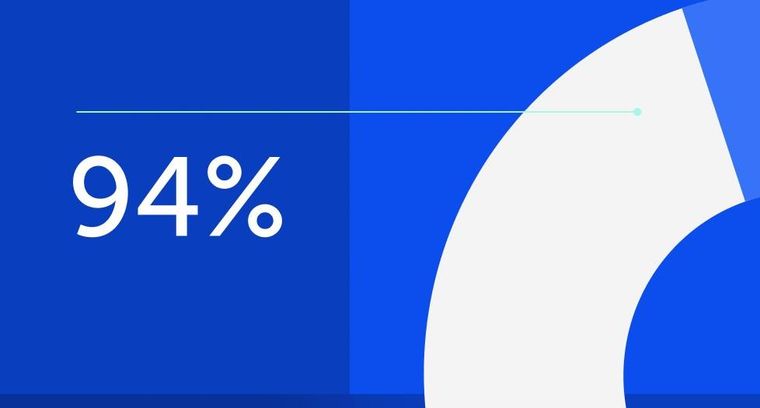
94% of researchers rate our articles as excellent or good
Learn more about the work of our research integrity team to safeguard the quality of each article we publish.
Find out more
ORIGINAL RESEARCH article
Front. Sustain. Food Syst., 26 October 2021
Sec. Agroecology and Ecosystem Services
Volume 5 - 2021 | https://doi.org/10.3389/fsufs.2021.712136
Forage grasses and legumes are the principal source of nutrition for most ruminant livestock in developing countries. Raising yields of forage crops can increase the availability and affordability of livestock products as well as reduce pressure on increasingly scarce land resources by enabling greater herd densities on existing pasture. However, the economic significance of cultivated forage crops in developing countries is not well-understood. We provide estimates of the present area and production value of cultivated forage crops as well as review evidence on the extent of adoption of CGIAR-derived improved varieties of cultivated forage species and their economic impact in developing countries. There are at least 159 million hectares under cultivated forage crops producing yield worth around $63 billion per year (at 2014-2016 prices). Latin America accounts for about 85% of this forage crop area. CGIAR forage breeding programs have developed and helped disseminate improved varieties of Brachiaria, Stylosanthes, Vigna unguiculata, and Calliandra spp., which by 2015 had been adopted on over 12 million hectares producing economic benefits of over $5.8 billion/year.
Forages - largely grasses and legumes - are the principal source of nutrition for most ruminant livestock in developing countries, thus contributing to the supply of nutrient-dense foods like meat and milk as well as products like leather and wool. In addition to using rangelands and native or naturalized pastures for grazing, farmers seed pastures with improved grasses and legumes and cultivate forage crops for e.g., hay, silage and fresh feed. More recently, i.e., during the last 4–5 decades, plant breeders have made important contributions to livestock productivity by developing high yielding forage varieties with tolerances to biotic and abiotic stresses (e.g., Miles et al., 2006; Miles and Hare, 2007; Aguirre et al., 2013; Cardoso et al., 2013; Rao et al., 2016; Hernandez et al., 2017; Abd El-Naby et al., 2019). Raising yields of forage crops can increase the availability and affordability of livestock products as well as reduce pressure on increasingly scarce land resources by enabling greater herd densities on existing pasture. However, the economic significance of cultivated forage crops in developing countries is not well-understood. The (FAO FAOSTAT Food and Agricultural Data), for example, publishes only a few statistics on forage crop area. Lack of basic information on the extent and value of forage crops is a constraint to making informed judgments about the economic potential of forage crop improvement and how forage crops might rank in public and private investment priorities.
The objective of this paper is to review and assess available evidence on the extent and economic significance of cultivated forage crops in developing countries. In particular, we attempt to (i) estimate the present area and production value of cultivated forage crops; and (ii) review evidence on the extent of adoption of CGIAR-derived improved varieties of cultivated forage species and their economic impact. Our geographic focus includes all Latin America and the Caribbean except the southern cone countries of South America (Argentina, Chile, Paraguay and Uruguay), developing countries in Asia except China, Mongolia and North Korea, and Africa.
We define “cultivated forage crops” as any grass, legume or food-feed crop species that has been deliberately seeded by farmers to provide grazing and fodder for livestock. In FAO land use statistics, area sown to cultivated forage crops is sometimes classified as permanent pasture and sometimes as cropland. FAO limits “cropland” to areas cultivated at least once every 5 years. Since many forage species are perennials that are only periodically reseeded to reinvigorate their productivity, they don't always meet the criterion as cropland pasture. In Brazil, for example, “planted pastures” (distinguished from “native pastures” in the Brazilian Agricultural Census) are typically reseeded every 8–10 years (Jank et al., 2014). Thus, FAO defines these areas as part of permanent pasture. For the United States, however, area under alfalfa, clover and other hay and forage crops, which tend to be reseeded every 1–5 years, are considered “cropland pastures” and classified as part of cropland.
In practice it is often difficult to make a sharp distinction between cropland pastures and permanent pastures and perennial cut-and carry forages by this definition. In the U.S. case, for example, an apparent decline of 50 million acres of cropland between the 2007 and 2012 Agricultural Censuses was mostly due to a reclassification of some “cropland pasture” as permanent grassland. FAO estimates that in 2015, New Zealand had 10.7 million hectares of permanent pastures and 0.5 million hectares as cropland, while the USGS GFSAD30 Project, using satellite imagery to measure the extent of global cropland, estimated 8.2 million hectares of cropland for New Zealand—more than 20 times the national estimate (Teluguntla et al., 2017). Much of the land in New Zealand's pastures was at some time in the past seeded with exotic grasses, and is counted as cropland by GFSAD30 but as permanent pasture by FAO. Similarly, the 2017 Brazilian Agricultural Census identifies 112 million hectares as planted pastures and 47 million hectares as natural pastures. As note above FAO classifies both as part of permanent pasture.
To determine area under cultivated forage crops, we canvas national statistical sources in developing countries and other secondary sources. While the FAO does not regularly publish statistics on forage crops, it has made some effort to collect them, which we obtained. Some national statistical offices also publish estimates of cultivated grasslands and forages, particularly in Latin America. Comprehensive estimates of forage crop yield and value at the national level, however, are quite scarce. For this we rely on limited survey evidence and expert opinion. For countries with no national statistics on forage crops—primarily in Sub-Saharan Africa—we “back out” an estimate of the value of forage crops from surveys of dairy production, an approach developed by Gonzalez et al. (2016). Dairies are a primary user of forage crops. Cows and heifers can be grazed in fields during the growing season and fed cut hay and fresh forage while being milked in stalls and during the off-season. Surveys of dairy farmers reveal how much forage and fodder are fed to cattle and the share of these feed sources in total production costs. Scaling these figures by the quantity of milk produced in mixed crop-livestock systems provides a national-level estimate of the value of forages. The economic impact of improved forage crops has also been assessed by comparing the productivity of dairy cows fed native grasses vs. improved forage varieties (Holmann et al., 2004).
Besides Holmann et al. (2004), there are a limited number of studies that have investigated the adoption and economic impact of improved forage varieties in developing countries. White et al. (2013) provide an extensive review of this evidence for a number of different forage species. We add to this by summarizing evidence from some new studies published since their review, but limit our attention to innovations developed through collaboration between CGIAR international agricultural research centers (principally CIAT—now the Alliance of Bioversity International and CIAT- and ILRI) and national agricultural research programs.
Available national statistics on the extent of cultivated forage crops in developing countries are most complete for Latin America. According to estimates compiled from national statistical sources and FAO, Latin America and the Caribbean region has about 137 million hectares in cultivated forage crops, followed by 12 million hectares in South and Southeast Asia, and 6.5 million hectares in Central-West Asia and North Africa (Table 1). The distribution of forage crop area is highly skewed toward Latin America, with Brazil alone accounting for 71% of this area.
Numerous species make up these forages. They include tropical grasses like Brachiaria (now Urochloa) spp. and Panicum maximum (now Megathyrsus maximus) as well as legumes like Stylosanthes spp., Leucaena leucocephala and Vigna unguiculata (cowpea). Brachiaria spp., originally from Africa, is probably the most widely cultivated forage type, accounting for a significant share of the area in Latin America. Trifolium alexandrinum (Egyptian clover or berseem) is the dominant species in Egypt, and alfalfa is widely used in northern Africa. In fact, more than 600 species of grasses are currently sued for grazing and feeding livestock (Clayton, 1983). These tropical forages often thrive in poor soils, the legumes being capable of obtaining nitrogen through biological nitrogen fixation (BNF), and provide hay and fodder that is relatively rich in protein and other important nutrients for ruminant livestock. Unharvested biomass and livestock manure increase organic matter, increase soil water holding capacity, and provide nutrients for subsequent crops, and thus forage crops are often used in rotation with cereals and other field crops (Abd El-Naby et al., 2019; Mazumder et al., 2021).
The gross value of cultivated forages is given by the product of their area, yield, and price. However, utilized yield from grazed land is difficult to measure, and even when harvested may only be used on the farms where they are grown. Thus, market information on quantities traded and prices received for forages is very limited. Available national estimates recorded in the unpublished FAO data on forage crops show annual forage crop yield ranging from 3.4 tons/hectare in Bolivia to 70 tons/hectare under irrigation in Egypt, but with most available estimates in the neighborhood of 10 tons/hectare. We assume an average yield of 10 tons/ha/year (dry weight) when yield estimates are missing from national statistics. For the unit value of forage crops, we assume it is correlated with feed grain prices, as they are to some degree substitutes for one another. Based on expert consultation and some limited market data, Burkart (2020) estimated a standard rule of thumb for the price of forages (on a fresh weight basis) to be on average 18% of the local price of maize (Zea mays), the primary world feed grain. For the purpose of this study, we assume a global average forage price of $36 per ton of dry weight in 2015 dollars, which is 18% of FAO's estimate of the global average price of maize in 2014–2016 (or $201/ton).
For Sub-Saharan African countries lacking estimates of forage crop area, we derive an estimate of the value of cultivated forages based their share of feed costs in dairy production. This procedure requires three pieces of information: (i) national cow milk production, which we take from FAO, (ii) the share of dairy milk produced in mixed crop-livestock systems (where harvested forages are utilized), and (iii) the cost of forages fed to cows in this system expressed as a share of milk value. For East African countries, Gonzalez et al. (2016) provide estimates of (ii) and (iii), which are drawn from surveys of small-holder dairies in these countries. For West and Southern Africa, we derived tentative estimates of these parameters through consultation with dairy experts from national research programs and CGIAR centers based in Sub-Saharan Africa.
The area and value of cultivated forage crops in Sub-Saharan African countries (other than Sudan and South Africa, for which FAO has unpublished estimates of forage crop area) is presented in Table 2. These estimates suggest that forage crops may be cultivated on as much as 3.3 million hectares yielding a forage value of $1,205 million per year in these countries. This area and value are about evenly split between Eastern and Southern Africa, with Western Africa accounting for <1% of the total. Apart from South Africa and Sudan, these estimates are derived from the economic significance of dairy production from mixed crop-livestock systems. However, apart from the estimates provided by Gonzalez et al. (2016) for Eastern African countries, there is little information available on forage use in dairies or other livestock systems exist for other parts of Africa, so these figures should be considered as tentative and approximate.
The information from Tables 1 and 2 are combined in Table 3 to summarize our estimates of the area and economic significance of cultivated forage crops for major regions of the developing world. Drawing on data from the 2012 to 2018 period, cultivated forage crops in developing countries had a value of $63 billion, comparable to the gross value of cassava and sweet potato produced in developing countries ($60 billion, combined). In terms of area, cultivated forages were grown on around 159 million hectares. This amounts to 6.6% of total agricultural land in these countries, and almost half of all agricultural land in Brazil. It is somewhat less than the 167 million hectares sown to rice world-wide.
Raising ruminant livestock productivity by improving the yield and quality of forage crops can have significant social, economic and environmental impacts. White et al. (2013) estimated that improved forage crop varieties have been adopted on at least 118 million hectares in developing countries, but that impacts have been assessed for less than half of this area. Table 4 summarizes evidence on the adoption and impact of improved forage varieties developed by CGIAR agricultural research centers in collaboration with national research programs. To assess the extent of adoption and productivity impact of improved forages, these studies use farm surveys, forage seed sales, and experimental and on-farm trials comparing improved and traditional varieties. Since forages are mostly used on-farm and not sold as a cash crop, economic impacts are often derived from estimates the net value of increased meat and milk production when improved forages are used on beef and dairy farms, rather than from a market valuation of higher forage yields (see Holmann et al., 2004). According to these estimates, by 2010–2015, improved CGIAR-related forage crop varieties had been adopted on around 12.3 million hectares in developing countries, generating annual net benefits of $5.8 billion compared with production using traditional or unimproved forage varieties. This net benefit value does not include China, where White et al. (2013) estimated that CGIAR-related Stylosanthes varieties had been adopted on at least 200,000 hectares.
Table 4. Adoption and impact of CGIAR-related improved forage crop varieties in developing countries.
The great majority of the economic benefits from these improved CGIAR-related forages have accrued in Latin America, where dairy and beef farms are relatively large. Smallholder farmers, which predominate in Asia and Sub-Saharan Africa, account for 19% of the adoption area and 4% of the benefits from improved forages reported in Table 4. Nonetheless, it would seem that there is considerable potential for diffusion of improved forage crops amongst small-holder farmers and possibly also pastoralists, especially in Sub-Saharan Africa, which has more than 700 million hectares of native pastures. Moreover, because of small farm size the number of beneficiaries is likely to be large. Maass et al. (2015) report that efforts to disseminate hybrid Brachiaria cultivars in East Africa have led to adoption on at least 1,000 hectares benefitting 20,000 farm households.
Another important dimension of forage crop development are their potential environmental impacts. The introduction of forage species capable of obtaining plant-associated BNF and their ability to access and use water and nutrients efficiently means that these forage crops can often thrive on relatively poor soils. Studies on the impact of Stylosanthes adoption in West African crop fallows, for example, accounted for their positive yield impact on cereal crops grown in rotation (Elbasha et al., 1999). Regarding land use conversion, studies have confirmed that at the global level, agricultural intensification has been land sparing (Byerlee et al., 2014; Villoria, 2019). However, on the forest frontier, technological improvements that improve the profitability of ruminant livestock could increase incentives to expand pastures at the expense of nearby forest areas (Angelsen and Kaimowitz, 2001). At the same time, productivity improvements can substantially improve resource efficiency in existing production systems, reducing, for example, GHG emissions per ton of meat and milk produced (Gerber et al., 2013).
Kaimowitz and Angelsen (2008) identify several conditioning factors determining whether technological progress in agriculture may lead to more or less deforestation: the factor intensities of new technologies (e.g., how much labor per hectare or per animal they require), the degree to which farmers are labor- or capital-constrained, the size and growth of the market they face for their meat and milk products, and how accessible forest land is for expansion and frontier settlement. To both reap the large economic (and environmental) benefits from technological progress and protect against real environmental risks, policy makers may be able to offer farmers and ranchers improved technologies in return for accepting other policies that limit their ability to expand their pastures into ecologically sensitive areas (Angelsen and Kaimowitz, 2001). Public-private efforts in that regard have in fact been initiated in many Latin American countries, such as the Tropical Forest Alliance (TFA) (MADS, 2019), the Colombian Roundtable for Sustainable Beef and Dairy (MGS-COL) (Tapasco et al., 2019), and the Nationally Appropriate Mitigation Actions (NAMA)—which are active in several countries including Costa Rica and Colombia. These efforts generally use market incentives (through certification) to support adoption of sustainable technologies that include limits on deforestation.
In this paper, we sought to develop estimates of the economic significance of cultivated forage crops in developing countries. Lack of statistics and systematic assessments on forage crops limits informed judgment on the value and potential of public and private investment in forage crop development. For example, economic analysis to help inform CGIAR research investment priorities in crop breeding programs (Wiebe et al., 2021) excluded forage crops due to the absence of such essential data.
Our estimates suggest that across Latin America, Africa, and Asia (excluding China and southern cone countries of South America), there is likely to be at least 159 million hectares under cultivated forage crops producing yield worth around $63 billion per year (at 2014–2016 prices). This does not include land in native pastures, estimated by FAO to be around 1.6 billion hectares in this set of countries. Latin America, which has the best data on forage crops, accounts for about 85% of the estimated cultivated forage crop area in developing countries. Data on forage crops in Sub-Saharan Africa, on the other hand, are very sparse, and our estimates for this region are derived indirectly by extrapolating survey evidence on the use of forages in dairy production. Excluding South Africa, this approximation suggests there may be 1.8 million hectares of cultivated forage crops currently grown in Sub-Saharan Africa, mostly in Eastern African countries (and 3.3. million hectares in South Africa is included).
The paper also reviews evidence on the economic impact of CGIAR programs in forage crop improvement. With relatively modest R&D investment, CGIAR forage breeding programs have achieved significant economic impacts. Improved varieties of Brachiaria, Stylosanthes, Vigna unguiculata, and Calliandra spp. have been adopted on over 12 million hectares in Latin America, Asia and Sub-Saharan Africa, producing net economic benefits of over $5.8 billion/year compared with productivity using traditional cultivars. Most of these economic impacts have occurred in Latin America and on farms that are relatively large. Impacts on small-holder farmers in Asia and Africa have been more limited but still significant—about 2.4 million hectares of adoption producing net benefits of $229 million/year in these regions. Comparing the size of documented impacts to our estimates of the current value of forage crops, CGIAR technologies would appear to account for about 10% of the annual economic output from forage crops in Latin America ($5.6 billion of impact out of $53.0 billion of total forage crop value) but only 1–2% of forage crop output in Asia and Sub-Saharan Africa (not including dual-purpose crops like cowpea).
Another important dimension of impact, and not fully resolved in empirical studies, is how improved forage crops and other technologies designed to intensify ruminant livestock production affect land use and the environment. While at the global level, agricultural technical change has likely been land saving, it is possible that in land abundant countries, improvements in agricultural productivity near the forest frontier may increase incentives to convert forests to cropland and pastures. To reap the benefits of technological change and protect critical environmental resources may require complementary policies or initiatives that both support agricultural R&D and protect critical ecologically-rich forest lands and natural resources.
The original contributions presented in the study are included in the article/supplementary material, further inquiries can be directed to the corresponding authors.
KF, MP, and SB contributed to conception and design of the study. KF organized the database and SB contributed to it. KF performed the statistical analysis and wrote the first draft of the manuscript. All authors contributed to manuscript revision, read, and approved the submitted version.
This work was supported by the U.S. Agency for International Development and the U.S. Department of Agriculture, Economic Research Service. This work was funded by the CGIAR Research Program on Livestock. The funders had no role in the design of the study; in the collection, analyses, or interpretation of data; in the writing of the manuscript, or in the decision to publish the results.
The findings and conclusions are those of the authors and should not be construed to represent any official USAID, USDA or U.S. Government determination or policy.
The authors declare that the research was conducted in the absence of any commercial or financial relationships that could be construed as a potential conflict of interest.
All claims expressed in this article are solely those of the authors and do not necessarily represent those of their affiliated organizations, or those of the publisher, the editors and the reviewers. Any product that may be evaluated in this article, or claim that may be made by its manufacturer, is not guaranteed or endorsed by the publisher.
This work was carried out as part of the CGIAR Research Program on Livestock. We thank all donors who globally support our work through their contributions to the CGIAR System. CGIAR is a global research partnership for a food-secure future. Its science is carried out by 15 Research Centers in close collaboration with hundreds of partners across the globe. We also thank the reviewers for their constructive comments that helped to improve the manuscript.
Abd El-Naby, Z. M., Hafez, W. A. E.-K., and Hashem, H. A. (2019). Remediation of salt-affected soil by natural and chemical amendments to improve berseem clover yield and nutritive quality. Afr. J. Range Forage Sci. 36:49–60. doi: 10.2989/10220119.2018.1518929
Agricultural Census (2007). Censo Agricola, Ganadero y Forestal. Instituto Nacional de Estadistica y Geografia (INEGI).
Agricultural Census (2017). Censo Agropecuario 2017-Resultados Preliminaries. Instituto Brasilerio de Geografia e Estatistica (IBGE).
Agricultural Census (2018). Encuesta de Superficie y Produccion Agropecuaria Continua Ecuador. Instituto Nacional de Estadistica y Censos (INEC).
Aguirre, L. M., Cardona, C., Miles, J. W., and Sotelo, G. (2013). Characterization of resistance to adult spittlebugs (Hemiptera: Cercopidae) in Brachiaria spp. J. Econ. Entomol. 106, 1871–1877. doi: 10.1603/EC11189
Angelsen, A., and Kaimowitz, D., (eds.). (2001). Agricultural Technologies and Tropical Deforestation. Wallingford: CABI Publishing and Center for International Forestry Research (CIFOR).
Burkart, S. (2020). Survey of Forage and Feed Grain Prices in Developing Countries. Cali: Unpublished mimeo, International Center for Tropical Agricultural (CIAT).
Byerlee, D., Stevenson, J., and Villoria, N. (2014). Does intensification slow crop land expansion or encourage deforestation? Global Food Sec. 3, 92–98. doi: 10.1016/j.gfs.2014.04.001
Cardoso, J. A., Jiminez, J., Rincón, J., Guevara, E., Hoek, R., van der Jarvis, A., et al. (2013). Advances in improving tolerance to waterlogging in Brachiaria grasses. Trop. Grass. 1, 197–201. doi: 10.17138/TGFT(1)197-201
Clayton, W. D. (1983). “Tropical grasses,” in Genetic Resources of Forage Plants, eds J. G. MclIover and R. A. Bray. (Canberra: CSIRO), 39–46.
Elbasha, E., Thornton, P., and Tarawali, G. (1999). An Ex-Post Economic Impact Assessment of Planted Forages in West Africa. ILRI Impact Assessment Series 2. Nairobi: ILRI.
FAO FAOSTAT Food Agricultural Data, . Rome: Food Agriculture Organization (FAO) of the United Nations. Available online at: http://www.fao.org/faostat/en/#data. (accessed October 7 2021).
FAO. (2017). FAOSTAT online dataset. FAO Perm Pasture-Cultivated. Rome: Food and Agriculture Organization. (accessed April, 2017).
Gerber, P. J., Steinfeld, H., Henderson, B., Mottet, A., Opio, C., Dijkman, J., et al. (2013). Tackling Climate Change Through Livestock - A Global Assessment of Emissions and Mitigation Opportunities. Rome: Food and Agriculture Organization of the United Nations (FAO).
Gonzalez, C., Schiek, B., Mwendia, S., and Prager, S. (2016). Improved Forages and Milk Production in East Africa. A Case Study in the Series: Economic Foresight for Understanding the Role of Investments in Agriculture for the Global Food System. Cali: Centro Internacional de Agricultura Tropical (CIAT).
Hernandez, L. M., Sotelo, G., Bonilla, X., Álvarez, E., Miles, J., and Worthington, M. (2017). Phenotyping Brachiaria genotypes to assess Rhizoctonia resistance by comparing three inoculum types. Plant Dis. 101, 916–923. doi: 10.1094/PDIS-08-16-1160-RE
Holmann, F., Rivas, L., Argel, P., and Perez, E. (2004). Impact of the adoption of Brachiaria grasses: Central America and Mexico. Livestock Res. Rural Dev. 16. Available onilne at: http://www.lrrd.org/lrrd16/12/holm16098.htm
ISPC. (2018). Tropical Forages and the Diffusion of Brachiaria Cultivars in Latin America. Rome: Brief Number 70, Standing Panel on Impact Assessment, CGIAR Independent Science and Partnership Council (ISPC), Science Council Secretariat.
Jank, L., Barrious, S., do Valle, C., Simeao, R., and Alves, G. (2014). The value of improved pastures to Brazilian beef production. Crop Pasture Sci. 65, 1132–1137. doi: 10.1071/CP13319
Kaimowitz, D., and Angelsen, A. (2008). Will livestock intensification help save Latin America's tropical forests? J. Sust. Forest. 27, 6–24. doi: 10.1080/10549810802225168
Kristjanson, P., Okike, I., Tarawali, S., Singh, B., and Manyong, V. (2005). Farmers' perceptions of benefits and factors affecting the adoption of improved dual-purpose Cowpea in the Dry Savannas of Nigeria. Agric. Econ. 32, 195–210. doi: 10.1111/j.0169-5150.2005.00338.x
Kristjanson, P., Tarawali, S., Okike, I., Singh, B., Thornton, P., Manyong, V., et al. (2001). Genetically Improved Dual-purpose Cowpea: Assessment of Adoption and Impact in the Dry Savanna Region of West Africa. Nairobi: ILRI.
Labarta, R., Martínez, J. M., Yaccelga, A., Reyes, B., Gómez, L., Maredia, M., et al. (2017). Assessing the Adoption and Economic and Environmental Impacts of Brachiaria Grass Forage Cultivars in Latin America Focusing in the Experience of Latin America. Research Report to the Standing Panel of Impact Assessment (SPIA) under the SIAC project. Cali: Colombia.
Maass, B. L., Midega, C. A. O., Mutimura, M., Rahetlah, V. B., Salgado, P., Kabirizi, J. M., et al. (2015). Homecoming of Brachiaria: improved hybrids prove useful for African animal agriculture. East Afr. Agric. Forest. J. 81, 71–78. doi: 10.1080/00128325.2015.1041263
MADS. (2019). Cadenas de carne y leche firman acuerdos de cero deforestación que protegerán bosques y páramos en Colombia. Available online at: https://www.minambiente.gov.co/index.php/noticias/4292-cadenas-de-carne-y-leche-firman-acuerdos-de-cero-deforestacion-que-protegeran-bosques-y-paramos-en-colombia (accessed January 5, 2021).
Mazumder, P., Akhil, P., Jyoti Khwairakpam, M., Mishra, U., and Kalamdhad, A. S. (2021). Enhancement of soil physico-chemical properties post compost application: optimization using response surface methodology comprehending central composite design. J. Environ. Manage 289:112461. doi: 10.1016/j.jenvman.2021.112461
Miles, J. W., Cardona, C., and Sotelo, G. (2006). Recurrent selection in a synthetic brachiariagrass population improves resistance to three spittlebug species. Crop. Sci. 46, 1088–1093. doi: 10.2135/cropsci2005.06-0101
Miles, J. W., and Hare, M. D. (2007). Plant breeding and seed production of apomictic tropical forage grasses. Bioforsk Fokus. 2, 74–81. Available online at: https://hdl.handle.net/10568/88944
National Statistics (2011). Censo Nacional Agropecuario. Instituto Nacional de Estadistica y Censo (INEC).
National Statistics (2015). Censo Nacional Agropecuario 2013 Bolivia. Instituto Nacional de Estadistica (INE).
National Statistics (2016). Statistical Yearbook. Cairo: Central Agency for Public Mobilization and Statistics (CAPMAS).
Place, F., Ralph, R. L., Maina, L., Franzel, S., Sinja, J., and Wanjiku, J. (2009). The Impact of Fodder Trees on Milk Production and Income Among Smallholder Dairy Farmers in East Africa and the Role of Research. ICRAF Occasional Paper No. 12. Nairobi: World Agroforestry Centre.
Rao, I. M., Miles, J. W., Beebe, S. E., and Horst, W. J. (2016). Root adaptations to soils with low fertility and aluminium toxicity. Ann. Bot. 118, 593–605. doi: 10.1093/aob/mcw073
Tapasco, J., LeCoq, J. F., Ruden, A., Rivas, J. S., and Ortíz, J. (2019). The livestock sector in colombia: toward a program to facilitate large-scale adoption of mitigation and adaptation practices. Front. Sust. Food Syst. doi: 10.3389/fsufs.2019.00061
Tarawali, G., Manyong, V., Carsky, R., Vissoh, P., Osei-Bonsu, P., and Galiba, M. (1999). Adoption of improved fallows in West Africa: lessons from Mucuna and Stylo case studies. Agrofor. Syst. 47, 93–122. doi: 10.1023/A:1006270122255
Teluguntla, P., Thenkabail, P., Xiong, J., Gumma, M., Congalton, R., Oliphant, A., et al. (2017). NASA Making Earth System Data Records for Use in Research Environments (MEaSUREs) Global Food Security-support Analysis Data (GFSAD) Cropland Extent 2015 Australia, New Zealand, China, Mongolia 30 m V001 [Data set]. Sioux Falls: NASA EOSDIS Land Processes DAAC.
Villoria, N. B. (2019). Technology spillovers and land use change: empirical evidence from global agriculture. Am. J. Agric. Econ. 101, 870–93. doi: 10.1093/ajae/aay088
White, D. S., Peters, M., and Horne, P. (2013). Global Impacts from improved tropical forages: a meta-analysis revealing overlooked benefits and costs, evolving values and new priorities. Trop. Grass. 1, 12–24. doi: 10.17138/TGFT(1)12-24
Keywords: variety adoption, research impact, CGIAR, Brachiaria, economic value
Citation: Fuglie K, Peters M and Burkart S (2021) The Extent and Economic Significance of Cultivated Forage Crops in Developing Countries. Front. Sustain. Food Syst. 5:712136. doi: 10.3389/fsufs.2021.712136
Received: 02 June 2021; Accepted: 29 September 2021;
Published: 26 October 2021.
Edited by:
Johann G. Zaller, University of Natural Resources and Life Sciences Vienna, AustriaReviewed by:
Nirmalendu Basak, Central Soil Salinity Research Institute (ICAR), IndiaCopyright © 2021 Fuglie, Peters and Burkart. This is an open-access article distributed under the terms of the Creative Commons Attribution License (CC BY). The use, distribution or reproduction in other forums is permitted, provided the original author(s) and the copyright owner(s) are credited and that the original publication in this journal is cited, in accordance with accepted academic practice. No use, distribution or reproduction is permitted which does not comply with these terms.
*Correspondence: Keith Fuglie, S2VpdGguZnVnbGllQHVzZGEuZ292; Stefan Burkart, cy5idXJrYXJ0QGNnaWFyLm9yZw==
Disclaimer: All claims expressed in this article are solely those of the authors and do not necessarily represent those of their affiliated organizations, or those of the publisher, the editors and the reviewers. Any product that may be evaluated in this article or claim that may be made by its manufacturer is not guaranteed or endorsed by the publisher.
Research integrity at Frontiers
Learn more about the work of our research integrity team to safeguard the quality of each article we publish.