- 1Department of International Health, Bloomberg School of Public Health, Johns Hopkins University, Baltimore, MD, United States
- 2Nitze School of Advanced International Studies, Johns Hopkins University, Washington, DC, United States
- 3Charles H. Dyson School of Applied Economics and Management, Cornell University, Ithaca, NY, United States
- 4Department of Nutritional Sciences, School of Public Health, University of Michigan, Ann Arbor, MI, United States
- 5Department of Global Health and Population, Harvard T. H. Chan School of Public Health, Harvard University, Boston, MA, United States
- 6Berman Institute of Bioethics, Johns Hopkins University, Baltimore, MD, United States
Food systems have a profound impact on diets, nutrition, health, economic development, and environmental sustainability. Yet their complexity poses a persistent challenge in identifying the policy actions that are needed to improve human and planetary health outcomes. Typologies are a useful classification tool to identify similarities and differences among food systems, while reducing this analytical complexity. This study presents a new food system typology, implemented at the country level using parsimonious data that characterize food supply chains, food environments, consumer-related factors, and key outcomes, including dietary intake, nutritional status, health, and environmental impacts. Five food system types are identified: rural and traditional; informal and expanding; emerging and diversifying; modernizing and formalizing; and industrial and consolidated. Patterns across the five system types in key outcome variables align with narratives provided by the food systems and nutrition transition literature, demonstrating the usefulness of this classification method. Substantial heterogeneity nonetheless still exists within individual food system types. Therefore, the recommended use of the typology is in early stages of hypothesis generation, to identify potential risk factors or constraints in the food system that can be explored further at national and sub-national levels.
Introduction
In the past decade, the critical role of food systems in shaping human and planetary health has been firmly established (Popkin et al., 2012; Springmann et al., 2018; Willett et al., 2019). This has led policymakers to seek out actions to reorient their food systems toward healthier, more sustainable outcomes, while continuing to promote socio-economic development, resilience, and poverty reduction. However, conceptual issues in characterizing food systems and their various components have challenged global and national discussions. These issues stem in part from the complexity of food systems, which are defined as the combination of all the diverse elements and processes involved in producing, processing, distributing, marketing, preparing, and consuming food. These elements and processes also vary over time and across spatial scales (HLPE, 2017).
Typologies are a classification tool that can support researchers and policymakers in conceptualizing and analyzing food systems. In their most basic sense, typologies offer a system for ordering and grouping entities according to their similarities. Statistically, the goal is to minimize variation within a group and maximize variation between groups (Bailey, 1994). Once grouped according to a clear set of criteria, the reduced dimensionality in the data makes it easier to compare and contrast entities, thus enhancing and accelerating learning. When applied to national food systems, a typology can help to identify countries with similar food systems that may be more likely to share common drivers of dietary, economic, and environmental change and be responsive to similar policy actions or technological or institutional innovations. It can likewise help demarcate the boundaries beyond which lessons from one context may not translate well to another.
Efforts to classify types of agriculture have existed since the late 19th century and in the 1960s, the International Geographical Union led a Commission on Agricultural Typology, which had the goal of systematically ordering knowledge of agricultural geography to better inform agricultural development programs (Kostrowicki, 1977). While at the time the concept of a food system was not fully formed, a systems lens was being applied to agriculture and typologies were deemed appropriate for synthesizing the multi-dimensional, interconnected qualities of a system. Given growing interest in broader systems framings, and evidence that most of the revenue and many of the environmental and social impacts of consumer food purchases accrue downstream from farms, the time is ripe to develop food systems typologies (Yi et al., 2021).
A preliminary scoping review was conducted to assess how previous typologies related to food systems were developed. Searches were conducted during the summer of 2018 and again in fall 2020 in PubMed, Web of Science, and Scopus databases (see search terms used in Appendix 1, Supplementary Material). The review sought to explore the different components of food systems that typologies aimed to characterize, the variables used to define the typologies, and the methods used to develop typologies, with the goal of adapting and building upon elements that were appropriate for the current study.
The search yielded 317 relevant articles, which were complemented with food systems-related reports from Internet searches of the gray literature published in recent years. Illustrative examples of the typologies identified and the components of food systems they aimed to characterize are included in Table 1. One limitation of food systems research to date has been the tendency to focus narrowly on individual components of the system (e.g., agriculture, supply chains, or food environments), rather than the system as a whole (Sobal et al., 1998; Ingram, 2011). This trend is also present in food systems-related typologies, which most commonly focus on individual components of the food system. In the agricultural sector they have been used extensively to characterize farming households and patterns, while the relatively less developed field of food environment research has also made use of typologies to characterize neighborhoods or specific types of outlets where consumers shop for food.
Typologies describing food systems as a whole were less common and tended to utilize conceptual, non-empirical methods of categorizing food systems. Our intention was to build a typology that reflected food systems comprehensively, using available national data that could be operationalized for use in analysis. Quantitative methods were therefore deemed more appropriate than conceptual typologies, which present theorized (or ideal) food system types as opposed to empirical cases, often based on expert judgement (Bailey, 1994), and may not readily facilitate straightforward classification and utilization in analysis.
The approach we adopted was closest to that used in the Global Nutrition Report 2015: Actions and Accountability to Advance Nutrition and Sustainable Development (IFPRI, 2015) and the Committee on World Food Security's High-Level Panel of Experts (HLPE) report, Nutrition and Food Systems (HLPE, 2017). Both of those reports developed typologies that moved beyond agricultural systems, using a purposefully selected set of indicators to represent the broader food system, including production, food environment and consumers. We have aimed to improve on those previous efforts in both the selection of our typology indicators and in our methods of classification, as described in more detail in the Methods.
A prerequisite for developing a typology is the identification of a parsimonious set of core features that should be used to group individual elements, in our case countries. Variables that do not demonstrate sufficient heterogeneity across the sample of countries or do not address the diverse components of a food system may not generate an informative typology. The chosen variables should also be universally relevant for describing food systems across all types.
Typologies may mask meaningful heterogeneity in food systems, especially at smaller spatial scales than country-level. Though they must generate mutually exclusive classes, it is also true that there will always be some countries that are close to the boundary between two types. For these countries, there is more uncertainty with regard to classification in a given type, which may be sensitive to specific decisions related to the variables chosen, how thresholds are set, and how criteria are weighted. Typologies should therefore not replace local context analysis, but rather serve as a starting point for hypothesis generation of how food systems may better contribute to human and planetary health, and socio-economic outcomes.
This study presents a newly developed food systems typology, which is used to classify 155 countries into five food system types. The food system types are described and diet, nutrition, and environmental sustainability outcomes are compared across the typology as a means of exploring the patterns of different outcomes across food system transformations. This typology is currently featured on the GAIN and Johns (2020) and underpins the contextualized analysis of the Cornell Atkinson—Nature Sustainability expert panel on “Innovations to build sustainable, equitable, inclusive food value chains” (Barrett C. et al., forthcoming).
Methods
Two principal methodological challenges arise when constructing a quantitative typology: (1) the choice of food system dimensions to incorporate in the typology and the variables that should be used to measure those dimensions; and (2) the choice of technique for grouping countries into different categories based on those dimensions (Kostrowicki, 1977).
Choosing Typology Variables
This study relied on a limited number of purposefully selected variables to inform the typology. Several earlier food system typologies included in the scoping review, while discussing implications for the broader food system, including nutrition and health outcomes, were primarily informed by the agricultural transformation process and relied solely on agricultural employment and income per capita to classify countries (McCullough et al., 2010; Pingali et al., 2015). Other typologies, particularly those with a targeted focus on farming households or farming systems, utilized large quantities of indicators (Madry et al., 2013; Lopez-Ridaura et al., 2018). For the purposes of the study, this was not preferred for several reasons. First, a large number of variables would result in more missing data, which would reduce our sample size. Second, these methods frequently rely on more advanced statistical classification techniques to process large quantities of data that were disadvantageous due to the nature of our data (as discussed in the following section). Lastly, using a more parsimonious set of indicators facilitated clearer identification of the influence of each variable, and easier communication of results.
Four variables were identified to serve as the basis for the food systems typology. These variables were selected according to the following criteria: (1) the group of indicators chosen should reflect the different components of the food system; (2) the literature should support the indicators' association with food system patterns and transitions; and (3) indicators should have high global coverage, with availability for at least 100 countries, and reasonably balanced representation across different regions and income group classifications. Indicators that had been included in previous typologies from the scoping review were also prioritized.
A food systems conceptual framework was developed to guide implementation of the first criteria, shown in Figure 1. This framework was adapted for the Food Systems Dashboard from recent high-level reports that had broad input from researchers, representing different food systems-related disciplines, also incorporating more recent conceptual work drawing attention to the external and individual dimensions of the food environment (Global Panel on Agriculture Food Systems for Nutrition, 2016; HLPE, 2017; Turner et al., 2018). The framework includes as its core components food supply chains, food environments, consumers (made up of their individual factors and behaviors), as well as external drivers of food systems. Each of these components is also broken up into various sub-components. The goal for the typology was to select variables that would describe at least one key feature of each of the core components of the framework. Variables assessing diet, nutrition, and health were excluded from the construction of the typology because conceptually, these were considered as outcomes of food system processes as opposed to components within the food system.
Agricultural value added per worker (in constant 2010 USD) was selected to represent food supply chains in our typology. Improvements in agricultural productivity are a key driver of agricultural transformation, in which countries transition from having a large agricultural labor force with low productivity, to a reduced, yet more productive agricultural labor force, fueled by technological change, as workers join non-agricultural sectors (World Bank, 2008). Especially in the early stages of agricultural development, revenues from productivity gains are needed for reinvestment in agricultural research and supply chain infrastructure (Timmer, 1988). This indicator also has strong relationships with different malnutrition burdens, with forms of undernutrition high where agricultural productivity is low and obesity higher where productivity is high, but micronutrient deficiencies persisting even among countries with very high productivity (Gómez et al., 2013). This indicator was also selected for the Global Nutrition Report 2015 typology (IFPRI, 2015).
Food environments are addressed in the typology through two variables. The first reflects the diversity of foods available in a country, measured as the percent of dietary energy from cereals, roots, and tubers (i.e., staple foods). A lower percentage reflects greater availability of nutrient-dense non-staple foods, which low-income countries achieve mainly through diversification of production, while high and middle-income countries achieve more through trade (Remans et al., 2014). This indicator was also incorporated in the Global Nutrition Report 2015 and HLPE report typologies (IFPRI, 2015; HLPE, 2017).
The second variable measures the number of supermarkets (per 100,000 inhabitants) within a country, which can signal the stage of development of a country's modern food retail sector, as well as the well-documented influence of supermarkets in driving upstream changes in food supply chains, including farming, distribution, and processing (Reardon et al., 2003; Reardon and Timmer, 2007). Growth in modern food retail also typically predates and facilitates transformation of food service operations to accommodate increased consumption of food away from home (Barrett C. B. et al., forthcoming). This variable was not included in either the Global Nutrition Report 2015 or HLPE typologies (IFPRI, 2015; HLPE, 2017). Due to its strong basis in the food system and nutrition-transition-related literature, it fills an important gap.
The final variable is urban population as a percent of total population. While this is an external driver of food systems within the framework adopted, here it is used as a proxy measure for consumer-related factors. Urbanization is a powerful demographic driver of change to the built food system, lengthening food supply chains, and shifting the composition of food retailers and food products (Satterthwaite et al., 2010; Reardon and Zilberman, 2018; Barrett C. B. et al., forthcoming). Urbanization is associated with dramatic changes in consumer behaviors, reflected in lifestyles (e.g., greater female workforce participation and food consumption away from home) and shifts in food preferences (e.g., increased purchase of prepared foods) (Ranganathan et al., 2016; Barrett C. B. et al., forthcoming). Lacking standardized data on important characteristics of consumers within food systems, including situational factors such as their time resources and mobility, urbanization was viewed as reasonable approximation, which likely correlates with these consumer factors.
An ideal set of indicators to reflect the core dimensions of a food system might include other variables that are not part of our typology. For example, while supermarkets are a driving force in food systems transitions, the size and nature of the informal food sector may also be an important characteristic that distinguishes different classes of food systems. Food safety, availability of cold chains, and more detailed information about food processing and other stages of food supply chains are likewise important features. However, most of these elements are not well-characterized and data are not routinely gathered. Therefore, we believe the indicators included in this typology are the best set presently available.
The four variables included in the typology were sourced from the World Bank, the Food and Agriculture Organization (FAO) Food Balance Sheets, and Euromonitor International in October 2020 (FAO, 2016; Euromonitor International, 2018b; United Nations Population Division, 2019; United Nations Population Division and World Bank, 2019; World Bank OECD ILO, 2019). The sample of 155 countries includes only those for which data was available for all of the indicators.
Technique for Classifying Food System Types
Food system types were classified using a relatively simple quantitative method, based on ranking and scoring countries for each of the four chosen indicators. More advanced statistical methods, including principal component analysis (PCA), cluster analysis, and latent variable modeling have been used extensively in developing typologies for distinct components of food systems. Several of these methods were tested, but did not perform well, primarily due to skewed distributions (particularly for agricultural productivity and supermarkets), which resulted in food system types consisting of a very small number of outlier countries. Logarithmic or inverse-rank based transformations are possible, but using only four input variables, these methods also typically result in a smaller number of clusters (or types). For the current typology, it was decided a priori that five food system types was optimal and that fewer would fail to capture the heterogeneity that exists within regions, for example, regionally in Africa, where the expert judgment of the study team was that more than a single country-level food system type predominates.
Countries were first ranked from highest to lowest on each indicator, under the hypothesis that higher values were associated with more “modern” food systems, and lower values more “traditional” food systems. The ranking was inverted in the case of the share of dietary energy from cereals, roots, and tubers, which is theorized to be lower in more modern food systems.
A score for each country was assigned based on the sum of its ranks on each of the four indicators. For example, if a country ranked 10th on agriculture value added, 15th on share of dietary energy from cereals roots and tubers, 17th on number of supermarkets per 100,000 population, and 8th on urbanization, it received a score of 50. Once scores were calculated for each country, all countries were sorted from lowest score to highest score. The typology was then created by separating the distribution of scores into quintiles, with the lowest quintile representing the most modern food system type and the highest quintile representing the most traditional food system type.
Using this method, countries are organized into food system types according to their rank-based position relative to each other, rather than the absolute values for each of the typology variables, and there are roughly an equal number of countries in each food system type (ties result in slightly different numbers per type). An alternative method could derive equal intervals from the sample distribution and score each country according to the interval it falls within, however, this method is similarly constrained by skewed distributions. It should be noted that classification based on relative rankings means that the absolute thresholds separating food system types will shift over time; for example, our typology methods will continue to assign countries with lower relative percentages of urban population toward the “rural” end of the typology, but as global trends in urbanization continue, this group will be much less rural than it was at baseline, and likely more similar to the urban food system types. Therefore, these methods are most appropriate for classifying food system types at a given point in time and may also be repeated within short-time frames (e.g., 5 years) to monitor how individual countries change position relative to each other. Monitoring longer-term changes will require revisiting the definitions of the food system types that make up the typology, recognizing that global shifts in the baseline characteristics will have taken place.
The HLPE report used a similarly straightforward method of classifying countries into three food system types, wherein countries had a “modern” food system type if they were above the median on all four typology indicators that report included, a “traditional” food system if they were below the median on all four, and a “mixed” food system if they had a mix of above and below median values (HLPE, 2017). In contrast, the Global Nutrition Report 2015 ranked countries on each of their three typology indicators, then color-coded them by quartile, and visually inspected the distributions to delineate five food system types (IFPRI, 2015). The method used here is closest to that used by the Global Nutrition Report, but has attempted to use a clearer, more specific method for separating out the five food system types.
Stylized descriptions were also prepared for each of the food system types, based on published literature describing food system transitions as well as comparisons of specific variables across the food system types, as presented in the Results. These descriptions were primarily meant to give a more user-friendly narrative to complement the typology, for practitioners and policy makers visiting the Food System Dashboard. They are shared in Appendix 2, Supplementary Material which are reproduced from the Cornell Atkinson—Nature Sustainability expert panel on “Innovations to build sustainable, equitable, inclusive food value chains” (Barrett C. et al., forthcoming).
Results
After excluding countries with missing data, 155 countries were classified into five food system types: rural and traditional; informal and expanding; emerging and diversifying; modernizing and formalizing; and industrial and consolidated. These types are exhaustive and mutually exclusive. The countries included in the typology represent 97% of the global population, 93% of global land area, and 97% of global gross domestic product (GDP).
By design, the mean values for each typology variable follow a gradient from rural to industrial food system types, as shown in Table 2. These transitions are not linear. There are abrupt increases in agricultural productivity and supermarkets for modernizing and formalizing, and industrial and consolidated food system types, due to positive skewness in the distribution of those variables. As is common with typologies, some countries are located near the center of a given type, while others are closer to the margin between two different types. The similarities between food system types are evident by the overlapping interquartile ranges of urbanization, share of dietary energy from staples, and supermarkets.
Most regions of the world are characterized by multiple food system types (Figure 2). While there is a general trend toward more modernized food system types from lower-income regions to higher-income regions, and the vast majority of countries in Europe and North America have industrial and consolidated food system types, most regions of the world have several different types, reflecting the different stages of food system transformation that can be found even among countries that are geographically close to each other. Latin America and the Caribbean contain four food system types and all five can be found in the Middle East.
Industrial and consolidated food systems and modernizing and formalizing food systems account for a smaller share of the global population (13 and 11% respectively), yet occupy a larger share of global land area, while the other three types have a relatively larger share of the global population, with a smaller share of global land area (Figure 3). Nearly one-third of the global population lives in a country characterized as having a rural and traditional food system.
The four typology variables are each highly correlated with income, as measured by gross domestic product (GDP) per capita, with Spearman correlation coefficients ranging from |0.76| to |0.90|. There are well-established associations between income growth and structural changes to agriculture, processing, food trade, retail, and demand-side factors (e.g., food preferences and consumer food budget shares), in addition to its associations with broader socio-demographic transitions (Timmer, 1988; Popkin, 1999; Reardon et al., 2003; Ranganathan et al., 2016). The relationship between food system type and GDP per capita is also evident in Figure 31, by the increase in bubble sizes from the more rural types to more industrial types.
The question may be asked therefore, what additional insight can the food system typology offer beyond income group classification? The relationship between income growth and structural changes affecting the food system are not as straightforward as they may seem. For example, while agricultural productivity gains were a cornerstone of the economic development achieved by the United States and many Asian and European countries, they have been neither necessary nor sufficient for many developing countries embarking on modern development pathways (Timmer, 1988; Gollin, 2010). Similarly, many African countries have rapidly urbanized without experiencing sustained income growth (Fay and Opal, 2000). As shown in Table 3, there is an obvious trend from rural to modern food system types, moving from low to high-income groups. Not all high-income countries have industrial and consolidated food systems, however, and middle-income countries are especially heterogeneous in their food systems. This food system typology offers a more nuanced characterization of national food systems than income groups would alone, using variables that more directly measure it.
Validating the Food Systems Typology
Given the parsimonious nature of the typology, and in the absence of a true “gold standard” measure of a national food system, determining the extent to which the typology could reveal patterns in other food system variables that were not included in its development may offer a qualitative means of validating the typology. Data related to a variety of food supply chain and food environment-related factors were gathered and compared across the five food system types, as shown in Figure 42.
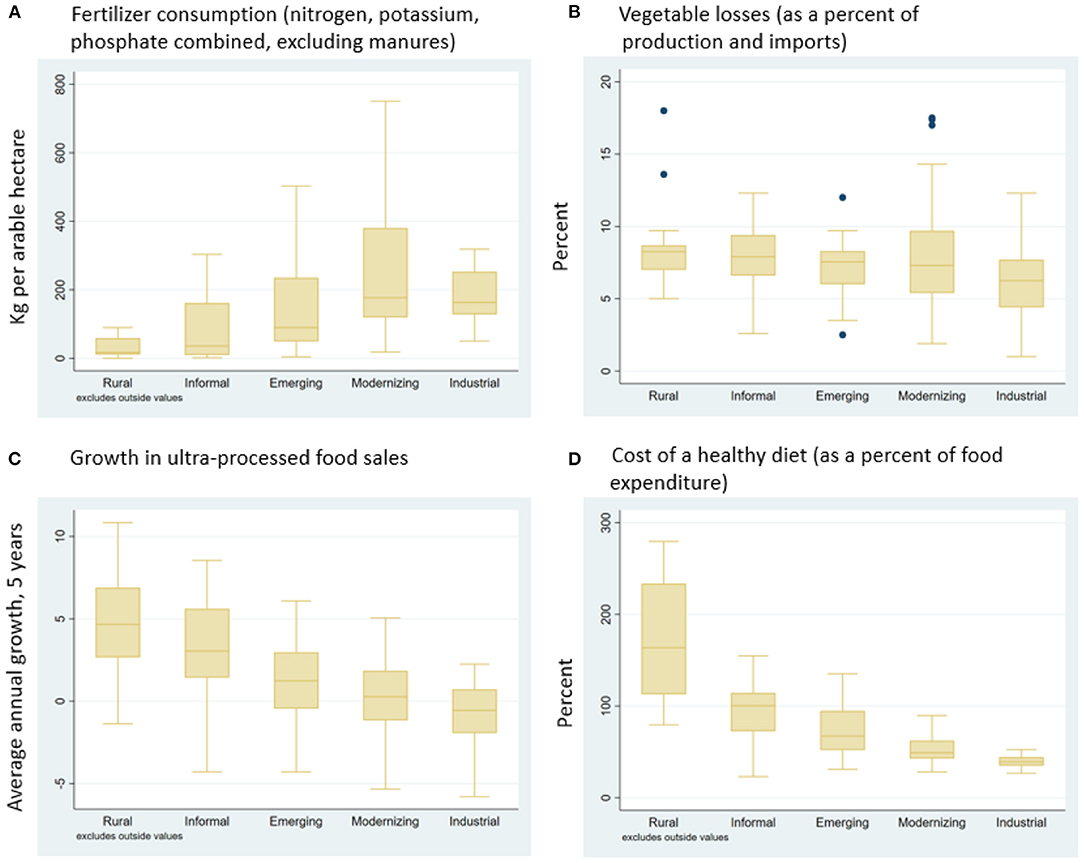
Figure 4. Comparison of supply chain and food environment variables across the food system types. Note that outliers are excluded from graphs (A,C,D).
Average fertilizer consumption increased from rural and traditional to modernizing and formalizing food system types, leveling off with industrial and consolidated food systems. However, there was large variation especially among emerging and diversifying and modernizing and formalizing food systems, and significant overlap in the distributions across each type. These wide and overlapping distributions held true for the comparison of vegetable losses as well, indicating less distinction between food system types. However, there was still a general declining trend, which should be expected given that cold chains typically emerge alongside supermarkets and modern retail (Reardon et al., 2003).
Average annual growth from 2013 to 2018 in the retail value of ultra-processed food sales was highest in rural and traditional food systems, with a median of 4.7%, steadily declining to −0.6% for industrial and consolidated food systems, where it is lowest. Though the number of supermarkets in rural and traditional food systems is the lowest compared to other food system types, this group contains many of the countries where the growth in modern retail is happening fastest, which fuels growth in sales of ultra-processed foods. Even in rural areas where supermarkets have yet to be established, these foods may reach consumers through modern-to-traditional food supply chains (Gomez and Ricketts, 2013).
The affordability of a healthy diet was compared using the cost of a healthy diet, based on the minimum cost of following national food-based dietary guidelines, as a percent of national average food expenditure (FAO, 2020). Analysis in the State of Food Security and Nutrition in the World 2020 found that healthy diets were most unaffordable in the Global South and in low-income countries (FAO, 2020). Here, nearly all countries with rural and traditional food systems have a cost of a healthy diet that is greater than food expenditure, meaning that the average income households have available for food is not sufficient to follow food-based dietary guidelines. In contrast, other food system types, especially the modernizing and formalizing and industrial and consolidated food systems groups, have healthy diets that are almost uniformly lower in cost than average food expenditure.
Variables describing diet, nutrition, health, or environmental sustainability outcomes were not used in the classification of countries into different food system types. However, we examined patterns in these outcomes across the typology and whether they aligned with hypothesized linkages in the food systems and nutrition transition literature. These comparisons are presented in Figure 53. Note that dietary intake estimates presented are derived from modeling, in the absence of comparable dietary intake data across countries (GBD 2017 Risk Factor Collaborators, 2018).
Median estimated vegetable intake among rural and traditional food systems was 62 grams per day, the lowest among the five types, while intake in the modernizing and formalizing type was 196 grams per day, the highest, and about 20 grams per day greater than industrial and consolidated countries. It should be noted that the median vegetable intake is below the WHO recommendation of 200 grams per day across all food system types. In contrast, estimated average red meat intake increased steadily over the five food system types, with industrial and consolidated countries estimated to be consuming a median of 48 grams per day. These patterns are consistent with the correlation between income and the food system typology, and Bennett's Law, which states that as income increases, households diversify their diets, spending more of their income on non-staples, such as vegetables and red meat. There was a nearly linear decrease in the average prevalence of anemia among women of reproductive age from countries in the rural and traditional food system type to industrial and consolidated food systems. The prevalence of adult obesity began to climb first among informal and expanding food system types, then was relatively comparable across emerging and diversifying, modernizing and formalizing, and industrial and consolidated food systems. That the largest increases in obesity prevalence take place in the earlier stages of food systems transitions may reflect the changing nature of the nutrition transition, now taking hold in developing countries at an accelerated pace (Popkin, 2012).
Lastly, a set of environmental outcomes were compared across the five food system types (Figure 6)4. Data assessing the ecological footprint of food production were obtained from the Global Footprint Network5. This measures the amount of biologically productive land and water required for all of the resources harvested and waste generated by a country, including from primary production, the built-up surfaces that support food production, and land needed to absorb carbon dioxide emissions from food production6 (Lin et al., 2019). The median ecological footprint of production increases from rural and traditional food systems to industrial and consolidated food systems. Its variance is especially high among industrial and consolidated food systems with a skewed distribution, indicating the presence of some countries with especially high footprints, despite a median which is similar to modernizing and formalizing types.
Measures of the environmental impact of food consumption were also compared across the typology, relating to several planetary boundaries analyzed by the EAT-Lancet Commission on Healthy Diets from Sustainable Food Systems, which define the safe operating space for food systems, including: climate change; freshwater use; and phosphorous and nitrogen cycling (Willett et al., 2019).
Greenhouse gas (GHG) emissions from food consumption are largely driven by animal-source foods, especially ruminant meat, which are more GHG-intensive than other foods (Poore and Nemecek, 2018; Willett et al., 2019; WWF, 2020). Therefore, the increasing trend in red meat consumption shown in Figure 5, panel b explains the gradual increase in GHG emissions from rural and traditional food systems to industrial and consolidated food systems. Variance within food system types partially results from heterogeneity in production efficiencies, due to varying production systems and management practices, with inefficiency also associated with higher GHG emissions. This is evident in the wide boxplots for rural and traditional, informal and expanding, and emerging and diversifying food systems, where inefficient low-productivity crop and livestock farming coexists alongside commercial farming. The smaller variance within industrial and consolidated food systems reflects the more uniformly commercial objectives of farms, with tighter profit margins that require more homogenous management practices and use of technology (Coelli, 1995).
Similarly, per capita water use from food consumption shows a positive trend from rural and traditional to industrial and consolidated food systems, which partially reflects the increasing volume of consumption and higher consumption of animal-source foods, which have a higher average water footprint than grains and most other plant-based foods (Hoekstra and Chapagain, 2007; Mekonnen and Hoekstra, 2010, 2011). Water withdrawals in more traditional food systems are primarily directed toward grains, though poor irrigation technology can result in higher evaporative losses, which may explain why many countries in rural, informal, and emerging food systems still have high water use per capita from food consumption (Jägermeyr et al., 2015).
Eutrophication from food consumption, measured as grams of phosphate equivalents per person, demonstrates the clearest pattern of increase along the typology. Nitrogen and phosphorous fertilizers, when used in excess, lead to runoff of these nutrients into streams, rivers, and coastal ecosystems, where they cause eutrophication, reducing oxygen levels and eventually causing dead zones to form, where aquatic life cannot survive (Diaz and Rosenberg, 2008). Fertilizer application is unevenly distributed globally. Industrial and consolidated food systems, as well as rapidly growing agricultural sectors in emerging and diversifying and modernizing and formalizing countries, have used fertilizer as a means of increasing yields and meeting food security objectives, often in excess of crop nutrient removals, while in many rural and traditional food systems, fertilizer application is still insufficient to restore nutrient-depleted soils (Vitousek et al., 2009). This partially explains crop yield gaps, as well as the pattern in eutrophication from food consumption we see in the food system typology.
Discussion
The complexity of food systems is evident in the conceptual frameworks that seek to depict them, which describe seemingly infinite elements, processes, and actors, and the interactions among them. The food system typology presented here provides a tool for reducing some of this complexity in the analysis of food systems. It does this first by classifying similar country-level food systems into mutually exclusive types, which can be compared as groups rather than carrying out large series of comparisons of individual countries. Second, it provides a composite characterization of food systems through use of a parsimonious set of variables that correlate well with other food system characteristics, which may be easier to compare than a large number of individual variables, when the goal is to compare food systems broadly, as systems rather than individual components.
The methods used produced a typology of five distinct food system types. A core set of four variables were purposefully selected to represent the key components of food systems, and a simple classification technique, using rank-based quintiles, separated 155 countries into these five types. The method of validation involved comparing the five food system types on other food system characteristics not used in the construction of the typology, and a range of diet, nutrition, health, and environmental sustainability outcomes. For the majority of between-type comparisons on these indicators, there was a reasonably limited amount of overlap between interquartile ranges (displayed in boxplots) and the patterns in median values aligned with relationships put forth in food systems transformation and nutrition transition literature.
In contrast with previous typologies developed in the fields of agriculture and food systems (noted in Table 1), this typology reflects the full set of related components that make up a food system, including supply chains, food environments, and consumers, to avoid overreliance on any individual component on its own. By utilizing quantitative methods for classification, it also provides an alternative to conceptual typologies, producing empirical cases that may be compared and utilized in statistical analyses. These quantitative methods also allow a more concrete assessment of how well-certain countries fit into a given type, whether they are located in the center of that type (i.e., the middle-range of an indicator distribution) or whether they are more on the margins. Two relatively recent typologies for food systems—the 2015 Global Nutrition Report and the HLPE Food Systems and Nutrition Report—also used similar quantitative methods (IFPRI, 2015; HLPE, 2017). However, this typology adds key dimensions that were not included in those previous typologies, particularly the size of the modern retail sector, as indicated by quantity of supermarkets, which has well-documented upstream and downstream influences on food systems. It also presents a more straightforward, transparent method of classification and attempts to systematically validate the typology through comparisons with a range of other variables.
Case Study: Positive Deviance in Mediterranean Food Environments
The typology groups together food systems that are relatively similar. However, meaningful heterogeneity still exists within these groups on individual characteristics, and one possible way to learn from the typology may be to identify cases of positive deviance. Ultra-processed foods are formulated by combining cheap, industrial sources of dietary energy and nutrients with additives meant to enhance palatability and convenience for consumers. They are energy-dense, often high in added sugar, salt, fats, and oils, and have been associated with obesity and cardiovascular disease (Monteiro et al., 2019). Industrial and consolidated food system types are likely to have the highest availability of ultra-processed foods (measured in our dataset as retail value of sales per capita), due to the influence of modern retail, among other drivers.
However, deeper analysis into the countries included in this group reveals that this is not uniformly true. Cyprus, Greece, Italy, and Spain all rank in the bottom 25th percentile, with ultra-processed food sales < USD $550 per capita, while countries above the 75th percentile, such as the United States, are roughly double this amount (Euromonitor International, 2020). An obvious commonality among the countries toward the lower end of this distribution is their concentration in the Mediterranean region. Unique features of the Mediterranean food environment have been hypothesized to contribute to adherence to the Mediterranean diet, including a diversity of food store types, such as food markets and small, specialized vendors of fruits and vegetables, fish, and other foods (Díez et al., 2019). Therefore, despite these countries still having high availability of supermarkets, consumers have a range of other options to choose from when shopping, which may enable easier access to foods that are typical of the Mediterranean diet, while limiting their exposure to ultra-processed foods commonly found in supermarkets.
Further analysis may also seek to identify differences in food-based dietary guidelines and other food-related messaging between countries with high vs. low ultra-processed food sales. For countries in transitioning food systems, which are yet to fully industrialize and consolidate, these analyses may yield helpful lessons about the potential risks and benefits of different food system transformation pathways, their effects on the make-up of food environments, and possible implications for diets.
A key limitation for the typology has been the spatial granularity of the data it is based on. Multiple food system types may coexist within a country. With more disaggregated data at the region or district-level, for example, the typology could also be implemented sub-nationally and may reveal some of this heterogeneity. However, lacking access to that subnational data, the typology could only be applied at the country-level and is therefore better interpreted as a characterization of the predominant food system type within a country and not the type that applies uniformly to all areas of a country. It may be of great value in the future to collect standardized indicators at finer spatial scales and more frequently in order to facilitate more granular characterization of food systems and cross-locational learning.
Another limitation is the extent to which the food system typology may explain patterns of environmental sustainability-related outcomes. The conceptual framework adopted sought primarily to depict food system elements and relationships that influence diet outcomes. While diets are extremely relevant for environmental outcomes, and shifting dietary patterns is a key lever for remaining within planetary boundaries, there are other key dimensions related to the production possibilities of land and natural resource availability that vary spatially, over agro-ecological zones and regions, and those are not incorporated in the construction of the typology.
Lastly, due to our use of relative rankings to score and classify countries, attempts to re-classify countries in the future will result in roughly the same number of countries within each food system type, but definitions (i.e., typology indicator ranges) that have shifted. Over a long period of time, these shifts from the baseline measurement may be so great that they warrant a re-assessment of the definitions of each food system type. Other indicators may also emerge in the future that reflect dimensions of the food system that have taken on new relevance.
This typology may serve as a useful tool for policymakers who are in the early stages of articulating food systems strategies for improving human and planetary health outcomes. It does this by providing a more targeted sample of countries from which national stakeholders can draw helpful comparisons. Successful policies and programs in countries identified as having the same or similar food system types may stand a greater chance of having positive impacts in their own context; in contrast, comparisons among a sample of unclassified countries may be less helpful due to the larger systemic differences that exist, even among countries from the same region. Policymakers may also use the typology to weigh the advantages and disadvantages of different food system types and identify benchmarks that can provide targets for future progress. For example, an analysis of vegetable intake could be conducted among countries in the same or neighboring food system types that would allow policymakers to identify policies, investments in socio-technical innovations, or other food system factors that have enabled some countries to achieve higher intake. This could similarly accelerate the exploration of barriers to vegetable intake in countries with weaker performance. Two case studies accompany this article to illustrate further the ways that the typology may facilitate policy analysis.
Transitioning toward the modern pole of the typology is not exclusively associated with desirable outcomes, as evidenced by the higher burden of obesity and larger environmental footprint of industrial and consolidated food systems. This typology should be used in parallel with more local context analysis, and more in-depth analysis into specific bottlenecks, in order for policymakers to chart optimal pathways of food systems transformation toward healthier, more sustainable diets.
Case Study: Cross-Country Comparisons of Vegetable Losses
The typology may also enhance learning from cross-country comparisons. As an example, Guinea-Bissau and Burkina Faso are both classified as having rural and traditional food system types and both are located in the West Africa region, with tropical climates, but they differ significantly in their performance on vegetable losses. In 2018, Guinea-Bissau experienced a post-harvest loss of 18% of the total vegetables it produced domestically and imported, while Burkina Faso only lost 6.8% (Food Agriculture Organization, 2018a,b). Exploring the reasons for this difference can be aided by the typology, which ensures comparisons within food system types that are more “apples-to-apples,” meaning that large structural differences are less likely to exist within two countries from the same group, and that differences that do exist may be more modifiable in shorter time periods.
Guinea-Bissau and Burkina Faso find themselves in relatively similar positions within the agricultural development process, with agricultural sectors that contribute a large percentage of national GDP and employ the majority of the labor force, which is made up primarily of smallholder farmers. Agricultural revenues are concentrated in single commodities in both countries, with 95% of Guinea-Bissau's exports coming from cashew nuts (Paviot et al., 2019) and 60% of Burkina Faso's agricultural exports made up of cotton (World Bank, 2017). Development strategies in both countries are focused on improving agricultural production as well as investing in infrastructure for better market access, with weak roads and limited access to electricity in rural areas posing as critical bottlenecks for growth.
Diversification of agriculture is also a priority in both countries, though Burkina Faso may have a head start. In recent years, it has experienced improvements in mango and onion yields, and has established itself as an exporter of these products to neighboring countries as well as international markets. These exports could grow further as Burkina Faso continues to invest in its “growth poles,” designated geographic areas where key infrastructure (electricity, irrigation, roads and railways) are brought together to strengthen value chains (IFC, 2019). Vegetable yields in Burkina Faso are also 9.8 tons per hectare, compared to 6.2 tonnes per hectare in Guinea-Bissau, and a regional average of 5.7 tons per hectare (FAO, 2020). These signs could point to a more market-oriented agricultural management approach that is taking hold in Burkina Faso, which may also work to reduce vegetable losses. Meanwhile, there are unique challenges Guinea-Bissau may be faced with, including a climate that is relatively more humid compared to the savannah regions of Burkina Faso, and climate change related shocks, including floods, that threaten its agriculture and infrastructure (Paviot et al., 2019).
No easy answers emerge from a desktop comparison of countries, however the typology can help point to areas that are worth exploring more in-depth. Vegetable losses may be affected by a range of agro-ecological and management-related factors, and policies and actions that have been successful in one country—be they large-scale infrastructure investments or incentives offered to smallholders—may be worth considering in countries within similar food system types. More contextualized analysis in-country is needed to identify these levers and evaluate their feasibility.
Data Availability Statement
The original contributions presented in the study are included in the article/Supplementary Material, further inquiries can be directed to the corresponding author.
Author Contributions
JF conceived of the presented idea and supervised the project. QM developed the typology by carrying out the scoping review, identifying appropriate methods, gathering data, conducting computations, led the writing of the manuscript, including producing tables, figures, writing case studies, incorporated feedback, and finalized the manuscript. JF, AJ, and AH provided feedback during the development of the methods. AJ and AH provided support in drafting the stylized typology descriptions. CB and JF provided edits on early drafts of the manuscript. JF, CB, AJ, AH, and RM provided feedback on revised drafts. All authors contributed to the article and approved the submitted version.
Funding
The Food Systems Dashboard and this work received support from the Nourish People and Planet Initiative at the Rockefeller Foundation. QM receives additional doctoral funding support from the Center for a Livable Future-Lerner Fellowship Program.
Conflict of Interest
The authors declare that the research was conducted in the absence of any commercial or financial relationships that could be construed as a potential conflict of interest.
Publisher's Note
All claims expressed in this article are solely those of the authors and do not necessarily represent those of their affiliated organizations, or those of the publisher, the editors and the reviewers. Any product that may be evaluated in this article, or claim that may be made by its manufacturer, is not guaranteed or endorsed by the publisher.
Acknowledgments
The authors are grateful to Mario Herrero for expertise and advice provided in the analysis of environmental impacts from food consumption.
Supplementary Material
The Supplementary Material for this article can be found online at: https://www.frontiersin.org/articles/10.3389/fsufs.2021.746512/full#supplementary-material
Footnotes
1. ^Data sources: Euromonitor International (2018a); United Nations Population Division and World Bank (2019); World Bank Eurostat-OECD (2019).
2. ^Data sources: Food and Agriculture Organization (2016); Euromonitor International (2018a); Food Agriculture Organization (2018b); FAO (2020).
3. ^Data sources: World Health Organization (2016); Abarca-Gómez et al. (2017); GBD 2017 Risk Factor Collaborators (2018).
4. ^Data sources: WWF (2020); Global Footprint Network (2021).
5. ^https://www.footprintnetwork.org/
6. ^Ecological Footprint is measured in units of global hectares, which weights land according to biological productivity, thus enabling comparisons across different land types.
References
Abarca-Gómez, L., Abdeen, Z. A., Hamid, Z. A., Abu-Rmeileh, N. M., Acosta-Cazares, B., Acuin, C., et al. (2017). Worldwide trends in body-mass index, underweight, overweight, and obesity from 1975 to 2016: a pooled analysis of 2416 population-based measurement studies in 128·9 million children, adolescents, and adults. Lancet 390, 2627–2642. doi: 10.1016/S0140-6736(17)32129-3
Alvarez, S., Timler, C. J., Michalscheck, M., Paas, W., Descheemaeker, K., Tittonell, P., et al. (2018). Capturing farm diversity with hypothesis-based typologies: an innovative methodological framework for farming system typology development. PLoS ONE 13:e0194757. doi: 10.1371/journal.pone.0194757
Andersen, E., Elbersen, B., Godeschalk, F., and Verhoog, D. (2007). Farm management indicators and farm typologies as a basis for assessments in a changing policy environment. J. Environ. Manage. 82, 353–362. doi: 10.1016/j.jenvman.2006.04.021
Baer-Nawrocka, A., and Sadowski, A. (2019). Food security and food self-sufficiency around the world: a typology of countries. PLoS ONE 14:e0213448. doi: 10.1371/journal.pone.0213448
Bailey, K. (1994). “Typologies and taxonomies in social science, in Typologies and Taxonomies: An Introduction to Classification Techniques (New York, NY: SAGE Publications, Inc), 1–16. doi: 10.4135/9781412986397.n1
Barrett, C., Benton, T., Fanzo, J., Herrero, M., and Nelson, R. (forthcoming). Socio-Technical Innovation Bundles for Agri-Food Systems Transformation. London: Palgrave Macmillan.
Barrett C. B. Reardon T. Swinnen J. Zilberman D. (forthcoming). Agri-food value chain revolutions in low-and middle-income countries. J. Econ. Lit.
Bean, M., and Sharp, J. S. (2011). Profiling alternative food system supporters: the personal and social basis of local and organic food support. Renew. Agric. Food Syst. 26, 243–254. doi: 10.1017/S1742170511000032
Carbone, A. (2018). Foods and places: comparing different supply chains. Agriculture 8:6. doi: 10.3390/agriculture8010006
Coelli, T. J. (1995). Recent developments in frontier modelling and efficiency measurement. Aust. J. Agric. Econ. 39, 219–245. doi: 10.1111/j.1467-8489.1995.tb00552.x
Diaz, R. J., and Rosenberg, R. (2008). Spreading dead zones and consequences for marine ecosystems. Science 321, 926–929. doi: 10.1126/science.1156401
Dicecca, R., Pascucci, S., and Conto, F. (2016). Understanding reconfiguration pathways of agri-food value chains for smallholder farmers. Br. Food J. 118, 1837–1862. doi: 10.1108/BFJ-05-2016-0194
Díez, J., Bilal, U., and Franco, M. (2019). Unique features of the Mediterranean food environment: Implications for the prevention of chronic diseases Rh: Mediterranean food environments. Eur. J. Clin. Nutr. 72, 71–75.
Downs, S. M., Ahmed, S., Fanzo, J., and Herforth, A. (2020). Food environment typology: advancing an expanded definition, framework, and methodological approach for improved characterization of wild, cultivated, and built food environments toward sustainable diets. Foods (Basel, Switzerland) 9:532. doi: 10.3390/foods9040532
Ericksen, P. J. (2008). Conceptualizing food systems for global environmental change research. Glob. Environ. Change. 18, 234–245. doi: 10.1016/j.gloenvcha.2007.09.002
Euromonitor International (2018a). Growth in Ultra-Processed Food Sales [Data set]. In Passport Database. Euromonitor International.
FAO (2016). Share of Dietary Energy Supply Derived from Cereals, Roots, and Tubers (kcal/cap/day) (3-year average). Available online at: http://www.fao.org/faostat/en/#data/FS (accessed October 20, 2020).
FAO IFAD, UNICEF, WFP, and WHO. (2020). The State of Food Security and Nutrition in the World 2020: Transforming Food Systems for Affordable Healthy Diets. FAO.
Fay, M., and Opal, C. (2000). Urbanization Without Growth: A Not-So-Uncommon Phenomenon. Policy Research Working Paper No. 2412 (Policy Research Working Papers). The World Bank. doi: 10.1596/1813-9450-2412
Feuillet, T., Charreire, H., Roda, C., Ben Rebah, M., Mackenbach, J. D., Compernolle, S., et al. (2016). Neighbourhood typology based on virtual audit of environmental obesogenic characteristics. Obes. Rev. 17(Suppl. 1), 19–30. doi: 10.1111/obr.12378
Food Agriculture Organization (2016). Fertilizer Consumption (Kilograms Per Hectare of Arable Land) [Data set]. In World Development Indicators. The World Bank. Available online at: https://data.worldbank.org/indicator/AG.CON.FERT.ZS
Food and Agriculture Organization (2018a). Land Area, Sq. Km [Data set]. In World Development Indicators. The World Bank. Available online at: https://data.worldbank.org/indicator/AG.LND.TOTL.K2 (accessed October 20, 2020).
Food and Agriculture Organization (2018b). Vegetable Losses [Data set]. In Food Balance Sheets. FAO. Available online at: http://www.fao.org/faostat/en/#data/FBS (accessed June 01, 2021).
GAIN Johns Hopkins University (2020). Food Systems Dashboard. Available online at: https://foodsystemsdashboard.org/ (accessed July 18, 2021).
GBD 2017 Risk Factor Collaborators (2018). Global, regional, and national comparative risk assessment of 84 behavioural, environmental and occupational, and metabolic risks or clusters of risks for 195 countries and territories, 1990–2017: a systematic analysis for the global burden of disease study 2017. Lancet 392, 1923–1994. doi: 10.1016/S0140-6736(18)32225-6
Global Footprint Network (2021). Ecological Footprint from Food Production [Data set]. In National Footprint and Biocapacity Accounts 2021. Available online at: http://data.footprintnetwork.org/ (accessed March 1, 2021).
Global Panel on Agriculture and Food Systems for Nutrition (2016). Food systems and diets: Facing the challenges of the 21st century. IFPRI.
Gollin, D. (2010). “Agricultural productivity and economic growth,” in Handbook of Agricultural Economics, Vol. 4, eds B. L. Gardner, and G. C. Rousser (Amsterdam: Elsevier), 3825–3866. doi: 10.1016/S1574-0072(09)04073-0
Gómez, M. I., Barrett, C. B., Raney, T., Pinstrup-Andersen, P., Meerman, J., Croppenstedt, A., et al. (2013). Post-green revolution food systems and the triple burden of malnutrition. Food Policy 42, 129–138. doi: 10.1016/j.foodpol.2013.06.009
Gomez, M. I., and Ricketts, K. D. (2013). Food value chain transformations in developing countries: selected hypotheses on nutritional implications. Food Policy 42, 139–150. doi: 10.1016/j.foodpol.2013.06.010
Gunia, M., Mandonnet, N., Arquet, R., de la Chevrotière, C., Naves, M., Mahieu, M., et al. (2010). Production systems of creole goat and their implications for a breeding programme. Anim.: Int. J. Anim. Biosci. 4, 2099–2105. doi: 10.1017/S1751731110001412
Hajer, M. A., Westhoek, H., Ingram, J., Van Berkum, S., and Özay, L. (2016). Food Systems and Natural Resources. UNEP.
HLPE (2017). Nutrition and Food Systems. A Report by the High Level Panel of Experts on Food Security and Nutrition. HLPE.
Hobbs, M., Green, M. A., Wilkins, E., Lamb, K. E., McKenna, J., and Griffiths, C. (2019). Associations between food environment typologies and body mass index: evidence from Yorkshire, England. Soc. Sci. Med. 239:112528. doi: 10.1016/j.socscimed.2019.112528
Hoekstra, A. Y., and Chapagain, A. K. (2007). Water footprints of nations: water use by people as a function of their consumption pattern. Water Resour. Manage. 21, 35–48. doi: 10.1007/s11269-006-9039-x
IFC (2019). Creating Markets in Burkina Faso: Growing Burkina Faso's Private Sector and Harnessing it to Bolster Economic Resilience. Washington, DC: International Finance Corporation.
IFPRI (2015). Global Nutrition Report 2015: Actions and Accountability to Advance Nutrition and Sustainable Development. International Food Policy Research Institute.
Ingram, J. (2011). A food systems approach to researching food security and its interactions with global environmental change. Food Sec. 3, 417–431. doi: 10.1007/s12571-011-0149-9
Jägermeyr, J., Gerten, D., Heinke, J., Schaphoff, S., Kummu, M., and Lucht, W. (2015). Water savings potentials of irrigation systems: global simulation of processes and linkages. Hydrol. Earth Syst. Sci. 19, 3073–3091. doi: 10.5194/hess-19-3073-2015
Kostrowicki, J. (1977). Agricultural typology concept and method. Agric. Syst. 2, 33–45. doi: 10.1016/0308-521X(77)90015-4
Le Noë, J., Billen, G., and Garnier, J. (2017). How the structure of agro-food systems shapes nitrogen, phosphorus, and carbon fluxes: the generalized representation of agro-food system applied at the regional scale in France. Sci. Total Environ. 586, 42–55. doi: 10.1016/j.scitotenv.2017.02.040
Lin, D., Hanscom, L., Martindill, J., Borucke, M., Cohen, L., Galli, A., et al. (2019). Working Guidebook to the National Footprint and Biocapacity Accounts. Global Footprint Network.
Lopez-Ridaura, S., Frelat, R., van Wijk, M. T., Valbuena, D., Krupnik, T. J., and Jat, M. L. (2018). Climate smart agriculture, farm household typologies and food security: an ex-ante assessment from Eastern India. Agric. Syst. 159, 57–68. doi: 10.1016/j.agsy.2017.09.007
Madry, W., Mena, Y., Roszkowska-Madra, B., Gozdowski, D., Hryniewski, R., and Castel, J. M. (2013). An overview of farming system typology methodologies and its use in the study of pasture-based farming system: a review. Span. J. Agric. Res. 11, 316–326. doi: 10.5424/sjar/2013112-3295
McCullough, E. B., Pingali, P. L., and Stamoulis, K. G. (2010). “Small farms and the transformation of food systems: an overview,” in Looking East, Looking West: Organic and Quality Food Marketing in Asia and Europe, eds R. Haas, and V. Canavari, B. Slee, C. Tong, and B. Anurugsa (Taylor and Francis: Oxfordshire), 47–83.
Mekonnen, M., and Hoekstra, A. Y. (2010). The Green, Blue and Grey Water Footprint of Farm Animals and Animal Products. Volume 2: Appendices. Available online at: https://digitalcommons.unl.edu/wffdocs/82/ (accessed March 1, 2021).
Mekonnen, M. M., and Hoekstra, A. Y. (2011). The green, blue and grey water footprint of crops and derived crop products. Hydrol. Earth Syst. Sci. 15, 1577–1600. doi: 10.5194/hess-15-1577-2011
Monteiro, C. A., Cannon, G., Lawrence, M., Da Costa Louzada, M. L., and Machado, P. P. (2019). Ultra-Processed Foods, Diet Quality, and Health Using the Nova Classification System. Rome: FAO.
Myers, C. A., Denstel, K. D., and Broyles, S. T. (2016). The context of context: examining the associations between healthy and unhealthy measures of neighborhood food, physical activity, and social environments. Prevent. Med. 93, 21–26. doi: 10.1016/j.ypmed.2016.09.009
Nsamzinshuti, A., Janjevic, M., Rigo, N., and Ndiaye, A. B. (2018). “Short supply chains as a viable alternative for the distribution of food in urban areas? Investigation of the performance of several distribution schemes,” in Sustainable Freight Transport: Theory, Models, and Case Studies, eds V. Zeimpekis, E. Aktas, M. Bourlakis, and I. Minis (New York, NY: Springer International Publishing), 99–119. doi: 10.1007/978-3-319-62917-9_7
Paviot, M. C., Bresnyan, E. W., Diaz, A., Mishu, S., Ba, A., and Grosclaude, M. (2019). Guinea-Bissau: Unlocking Diversification to Unleash Agricultural Growth. Washington, DC: The World Bank.
Pingali, P., Ricketts, K., and Sahn, D. E. (2015). “Agriculture for nutrition,” in The Fight Against Hunger and Malnutrition: The Role of Food, Agriculture, and Targeted Policies, ed D. E. Sahn (Oxford: Oxford University Press), 165–191. doi: 10.1093/acprof:oso/9780198733201.003.0008
Poore, J., and Nemecek, T. (2018). Reducing food's environmental impacts through producers and consumers. Science 360, 987–992. doi: 10.1126/science.aaq0216
Popkin, B. M. (1999). Urbanization, lifestyle changes and the nutrition transition. World Dev. 27, 1905–1916. doi: 10.1016/S0305-750X(99)00094-7
Popkin, B. M. (2012). “The Nutrition Transition is Speeding Up: A Global Perspective,” in Nutritional Health: Strategies for Disease Prevention, 3rd Edn, eds N. Temple, T. Wilson, and J. D. Jacobs (Totowa, NJ: Humana Press Inc), 85–99. doi: 10.1007/978-1-61779-894-8_5
Popkin, B. M., Adair, L. S., and Ng, S. W. (2012). Global nutrition transition and the pandemic of obesity in developing countries. Nutr. Rev. 70, 3–21. doi: 10.1111/j.1753-4887.2011.00456.x
Ranganathan, J., Vennard, D., Waite, R., Dumas, P., Lipinski, B., Searchinger, T. I. M., et al. (2016). Shifting Diets for a Sustainable Food Future. World Resources Institute. Avaiable online at: https://www.wri.org/research/shifting-diets-sustainable-food-future (accessed March 1, 2021).
Reardon, T., Timmer, C. P., Barrett, C. B., and Berdegué, J. (2003). The rise of supermarkets in Africa, Asia, and Latin America. Am. J. Agric. Econ. 85, 1140–1146. doi: 10.1111/j.0092-5853.2003.00520.x
Reardon, T., and Zilberman, D. (2018). “Climate smart food supply chains in developing countries in an era of rapid dual change in agrifood systems and the climate,” in Climate Smart Agriculture, Vol. 52, eds L. Lipper, N. McCarthy, D. Zilberman, S. Asfaw, and G. Branca (Berlin: Springer), 335–351. doi: 10.1007/978-3-319-61194-5_15
Reardon, T., and Timmer, C. P. (2007). “Transformation of markets for agricultural output in developing countries since 1950: how has thinking changed?” in Handbook of Agricultural Economics, Vol. 3, ed R. Evenson and P. Pingali, (Amsterdam: Elsevier), 2807–2855. doi: 10.1016/S1574-0072(06)03055-6
Remans, R., Wood, S. A., Saha, N., Anderman, T. L., and DeFries, R. S. (2014). Measuring nutritional diversity of national food supplies. Glob. Food Sec. 3, 174–182. doi: 10.1016/j.gfs.2014.07.001
Satterthwaite, D., McGranahan, G., and Tacoli, C. (2010). Urbanization and its implications for food and farming. Philos. Trans. R. Soc. B: Biol. Sci. 365, 2809–2820. doi: 10.1098/rstb.2010.0136
Sobal, J., Khan, L. K., and Bisogni, C. (1998). A conceptual model of the food and nutrition system. Soci. Sci. Med. 47, 853–863. doi: 10.1016/S0277-9536(98)00104-X
Springmann, M., Clark, M., Mason-D'Croz, D., Wiebe, K., Bodirsky, B. L., Lassaletta, L., et al. (2018). Options for keeping the food system within environmental limits. Nature 562, 519–525. doi: 10.1038/s41586-018-0594-0
Tefft, J., Jonasova, M., Adjao, R., and Morgan, A. (2017). Food Systems for an Urbanizing World. World Bank and FAO. Avaiable online at: https://openknowledge.worldbank.org/handle/10986/32502 (accessed October 20, 2020).
Timmer, P. C. (1988). “The agricultural transformation,” in Handbook of Development Economics, Vol. 1, eds H. Chenery and T. N. Srinivasan (Amsterdam: Elsevier), 275–331. doi: 10.1016/S1573-4471(88)01011-3
Timperio, A., Crawford, D., Leech, R. M., Lamb, K. E., and Ball, K. (2018). Patterning of neighbourhood food outlets and longitudinal associations with children's eating behaviours. Prevent. Med. 111, 248–253. doi: 10.1016/j.ypmed.2018.03.003
Tonumaipe'a, D., Cammock, R., and Conn, C. (2021). Food havens not swamps: a strength-based approach to sustainable food environments. Health Promot. Int. doi: 10.1093/heapro/daab021
Tudisca, S., Di Trapani, A. M., Sgroi, F., Testa, R., and Giamporcaro, G. (2014). Role of alternative food networks in Sicilian farms. Int. J. Entrepreneursh. Small Bus. 22, 50–63. doi: 10.1504/IJESB.2014.062130
Turner, C., Aggarwal, A., Walls, H., Herforth, A., Drewnowski, A., Coates, J., et al. (2018). Concepts and critical perspectives for food environment research: a global framework with implications for action in low-and middle-income countries. Glob. Food Sec. 18, 93–101. doi: 10.1016/j.gfs.2018.08.003
Tyrrell, R. L., Greenhalgh, F., Hodgson, S., Wills, W. J., Mathers, J. C., Adamson, A. J., et al. (2017). Food environments of young people: linking individual behaviour to environmental context. J. Public Health 39, 95–104. doi: 10.1093/pubmed/fdw019
United Nations Population Division (2019). Urban Population (% of Total Population). Available online at: https://data.worldbank.org/indicator/SP.URB.TOTL.IN.ZS (accessed October 20, 2020).
United Nations Population Division World Bank (2019). Population, Total. Available online at: https://data.worldbank.org/indicator/SP.POP.TOTL (accessed October 20, 2020).
Vitousek, P. M., Naylor, R., Crews, T., David, M. B., Drinkwater, L. E., Holland, E., et al. (2009). Nutrient imbalances in agricultural development. Science 324, 1519–1520. doi: 10.1126/science.1170261
Willett, W., Rockström, J., Loken, B., and Springmann, M. (2019). Food in the Anthropocene: the EAT–Lancet Commission on healthy diets from sustainable food systems. Lancet. 393, 447–492. doi: 10.1016/S0140-6736(18)31788-4
World Bank (2017). Burkina Faso: Agriculture as a Powerful Instrument for Poverty Reduction, Research Brief . The World Bank.
World Bank OECD ILO (2019). Agriculture, Forestry, and Fishing, Value Added Per Worker (Constant 2010 US$). Available online at: https://data.worldbank.org/indicator/NV.AGR.EMPL.KD (accessed October 20, 2020).
World Bank Eurostat-OECD (2019). GDP Per Capita, PPP (Current International $) [Data set]. In World Development Indicators. The World Bank. Available online at: https://data.worldbank.org/indicator/NY.GDP.PCAP.PP.CD (accessed October 20, 2020).
World Health Organization (2016). Prevalence of Anaemia in Women of Reproductive Age [Data set]. In Global Health Observatory. WHO. Available online at: https://apps.who.int/gho/data/view.main.ANAEMIAWOMENPREVANEMIAv (accessed October 20, 2020).
Keywords: food systems, typology, nutrition, sustainability, agriculture
Citation: Marshall Q, Fanzo J, Barrett CB, Jones AD, Herforth A and McLaren R (2021) Building a Global Food Systems Typology: A New Tool for Reducing Complexity in Food Systems Analysis. Front. Sustain. Food Syst. 5:746512. doi: 10.3389/fsufs.2021.746512
Received: 24 July 2021; Accepted: 13 October 2021;
Published: 18 November 2021.
Edited by:
Marrit Van Den Berg, Wageningen University and Research, NetherlandsReviewed by:
Ruerd Ruben, Wageningen University and Research, NetherlandsPolly Joanna Ericksen, International Livestock Research Institute (ILRI), Kenya
Copyright © 2021 Marshall, Fanzo, Barrett, Jones, Herforth and McLaren. This is an open-access article distributed under the terms of the Creative Commons Attribution License (CC BY). The use, distribution or reproduction in other forums is permitted, provided the original author(s) and the copyright owner(s) are credited and that the original publication in this journal is cited, in accordance with accepted academic practice. No use, distribution or reproduction is permitted which does not comply with these terms.
*Correspondence: Quinn Marshall, qmarsha1@jhu.edu