambros, C. S., Rodrigues, E. N. L., Teixeira, R. A., Vieira, A. D. H. N., de Almeida, H. S., et al. (2020). Increased grazing intensity in pastures reduces the abundance and richness of ground spiders in an integrated crop-livestock system. Agron. Sustain. Dev. 40:1. doi: 10.1007/s13593-019-0604-0
Garrett, R. D., Ryschawy, L. W., Bell, O., Cortner, J., Ferreira Garik, A. V. N., et al. (2020). Drivers of decoupling and recoupling of crop and livestock systems at farm and territorial scales. Ecol. Soc. 25:art24. doi: 10.5751/ES-11412-250124
Garrett, R. D., Niles, M. T., Gil, J. D. B., Gaudin, A., Chaplin-Kramer, R., Assmann, A., et al. (2017). Social and ecological analysis of commercial integrated crop livestock systems: current knowledge and remaining uncertainty. Agric. Syst. 155, 136–146. doi: 10.1016/j.agsy.2017.05.003
Gaudin, A. C., Tolhurst, T. N., Ker, A. P., Janovicek, K., Tortora, C., Martin, R. C., et al. (2015). Increasing crop diversity mitigates weather variations and improves yield stability. PLoS ONE 10:e0113261. doi: 10.1371/journal.pone.0113261
Gerber, P. J., Steinfeld, H., Henderson, B., Mottet, A., Opio, C., Dijkman, J., et al. (2013). Tackling Climate Change Through Livestock: A Global Assessment of Emissions and Mitigation Opportunities. Rome: FAO.
Gil, J. D. B., Garrett, R., and Berger, T. (2016). Determinants of crop-livestock integration in Brazil: evidence from the household and regional levels. Land Use Policy 59, 557–568. doi: 10.1016/j.landusepol.2016.09.022
Guzman, A., Montes, M., Hutchins, L., DeLaCerda, G., Yang, P., Kakouridis, A., et al. (2021). Crop diversity enriches arbuscular mycorrhizal fungal communities in an intensive agricultural landscape. New Phytol. 231, 447–459. doi: 10.1111/nph.17306
Hooper, D. U., Chapin Iii, F. S., Ewel, J. J., Hector, A., Inchausti, P., Lavorel, S., et al. (2005). Effects of biodiversity on ecosystem functioning: a consensus of current knowledge. Ecol. Monogr. 75, 3–35. doi: 10.1890/04-0922
IPCC (2006). Good Practice Guidance on Land Use Change and Forestry in National Greenhouse Gas Inventories. Tokyo: Intergovernmental Panel on Climate Change.
Jacoboski, L. I., Paulsen, R. K., and Hartz, S. M. (2017). Bird-grassland associations in protected and non-protected areas in southern Brazil. Perspect. Ecol. Conserv. 15, 109–114. doi: 10.1016/j.pecon.2017.05.002
Jouan, J., De Graeuwe, M., Carof, M., Baccar, R., Bareille, N., Bastian, S., et al. (2020). Learning interdisciplinarity and systems approaches in agroecology: experience with the serious game SEGAE. Sustainability 12:4351. doi: 10.3390/su12114351
Katsvairo, T. W., Wright, D. L., Marois, J. J., Hartzog, D. L., Rich, J. R., and Wiatrak, P. J. (2006). Sod–livestock integration into the peanut–cotton rotation: a systems farming approach. Agron. J. 98, 1156–1171. doi: 10.2134/agronj2005.0088
Kirkegaard, J. A., Sprague, S. J., Hamblin, P. J., Graham, J. M., and Lilley, J. M. (2012). Refining crop and livestock management for dual-purpose spring canola (Brassica napus). Crop Pasture Sci. 63, 429–443. doi: 10.1071/CP12163
Kremen, C., and Merenlender, A. M. (2018). Landscapes that work for biodiversity and people. Science 362:eaau6020. doi: 10.1126/science.aau6020
Kunrath, T. R., de Albuquerque Nunes, P. A., de Souza Filho, W., Cadenazzi, M., Bremm, C., Martins, A. P., et al. (2020). Sward height determines pasture production and animal performance in a long-term soybean-beef cattle integrated system. Agr. Syst. 177:102716. doi: 10.1016/j.agsy.2019.102716
Laca, E. A. (2021). Multi-scape interventions to match spatial scales of demand and supply of ecosystem services. Front. Sustain. Food Syst. 4:289. doi: 10.3389/fsufs.2020.607276
Lemaire, G., Gastal, F., Franzluebbers, A., and Chabbi, A. (2015). Grassland-cropping rotations: an avenue for agricultural diversification to reconcile high production with environmental quality. Environ. Manage. 56, 1065–1077. doi: 10.1007/s00267-015-0561-6
Lindborg, R., Gordon, L. J., Malinga, R., Bengtsson, J., Peterson, G., Bommarco, R., et al. (2017). How spatial scale shapes the generation and management of multiple ecosystem services. Ecosphere 8:e01741. doi: 10.1002/ecs2.1741
Lovell, S. T., and Johnston, D. M. (2009). Creating multifunctional landscapes: how can the field of ecology inform the design of the landscape? Front. Ecol. Environ. 7, 212–220. doi: 10.1890/070178
Martins, A. P., Costa, S. E. V. G.A., Anghinoni, I., Kunrath, T. R., Balerini, F., Cecagno, D., et al. (2014). Soil acidification and basic cation use efficiency in an integrated no-till crop–livestock system under different grazing intensities. Agric. Ecosyst. Environ. 195, 18–28. doi: 10.1016/j.agee.2014.05.012
Martins, A. P., Denardin, L. G. O., Borin, J. B. M., Carlos, F. S., Barros, T., Ozório, D. V. B., et al. (2017). Short-term impacts on soil-quality assessment in alternative land uses of traditional paddy fields in southern Brazil. Land Degrad. Dev. 28, 534–542. doi: 10.1002/ldr.2640
Millennium Ecosystem Assessment (2005). Ecosystems and Human Well-being: Biodiversity Synthesis. Washington DC: World Resources Institute.
Modernel, P., Rossing, W. A., Corbeels, M., Dogliotti, S., Picasso, V., and Tittonell, P. (2016). Land use change and ecosystem service provision in Pampas and Campos grasslands of southern South America. Environ. Res. Lett. 11:113002. doi: 10.1088/1748-9326/11/11/113002
Moojen, F. G. (2021). A Co-design Methodology to Reconnect Crop and Livestock in Agricultural Systems: Farm Coaching, Serious Games and Advisory Roles on the Development of Innovative Ideas. (thesis). [Porto Alegre (RS)]: Federal University of Rio Grande do Sul.
Moonen, A. C., and Bàrberi, P. (2008). Functional biodiversity: an agroecosystem approach. Agric. Ecosyst. Environ. 127, 7–21. doi: 10.1016/j.agee.2008.02.013
Moraine, M., Duru, M., and Therond, O. (2017). A social-ecological framework for analyzing and designing integrated crop–livestock systems from farm to territory levels. Renew. Agric. Food Syst. 32, 43–56. doi: 10.1017/S1742170515000526
Mori, A. S., Furukawa, T., and Sasaki, T. (2013). Response diversity determines the resilience of ecosystems to environmental change. Biol. Rev. 88, 349–364. doi: 10.1111/brv.12004
Niles, M. T., Garrett, R. D., and Walsh, D. (2017). Ecological and economic benefits of integrating sheep into viticulture production. Agron. Sustain. Dev. 38:1. doi: 10.1007/s13593-017-0478-y
Odum, E. P. (1969). The strategy of ecosystem development. Science 164, 262–270. doi: 10.1126/science.164.3877.262
Orford, K. A., Murray, P. J., Vaughan, I. P., and Memmott, J. (2016). Modest enhancements to conventional grassland diversity improve the provision of pollination services. J. Appl. Ecol. 53, 906–915. doi: 10.1111/1365-2664.12608
Paramesh, V., Sreekanth, G. B., Chakurkar, E. B., Chethan Kumar, H. B., Gokuldas, P., Manohara, K. K., et al. (2020). Ecosystem network analysis in a smallholder integrated crop–livestock system for coastal lowland situation in tropical humid conditions of India. Sustainability 12:5017. doi: 10.3390/su12125017
Peterson, C. A., Bell, L. W., de Faccio Carvalho, P. C., and Gaudin, A. C. M. (2020). Resilience of an integrated crop–livestock system to climate change: a simulation analysis of cover crop grazing in Southern Brazil. Front. Sustain. Food Syst. 4:604099. doi: 10.3389/fsufs.2020.604099
Peterson, C. A., Eviner, V. T., and Gaudin, A. C. M. (2018). Ways forward for resilience research in agroecosystems. Agric. Syst. 162, 19–27. doi: 10.1016/j.agsy.2018.01.011
Piñeiro, G., Pinto, P., Arana, S., Sawchik, J., Díaz, J. I., Gutiérrez, F., et al. (2014). Cultivos de Servicio: integrando la ecología con la producción agrícola. XXVI Reunión Argentina de Ecología. Chubut, Argentina: Universidad Nacional de la Patagonia San Juan Bosco, 02–05.
Pinto, P., Long, M. E. F., and Piñeiro, G. (2017). Including cover crops during fallow periods for increasing ecosystem services: is it possible in croplands of Southern South America? Agric. Ecosyst. Environ. 248, 48–57. doi: 10.1016/j.agee.2017.07.028
Portugal, T. B., Campos, B. M., Martin, D. M., Moraes, R. F., Schuster, M. Z., Szymczak, L. S., et al. (2018). “Componente pastoril em sistema integrado de produção agropecuária em área de proteção ambiental,” in Boletim Técnico do Núcleo de Inovação Tecnológica em Agropecuária, eds R. Dominschek, S. Kruchelski, L. Deiss, T. B. Portugal, L. G. O. Denardin, A. P. Martins, et al. (Curitiba, PR: UFPR), 59–77.
Prevedello, J. A., Almeida-Gomes, M., and Lindenmayer, D. B. (2018). The importance of scattered trees for biodiversity conservation: a global meta-analysis. J. Appl. Ecol. 55, 205–214. doi: 10.1111/1365-2664.12943
Price, R. J., Nicholson, C., and McGuckian, N. (2009). Contesting targets as a measurement of success in agricultural extension: a case study of the Grain & Graze Change-on-farm strategy. Anim. Prod. Sci. 49, 941–955. doi: 10.1071/AN09034
Rakkar, M. K., Blanco-Canqui, H., Drijber, R. A., Drewnoski, M. E., MacDonald, J. C., and Klopfenstein, T. (2017). Impacts of cattle grazing of corn residues on soil properties after 16 years. Soil Sci. Soc. Am. J. 81, 414–424. doi: 10.2136/sssaj2016.07.0227
Ramos, M. E., Robles, A. B., Sanchez-Navarro, A., and Gonzalez-Rebollar, J. L. (2011). Soil responses to different management practices in rainfed orchards in semiarid environments. Soil Tillage Res. 112, 85–91. doi: 10.1016/j.still.2010.11.007
Rao, S., and Stephen, W. P. (2009). Bumble bee pollinators in red clover seed production. Crop Sci. 49, 2207–2214. doi: 10.2135/cropsci2009.01.0003
Reicosky, D. C., Calegari, A., dos Santos, D. R., and Tiecher, T. (2021). “Cover crop mixes for diversity, carbon and conservation agriculture,” in Cover Crops and Sustainable Agriculture, eds R. Islam and B. Sherman (Boca Ratón, FL: CRC Press), 169–208.
Ritchie, M. E., and Olff, H. (1999). Spatial scaling laws yield a synthetic theory of biodiversity. Nature 400, 557–560. doi: 10.1038/23010
Roese, A. D., Zielinski, E. C., and May De Mio, L. L. (2020). Plant diseases in afforested crop-livestock systems in Brazil. Agric. Syst. 185:102935. doi: 10.1016/j.agsy.2020.102935
Rundlöf, M., Lundin, O., and Bommarco, R. (2018). Annual flower strips support pollinators and potentially enhance red clover seed yield. Ecol. Evol. 8, 7974–7985. doi: 10.1002/ece3.4330
Russelle, M. P., Entz, M. H., and Franzluebbers, A. J. (2007). Reconsidering integrated crop–livestock systems in North America. Agron. J. 99, 325–334. doi: 10.2134/agronj2006.0139
Ryschawy, J., Joannon, A., Choisis, J. P., Gibon, A., and Le Gal, P. Y. (2014). Participative assessment of innovative technical scenarios for enhancing sustainability of French mixed crop-livestock farms. Agric. Syst. 129, 1–8. doi: 10.1016/j.agsy.2014.05.004
Ryschawy, J., Tiffany, S., Gaudin, A., Niles, M. T., and Garrett, R. D. (2021). Moving niche agroecological initiatives to the mainstream: a case-study of sheep-vineyard integration in California. Land Use Policy 109:105680. doi: 10.1016/j.landusepol.2021.105680
Ryschawy, J., Martin, G., Moraine, M., Charmeau, A., and Pelletier, A. (2018). Using the serious game Dynamix to design trade relationships among grain and livestock farmers in Ariège. Fourrages 2018, 207–212.
Salvini, G., van Paassen, A., Ligtenberg, A., Carrero, G. C., and Bregt, A. K. (2016). A role-playing game as a tool to facilitate social learning and collective action towards Climate Smart Agriculture: lessons learned from Apuí, Brazil. Environ. Sci. Policy 63, 113–121. doi: 10.1016/j.envsci.2016.05.016
Sanderson, M. A., Archer, D., Hendrickson, J., Kronberg, S., Liebig, M., Nichols, K., et al. (2013). Diversification and ecosystem services for conservation agriculture: Outcomes from pastures and integrated crop–livestock systems. Renew. Agric. Food Syst. 28, 129–144. doi: 10.1017/S1742170512000312
Schmitt, J., Portela, V. O., Santana, N. A., Freiberg, J. A., Bell,é, C., Pacheco, D., et al. (2021). Effect of grazing intensity on plant-parasitic nematodes in an integrated crop–livestock system with low plant diversity. Appl. Soil Ecol. 163:103908. doi: 10.1016/j.apsoil.2021.103908
Schuster, M. Z., Pelissari, A., de Moraes, A., Harrison, S. K., Sulc, R. M., Lustosa, S. B. C., et al. (2016). Grazing intensities affect weed seedling emergence and the seed bank in an integrated crop livestock system. Agric. Ecosyst. Environ. 232, 232–239. doi: 10.1016/j.agee.2016.08.005
Sekaran, U., Kumar, S., and Gonzalez-Hernandez, J. L. (2021). Integration of crop and livestock enhanced soil biochemical properties and microbial community structure. Geoderma 381:114686. doi: 10.1016/j.geoderma.2020.114686
Soussana, J.-F., and Lemaire, G. (2014). Coupling carbon and nitrogen cycles for environmentally sustainable intensification of grasslands and crop-livestock systems. Agric. Ecosyst. Environ. 190, 9–17. doi: 10.1016/j.agee.2013.10.012
Stark, F., González-García, E., Navegantes, L., Miranda, T., Poccard-Chapuis, R., Archimède, H., et al. (2018). Crop-livestock integration determines the agroecological performance of mixed farming systems in Latino-Caribbean farms. Agron. Sustain. Dev. 38:4. doi: 10.1007/s13593-017-0479-x
Szymczak, L. S., de Faccio Carvalho, P. C., Lurette, A., de Moraes, A., de Albuquerque Nunes, P. A., Martins, A. P., et al. (2020). System diversification and grazing management as resilience-enhancing agricultural practices: the case of crop-livestock integration. Agric. Syst. 184:102904. doi: 10.1016/j.agsy.2020.102904
Tilman, D., Fargione, J., Wolff, B., D'antonio, C., Dobson, A., Howarth, R., et al. (2001). Forecasting agriculturally driven global environmental change. Science 292, 281–284. doi: 10.1126/science.1057544
Tittonell, P. (2020). Assessing resilience and adaptability in agroecological transitions. Agric. Syst. 184:102862. doi: 10.1016/j.agsy.2020.102862
Tonitto, C., David, M. B., and Drinkwater, L. E. (2006). Replacing bare fallows with cover crops in fertilizer-intensive cropping systems: a meta-analysis of crop yield and N dynamics. Agric. Ecosyst. Environ. 112, 58–72. doi: 10.1016/j.agee.2005.07.003
Tracy, B. (2007). “The ecology of integrated crop-livestock systems,” in Proceedings of the International Symposium on Integrated Crop-Livestock Systems, eds A. de Moraes and P. C. de Faccio Carvalho (Curitiba, PR: UFPR), 1–10.
Ulanowicz, R. E., Goerner, S. J., Lietaer, B., and Gomez, R. (2009). Quantifying sustainability: resilience, efficiency and the return of information theory. Ecol. Complex. 6, 7–36. doi: 10.1016/j.ecocom.2008.10.005
Van Oijen, M., Barcza, Z., Confalonieri, R., Korhonen, P., Kröel-Dulay, G., Lellei-Kovács, E., et al. (2020). Incorporating biodiversity into biogeochemistry models to improve prediction of ecosystem services in temperate grasslands: review and roadmap. Agronomy 10:259. doi: 10.3390/agronomy10020259
Van Rijn, P. C. J., and Wäckers, F. L. (2016). Nectar accessibility determines fitness, flower choice and abundance of hoverflies that provide natural pest control. J. Appl. Ecol. 53, 925–933. doi: 10.1111/1365-2664.12605
Verhoeven, J. T., Arheimer, B., Yin, C., and Hefting, M. M. (2006). Regional and global concerns over wetlands and water quality. Trends Ecol. Evol. 21, 96–103. doi: 10.1016/j.tree.2005.11.015
Villarino, S. H., Pinto, P., Jackson, R. B., and Piñeiro, G. (2021). Plant rhizodeposition: a key factor for soil organic matter formation in stable fractions. Sci. Adv. 7:eabd3176. doi: 10.1126/sciadv.abd3176
Wezel, A., Casagrande, M., Celette, F., Vian, J. F., Ferrer, A., and Peign,é, J. (2014). Agroecological practices for sustainable agriculture. A review. Agron. Sustain. Dev. 34, 1–20. doi: 10.1007/s13593-013-0180-7
Wilson, C. H., Strickland, M. S., Hutchings, J. A., Bianchi, T. S., and Flory, S. L. (2018). Grazing enhances belowground carbon allocation, microbial biomass, and soil carbon in a subtropical grassland. Global Change Biol. 24, 2997–3009. doi: 10.1111/gcb.14070
Wittwer, R. A., Dorn, B., Jossi, W., and van der Heijden, M. G. (2017). Cover crops support ecological intensification of arable cropping systems. Sci. Rep. 7, 1–12. doi: 10.1038/srep41911
Wood, S. L., Jones, S. K., Johnson, J. A., Brauman, K. A., Chaplin-Kramer, R., Fremier, A., et al. (2018). Distilling the role of ecosystem services in the sustainable development goals. Ecosyst. Serv. 29, 70–82. doi: 10.1016/j.ecoser.2017.10.010
Keywords: biodiversity, ecosystem services, foodscapes, integrated crop-livestock systems, mixed crop-livestock, resilience
Citation: de Faccio Carvalho PC, de Albuquerque Nunes PA, Pontes-Prates A, Szymczak LS, de Souza Filho W, Moojen FG and Lemaire G (2021) Reconnecting Grazing Livestock to Crop Landscapes: Reversing Specialization Trends to Restore Landscape Multifunctionality. Front. Sustain. Food Syst. 5:750765. doi: 10.3389/fsufs.2021.750765
Received: 31 July 2021; Accepted: 27 September 2021;
Published: 21 October 2021.
Edited by:
Pablo Gregorini, Lincoln University, New ZealandReviewed by:
Pablo Chilibroste, Universidad de la República, UruguayAnita Fleming, Lincoln University, New Zealand
Copyright © 2021 de Faccio Carvalho, de Albuquerque Nunes, Pontes-Prates, Szymczak, de Souza Filho, Moojen and Lemaire. This is an open-access article distributed under the terms of the Creative Commons Attribution License (CC BY). The use, distribution or reproduction in other forums is permitted, provided the original author(s) and the copyright owner(s) are credited and that the original publication in this journal is cited, in accordance with accepted academic practice. No use, distribution or reproduction is permitted which does not comply with these terms.
*Correspondence: Paulo César de Faccio Carvalho, cGF1bG9jZmMmI3gwMDA0MDt1ZnJncy5icg==