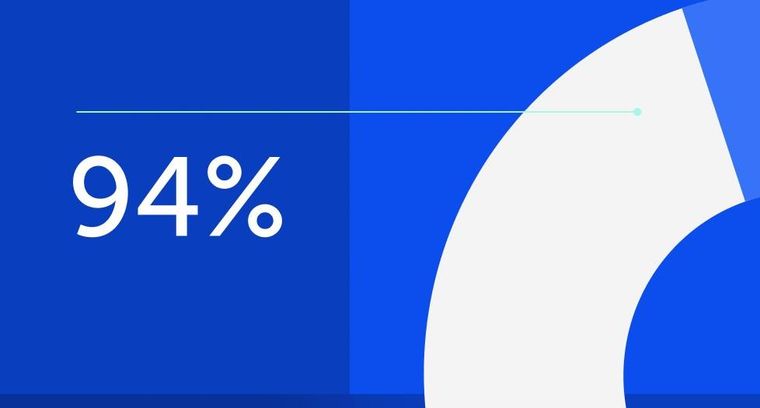
94% of researchers rate our articles as excellent or good
Learn more about the work of our research integrity team to safeguard the quality of each article we publish.
Find out more
MINI REVIEW article
Front. Sustain. Food Syst., 27 January 2022
Sec. Waste Management in Agroecosystems
Volume 5 - 2021 | https://doi.org/10.3389/fsufs.2021.745894
As the world population increases, food demand and agricultural activity will also increase. However, ~30–40% of the food produced today is lost or wasted along the production chain. Increasing food demands would only intensify the existing challenges associated with agri-food waste management. An innovative approach to recover the resources lost along the production chain and convert them into value-added product(s) would be beneficial. An alternative solution is the use of the larvae of the black soldier fly (BSFL), Hermetia illucens L., which can grow and convert a wide range of organic waste materials into insect biomass with use as animal feed, fertilizer and/or bioenergy. However, the main concern when creating an economically viable business is the variability in BSFL bioconversion and processing due to the variability of the substrate. Many factors, such as the nutritional composition of the substrate heavily impact BSFL development. Another concern is that substrates with high lignin and cellulose contents have demonstrated poor digestibility by BSFL. Studies suggest that pretreatment methods may improve the digestibility and biodegradability of the substrate by BSFL. However, a systematic review of existing pretreatment methods that could be used for enhancing the bioconversion of these wastes by BSFL is lacking. This paper provides a state-of-the-art review on the potential pretreatment methods that may improve the digestibility of substrates by BSFL and consequently the production of BSFL. These processes include but are not limited to, physical (e.g., mechanical and thermal), chemical (alkaline treatments), and biological (bacterial and fungal) treatments.
Meeting global food demands within our planetary boundaries requires recycling nutrients currently wasted within the agri-food system (Willett et al., 2019). Today, more than 1.3 billion tons of food are lost or wasted each year (Gustavsson et al., 2011). Especially in low- and middle-income countries, a lack of managing these wastes leads to adverse public health and environmental effects (Hoornweg and Bhada-Tata, 2012). Instead, utilizing nutrients in agri-food wastes contributes to more sustainable food production for the growing global population, projected to surpass nine billion by 2050 (United Nations, 2017).
An emerging solution for recycling of agri-food wastes into value-added products for food production uses black soldier fly larvae (BSFL, Hermetia illucens L.) (Figure 1A) (Gold et al., 2018). BSFL convert many agri-food wastes (e.g. manures, agricultural crops and residues and milling side streams) (Gold et al., 2018, 2020a,b) into protein-rich insect biomass that can efficiently substitute current livestock feed ingredients, such as soybean and fishmeal, leaving behind a compost-like residue (i.e., insect frass) for fertilizer applications (Klammsteiner et al., 2020). In addition, agri-food waste bioconversion with BSFL can reduce greenhouse gas emissions compared to biowaste composting, and produce BSFL-based feeds with a lower environmental impact than current livestock feed ingredients (Smetana et al., 2016, 2019; Gold et al., 2018; Mertenat et al., 2019). However, there are some trade-offs when pursuing the pathway of BSFL recycling of agri-food waste as these substrates do not typically result in high BSFL performance.
Figure 1. (A) Schematic of the BSFL process for the production of insect-based feed, bioenergy, and fertilizer from agri-food wastes and byproducts. (B) Schematic of the structural change of lignocellulosic components after physical, chemical, and biological pretreatments as part of substrate preparation. Underlined pretreatments are reviewed in this study. Adapted from Mosier et al. (2005) and Dortmans et al. (2021).
One key challenge of BSFL bioconversion is the frequently low process performance with some of the most abundant and affordably sourced agri-food wastes (Gold et al., 2018; Lalander et al., 2019). For example, the bioconversion rate, that is the amount of dry larval biomass produced per unit of waste, is 1.9–6% for animal manures (Gold et al., 2018; Liu et al., 2018), 5% for brewery side streams (Liu et al., 2018) and 4–5% for fruit and vegetable wastes (Lalander et al., 2019; Somroo et al., 2019). These bioconversion rates are low relative to those for food wastes (13–21%) (Nyakeri et al., 2017; Lalander et al., 2019). BSFL development is affected by highly biodegradable macronutrients such as proteins, carbohydrates, and lipids (Nguyen et al., 2013). The low performance of some agri-food wastes has frequently been associated with their high crude fiber content containing lignocellulose, which has poor biodegradability. The lignocellulosic composition primarily consists of cellulose (~30–50%), hemicellulose (~20–35%) and lignin (~10–25%) (Table 1) (Liu et al., 2008). Despite contradictory results in the literature, where Rehman et al. (2017) reported BSFL reduced lignin and cellulose in dairy manure by 31 and 50%, respectively, these lignocellulosic fractions are thought to be mostly indigestible by BSFL and negatively correlated with process performance (Liu et al., 2018; Gold et al., 2020a,b). Since the process performance and bioconversion of these agri-food wastes are decisive for the affordability and sustainability of BSFL-based products (Smetana et al., 2019), solutions to enhance process performance and conversion are urgently needed if these wastes will be used for BSFL production.
The structural characteristics of lignocellulosic substrates (i.e., lignin presence, high cellulose crystallinity, water insolubility, and resistance to depolymerization) are common problems affecting the efficient valorization of many agri-food wastes (Agbor et al., 2011). Various physical, chemical, and biological substrate pretreatments have been extensively studied to enhance the conversion of lignocellulosic substrates for bioenergy yields (e.g., biogas, methane and ethanol) from anaerobic digestion and fermentation, ethanol production, (vermi)-composting and, to a lesser extent BSFL rearing performance (Hamelinck et al., 2005; Mosier et al., 2005; Carlsson et al., 2012; Kim, 2013). The overall bioconversion process performance, and economic viability are greatly affected by the availability and degradability of the substrate. The goal of substrate pretreatment is to degrade lignin, reduce the crystalline structure of cellulose (Figure 1B), and increase pore size and surface/volume ratio, making it more suitable for digestion by larval (e.g., insect-based bioconversion) enzymes (Mosier et al., 2005; Kim, 2013).
Implementation of pretreatments in BSFL production, could potentially enhance bioconversion efficiency and reliability across various agri-food wastes. However, few studies have investigated the effects of physical, chemical (Liu et al., 2021), and biological pretreatments (Isibika et al., 2019) on BSFL bioconversion. The goal of this review is to evaluate state-of-the-art pretreatment methods used for biogas production and (vermi-) composting, providing direction for future research activities. Due to the use of semi-solid substrates and the importance of microbiological processes, these waste conversion processes are probably most similar to BSFL bioconversion and thus the focus of this review. To synthesize the most relevant research for bioenergy/biogas production and (vermi-) composting, only those published in the last 20 years were considered (see Supplementary Figure 1, for the four-step approach conducted for this review). To enable economically viable waste management and feed production, technologies considered by previous studies to have high energy demands (e.g., sonication, microwave, pulsed electric field, steam explosion), or include costly additives (e.g., commercial cellulolytic enzymes), are not discussed in detail in this review.
Physical pretreatment is a widely applied substrate pretreatment for improving biogas production and composting. Physical pretreatment alters the lignocellulosic characteristics (e.g., reduce crystallinity, increase surface area, and increase porosity) using mechanical, thermal, and electromagnetic processes (Atelge et al., 2020). In contrast to chemical and biological pretreatments, physical pretreatments do not require additives (e.g., chemicals, microbes), but depending on the technology, substrate type and the process target, they can demand energy (i.e., electricity and heat) requirements of 3–55 kWh/t (Kratky and Jirout, 2011; Menardo et al., 2012; Bhagwat et al., 2015).
Mechanical pretreatment can reduce substrate particle size with various choppers and mills (e.g., hammer mill, knife mill, bead mill, wet disk mill). Grinding and chopping typically involve fracturing the substrate down to diameters of <2 mm and 10–30 mm, respectively (Oyedeji et al., 2020). These technologies are considered more suitable for substrates with moisture contents lower than 15%, while colloid mills, extruders, and expanders are suitable for substrates with moisture contents over 15–20% (wet basis) (Kratky and Jirout, 2011).
Reduced substrate particle size has been shown to positively correlate with biogas or methane yield (Victorin et al., 2020), in addition to increasing cellulose degradation (Sharma et al., 1988). Mechanical pretreatments, such as grinding, extrusion, and mechanical disintegration, have already been applied in full-scale for animal manure, municipal solid waste, and agricultural crops and residues (Carrère et al., 2016). Particle size reduction, ranging from ≤ 2 mm to 5 cm for manures, fruit/vegetable waste, and crop and harvesting residues has shown to increase methane production/yield by 16–89% in comparison to untreated substrates (see Supplementary Table 2) (Sharma et al., 1988; Angelidaki and Ahring, 2000; Mshandete et al., 2006; Menardo et al., 2012). For example, methane production increased by 16% and 23% for cattle manure and sisal fiber, respectively, when reduced to 2 mm average particle size (Angelidaki and Ahring, 2000; Mshandete et al., 2006). The increased surface area provides greater access for microorganisms, which positively impacts methane production/yield. However, the benefit of particle size reduction is very dependent on the substrate. For example, Menardo et al. (2012) reported a methane yield increase of 57% and 19% for wheat straw and barley straw, respectively, when reduced to 5 cm, but did not observe the same effect for rice straw. Palma et al. (2019) suggested that the mechanical pretreatment efficiency may also be substrate-dependent for BSFL bioconversion, as decreasing almond hull particle size with a hammer mill from 6 to 4 mm decreased larval mass by 10%. The authors concluded that BSFL may benefit from aeration due to its larger particle size. In contrast to anaerobic digestion, BSFL based conversion is an aerobic process, requiring oxygen to function. Thus, porosity and airflow play a role, and a more compacted substrate with lower particle size may lead to a reduced oxygen supply for BSFL. However, more research is needed to identify the optimal particle size for efficient BSFL bioconversion.
Another common physical pretreatment worth considering is thermal pretreatment, as the main goal is to increase the solubility of the organic matter, improving biodegradability and increasing biogas production and the efficiency of composting (Appels et al., 2010; Cesaro and Belgiorno, 2014; Cao et al., 2019). Thermal pretreatment is highly dependent on temperature, time, and, in some cases pressure, with temperatures above 100°C and processing times ranging from 15 to 120 min with 2–9 bar pressure (Carrère et al., 2010; Atelge et al., 2020). However, these operating conditions may not be suitable for BSFL processing because of the inactivation of potentially beneficial microorganisms present in the substrate (Gold et al., 2020a,b). For example, Isibika et al. (2019) attributed the 15 and 19% decrease in BSFL larval mass and bioconversion rate, respectively, on banana peels following pretreatment for 60 min at 120°C at 2 bar, to release of toxic tannins. The production/release of phenolic compounds at high temperatures (e.g. >160°C) could potentially inhibit larval and microbial metabolism (Müller, 2000; Hendriks and Zeeman, 2009). In general, low temperature thermal pretreatment (e.g., <100°C) has shown to increase methane and biogas production/yield for animal manures and agricultural crops and residues by 14–62% compared to untreated substrates (see Supplementary Table 2) (Menardo et al., 2012; Nava-Valente et al., 2021; Senol, 2021). However, thermal pretreatment at 70°C for 24 h for buckwheat hull had no significant effect on methane yield as lignocellulose degradation was not observed (Mirko et al., 2021).
Therefore, pretreatment at lower temperatures (80–90°C) and longer holding times (≥60 min) could be a more suitable treatment condition of the substrate before BSFL bioconversion, in addition to achieving pasteurization of the substrate (i.e., inactivating relevant vegetative pathogens that may still be present). Similar treatment settings increased biogas production from agricultural crops and residues by 60%, when pretreated at 80–90°C for 30–60 min (Menardo et al., 2012, see Supplementary Table 2). Thermal pretreatment at 90°C for 4 h increased the composting efficiency of animal manure mixed with agricultural crops and residues, increasing humic substances and stable forms of organic matter by 14–18% (Huang et al., 2019; Zhu et al., 2021, see Supplementary Table 2). Overall, thermal pretreatment at these lower temperatures has demonstrated positive effects on biogas production and composting.
Chemical pretreatments use oxidizing agents (e.g., H2O2), acids (e.g., H2SO4 and HCl), alkalis, and ozone to break down lignin and/or hemicelluloses while increasing the accessibility of cellulose (Carrère et al., 2010; Behera et al., 2014). The efficiency of chemical pretreatment is highly substrate-dependent and requires optimization of chemicals, dose, and treatment time for optimal results.
Acid pretreatments typically use either concentrated or diluted acids. For concentrated acids, a concentration above 30% (w/v) is usually needed and carried out at temperatures lower than 100°C for several hours. However, a major disadvantage is that a high concentration leads to high corrosiveness, thus requiring a setup that is resistant to corrosion (Sun and Cheng, 2002). Additionally, a neutralizing agent is needed to increase the pH. For the diluted form, an acid concentration of 0.5 to 5% (w/v) can be used, but at high temperatures (120–215°C) for a few minutes (Sun and Cheng, 2002). As mentioned in the section on physical pretreatments, high temperatures may not be suitable for BSFL bioconversion. Therefore, our review focuses on alkaline treatments due to their milder conditions compared to acid treatment (Kim et al., 2016) and because of their proven effect on high-lignin substrates, such as agricultural crops and residues, municipal solid waste, and animal manure (Carrère et al., 2016).
Treatment of substrates with alkaline chemicals (e.g., sodium hydroxide, lime, and ammonia) has been most widely studied because of its effectiveness in lignin degradation, increasing access to cellulose and hemicellulose for microbial decomposition (Carrère et al., 2011; Bochmann and Montgomery, 2013). A neutralizing agent, such as sulfuric acid, may be needed to reduce pH after alkaline pretreatment.
Pretreatment of lignocellulosic substrates with 1–10% sodium hydroxide (NaOH) is the most commonly used alkaline pretreatment, with typical residence times between 1 and 24 h (Sambusiti et al., 2013; Taherdanak and Zilouei, 2014, see Supplementary Table 3). Agricultural crops and residues pretreated with NaOH at ambient temperature (e.g., 20–25°C), have shown to increase biogas or methane production by 13–89% (Supplementary Table 3) (Neves et al., 2006; He et al., 2008; Taherdanak and Zilouei, 2014). However, there could be a threshold where NaOH pretreatment is no longer effective, e.g., if lignin content exceeds 26% (Sun and Cheng, 2002). Liu et al. (2021) tested alkaline pretreatment (NaOH + H2O2 for 6 h at 30 °C) on the lignocellulosic content of rice straw before BSFL rearing. Prior to BSFL bioconversion, the authors observed a decrease in hemicellulose and lignin contents by 22, and 68%, respectively, and an increase in cellulose by 25% in the substrate. The authors found that following pretreatment, cellulose decomposition by BSFL was 10% higher (Supplementary Table 3). Surprisingly, this did not significantly change rice straw reduction by BSFL compared to the untreated control. However, the results are still promising, given that pretreatment increased the harvested larval biomass by 32%.
Additional alkaline pretreatments use lime [Ca(OH)2], aqueous ammonia, or urea to maximize biogas/methane production (17–173%) for animal manure, municipal organic solid waste, and agricultural crops and residues (Supplementary Table 3) (López Torres and Espinosa Lloréns, 2008; Jurado et al., 2013b; Liang et al., 2014; Mirtsou-Xanthopoulou et al., 2014; Antonopoulou and Gavala, 2015). Lime pretreatment was shown to enhance biogas production with municipal organic solid waste by 173% (López Torres and Espinosa Lloréns, 2008), but showed contradicting results with animal manure (Niasar et al., 2011). Aqueous ammonia has gained increased attention because of its effectiveness in lignin removal or modification of substrates while preserving the total carbohydrate content (Mirtsou-Xanthopoulou et al., 2014; Carrère et al., 2016). Pretreatment with the soaking aqueous ammonia method using 3 g ammonia/1g DM at 22°C and an optimal pretreatment time of 3 days was found to increase methane yield by 37–104% for animal manure and agricultural crops and residues (Jurado et al., 2013a,b; Mirtsou-Xanthopoulou et al., 2014). However, using large quantities of aqueous ammonia would not be suitable for BSFL production. But, the addition of aqueous ammonia at a lower dose of 1% to banana peels, pretreated for 7 days, increased crude fiber by 5% and demonstrated a positive increase in larval biomass and bioconversion by 31 and 33%, respectively (Isibika et al., 2019). However, at a dose of 0.8% larval bioconversion decreased by 1% compared to the control. Interestingly, urea pretreatment has been used to increase the nutritive value of ruminant feeds (e.g., rice and wheat straw) (van Kuijk et al., 2015) by breakdown of lignin (Yao et al., 2018). Palma et al. (2019), observed an increase in larval biomass by 10% when supplementing almond hulls with urea, changing the C/N ratio (i.e., carbon to nitrogen ratio) from 16 to 32. Ammonia and urea can increase the digestibility of lignocellulosic substrates for BSFL; however, it remains unclear whether these effects stem from improved digestibility of the substrates due to chemical fiber decomposition or microbial conversion of the added non–protein nitrogen and/or degraded fibers into protein digestible by BSFL (Isibika et al., 2019).
Biological pretreatments add bacteria, fungi, yeasts or mixtures of microorganisms to substrates for the decomposition of lignin and/or cellulose by microbial enzymes (e.g. lignin modifying enzymes (LME) and cellulase, respectively) (Galbe and Zacchi, 2012; Atelge et al., 2020). The advantages of biological pretreatments over physical and chemical pretreatments are that they require less energy, no chemicals, and necessary microorganisms can be isolated from substrates (Mishra et al., 2018). Disadvantages include the slow decomposition rate and limited control over the process, possibly making it less suitable for industrial scale (Agbor et al., 2011). Various factors can influence the effectiveness of biological pretreatments, such as temperature, moisture content, incubation time, carbon and nitrogen sources, and microbial species (Sharma et al., 2019). These factors should be optimized to improve the decomposition rate.
Fungal pretreatments have been studied extensively for biogas production. Among the candidate fungi, white-rot fungi (e.g., Phanerochaete chrysosporium, Pleurotus ostreatus, and Ceriporiopsis subvermispora) possess enzymes for lignin degradation (Wan and Li, 2012). Pretreatment of agricultural crops and residues with white-rot fungi for 14–40 days was shown to decrease substrate lignin by 16–33% (Mustafa et al., 2016; Alexandropoulou et al., 2017) (Supplementary Table 4). This has led to an increase in methane yield or biogas production from 6 to 154%, but has also shown to decrease methane yield by 9% (Supplementary Table 4) (Liu et al., 2014; Zhao et al., 2014; Mustafa et al., 2016; Alexandropoulou et al., 2017). As indicated by the range of results among studies, the effectiveness of the fungi is highly substrate- and species-dependent. For example, C. subvermispora, a wood-decaying fungus, was more effective at degrading woody substrates than herbaceous substrates (e.g., wheat straw and soybean straw) (Wan and Li, 2012). Brown-rot fungi (e.g., Serpula lacrymans) were more effective degraders of hemicellulose and cellulose, suggesting they are suitable for substrates low in lignin but high in cellulose (Wan and Li, 2012). Soft-rot fungi (e.g., Ceratocystis spp.) have shown to degrade cellulose and hemicellulose on woody substrates (Madadi and Abbas, 2017).
Results of Isibika et al. (2019) confirmed that substrate fungal pretreatment can also increase BSFL rearing performance but highlight that pretreatment time and fungi choice can highly influence the results. Pretreatment of banana peels with Trichoderma reesei for 7–21 days increased larval mass by 26–70% and bioconversion ratio from 14% (after 7 days pretreatment) to 61% (after 14 days pretreatment). However, pretreatment of banana peels with Rhizopus oligosporus decreased bioconversion rate by 7.5% when pretreated for 7 days, potentially due to the low fiber degradation of 10% compared to T. reesie (40–53%).
Although fungi are the main source of lignocellulosic enzymes (Taha et al., 2015), many bacteria also possess enzymes that are effective degraders of lignocellulosic fractions, such as the genera Bacillus (e.g., Bacillus subtilis), and Streptomyces, which increased biogas production for agricultural crops and residues by 11 to 42% compared to those without pretreatment (Zhong et al., 2011; Zhao et al., 2019) (Supplementary Table 4). These strains may be present in the larval gut and could potentially improve the digestion of lignocellulose by isolating these strains and using them as a pretreatment for BSFL. Additionally, as discussed by Gorrens et al. (2021), the addition of dominant endogenous bacteria, such as Enterococcus spp. and Providencia spp. during BSFL rearing can positively influence the bioconversion of difficult to digest substrates into valuable biomass due to the production of lignocellulose-decomposing enzymes (Jiang et al., 2019). Similarly, pectinase activity was described by Callegari et al. (2020) for Klebsiella spp. isolated from BSFL.
In the last decade, interest in using bacteria on animal manure and agricultural crops and residues for BSFL rearing has increased, but as a co-treatment (i.e., bacteria are added together with larvae at the beginning of rearing) (Yu et al., 2011; Zheng et al., 2012; Somroo et al., 2019). On soybean curd residue, Lactobacillus buchneri increased the bioconversion rate and larval mass by 38 and 39%, respectively (Somroo et al., 2019). Additionally, Mazza et al. (2020) inoculated chicken manure with isolated pure bacterial strains from larval eggs and gut and evaluated their effect on larval performance. From the bacterial strains tested, Kocuria marina, Proteus mirabilis, and B. subtilis improved larval mass by 15–19% compared to the control (without bacteria). In contrast, Gordonia sihwensis and Micrococcus luteus led to a decrease in larval mass by up to 1% (Supplementary Table 4). Substrate characteristics such as lignin and hemicellulose content and the cellulose crystallinity have shown to affect enzyme activity (Taha et al., 2015).
Future research on biological pretreatment should evaluate whether the use of white-rot fungi, T. reesei, or bacteria such as B. subtilis, L. buchneri, Pseudomonas spp., Enterococcus spp., and Providencia spp. would enhance the production of bacteria that excrete cellulosic enzymes (e.g., cellulase) which have been identified in the BSFL gut (Callegari et al., 2020), thus increasing digestion of the cellulose while improving overall conversion efficiency. Taha et al. (2015) observed lignocellulosic enzymatic increase from fungi and bacteria between 3 and 12 days; therefore, a minimum pretreatment time of 3 days should be studied.
Similar to composting and anaerobic digestion, individual mechanical, chemical and biological pretreatments, or a combination of these, may improve BSFL bioconversion performance with lignocellulosic substrates. As demonstrated by the variable efficiencies reported in this review and the unknown effects of treatments on BSFL, laboratory and pilot-scale studies are needed to determine transferability for industrial application. These efforts should work toward broad substrate-specific process recommendations (e.g. particle size, temperature, chemical and dose) and gaining further understanding of the underlying effects on the BSFL digestion process.
Based on this review, bioconversion efficiency could be determined by mechanical pretreatment of agricultural crops and residues, municipal organic solid waste, and animal manure (e.g., with <6 mm particle size). Because mechanical pretreatment may release water (e.g. in fruit and vegetable wastes) bound behind cell walls, substrate dewatering or addition of a drier substrate (e.g. paper) might be required to produce a suitable BSFL substrate. Thermal treatments with lower temperature treatments and longer holding times (e.g. 80–90°C for >60 min) may provide positive effects for some substrates. However, this increases energy requirements, and depending on the energy source could impact the environmental benefits of BSFL products in comparison to current animal feed benchmarks such as fish meal.
Previous studies have shown improved performance with NaOH, urea, and ammonia treatments for all lignocellulosic substrates. For urea and ammonia pretreatment, doses of ≥1% with pretreatment times of ≤ 7 days could be effective. Future research should focus on increasing process improvements beyond those reported and assess whether chemical pretreatments have an influence on downstream processing (e.g. quality of feed and fertilizer products) to justify the additional operational resource requirements of chemical addition for BSFL rearing.
Biological pretreatments (e.g. with white-rot fungi or T. reesei) have shown to effectively delignify substrates and may stimulate microbial enzyme activities that will further improve digestibility by BSFL. Treatment with T. reesei for 14 days positively increased BSFL bioconversion. Additionally, the use of bacterial pretreatments, such as B. subtilis, with a minimum pretreatment time of 3 days, could also enhance microbial enzymatic activities. However, similar to chemical pretreatment, the dose and treatment time for biological pretreatments need to be optimized, as typical pretreatment times used (20–30 days) may not be feasible.
For all pretreatments, efficiency gains need to be balanced with additional capital and operational resources, such as investment, labor, time, process additive cost, and energy. An economic assessment of the BSFL production chain should be conducted to evaluate the minimum process and bioconversion needed to justify the use of pretreatment for agri-food wastes. Ultimately, life cycle assessments should evaluate the effects substrate pretreatment may have across the different environmental impact categories.
DP performed the main topic review, drafted the original manuscript, and conducted revisions. MG contributed with manuscript revision, ideas, feedback, and writing throughout manuscript development. DV contributed with manuscript revision and feedback. CZ and AM performed manuscript revision and supervised the work presented. AM provided the financial support. All authors contributed to the article and approved the submitted version.
This work was supported by the European Union's Horizon 2020 Research and Innovation Program under grant agreement no. 861976 project SUStainable INsect CHAIN (SUSINCHAIN).
The authors declare that the research was conducted in the absence of any commercial or financial relationships that could be construed as a potential conflict of interest.
All claims expressed in this article are solely those of the authors and do not necessarily represent those of their affiliated organizations, or those of the publisher, the editors and the reviewers. Any product that may be evaluated in this article, or claim that may be made by its manufacturer, is not guaranteed or endorsed by the publisher.
The authors would like to sincerely thank the European Union's Horizon 2020 project SUSINCHAIN partners from work package 2 (Inagro and for Farmers Corporate Services BV) for their valuable feedback on selecting pretreatment technologies with potential to improve insect production.
The Supplementary Material for this article can be found online at: https://www.frontiersin.org/articles/10.3389/fsufs.2021.745894/full#supplementary-material
Agbor, V. B., Cicek, N., Sparling, R., Berlin, A., and Levin, D. B. (2011). Biomass pretreatment: Fundamentals toward application. Biotechnol. Adv. 29, 675–685. doi: 10.1016/j.biotechadv.2011.05.005
Alexandropoulou, M., Antonopoulou, G., Fragkou, E., Ntaikou, I., and Lyberatos, G. (2017). Fungal pretreatment of willow sawdust and its combination with alkaline treatment for enhancing biogas production. Environ. Manag. 203, 704–713. doi: 10.1016/j.jenvman.2016.04.006
Angelidaki, I., and Ahring, B. K. (2000). Methods for increasing the biogas potential from the recalcitrant organic matter contained in manure. Water Sci. Technol. 41, 189–194. doi: 10.2166/wst.2000.0071
Ansari, M., Zafar, U., Ejaz, U., Sohail, M., Pirzada, A., and Aman, A. (2021). Comparison of composting of chemically pretreated and fermented sugarcane bagasse for zero-waste biorefinery. J. Mater. Cycles Waste Manag. 23, 911–921. doi: 10.1007/s10163-021-01176-w
Antonopoulou, G., and Gavala, H. N. (2015). The effect of aqueous ammonia soaking pretreatment on methane generation using different lignocellulosic biomasses. Waste Biomass Valorization 6, 281–291. doi: 10.1007/s12649-015-9352-9
Appels, L., Degrève, J., Van der Bruggen, B., Van Impe, J., and Dewil, R. (2010). Influence of low temperature thermal pre-treatment on sludge solubilisation, heavy metal release and anaerobic digestion. Bioresour. Technol. 101, 5743–5748. doi: 10.1016/j.biortech.2010.02.068
Atelge, M. R., Atabani, A. E., Banu, J. R., Krisa, D., Kaya, M., Eskicioglu, C., et al. (2020). A critical review of pretreatment technologies to enhance anaerobic digestion and energy recovery. Fuel 270:117494. doi: 10.1016/j.fuel.2020.117494
Behera, S., Arora, R., Nandhagopal, N., and Kumar, S. (2014). Importance of chemical pretreatment for bioconversion of lignocellulosic biomass. Renew. Sustain. Energy Rev. 36, 91–106. doi: 10.1016/j.rser.2014.04.047
Bhagwat, S., Ratnaparkhe, S., and Kumar, A. (2015). Biomass pre-treatment methods and their economic viability for efficient production of biofuel. Br. Biotechnol. J. 8, 1–17. doi: 10.9734/BBJ/2015/18284
Bochmann, G., and Montgomery, L. F. R. (2013). “Storage and pre-treatment of substrates for biogas production,” in The Biogas Handbook: Science, Production and Applications, eds A. Wellinger, J. Murphy, and D. Baxter (Sawston: Woodhead Publishing), 85–103. doi: 10.1533/9780857097415.1.85
Callegari, M., Jucker, C., Fusi, M., Leonardi, M. G., Daffonchio, D., Borin, S., et al. (2020). Hydrolytic profile of the culturable gut bacterial community associated with Hermetia illucens. Front. Microbiol. 11:1965. doi: 10.3389/fmicb.2020.01965
Cao, Y., Wang, J., Huang, H., Sun, E., Butterly, C., Xu, Y., et al. (2019). Spectroscopic evidence for hyperthermophilic pretreatment intensifying humification during pig manure and rice straw composting. Bioresour. Technol. 294:122131. doi: 10.1016/j.biortech.2019.122131
Carlsson, M., Lagerkvist, A., and Morgan-Sagastume, F. (2012). The effects of substrate pre-treatment on anaerobic digestion systems: a review. Waste Manag. 32, 1634–1650. doi: 10.1016/j.wasman.2012.04.016
Carrère, H., Antonopoulou, G., Affes, R., Passos, F., Battimelli, A., Lyberatos, G., and Ferrer, I. (2016). Review of feedstock pretreatment strategies for improved anaerobic digestion: from lab-scale research to full-scale application. Bioresour. Technol. 199, 386–397. doi: 10.1016/j.biortech.2015.09.007
Carrère, H., Dumas, C., Battimelli, A., Batstone, D. J., Delgenès, J. P., Steyer, J. P., et al. (2010). Pretreatment methods to improve sludge anaerobic degradability : a review. Hazard. Mater. 183, 1–15. doi: 10.1016/j.jhazmat.2010.06.129
Carrère, H., Monlau, F., Barakat, A., Dumas, C., and Jean-Philippe, S. (2011). Biogas From Lignocellulosic Biomass : Interest of Pretreatments. International Congress Progress in Biogas II, Mar 2011, Stuttgart, Germany. (hal-02749537).
Cesaro, A., and Belgiorno, V. (2014). Pretreatment methods to improve anaerobic biodegradability of organic municipal solid waste fractions. Chem. Eng. J. 240, 24–37. doi: 10.1016/j.cej.2013.11.055
Chen, S., Liao, W., C, L., Wen, Z., Kincaid, R. L., Harrison, J. H., et al. (2003). Value-Added Chemicals From Animal Manure. Richland, WA: Pacific Northwest National Laboratory. doi: 10.2172/15009485
Dortmans, B. M. A., Egger, J., Diener, S., and Zurbrügg, C. (2021). Black Soldier Fly Biowaste Processing - A Step-by-Step Guide, 2nd Edn. (Eawag: Swiss Federal Institute of Aquatic Science and Technology).
Edwiges, T., Frare, L., Mayer, B., Lins, L., Triolo, J. M., Flotats, X., et al. (2018). Influence of chemical composition on biochemical methane potential of fruit and vegetable Waste Manag. 71, 618–625. doi: 10.1016/j.wasman.2017.05.030
Galbe, M., and Zacchi, G. (2012). Pretreatment: the key to efficient utilization of lignocellulosic materials. Biomass Bioenergy 46, 70–78. doi: 10.1016/j.biombioe.2012.03.026
Gold, M., Binggeli, M., Kurt, F., de Wouters, T., Reichlin, M., Zurbrügg, C., et al. (2020a). Novel experimental methods for the investigation of Hermetia illucens (Diptera: Stratiomyidae) larvae. J. Insect Sci. 20, 1–9. doi: 10.1093/jisesa/ieaa057
Gold, M., Cassar, C. M., Zurbrügg, C., Kreuzer, M., Boulos, S., Diener, S., et al. (2020b). Biowaste treatment with black soldier fly larvae: increasing performance through the formulation of biowastes based on protein and carbohydrates. Waste Manag. 102, 319–329. doi: 10.1016/j.wasman.2019.10.036
Gold, M., Tomberlin, J. K., Diener, S., Zurbrügg, C., and Mathys, A. (2018). Decomposition of biowaste macronutrients, microbes, and chemicals in black soldier fly larval treatment: a review. Waste Manag. 82, 302–318. doi: 10.1016/j.wasman.2018.10.022
Gorrens, E., Van Moll, L., Frooninckx, L., De Smet, J., and Van Campenhout, L. (2021). Isolation and identification of dominant bacteria from black soldier fly larvae (Hermetia illucens) envisaging practical applications. Front. Microbiol. 12:665546. doi: 10.3389/fmicb.2021.665546
Gustavsson, J., Cederberg, C., Sonesson, U., Otterdijk, V. R., and Meybeck, A. (2011). Global Food Losses and Food Waste. Rome: FAO.
Hamelinck, C. N., Van Hooijdonk, G., and Faaij, A. P. C. (2005). Ethanol from lignocellulosic biomass: techno-economic performance in short-, middle- and long-term. Biomass Bioenergy 28, 384–410. doi: 10.1016/j.biombioe.2004.09.002
Hartmann, H., and Ahring, B. K. (2005). Anaerobic digestion of the organic fraction of municipal solid waste: influence of co-digestion with manure. Water Res. 39, 1543–1552. doi: 10.1016/j.watres.2005.02.001
He, Y., Pang, Y., Liu, Y., Li, X., and Wang, K. (2008). Physicochemical characterization of rice straw pretreated with sodium hydroxide in the solid state for enhancing biogas production. Energy Fuels 22, 2775–2781. doi: 10.1021/ef8000967
Hendriks, A. T. W. M., and Zeeman, G. (2009). Pretreatments to enhance the digestibility of lignocellulosic biomass. Bioresour. Technol. 100, 10–18. doi: 10.1016/j.biortech.2008.05.027
Hoornweg, D., and Bhada-Tata, P. (2012). What a Waste: A Global Review of Solid Waste Management. Washington, DC: World Bank.
Huang, Y., L, D., Shah, G. M., Chen, W., Wang, W., Xu, Y., and Huang, H. (2019). Hyperthermophilic pretreatment composting significantly accelerates humic substances formation by regulating precursors production and microbial communities. Waste Manag. 92, 89–96. doi: 10.1016/j.wasman.2019.05.021
Isibika, A., Vinnerås, B., Kibazohi, O., Zurbrügg, C., and Lalander, C. (2019). Pre-treatment of banana peel to improve composting by black soldier fly (Hermetia illucens (L.), Diptera: Stratiomyidae) larvae. Waste Manag. 100, 151–160. doi: 10.1016/j.wasman.2019.09.017
Jiang, C. L., Jin, W. Z., Tao, X. H., Zhang, Q., Zhu, J., Feng, S. Y., et al. (2019). Black soldier fly larvae (Hermetia illucens) strengthen the metabolic function of food waste biodegradation by gut microbiome. Microb. Biotechnol. 12, 528–543. doi: 10.1111/1751-7915.13393
Jurado, E., Gavala, H. N., and Skiadas, I. V. (2013a). Enhancement of methane yield from wheat straw, miscanthus and willow using aqueous ammonia soaking. Environ. Technol. 34, 2069–2075. doi: 10.1080/09593330.2013.826701
Jurado, E., Skiadas, I. V., and Gavala, H. N. (2013b). Enhanced methane productivity from manure fibers by aqueous ammonia soaking pretreatment. Appl. Energy 109, 104–111. doi: 10.1016/j.apenergy.2013.03.075
Kanauchi, O., Mitsuyama, K., and Araki, Y. (2001). Development of a functional germinated barley foodstuff from brewer's spent grain for the treatment of ulcerative colitis. J. Am. Soc. Brewing Chem. 59, 59–62. doi: 10.1094/ASBCJ-59-0059
Kim, J. S., Lee, Y. Y., and Kim, T. H. (2016). A review on alkaline pretreatment technology for bioconversion of lignocellulosic biomass. Bioresour. Technol. 199, 42–48. doi: 10.1016/j.biortech.2015.08.085
Kim, T. H. (2013). “Pretreatment of lignocellulosic biomass,” in Bioprocessing Technologies in Biorefinery for Sustainable Production of Fuels, Chemicals, and Polymers, eds Y. Shang-Tian, H. A. El-Enshasy, and N. Thongchul (Hoboken, NJ: John Wiley & Sons, Inc.), (pp. 91–109). doi: 10.1002/9781118642047.ch6
Klammsteiner, T., Turan, V., Fern, M., Oberegger, S., and Insam, H. (2020). Suitability of black soldier fly frass as soil amendment and implication for organic waste hygienization. Agronomy 10, 1–12. doi: 10.3390/agronomy10101578
Kratky, L., and Jirout, T. (2011). Biomass Size Reduction Machines for Enhancing Biogas Production. Chem. Eng. Technol. 34, 391–399. doi: 10.1002/ceat.201000357
Lalander, C., Diener, S., Zurbrügg, C., and Vinnerås, B. (2019). Effects of feedstock on larval development and process efficiency in waste treatment with black soldier fly (Hermetia illucens). J. Cleaner Prod. 208, 211–219. doi: 10.1016/j.jclepro.2018.10.017
Li, Q., Zheng, L., Qiu, N., Cai, H., Tomberlin, J. K., and Yu, Z. (2011). Bioconversion of dairy manure by black soldier fly (Diptera: Stratiomyidae) for biodiesel and sugar production. Waste Manag. 31, 1316–1320. doi: 10.1016/j.wasman.2011.01.005
Liang, Y. G., Cheng, B., Si, Y., Bin Cao, D. J., Nie, E., Tang, J., et al. (2014). Physicochemical changes of rice straw after lime pretreatment and mesophilic dry digestion. Biomass Bioenergy 71, 106–112. doi: 10.1016/j.biombioe.2014.10.020
Liu, C., Wang, C., Yao, H., and Chapman, S. J. (2021). Pretreatment is an important method for increasing the conversion efficiency of rice straw by black soldier fly larvae based on the function of gut microorganisms. Sci. Total Environ. 762:144118. doi: 10.1016/j.scitotenv.2020.144118
Liu, S., Li, X., Wu, S., He, J, Pang, C., Deng, Y., and Dong, R. (2014). Fungal Pretreatment by Phanerochaete chrysosporium for enhancement of biogas production from corn stover silage. Appl. Biochem. Biotechnol. 174, 1907–1918. doi: 10.1007/s12010-014-1185-7
Liu, Z., Minor, M., Morel, P. C. H., and Najar-Rodriguez, A. J. (2018). Bioconversion of three organic wastes by black soldier fly (Diptera: Stratiomyidae) larvae. Environ. Entomol. 47, 1609–1617. doi: 10.1093/ee/nvy141
Liu, Z. L., Saha, B. C., and Slininger, P. J. (2008). “Lignocellulosic biomass convertion to ethanol by Saccharomyces,” in Bioenergy, eds J. D. Wall, C. S. Harwood, and A. Demain (Washington, DC: ASM Press), 17–36. doi: 10.1128/9781555815547.ch2
López Torres, M., and Espinosa Lloréns, M. del C. (2008). Effect of alkaline pretreatment on anaerobic digestion of solid wastes. Waste Manag. 28, 2229–2234. doi: 10.1016/j.wasman.2007.10.006
Madadi, M., and Abbas, A. (2017). Lignin degradation by fungal pretreatment: a review. J. Plant Pathol. Microbiol. 8:2. doi: 10.4172/2157-7471.1000398
Mazza, L., Xiao, X., ur Rehman, K., Cai, M., Zhang, D., Fasulo, S., et al. (2020). Management of chicken manure using black soldier fly (Diptera: Stratiomyidae) larvae assisted by companion bacteria. Waste Manag. 102, 312–318. doi: 10.1016/j.wasman.2019.10.055
Menardo, S., Airoldi, G., and Balsari, P. (2012). The effect of particle size and thermal pre-treatment on the methane yield of four agricultural by-products. Bioresour. Technol. 104, 708–714. doi: 10.1016/j.biortech.2011.10.061
Meneguz, M., Schiavone, A., Gai, F., Dama, A., Lussiana, C., Renna, M., et al. (2018). Effect of rearing substrate on growth performance, waste reduction efficiency and chemical composition of black soldier fly (Hermetia illucens) larvae. J. Sci. Food Agric. 98, 5776–5784. doi: 10.1002/jsfa.9127
Mertenat, A., Diener, S., and Zurbrügg, C. (2019). Black soldier fly biowaste treatment – assessment of global warming potential. Waste Manag. 84, 173–181. doi: 10.1016/j.wasman.2018.11.040
Mirko, C., Pezzolla, D., Chiara, T., and Giovanni, G. (2021). Pretreatments for enhanced biomethane production from buckwheat hull: Effects on organic matter degradation and process sustainability. J. Environ. Manag. 285:112098. doi: 10.1016/j.jenvman.2021.112098
Mirtsou-Xanthopoulou, C., Jurado, E., Skiadas, I. V., and Gavala, H. N. (2014). Effect of aqueous ammonia soaking on the methane yield and composition of digested manure fibers applying different ammonia concentrations and treatment durations. Energies 1014, 4157–4168. doi: 10.3390/en7074157
Mishra, S., Singh, P. K., Dash, S., and Pattnaik, R. (2018). Microbial pretreatment of lignocellulosic biomass for enhanced biomethanation and waste management. 3 Biotech 8:458. doi: 10.1007/s13205-018-1480-z
Mosier, N., Wyman, C., Dale, B., Elander, R., Lee, Y. Y., Holtzapple, M., et al. (2005). Features of promising technologies for pretreatment of lignocellulosic biomass. Bioresour. Technol. 96, 673–686. doi: 10.1016/j.biortech.2004.06.025
Mshandete, A., Björnsson, L., Kivaisi, A. K., Rubindamayugi, M. S. T., and Mattiasson, B. (2006). Effect of particle size on biogas yield from sisal fibre waste. Renew. Energy 31, 2385–2392. doi: 10.1016/j.renene.2005.10.015
Müller, J. A. (2000). Pretreatment processes for the recycling and reuse of sewage sludge. Water Sci. Technol. 42, 167–174. doi: 10.2166/wst.2000.0197
Mussatto, S. I., Dragone, G., and Roberto, I. C. (2006). Brewers' spent grain: generation, characteristics and potential applications. J. Cereal Sci. 43, 1–14. doi: 10.1016/j.jcs.2005.06.001
Mustafa, A. M., Poulsen, T. G., and Sheng, K. (2016). Fungal pretreatment of rice straw with Pleurotus ostreatus and Trichoderma reesei to enhance methane production under solid-state anaerobic digestion. Appl. Energy 180, 661–671. doi: 10.1016/j.apenergy.2016.07.135
Nava-Valente, N., Del Ángel-Coronel, O. A., Atenodoro-Alonso, J., and Lopez-Escobar, L. A. (2021). Effect of thermal and acid pre-treatment on increasing organic loading rate of anaerobic digestion of coffee pulp for biogas production. Biomass Conv. Bioref . 1–14. doi: 10.1007/s13399-021-01529-3
Neves, L., Ribeiro, R., Oliveira, R., and Alves, M. M. (2006). Enhancement of methane production from barley waste. Biomass Bioenergy 30, 599–603. doi: 10.1016/j.biombioe.2005.12.003
Nguyen, T. T. X., Tomberlin, J. K., and Vanlaerhoven, S. (2013). Influence of resources on Hermetia illucens (Diptera: Stratiomyidae) larval development. J. Med. Entomol. 50, 898–906. doi: 10.1603/ME12260
Niasar, H. S., Karimi, K., Zilouei, H., Salehian, P., and Jeihanipour, A. (2011). Effects of lime pretreatment on biogas production from dairy cattle manure. Minerva Biotec 23, 77–82.
Nyakeri, E. M., Ogola, H. J. O., Ayieko, M. A., and Amimo, F. A. (2017). Valorisation of organic waste material: growth performance of wild black soldier fly larvae (Hermetia illucens) reared on different organic wastes. J. Insects Food Feed 3, 193–202. doi: 10.3920/JIFF2017.0004
Oyedeji, O., Gitman, P., Qu, J., and Webb, E. (2020). Understanding the impact of lignocellulosic biomass variability on the size reduction process: a review. ACS Sustain. Chem. Eng. 8, 2327–2343. doi: 10.1021/acssuschemeng.9b06698
Palma, L., Fernandez-Bayo, J., Niemeier, D., Pitesky, M., and VanderGheynst, J. S. (2019). Managing high fiber food waste for the cultivation of black soldier fly larvae. NPJ Sci. Food 3:15. doi: 10.1038/s41538-019-0047-7
Rao, M. S., and Singh, S. P. (2004). Bioenergy conversion studies of organic fraction of MSW: Kinetic studies and gas yield-organic loading relationships for process optimisation. Bioresour. Technol. 95, 173–185. doi: 10.1016/j.biortech.2004.02.013
Rehman, K. U., Cai, M., Xiao, X., Zheng, L., Wang, H., Soomro, A. A., et al. (2017). Cellulose decomposition and larval biomass production from the co-digestion of dairy manure and chicken manure by mini-livestock (Hermetia illucens L.). J. Environ. Manag. 196, 458–465. doi: 10.1016/j.jenvman.2017.03.047
Sambusiti, C., Monlau, F., Ficara, E., Carrère, H., and Malpei, F. (2013). A comparison of different pre-treatments to increase methane production from two agricultural substrates. Appl. Energy 104, 62–70. doi: 10.1016/j.apenergy.2012.10.060
Senol, H. (2021). Effects of NaOH, thermal, and combined NaOH-thermal pretreatments on the biomethane yields from the anaerobic digestion of walnut shells. Environ. Sci. Pollut. Res. 28, 21661–21673. doi: 10.1007/s11356-020-11984-6
Sharma, H. K., Xu, C., and Qin, W. (2019). Biological pretreatment of lignocellulosic biomass for biofuels and bioproducts: an overview. Waste Biomass Valorization 10, 235–251. doi: 10.1007/s12649-017-0059-y
Sharma, S. K., Mishra, I. M., Sharma, M. P., and Saini, J. S. (1988). Effect of particle size on biogas generation from biomass residues. Biomass 17, 251–263. doi: 10.1016/0144-4565(88)90107-2
Shumo, M., Osuga, I. M., Khamis, F. M., Tanga, C. M., Fiaboe, K. K. M., Subramanian, S., et al. (2019). The nutritive value of black soldier fly larvae reared on common organic waste streams in Kenya. Sci. Rep. 9, 1–13. doi: 10.1038/s41598-019-46603-z
Smetana, S., Palanisamy, M., Mathys, A., and Heinz, V. (2016). Sustainability of insect use for feed and food: life cycle assessment perspective. J. Cleaner Prod. 137, 741–751. doi: 10.1016/j.jclepro.2016.07.148
Smetana, S., Schmitt, E., and Mathys, A. (2019). Sustainable use of Hermetia illucens insect biomass for feed and food: attributional and consequential life cycle assessment. Resources, Conserv. Recycling 144, 285–296. doi: 10.1016/j.resconrec.2019.01.042
Somroo, A. A., ur Rehman, K., Zheng, L., Cai, M., Xiao, X., Hu, S., et al. (2019). Influence of Lactobacillus buchneri on soybean curd residue co-conversion by black soldier fly larvae (Hermetia illucens) for food and feedstock production. Waste Manag. 86, 114–122. doi: 10.1016/j.wasman.2019.01.022
Sun, Y., and Cheng, J. (2002). Hydrolysis of lignocellulosic materials for ethanol production: a review. Bioresour. Technol. 83, 1–11. doi: 10.1016/S0960-8524(01)00212-7
Taha, M., Shahsavari, E., Al-Hothaly, K., Mouradov, A., Smith, A. T., Ball, A. S., et al. (2015). Enhanced biological straw saccharification through coculturing of lignocellulose-degrading microorganisms. Appl. Biochem. Biotechnol. 175, 3709–3728. doi: 10.1007/s12010-015-1539-9
Taherdanak, M., and Zilouei, H. (2014). Improving biogas production from wheat plant using alkaline pretreatment. Fuel 115, 714–719. doi: 10.1016/j.fuel.2013.07.094
United Nations (2017). World Population Prospects: The 2017 Revision, Key Findings, And Advance Tables. New York, NY: United Nations.
van Kuijk, S. J. A., Sonnenberg, A. S. M., Baars, J. J. P., Hendriks, W. H., and Cone, J. W. (2015). Fungal treated lignocellulosic biomass as ruminant feed ingredient: a review. Biotechnol. Adv. 33, 191–202. doi: 10.1016/j.biotechadv.2014.10.014
Victorin, M., Davidsson, Å., and Wallberg, O. (2020). Characterization of mechanically pretreated wheat straw for biogas production. Bioenergy Res. 13, 833–844. doi: 10.1007/s12155-020-10126-7
Wan, C., and Li, Y. (2012). Fungal pretreatment of lignocellulosic biomass. Biotechnol. Adv. 30, 1447–1457. doi: 10.1016/j.biotechadv.2012.03.003
Willett, W., Rockström, J., Loken, B., Springmann, M., Lang, T., Vermeulen, S., et al. (2019). Food in the anthropocene: the EAT–lancet commission on healthy diets from sustainable food systems. Lancet Commissions 393, 447–492. doi: 10.1016/S0140-6736(18)31788-4
Yao, Y., Bergeron, A. D., and Davaritouchaee, M. (2018). Methane recovery from anaerobic digestion of urea-pretreated wheat straw. Renew. Energy 115, 139–148. doi: 10.1016/j.renene.2017.08.038
Yu, G., Cheng, P., Chen, Y., Li, Y., Yang, Z., Chen, Y., et al. (2011). Inoculating poultry manure with companion bacteria influences growth and development of black soldier fly (Diptera: Stratiomyidae) larvae. Environ. Entomol. 40, 30–35. doi: 10.1603/EN10126
Zhao, J., Zheng, Y., and Li, Y. (2014). Fungal pretreatment of yard trimmings for enhancement of methane yield from solid-state anaerobic digestion. Bioresour. Technol. 156, 176–181. doi: 10.1016/j.biortech.2014.01.011
Zhao, Y., Xu, C., Ai, S., Wang, H., Gao, Y., Yan, L., et al. (2019). Biological pretreatment enhances the activity of functional microorganisms and the ability of methanogenesis during anaerobic digestion. Bioresour. Technol. 290:121660. doi: 10.1016/j.biortech.2019.121660
Zheng, L., Hou, Y., Li, W., Yang, S., Li, Q., and Yu, Z. (2012). Biodiesel production from rice straw and restaurant waste employing black soldier fly assisted by microbes. Energy 47, 225–229. doi: 10.1016/j.energy.2012.09.006
Zhong, W., Zhang, Z., Luo, Y., Sun, S., Qiao, W., and Xiao, M. (2011). Effect of biological pretreatments in enhancing corn straw biogas production. Bioresour. Technol. 102, 11177–11182. doi: 10.1016/j.biortech.2011.09.077
Zhu, B., Zhang, R., Gikas, P., Rapport, J., Jenkins, B., and Li, X. (2010). Biogas production from municipal solid wastes using an integrated rotary drum and anaerobic-phased solids digester system. Bioresour. Technol. 101, 6374–6380. doi: 10.1016/j.biortech.2010.03.075
Keywords: Hermetia illucens, waste management, lignocellulosic biomass, bioconversion, substrate digestibility, biodegradable, Fiber, lignin
Citation: Peguero DA, Gold M, Vandeweyer D, Zurbrügg C and Mathys A (2022) A Review of Pretreatment Methods to Improve Agri-Food Waste Bioconversion by Black Soldier Fly Larvae. Front. Sustain. Food Syst. 5:745894. doi: 10.3389/fsufs.2021.745894
Received: 22 July 2021; Accepted: 20 December 2021;
Published: 27 January 2022.
Edited by:
Raul Moral, Miguel Hernández University of Elche, SpainReviewed by:
Warmadewanthi Warmadewanthi, Sepuluh Nopember Institute of Technology, IndonesiaCopyright © 2022 Peguero, Gold, Vandeweyer, Zurbrügg and Mathys. This is an open-access article distributed under the terms of the Creative Commons Attribution License (CC BY). The use, distribution or reproduction in other forums is permitted, provided the original author(s) and the copyright owner(s) are credited and that the original publication in this journal is cited, in accordance with accepted academic practice. No use, distribution or reproduction is permitted which does not comply with these terms.
*Correspondence: Alexander Mathys, YWxleGFuZGVyLm1hdGh5c0BoZXN0LmV0aHouY2g=
Disclaimer: All claims expressed in this article are solely those of the authors and do not necessarily represent those of their affiliated organizations, or those of the publisher, the editors and the reviewers. Any product that may be evaluated in this article or claim that may be made by its manufacturer is not guaranteed or endorsed by the publisher.
Research integrity at Frontiers
Learn more about the work of our research integrity team to safeguard the quality of each article we publish.