- 1Department of Food, Agricultural, and Biological Engineering, Ohio State University, Columbus, OH, United States
- 2The Nature Conservancy, Columbus, OH, United States
- 3Department of Agricultural, Environmental, and Development Economics, Ohio State University, Columbus, OH, United States
- 4Translational Data Analytics Institute, Ohio State University, Columbus, OH, United States
- 5Agricultural Research Service, United States Department of Agriculture, Columbus, OH, United States
- 6Ohio State University Extension, Columbus, OH, United States
- 7Herbert Wertheim School of Public Health and Human Longevity Science, University of California, San Diego, San Diego, CA, United States
- 8School of Environment and Natural Resources, Ohio State University, Columbus, OH, United States
- 9Department of Civil, Environmental, and Geodetic Engineering, Ohio State University, Columbus, OH, United States
- 10Sustainability Institute, Ohio State University, Columbus, OH, United States
Legacy nutrients stored in agricultural soils are a substantial component of riverine nutrient discharge contributing to the eutrophication of aquatic ecosystems. These nutrient loads can persist and delay water quality initiatives, for example, those of the Great Lakes Water Quality Agreement which seek to reduce phosphorus (P) loads entering the Western Lake Erie Basin. In this watershed, approximately 5% of fields have P concentrations 2.5-fold greater than the maximum agronomic recommendations for corn and soybeans. Fields with these elevated-P concentrations (>100 mg P kg−1 soil) act as a source of legacy-P and discharge greater P loads. Implementing best management practices to treat runoff from these fields is desirable but finding them has been a challenge as soil test data are proprietary information creating an asymmetric information barrier. To overcome this barrier, we formed a public-private partnership that included agricultural retailers who conduct soil testing for farmers. Agricultural retailers who partnered with this project provided their soil P data and contacted farmers to gauge their interest, maintaining privacy for farmers until they expressed interest. Only 3.8% of soil samples in the provided data had elevated-P concentrations. In many cases, these elevated-P soils were confined to zones within fields, and 13% of fields had at least one elevated-P zone. We pursued these elevated-P fields as research sites for the implementation and monitoring of management practices. The agricultural retailers contacted 77 farmers with surveys, and 25 responded with interest in meeting the research team to discuss the project. Following a preliminary evaluation with the spatial data of fields operated by interested farmers, visits were arranged so that 12 research sites could be located. As indicated through the surveys, discussions with farmers, and soil data, many of the fields had accumulated elevated-P due to historic land-use (livestock, manure, or biosolid application) creating legacy sources. We conclude that public-private partnerships featuring agricultural retailers are a promising tool that may help overcome asymmetric information barriers to finding and managing agricultural fields with legacy-P that that disproportionately contribute to nutrient runoff.
Introduction
Legacy nutrients stored in agricultural soils pose a serious threat to downstream waterbodies as they steadily leach into surface and subsurface runoff. Across the world, agricultural discharge of nutrients has contributed to the eutrophication of many aquatic ecosystems (Smith et al., 2006; Diaz and Rosenberg, 2008). Reducing agricultural nutrient loads requires collaboration between public and private entities to efficiently manage this diffuse pollution. Nutrient management plans such as the Chesapeake Bay Program (2014), the European Economic Community (EEC) (1991), and the Great Lakes Water Quality Agreement (GLWQA; International Joint Commission, 2012) have largely targeted agricultural sources. Despite substantial efforts to improve water quality, the response is often slower than desired, with legacy sources implicated as one reason for the lag in meeting desired water quality improvements (Sharpley et al., 2013; Melland et al., 2018; Van Meter et al., 2018). Further demonstrating the relevance and widespread nature of this problem, a study of 143 watersheds across the United States concluded that legacy sources contributed to riverine export of phosphorus (P) in 49 watersheds at an average rate of 86 kg P km−2, compared to 56 kg P km−2 in 94 watersheds where P was still being applied (Stackpoole et al., 2019).
One objective of the GLWQA is focused on reducing phosphorus loads since this nutrient supports the growth of cyanobacteria and algae in Lake Erie (International Joint Commission, 2012). In the Western Lake Erie Basin (WLEB), the single greatest contributor to P loads is the agriculturally-intensive Maumee River watershed (Maccoux et al., 2016). Separate studies estimated that legacy-P sources account for 34–66% (Stackpoole et al., 2019) or 39–49% (Kast et al., 2021) of the annual total P load discharged by the Maumee River. The GLWQA and subsequent action plans (Ohio Lake Erie Commission, 2018; United States Environmental Protection Agency, 2018) have established annual loading targets of 860 metric tons of total P and 186 metric tons of dissolved reactive P for the Maumee Basin—a 40% reduction from 2008 loads (Ohio Lake Erie Commission, 2018). Reducing legacy-P loads alone would make considerable progress toward meeting the stated water quality goals.
Legacy-P may persist for decades after its accumulation in the soil, masking water quality improvements as soil P reserves are gradually drawn down under revised nutrient application rates (Jarvie et al., 2013; Williams et al., 2015; Vollmer-Sanders et al., 2016; Liu et al., 2018). Recent reviews of soil testing laboratory data from the WLEB found that over 5% of soil samples in the region have a P concentration that exceeds 100 mg kg−1 soil (Williams et al., 2015; Dayton et al., 2020). This concentration is 2.5-fold greater than the maximum of 40 mg P kg−1 soil recommended by the Tri-State guidelines for corn and soybeans, which are the dominant crops in the WLEB (Culman et al., 2020a). Beyond this concentration, additional phosphorus application is not expected to improve agronomic yields (Culman et al., 2020a). This means that these fields will be a source of legacy-P as there is minimal fresh application of this nutrient from fertilizer inputs. Numerous studies have also linked elevated-P soils to disproportionately greater loads of P in runoff (e.g., Mcdowell et al., 2002; Vadas et al., 2005; Wang et al., 2012; Duncan et al., 2017). This means that elevated-P soils act as critical source areas of P loads within the watershed (Thomas et al., 2016). Modeling has suggested that targeting best management practices (BMPs) to these critical source areas will result in greater P reduction (Ghebremichael et al., 2013; Scavia et al., 2017; Teshager et al., 2017; Martin et al., 2021). This includes using a variety of in-field (e.g., cover crops, subsurface placement of fertilizer) and structural, edge-of-field practices (e.g., wetlands, riparian buffers) that capture, store, and treat surface and subsurface runoff. Locating the fields with elevated-P soils can therefore be expected to simultaneously (1) maximize the performance of these conservation measures by placing BMPs near sources of greater P loads, while (2) addressing legacy-P in the watershed.
Despite the knowledge that elevated-P fields exist, their locations are largely unknown because soil data are proprietary information. This creates an asymmetric information barrier to management programs, where the knowledge of the optimal fields that could benefit from BMPs is obscured to managers. Partnering with individuals and organizations who hold this knowledge can overcome this asymmetric information barrier. In the WLEB watershed, many farmers consult with agricultural retailers when designing and implementing nutrient management plans for their operations: nearly half of agricultural operators in the WLEB watershed rely on an agricultural retailer (also known as a nutrient service provider or crop consultant) to apply fertilizer (Prokup et al., 2017). Agricultural retailers also are responsible for soil testing thus possessing the asymmetric knowledge that could lead to locating elevated-P fields and thus targeted BMP implementation. Agricultural retailers often have more limited knowledge about BMPs that could be used to reduce edge-of-field nutrient losses, but because of their trusted relationships with farmers it has been suggested that they could become non-traditional actors promoting conservation activity with farmers (Vollmer-Sanders et al., 2016; Eanes et al., 2017). Partnering with agricultural retailers will allow for the identification of the elevated-P fields through shared data while enhancing the roles of retailers in promoting the adoption of BMPs by farmers.
Public-private partnership (PPPs) are arrangements between private and public entities in which resources, responsibilities, risks and rewards are shared between the partners in pursuit of a mutually agreed upon objective (Kwak et al., 2009). PPPs provide unique capabilities by combining the resources and authority available to the private and public partners, but at the cost of coordination and monitoring needed to ensure partners' alignment with the objective and performance of agreed upon tasks. While the majority of the literature on PPPs focuses on projects such as transportation and energy infrastructure (World Bank, 2017), several authors suggest or explore the use of PPPs to deliver environmental damage mitigation in agriculture (Calvo, 1998; Lawrence et al., 2014; Benson et al., 2018). The novelty of our contribution is the development of a PPP to specifically address the crucial informational asymmetry that impedes effective management of elevated-P fields. In our PPP, private partners contribute improved information concerning target fields and the public sector partner provides financial and technical resources to mitigate P losses from these fields.
As part of a publicly-funded grant, we formed a PPP in the WLEB with the objective to find 12 elevated-P fields to serve as research sites to target legacy-P loads. We worked with four agricultural retailers in this study who were willing to share deidentified soil test data and approach their farmer customers to participate in this study. In addition to guiding the selection of field sites, we used data provided by the agricultural retailers to increase knowledge about the extent and distribution of elevated-P soils across the basin. Specifically, we quantified the number of elevated-P zones within fields and then calculated the average soil test phosphorus (STP) for these fields. Additionally, we correlated STP concentrations with soil texture and other micronutrients that could help predict potential water quality impacts (Hanrahan et al., 2020a,b) and historical management activities (e.g., manure or biosolid application; Mantovi et al., 2003; Popovic et al., 2012). Following the initial contact by agricultural retailers with farmers, the research team evaluated the suitability of fields for BMPs and hydrological monitoring. This study demonstrates the value of forming public-private partnerships to identify and manage sites that act both as a source of legacy-P and disproportionately contribute to degradation of ecosystem services.
Materials and Methods
Formation of Public-Private Partnership
Our PPP was initiated by a research team from Ohio State University (OSU and Extension), the United States Department of Agriculture-Agricultural Research Service (USDA-ARS), and The Nature Conservancy (TNC). Early in 2017, we engaged the Ohio Agribusiness Association (OABA) and 4R Nutrient Stewardship Council with an interest in building a public-private partnership. The OABA is a trade association made up of businesses that serve Ohio agriculture and is the administrator of the 4R Nutrient Stewardship Certification Program for agricultural retailers (sometimes referred to as nutrient service providers) who follow established guidelines for fertilizer application (Vollmer-Sanders et al., 2016). The OABA introduced the project team to four 4R-certified agricultural retailers in the Maumee River watershed whom they thought would consider partnering on our project. These groups included an independent consulting group (consulting with farmers on 90 km2 in the WLEB), two farmer cooperatives (1,030–1,350 km2), and a national corporation (2,370 km2). These agricultural retailers agreed to join the partnership and to (1) share de-identified soil test data; (2) deliver and participate in regular surveys and interviews to evaluate the effectiveness of the partnership; and (3) serve as a point of contact between researchers and farmers to find participants for this study (Figure 1). For their participation, each agricultural retailer received an annual stipend (US$2,000) across the five years of the partnership.
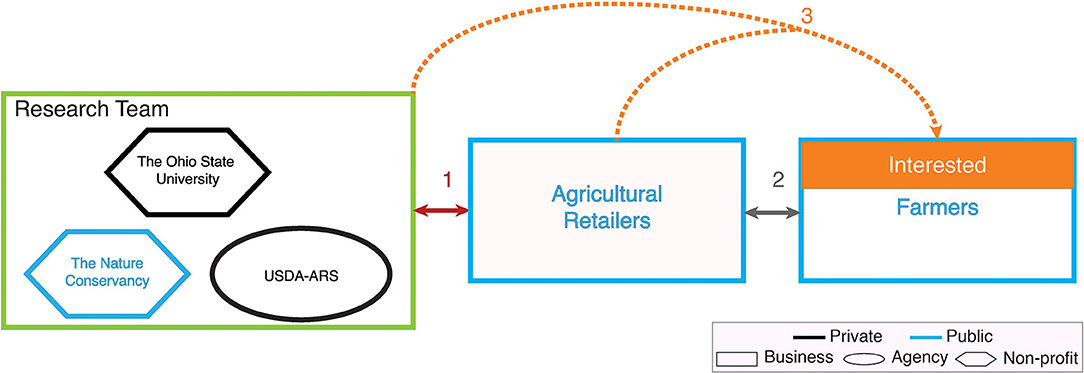
Figure 1. The structure of the Public-Private Partnership. This partnership involved a mixture of public/private businesses, agencies, and non-profit organization (see lower-right legend). The research team included representation from a public, governmental agency; a public, non-profit organization; and a private, non-profit organization. Each number represents the steps in the formation of our public-private partnership. (1) Three agricultural retailers agreed to share their soil test phosphorus data with the research team. (2) The research team then requested the retailers to each contact 25 farmers about their interest in participating with the research project returning information from those who were interested. (3) The research team evaluated only the fields of interested farmers (highlighted orange) and arranged meetings with them and the agricultural retailers to discuss their enrollment in the conservation and monitoring program.
Following the formation of the PPP with the four agricultural retailers, municipal-level legislation was passed granting guardianship rights to the citizens of the city of Toledo, Ohio. The resulting Lake Erie Bill of Rights (LEBoR) allowed citizens of Toledo to file lawsuits against Lake Erie polluters across jurisdictions (Henry, 2019). This ruling was subsequently challenged in and invalidated by the Ohio Supreme Court (Drewes Farms Partnership v. City of Toledo, Ohio, 2020). As a result, and despite their continued support and involvement in an advisory role in the project, one of the agricultural retailers withdrew their commitment to share data or contact farmers for the purpose of identifying research sites. Specifically, this retailer expressed concerns that their involvement could negatively impact their business. We continued the project with three agricultural retailers who participated fully in this study.
Collection of Data Used to Locate Elevated-P Fields
We set a threshold for elevated-P at a soil test phosphorus (STP) concentration of ≥100 mg P kg−1 soil, which is 2.5-fold the regional recommended agronomic STP concentration for corn and soybean (Culman et al., 2020a). Units for STP were expressed in equivalents of Mehlich-3P as most commercial soil testing laboratories in the region use this extraction method (Culman et al., 2020b). Previously, the Bray-P1 method was used in setting the Tri-State standards (Vitosh et al., 1995), and two of the partnered agricultural retailers still used Bray-P1 equivalents in their operations. To account for this, we multiplied the Bray P1 values by 1.35 to convert them into Mehlich-3P equivalents (Culman et al., 2020b).
The three agricultural retailers used different methods of sampling and analysis to evaluate STP levels in fields: composite, grid, or zone. In composite sampling, multiple soil cores are collected and composited prior to analysis resulting in a single STP concentration. For the other two approaches, multiple samples are taken from within the same field: gridded sampling used a standard-sized area, while zone sampling could be designed based on soil types, yields, or other field-specific data. For this study, we referred to each field area as a “zone.” We considered any agricultural field with at least one elevated-P zone to be a candidate for monitoring and management due to limited auxiliary knowledge about the field.
The data supplied by the three agricultural retailers (identified individually as AR_[1–3]) varied substantially. Specifically, AR_1 shared their most recent data (2016–2017) in a format that included a de-identified client identification number, field name, and zone with corresponding STP data. Likewise, AR_2 shared recent data (2015–2018) and included other geochemical analyses. Finally, AR_3 only shared their STP data for the elevated-P fields. Since AR_3 provided an incomplete dataset, it was excluded from an analysis of STP concentrations distributed across the basin. Some data provided by AR_1 and AR_2 included repeated measurements for a particular field, and in those cases only the most recent data was included for this analysis. Through discussions with the agricultural retailers, we learned some data referred to small vegetable gardens (n = 48) or local school district properties such as baseball and football fields (n = 17). We removed garden and schoolyard data, leaving a combined dataset that included 47,093 P measurements across 35,951 distinct agricultural fields (i.e., an average of 1.3 zones per field).
Ultimately, the objective of this initial data screening was to identify 25 farmers from each agricultural retailer so we could inquire about their willingness to participate in this study. In an iterative process, we started by selecting a list of fields, preferentially seeking those with the highest concentration and multiple zones with STP ≥100 mg kg−1 soil. Working with the agricultural retailers we arrived at a total of 77 current customers who manage elevated-P fields (25/26/26 farmers from AR_1/AR_2/AR_3, respectively). To get a better understanding of the fields managed by these 77 customers, we asked the agricultural retailers to complete a questionnaire asking for information on 106 fields managed by these farmers. We preferentially selected fields with the greatest STP concentrations and/or the greatest number of elevated-P zones. The requested information included: the operation size for the farmer (total acreage); geographic location; number of elevated-P fields managed; field size; adjacency of the field to open water; current fertilizer type; method of fertilizer application; who applied the fertilizer; soil type(s); tillage practice(s); and current/past land uses.
We then developed a survey to be delivered by the agricultural retailers to the 77 farmers managing elevated-P fields (Appendix A). We gave each agricultural retailer general instructions on how to deliver this survey, allowing for electronic or in-person discussions. We provided a brief explanation of our project followed by an inquiry to gauge the interest of the farmer on a scale of 1–5, with 1 being “not at all interested” and 5 being “extremely interested.” The survey also included questions pertaining to whether the fields in question were owned or rented; the farmer's participation in other conservation programs and practices; and a set of demographic characteristics. Farmers who responded with an interest of ≥3 (moderately, very, or extremely interested) were considered interested in participating in the research project. One exception involved a customer of one of the agricultural retailers who expressed interest in the project after attending an outreach event that mentioned our project.
We requested GIS information from the agricultural retailers on fields belonging to interested farmers; this inquiry yielded spatial information on 52 elevated-P fields. This included one field that was later selected as a research site that was not located through retailers. Rather, this field was brought to our attention by the owners of a field who had chosen to participate in our study. At least one field was selected from each of the interested farmers. Within the defined zones, we analyzed for relationships with STP concentrations between predominant soil types (United States Department of Agriculture, 2013) and other soil test data.
For the purpose of monitoring nutrient loads, we sought elevated-P fields that were hydrologically isolated with concentrated flow paths. The initial step in finding these isolated fields involved downloading LiDAR elevation data from the publicly available Ohio Statewide Imagery Program (OSIP) that was input into the Personal Computer Storm Water Management Model (PCSWMM; Computational Hydraulics International, version 7.2). We delineated hydrologic flow paths to screen for potential fields. We then followed up with the agricultural retailers to arrange a meeting involving our team of researchers and the farmers managing the fields in the presence of a representative from the retailer. This meeting allowed us to ground-truth the surface flow paths, but also allowed identification of subsurface drainage outlets on the sites. We further discussed with the farmers their willingness to allow us to implement and monitor the effects of BMPs on these sites with the goal of obtaining 12 new research sites.
Data Analysis
We analyzed soil chemistry data in R (version 3.6.0; R Core Team, 2019) with images created in the grammar of graphics, “ggplot2,” package (Wickham, 2016). We used the cumulative density function to evaluate the distribution of STP values and arithmetic mean concentrations across fields. Additionally, we calculated the 95% confidence interval for field average STP concentrations using the student's t-test to estimate the average field concentration as a function of the target concentration for elevated-P. Next, we analyzed the spatial data provided on the subset of 52 fields selected from farmers interested in participating in the study. We tested for linear relationships between STP and other geochemical data (n = 12) using the Pearson correlation coefficients to calculate the R2. P-values were adjusted using the Bonferroni method to detect statistical significance (α = 0.05). We used the post-hoc Kruskal-Nemenyi test to detect pairwise differences in STP concentrations by different soil textures using the”PMCMR” package (Pohlert, 2014).
Six ordinary-least-square (OLS) regression models were developed to analyze the relationship between field STP concentrations with the data collected about farmers and their operations through the agricultural retailers. While this analysis was not critical to identifying the fields for the study, it helps to answer the broader question about field and farm operator characteristics typically associated with elevated-P fields. The dependent variable for this analysis was the maximum reported STP concentration for any zone on each elevated-P field. The main independent variables were: the size of the farm operation (small, medium, large); whether or not the field is adjacent to an open water source; indicators for fertilizer application methods (broadcasting and starter, variable rate application, flat rate application, broadcasting only); indicators for past land use (livestock, crop, or unknown); whether or not manure application is practiced; whether or not the operation uses only inorganic fertilizer; whether or not the farmer practices conservation tillage; indicators for cover crops, crop rotation practices (corn/soybean or corn/soybean/wheat), or any other conservation practices; and the interest level of the farmer to participate in the program measured on a scale from 1 to 5 where 1 means not interested at all and 5 means extremely interested. As no farmer responded with an interest level of 2, we rescaled these values to 1–4 in these OLS models. We also included an indicator variable denoting if the field is in a county from the northwestern region of Ohio (Table S1).
The first model (1) included all variables collected through the questionnaire and survey. The second model (2) divided conservation tillage, previously an indicator value, into two categories: no-till and strip-till. The third model (3) converted the crop rotation to an indicator variable for whether wheat was included. The fourth model (4) converted the 1–5 scale measuring farmers' interest level to participate in a PPP into an indicator denoting whether (≥3) or not (1) farmers showed some interest. The fifth model (5) created an indicator variable for whether the land use had previously included livestock and another indicator for whether past land use included crops (with unknown past land use as the omitted category). In the final model (6) we omitted the indicator variable for the practice of manure application. All models included geographic area fixed effects (i.e., northwest Ohio region). We evaluated the models using corrected Akaike Information Criterion (AICC) selecting for the lowest value (Burnham and Anderson, 2002).
Results
Elevated-P Concentrations in the Western Lake Erie Basin
STP measurements obtained from two of the participating agricultural retailers ranged from 2–1,580 mg kg−1 soil (Figure 2A). While 34% of the data were above the agronomic recommended maximum of 40 mg P kg−1 soil, 3.8% of the data were above the elevated-P field threshold of 100 mg P kg−1 soil. Thirteen percent (926/6,970) of fields had at least one elevated-P zone. For 563 of these fields, though, there was only one elevated-P zone, and these areas often represented less than half of the number of zones sampled across the field (Figure 2B). Alternatively, 129 of the elevated-P fields had STP ≥100 mg kg−1 soil in every sampled zone. This included 75 fields that had multiple zones, while the other 54 consisted of a single soil sample. With these variations in STP concentrations across fields, elevated-P fields had an average STP concentration of 91 ± 4.9 mg P kg−1 soil (Figure S1). In selecting the farmers to engage in the search for research sites, we used this data to identify those operations that had the greatest STP concentrations and/or most zones of elevated-P.
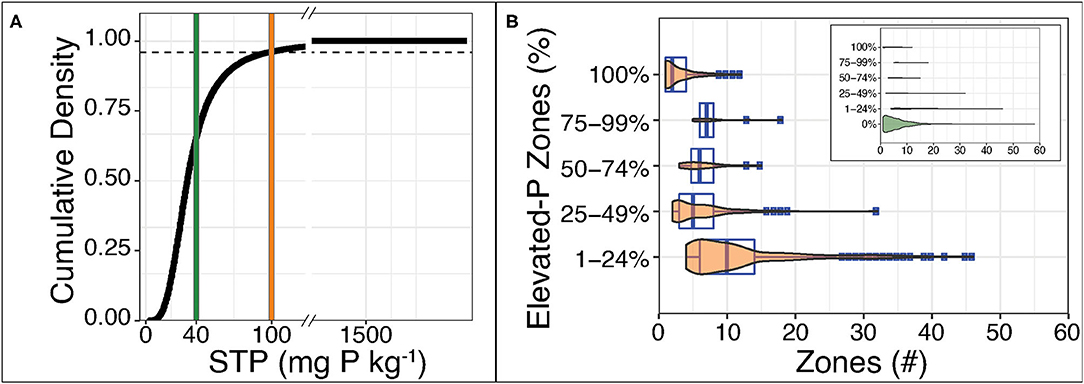
Figure 2. (A) Distribution of soil test phosphorus (STP) values (in Mehlich-3 equivalents) across fields in the combined data provided by AR_1 and AR_2. The black line shows the cumulative density of STP concentrations. Vertical lines highlight the concentrations for the recommended agronomic need (40 mg P kg−1 soil; green) and the threshold set for defining elevated-P fields in the region (100 mg P kg−1 soil; orange). (B) Percent of zones with elevated-P compared to the total number of zones on the field. Violins are scaled such that the area within each violin is representative of fields in that category. The inset graph includes the relative number of fields without any elevated-P zone. Violins are underlain by boxplots to show the median, interquartile range, and outliers.
Identifying Elevated-P Fields for BMP Implementation and Monitoring
Among the 77 farmers initially identified with elevated P fields, we received a positive response (≥ 3) from 32% of these farmers, reducing the potential number of study sites to ~170 fields and 25 interested farmers (Figure 3). Two of the agricultural retailers received interest from 10 farmers each (out of 25 or 26), while the other retailer only received interest from 5/26 farmers. One noteworthy difference between these three retailers was in how the research team communicated with them to deliver the survey. For the two agricultural retailers that received interest from 10 farmers, we had direct contact with the person who delivered the survey to the farmer. However, the research team had contact with only a division manager at the other retailer—with a corporate structure—and not with the crop consultants who delivered the survey to the farmers. This highlights a challenge of constructing and managing PPPs involving multiple private entities.
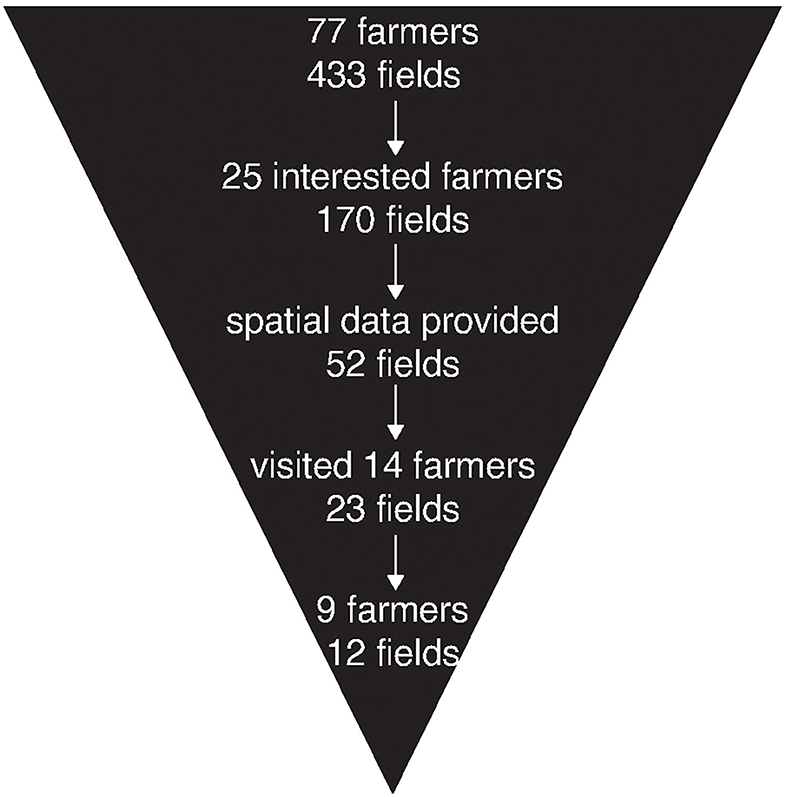
Figure 3. The process of finding elevated-P fields for BMP implementation and monitoring. Initially, 77 farmers were contacted to find interested participants. A selection of potential sites was chosen from the pool of interested farmers and spatial information was requested on these fields. Following a hydrological assessment using the spatial data, fields were visited to assess the suitability of a practice at each site. Ultimately, 12 fields were identified for monitoring and BMP implementation.
Of the 170 elevated-P fields with interested farmers, we requested spatial information on 52 fields so that they could be evaluated as candidates for in-person visits (Figure 4). From these 52 fields we determined that 32 were fit for surface water monitoring through our PCSWMM delineations. Specifically, 14 were preferable as candidates based on greater STP concentrations and isolated flow paths that crossed the elevated-P zone, while the other 18 were less suitable but still given consideration (e.g., Figure 4). Based upon the rankings by the research team, we visited 23 of these fields, at which point we had selected the 12 final fields for monitoring and BMP implementation.
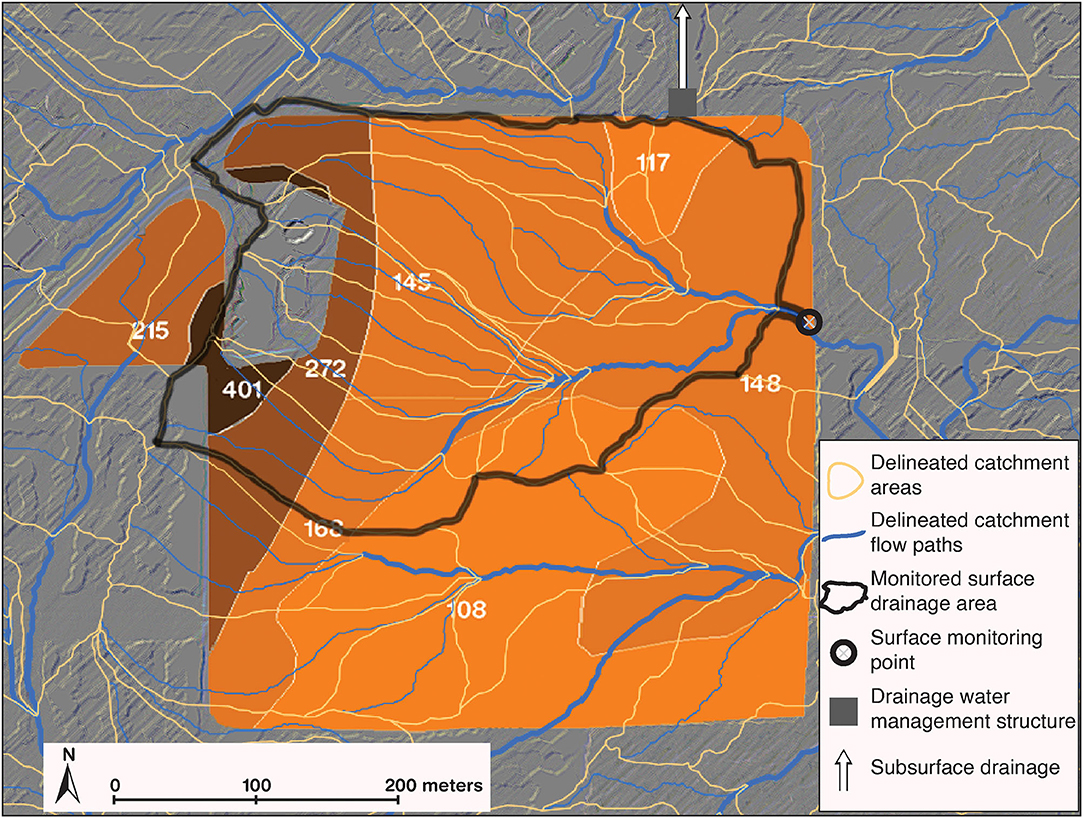
Figure 4. Surface water flow paths across a field (Site #2, Table 1) selected for this study. This site image depicts the isolated and concentrated hydrology sought for monitoring following the delineation of catchment areas and their respective flow paths (see legend in the bottom-right). Areas of the field are labeled and shaded according to the local STP concentration in units of mg P kg−1 soil.
In the quest to find 12 elevated-P fields for this study, site visits were necessary to confirm surface flow paths, ensure hydrologic isolation from other sources of water, and locate subsurface drainage outlets. Generally, two cases were presented: (1) the flow paths were confirmed through observation of erosion and/or farmer knowledge, or (2) no signs of erosion were present, and farmers stated they had not observed surface runoff in the past—this was common for highly permeable, sandy soils, which are present in many of the second cases. Site visits were also used to discuss and identify the location of subsurface drainage tiles on the sites. Of the 23 fields that were visited, 18 featured subsurface drainage tiles. Most of the farmers knew the location of tile outlets but only some possessed maps showing the locations of tiles. Selected sites were chosen based on the ability to install monitoring equipment to capture both surface and subsurface flow, when possible, with limited off-site contributions.
The final discussion between the farmers and research team was about which BMPs would be suitable for a particular field. We arranged visits with 14 farmers to discuss practices on their fields. Two of these farmers opted out of the research after discussing BMPs on their fields: one was considering selling their land for commercial development, while the other was not interested in additional conservation measures on a field already utilizing several. The PPP was able to successfully recruit nine farmers/land-owners who were willing to allow installation of new BMPs and subsequent monitoring on 12 elevated-P fields (Table 1). The three remaining farmers showed interest after meeting, but their fields were not selected as there were monitoring and/or sampling challenges at these locations. At five of the selected sites, no BMP was chosen in the initial stages, but monitoring equipment was installed to begin collecting data. These data will be used to inform the future management strategies to be adopted in the latter stages of the research, so that the most appropriate management action can be taken. Two sites have had drainage water management and monitoring equipment successfully installed. The remaining five sites have edge-of-field practices installed including two constructed wetlands and three subsurface-fed phosphorus filter beds.
Our selection process in collaboration with the agricultural retailers successfully identified 12 elevated-P fields for research sites with average field STP concentrations ranging from 76–401 mg P kg−1 soil. This equated to an average field STP concentration of 247 mg P kg−1. We were also successful in identifying sites with elevated-P zones that featured many different soil textures present in the region, with “clay” being the only soil texture that was not represented. For eight of these sites, the likely origin of elevated-P could be traced back to the application of manure or municipal biosolids as fertilizer, with one field having a history of livestock operations which were currently still present on site. Another site (#11) had been used for vegetable production in the past and may have received manure prior to a transfer in ownership to the current farmer. Site #6 had been previously used as an orchard with no known manure applications. The reasons for elevated-P on the remaining two sites remained unknown following our discussions with the farmers.
Characteristics of Elevated-P Fields
In addition to identifying the 12 elevated-P fields to be used as research sites, we identified field, farm, and farmer characteristics associated with elevated-P fields. The three agricultural retailers supplied geospatial and additional geochemical data on a selection of 52 fields from the farmers who expressed interest in adopting BMPs. Elevated-P was correlated (R2 > 0.16; p < 0.001) with the concentration of copper, iron, and zinc, in order from weakest to strongest correlation (Table 2). Additionally, there were significant correlations between S and Mn with P. All significant relationships were positive, meaning that the concentrations of these metals and sulfur were greater in the zones with greater STP.
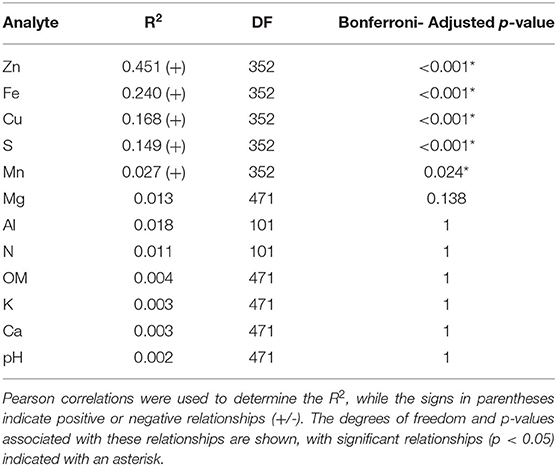
Table 2. Relationship between soil test phosphorus (STP) and soil chemistry data averaged across the selected 52 elevated-P fields from interested farmers.
There was also a statistically significant relationship between soil texture and STP concentrations on the 52 elevated-P fields. Generally, the greater P concentrations tended to be found in zones with sand or sandy loam textures (Figure 5). The three sandiest textures (“sand,” “loamy sand,” and “sandy loam”) accounted for 113 of the 188 elevated-P zones in the fields we analyzed. Of individual soil textures, though, silty clay loam zones were where the greatest number of elevated-P zones were identified (50). Proportionally only 23% of these “silty clay loam” zones had STP ≥ 100 mg kg−1 soil, while this was the case for over 75% of the “sand” zones. This means that in addition to having generally greater STP, these zones were also more frequently the elevated-P zones within these fields, though the reason for this relationship was outside the scope of this analysis.
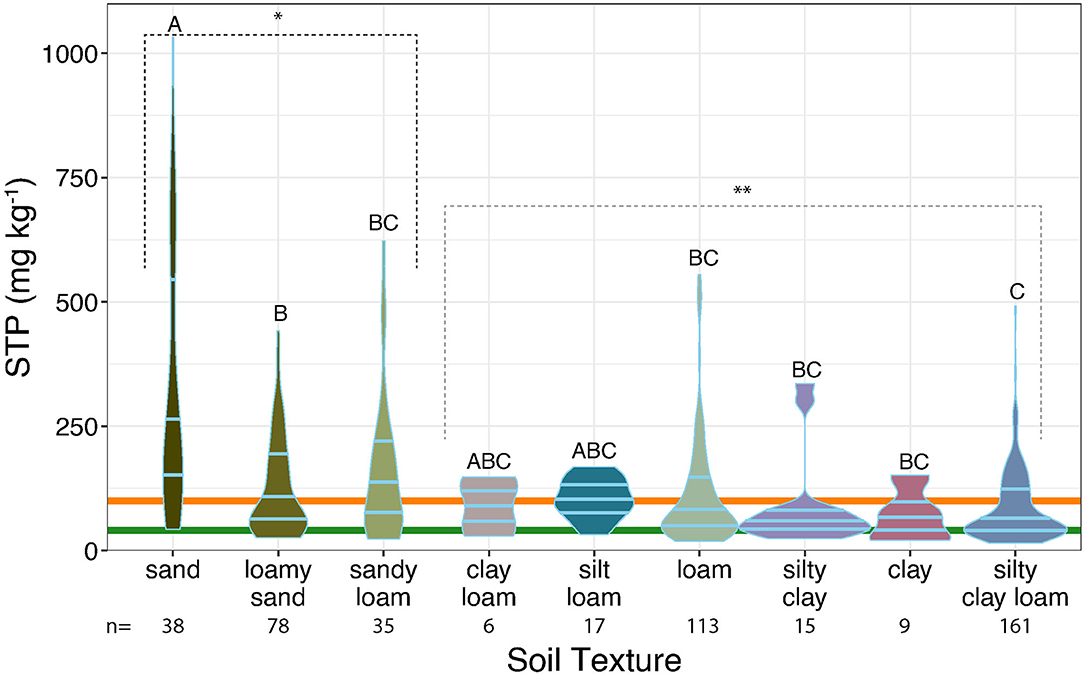
Figure 5. The distribution of STP concentrations by soil texture using violin plots on the selection of 52 elevated-P fields from interested farmers. The light blue lines correspond to the 25-50-75th quantiles. The number (n) of zones for each soil type is shown below the soil types. Groups were compared using the post-hoc Kruskal-Nemenyi test with letters above the violins representing significant differences for individual soil textures (α < 0.05). This test was also run for sandy against non-sandy soil textures as indicated by asterisks above the gray dashed-lines. Orange and green horizontal lines are placed at the 100 and 40 mg P kg−1 thresholds, respectively.
Of the six OLS models developed, we selected the 5th OLS regression model since it had the lowest AICC value (Table S2). This model revealed a positive correlation between maximum STP on a field and past livestock use, current use of only inorganic fertilizers, the use of cover crops, and conservation practices other than conservation tillage or cover crops such as small grains in rotation, filter strips, riparian buffers, and grassed waterways (Table 3). On the other hand, there was a negative correlation between maximum STP on a field, a past land use of solely commodity crops, and current common crop rotations (corn/soy or corn/soy/wheat). Importantly, there was also a negative correlation between maximum STP and interest in participating in this study. In other words, STP concentrations were higher on farms where livestock were present in the past or where the past land use was not just commodity crops, and where current practices involve only inorganic fertilizers, cover crops, atypical crop rotations (i.e., those including vegetables, alfalfa, or two consecutive years of soybeans), and conservation practices like small grains in rotation, filter strips, riparian buffers, and grassed waterways. In addition, farmers with greater STP concentrations were less likely to be interested in participating in our program.
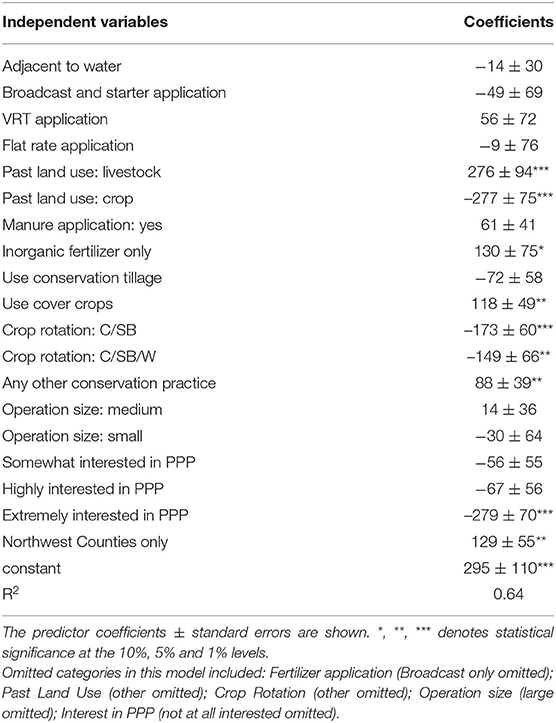
Table 3. Factors associated with max soil test phosphorus concentrations (mg STP kg−1 soil) on each field.
Discussion
Our PPP was successful in recruiting farmers operating on private, agricultural lands to participate in monitoring and conservation practice assessment to mitigate runoff from elevated-P fields. Frequently, conservation efforts involve the direct voluntary adoption of BMPs or conservation measures such as with the USDA National Resource Conservation Service's (NRCS) Wetland Reserve Program that has sought to restore wetland habitat on agricultural lands since its creation in the 1990 Farm Bill (P.L. 101-624; Benson et al., 2018). While farmer engagement in these voluntary government programs has had some success (e.g., Bosch et al., 1995), many have argued that not enough conservation is happening on private lands (Wilson et al., 2013; Osmond et al., 2019). Likewise, PPPs involving industrial entities, such as the National Forum on Non-point Source Pollution, have been effective at mitigating agricultural pollution using voluntary incentive programs sponsored by the private sector (Calvo, 1998). Examples from the National Forum included an educational program developed by the Coors Brewing Company to encourage the adoption of BMPs by barley growers, with monetary rewards given to the Future Farmers of America chapters for their role in recruiting farmers to install riparian buffers (Calvo, 1998). Within the WLEB, the 4R program is an example of another PPP that was able to make substantial progress in adoption (35% of acres enrolled within two years) by choosing to certify agricultural retailers rather than individual farmers (Vollmer-Sanders et al., 2016). In many ways, our PPP operated on a similar path as the 4R Nutrient Stewardship Certification program, but it faced different barriers due to its narrower scope. That is, the proportion of fields that could benefit from the array of 4R practices is relatively large compared to the proportion of fields with elevated-P concentrations which require mitigation through other means including edge-of-field practices. This makes identifying the small number of operators who are willing to consider mitigation of elevated-P fields a more precise task that is likely ill-suited to general mechanisms used to enroll farmers into other programs. Our PPP is a rare example of such a partnership that leveraged asymmetric information held by private entities to ensure resources were spent more efficiently. In other words, payments were made to the participating agricultural retailers to locate fields where we expect a return on this investment to be realized through greater load reductions.
Of the farmers with elevated-P fields who were contacted through the agricultural retailers, 32% were interested in participating in the study. This rate of response was more favorable than a regional effort to install wetlands in which only 1.5% of farmers responded to a mailed survey (Berkowitz et al., 2020). Our project likely benefitted from both the fact that retailers initiated the conversation with the farmers and the fact that we did not target a single BMP, especially wetlands which commonly require taking land out of production (Berkowitz et al., 2020). Through our experience, we found that we received greater interest when we worked directly with the agricultural retailers who contacted farmers to complete the initial survey. On the other hand, we did not have this interaction with the retailers from the corporate partner. With that group we explained our program to a division manager who sent the survey to branch offices to be dispersed to the farmers through the crop consultants working with each farmer. This resulted in identifying only five interested farmers compared to 10 from each of the other agricultural retailers, though these were not significantly different proportions based on our limited sample sizes (n = 25/26/26). These results indicate that working more closely with agricultural retailers, who are entities trusted by farmers (Lawrence et al., 2014; Enloe et al., 2017), may aid in recruiting volunteers.
While we designed our PPP to maintain the privacy of individual farmers (all shared data were de-identified until an individual farmer expressed interest), this remained a concern throughout the process. One of the agricultural retailers mentioned that several farmers they contacted were concerned about how their involvement could expose them to potential lawsuits. This concern may have arisen in part due to the passage of the Lake Erie Bill of Rights (LEBoR) around the time agricultural retailers were contacting farmers (Henry, 2019). In fact, one of the retailers withdrew their commitment to share their data explicitly due to the LEBoR. This agricultural retailer was worried that their involvement in the PPP could damage business if farmers became aware of their involvement. We suspect that similar concerns about privacy were a factor in the interest level of farmers and led to the negative correlation between this interest and the STP concentrations of fields. In other words, farmers with greater STP concentrations were more concerned with being involved in the project that could potentially expose their identity. As a public entity, the research team at OSU evaluated concerns about public records requests early in the process of identifying sites, and we felt that there were sufficient legal arguments that identifying information shared confidentially would not be subject to these requests. In the case that a similar PPP be developed, it will be important to consider the manner in which identifying information shared with public entities can be protected from the Freedom of Information Act (5 U.S.C. § 552) or other public records requests (ORC 149.43) to preserve participating farmer anonymity.
The data supplied by the agricultural retailers in our partnership had a lesser percentage of elevated-P samples (3.8% vs. >5%) compared to aggregate data provided by the soil testing laboratories in the region (Dayton et al., 2020). One potential explanation for this was the fact that we worked with 4R-certified agricultural retailers who were less likely to over-apply nutrients on these fields. Working through the agricultural retailers again provided a unique benefit to this research as it revealed that these elevated-P zones were often isolated areas within a field. Specifically, 13% of the fields had at least one zone of elevated-P. This means that management would need to be extended to a greater number of fields and may need to be tailored to treat the runoff coming from these specific areas. In our process to find research sites, though, one of the challenges was finding isolated surface flow paths across elevated-P zones or having precise knowledge of the subsurface tile network making it difficult to intercept the runoff from these elevated-P zones. While this posed a challenge for monitoring, dispersed flow across the field also affected the selection of BMPs. Some practices are better suited to be applied across whole fields and not limited to isolated areas (e.g., cover crops, gypsum application) making them more ideal for where water flows in multiple directions or where there is more than one discharge point on the field. An adaptive approach that considers these factors is necessary for these conservation efforts where edge-of-field BMPs may be more appropriate for fields with consolidated flow paths while in-field practices could be implemented more generally.
The analysis of spatial field data and survey data provided by the agricultural retailers about the farmer and their operation revealed important information about the history of elevated-P fields and some characteristics that could influence the rate of P-loss. With cover crops, for example, studies have shown that P is moved from the subsurface to the surface soil layer which could explain why this practice was associated with elevated-P in our study (Soltangheisi et al., 2020). Alternatively, farmers who were concerned that P concentrations were too high in their fields may have been more likely to plant cover crops or engage in other conservation practices, leading to the positive correlation. It was also apparent that a history of livestock and, to a lesser extent, manure application was correlated with maximum STP concentrations measured within a single zone of the field. Notably, eight of our selected research sites had a known history of livestock, manure application, or biosolid application on the fields. Further supporting the relationship between manure application and elevated-P were its correlation with heavy metals (Zn, Fe, Cu) and sandier soil types. Agricultural soils are known to become enriched with these metals following manure applications (Mantovi et al., 2003; Popovic et al., 2012), while historic application of manure to make sandy soils more productive has been associated with legacy-P loading in northern Europe (Chardon and Schoumans, 2007). Anecdotally, a few farmers and agricultural retailers mentioned how sandy soils were better suited to historic manure applications as they dried out more quickly in the winter or spring that allowed more frequent, historic applications.
The association of elevated-P with sandier soil types may also have implications for the transport of this nutrient into drainage from these fields. There is a common expectation that clay has a high capacity for P sorption (Mcdowell et al., 2002; Arai and Livi, 2013; Laakso et al., 2017; Xu et al., 2020). While some cases have demonstrated lesser P concentrations in discharge from clay soils (Mcdowell et al., 2002), others have shown the opposite to be true (van Es et al., 2004; Xu et al., 2020). Additionally, a desorption experiment revealed that the sand portion—separated from its parent soil—released P at a slower rate, which was attributed to aluminosilicate surfaces on the sand particles (Arai and Livi, 2013). Al, Fe, Ca, and organic matter have all been indicated as soil components that limit P-losses from soils (Mcdowell et al., 2002; Hanrahan et al., 2020b; Xu et al., 2020). However, in our data, we found no correlation between the concentration of any of these elements and STP across the elevated-P fields with the exception of iron (Fe). This means that these elements were unlikely to have played a role in the development of elevated-P soils, but they may still become more important as water quality is monitored from these sites. One of the more interesting opportunities with the water quality data will be to explore the relationship between P loads and sand/silt/clay content as the research sites selected from the PPP included elevated-P soils across a variety of soil types.
Several different practices were selected for the research sites in the PPP so that the water quality monitoring can be used to guide the selection of BMPs best suited to reduce P runoff from for elevated-P fields. There were concerns about drainage water management (DWM) on elevated-P fields as research has shown that this practice can lead to increased P loads, although this varied seasonally and annually (Gilliam and Skaggs, 1986; Valero et al., 2007; Saadat et al., 2018). Despite a lack of certainty with its effects on P-loads, DWM also has the potential to reduce nitrogen and increase agricultural productivity making it a desirable practice for farmers (Gilliam and Skaggs, 1986; Pease et al., 2018). There were similar concerns about building wetlands on these sites with elevated-P soils as these systems may also cause the release of P under anaerobic conditions (Land et al., 2016). For this project, the upper-most soils were removed to reduce the risk of P leaching after wetland construction and flooding. Over time, there may be diminishing returns from wetlands as the sediment becomes saturated with P (Mitsch et al., 2012). P-filters were another intriguing system within the context of this project. These systems are especially efficient in reducing P—a steel-slag filter at one of the USDA-ARS sites reduced as much as 55% of the dissolved P (Penn et al., 2020). The three systems designed in this study appealed to the farmers as their size did not reduce the amount of arable land or interfere with standard operations on the field. Much like with wetlands, these systems are expected to yield diminishing returns over time (Qin et al., 2018). Part of the research with the new sites will include using new materials and different configurations to improve the design of P-filters. At five sites, only monitoring has been established to allow data collection and analysis to determine what practice would be most suitable for each site. The edge-of-field BMPs already selected are still an option though other in-field BMPs will be considered (e.g., cover crops [NRCS #340] or gypsum application [NRCS #333]). Across all sites, management was funded by the research project with no cost to the farmers contributing to their interest in participating.
Expansion or replication of our PPP will require new sources of funding so that a greater number of elevated-P sites can receive management. Additional efforts to identify who might be more interested in participating in future partnerships revealed no significant differences when contrasting the uninterested vs. interested farmers on characteristics such as farm size, total number of elevated-P fields, maximum STP value, type of rotation, or whether a cover crop was being used on the field of interest. Our research will continue to explore the best way to carry this partnership forward by fostering the relationship between agricultural retailers and environmental managers in the watershed. Our research intends to address a gap in knowledge about the sustainability of PPPs in the area of conservation (Pinz et al., 2018). We expect the results of our continued research to show that the cost of obtaining asymmetric information by including agricultural retailers is outweighed by the benefits realized through enhanced P-load reductions by locating these practices on or near elevated-P fields.
Legacy nutrients can delay the success of water quality initiatives, but finding these sources has been challenging (Jarvie et al., 2013; Sharpley et al., 2013; Powers et al., 2016). We were able to overcome this barrier by partnering with agricultural retailers who found farmers who were willing to adopt conservation practices on elevated-P fields. As documented, these sites had developed the elevated-P concentrations due to past management and land-use, considering minimal new fertilizer (starter was applied on the sites). Therefore, our sites are expected to be a source of legacy-P discharged at disproportionately greater concentrations (Vadas et al., 2005; King et al., 2018). Our endeavors identified many elevated-P fields suitable for conservation practices with farmers willing to adopt them beyond the 12 research sites selected for our study. This process demonstrated that public-private partnerships that include agricultural retailers are a unique tool to help locate and treat the sources of disproportionate and legacy-P loads that impair water quality around the world.
Data Availability Statement
The raw data supporting the conclusions of this article will be made available by the authors, without undue reservation.
Ethics Statement
The studies involving human participants were reviewed and approved by the Ohio State University Office of Responsible Research Practices. Written informed consent for participation was not required for this study in accordance with the national legislation and the institutional requirements. Written informed consent was not obtained from the individual(s) for the publication of any potentially identifiable images or data included in this article.
Author Contributions
JD'A, MK, KWK, GL, BER, RSW, RJW, and JFM conceived of and designed the research. MRB, NDS, MMLJ, CS, TP, ERS, and JFM acquired and analyzed data. MRB and JFM wrote and edited the manuscript. TP, ERS, RSW, and BER assisted in writing sections of this manuscript. All authors reviewed, contributed, and approved the submitted version of this article.
Funding
Funding was provided through a USDA National Institute of Food and Agricultural Grant #2018-68011-28370.
Conflict of Interest
The authors declare that the research was conducted in the absence of any commercial or financial relationships that could be construed as a potential conflict of interest.
Publisher's Note
All claims expressed in this article are solely those of the authors and do not necessarily represent those of their affiliated organizations, or those of the publisher, the editors and the reviewers. Any product that may be evaluated in this article, or claim that may be made by its manufacturer, is not guaranteed or endorsed by the publisher.
Acknowledgments
We would like to thank the Ohio Agribusiness Association for supporting this project, especially identifying the four agricultural retailers that are included in the PPP. We would like the recognize that Hugh Walpole assisted in the preliminary analysis of data collected about farmers and their operations through the agricultural retailers. We would also like to thank Chad Penn and Stan Livingston for their help in designing and installing the phosphorus filter systems in this project.
Supplementary Material
The Supplementary Material for this article can be found online at: https://www.frontiersin.org/articles/10.3389/fsufs.2021.742817/full#supplementary-material
References
Arai, Y., and Livi, K. J. (2013). Underassessed phosphorus fixation mechanisms in soil sand fraction. Geoderma 192, 422–429. doi: 10.1016/j.geoderma.2012.06.021
Benson, C. E., Carberry, B., and Langen, T. A. (2018). Public–private partnership wetland restoration programs benefit species of greatest conservation need and other wetland-associated wildlife. Wetlands Ecol. Manage. 26, 195–211. doi: 10.1007/s11273-017-9565-8
Berkowitz, J. F., Schlea, D. A., VanZomeren, C. M., and Boles, C. M. (2020). Coupling watershed modeling, public engagement, and soil analysis improves decision making for targeting P retention wetland locations. J. Great Lakes Res. 46, 1331–1339. doi: 10.1016/j.jglr.2020.06.016
Bosch, D. J., Cook, Z. L., and Fuglie, K. O. (1995). Voluntary versus mandatory agricultural policies to protect water quality: adoption of nitrogen testing in Nebraska. Appl. Econ. Perspect. Policy 17, 13–24. doi: 10.2307/1349651
Burnham, K. P., and Anderson, D. R. (2002). A Practical Information-Theoretic Approach. New York, NY: Springer.
Calvo, G. (1998). Voluntary public-private nonpoint source pollution projects: a welcome response to regulatory shortcomings under the Clean Water Act. Great Plains Nat. Resources J. 3:159.
Chardon, W. J., and Schoumans, O. F. (2007). Soil texture effects on the transport of phosphorus from agricultural land in river deltas of Northern Belgium, The Netherlands and North-West Germany. Soil Use Manage. 23, 16–24. doi: 10.1111/j.1475-2743.2007.00108.x
Chesapeake Bay Program (2014). Chesapeake Watershed Agreement. Available online at: https://www.chesapeakebay.net/documents/FINAL_Ches_Bay_Watershed_Agreement.withsignatures-HIres.pdf (accessed September 20, 2021).
Culman, S., Fulford, A., Camberato, J., and Steinkey, K. (2020a). Tri-State Fertilizer Recommendations. Bulletin 974. Columbus, OH: College of Food, Agricultural, and Environmental Sciences; The Ohio State University. Available online at: https://extensionpubs.osu.edu/tri-state-fertilizer-recommendations-for-corn-soybean-wheat-and-alfalfa/ (accessed November 17, 2020).
Culman, S. W., Mann, M., Sharma, S., Saeed, M. T., Fulford, A. M., Lindsey, L. E., et al. (2020b). Calibration of Mehlich-3 with Bray P1 and ammonium acetate in the Tri-State Region of Ohio, Indiana and Michigan. Commun. Soil Sci. Plant Anal. 51, 86–97. doi: 10.1080/00103624.2019.1695825
Dayton, E. A., Shrestha, R. K., Fulford, A. M., Love, K. R. W., Culman, S., and Lindsey, L. E. (2020). Soil test phosphorus and phosphorus balance trends: a county-level analysis in Ohio. Agron. J. 1, 1617–1624. doi: 10.1002/agj2.20146
Diaz, R. J., and Rosenberg, R. (2008). Spreading dead zones and consequences for marine ecosystems. Science 321, 926–929. doi: 10.1126/science.1156401
Drewes Farms Partnership v. City of Toledo, Ohio. (2020). 441 F. Supp. 3d 551. United States District Court, Northern District of Ohio, Western Division. Available online at: https://casetext.com/case/drewes-farms-pship-v-city-of-toledo
Duncan, E. W., King, K. W., Williams, M. R., LaBarge, G., Pease, L. A., Smith, D. R., et al. (2017). Linking soil phosphorus to dissolved phosphorus losses in the Midwest. Agric. Environ. Lett. 2, 1–5. doi: 10.2134/ael2017.02.0004
Eanes, F. R., Singh, A. S., Bulla, B. R., Ranjan, P., Prokopy, L. S., Fales, M., et al. (2017). Midwestern US farmers perceive crop advisers as conduits of information on agricultural conservation practices. Environ. Manage. 60, 974–988. doi: 10.1007/s00267-017-0927-z
Enloe, S. K., Schulte, L. A., and Tyndall, J. C. (2017). Public–private partnerships working beyond scale challenges toward water quality improvements from private lands. Environ. Manage. 60, 574–587. doi: 10.1007/s00267-017-0905-5
European Economic Community (EEC). (1991). Council Directive Concerning the Protection of Waters Against Pollution Caused by Nitrates From Agricultural Sources. Official Journal of the European Communities, 91/676/EEC.
Ghebremichael, L. T., Veith, T. L., and Hamlett, J. M. (2013). Integrated watershed-and farm-scale modeling framework for targeting critical source areas while maintaining farm economic viability. J. Environ. Manage. 114, 381–394. doi: 10.1016/j.jenvman.2012.10.034
Gilliam, J. W., and Skaggs, R. W. (1986). Controlled agricultural drainage to maintain water quality. J. Irrig. Drain. Eng. 112, 254–263. doi: 10.1061/(ASCE)0733-9437(1986)112:3(254)
Hanrahan, B., King, K. W., and Williams, M. R. (2020b). Controls on subsurface nitrate and dissolved reactive phosphorus losses from agricultural fields during precipitation-driven events. Sci. Total Environ. 754:142047. doi: 10.1016/j.scitotenv.2020.142047
Hanrahan, B. R., King, K. W., Macrae, M. L., Williams, M. R., and Stinner, J. H. (2020a). Among-site variability in environmental and management characteristics: Effect on nutrient loss in agricultural tile drainage. J. Great Lakes Res. 46, 486–499. doi: 10.1016/j.jglr.2020.02.004
Henry, T. (2019). Lake Erie Bill of Rights Gets Approval from Toledo Voters. Toledo Blade. Available online at: www.toledoblade.com/local/politics/2019/02/26/Lake-Erie-Bill-of-Rights-gets-approval-from-Toledo-voters/stories/20190226159 (accessed February 27, 2019).
International Joint Commission (2012). Great Lakes Water Quality Agreement. Available online at: https://binational.net//wp-content/uploads/2014/05/1094_Canada-USA-GLWQA-_e.pdf (accessed February 1, 2019).
Jarvie, H. P., Sharpley, A. N., Spears, B., Buda, A. R., May, L., and Kleinman, P. J. (2013). Water quality remediation faces unprecedented challenges from “legacy phosphorus.” Environ. Sci. Technol. 47, 8997–8998. doi: 10.1021/es403160a
Kast, J. B., Apostel, A. M., Kalcic, M. M., Muenich, R. L., Dagnew, A., Long, C. M., et al. (2021). Source contribution to phosphorus loads from the Maumee River watershed to Lake Erie. J. Environ. Manage. 279:111803. doi: 10.1016/j.jenvman.2020.111803
King, K. W., Williams, M. R., LaBarge, G. A., Smith, D. R., Reutter, J. M., Duncan, E. W., et al. (2018). Addressing agricultural phosphorus loss in artificially drained landscapes with 4R nutrient management practices. J. Soil Water Conserv. 73, 35–47. doi: 10.2489/jswc.73.1.35
Kwak, Y. H., Chih, Y., and Ibbs, C. W. (2009). Towards a comprehensive understanding of public private partnerships for infrastructure development. Calif. Manage. Rev. 51, 51–78. doi: 10.2307/41166480
Laakso, J., Uusitalo, R., Leppänen, J., and Yli-Halla, M. (2017). Sediment from agricultural constructed wetland immobilizes soil phosphorus. J. Environ. Qual. 46, 356–363. doi: 10.2134/jeq2016.09.0336
Land, M., Granéli, W., Grimvall, A., Hoffmann, C. C., Mitsch, W. J., Tonderski, K. S., et al. (2016). How effective are created or restored freshwater wetlands for nitrogen and phosphorus removal? A systematic review. Environ. Evid. 5:9. doi: 10.1186/s13750-016-0060-0
Lawrence, D. J., Moody, L., and Clearfield, F. B. (2014). A public-private partnership to enhance the voluntary approach to nutrient stewardship. J. Soil Water Conserv. 69, 36A−38A. doi: 10.2489/jswc.69.2.36A
Liu, J., Cade-Menun, B., Yang, J., Hu, Y., Liu, C., Tremblay, J., et al. (2018). Long-term land use affects phosphorus speciation and the composition of phosphorus cycling genes in agricultural soils. Front. Microbiol. 9:1643. doi: 10.3389/fmicb.2018.01643
Maccoux, M. J., Dove, A., Backus, S. M., and Dolan, D. M. (2016). Total and soluble reactive phosphorus loadings to Lake Erie: a detailed accounting by year, basin, country, and tributary. J. Great Lakes Res. 42, 1151–1165. doi: 10.1016/j.jglr.2016.08.005
Mantovi, P., Bonazzi, G., Maestri, E., and Marmiroli, N. (2003). Accumulation of copper and zinc from liquid manure in agricultural soils and crop plants. Plant Soil. 250, 249–257. doi: 10.1023/A:1022848131043
Martin, J. F., Kalcic, M. M., Aloysius, N., Apostel, A. M., Brooker, M. R., Evenson, G., et al. (2021). Evaluating management options to reduce Lake Erie algal blooms using an ensemble of watershed models. J. Environ. Manage. 280:111710. doi: 10.1016/j.jenvman.2020.111710
Mcdowell, R., Sharpley, A., and Withers, P. (2002). Indicator to predict the movement of phosphorus from soil to subsurface flow. Environ. Sci. Technol. 36, 1505–1509. doi: 10.1021/es015675w
Melland, A. R., Fenton, O., and Jordan, P. (2018). Effects of agricultural land management changes on surface water quality: a review of meso-scale catchment research. Environ Sci Policy 84, 19–25. doi: 10.1016/j.envsci.2018.02.011
Mitsch, W. J., Zhang, L., Stefanik, K. C., Nahlik, A. M., Anderson, C. J., Bernal, B., et al. (2012). Creating wetlands: primary succession, water quality changes, and self-design over 15 years. Bioscience 62, 237–250. doi: 10.1525/bio.2012.62.3.5
Ohio Lake Erie Commission (2018). State of Ohio's Domestic Action Plan. Available online at: https://lakeerie.ohio.gov/Portals/0/Ohio%20DAP/DAP%201-1%20FINAL%202018-08-27.pdf (accessed February 1, 2019).
Osmond, D. L., Shober, A. L., Sharpley, A. N., Duncan, E. W., and Hoag, D. (2019). Increasing the effectiveness and adoption of agricultural phosphorus management strategies to minimize water quality impairment. J. Environ. Qual. 48, 1204–1217. doi: 10.2134/jeq2019.03.0114
Pease, L. A., Fausey, N. R., Martin, J. F., and Brown, L. C. (2018). Weather, landscape, and management effects on nitrate and soluble phosphorus concentrations in subsurface drainage in the western Lake Erie basin. Trans. ASABE 61, 223–232. doi: 10.13031/trans.12287
Penn, C., Livingston, S., Shedekar, V., King, K., and Williams, M. (2020). Performance of field-scale phosphorus removal structures utilizing steel slag for treatment of surface and subsurface drainage. Water 12:443. doi: 10.3390/w12020443
Pinz, A., Roudyani, N., and Thaler, J. (2018). Public–private partnerships as instruments to achieve sustainability-related objectives: the state of the art and a research agenda. Public Manage. Rev. 20, 1–22. doi: 10.1080/14719037.2017.1293143
Pohlert, T. (2014). The Pairwise Multiple Comparison of Mean Ranks Package (PMCMR). Version 1. Available online at: https://CRAN.R-project.org/package=PMCMR (accessed December, 19, 2019).
Popovic, O., Hjorth, M., and Stoumann Jensen, L. (2012). Phosphorus, copper and zinc in solid and liquid fractions from full-scale and laboratory-separated pig slurry. Environ. Technol. 33, 2119–2131. doi: 10.1080/09593330.2012.660649
Powers, S. M., Bruulsema, T. W., Burt, T. P., Chan, N. I., Elser, J. J., Haygarth, P. M., et al. (2016). Long-term accumulation and transport of anthropogenic phosphorus in three river basins. Nat. Geosci. 9:353. doi: 10.1038/ngeo2693
Prokup, A., Wilson, R., Zubko, C., Heeren, A., and Roe, B. (2017). 4R Nutrient Stewardship in the Western Lake Erie Basin. Columbus, OH: The Ohio State University, School of Environment and Natural Resources. Available online at: https://4rcertified.org/wp-content/uploads/2017/02/Prokup.etal_.2017.4R-Nutrient-Stewardship-in-the-western-Lake-Erie-basin.pdf (accessed June 30, 2021).
Qin, Z., Shober, A. L., Scheckel, K. G., Penn, C. J., and Turner, K. C. (2018). Mechanisms of phosphorus removal by phosphorus sorbing materials. J. Environ. Qual. 47, 1232–1241. doi: 10.2134/jeq2018.02.0064
R Core Team (2019). R: A Language and Environment for Statistical Computing. Vienna: R Foundation for Statistical Computing. Available online at: https://www.R-project.org/ (accessed December 19, 2019).
Saadat, S., Bowling, L., Frankenberger, J., and Kladivko, E. (2018). Nitrate and phosphorus transport through subsurface drains under free and controlled drainage. Water Res. 142, 196–207. doi: 10.1016/j.watres.2018.05.040
Scavia, D., Kalcic, M., Muenich, R. L., Read, J., Aloysius, N., Bertani, I., et al. (2017). Multiple models guide strategies for agricultural nutrient reductions. Front. Ecol. Environ. 15, 126–132. doi: 10.1002/fee.1472
Sharpley, A., Jarvie, H. P., Buda, A., May, L., Spears, B., and Kleinman, P. (2013). Phosphorus legacy: overcoming the effects of past management practices to mitigate future water quality impairment. J. Environ. Qual. 42, 1308–1326. doi: 10.2134/jeq2013.03.0098
Smith, V. H., Joye, S. B., and Howarth, R. W. (2006). Eutrophication of freshwater and marine ecosystems. Limnol. Oceanogr. 51, 351–355. doi: 10.4319/lo.2006.51.1_part_2.0351
Soltangheisi, A., Teles, A. P. B., Sartor, L. R., and Pavinato, P. S. (2020). Cover cropping may alter legacy phosphorus dynamics under long-term fertilizer addition. Front. Environ. Sci. 8:13. doi: 10.3389/fenvs.2020.00013
Stackpoole, S. M., Stets, E. G., and Sprague, L. A. (2019). Variable impacts of contemporary versus legacy agricultural phosphorus on US river water quality. PNAS 116, 20562–20567. doi: 10.1073/pnas.1903226116
Teshager, A. D., Gassman, P. W., Secchi, S., and Schoof, J. T. (2017). Simulation of targeted pollutant-mitigation-strategies to reduce nitrate and sediment hotspots in agricultural watershed. Sci. Total Environ. 607–608, 1188–1200. doi: 10.1016/j.scitotenv.2017.07.048
Thomas, I. A., Mellander, P.-., Murphy, P. N. C., Fenton, O., Shine, O., Djodjic, F., et al. (2016). A sub-field scale critical source area index for legacy phosphorus management using high resolution data. Agric. Ecosyst. Environ. 233, 238–252. doi: 10.1016/j.agee.2016.09.012
United States Department of Agriculture (2013). Web Soil Survey. Soil Survey Staff. Washington, DC: Natural Resources Conservation Service. Available online at: http://websoilsurvey.sc.egov.usda.gov/ (accessed July 31, 2019).
United States Environmental Protection Agency (2018). US Action Plan for Lake Erie. Available online at: https://www.epa.gov/sites/production/files/2018-03/documents/us_dap_final_march_1.pdf (accessed February 1, 2019).
Vadas, P. A., Kleinman, P. J., Sharpley, A. N., and Turner, B. L. (2005). Relating soil phosphorus to dissolved phosphorus in runoff: a single extraction coefficient for water quality modeling. J. Environ. Qual. 34, 572–580. doi: 10.2134/jeq2005.0572
Valero, C. S., Madramootoo, C. A., and Stämpfli, N. (2007). Water table management impacts on phosphorus loads in tile drainage. Agric. Water Manage. 89, 71–80. doi: 10.1016/j.agwat.2006.12.007
van Es, H. M., Schindelbeck, R. R., and Jokela, W. E. (2004). Effect of manure application timing, crop, and soil type on phosphorus leaching. J. Environ. Qual. 33, 1070–1080. doi: 10.2134/jeq2004.1070a
Van Meter, K. J., Van Cappellen, P., and Basu, N. B. (2018). Legacy nitrogen may prevent achievement of water quality goals in the Gulf of Mexico. Science 360:eaar4462. doi: 10.1126/science.aar4462
Vitosh, M. L., Johnson, J. W., and Mengel, D. B. (1995). Tri-state Fertilizer Recommendations for Corn, Soybeans, Wheat and Alfalfa. E-2567. East Lansing, MI: Michigan State University Extension. Available online at: https://archive.lib.msu.edu/DMC/Ag.%20Ext.%202007-Chelsie/PDF/e2567.pdf (accessed November 17, 2020).
Vollmer-Sanders, C., Allman, A., Busdeker, D., Moody, L. B., and Stanley, W. G. (2016). Building partnerships to scale up conservation: 4R Nutrient Stewardship Certification Program in the Lake Erie watershed. J. Great Lakes Res. 42, 1395–1402. doi: 10.1016/j.jglr.2016.09.004
Wang, Y. T., Zhang, T. Q., O'Halloran, I. P., Tan, C. S., Hu, Q. C., and Reid, D. K. (2012). Soil tests as risk indicators for leaching of dissolved phosphorus from agricultural soils in Ontario. Soil Sci. Soc. Am. J. 76, 220–229. doi: 10.2136/sssaj2011.0175
Wickham, H. (2016). ggplot2: Elegant Graphics for Data Analysis. Version 3.2. Available online at: https://ggplot2.tidyverse.org (accessed December 19, 2019).
Williams, M. R., King, K. W., Dayton, E., and LaBarge, G. A. (2015). Sensitivity analysis of the Ohio phosphorus risk index. Trans. ASABE 58, 93–102. doi: 10.13031/trans.58.10778
Wilson, R., Burnett, L., Ritter, T., Roe, B., and Howard, G. (2013). Farmers, Phosphorus and Water Quality: A Descriptive Report of Beliefs, Attitudes and Practices in the Maumee Watershed of Northwest Ohio. Columbus, OH: The Ohio State University, School of Environment and Natural Resources.
World Bank (2017). Public-Private Partnerships: Reference Guide Version 3. Washington, DC: World Bank.
Keywords: eutrophication, Lake Erie, conservation, edge-of-field, agricultural retailers, soil test phosphorus, asymmetric information barrier
Citation: Brooker MR, D'Ambrosio J, Jones MML, Kalcic M, King KW, LaBarge G, Panchalingam T, Roe BE, Schwab ER, Soldo C, Stoltzfus ND, Wilson RS, Winston RJ and Martin JF (2021) A Public-Private Partnership to Locate Fields for Implementation and Monitoring of Best Management Practices to Treat Legacy Phosphorus. Front. Sustain. Food Syst. 5:742817. doi: 10.3389/fsufs.2021.742817
Received: 16 July 2021; Accepted: 10 September 2021;
Published: 11 October 2021.
Edited by:
Shubiao Wu, Aarhus Institute of Advanced Studies, DenmarkReviewed by:
Neslihan Semerci, Marmara University, TurkeyEbrahim Tilahun, Marmara University, Turkey
Copyright © 2021 Brooker, D'Ambrosio, Jones, Kalcic, King, LaBarge, Panchalingam, Roe, Schwab, Soldo, Stoltzfus, Wilson, Winston and Martin. This is an open-access article distributed under the terms of the Creative Commons Attribution License (CC BY). The use, distribution or reproduction in other forums is permitted, provided the original author(s) and the copyright owner(s) are credited and that the original publication in this journal is cited, in accordance with accepted academic practice. No use, distribution or reproduction is permitted which does not comply with these terms.
*Correspondence: Michael R. Brooker, brooker.26@osu.edu