- 1Alliance of Bioversity International and International Center for Tropical Agriculture, Africa Hub, Nairobi, Kenya
- 2Alliance of Bioversity International and International Center for Tropical Agriculture, Hanoi, Vietnam
- 3Alliance of Bioversity International and International Center for Tropical Agriculture, Latin-America Hub, Cali, Colombia
- 4Alliance of Bioversity International and International Center for Tropical Agriculture, Rome, Italy
- 5Department of Environmental System Science, ETH Zurich, Zurich, Switzerland
- 6Department of Human Ecology, Rutgers University, New Brunswick, NJ, United States
- 7Department of Soil Ecology, Bayreuth Center of Ecology and Environmental Research, University of Bayreuth, Bayreuth, Germany
- 8Faculty of Tropical AgriSciences, Czech University of Life Sciences Prague, Prague, Czechia
- 9Mohammed VI Polytechnic University, AgroBioSciences, Agricultural Innovations and Technology Transfer Centre (AITTC), Ben Guerir, Morocco
- 10Farming Systems Ecology, Wageningen University & Research, Wageningen, Netherlands
- 11The Nature Conservancy, Arlington, VA, United States
- 12International Center for Agricultural Research in the Dry Areas, Tunis, Tunisia
- 13International Center for Agricultural Research in the Dry Areas, Amman, Jordan
- 14Mazingira Centre, Sustainable Livestock Systems, International Livestock Research Institute, Nairobi, Kenya
Livestock are critical for incomes, livelihoods, nutrition and ecosystems management throughout the global South. Livestock production and the consumption of livestock-based foods such as meat, cheese, and milk is, however, under global scrutiny for its contribution to global warming, deforestation, biodiversity loss, water use, pollution, and land/soil degradation. This paper argues that, although the environmental footprint of livestock production presents a real threat to planetary sustainability, also in the global south, this is highly contextual. Under certain context-specific management regimes livestock can deliver multiple benefits for people and planet. We provide evidence that a move toward sustainable intensification of livestock production is possible and could mitigate negative environmental impacts and even provide critical ecosystem services, such as improved soil health, carbon sequestration, and enhanced biodiversity on farms. The use of cultivated forages, many improved through selection or breeding and including grasses, legumes and trees, in integrated crop-tree-livestock systems is proposed as a stepping stone toward agroecological transformation. We introduce cultivated forages, explain their multi-functionality and provide an overview of where and to what extent the forages have been applied and how this has benefited people and the planet alike. We then examine their potential to contribute to the 13 principles of agroecology and find that integrating cultivated forages in mixed crop-tree-livestock systems follows a wide range of agroecological principles and increases the sustainability of livestock production across the globe. More research is, however, needed at the food system scale to fully understand the role of forages in the sociological and process aspects of agroecology. We make the case for further genetic improvement of cultivated forages and strong multi-disciplinary systems research to strengthen our understanding of the multidimensional impacts of forages and for managing agro-environmental trade-offs. We finish with a call for action, for the agroecological and livestock research and development communities to improve communication and join hands for a sustainable agri-food system transformation.
Importance of Sustainable Livestock Production Systems
Even though the role of animal based proteins as part of a sustainable twenty-first century food system is a highly debated topic (Meybeck and Gitz, 2017), the livestock sector currently plays a key role in food and nutrition security, particularly in developing countries. Livestock products (meat, milk and eggs) contribute 15% and 31% of the global per capita calorie and protein supply, respectively (Godde et al., 2021). Large regional differences characterize the nutritional contributions of livestock, with low intakes of animal-source food in the Global South compared with excesses in the Global North (Meyfroidt, 2018). Livestock are kept by more than half of rural households (FAO, 2018, 2021), with more than 844 million people worldwide receiving some income from agriculture, and the livestock sector contributing about 40% of the value-added in agriculture (Gontijo de Lima et al., 2015).
In general, family farming—often by smallholders cultivating less than two hectares—is still the predominant form of livestock production in the global South, in terms of numbers as well as occupied area (Lowder et al., 2021). On these family farms, livestock production mainly occurs in mixed crop-livestock systems (Herrero et al., 2010), where livestock has a multitude of functions, ranging from the provision of food, nutrition, income and risk reduction to farmers as well as the contribution of essential nutrients and draft power to reduce drudgery and improve crop productivity. The farms are further connected to—mostly local, regional, and national—markets where they generate a plethora of other jobs along livestock value chains (Lie et al., 2017; Bravo et al., 2018; Enciso et al., 2018).
In response to increasing demand for livestock products, these traditionally mixed systems increasingly intensify and are thereby replaced by specialized livestock production systems with spatially decoupled crop and livestock production and high levels of resource depletion and/or environmental pollution (Garrett et al., 2017a; Jin et al., 2020). For instance, about 51% of total feed nitrogen (N) in China was imported in 2015, greatly increasing energy requirements for transport, greenhouse gas (GHG) emissions abroad, and causing nutrient surpluses in China (Du et al., 2018; Zhang et al., 2020). The spatial decoupling of crop and livestock production is further associated with smaller fractions of manure returned to cropland and larger losses of manure N to surface and ground waters and GHG emissions (Bai et al., 2018). Hence, specialized crop production systems increasingly rely on synthetic fertilizers, and have higher environmental costs per unit of crop product (Zhao et al., 2017). Lastly, the proportion of grain-based feed ingredients and thus direct competition with human nutrition typically increases in the specialized livestock production systems. At the same time, their dependence on antibiotics and growth promoters is harmful for public health (antibiotic resistance, foodborne, and zoonotic diseases) (Peterson et al., 2020).
Globally, the livestock sector has a huge environmental footprint. It is responsible for emitting 14.5% of the total anthropogenic GHG emissions (Adegbeye et al., 2020), 33% of the total reactive nitrogen emissions (Mueller and Lassaletta, 2020), and is utilizing 30% of the total ice-free land area (Havlík et al., 2012). While large regional differences exist, many of the current livestock production systems in the tropics are responsible for undesirable environmental effects. Expansion of grazing land for livestock is a major driver for deforestation especially in Latin America, leading to about 57% of pasture land replacement with forests over the last decades (Graesser et al., 2015). Overgrazing in pasture and rangelands has resulted in severe soil degradation through compaction and erosion (Martinez and Zinck, 2004), especially in the drylands, with SOC losses creating a large carbon deficit in soils globally (Sanderman et al., 2017). In addition, livestock production is associated with biodiversity loss and high water use (Alkemade et al., 2013; Heinke et al., 2020) Among the most recognized and studied side effects of livestock production related to environmental damage in the tropical areas are: GHG emissions contributing to global warming, deforestation, biodiversity loss, high water use, and land/soil degradation (Martinez and Zinck, 2004; Alkemade et al., 2013; Chirinda et al., 2019; Boddey et al., 2020; Butterbach-Bahl et al., 2020). Widely publicized recent reports, such as EAT-Lancet (Willett et al., 2019), prompted a wave of media outreach arguing that one of the main solutions to the climate change and human health crises, globally, is to eat no or little animal-source foods (Paul et al., 2020a). Although we concur that the growing demand for livestock products presents a threat to environmental sustainability, we question the notion that stopping livestock production altogether is the most suitable or feasible option. Firstly, the political will is lacking and the necessary behavioral change of the majority of consumers is unlikely to occur (Winders and Ransom, 2019). Under these circumstances, it is important to have complimentary strategies that do not eliminate livestock but instead transform its production to reduce the environmental damages from the livestock sector. Secondly, livestock is not only of vital importance for low-income societies in socio-economical terms, but—when managed well—also plays various complex and often positive environmental and social benefits (Paul et al., 2020b). To reduce the consumption of animal source food could be a valid option for the Global North where diets show an excess in protein and energy consumption, but not for low and middle income countries where most people are under recommended nutrition standards. There, it is, thus, critical to identify sustainable management strategies. These strategies should be applicable to the local context, socially-acceptable, economically viable and avoid the environmental degradation that in the long-term undermines their existence.
Agroecology has been put forward as a solution to modern crises such as climate change and malnutrition, contrasting with the dominant industrial agricultural model based on the use of external inputs (Wezel et al., 2020), while improved forages have been proposed as an important entry point for the sustainable intensification of livestock production systems (Rao et al., 2015). This paper takes a closer look at and links up both these proposed solutions. It explores the benefits of including improved forages in integrated crop-livestock-tree systems and investigates the role of such forage-based systems in agroecological transformation. We thereby specifically focus on mixed cropping systems and cultivated forages in the tropics, i.e., crops that are specifically grown as animal feed, be it for grazing or cut-and-carry purposes; and exclude from our analyses the native and naturalized pastures and rangelands.
Based on a review of literature and expert opinion, we aim to demonstrate the importance of cultivated tropical forages, with their emerging environmental co-benefits, for ensuring sustainable livestock production based on agroecological principles. In section The Agroecological Framework, it starts by briefly introducing agroecology as (i) a science, (ii) a practice and (iii) a movement supporting the application of 13 principles—and their underlying values—to the design of farming and food systems. The next section, section Ensuring System Sustainability Through Integrating Improved Forages in Mixed Crop-Tree-Livestock Systems in the Tropics, summarizes how cultivated forages have been put into practice by farmers in the global south and how this provides benefits across different sustainability domains and barriers to further adoption at scale. Section Contributions of Improved Cultivated Forages to Agroecological Transformation proceeds by (i) outlining through which pathways and mechanisms this practice is in line with each of the agroecological principles and (ii) assessing to which extent applying these principles is covered in the scientific literature about forage-based livestock production systems in the tropics. Based on field experience and literature review, we summarize our understanding of the mechanisms and pathways through which the integration of forages in animal production systems can contribute or has shown to contribute to each of the 13 agroecological principles. Based on this understanding, search strings were developed for agroecology as a whole and separately for each principle. They were combined with a general search string capturing the integration of cultivated forages in smallholder mixed crop-tree-livestock systems in the tropics (see Supplementary Material). We report the number of hits in Web of Science as a metric for the availability of evidence of this contribution from the perspective of the scientific community. After reviewing the science at the forage-agroecology nexus, section Future Outlook finally identifies critical knowledge gaps and recommends the next steps for scaling up the contribution of cultivated tropical forages to the agroecological transformation of agri-food systems.
The Agroecological Framework
The principles of agroecology have evolved in history, from agriculture-centered to a holistic food system approach (Gliessman, 2018; Wezel et al., 2020). The most common definition of agroecology, “the application of ecological concepts and principles to the design and management of sustainable agroecosystems, or the science of sustainable agriculture,” has recently evolved into an integrated concept bringing the three dimensions of sustainability—ecological, economic, and social—to all parts of the food system. The approach is grounded in ecological thinking where a holistic, systems-level understanding of food system sustainability is required (Gliessman, 2018). An agroecological perspective on agri-food systems links the nutritional value of food and dietary choices to the environmental and social impacts of food production (Lamine and Dawson, 2018). Hilbeck et al. (2015) write that “agroecology is neither a defined system of production nor a production technique. It is a set of principles and practices intended to enhance the sustainability of a farming system, and it is a movement that seeks a new way of food production. Scholars thereby agree that the term incorporates three components (IFOAM EU, 2019). First, it is a scientific discipline, studying the ecology of agricultural systems. Second, it has evolved into a set of agricultural practices. Finally, it has turned into a movement that incorporates social justice, food sovereignty and the preservation of cultural identities (Méndez et al., 2013). As such, it operates at different levels and engages different stakeholders ranging from scientists to farmers and communities in the context of the sustainable agri-food systems.
As happens with multi-dimensional concepts, operationalization often ends up focusing on one or a few components and fails to maintain a holistic approach. While promoting unidimensional agroecological practices, often mainly technical, still contributes to an agroecological transformation, these approaches are less sustainable as they often lack the sociopolitical support needed e.g., to reverse the power balance with conventional agriculture (Le Coq et al., 2020). Practically, neglecting the multidimensionality of the agroecology concept results in confusion with other concepts like organic agriculture, conservation agriculture, nature-positive agriculture or the more recent regenerative agriculture. Organic and conservation agriculture are based on simple principles around soil fertility management at plot level, aiming at avoiding the use of agrochemical and protecting the soil through permanent soil cover. The two differ in their market orientation, with organic agriculture strongly driven by product certification. Regenerative agriculture proposes a more holistic approach, trying to reconcile agroecology and sustainable intensification under the same banner, but seems to generate just more confusion (Giller et al., 2021). Nature-positive solutions, in turn, are less specific and englobe anything where nature works to address societal challenges, in agriculture or other sectors (Seddon et al., 2021), which includes the agroecology concept. The difference would be that nature-positive agriculture focusses on practices, whereas agroecology focusses on processes. But a common feature between all these different concepts is their meager integration of the livestock component. Until 2015, only 5% of indexed studies concerning agroecology dealt with livestock (Soussana et al., 2015).
As the concept gains prominence as a way to sustainably transform agriculture and food systems, particularly in a post-COVID world (Altieri and Nicholls, 2020), attempts to recognize all its dimensions and make it operational have culminated recently with the development of a clear framework and evaluation tool (FAO, 2018, 2021; Mottet et al., 2020). The framework is composed of ten interlinked and interdependent elements: (i) diversity, (ii) synergies, (iii) efficiency, (iv) resilience, (v) recycling, (vi) co-creation and sharing of knowledge, (vi) human and social values, (vii) culture and food traditions, (viii) responsible governance, (ix) circular, and (x) solidarity economy. The first five describe common characteristics of agroecological systems, the sixth and seventh describe foundational practices and innovation approaches, and the last three describe context features and enabling environment (FAO, 2018, 2021). These 10 elements imply a series of requirements for farming system management that can be articulated in 13 principles: recycling, input reduction, soil health, animal health, biodiversity, synergy, economic diversification, co-creation of knowledge, social values and diets, fairness, connectivity, land and natural resource governance, and participation (Wezel et al., 2020). A farming system that scores high in these principles can be seen as transitioning toward a sustainable food system via agroecological transformation. Figure 1 presents a schematic overview of the different agroecological principles at play in a mixed crop-tree-livestock farm.
In section Contributions of Improved Cultivated Forages to Agroecological Transformation, we assess the role of improved tropical forages as a potential catalyst for enabling livestock systems to contribute to the 13 principles and support an agroecological transformation. As a background, the next section defines improved forages, summarizes documented uptake, the multi-dimensional impacts of this uptake and barriers to more wide-spread uptake.
Ensuring System Sustainability Through Integrating Improved Forages in Mixed Crop-Tree-Livestock Systems in the Tropics
Livestock production in the global South takes place in a variety of livestock production systems. The grassland-based systems, in which crop-based agriculture is minimal, cover the largest areas (Robinson et al., 2011), while most production (i.e., meat, milk, eggs) occurs in mixed crop-livestock systems (Herrero et al., 2010). Cultivated forages include a wide variety of sown or planted grasses, herbaceous legumes, trees and shrubs (mostly legumes) that are integrated in a variety of mixed systems, including intensive or extensive mixed agricultural systems with grazing or cut-and-carry systems, agro-pastoral and silvo-pastoral systems (Rao et al., 2015). In Latin America and the Caribbean, permanent pastures are the most common use of forages, while in Sub-Saharan Africa and Southeast Asia cut-and-carry systems prevail.
There exists a large diversity of forages allowing adaptation to various production contexts. The so-called genetic improvement of tropical forages is relatively recent and was for several decades relying heavily on the agronomic selection of wild relatives. The agronomic/genetic evaluation of forages has been focused not only on productivity and feed quality but also on tolerance to biotic (insects, diseases) and abiotic (low soil fertility, aluminum toxicity, drought, waterlogging) stress factors. Through this selection from the wild it was possible to identify superior germplasm which resulted in substantial and sustainable productivity gains (per head and per unit area) as well as enhanced resilience (e.g., Peters et al., 2013; Rao et al., 2015; Schultze-Kraft et al., 2018). Recently the importance of bred forages has increased (Jank et al., 2014) and this has allowed attention to specific constraints, where diversity in the natural populations reached limitations in identifying productive, nutritive and stress-tolerant materials. For example, in well-drained environments in Latin America and the Caribbean with a wide distribution of Urochloa (previously known as Brachiaria; Cook et al., 2020) decumbens, resistance to a major insect, spittlebug, became an issue to be addressed by the breeding efforts, while for waterlogged environments there remains a scarcity of high-quality forages (Argel et al., 2007). Bred forages with a combination of desirable traits (e.g., productivity, quality and resistance to biotic and abiotic factors) are also attractive to seed suppliers for targeting specific agro-ecological niches, allowing a greater market differentiation providing incentives for development of the forage seed sector. For example, in the case of crop-livestock systems in Latin America and the Caribbean (LAC), we see expanding demand for forages requiring soil fertility management and greater attention to environmental concerns. There is also an increasing demand for shade-tolerant forages for silvopastoral systems with high resilience to vulnerable climates with extreme and unpredictable weather conditions. Throughout the rest of this paper we will use the term “improved forages” when we refer to forages that have gone through a process of agronomic selection from wild relatives or breeding and selection leading to genetic gain in desirable traits.
At first sight, such improved forages seem similar to the high yielding crops such as wheat and rice, widely promoted by the international agricultural research centers in the 1960s and 1970s and adopted as part of the Green Revolution (Byerlee and Lynam, 2020). We do, however, not expect the well-documented drawbacks, such as high input prices, environmental pollution and increased inequality, of the green revolution to re-occur with improved forages. First, the technology in itself differs significantly, with the improved forages not requiring intensive application of pesticides, herbicides and synthetic fertilizers. On the contrary, many have been selected or are specifically bred for their capacity to perform well in marginal areas facing climate variability and change, low fertility or acid soils, water logging, and for pest and disease resistance. In addition, they are being promoted as a component of mixed cropping systems to improve the overall system performance and efficiency in using local resources. Finally, a wide variety of forage species and varieties, including indigenous trees and so-called neglected or orphan crops, are considered for system improvement.
Decades of efforts to promote cultivated forages for their productivity and environmental benefits have contributed to widespread adoption, particularly grasses in LAC (White et al., 2013; Baptistella et al., 2020, REDE ILPF ref). It is worthwhile to have a closer look at some successful scaling examples. Maass et al. (2015) estimated that the adoption of hybrid Urochloa cultivars in East Africa was about 1,000 hectares (20,000 households). Labarta et al. (2017) and ISPC (2018) reported that adoption of improved Urochloa cultivars in Colombia, Peru, Nicaragua, Costa Rica and Honduras occurred on approximately 7.9 million hectares. According to White et al. (2013), Stylosanthes varieties (from the CGIAR genebank) have been adopted on at least 200,000 hectares. Valentim and Andrade (2005) estimated the early adoption of Arachis pintoi for the Amazon region of Brazil to have reached 1,000 cattle producers and to have generated a gross profit of US$ 4,000 per year per producer. Wunscher et al. (2004) and Lascano et al. (2005) reported a successful early adoption of Arachis pintoi in Colombia and Costa Rica.
The benefits of integrating improved forages in livestock production systems have previously been described as part of the LivestockPlus concept (Rao et al., 2015). The authors describe how the sustainable intensification of forage-based systems, combining genetic, ecological and socio-economic intensification processes, increases the efficiency of the systems, has the potential to improve livelihoods, and yields a range of environmental co-benefits—including improved soil health, reduced erosion, reduced GHG emissions and improved GHG balances (emissions vs. carbon accumulation/life cycle), and improved adaptation to climate variability and change. Figure 2 illustrates how forages can be integrated in mixed crop-tree-livestock systems and summarizes how this positively impacts on livelihoods and the environment.
The relatively wide adoption of improved tropical forages in LAC has convincingly demonstrated their capacity to increase productivity while reducing livestock-related GHG emissions per unit product. On one side, their ability to increase soil carbon sequestration has been demonstrated (Fisher et al., 1994) while the ability of certain grasses (e.g., Urochloa and Megathyrsus) to modulate the rhizosphere interactions through biological nitrification inhibition has proven to reduce soil-borne N2O emissions up to 60% (compared to similar genotypes without this ability) either after fertilization or urine deposition (Subbarao et al., 2009; Byrnes et al., 2017). Another strategy is the improvement of cattle diets through supplementation with forage legumes, which has the potential to reduce up to 67% cattle enteric CH4 emissions based on a legume (i.e., Leucaena) inclusion proportion of 36% when compared to a grass alone diet (Gaviria-Uribe et al., 2020; Montoya-Flores et al., 2020).
In addition to these environmental co-benefits there is a huge body of evidence about their economic benefits. Zooming into forage grasses, the implementation of improved forage-based cattle production systems in Latin America, for example, increases the Internal Rate of Return (IRR)1 by 10–100% compared to traditional grazing systems (Seré and Estrada, 1982; Seré et al., 1993). The implementation of improved Urochloa brizantha cultivars in Colombian beef cattle systems is expected to reduce the producer's risk of obtaining economic losses and lead to economic benefits of US$ 11.3 million at the national level (2022–2048) from which 62.5% would fall on the producer and 37.5% on the consumer. Supplementation by 35% with the forage oats (Avena sativa AV25T cv. Altoandina) in a Kikuyu grass dairy system increases the net present value (NPV)2 by >100% when compared with a Kikuyu monoculture and leads to an IRR of 49.9% (Rivas and Holmann, 2000). The implementation of spittlebug-resistant Urochloa hybrids was estimated to have potential benefits equivalent to 43% of Colombia's beef and dairy production volume of 2003 (Rivas and Holmann, 2004a,b). The implementation of different planted forages in West Africa during the period from 1977 to 1997 was estimated to result in an social internal rate of return3 on investments of 38% over 20 years (Elbasha et al., 1999).
Examples also abound around the dual economic-environmental benefits associated with forage legumes. The introduction of forage legumes in the crop-livestock systems of Nicaragua has proven benefits to tackling degradation and restoring land and soil health. When introduced into the smallholder traditional crop-livestock production system of the Nicaraguan hillsides, Canavalia brasiliensis derived on average 69% of its N from the atmosphere by symbiotic N2-fixation, and increased the soil N balance when used as green manure (Douxchamps et al., 2010). In this case, 12% of the N from Canavalia was recovered in the subsequent maize crop (Douxchamps et al., 2011). However, when used as forage to increase milk yields and annual net income, Canavalia bears the risk of triggering soil N depletion, unless animal manure is recycled. Therefore, biophysical and socioeconomic trade-offs must be carefully balanced at the farm level to maximize nutrient use efficiency and ensure a sustainable farming system intensification (Douxchamps et al., 2014). Pastures on highly weathered soil in forest margins in Caquetá, Colombia increased dry matter and N/protein yield in farmers pastures containing legumes; because of additional N input via symbiotic N2 fixation; greater P uptake in productive grass-legume than grass-alone pastures in spite of low plant available P in soils, which likely resulted in greater P recycling (Villegas et al., 2020). Furthermore, the inclusion of the legume Arachis pintoi in grass-legume associations in the same study area doubles beef and milk production and leads to an IRR of between 19.3 and 21.1%, which is significantly higher than for a traditional production system (Rivas and Holmann, 2000). For Costa Rica, grass-legume associations with Arachis pintoi and Cratylia argentea (Rivas and Holmann, 2000) lead to an estimated 30% reduction in production costs per kilogram of milk (Peters et al., 2001). Profitability evaluations in Costa Rica, Michoacán (Mexico) and the Colombian Caribbean region report an IRR that oscillates around 33% for a Leucaena leucocephala-grass association (Jimenez-Trujillo et al., 2011; González, 2013; Murgueitio et al., 2015). The inclusion of Leucaena diversifolia in a Urochloa brizantha cv. Cayman hybrid production system in Colombia is financially profitable and improves all risk and performance indicators when compared with Cayman as a monoculture. This legume increases the Net Present Value (NPV) and the IRR and decreases the minimum area required for generating two basic salaries, the payback period and the risk of obtaining economic loss (Enciso et al., 2020). Also in south-east Asia, forage legumes have proven to play multiple roles, supporting at farm level an increase of N recycling intensity, of N balances and of land productivity. However, the magnitude of the effects there depends strongly on the type of farming system, with more important effects where potential for improvement was high (Epper et al., 2019). While in Queensland, Australia, Leucaena leucocephala has been identified as the most productive and profitable legume, doubling the gross margin (expressed per unit of area), when compared with perennial grasses. At the regional level, economic benefits from the adoption of L. leucocephala have been estimated to be more than US$ 69 million/yr for 2006 in a planted area of 150,000 ha (Shelton and Dalzell, 2007; Bowen et al., 2016).
Also tree-based forage species have been demonstrated to have multiple benefits. Pilot sites in Mali, Burkina Faso and Niger, for example, show that more successful restoration outcomes are achieved when combining slow-growing indigenous trees or shrubs with fast growing native fodder species for livestock (Sacande and Berrahmouni, 2016). Fodder species have been used to incentivise restoration for example in Burkina Faso (Vinceti, 2020) leading to more resilient restoration outcomes and great adoption of restoration by farmers. Dry forest species can provide critical reserves during extreme drought offering important food and fodder for communities (Valette, 2019). Early effects of silvopastoral systems with improved forages also show improved soil health and increased abundance and diversity of soil macrofauna as documented by e.g., Barros et al. (2003); Lira et al. (2020), and Vazquez et al. (2020). Mixed systems with a strong tree component are thus gaining prominence because of their true multiple environmental wins: increased soil quality, GHG emission mitigation, higher biodiversity and improved water use efficiency.
As a final example, cactus pear (Opuntia ficus-indica) is gaining increasing interest across the globe because of its unique features that could help alleviate hunger in arid regions thanks to its ability to survive in harsh conditions. This spineless species is not invasive and is used as livestock feed that can improve meat and milk production for cash earnings, while helping to reduce groundwater use through its high-water use efficiency (species with CAM photosynthetic pathway). Furthermore, its evergreen cladodes can provide “at any time of the year” high palatable green fodder with a high Ca to P ratio. Despite its low crude protein and fiber content, the cactus pear cladodes are high in water, sugars, ash and vitamins A and C representing a digestible energy-rich feed when incorporated into livestock diets (Rocha Filho et al., 2021). Because of their high-water content, cactus pears also reduce the need for livestock watering. In fact, cactus pear is a very versatile, resilient crop. It is very easy to establish and able to grow on lands where no other crops can grow. Cactus pear is a multi-functional plant that can be utilized to restore degraded land, control soil and water erosion, regulate climate through carbon sequestration, and its fruits and cladodes are consumed by humans (Inglese et al., 2018; Hassan et al., 2019).
Even though the research on gender and social benefits has started later, good evidence on positive impacts in that dimension of sustainability is also emerging. A case study from Kenya shows that the adoption of improved planted forages in dairy systems leads to additional roles of women in feed and dairy production and thus more control over the derived incomes from the production system, but also to higher labor burdens, which might affect technology adoption (Lukuyu et al., 2021). Ba et al. (2013) report an average of 50% reduction in amount of labor and time spent by smallholder farmers in supplying forages to their animals in south Central Vietnam. The adoption of Urochloa hybrids and other improved forages in Ugandan pig production systems has led to time savings among male and female farmers (reduced time for collecting feed) and thus made it possible for the producers to engage in other economic activities (e.g., farming, small-scale enterprises). It also changed the decision-making structures in the households and empowered women to join their husbands in the decision on which forage to adopt and how to grow and manage it (Lukuyu et al., 2020). In Ethiopia and Kenya, women and youth are increasingly starting to engage in forage businesses, from which they retain income, and which is a promising pathway for women's economic empowerment (Njuguna-Mungai et al., under review).
Despite the growing evidence on the multiple benefits of integrating cultivated forages in mixed crop-tree-livestock systems and some successful scaling examples, overall the adoption rates of improved forages remain relatively low, especially outside Brazil and Latin America. Many of the determining factors for the adoption of forage technologies have been studied and include risk factors (perception of risk about future returns from implementing the technology, risk aversion of the producer) (e.g., Marra et al., 2003; van Winsen et al., 2014; Trujillo-Barrera et al., 2016), the availability of commercial seeds, forage establishment costs, the availability of technical information on the establishment and management, the promotion and availability of knowledge about potential benefits and risks (CIAT, 2004; Wunscher et al., 2004; Lascano et al., 2005), labor requirements (Kaimowitz and Angelsen, 2008), farm size and farm management, the proximity to input markets (ISPC, 2018), the growth of output markets (Kaimowitz and Angelsen, 2008), as well as the general access to productive inputs (e.g., fertilizer, manure, pesticides), capital (e.g., credits, payments for ecosystem services, product differentiation) (e.g., Charry et al., 2019), and extension/technical assistance (Ruiz et al., 2016; Bravo et al., 2018; Enciso et al., 2018; Charry et al., 2019), social capital, and membership of farmer groups (Oulu, 2020). Likewise, structural conditions can influence the adoption of improved forages, such as the prevailing extensive nature of the cattle production systems, low land prices (which can lead to an expansion of area instead of intensification) (White et al., 2001), land tenure rights (Kaimowitz and Angelsen, 2008), land speculation (Smith et al., 1997), political violence and warfare (ISPC, 2018), and missing regulatory and monitoring frameworks. When it comes to promoting the adoption of forage technologies, it is also important to analyze and understand how livestock producers make their decisions and how their decision-making process is influenced by factors such as trust (in the information provided or in its sources), social networks and socio-cultural contexts (e.g., Jones et al., 2013; Martínez-García et al., 2013; Rossi Borges and Oude Lansink, 2016; Ambrosius et al., 2019; Hidano et al., 2019).
Contributions of Improved Cultivated Forages to Agroecological Transformation
As partly demonstrated in the previous section, integrating improved forages in mixed crop-tree-livestock systems is associated with a wide variety of practice changes. These changes include agronomic and animal husbandry practice change, awareness creation, capacity building, and multi-stakeholder engagement approaches to actions associated with the broader food systems, such as waste reductions and dietary shifts. As amply described in the scientific literature (see Table 1), they thereby align well to all 13 agroecological principles.
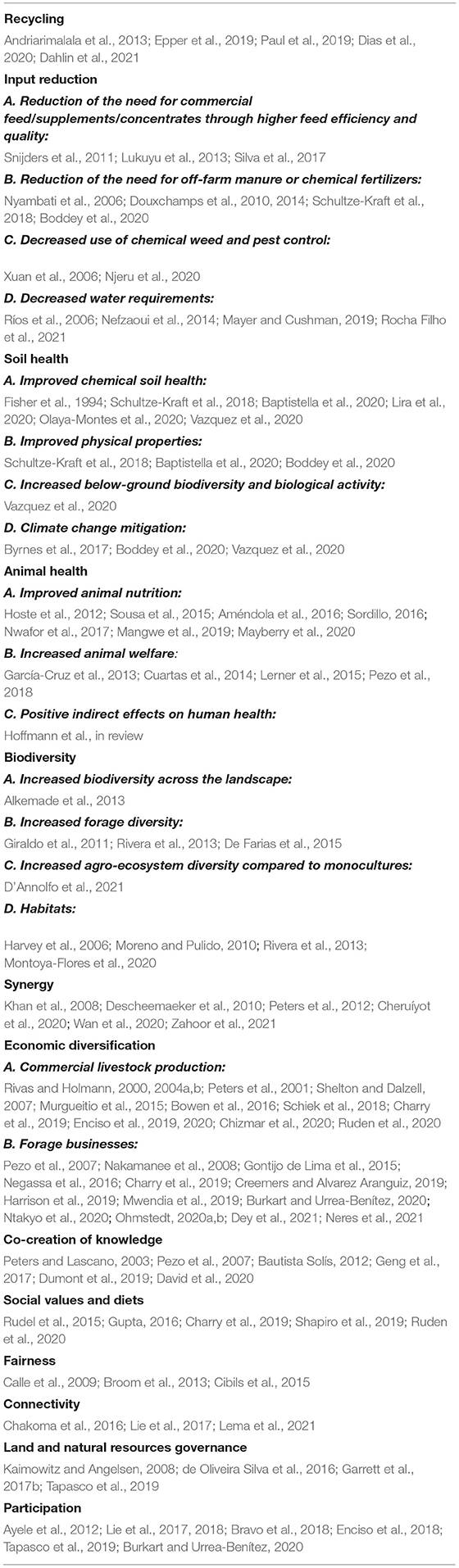
Table 1. Key references describing the contribution of tropical forages in mixed crop-tree-livestock (MCTL) systems to the 13 agroecological principles described by Wezel et al. (2020).
The first principle, recycling, prescribes to use local renewable resources as much as possible and close as far as possible resource cycles of nutrients and biomass. Forages take up nutrients available in the system, including from deep soil layers, and make these available to livestock. This results in improved nutrient use efficiency. More options to close nutrient cycles through animal manure also exist. In terms of input reduction, the second principle, forages are associated with a reduced need for external inputs, such as feeds, agro-chemicals and water. First, they are associated with a reduction of the need for commercial feed/supplements/concentrates through higher feed efficiency and quality. Well-managed high-quality forages can eliminate or minimize the need for concentrates by moderate producing animals, because intensive utilization of forages (cutting or grazing at the right moment of the phenology) increases the production of metabolizable energy and protein per unit of area. Second, they often are associated with a reduction of the need for off-farm manure or chemical fertilizers. This is facilitated through symbiotic N2 fixation by forage legumes and the use of forages (partly/fully) as green manure. In addition, there is higher availability of on-farm animal manure because of increased livestock productivity (through higher stocking rates and better-fed animals) and increased availability of crop residues for soil amendments as they can be replaced by forages in the feed basket. Third, the use of forages as a cover crop reduces the need for weeding and chemical weed control, while the use of forages with genetic tolerance against certain pests and diseases or the use of forages in the push-pull system replaces chemical pest control measures (e.g., against stemborer and striga). Fourth, forages are associated with decreased water requirements. Increased soil water retention and infiltration is observed as a result of forages used as a cover crop or green manure to improve soil structure and limit run-off and in the case of improved forages established in areas previously covered by degraded pastures. Drought-tolerant and water-saver forages reduce dependence on water for irrigation compared to currently used forages grown in similar conditions.
Integrating cultivated forages in the systems enhances different dimensions of soil health, the third principle. The chemical soil health is improved through root exudation or forages used as green manure, through the stimulation of nutrient cycling, soil organic matter (SOM) accumulation, increased soil carbon stocks and sequestration. The physical soil properties are improved as a result of increased soil aggregation, improved soil structure and aeration, increases in particulate organic matter in soil, roots remaining in soil after harvest/grazing, forages as green manure or cover crop, or the use of forages to prevent soil erosion. Below-ground biodiversity and biological activity is increased through increased soil microbial diversity and activity, presence of rhizobia. Diverse pastures (mix of various species) of diverse functions (secondary compounds, root system) improve the conditions for biological activity at deeper horizons, while increased use of tree-based forages can improve soil quality through improved mycorrhizal networks. The integration of forages, with their capacity to sequester and store carbon in the soil and to inhibit biological nitrification, finally, can significantly enhance the climate change mitigation function of the soil.
Different mechanisms are at play for improving animal health and welfare, the fourth agroecological principle. High-quality forages (incl. legumes) in the systems improve the overall quality and quantity feeding and thus animal health, amongst others through enhanced immunity and resistance to pathogens. The conservation of forages (e.g., hay, silage, pellets) thereby increases the availability of feed during seasons where scarcity of feed leaves the animals most vulnerable to disease. Forages from diverse pastures (a mix of various species) complement each other in their contents of critical nutrients for the animal and secondary compounds. Some can, for example, be more efficient in utilizing P or pumping Cu or Mg, providing balanced nutrients and secondary compounds (antibloat, antiparasite agents), while recent results indicate that bioactive tanniniferous plants represent a valuable option as an alternative to commercial drugs for the control of gastrointestinal nematodes. Animal welfare is increased in silvopastoral systems. The trees/shade create more favorable microclimatic conditions and reduce heat stress, which has in turn been associated with more stable social/hierarchical behavior. In addition to animal health and welfare, also positive indirect effects on human health have been documented. Improved plant health, including those of forages, under minimal use of agrochemicals improves animal and human health through reduced exposure to chemical residues. Well-fed animals require less antibiotics thus reducing the need for antibiotics and risk of antimicrobial resistance. Well-fed and healthy animals cause a lower pathogen load in manure that can be transmitted through the food chain and feeding healthy forages can reduce feeding of feeds with high aflatoxins such as maize in East Africa.
The fifth agroecological principle, biodiversity, can be enhanced by increasing biodiversity across the landscape. Enhancing land productivity, through high-yielding forages, can spare land for biodiversity conservation and prevent the need for further land conversion to agriculture. The introduction of alternative forage species increases the diversity of species and genetic resources at farm and landscape level as compared to grass monocultures or degraded/intensively-managed pastures. This can include the use (and in-situ conservation) of local/neglected species. The broader variety of forage species in combination with reduced use of chemical weed/pest control is likely to attract/maintain wider diversity of e.g., pollinators and below-ground fauna. such well-managed pastures increase the natural introduction of native plant species with desired feeding value and resilience to extreme environmental conditions. In silvopastoral systems, the presence of shrubs and trees has been demonstrated to have a positive impact on biodiversity by creating complex habitats for wild animals and plants and harboring a richer soil biota as compared to conventional grazing systems. Cultivated forages enhance positive ecological interactions and complementarities among system components at the interface between the system's soil, plant, and animal components and thus align well with the sixth agroecological principle of synergy. Using for example tree-based forages can help to increase on-farm above and below ground carbon storage, leading to additional climate mitigation benefits.
Different mechanisms contribute to economic diversification, the seventh principle. In first instance, forages enable further commercialization of livestock production. Feed represents the highest cost of production in any livestock system and cultivated forages can substantially reduce the feed input costs. In combination with enhanced productivity, this results in increased rates of return and opens opportunities for income diversification with cattle fattening or commercial milk production. Also the forages in themselves allow for income-diversification. Income-generating opportunities along the forage value chain include forage seed supply, marketing and distribution, the sale of hay, silage, pellets and timber or fruits in the case of forage trees.
Approaches that encourage co-creation of knowledge and horizontal learning used in research and development efforts around cultivated and improved forages include: on-farm variety trials and participatory monitoring and evaluation, capacity building and knowledge exchange activities such as field days and farmer exchanges. These approaches promote farmer-to-farmer contacts as well as more equal relationships between farmers and researchers. This encourages sharing knowledge and skills and triggers innovation in combination with encouraging community-level seed production and “passing on the gift,” the existing technology (and associated management practices) scale out quickly.
In terms of social values and diets, principle number nine, animal sourced foods (ASF) are an important source of proteins and readily available micro-nutrients, especially important for improving the nutritional status of especially young children and pregnant and lactating women. Integrating cultivated forages in livestock production systems can increase both the quantity and quality of ASF production. The forages also enable the production of sustainably produced ASF, with simultaneous social, economic and environmental benefits.
Efforts to ensure the affordability of quality and environmentally-friendly animal products and the creation of opportunities for smallholders, including for women and youth align well to principle ten, fairness. Forages support dignified and robust livelihoods along the livestock value chains. In line with connectivity, the eleventh principle, local feed, seed, and ASF production allow re-embedding food systems into local economies. Actors along the forages and ASF value chains have more proximity and confidence and are better connected to markets. Principle twelve, land and natural resources governance, prescribes to strengthen institutional arrangements to improve, including the recognition and support of family farmers, smallholders and peasant food producers as sustainable managers of natural and genetic resources. Forages create a need for land-use planning and offer opportunities for development of new resource management strategies, for instance to mitigate soil degradation (e.g., fanya juu terraces). Participatory land-use planning processes can ensure the optimal use of land areas that would not be suitable for crops, use in rotation/intercropping/life barriers/under trees and at times promote land use options for carbon-neutral agriculture. In line with the last principle, participation, the Forage community has started to apply a wide array of participatory approaches. Through participatory system dynamics modeling and participation in multi-stakeholder innovation platforms or round-table discussions, farmers can be included in the design of livestock and forage sector strategies. These approaches promote equal relationships and balanced powers between farmers, researchers and policy makers.
Between 2005 and 2021, a total of 1,183 peer-reviewed publications addressed the use of cultivated forages in smallholder systems. The most studied principles concerning the forages are economic diversification, social values and diets, biodiversity, and recycling, all illustrated by more than 200 peer-reviewed publications, mostly at farm scale. Animal health renders 126 hits, then the other principles with less than a hundred. Connectivity was the least represented, with only five hits. These results show that the most evident agroecological impact of forages, according to the scientific community, can be observed in terms of market opportunities and income diversification. The high number of hits for social value and diets illustrates how high the topic of animal-source food and vegetarianism is currently on the global agenda. The principle of biodiversity includes particularly papers reporting options to include forages in rotation or intercropping with different types of systems and pastures' diversity. Finally, the capacity of forages to provide options to close nutrient cycles at the farm level was well-documented. The scientific community's interest in these topics has evolved: social values and diets are high on the agenda since 2012, recycling emerged a bit later in 2015, while economic diversification and biodiversity display a sawtooth but generally increasing interest (Figure 2). Besides connectivity and participation, which are both only sporadically addressed, the documentation of the other principles increased during the period 2005–2020, with some promising peaks for animal health and synergy. More research is needed at the food system scale to fully understand the role of forages in agroecology, particularly on sociological and process aspects, which are both at the core of the four principles less documented. This also indicates a yet to be filled opportunity for forage experts to engage more with the agroecological movement and make forages part of sustainable agri-food system transformation. The finding that despite the existence of scientific literature about cultivated forages and each of the agroecological principles, only 38 out of the 1,183 publications in our WoS search explicitly mention agroecology corroborates this action gap.
Future Outlook
As illustrated in sections Ensuring System Sustainability Through Integrating Improved Forages in Mixed Crop-Tree-Livestock Systems in the Tropics and Contributions of Improved Cultivated Forages to Agroecological Transformation, there is increased research interest and understanding of the economic, social and agroecological dynamics related to improved forages and their integration in mixed crop-tree-livestock systems. However, several knowledge and technology gaps still exist. At the actual technology level, it is important to continue the genetic improvement and identify or develop forage varieties tolerant to a wide range of biotic and abiotic stress factors. Supported by state-of-art genomics and phenomics, this can be done more efficiently and rapidly than before (Chang et al., 2019). Ensuring genetic diversity at forage level provides an insurance with respect to the impact of biotic and abiotic stress factors on yield and quality (Finckh, 2008). Livestock production, however, does not only take place in heterogeneous agro-climatic conditions, but also in a wide diversity in farm systems, and socioeconomic or policy contexts (Umunezero et al., 2016). To guide the choice of forage species and their integration into farming systems more systems agronomy is needed to produce robust socio-ecological niches for various systems that can be scaled (Paul et al., 2020c). This must be combined with increased research investments in the forages-soil health nexus which seem to have remained stable but low, with <100 WoS hits in total (Figure 3).
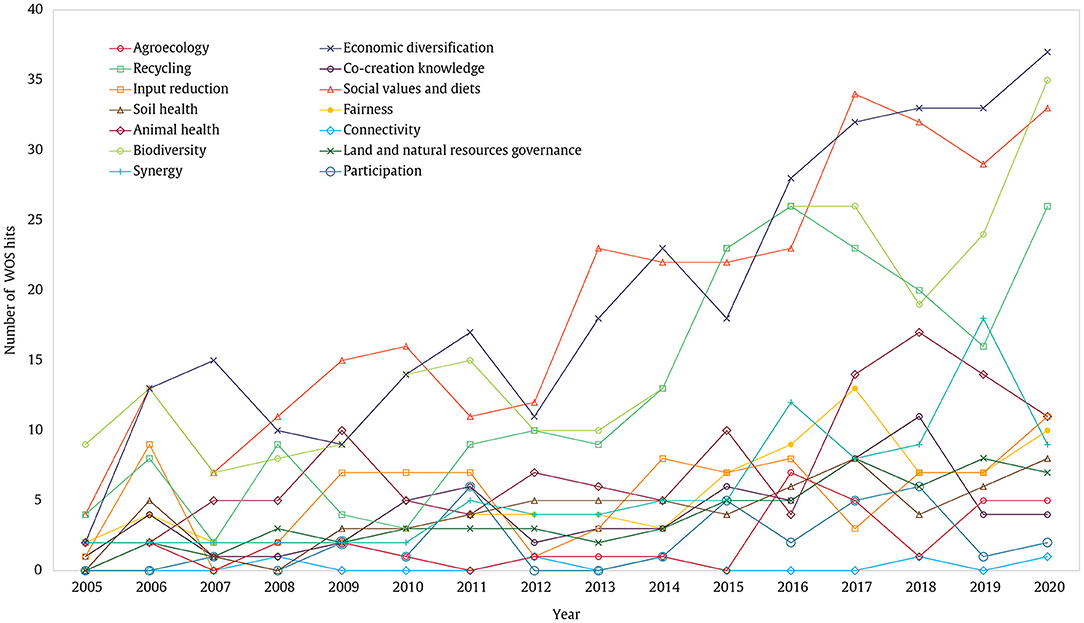
Figure 3. Evolution of the interest of the scientific community for the different nexi between forages and principles.
Further research is also required to strengthen our understanding of the multiple interacting impacts of improved forages at the food system level. An increased understanding of particularly the social dimension has a lot to offer, also in terms of understanding the drivers, underlying causes and impacts of changes linked to the productivity, economic, environmental and human dimensions (Rietveld et al., 2021), while our WoS search results show a low coverage of these issues in the scientific literature. Based on empirical data, foresight analyses and farming systems modeling can be used to estimate multidimensional impacts of forages and for reducing agro-environmental trade-offs (Groot et al., 2012; Paul et al., 2020c). In addition to developing context-specific data on the potential trade-offs associated with integrating forages in mixed crop-tree-livestock systems, a better understanding of what drives uptake of improved forages, especially within agroecological initiatives, is needed for guiding large-scale investments and supporting the decision-making processes around that.
At a more immediate action level, to ensure agroecological-based farming sustainability, there is a need for demand for the resultant products driven by sufficient public attention. To achieve the level of attention that results in changes in policy and consumer demand, there is a need for influential communication targeting policymakers and the different publics. Raising awareness at different decision-making levels should aim to differentiate, label and promote livestock products derived from agroecosystems based on agroecological principles. Concurrently, cultivated forages should be promoted as a versatile and multi-purpose crop through public campaigns (social media, workshops, leaflets, lobbying) (Louhaichi et al., 2018). However, from the literature search (Figure 2) these aspects seem to be understudied which would imply limited innovation in awareness raising. Yet, by highlighting the evidence-based benefits of integrating cultivated forages in agroecosystems, we can increase the visibility of crop-livestock systems and inform the flow of scaling-up investments. In addition, promotional and educational activities, along with results from further research involving farmer participation, in combination economic incentives, such as payments for ecosystem services and the development of inclusive business models, should be further explored (Schultze-Kraft et al., 2018).
Conclusion
The environmental and social consequences of the prevailing agri-food system have sparked a lively societal discussion on how to feed an increasing population in a socio-ecologically sustainable and equitable way. In response, agroecology has been presented as a practice, scientific discipline, and socio-political movement that applies ecological concepts in the sustainable management of agricultural systems. Although some literature highlights the important role livestock play in sustainable food systems and specifically agroecology, the prevailing narrative, especially so in the popular media, argues that one of the leading solutions to climate change and human health crises is to eat no or little animal-source foods.
In this paper, however, we point out that the narrow climate/diet framing misses the valuable role livestock can play, especially for family farmers in the south. Integrated systems present an opportunity to improve livestock production, support livelihoods, enhance and protect biodiversity, close nutrient loops etc. and forages play a key role in catalyzing this transformation. Scientific literature and documented practice change by farmers indicate that integrating cultivated forages in mixed crop-tree-livestock systems follows a wide range of agroecological principles and increases the sustainability of livestock production across the globe. We, therefore, have reason to believe that livestock production in the tropics based on improved forages can boost the sustainability indicators of this system, moving toward an agroecological transformation of the food system. It is, however, clear that a lot of this promise is yet to materialize and calls for an urgent coming together of the agroecological and livestock research and development communities. The specific role of the scientific community is therein to generate and use nuanced evidence on what is possible and what is not (taking multi-scale trade-offs into account). As part of the overall movement, they can help ensuring that forages gain more prominence in agroecological initiatives and that more investments are made in sustainable agri-food system transformation with explicit livestock and forage components.
Author Contributions
AN, SD, JA, and BP: conceptualized the study and did a general literature review. DV: conducted the quantitative literature search in Web of Science. AN: led the writing of all drafts and revisions. MPe: contributed to forage breeding and agronomy and provided overall scientific oversight. CK: tree-based systems. MPu: study design. TR and MW: context and framing. EV, NT, and AO: soil health. SN: soil organic carbon. CP-P: adoption factors. NC: GHGe. SB: economics and adoption. All authors gaps and conclusions.
Funding
This work was undertaken as part of the CGIAR Research Program on Livestock, which is carried out with support from the CGIAR Fund to Donors and bilateral funding agreements (https://www.cgiar.org/funders).
Conflict of Interest
The authors declare that the research was conducted in the absence of any commercial or financial relationships that could be construed as a potential conflict of interest.
The handling editor is currently editing co-organizing a Research Topic with several of the authors AN, SB, NC, MPe and confirms the absence of any other collaboration.
Publisher's Note
All claims expressed in this article are solely those of the authors and do not necessarily represent those of their affiliated organizations, or those of the publisher, the editors and the reviewers. Any product that may be evaluated in this article, or claim that may be made by its manufacturer, is not guaranteed or endorsed by the publisher.
Acknowledgments
We wish to extend a special thank to Jose Luis Urrea Benitez for designing Figure 1 and contributing to Figure 2. For Figure 2, we further want to acknowledge the skillful design by Tara Todras-Whitehill and Mireille Ferrari and beautiful illustrations by Sonja Niederhumer.
Supplementary Material
The Supplementary Material for this article can be found online at: https://www.frontiersin.org/articles/10.3389/fsufs.2021.742842/full#supplementary-material
Footnotes
1. ^The IRR is a financial indicator for estimating the profitability of potential investment projects. Although the IRR calculations are based on the same formula used for estimating the Net Present Value (NPV) of an investment project, it does not estimate the actual dollar value of the project but the expected annual return. Those potential investments with the highest IRR are generally the ones most desirable.
2. ^The NPV is an economic indicator that describes the difference between the present values of cash in- and outflows over a defined period of time and is used in investment planning for analyzing the profitability of a potential investment. The NPV considers the time value of money, is used to compare different investment alternatives, and relies on a discount rate related to the cost of required capital for making the investment. Investment options with a negative NPV are most likely not profitable and should be neglected.
3. ^The social IRR is a financial indicator that refers to the costs and benefits to society of a potential investment. It considers the opportunity costs of people not participating and the full cost of a potential investment for society, which makes it different from the general IRR indicator which only considers costs at the individual level. Apart from potential productivity increases derived from a potential investment, the social IRR also considers a broad range of possible non-economic benefits, such as better nutrition or a higher availability of end products.
References
Adegbeye, M. J., Ravi Kanth Reddy, P., Obaisi, A. I., Elghandour, M. M. M. Y., Oyebamiji, K. J., Salem, A. Z. M., et al. (2020). Sustainable agriculture options for production, Greenhouse gasses and pollution alleviation, and nutrient recycling in emerging and transitional nations - An overview. J. Clean. Product. 242:118319. doi: 10.1016/j.jclepro.2019.118319
Alkemade, R., Reid, R. S., van den Berg, M., de Leeuw, J., and Jeuken, M. (2013). Assessing the impacts of livestock production on biodiversity in rangeland ecosystems. Proc. Natl. Acad. Sci. U.S.A. 110, 20900–20905. doi: 10.1073/pnas.1011013108
Altieri, M. A., and Nicholls, C. I. (2020). Agroecology and the emergence of a post COVID-19 agriculture. Agric. Hum. Values 37, 525–526. doi: 10.1007/s10460-020-10043-7
Ambrosius, F. H. W., Hofstede, G. J., Bokkers, E. A. M., Bock, B. B., and Beulens, A. J. M. (2019). The social influence of investment decisions: a game about the Dutch pork sector. Livestock Sci. 220, 111–122. doi: 10.1016/j.livsci.2018.12.018
Améndola, L., Solorio, F. J., Ku-Vera, J. C., Améndola-Massiotti, R. D., Zarza, H., and Galindo, F. (2016). Social behaviour of cattle in tropical silvopastoral and monoculture systems. Animal 10, 863–867. doi: 10.1017/S1751731115002475
Andriarimalala, J. H., Rakotozandriny, J. D. N., Andriamandroso, A. L. H., Penot, E., Naudin, K., Dugué, P., et al. (2013). Creating synergies between conservation agriculture and cattle production in crop-livestock farms: a study case in the Lake Alaotra Region of Madagascar. Exp. Agric. 49, 352–365 doi: 10.1017/S0014479713000112
Argel, M., Pedro, J., Miles, J. W., Guiot García, J. D., Cuadrado Capella, H., and Lascano, C. E. (2007). Cultivar Mulato II (Brachiaria hybrid CIAT 36087): A High-Quality Forage Grass, Resistant to Spittlebugs and Adapted to Well-Drained, Acid Tropical Soils. CIAT.
Ayele, S., Duncan, A., Larbi, A., and Khanh, T. T. (2012). Enhancing innovation in livestock value chains through networks: lessons from fodder innovation case studies in developing countries. Sci. Public Policy 39, 333–346. doi: 10.1093/scipol/scs022
Ba, N. X., Lane, P. A., Parsons, D., Van, N. H., Khanh, H. L. P., Corfield, J. P., et al. (2013). Forages improve livelihoods of smallholder farmers with beef cattle in South Central Coastal Vietnam. Trop. Grasslands Forrajes Tropicales 1, 225–229. doi: 10.17138/TGFT(1)225-229
Bai, Z., Lee, M. R. F., Ma, L., Ledgard, S., Oenema, O., Velthof, G. L., et al. (2018). Global environmental costs of China's thirst for milk. Glob. Change Biol. 24, 2198–2211. doi: 10.1111/gcb.14047
Baptistella, J. L. C., Andrade, S. A. L., Favarin, J. L., and Mazzafera, P. (2020). Urochloa in tropical agroecosystems. Front. Sustain. Food Syst. 4:119. doi: 10.3389/fsufs.2020.00119
Barros, E., Neves, A., Blanchart, E., Fernandes, E. C. M., Wandelli, E., Lavelle, P., et al. (2003). Development of the soil macrofauna community under silvopastoral and agrosilvicultural systems in Amazonia. Pedobiologia 47, 273–280. doi: 10.1078/0031-4056-00190
Bautista Solís, P. (2012). Are we learning? Strengthening local people's capacities to facilitate the recuperation of degraded pasture lands in Central America (Ph.D. thesis). CATIE, Bangor University, Bangor, United Kingdom.
Boddey, R. M., Casagrande, D. R., Homem, B. G. C., and Alves, B. J. R. (2020). Forage legumes in grass pastures in tropical Brazil and likely impacts on greenhouse gas emissions: a review. Grass For. Sci. 75, 357–371. doi: 10.1111/gfs.12498
Bowen, MK, Chudleigh, F, Buck, S, and Hopkins, K. (2016). Productivity and profitability of forage options for beef production in the subtropics of northern Australia. Anim. Product. Sci. 58, 332–342. doi: 10.1071/AN16180
Bravo, A., Enciso, K., Hurtado, J. J., del Cairo, J. R., Jäger, M., and Charry, A. (2018). Estrategia Sectorial de la Cadena de Ganadería Doble Propósito en Guaviare, Con Enfoque Agroambiental y Cero Deforestación. Cali: Centro Internacional de Agricultura Tropical (CIAT). Available online at: https://hdl.handle.net/10568/91289 (accessed November 14, 2019).
Broom, D. M., Galindo, F. A., and Murgueitio, E. (2013). Sustainable, efficient livestock production with high biodiversity and good welfare for animals. Proc. R. Soc. B Biol. Sci. 280:20132025. doi: 10.1098/rspb.2013.2025
Burkart, S., and Urrea-Benítez, J. L. (2020). The Colombian Roundtable for Sustainable Beef and Dairy: 2020 Activities. Cali: Alliance of Bioversity International and CIAT.
Butterbach-Bahl, K., Gettel, G., Kiese, R., Fuchs, K., Werner, C., Rahimi, J., et al. (2020). Livestock enclosures in drylands of Sub-Saharan Africa are overlooked hotspots of N 2 O emissions. Nat. Commun. 11, 1–6 doi: 10.1038/s41467-020-18359-y
Byerlee, D., and Lynam, J. K. (2020). The development of the international center model for agricultural research: a prehistory of the CGIAR. World Dev. 135:105080. doi: 10.1016/j.worlddev.2020.105080
Byrnes, R. C., Nùñez, J., Arenas, L., Rao, I., Trujillo, C., Alvarez, C., et al. (2017). Biological nitrification inhibition by Brachiaria grasses mitigates soil nitrous oxide emissions from bovine urine patches. Soil Biol. Biochem. 107, 156–163. doi: 10.1016/j.soilbio.2016.12.029
Calle, A., Montagnini, F., and Zuluaga, A. F. (2009). Farmer's perceptions of silvopastoral system promotion in Quindío, Colombia. Bois et forets des tropiques 300, 79–94. doi: 10.19182/bft2009.300.a20417
Chakoma, I., Gwiriri, L. C., Manyawu, G., Dube, S., Shumba, M., and Gora, A. (2016). Forage seed production and trade as a pathway out of poverty in the smallholder sector: lessons from the Zimbabwe Crop Livestock Integration for Food Security (ZimCLIFS) project. Afr. J. Range Forage Sci. 33, 181–184. doi: 10.2989/10220119.2016.1173097
Chang, T.-G., Chang, S., Song, Q.-F., Perveen, S., and Zhu, X.-G. (2019). Systems models, phenomics and genomics: three pillars for developing high-yielding photosynthetically efficient crops. in silico Plants 1:diy003. doi: 10.1093/insilicoplants/diy003
Charry, A., Narjes, M., Enciso, K., Peters, M., and Burkart, S. (2019). Sustainable intensification of beef production in Colombia - chances for product differentiation and price premiums. Agric. Food Econ. 7:22. doi: 10.1186/s40100-019-0143-7
Cheruíyot, D., Midega, C. A. O., Pittchar, J. O., Pickett, J. A., and Khan, Z. R. (2020). Farmers' perception and evaluation of Brachiaria Grass (Brachiaria spp.) genotypes for smallholder cereal-livestock production in East Africa. Agriculture 10:268. doi: 10.3390/agriculture10070268
Chirinda, N., Loaiza, S., Arenas, L., Ruiz, V., Faverín, C., Alvarez, C., et al. (2019). Adequate vegetative cover decreases nitrous oxide emissions from cattle urine deposited in grazed pastures under rainy season conditions. Sci. Rep. 9, 1–9. doi: 10.1038/s41598-018-37453-2
Chizmar, S., Castillo, M., Pizarro, D., Vasquez, H., Bernal, W., Rivera, R., et al. (2020). A discounted cash flow and capital budgeting analysis of silvopastoral systems in the Amazonas region of Peru. Land 9:353. doi: 10.3390/land9100353
CIAT (2004). Tropical Grasses and Legumes: Optimizing Genetic Diversity for Multipurpose Use (Project IP5). Annual Report 2004. Cali: Centro Internacional de Agricultura Tropical.
Cibils, A. F., McGrew, D., Kassambara, B., Bengaly, K., Sissoko, B., and Acharya, R. N. (2015). Challenges and opportunities for agro-pastoral livestock smallholders in Mali. Outlook Agric. 44, 69-80. doi: 10.5367/oa.2015.0198
Cook, B. G., Pengelly, B. C., Schultze-Kraft, R., Taylor, M., Burkart, S., Cardoso Arango, J. A., et al. (2020). Tropical Forages: An Interactive Selection Tool. Cali: International Center for Tropical Agriculture (CIAT). Nairobi: International Livestock Research Institute (ILRI).
Creemers, J. J. H. M., and Alvarez Aranguiz, A. (2019). White Paper: Pathways to Intensify Sustainable Forage Production in Kenya - NEADAP. Available online at: https://edepot.wur.nl/504127
Cuartas, C. C. A., Naranjo, R. J. F., Tarazona, M. A. M., Murgueitio, R. E., Chará, O. J. D., Ku-Vera, J. C., et al. (2014). Contribution of intensive silvopastoral systems to animal performance and to adaptation and mitigation of climate change. Revista Colombiana de Ciencias Pecuarias 27, 76–94. doi: 10.17533/udea.rccp
Dahlin, A. S., Mukangango, M., Naramabuye, F. X., Nduwamungu, J., and Nyberg, G. (2021). Effect of grass-diet and grass-legume-diet manure applied to planting holes on smallholder maize production in Rwanda. Field Crops Res. 263:108057. doi: 10.1016/j.fcr.2021.108057
D'Annolfo, R., Gemmill-Herren, B., Amudavi, D., Shiraku, H. W., Piva, M., and Garibaldi, L. A. (2021). The effects of agroecological farming systems on smallholder livelihoods: a case study on push-pull system from Western Kenya. Int. J. Agric. Sustain. 19, 56–70, doi: 10.1080/14735903.2020.1822639
David, M., Bernard, B., Anthony, E., Michael, M. S., James, W., Andrew, A., et al. (2020). The role of indigenous knowledge (IK) in adaptation to drought by agropastoral smallholder farmers in Uganda. Indian J. Trad. Knowledge19, 44–52.
De Farias, P. M., Arellano, L., Hernández, M. I. M., and Ortiz, S. L. (2015). Response of the copro-necrophagous beetle (Coleoptera: Scarabaeinae) assemblage to a range of soil characteristics and livestock management in a tropical landscape. J. Insect Conserv. 19, 947–960. doi: 10.1007/s10841-015-9812-3
de Oliveira Silva, R., Barioni, L. G., Hall, J. A. J., Matsuura, M. F., Albertini, T. Z., Fernandes, F. A., et al. (2016). Increasing beef production could lower greenhouse gas emissions in Brazil if decoupled from deforestation. Nat. Clim. Change 6, 493–497. doi: 10.1038/nclimate2916
Descheemaeker, K., Amede, T., and Haileslassie, A. (2010). Improving water productivity in mixed crop-livestock farming systems of sub-Saharan Africa. Agric. Water Manage. 97, 579–586. doi: 10.1016/j.agwat.2009.11.012
Dey, B., Notenbaert, A., Makkar, H., Mwendia, S., Peters, M., and Sahlu, Y. (2021). Forage Seed Systems and Feed Reserves: Business Propositions, Case for Ethiopia. Washington, DC: USAID.
Dias, M., Costa, K., Severiano, E., Bilego, U., Neto, A., Almeida, D., et al. (2020). Brachiaria and Panicum maximum in an integrated crop-livestock system and a second-crop maize system in succession with soybean. J. Agric. Sci. 158, 206–217. doi: 10.1017/S0021859620000532
Douxchamps, S., Frossard, E., Bernasconi, S. M., van der Hoek, R., Schmidt, A., Rao, I. M., et al. (2011). Nitrogen recoveries from organic amendments in crop and soil assessed by isotope techniques under tropical field conditions. Plant Soil 341, 179–192. doi: 10.1007/s11104-010-0633-6
Douxchamps, S., Humbert, F., van der Hoek, R., Mena, M., Bernasconi, S. M., Schmidt, A., et al. (2010). Nitrogen balances in farmers' fields under alternative uses of a cover crop legume - a case study from Nicaragua. Nutr. Cycl. Agroecosyst. 88, 447–462. doi: 10.1007/s10705-010-9368-2
Douxchamps, S., Rao, I. M., Peters, M., van der Hoek, R., Schmidt, A., Martens, S., et al. (2014). Farm-scale trade-offs between legume use as forage vs. green manure: the case of Canavalia brasiliensis. Agroecol. Sustain. Food Syst. 38, 25–45. doi: 10.1080/21683565.2013.828667
Du, Y., Ge, Y., Ren, Y., Fan, X., Pan, K., Lin, L., et al. (2018). A global strategy to mitigate the environmental impact of China's ruminant consumption boom. Nat Commun. 9:4133. doi: 10.1038/s41467-018-06381-0
Dumont, E. S., Bonhomme, S., Pagella, T. F., and Sinclair, F. L. (2019). Structured stakeholder engagement leads to development of more diverse and inclusive agroforestry options. Exp. Agric. 55, 252–274. doi: 10.1017/S0014479716000788
Elbasha, E., Thornton, P. K., and Tarawali, G. (1999). An Ex-post Economic Assessment of Planted Forages in West Africa. Nairobi: International Livestock Research Institute.
Enciso, K., Bravo, A., Charry, A., Rosas, G., Jäger, M., Hurtado, J. J., et al. (2018). Estrategia Sectorial de la Cadena de Ganadería Doble Propósito en Caquetá, Con Enfoque Agroambiental y cero Deforestación. Cali: Centro Internacional de Agricultura Tropical (CIAT). Available online at: https://hdl.handle.net/10568/91981 (accessed October 21, 2019).
Enciso, K., Castillo, J., Burkart, S., Avellaneda, Y., Vargas, J., Campuzano, L. F., et al. (2020). Evaluación Económica de la Avena Forrajera Altoandina para Sistemas Lecheros del Trópico Alto de Colombia. Mosquera. Available online at: https://hdl.handle.net/10568/111083
Enciso, K., Sotelo, M., Peters, M., and Burkart, S. (2019). The inclusion of Leucaena diversifolia in a Colombian beef cattle production system: an economic perspective. Trop. Grasslands Forrajes Tropicales 7, 359–369. doi: 10.17138/tgft(7)359-369
Epper, C. A., Paul, B., Burra, D. D., Phengsavanh, P., Ritzema, R. S., Syfongxay, C., et al. (2019). Nutrient flows and intensification options for smallholder farmers of the Lao uplands. Agric. Syst. 177:102694. doi: 10.1016/j.agsy.2019.102694
FAO (2018). Livestock and Agroecology. Available online at: http://www.fao.org/3/I8926EN/i8926en.pdf
FAO (2021). FAOSTAT Food and Agricultural Data. Food and Agriculture Organization (FAO) of the United Nations, Rome. Available online at: http://www.fao.org/faostat/en/#data
Finckh, M. (2008). Integration of breeding and technology into diversification strategies for disease control in modern agriculture. Eur. J. Plant Pathol. 121, 399–409. doi: 10.1007/s10658-008-9273-6
Fisher, M. J., Tao, I. M., Ayarza, M. A., Lascano, C. E., Sanz, J. I., Thomas, R. J., et al. (1994). Carbon storage by introduced deep-rooted grasses in the South American savannas. Nature 371, 236–238. doi: 10.1038/371236a0
García-Cruz, F., Ibrahim, M., and Pezo, D. (2013). “Los árboles en los potreros para la reducción del estrés calórico del ganado en los trópicos,” in Estado del recurso arbóreo en fincas ganaderas y su contribución en la producción en Rivas, Nicaragua, eds D. Sánchez, C. Villanueva, G. Rusch, M. Ibrahim, and F. DeClerck (Turrialba: CATIE), 36–41.
Garrett, R. D., Niles, M. T., Gil, J. D. B., Gaudin, A., Chaplin-Kramer, R., Assmann, A., et al. (2017b). Social and ecological analysis of commercial integrated crop livestock systems: current knowledge and remaining uncertainty. Agric. Syst. 155, 136–146. doi: 10.1016/j.agsy.2017.05.003
Garrett, R. D., Niles, M. T., Gil, J. D. B., Gaudin, A., Chaplin-Kramer, R., Assmann, A., Assmann, T. S., et al. (2017a). Social and ecological analysis of commercial integrated crop livestock systems: Current knowledge and remaining uncertainty. Agric. Syst. 155, 136–146.
Gaviria-Uribe, X., Bolivar, D. M., Rosenstock, T. S., Molina-Botero, I. C., Chirinda, N., Barahona, R., et al. (2020). Nutritional quality, voluntary intake and enteric methane emissions of diets based on novel Cayman grass and its associations with two Leucaena shrub legumes. Front. Vet. Sci. 7:764. doi: 10.3389/fvets.2020.579189
Geng, Y., Hu, G., Ranjitkar, S., Wang, Y., Bu, D., Pei, S., et al. (2017). Prioritizing fodder species based on traditional knowledge: a case study of mithun (Bos frontalis) in Dulongjiang area, Yunnan Province, Southwest China. J. Ethnobiol. Ethnomed. 13, 1–15. doi: 10.1186/s13002-017-0153-z
Giller, K. E., Hijbeek, R., Andersson, J. A., and Sumberg, J. (2021). Regenerative agriculture: an agronomic perspective. Outlook Agric. 50, 13-25. doi: 10.1177/0030727021998063
Giraldo, C., Escobar, F., Chara, J. D., and Calle, Z. (2011). The adoption of silvopastoral systems promotes the recovery of ecological processes regulated by dung beetles in the Colombian Andes. Insect Conserv. Divers. 4, 115–122. doi: 10.1111/j.1752-4598.2010.00112.x
Gliessman, S. (2018). Defining agroecology. Agroecol. Sustain. Food Syst. 42, 599–600. doi: 10.1080/21683565.2018.1432329
Godde, C. M., Mason-D'Croz, D., Mayberry, D. E., Thornton, P. K., and Herrero, M. (2021). Impacts of climate change on the livestock food supply chain; a review of the evidence. Glob. Food Security 28:100488. doi: 10.1016/j.gfs.2020.100488
Gontijo de Lima, F., Lee, S. T., Pfister, J. A., Sayuri Miyagi, E., Lage Costa, G., Dias da Silva, R., et al. (2015). The effect of ensiling and haymaking on the concentrations of steroidal saponin in two Brachiaria grass species. Cienc. Rural 45, 858–863. doi: 10.1590/0103-8478cr20141305
González, J. M. (2013). Costos y beneficios de un sistema silvopastoril intensivo (SSPi), con base en Leucaena leucocephala (Estudio de caso en el municipio de Tepalcatepec, Michoacán, México). Avances En Investigación Agropecuaria 17, 35–50.
Graesser, J., Aide, M. T., Grau, R. H., and Ramankutty, N. (2015). Cropland/pastureland dynamics and the slowdown of deforestation in Latin America. Environ. Res. Lett. 10:034017. doi: 10.1088/1748-9326/10/3/034017
Groot, J. C. J., Oomen, G. J. M., and Rossing, W. A. H. (2012). Multi-objective optimization and design of farming systems. Agric. Syst. 110, 63–77.
Harrison, S., Srisomporn, W., and Nakamanee, G. (2019). Production of Leucaena leucocephala ‘silage’ for sale as animal feed: a case study from Sikiew District, Northeast Thailand. Trop. Grasslands Forrajes Tropicales 7, 455–458. doi: 10.17138/tgft(7)455-458
Harvey, C. A., Medina, A., Sánchez Merlo, D., Vĺchez, S., Hernández, B., Saenz, J., et al. (2006). Patterns of animal diversity associated with different forms of tree cover retained in agricultural landscapes. Ecol. Appl. 16, 1986–1999.
Hassan, S., Inglese, P., Gristina, L., Liguori, G., Novara, A., Louhaichi, M., et al. (2019). Root growth and soil carbon turnover in Opuntia ficus-indica as affected by soil volume availability. Eur. J. Agron. 105, 104–110 doi: 10.1016/j.eja.2019.02.012
Havlík, P., Valin, H., Mosnier, A., Obersteiner, M., Baker, J. S., Herrero, M., et al. (2012). Crop productivity and the global livestock sector: implications for land use change and greenhouse gas emissions. Am. J. Agric. Econ. 95, 442–448. doi: 10.1093/ajae/aas085
Heinke, J., Lannerstad, M., Gerten, D., Havlík, P., Herrero, M., Notenbaert, A. M. O., et al. (2020). Water use in global livestock production-opportunities and constraints for increasing water productivity. Water Resour. Res. 56:12. doi: 10.1029/2019WR026995
Herrero, M., Thornton, P. K., Notenbaert, A. M., Wood, S., Msangi, S., Freeman, H. A., et al. (2010). Smart investments in sustainable food production: revisiting mixed crop-livestock systems. Science 327, 822–825. doi: 10.1126/science.1183725
Hidano, A., Gates, M. C., and Enticott, G. (2019). Farmers' decision making on livestock trading practices: cowshed culture and behavioral triggers amongst New Zealand Dairy Farmers. Front. Vet. Sci. 7:130. doi: 10.3389/fvets.2019.00320
Hilbeck, A., Hoffmann, U., Home, R., Levidow, L., Muller, A., Nelson, E., et al. (2015). Feeding the People - Agroecology for Nourishing the World and Feeding the Agri-Food System. IFOAM EU, 2015. Available online at: https://www.ifoameu.org/sites/default/files/ifoameu_policy_ffe_feedingthepeople.pdf
Hoffmann, V., Paul, B., Falade, T., Ramankutty, N., Moodley, A., and Olawoye, J. (in review). A one health approach to plant health. Submitted to food policy.
Hoste, H., Martinez-Ortiz-De-Montellano, C., Manolaraki, F., Brunet, S., Ojeda-Robertos, N., Fourquaux, I., et al. (2012). Direct and indirect effects of bioactive tannin-rich tropical and temperate legumes against nematode infections. Vet. Parasitol. 186, 18–27. doi: 10.1016/j.vetpar.2011.11.042
IFOAM EU (2019). Position Paper on Agro-Ecology. Available online at: https://www.organicseurope.bio/content/uploads/2020/06/ifoameu_position_paper_agroecology.pdf?dd
Inglese, P., Mondragon, C., Nefzaoui, A., and Saenz, C. (2018). Crop Ecology, Cultivation and Uses of Cactus Pear. Food and Agriculture Organization of the United Nations and the International Center for Agricultural Research in the Dry Areas. Rome.
ISPC (2018). Tropical Forages and the Diffusion of Brachiaria Cultivars in Latin America. Rome: CGIAR Independent Science and Partnership Council.
Jank, L., Barrios, S. C., Valle, C. B., Simeaio, R. M., and Alves, G. F. (2014). The value of improved pastures to Brazilian beef production. Crop Past. Sci. 65, 1132–1137. doi: 10.1071/CP13319
Jimenez-Trujillo, J. A., Ibrahim, M., Pezo, D., Guevara-Hernandez, F., Gomez-Castro, H., Nahed-Toral, J., et al. (2011). Comparison of animal productivity and profitability between a silvopastoral system (Brachiaria brizantha associated with Leucaena leucocephala) and a conventional system (B. brizantha + chicken manure). Res. J. Biol. Sci. 6, 75–81. doi: 10.3923/rjbsci.2011.75.81
Jin, S., Zhang, B., Wu, B., Han, D., Hu, Y., Ren, C., et al. (2020). Decoupling livestock and crop production at the household level in China. Nat. Sustain. 4, 48–55. doi: 10.1038/s41893-020-00596-0
Jones, A. K., Jones, D. L., Edwards-Jones, G., and Cross, P. (2013). Informing decision making in agricultural greenhouse gas mitigation policy: a Best-Worst Scaling survey of expert and farmer opinion in the sheep industry. Environ. Sci. Policy 29, 46–56. doi: 10.1016/j.envsci.2013.02.003
Kaimowitz, D., and Angelsen, A. (2008). Will livestock intensification help save Latin America's tropical forests? J. Sustain. Forestry 27, 6–24. doi: 10.1080/10549810802225168
Khan, Z. R., Midega, C. A., Amudavi, D. M., Hassanali, A., and Pickett, J. A. (2008). On-farm evaluation of the ‘push-pull’ technology for the control of stemborers and striga weed on maize in western Kenya. Field Crops Res. 106, 224–233. doi: 10.1016/j.fcr.2007.12.002
Labarta, R., Martínez, J. M., Yaccelga, A., Reyes, B., Gómez, L., Maredia, M., et al. (2017). Assessing the Adoption and Economic and Environmental Impacts of Brachiaria Grass Forage Cultivars in Latin America Focusing in the Experience of Latin America. Research Report to the Standing Panel of Impact Assessment (SPIA) under the SIAC project. Cali.
Lamine, C., and Dawson, J. (2018). The agroecology of food systems: Reconnecting agriculture, food, and the environment. Agroecol. Sustain. Food Syst. 42, 629–636. doi: 10.1080/21683565.2018.1432517
Lascano, C. E., Peters, M., and Holmann, F. (2005). Arachis pintoi in the humid tropics of Colombia: a forage legume success story. Trop. Grasslands 39:220.
Le Coq, J., Sabourin, E., Bonin, M., Gresh, S., Marzin, J., Niederle, P., et al. (2020). Public policy support for agroecology in Latin America: lessons and perspectives. Glob. J. Ecol. 129–138. doi: 10.17352/gje.000032
Lema, Z., de Bruyn, L. A. L., Marshall, G. R., Roschinsky, R., and Duncan, A. J. (2021). Multilevel innovation platforms for development of smallholder livestock systems: how effective are they? Agric. Syst. 189:103047. doi: 10.1016/j.agsy.2020.103047
Lerner, A., Rudel, T. K., Schneider, L., McGroddy, M., Burbano, D., and Mena, C. (2015). The spontaneous emergence of silvo-pastoral landscapes in the Ecuadorian amazon: patterns and processes. Reg. Environ. Change 15, 357–371. doi: 10.1007/s10113-014-0699-4
Lie, H., Rich, K. M., and Burkart, S. (2017). Participatory system dynamics modelling for dairy value chain development in Nicaragua. Dev. Pract. 27, 785–800. doi: 10.1080/09614524.2017.1343800
Lie, H., Rich, K. M., van der Hoek, R., and Dizyee, K. (2018). An empirical evaluation of policy options for inclusive dairy value chain development in Nicaragua: a system dynamics approach. Agric. Syst. 164, 193–222. doi: 10.1016/j.agsy.2018.03.008
Lira, M. A. Jr, Fracetto, F. J. C., Ferreira, J. D. S., Silva, M. B., and Fracetto, G. G. M. (2020). Legume silvopastoral systems enhance soil organic matter quality in a subhumid tropical environment. Soil Sci. Society of America Journal 84, 1209–1218. doi: 10.1002/saj2.20106
Louhaichi, M., Kumar, S., Tiwari, S., Islam, M., Hassan, S., Yadav, O. P., et al. (2018). Adoption and utilization of Cactus Pear in South Asia-Smallholder farmers' perceptions. Sustainability 10:3625. doi: 10.3390/su10103625
Lowder, S. K., Sánchez, M. V., and Bertini, R. (2021). Which farms feed the world and has farmland become more concentrated? World Dev. 142:105455. doi: 10.1016/j.worlddev.2021.105455
Lukuyu, B., Baker, D., Baltenweck, I., Poole, J., Kabi, F., Katongole, C., et al. (2013). The Concentrate Feeds Supply Chain in Uganda: Emerging Trends and Implications on Quality and Access to Smallholder Farmers and Chain Efficiency. Heifer International and East Africa Dairy Development. Available online at: https://cgspace.cgiar.org/bitstream/handle/10568/34469/Concentrate%20Feeds%20Supply%20chain%20in%20Uganda.pdf?sequence=1
Lukuyu, B., Mutambo, I., and Ouma, E. (2020). Gender Dynamics and Social Implications of Improved Planted Forages in Smallholder Piggery Systems in Uganda. Nairobi: ILRI. Available online at: https://hdl.handle.net/10568/108297
Lukuyu, B., Mutambo, I., and Ouma, E. (2021). Gender Dynamics and Social Implications of Improved Planted Forages in Smallholder Dairy Systems in Kenya. Nairobi: ILRI. Available online at: https://hdl.handle.net/10568/113636
Maass, B. L., Midega, C. A. O., Mutimura, M., Rahetlah, V. B., Salgado, P., Kabirizi, J. M., et al. (2015). Homecoming of Brachiaria: improved hybrids prove useful for African animal agriculture. East Afr. Agric. Forest. J. 81, 71–78. doi: 10.1080/00128325.2015.1041263
Mangwe, M. C., Bryant, R. H., Beck, M. R., Beale, N., Bunt, C., and Gregorini, P. (2019). Forage herbs as an alternative to ryegrass-white clover to alter urination patterns in grazing dairy systems. Anim. Feed Sci. Technol. 252, 11–22. doi: 10.1016/j.anifeedsci.2019.04.001
Marra, M., Pannell, D. J., and Abadi Ghadim, A. (2003). The economics of risk, uncertainty and learning in the adoption of new agricultural technologies: where are we on the learning curve? Agric. Syst. 75, 215–234. doi: 10.1016/S0308-521X(02)00066-5
Martinez, L. J., and Zinck, J. A. (2004). Temporal variation of soil compaction and deterioration of soil quality in pasture areas of Colombian Amazonia. Soil Tillage Res. 75, 3–18. doi: 10.1016/j.still.2002.12.001
Martínez-García, C. G., Dorward, P., and Rehman, T. (2013). Factors influencing adoption of improved grassland management by small-scale dairy farmers in central Mexico and the implications for future research on smallholder adoption in developing countries. Livestock Sci. 152, 228–238. doi: 10.1016/j.livsci.2012.10.007
Mayberry, D., Hau, D. K., Dida, P. R., Bria, D., Praing, J., Mata, A. D., et al. (2020). Herbaceous legumes provide several options for increasing beef cattle productivity in eastern Indonesia. Anim. Product. Sci. 61 698–707 doi: 10.1071/AN20545
Mayer, J. A., and Cushman, J. C. (2019). Nutritional and mineral content of prickly pear cactus: a highly water-use efficient forage, fodder and food species. J. Agron. Crop Sci. 205, 625–634. doi: 10.1111/jac.12353
Méndez, V. E., Bacon, C. M., and Cohen, R. (2013). Agroecology as a transdisciplinary, participatory, and action-oriented approach. Agroecol. Sustain. Food Syst. 37, 3–18.
Meybeck, A., and Gitz, V. (2017). Sustainable diets within sustainable food systems. Proc. Nutr. Soc. 76, 1–11. doi: 10.1017/S0029665116000653
Meyfroidt, P. (2018). Trade offs between environment and livelihoods: bridging the global land use and food security discussions. Glob. Food Security 16, 9–16. doi: 10.1016/j.gfs.2017.08.001
Montoya-Flores, M. D., Molina-Botero, I. C., Arango, J., Romano-Muñoz, J. L., Solorio-Sánchez, F. J., Aguilar-Pérez, C. F., et al. (2020). Effect of dried leaves of Leucaena leucocephala on rumen fermentation, rumen microbial population, and enteric methane production in crossbred heifers. Animals 10:300. doi: 10.3390/ani10020300
Mottet, A., Bicksler, A., Lucantoni, D., De Rosa, F., Scherf, B., Scopel, E., et al. (2020). Assessing transitions to sustainable agricultural and food systems: a tool for agroecology performance evaluation (TAPE). Front. Sustain. Food Syst. 4:579154. doi: 10.3389/fsufs.2020.579154
Mueller, N. D., and Lassaletta, L. (2020). Nitrogen challenges in global livestock systems. Nature Food. 1, 400–401. doi: 10.1038/s43016-020-0117-7
Murgueitio, E., Barahona, R., Chará, J. D., Flores, M. X., Mauricio, R. M., and Molina, J. J. (2015). The intensive silvopastoral systems in Latin America sustainable alternative to face climatic change in animal husbandry. Cuban J. Agric. Sci. 49, 541–554.
Mwendia, S., Ohmstedt, U., and Peters, M. (2019). “Make money from forage hay production as a business” in Poster prepared for the Maziwa Zaidi Agribusiness Forum, Moshi, Tanzania (Cali: CIAT). Available online at: https://hdl.handle.net/10568/105484
Nakamanee, G., Srisomporn, W., Phengsavanh, P., Samson, J., and Stür, W. (2008). Sale of fresh forage - a new cash crop for smallholder farmers in Yasothon, Thailand. Trop. Grasslands 42, 65–74.
Nefzaoui, A., Louhaichi, M., and Ben Salem, H. (2014). Cactus as a tool to mitigate drought and to combat desertification. J. Arid Land Stud. 24, 121–124.
Negassa, A., Shapiro, B., Kidane, T., Abdena, A., and Hanson, J. (2016). Ex-Ante Assessment of Demand for Improved Forage Seed and Planting Materials Among Ethiopian Smallholder Farmers: A Contingent Valuation Analysis. ILRI Project Report. Nairobi: ILRI. Available online at: https://hdl.handle.net/10568/78657
Neres, M. A., Nath, C. D., and Hoppen, S. M. (2021). Expansion of hay production and marketing in Brazil. Heliyon 7:E06787. doi: 10.1016/j.heliyon.2021.e06787
Njeru, N. K., Midega, C. A. O., Muthomi, J. W., Wagacha, J. M., and Khan, Z. R. (2020). Impact of push-pull cropping system on pest management and occurrence of ear rots and mycotoxin contamination of maize in western Kenya. Plant Pathol. 69, 1644-1654. doi: 10.1111/ppa.13259
Njuguna-Mungai (under review). Gender dynamics surrounding the introduction of improved forages in Kenya and Ethiopia.
Ntakyo, R. P., Kirunda, H., Tugume, G., and Natuha, S. (2020). Dry season feeding technologies: assessing the nutritional and economic benefits of feeding hay and silage to dairy cattle in South-Western Uganda. Open J. Anim. Sci. 10, 627-648. doi: 10.4236/ojas.2020.103041
Nwafor, I. C., Shale, K., and Achilonu, M. C. (2017). Chemical composition and nutritive benefits of chicory (Cichorium intybus) as an ideal complementary and/or alternative livestock feed supplement. ScientificWorldJournal 2017:7343928. doi: 10.1155/2017/7343928
Nyambati, E. M., Sollenberger, L. E., Hiebsch, C. K., and Rono, S. C. (2006). On-farm productivity of relay-cropped mucuna and lablab in smallholder crop-livestock systems in northwestern Kenya. J. Sustain. Agric. 28, 97–116. doi: 10.1300/J064v28n01_09
Ohmstedt, U. (2020a). Forage Growing and on Farm Feeding of the Produced Forages: A Business Case. Nairobi: Alliance of Bioversity International and CIAT.
Ohmstedt, U. (2020b). Factsheets on Forage-Related Business Approaches for Kenya. Nairobi: Alliance of Bioversity International and CIAT.
Olaya-Montes, A., Llanos-Cabrera, M. P., Cherubin, M. R., Herrera-Valencia, W., Ortiz-Morea, F. A., and Silva-Olaya, A. M. (2020). Restoring soil carbon and chemical properties through silvopastoral adoption in the Colombian Amazon region. Land Degrad. Dev. 32, 3720–3730. doi: 10.1002/ldr.3832
Oulu, M., and Notenbaert, A. (2020). Adoption of Improved Forages in Western Kenya: Key Underlying Factors. [Presentation done November 24, 2020]. 46 slides. Available online at: https://hdl.handle.net/10568/110543
Paul, B. K., Butterbach-Bahl, K., Notenbaert, A., Nderi, A. N., and Ericksen, P. (2020a). Sustainable livestock development in low and middle income countries - shedding light on evidence-based solutions. Environ. Res. Lett. 16:011001. doi: 10.1088/1748-9326/abc278
Paul, B. K., Groot, J. C., Maass, B. L., Notenbaert, A. M., Herrero, M., and Tittonell, P. A. (2020c). Improved feeding and forages at a crossroads: farming systems approaches for sustainable livestock development in East Africa. Outlook Agric. 49, 13-20. doi: 10.1177/2F0030727020906170
Paul, B. K., Groot, J. C. J., Birnholz, C. A., Nzogela, B., Notenbaert, A., Woyessa, K., et al. (2019). Reducing agro-environmental trade-offs through sustainable livestock intensification across smallholder systems in Northern Tanzania. Int. J. Agric. Sustain. 18, 1–20. doi: 10.1080/14735903.2019.1695348
Paul, B. K., Maass, B., Koge, J., Notenbaert, A., Peters, M., Groot, J. G. J., et al. (2020b). Tropical forage technologies can deliver multiple benefits in Sub-Sahara Africa: a meta-analysis. Agron. Sustain. Dev. 40:22. doi: 10.1007/s13593-020-00626-3
Peters, M., Herrero, M., Fisher, M., Erb, K.-H., Rao, I., Subbarao, G. V., et al. (2013). Challenges and opportunities for improving eco-efficiency of tropical forage-based systems to mitigate greenhouse gas emissions. Trop. Grasslands Forrajes Trop. 1, 137–148. doi: 10.17138/TGFT(1)156-167
Peters, M., Horne, P., Schmidt, A., Holmann, F., Kerridge, P. C., Tarawali, S. A., et al. (2001). The Role of Forages in Reducing Poverty and Degradation of Natural Resources in Tropical Production Systems. Agricultural Research and Extension Network. Available online at: https://cdn.odi.org/media/documents/5218.pdf
Peters, M., and Lascano, C. E. (2003). Forage technology adoption: linking on-station research with participatory methods. Trop. Grasslands 37, 197–204.
Peters, M., Rao, I., Fisher, M., Subbarao, G., Martens, S., Herrero, M., et al. (2012). “Tropical forage-based systems to mitigate greenhouse gas emissions,” in CIAT. 2012. Eco-Efficiency: From Vision to Reality - Issues in Tropical Agriculture (Cali: CIAT).
Peterson, C. A., Deiss, L., and Gaudin, A. C. M. (2020). Integrated crop-livestock systems achieve comparable crop yields to specialized systems: a meta analysis. PLoS ONE 15:e0231840. doi: 10.1371/journal.pone.0231840
Pezo, D., Cruz, J., and Piniero, M. (2007). Escuelas de Campo para Ganaderos: Una estrategia para la rehabilitación y diversificación de fincas con pasturas degradadas. Archivos ALPA 15(Suppl. 1), 42–48.
Pezo, D., Ríos, N., Ibrahim, M., and Gómez, M. (2018). Silvopastoral Systems for Intensifying Cattle Production and Enhancing Forest Cover: The Case of Costa Rica. Washington, DC: World Bank.
Pulido, F., García, E., Obrador, J. J., and Moreno, G. (2010). Multiple pathways for tree regeneration in anthropogenic savannas: incorporating biotic and abiotic drivers into management schemes. J. Appl. Ecol. 47, 1272–1281. doi: 10.1111/j.1365-2664.2010.01865.x
Rao, I., Peters, M., Castro, A., Schultze-Kraft, R., White, D., Fisher, M., et al. (2015). LivestockPlus - the sustainable intensification of forage-based agricultural systems to improve livelihoods and ecosystem services in the tropics. Trop. Grassl. Forrajes Trop. 3, 59–82. doi: 10.17138/TGFT(3)59-82
Rietveld, A. M., Groot, J. C. J., and van der Burg, M. (2021). Predictable patterns of unsustainable intensification. Int. J. Agric. Sustain. 1–17. doi: 10.1080/14735903.2021.1940731
Ríos, N., Cárdenas, A. Y., Andrade, H. J., Ibrahim, M., Jiménez, F., Sancho, F., et al. (2006). Escorrentía superficial e infiltración en sistemas ganaderos convencionales y silvopastoriles en el trópico sub-húmedo de Nicaragua y Costa Rica. Agroforestería en las Américas 45, 66–71.
Rivas, L., and Holmann, F. (2000). Early Adoption of Arachis Pintoi in the Humid Tropics: The Case of Dual-Purpose Livestock Systems in Caquetá, Colombia. Livestock Research for Rural Development. Available online at: http://www.lrrd.org/lrrd12/3/riva123.htm
Rivas, L., and Holmann, F. (2004a). Impacto económico potencial de la adopción de nuevas Brachiarias resistentes a cercópidos. Pasturas Tropicales 26, 39–55.
Rivas, L., and Holmann, F. (2004b). Impacto de la Adopción de Híbridos de Brachiarias Resistentes al Salivazo: Colombia, México, y Centroamérica. Cali: Centro Internacional de Agricultura Tropical (CIAT).
Rivera, L. F., Armbrecht, I., and Calle, Z. (2013). Silvopastoral systems and ant diversity conservation in a cattle-dominated landscape of the Colombian Andes. Agric. Ecosyst. Environ. 181, 188–194. doi: 10.1016/j.agee.2013.09.011
Robinson, T. P., Thornton, P. K., Franceschini, G., Kruska, R. L., Chiozza, F., Notenbaert, A., et al. (2011). Global Livestock Production Systems. Rome: Food and Agriculture Organization of the United Nations (FAO) and International Livestock Research Institute (ILRI).
Rocha Filho, R. R., Santos, D. C., Véras, A. S. C., Siqueira, M. C. B., Novaes, L. P., Mora-Luna, R., et al. (2021). Can spineless forage cactus be the queen of forage crops in dryland areas? J. Arid Environ. 186:104426. doi: 10.1016/j.jaridenv.2020.104426
Rossi Borges, J. A., and Oude Lansink, A. G. J. M. (2016). Identifying psychological factors that determine cattle farmers' intention to use improved natural grassland. J. Environ. Psychol. 45, 89–96. doi: 10.1016/j.jenvp.2015.12.001
Rudel, T. K., Paul, B., White, D., Rao, I. M., Van Der Hoek, R., Castro, A., et al. (2015). LivestockPlus: forages, sustainable intensification, and food security in the tropics. Ambio 44, 685–693. doi: 10.1007/s13280-015-0676-2
Ruden, A., Castro, J. P., Gutiérrez, J. F., Koenig, S., and Arango, J. (2020). GANSO: New Business Model and Technical Assistance for the Professionalization of Sustainable Livestock Farming in the Colombian Orinoquia region. CCAFS Info Note. Cali: CGIAR Research Program on Climate Change, Agriculture and Food Security (CCAFS).
Ruiz, L. R., Burkart, S., Muñoz Quiceno, J. J., Enciso, K., Gutierrez Solis, J. F., Charry, A., et al. (2016). “Inhibiting factors and promotion strategies for increasing adoption levels of improved forages in cattle production,” in Tropentag 2016 “Solidarity in a Competing World Fair Use of Resources” (Vienna: Centro Internacional de Agricultura Tropical). Available online at: https://hdl.handle.net/10568/77030 (accessed August 28, 2019).
Sacande, M., and Berrahmouni, N. (2016). Community participation and ecological criteria for selecting species and restoring natural capital with native species in the Sahel: restoring natural capital in the Sahel. Restor. Ecol. 24, 479–488 doi: 10.1111/rec.12337
Sanderman, J., Heng, T., and Fiske, G. J. (2017). Soil carbon debt of 12,000 years of human land use. Proc. Natl Acad. Sci. U.S.A. 114, 9575–9580. doi: 10.1073/pnas.1706103114
Schiek, B., González, C., Mwendia, S., and Prager, S. D. (2018). Got forages? Understanding potential returns on investment in Brachiaria spp. for dairy producers in Eastern Africa. Trop. Grasslands Forrajes Tropicales 6, 117–133. doi: 10.17138/tgft(6)117-133
Schultze-Kraft, R., Rao, I. M., Peters, M., Clements, R. J., Bai, C., and Liu, G. (2018). Tropical forage legumes for environmental benefits: an overview. Trop. Grassl. Forrajes Trop. 6, 1–14. doi: 10.17138/TGFT(6)1-14
Seddon, N., Smith, A., Smith, P., Key, I., Chausson, A., Girardin, C., et al. (2021). Getting the message right on nature-based solutions to climate change. Glob. Change Biol. 27, 1518-1546. doi: 10.1111/gcb.15513
Seré, C., and Estrada, R. (1982). “Análisis económico de sistemas de ceba en pastos mejorados en los Llanos Orientales de Colombia,” in Referencias y ayudas utilizadas en el programa de capacitación científica en investigación para la producción y utilización de pastos tropicales, ed J. M. Spain (Cali, Colombia: Centro Internacional de Agricultura Tropical), 1063–1075.
Seré, C., Estrada, R., and Ferguson, J. (1993). Estudios de adopción e impacto en pasturas tropicales, investigación con pasturas en fincas. Centro Internacional de Agricultura Tropical (CIAT), Cali. Available online at: https://hdl.handle.net/10568/55753
Shapiro, M. J., Downs, S. M., Swartz, H. J., Parker, M., Quelhas, D., Kreis, K., et al. (2019). A systematic review investigating the relation between animal-source food consumption and stunting in children aged 6-60 months in low-and middle-income countries. Adv. Nutr. 10, 827–847. doi: 10.1093/advances/nmz018
Shelton, M., and Dalzell, S. (2007). Production, economic and environmental benefits of leucaena pastures. Trop. Grasslands 41, 174–190.
Silva, J. A., de Paula Sousa, D., de Paula, N. F., da Silva Carvalho, A. P., Gomes Macedo, B., da Costa Júnior, W. S., et al. (2017). Supplementation strategies for dairy cows kept in tropical grass pastures. Semina Ciências Agrárias 38, 401–416. doi: 10.5433/1679-0359.2017v38n1p401
Smith, J., Cadavid, J. V., Rincón, A., and Vera, R. (1997). Land speculation and intensification at the Frontier: a seeming paradox in the Colombian Savanna. Agric. Syst. 54, 501–520. doi: 10.1016/S0308-521X(96)00088-1
Snijders, P. J. M., Wouters, B. P., and Kariuki, J. N. (2011). Effect of Cutting Management and Nitrogen Supply on Yield and Quality of Napier grass (Pennisetum purpureum). Wageningen UR Livestock Research. Available online at: https://www.wur.nl/en/Publication-details.htm?publicationId=publication-way-343137363539
Sordillo, L. M. (2016). Nutritional strategies to optimize dairy cattle immunity. J. Dairy Sci.99, 4967–4982. doi: 10.3168/jds.2015-10354
Sousa, L. F., Maurício, R. M., Paciullo, D. S. C., Silveira, S. R., Ribeiro, R. S., Calsavara, L. H., et al. (2015). Forage intake, feeding behavior and bio-climatological indices of pasture grass, under the influence of trees, in a silvopastoral system. Trop. Grasslands Forrajes Tropicales 3, 129–141. doi: 10.17138/TGFT(3)129-141
Soussana J.-F Muriel, T., Philippe, L., and Bertrand, D. (2015). Agroecology: integration with livestock. In: International Symposium on Agroecology for Food Security and Nutrition (Rome: FAO), 225–249. Available online at: http://www.fao.org/3/a-i4729e.pdf
Subbarao, G. V., Nakahara, K., Hurtado, M. D. P., Ono, H., Moreta, D. E., Salcedo, A. F., et al. (2009). Evidence for biological nitrification inhibition in Brachiaria pastures. Proc. Natl. Acad. Sci. U.S.A. 106, 17302–17307. doi: 10.1073/pnas.0903694106
Tapasco, J., LeCoq, J. F., Ruden, A., Rivas, J. S., and Ortiz, J. (2019). The livestock sector in Colombia: toward a program to facilitate large-scale adoption of mitigation and adaptation practices. Front. Sustain. Food Syst. 3:61. doi: 10.3389/fsufs.2019.00061
Trujillo-Barrera, A., Pennings, J. M. E., and Hofenk, D. (2016). Understanding producers' motives for adopting sustainable practices: the role of expected rewards, risk perception and risk tolerance. Eur. Rev. Agric. Econ. 43, 359–382. doi: 10.1093/erae/jbv038
Umunezero, O., Mwendia, S., Paul, B. K., et al. (2016). Identifying and Characterizing Areas for Potential Forage Production in Rwanda. Cali: International Center for Tropical Agriculture (CIAT).
Valentim, J. F., and Andrade, C. M. S. (2005). Forage peanut (Arachis pintoi): a high yielding and high-quality tropical legume for sustainable cattle production systems in the western Brazilian Amazon. Trop. Grasslands 39:222.
Valette, M. (2019). How diverse is tree planting in the Central Plateau of Burkina Faso? Comparing small-scale restoration with other planting initiatives. Forests 10.3:227. doi: 10.3390/f10030227
van Winsen, F., de Mey, Y., Lauwers, L., Van Passel, S., Vancauteren, M., and Wauters, E. (2014). Determinants of risk behaviour: effects of perceived risks and risk attitude on farmer's adoption of risk management strategies. J. Risk Res. 19, 56–78. doi: 10.1080/13669877.2014.940597
Vazquez, E., Teutscherova, N., Lojka, B., Arango, J., and Pulleman, M. (2020). Pasture diversification affects soil macrofauna and soil biophysical properties in tropical (silvo) pastoral systems. Agric. Ecosyst. Environ. 302:107083. doi: 10.1016/j.agee.2020.107083
Villegas, D. M., Velasquez, J., Arango, J., Obregon, K., Rao, I. M., Rosas, G., et al. (2020). Urochloa grasses swap nitrogen source when grown in association with legumes in tropical pastures. Diversity 12:419. doi: 10.3390/d12110419
Vinceti, B. (2020). How is forest landscape restoration being implemented in Burkina Faso? Overview of ongoing initiatives. Sustainability 12:10430. doi: 10.3390/su122410430
Wan, N.-F., Zheng, X.-R., Fu, L.-W., Kiær, L. P., Zhang, Z., Chaplin-Kramer, R., et al. (2020). Global synthesis of effects of plant species diversity on trophic groups and interactions. Nat Plants 6, 503–510. doi: 10.1038/s41477-020-0654-y
Wezel, A., Herren, B. G., Kerr, R. B., Barrios, E., Rodrigues, A. L., and Sinclair, F. (2020). Agroecological principles and elements and their implications for transitioning to sustainable food systems. A review. Agron. Sustain. Dev. 40:40. doi: 10.1007/s13593-020-00646-z
White, D., Holmann, F., Fujisaki, S., Reategui, K., and Lascano, C. (2001). “Will intensifying pasture management in Latin America protect forests - Or is it the other way around?,” in Agricultural Technologies and Tropical Deforestation, eds A. Angelsen and D. Kaimowitz (Wallingford: CABI Publishing), 91–113. doi: 10.1079/9780851994512.0091
White, D., Peters, M., and Horne, P. (2013). Global impacts from improved tropical forages: a meta-analysis revealing overlooked benefits and costs, evolving values and new priorities. Trop. Grasslands Forrajes Tropicales 1, 12–24. doi: 10.17138/TGFT(1)12-24
Willett, W., Rockström, J., Loken, B., Springmann, M., Lang, T., Vermeulen, S., et al. (2019). Food in the anthropocene: the EAT-Lancet Commission on healthy diets from sustainable food systems. Lancet Commissions 393, 447–492. doi: 10.1016/S0140-6736(18)31788-4
Winders, W., and Ransom, E. (2019). Global Meat: The Social and Environmental Implications of the Expanding Meat Industry. Cambridge, MA: MIT Press. doi: 10.7551/mitpress/11868.001.0001
Wunscher, T., Schultze-Kraft, R., Peters, M., and Rivas, L. (2004). Early adoption of the tropical forage legume Arachis Pintoi in Huetar norte, Costa Rica. Exp. Agric. 40, 257–268. doi: 10.1017/S0014479703001583
Xuan, T. D., Elzaawely, A. A., Deba, F., Fukuta, M., and Tawata, S. (2006). Mimosine in Leucaena as a potent bio-herbicide. Agron. Sustain. Dev. 26, 89–97.
Zahoor, N., Abdullah, N., and Zakaria, N. (2021). The role of high performance work practices, work-family conflict, job stress and personality in affecting work life balance. Manage. Sci. Lett. 11, 1367–1378.
Zhang, X., Fang, Q., Zhang, T., Ma, W., Velthof, G. L., Hou, Y., et al. (2020). Benefits and trade-offs of replacing synthetic fertilizers by animal manures in crop production in China: a meta-analysis. Global Change Biol. 26, 888–900 doi: 10.1111/gcb.14826
Keywords: tropical forages, improved forages, cultivated forages, agroecology, mixed crop-tree-livestock systems, environmental co-benefits
Citation: Notenbaert AMO, Douxchamps S, Villegas DM, Arango J, Paul BK, Burkart S, Rao I, Kettle CJ, Rudel T, Vázquez E, Teutscherova N, Chirinda N, Groot JCJ, Wironen M, Pulleman M, Louhaichi M, Hassan S, Oberson A, Nyawira SS, Pinares-Patino CS and Peters M (2021) Tapping Into the Environmental Co-benefits of Improved Tropical Forages for an Agroecological Transformation of Livestock Production Systems. Front. Sustain. Food Syst. 5:742842. doi: 10.3389/fsufs.2021.742842
Received: 16 July 2021; Accepted: 15 October 2021;
Published: 11 November 2021.
Edited by:
Rein Van Der Hoek, Alliance Bioversity International and CIAT, FranceReviewed by:
Danilo Pezo, Centro Agronomico Tropical De Investigacion Y. Ensenanza Catie, Costa RicaSandrine Freguin Gresh, UMR ART-Dev, CIRAD, France
Copyright © 2021 Notenbaert, Douxchamps, Villegas, Arango, Paul, Burkart, Rao, Kettle, Rudel, Vázquez, Teutscherova, Chirinda, Groot, Wironen, Pulleman, Louhaichi, Hassan, Oberson, Nyawira, Pinares-Patino and Peters. This is an open-access article distributed under the terms of the Creative Commons Attribution License (CC BY). The use, distribution or reproduction in other forums is permitted, provided the original author(s) and the copyright owner(s) are credited and that the original publication in this journal is cited, in accordance with accepted academic practice. No use, distribution or reproduction is permitted which does not comply with these terms.
*Correspondence: An M. O. Notenbaert, a.notenbaert@cgiar.org