- 1Department of Food Sciences, University of Massachusetts, Amherst, MA, United States
- 2Department of Plant Sciences, North Dakota State University, Fargo, ND, United States
- 3Stockbridge School of Agriculture, University of Massachusetts, Amherst, MA, United States
Food quality improvements of fresh fruits targeting both food preservation and human health is essential to advance healthy dietary options and to mitigate imbalanced nutrition-linked non-communicable chronic disease (NCDs) challenges globally. Specifically, protective phenolic bioactives of fruits with dual functional benefits can be harnessed to advance innovations for improving nutritional quality and post-harvest shelf-life of perishable fruits. Based on this rationale the dual functional benefits of plant phenolics were harnessed using novel biological elicitation strategies to modulate phenolic bioactive-linked protective responses in apple during storage in two interrelated studies. Bioprocessed food-grade elicitors [water soluble chitosan oligosaccharide -(COS) and phenolic enriched oregano extracts-(OX)] were targeted as post-harvest dipping treatments (2 & 4 g/ L) and compared with diphenylamine (DPA) (1 & 2 g/L) to enhance phenolic-linked antioxidant and anti-diabetic (type 2 diabetes) relevant properties of Cortland apple during 3 months of storage (4°C). The selection of bio-elicitors and respective doses were based on the foundations of the previous related study, which resulted in reduction of superficial scald of Cortland apple during storage. Apples sampled over 3 months as aqueous and ethanol (12%) extracts of peel and pulp were analyzed separately for total soluble phenolic content, phenolic profile, antioxidant activity, and glucose metabolism relevant α-amylase and α-glucosidase enzyme inhibitory activities using in vitro assay models. Enhanced soluble phenolic content and associated antioxidant activity were observed in ethanol (12%) extracts of apple peel with 4 g/L COS elicitor treatments after 2 and 3 months of storage. High chlorogenic acid and quercetin derivatives were found in peel extracts of Cortland apple, while pulp extracts had high chlorogenic and gallic acids. Additionally, high α-glucosidase enzyme inhibitory activity, which is relevant for managing post-prandial hyperglycemia of type 2 diabetes was also observed in bio-elicited apple peel and pulp extracts. Therefore, results of these two interrelated studies indicate that bioprocessed food grade elicitor such as OX and COS can be recruited as a novel tool to enhance protective phenolic responses for improving type 2 diabetes targeted food quality and post-harvest storage quality of apple.
Introduction
Increasing awareness among consumers about the beneficial impact of balanced nutrition and diet on human health is driving the demand and market value of fresh fruits and vegetables globally (Porat et al., 2018). Among popular and nutritionally relevant fruits, apple (Malus domestica) ranks second in terms of consumption, and United States is the second largest producer in the world (USDA, 2020). The increasing popularity of apples as fresh and processed foods is mostly due to its nutritional attributes and overall perception of their health benefits (Endrizzi et al., 2015). High dietary fiber, essential micronutrients, vitamins, and dietary antioxidant profile of apple contributes to their well-rounded nutritional qualities (Musacchi and Serra, 2018). All these food qualities of apple are also pertinent for supporting balanced nutritional needs, while also providing wider protection against diet and lifestyle-influenced non-communicable chronic diseases (NCDs) (Hyson, 2011).
Previous studies indicated that higher consumption of apple is inversely related to reduced risks of several NCDs, such as cardiovascular diseases, asthma and pulmonary function, type 2 diabetes, inhibition of lipid oxidation, and potentially provides protection against certain types of cancers (Schiavano et al., 2015; Hodgson et al., 2016; Bondonno et al., 2017; Hua et al., 2018; de Oliveira Raphaelli et al., 2019). Some of these NCD preventative properties of apples are attributed to the composition and higher concentration of phenolic bioactives, like flavonoids and phenolic acids, which are naturally present and widely distributed in apple peel and pulp (Barbosa et al., 2012; Rana and Bhushan, 2016; Jakobek et al., 2020). In the United States, it is estimated that apple alone contributes to about 22% of total phenolics consumed from fruits making it the largest source of these human health protective bioactive compounds (Vinson et al., 2001; Chun et al., 2005). However, the composition and concentration of phenolic bioactives of apple alter significantly during post-harvest storage and processing stages, affecting both food quality and shelf-life (Lu et al., 2012; Francini and Sebastiani, 2013). Therefore, phenolic bioactive linked food quality improvements of apple can be targeted for dual functional benefits of enhancing human health protective functional properties as well as preservation qualities.
Most harvested apples are generally stored in controlled environmental chambers for over months, prior to its cold stored transportation and distribution in retail stores or fresh market. Long-term storage of apples facilitates the year-around supply of this popular and nutritionally beneficial fruit to consumers. However, once taken out from the controlled environmental condition of storage, food quality of apple deteriorates rapidly in the retail shelves resulting in browning and darkening of skin, commonly known as superficial scald (Jha et al., 2012; Bordonaba et al., 2013). Apart from the development of superficial scald, apple also becomes susceptible to different biotic and abiotic stresses resulting in poor fruit quality, post-harvest waste, and reduction of retail prices (Chai et al., 2020). Therefore, it is essential to develop and find novel post-harvest tools to counter superficial scald formation and improve overall food qualities of apple supporting fruit and retail industries, hence improving access to good quality fresh fruits to consumers.
In current post-harvest strategies, diphenylamine (DPA) or 1- methylcyclopropene (1-MCP) are commonly used to prevent superficial scald development and to improve preservation qualities in apples during long-term storage (Moggia et al., 2010; Du et al., 2017). Both DPA and 1-MCP are used as postharvest treatments and DPA is a key chemical residue that is detected in highest concentration in apple peel (Iñigo-Nuñez et al., 2010). With increasing consumers' preference for organic and naturally preserved fresh fruits, it is important to find alternative post-harvest tools to counter superficial scald development, while also improving food quality and shelf-life of stored apple (Denver and Jensen, 2014).
The development of superficial scald and deterioration of the nutritional qualities of apple are directly linked to oxidative breakdown in peel and pulp during storage. Previous studies reported that breakdown of redox regulation and subsequent oxidation of α-farnesene to conjugated triene (CT) is the major contributing factor in the development of superficial scald in apple (Lurie and Watkins, 2012; Karagiannis et al., 2020). Therefore, improving endogenous antioxidant protection and maintenance of redox balance is critical to address superficial scald development, while concurrently preventing post-harvest losses (Sabban-Amin et al., 2011; Bordonaba et al., 2013). Many bioactive antioxidants, like phenolics are widely distributed in apple peel, which potentially plays a critical protective functional role in preventing superficial scald as well as improving food qualities (Busatto et al., 2014, 2018). However, the functional role of phenolic antioxidants in superficial scald reduction is complex and not well understood. The higher concentration of water-soluble phenolic compounds can lead to enzymatic browning due to polymerization by polyphenol oxidase (PPO), while less oxidized phenolics can act as antioxidant and prevent oxidation of α-farnesene to conjugated triene (CT) (Lurie and Watkins, 2012). Therefore, the right balance of oxidation protective phenolics and other antioxidant enzymes in apple peel is important to reduce superficial scald and enzymatic browning at post-harvest stages. In the previous related study (Sarkar et al., 2018), we observed that improved redox regulation through up-regulation of anabolic pentose phosphate pathway (PPP) and subsequent biosynthesis of less oxidized phenolics and other antioxidant enzymes resulted in the reduction of superficial scald development in stored Cortland apple following post-harvest treatments with DPA and phenolic enriched oregano extracts (OX). Additionally, improved antioxidant enzyme regulation in apple peel was also observed in response to water soluble chitosan oligosaccharide (COS) dipping treatment (Sarkar et al., 2018). Due to the dual functional benefits of phenolic bioactives, we hypothesized that phenolic and antioxidant enrichment with post-harvest dipping treatments (DPA, OX, and COS) might also have relevance in improving human health targeted nutritional and phenolic bioactive qualities of apple.
High phenolic bioactive associated antioxidant and anti-diabetic relevant anti-hyperglycemic properties were observed in fresh and stored apples previously (Adyanthaya et al., 2010; Barbosa et al., 2010, 2012). Based on the promising findings of previous related studies, biological elicitation with food grade OX and COS as post-harvest treatments was advanced to improve phenolic bioactive associated antioxidant and anti-hyperglycemic properties in stored (for 3 months) Cortland apple. Additionally, the efficacy of biological elicitors was also compared with current commercial post-harvest treatment of DPA. Previously, post-harvest coating with edible chitosan resulted in reduced ethylene production, delayed ripening, higher retention of firmness and controlled decay as well as improved antifungal and antimicrobial properties of fresh cut and stored apple (Garrido Assis and de Britto, 2011; Qi et al., 2011; Shao et al., 2012; Zhang et al., 2020). Like COS, improvement in antimicrobial and post-harvest preservation properties of fruits and vegetables with exogenous application of phytochemical enriched oregano extracts was also observed (Kraśniewska et al., 2014; Sultanbawa, 2014; Li et al., 2020). These edible and natural elicitors can directly potentially provide antioxidant protection or can stimulate redox-protective anabolic responses of fruits during post-harvest storage and thereby improving their nutritional qualities and shelf-life.
Therefore, the aim of the present study was to examine the efficacy of edible COS and OX as post-harvest elicitor treatments on changes in phenolic-linked antioxidant and anti-hyperglycemic functionalities of Cortland apple peel and pulp during 3 months of storage. Total soluble phenolic content, phenolic compound profile, and inhibitory activity of carbohydrate metabolizing enzymes, like α-glucosidase and α-amylase of food grade extracts (aqueous and 12% ethanol) of apple peel and pulp were investigated separately using rapid in vitro model based assays. The wider aim of this study was to find effective and safe biological elicitors as an alternative to synthetic DPA and 1-MCP treatments to improve redox-linked post-harvest preservation and nutritional qualities of stored apple. Such bio-elicited and phenolic enriched apples can be targeted in dietary interventions to counter chronic oxidative stress and chronic hyperglycemia commonly associated with type 2 diabetes.
Materials and Methods
Apple Harvest
This biological elicitation study was conducted with apple cultivar “Cortland,” which is highly susceptible to superficial scald and post-harvest losses. The apples were grown at the Cold Spring Orchard (Belchertown, Massachusetts 01007, USA) of the University of Massachusetts, Amherst. Harvesting of apple were carried out on September 19, 2011, which was 143 days after the full bloom and is standard accepted practice for scald studies. Cortland apples are typically harvested after 150 days of full bloom and based on the maturity indicating 85% red color and 6.35 kg Firmness as followed by Massachusetts Agricultural Experimental Station, Belchertown, Massachusetts. In this study, 7 days early harvest was targeted to pre-dispose apples to superficial scald as described in the previous related study (Sarkar et al., 2018). Eighty to hundred fruits from one tree were collected in a bushel. Each bushel was considered as a biological replication unit. For seven treatments a total of 42 bushels of apple were harvested and targeted for biological elicitor treatments.
Biologically Based Elicitor Treatments
In this study seven post-harvest treatments as part of biological elicitation were targeted; T1- Control-dipped for 1 min in water, T2-diphenylamine (DPA)- apples dipped for 1 min in DPA 1 g/L solution, T3- dipped for 1 min in DPA 2 g/L solution, T4- bioprocessed oregano (OX) with minimum 7% rosmarinic acid (Barrington Nutritionals, Harrison, NY, USA)- dipped for 1 min in OX 2 g/L solution, T5-dipped for 1 min in OX 4 g/L solution, T6-water soluble chitosan oligosaccharide with ascorbic acid side chain (COS) (Kung Pung Bio, Jeju, South Korea)—dipped for 1 min in COS 2 g/L solution, T7- dipped for 1 min in COS 4 g/L solution. Following dipping treatments, apples were allowed to dry before being stacked on a pellet and kept in the cold storage (4°C) for 3 months (September 19th—December 18th). Immediately after harvest and during storage, 15 apples from each treatment block were taken out for evaluation, extraction, and to conduct biochemical analysis.
Extraction
Stored apples were first peeled to separate peel and pulp for extractions and then cut into small pieces. To understand the potential impact of elicitor treatments and post-harvest storage, peel and pulp were analyzed separately. Both peel and pulp of Cortland apple were extracted using distilled water (aqueous) and 12% ethanol (the solvents were targeted for food-grade use investigation). Peel extractions were performed by using 20 g of peel in 50 mL of distilled water and 5 g of peel in 15 mL of 12% ethanol. The peel refers to outer skin only. Pulp extractions were performed by using 100 g of pulp in 50 mL of water and 10 g of pulp in 20 mL of 12% ethanol. This included no outer skin (Figure 1).
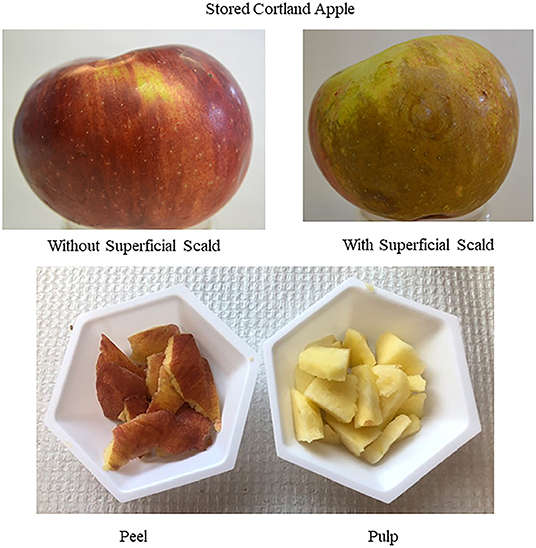
Figure 1. Stored “Cortland” apple with peel and pulp extracted separately using cold water and 12% ethanol.
Extractions were carried out by homogenizing using a Waring blender (CA, USA) for 3 min. The samples were then centrifuged at 15,000 g for 15 min. Supernatants were collected and stored at 4°C during the period of study. pH of the extracts was adjusted to 5.8–6.2 (to avoid interference of acidic pH toward in vitro enzyme inhibitory activities) and all biochemical analysis were carried out within a week of extraction.
Total Soluble Phenolic Assay
The total soluble phenolic content of Cortland apple peel and pulp was analyzed using the modified Folin-Ciocalteu method (Shetty et al., 1995). Initially, 0.5 mL of supernatant was transferred into a test tube and diluted with 0.5 mL of distilled water. The diluted (2 times) aliquot were then mixed with 1 mL of 95 % ethanol and 5 mL of distilled water. Then to each sample, 0.5 mL of 50 % (v/v) Folin-Ciocalteu reagent was added and mixed thoroughly with a vortex mixer. After 5 min, 1 mL of 5 % Na2CO3 was added, and the reaction mixture was allowed to stand for 60 min. The absorbance was read at 725 nm using Genesys UV/Visible spectrophotometer (Thermo Fisher Scientific, Waltham, MA, USA). The absorbance values were converted to total soluble phenolic content and were expressed in milligrams gallic acid equivalents per gram fresh weight (FW) of the sample. Standard curve was established using various concentrations of gallic acid dissolved in 95 % ethanol.
Antioxidant Activity by DPPH Radical Inhibition Assay
The antioxidant activity was determined by the DPPH (2, 2- diphenyl-1-picrylhydrazyl) free radical scavenging method modified from Kwon et al. (2006). A 250-μL aliquot of the sample extract was mixed with 1,250 μL of DPPH (60 μM in ethanol). Absorbance was measured at 517 nm using the Genesys UV/Visible spectrophotometer. The absorbance readings of samples were compared with the controls, containing 95% ethanol instead of sample extract. The percentage DPPH inhibition was calculated by:
α-Glucosidase Enzyme Inhibition Assay
The α-glucosidase enzyme inhibitory activity was determined by an assay modified from McCue et al. (2005). α-Glucosidase was assayed by using 50 μL of apple sample extracts and 100 μL of 0.1 M phosphate buffer (pH 6.9) containing α-glucosidase solution (1 U/mL) and was incubated in 96-well plates at 25°C for 10 min. After preincubation, 50 μL of 5 mM p-nitrophenyl-α-d-glucopyranoside solution in 0.1 M phosphate buffer (pH 6.9) was added in each well at timed intervals. The reaction mixtures were incubated at 25°C for 5 min. Before and after incubation, absorbance readings were determined at 405 nm by a microplate reader (Thermomax, Molecular Devices Co., Sunnyvale, CA) and compared with a control that had 50 μL of buffer solution instead of the extract. Dose dependency was evaluated using 25 μL and 10 μL of the sample, and the volume made up to 50 μL using 0.1 M phosphate buffer (pH 6.9) and using same protocol described above.
The α-glucosidase enzyme inhibitory activity was expressed as percentage inhibition using following formula:
α-Amylase Enzyme Inhibition Assay
The α-amylase enzyme inhibitory activity was determined by an assay modified from McCue and Shetty (2004). A total of 500 μL of undiluted, 1:2, and 1:5 dilution (due to the high sugar content and to investigate dose dependency) of sample extract and 500 μL of 0.02 M sodium phosphate buffer (pH 6.9 with 0.006 M NaCl) containing α-amylase enzyme solution (0.5 mg/mL) were incubated at 25°C for 10 min. After pre-incubation, 500 μL of a 1% starch solution in 0.02 M sodium phosphate buffer (pH 6.9 with 0.006 M NaCl) as substrate was added to each tube at timed intervals. Total of 1.0 mL of dinitro salicylic acid color reagent was added to stop the reaction. The reaction test tubes were then incubated in a boiling water bath for 5 min and cooled to room temperature. The reaction mixture was then diluted after adding 15 mL of distilled water, and the absorbance was measured at 540 nm using the Genesys UV/Visible spectrophotometer. The readings were compared with the controls, containing buffer instead of sample extract and sample blank containing buffer instead of enzyme. The percentage α-amylase enzyme inhibitory activity was calculated with the following equation:
High Performance Liquid Chromatography (HPLC) Analysis of Phenolic Profiles
The apple peel and pulp extracts (2 mL) were filtered through a 0.2 μm filter. A volume of 5 μL of extract was injected using an Agilent ALS 1100 auto sampler into an Agilent 1100 series HPLC (Agilent Technologies, Palo Alto, CA) equipped with a DAD 1100 diode array detector. The solvents used for gradient elution were (A) 10 mM phosphoric acid (pH 2.5) and (B) 100% methanol. The methanol concentration was increased to 60% for the first 8 min and to 100% over the next 7 min, then decreased to 0% for the next 3 min and was maintained for the next 7 min (total run time, 25 min). Agilent Zorbax SB-C18, 250 x 4.6 mm i.d., analytical column with packing material of 5 μm particle size was used with flow rate of 1 mL/min was maintained at room temperature. During each run, the absorbance was recorded at 226 and 306 nm and the chromatogram was integrated using Agilent Chemstation enhanced integrator. Pure standards of phenolic compounds in 100% methanol were used to calibrate the standard curves and to match the retention times.
Statistical Analysis
All experiments were performed with six replications. The statistically significant effect of apple sample (peel vs. pulp) (S), extraction solvent (water vs. 12% ethanol) (E), elicitor treatments (control, DPA, OX, and COS) (T), storage duration (D) and their respective 2-way (S × E; S × T; E × T; D × S; D × E; D × T), 3-way (S × E × T; D × S × E; D × S × T; D × E × T), and 4-way (D × S × E × T) interactions were determined using analysis of variance (ANOVA) of SAS (version 9.4; SAS Institute, Cary, NC) software. Statistically significant differences in soluble phenolic content, antioxidant activity, α-glucosidase, α-amylase (half-diluted) enzyme inhibitory activities, and chlorogenic acid content between main effects and their respective interactions were determined using Tukey's test at the 95% confidence level and presented in Supplementary Appendix 1. For α-amylase enzyme inhibition (undiluted apple peel and 1/5th diluted apple pulp) and phenolic profile (quercetin, p-coumaric, and gallic acids), statistical significance (p ≤ 0.05) between 3-way interactions of storage duration (D) × extraction solvent (E) × post-harvest elicitor treatment (T) were calculated and presented (Supplementary Appendix 2). Standard error for each investigated biochemical parameter was calculated using Microsoft Excel 2010 software.
Results and Discussion
Total Soluble Phenolic Content and Phenolic Profile
Total soluble phenolic content and phenolic compound profile of aqueous and ethanol (12%) extracts of bio-elicited apple peel and pulp were determined separately and compared during post-harvest storage. In this study, total soluble phenolic content of Cortland apple peel ranged between 0.35 and 1.44 mg GAE/ g F.W., and 0.05- 0.32 mg GAE/ g F.W. for pulp extracts (Figures 2A,B). Previously, similar level of soluble phenolic content was observed in stored apple peel and pulp (Adyanthaya et al., 2009, 2010; Barbosa et al., 2012). Overall, statistically significant differences in soluble phenolic content between apple sample (peel vs. pulp) (S), extraction solvent (water vs. 12% ethanol) (E), elicitor treatments (control, DPA, OX, & COS) (T), storage duration (D) and their respective 2-way (S × E; S × T; E × T; D × S; D × E; D × T), 3-way (S × E × T; D × S × E; D × S × T; D × E × T), and 4-way (D × S × E × T) interactions were observed at all post-harvest storage time points (Supplementary Appendix 1; Figures 2A,B). In all storage time points, significantly higher soluble phenolic content was observed in apple peel, when compared to apple pulp. Ethanol (12%) extracts of both apple peel and pulp had significantly higher total soluble phenolic content when compared to aqueous extracts of the same sample. Before storage and at 2 months of storage, ethanol (12%) extracts of apple peel treated with 4 g/L COS had significantly higher total soluble phenolic content, while after 3 months of storage, significantly higher phenolic content was observed in ethanol (12%) extracts of apple peel with 4 g/L OX elicitor treatment. Overall, significantly higher phenolic content was observed in apple peel at 2 months of storage irrespective of the extraction methods and elicitor treatments.
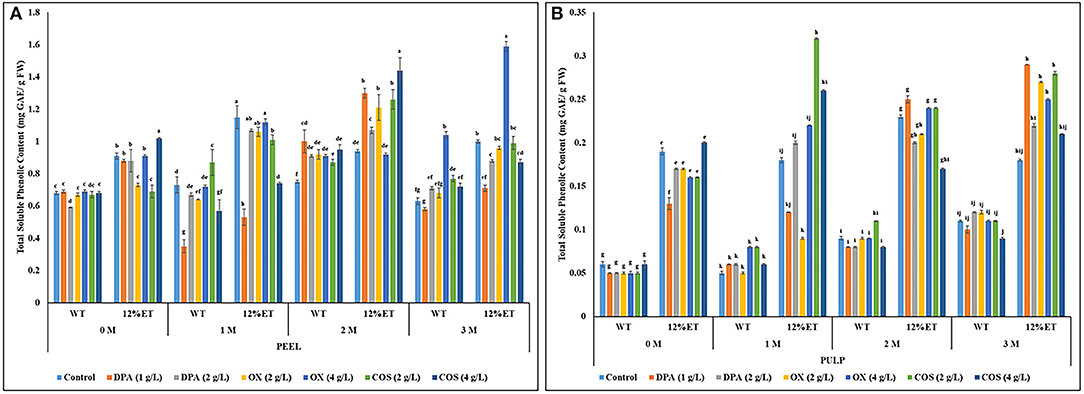
Figure 2. Total soluble phenolic content (mg Gallic Acid Equivalent/ g Fresh Weight) of aqueous (WT) and ethanol (12%ET) extracts of Cortland apple peel (A) and pulp (B) with elicitor treatments during 3 months of storage. Error bars represent standard errors (S.E.). Different lowercase letters indicate statistically significant (p ≤ 0.05) differences in total soluble phenolic content between sample (peel vs. pulp) × extraction solvent × post-harvest elicitor treatment interactions separately for each storage time point.
Initially, 1 g/L DPA treatment significantly reduced total soluble phenolic content in both aqueous and ethanol (12%) extracts of Cortland apple peel at 1 month of storage. As DPA treatment counters the imbalance in oxidative metabolism to reduce scald formation, it also potentially downregulates synthesis of oxidation susceptible soluble phenolic compounds and associated expression of key proteins such as lipoxygenase, oxidoreductase, and polyphenol oxidase responsible for enzymatic browning in apple peel (Du et al., 2017). In the current study, after 2 months of storage, no significant reduction of soluble phenolic content was observed with DPA dipping treatments, irrespective of concentrations and extraction methods. In the previous related study (Sarkar et al., 2018), lower superficial scald development was found with 1 and 2 g/L DPA, and 2 g/L OX post-harvest dipping treatments. Therefore, further studies with optimization of OX bio-elicitation dose between 2 and 4 g/L can provide better understanding to advance potential application of this food grade preservative to reduce superficial scald and to improve nutritionally relevant bioactive phenolics in stored apple. Another bio-elicitor (4 g/L COS) targeted in this study also resulted in higher phenolic content in apple peel and pulp, specifically after 1 and 2 months of storage and with ethanol (12%) extraction. The advantage with these food grade OX and COS dipping treatments is that it can be integrated into organic as well as conventional production systems and will not have any issues with potential chemical residues that could be detrimental to human health and the environment. However, more than soluble phenolic content, it is important to understand the impact of bio-elicitation on modulation of individual phenolic compounds of apple peel and pulp during storage.
In apple peel extracts, chlorogenic acid, quercetin, and p-coumaric acid were found as major phenolic compounds, while chlorogenic acid and gallic acid were major phenolics in pulp extracts (Table 1). At 1 and 2 months of storage, p-coumaric acid was also found in select bio-elicited apple pulp extracts. Statistically significant differences in chlorogenic acid content of apple peel and pulp between 3-way (S × E × T; D × S × E; D × S × T; D × E × T) and 4-way interactions (D × S × E × T) were observed (Supplementary Appendix 1; Table 1). Similarly, statistically significant effect of D × E × T interaction on quercetin content of aqueous and ethanol (12%) extracts of apple peel was also found (Supplementary Appendix 1; Table 1). Overall, higher quercetin content was found in ethanol (12%) extracts of apple peel after 1 and 3 months of storage, when compared with same peel extracts before storage. Previously, Barbosa et al. (2012) reported similar phenolic profile in peel and pulp extracts of stored apple. In this current study, higher concentration of individual phenolic acids was also observed in ethanol (12%) extracts, when compared with aqueous extracts of same peel and pulp samples. No statistically significant differences in gallic acid content of apple pulp extracts was observed between D × E × T interaction.
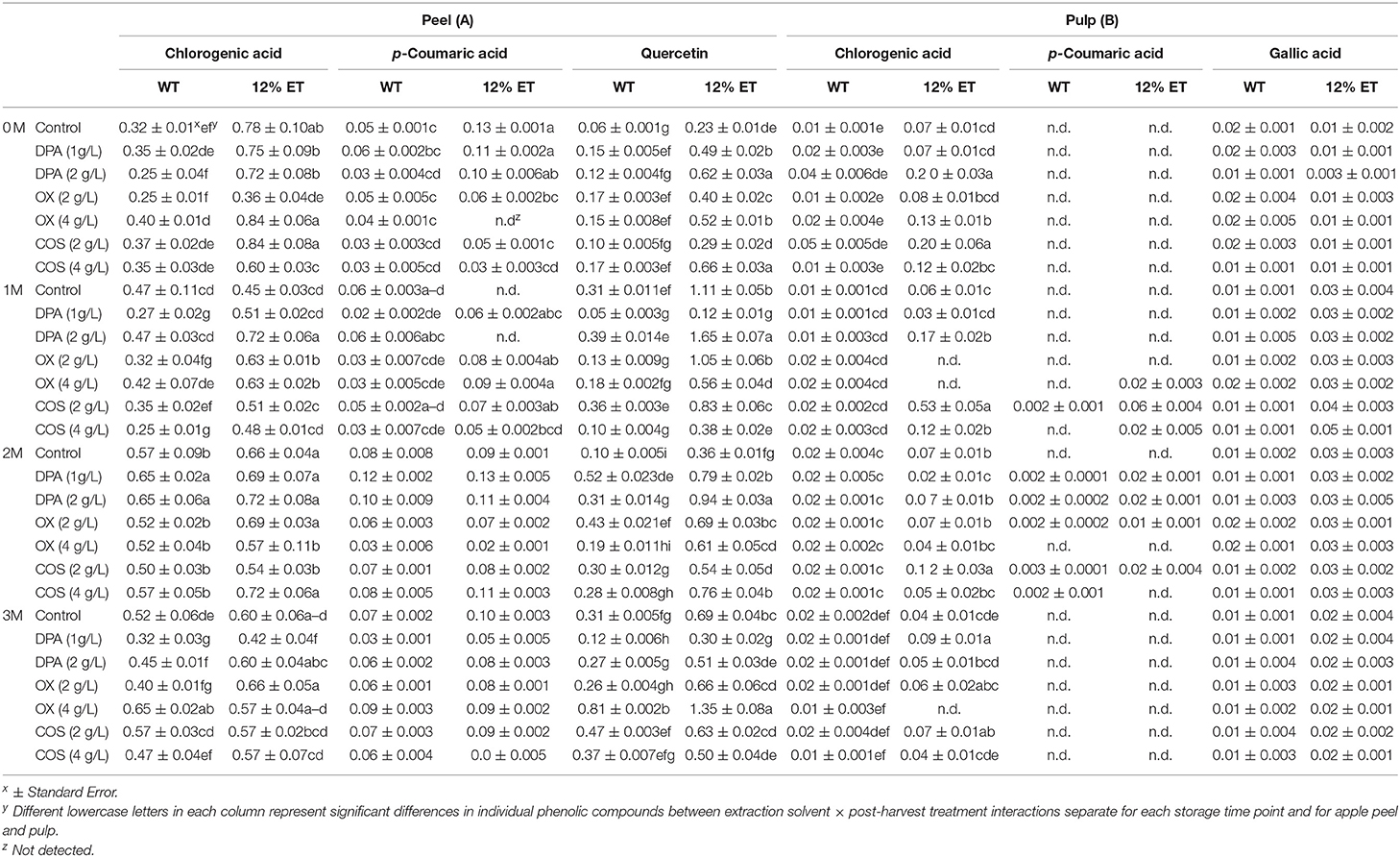
Table 1. Phenolic compound profile (μg/g Fresh Weight) of aqueous (WT) and ethanol (12%ET) extracts of Cortland apple peel (A) and pulp (B) with elicitor treatments (DPA, OX, and COS) during 3 months of storage.
Statistically significant effect of D × E × T interaction on p-coumaric acid content of apple peel was observed (Supplementary Appendix 2). Higher p-coumaric acid content was found in ethanol (12%) extracts of apple peel at 2 months of storage. For apple peel, before storage and at 1 month of storage time, p-coumaric acid content varied significantly between E × T interaction. Post-harvest dipping treatment with food grade 4 g/L OX and 2 g/L COS resulted in higher chlorogenic acid content in ethanol (12%) extracts of apple peel and pulp extracts at 3 months storage time point. Additionally, significantly higher quercetin content was found at 3 months stored apple peel extracts with 4 g/L OX bio-elicitor treatment and with ethanol (12%) extracts. At initial storage time points, 2 g/L DPA treatment resulted in higher quercetin content in ethanol (12%) extracts of apple peel. Therefore, these chemical and elicitor treatments might have modulated the biosynthesis and release of inducible phenolics like chlorogenic acid and quercetin in peel of stored Cortland apple. Such changes in phenolic composition of apple peel during storage is highly relevant for determining their post-harvest preservation as well as human health protective nutritional qualities.
Previously, strong correlation between higher concentration of chlorogenic acid in apple peel and superficial scald development was reported in “Granny Smith” apple (Busatto et al., 2014). However, further study with “Cortland” and “Red Delicious” apple revealed more complex relationship between phenolic biosynthesis and superficial scald development, and specific role of DPA and 1-MCP in modulating metabolic pathways and gene expression, which influences phenolic biosynthesis (Gong et al., 2021). The findings of this recent study (Gong et al., 2021) corroborated with our hypothesis and results of Sarkar et al. (2018), that more than total phenolic content, composition of phenolic compounds, specifically critical level of less oxidized phenolics, and their redox protective function influence superficial scald reduction in stored apples. Such phenolic linked antioxidant activity of apple peel and pulp is also relevant for potential dietary benefits of stored apples, especially in their role to counter chronic oxidative stress and associated metabolic breakdowns of type 2 diabetes.
Total Antioxidant Activity
Overall, total antioxidant activity of apple peel extracts varied between 16 and 86% inhibition, while in apple pulp extracts it ranged between 10 and 52% inhibition (Figures 3A,B). Statistically significant differences in DPPH-based antioxidant activity between apple sample (peel vs. pulp) (S), extraction solvent (water vs. 12% ethanol) (E), elicitor treatments (control, DPA, OX, and COS) (T), storage duration (D) and their respective 2-way (S × E; S × T; D × S; D × E; D × T), 3-way (S × E × T; D × S × E; D × S × T; D × E × T), and 4-way (D × S × E × T) interactions were observed, while effect of extraction × treatment (E × T) interaction on antioxidant activity was not statistically significant (Supplementary Appendix 1; Figures 3A,B). The antioxidant activity of apple peel and pulp extracts decreased with increase in storage time. However, the reduction in antioxidant activity was more significant in control (untreated), when compared to the elicitor treated apples. After 2 months of storage, ethanol (12%) extracts of 2 and 4 g/L COS treated apple peel had significantly higher antioxidant activity. Additionally, after 3 months of storage, OX and COS elicitation treatments with both concentrations (2 & 4 g/L) resulted in higher antioxidant activity in ethanol (12%) extracts of apple peel. Initially, before storage and at 1 month of storage time points, 2 g/L DPA chemical treatment had higher antioxidant activity in ethanol (12%) extracts of apple peel. Aqueous and ethanol (12%) extracts of untreated apple peel and ethanol (12%) extracts of untreated apple pulp had the lowest free radical linked antioxidant activity after 3 months of storage (16% in apple peel and 10% in apple pulp extracts). Overall, antioxidant activity of apple reduced after 2 months of storage irrespective of extraction methods and elicitor treatments. In previous related study (Sarkar et al., 2018), 4 g/L COS dipping treatment stimulated antioxidant enzyme responses in stored apple peel. Therefore, the high antioxidant activity in 4 g/L COS treated apple peel with ethanol (12%) extracts could have resulted from wider distribution of antioxidants in addition to the content of phenolic bioactives. Like COS, 4 g/L OX dipping treatment also showed higher retention of antioxidant activity in aqueous and ethanol (12%) extracts of apple peel and pulp after 3 months of storage, when compared with the sample from control treatment (Figures 3A,B).
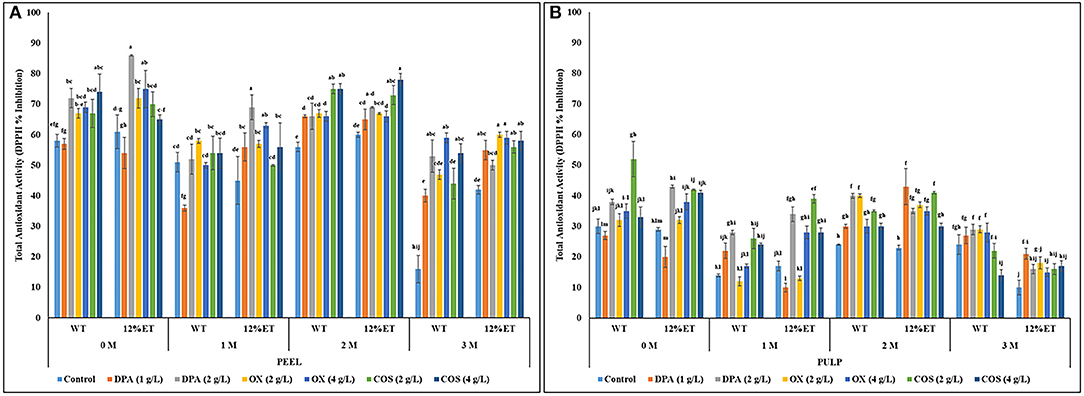
Figure 3. Total antioxidant activity (DPPH-based % inhibition) of aqueous (WT) and ethanol (12%ET) extracts of Cortland apple peel (A) and pulp (B) with elicitor treatments during 3 months of storage. Error bars represent standard errors (S.E.). Different lowercase letters indicate statistically significant (p ≤ 0.05) differences in total antioxidant activity between sample (peel vs. pulp) × extraction solvent × post-harvest elicitor treatment interactions separately for each storage time point.
Previously, Adyanthaya et al. (2009) reported a similar trend in antioxidant protection and role of both antioxidant enzymes and phenolic bioactives in improving post-harvest preservation qualities of stored apple. The higher antioxidant activity in 4 g/L COS and 4 g/L OX treated apple peel extracts (12% ethanol) after 3 months of storage might have relevance in countering oxidation of α-farnesene to conjugated triene (CT) and reducing superficial scald in apple. In addition to scald reduction, improved antioxidant activity is also pertinent for improving redox-linked preservation qualities and shelf-life of long term stored apples and other fruits. Upregulation of endogenous defense response, delayed ripening, extended shelf-life, and enhanced protection against biotic stresses in stored apple with COS post-harvest treatment was previously reported (Shao et al., 2012; Li et al., 2015; Zhang et al., 2020).
Additionally, apple is a good source of dietary antioxidants, and enhanced antioxidant activity with post-harvest elicitor treatments like COS and OX also has relevance in improving nutritionally linked food qualities of stored apple. The dietary benefit of apple is not just related to its antioxidant capacity, but also has wider functional benefits like anti-hyperglycemic and cardio-protective properties (Adyanthaya et al., 2010; Balasuriya and Rupasinghe, 2012; Barbosa et al., 2012).
Anti-hyperglycemic Property
Fruits that are rich sources of phenolic antioxidants, like apple, have previously shown inhibitory potentials against key carbohydrate modulating enzymes (Adyanthaya et al., 2010; Barbosa et al., 2010, 2012). Overall, very high α-glucosidase (~100 % inhibition in undiluted sample) and α-amylase enzymes inhibitory (90% in apple peel and 100 % inhibition in apple pulp extracts) activities were observed in all aqueous and ethanol (12%) extracts of apple peel and pulp. Due to high baseline α-glucosidase and α-amylase enzymes inhibitory activities that would prevent understanding of dose dependent responses of apple extracts, results of ½ and 1/5th diluted samples were assayed and presented (Tables 2, 3). Higher α-glucosidase enzyme inhibitory activity was reported in apple peel extracts, when compared to apple pulp extracts irrespective of extraction methods. Except for the effect of apple sample × extraction (S × E), statistically significant differences in α-glucosidase enzyme inhibitory activity (both in ½ & 1/5th) between apple sample (peel vs. pulp) (S), extraction solvent (water vs. 12% ethanol) (E), elicitor treatments (control, DPA, OX, and COS) (T), storage duration (D) and their respective 2-way (S × T; E × T; D × S; D × E; D × T), 3-way (S × E × T; D × S × E; D × S × T; D × E × T), and 4-way (D × S × E × T) interactions were observed (Supplementary Appendix 2; Table 2). Overall, higher α-glucosidase enzyme inhibitory activity was observed at 1 and 3 months of storage. After 3 months of storage, 4 g/L COS treated apple peel, especially with ethanol (12%) extracts had significantly higher α-glucosidase enzyme inhibitory activity. However, in ethanol (12%) extracts of apple pulp, 4 g/L OX elicitor treatment resulted in significantly higher α-glucosidase enzyme inhibitory activity (Table 3). Same food-grade elicitor treatment also resulted in higher antioxidant activity in ethanol (12%) extracts of apple peel after 3 months of storage.
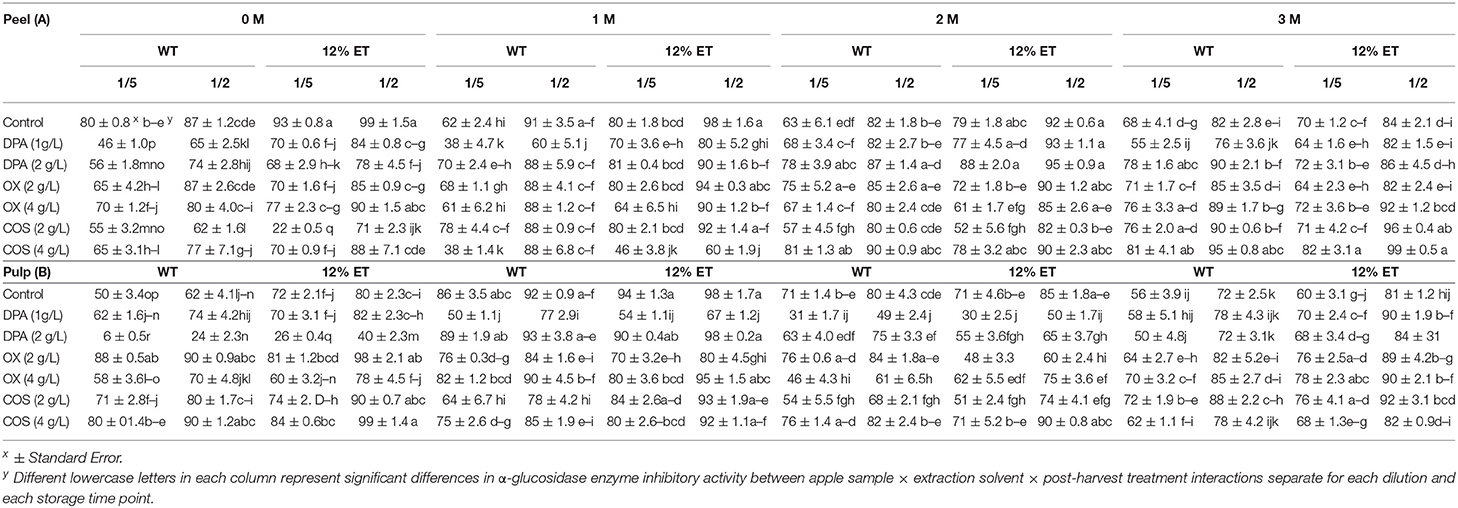
Table 2. α-Glucosidase enzyme inhibitory activity of aqueous (WT) and ethanol (12%ET) extracts of Cortland apple peel (A) and pulp (B) (1/5th and ½ diluted) with elicitor treatments (DPA, OX, and COS) during 3 months of storage.
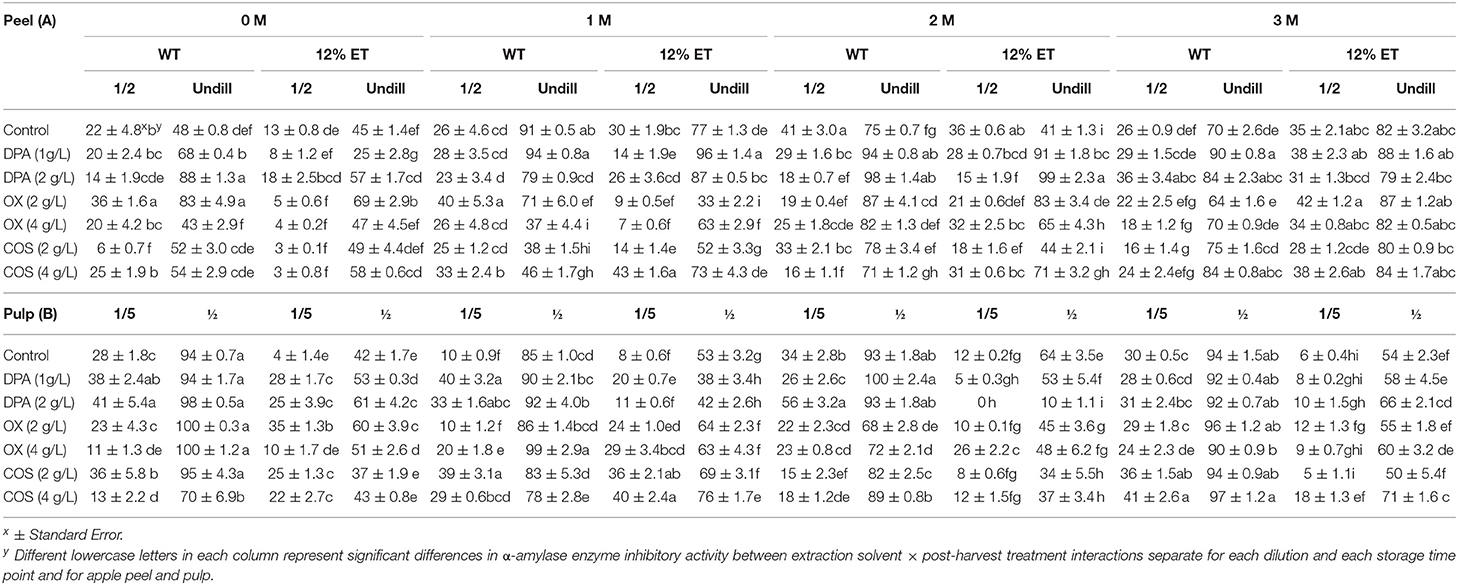
Table 3. α-Amylase enzyme inhibitory activity of aqueous (WT) and ethanol (12%ET) extracts of Cortland apple peel (A) (½ and undiluted) and pulp (B) (1/5th and ½ diluted) with elicitor treatments (DPA, OX, and COS) during 3 months of storage.
Previously, Adyanthaya et al. (2010) reported higher α-glucosidase enzyme inhibitory activity in stored apple peel extracts when compared with pulp extracts. Furthermore, moderate to high positive correlation between α-glucosidase enzyme inhibitory activity and phenolic content was also observed. However, the α-glucosidase enzyme inhibitory activity was cultivar specific as it was maintained at same level in Macintosh and Cortland cultivars over 3 months of storage, while it decreased for Empire and Mutsu cultivars (Adyanthaya et al., 2010). In the current study, α-glucosidase enzyme inhibitory activity of Cortland apple peel and pulp extracts (both aqueous and 12% ethanol) did not decrease significantly with increased storage time.
The results of the current study suggested that post-harvest dipping treatment of 4 g/L COS and 4 g/L OX might have better potential in sustaining higher α-glucosidase enzyme inhibitory activity and antioxidant activity in post-harvest stored apples. Changes in α-glucosidase enzyme inhibitory activity with post-harvest elicitor treatments and its's link to phenolic content and antioxidant activity is important to understand wider food qualities and potential health benefits of bio-elicited and stored apple.
Overall, statistically significant differences in α-amylase enzyme inhibitory activity (½ diluted) between D × S × E × T interaction were observed (Supplementary Appendix 1). For apple peel (undiluted) and pulp (1/5th diluted) extracts, statistically significant effect of D × E × T interaction on α-amylase enzyme inhibitory activity was also observed (Table 3). In both aqueous and ethanol (12%) extracts of apple peel, higher α-amylase enzyme inhibitory activity was found after 3 months of storage, while ethanol (12%) extracts of apple pulp (1/5th diluted) had higher α-amylase enzyme inhibitory activity before storage and at 1 month of storage. Among samples, higher α-amylase enzyme inhibitory activity was observed in apple pulp extracts when compared to apple peel extracts irrespective of extraction methods. When compared between extraction solvents, aqueous extracts had higher α-amylase enzyme inhibitory activity than ethanol extracts (12%). Previously, Barbosa et al. (2012) also reported higher α-amylase inhibitory activity in apple pulp than peel in both aqueous and ethanol (12%) extracts for all varieties in long-term stored apples. Furthermore, a positive correlation was reported between phenolic content and α-amylase inhibitory in long-term stored apple. In undiluted peel extracts (both aqueous and 12% ethanol), 1 g/L DPA treatment resulted in higher α-amylase enzyme inhibitory activity at 1 and 3 months of storage. However, for aqueous pulp extracts, 2 and 4 g/L OX and 4 g/ L COS treated sample had higher mean α-amylase enzyme inhibitory activity, specifically after 3 months of storage. Additionally, α-amylase enzyme inhibitory activity of apple peel extracts, especially in 12% ethanol extracts slightly increased over storage time, while opposite trend was observed in apple pulp extracts. Previously, inhibition of α-amylase enzyme by chlorogenic acid derived from apple was reported (Li et al., 2019). Similarly, inhibition of both α-amylase and α-glucosidase enzymes by quercetin was also observed (Oboh et al., 2015). Therefore, phenolic compounds like chlorogenic acid and quercetin found in apple peel might have played role in the high α-amylase and α-glucosidase enzymes inhibitory activities of stored apple extracts in the current study.
Conclusion
In this current study, higher retention of phenolic bioactives, which also resulted in sustained antioxidant and anti-hyperglycemic functional benefits in ethanol (12%) extracts of Cortland apple peel and pulp was observed with food-grade OX (4 g/L) and COS (4 g/L) elicitor treatments. However, phenolic-linked antioxidant and anti-hyperglycemic functional activity of apple peel and pulp varied significantly between sample (peel vs. pulp) × extraction solvent × post-harvest elicitor treatment × duration interactions. Overall, apple peel had significantly higher phenolic content and associated antioxidant and α-glucosidase enzyme inhibitory activity, while high α-amylase enzyme inhibitory activity was observed in apple pulp. Furthermore, bio-elicitation strategy with food grade elicitors like OX (4 g/L) and COS (4 g/L) as post-harvest dipping treatments can be recruited to sustain the anti-hyperglycemic functional benefits in stored apples. Results of this study indicate that well-preserved Cortland apple is a good dietary source that can be targeted as part of complimentary food support strategies to manage post-prandial glucose homeostasis targeting type 2 diabetes benefits. Future studies with more apple cultivars and longer storage time (more than 3 months) are required to better understand the impact of these bio-elicitors as food grade preservatives for reducing post-harvest storage loss and for improving human health targeted phenolic bioactive qualities in stored apple and other fruits.
Data Availability Statement
The raw data supporting the conclusions of this article will be made available by the authors, without undue reservation.
Author Contributions
CA, DS, DG, and KS: conceptualization and methodology. CA and DS: data curation, formal analysis, and writing—original draft. CA, DS, and DG: investigation. CA, DS, and KS: project administration and writing—review and editing. All authors contributed to the article and approved the submitted version.
Conflict of Interest
The authors declare that the research was conducted in the absence of any commercial or financial relationships that could be construed as a potential conflict of interest.
Publisher's Note
All claims expressed in this article are solely those of the authors and do not necessarily represent those of their affiliated organizations, or those of the publisher, the editors and the reviewers. Any product that may be evaluated in this article, or claim that may be made by its manufacturer, is not guaranteed or endorsed by the publisher.
Acknowledgments
We wish to thank the Cold Spring Orchard and Plant, Soil, and Insect Sciences facility of the University of Massachusetts at Belchertown, MA, where apples were grown, harvested, and stored for this study.
Supplementary Material
The Supplementary Material for this article can be found online at: https://www.frontiersin.org/articles/10.3389/fsufs.2021.709384/full#supplementary-material
References
Adyanthaya, I., Kwon, Y. I., Apostolidis, E., and Shetty, K. (2009). Apple postharvest preservation is linked to phenolic content and superoxide dismutase activity. J. Food Biochem. 33, 535–556. doi: 10.1111/j.1745-4514.2009.00236.x
Adyanthaya, I., Kwon, Y. I., Apostolidis, E., and Shetty, K. (2010). Health benefits of apple phenolics from postharvest stages for potential type 2 diabetes management using in vitro models. J. Food Biochem. 34, 31–49. doi: 10.1111/j.1745-4514.2009.00257.x
Balasuriya, N., and Rupasinghe, H. V. (2012). Antihypertensive properties of flavonoid-rich apple peel extract. Food Chem. 135, 2320–2325. doi: 10.1016/j.foodchem.2012.07.023
Barbosa, A. C. L., Pinto, M. D. S., Sarkar, D., Ankolekar, C., Greene, D., and Shetty, K. (2010). Varietal influences on antihyperglycemia properties of freshly harvested apples using in vitro assay models. J. Med. Food 13, 1313–1323. doi: 10.1089/jmf.2009.0273
Barbosa, A. C. L., Pinto, M. D. S., Sarkar, D., Ankolekar, C., Greene, D., and Shetty, K. (2012). Influence of varietal and pH variation on antihyperglycemia and antihypertension properties of long-term stored apples using in vitro assay models. J. Food Biochem. 36, 479–493. doi: 10.1111/j.1745-4514.2011.00554.x
Bondonno, N. P., Bondonno, C. P., Ward, N. C., Hodgson, J. M., and Croft, K. D. (2017). The cardiovascular health benefits of apples: whole fruit vs. isolated compounds. Trends Food Sci. Technol. 69, 243–256. doi: 10.1016/j.tifs.2017.04.012
Bordonaba, J. G., Matthieu-Hurtiger, V., Westercamp, P., Coureau, C., Dupille, E., and Larrigaudière, C. (2013). Dynamic changes in conjugated trienols during storage may be employed to predict superficial scald in ‘Granny Smith' apples. LWT-Food Sci. Technol. 54, 535–541. doi: 10.1016/j.lwt.2013.06.025
Busatto, N., Farneti, B., Commisso, M., Bianconi, M., Iadarola, B., Zago, E., et al. (2018). Apple fruit superficial scald resistance mediated by ethylene inhibition is associated with diverse metabolic processes. Plant J. 93, 270–285. doi: 10.1111/tpj.13774
Busatto, N., Farneti, B., Tadiello, A., Vrhovsek, U., Cappellin, L., Biasioli, F., et al. (2014). Target metabolite and gene transcription profiling during the development of superficial scald in apple (Malus x domestica Borkh). BMC Plant Biol. 14, 1–13. doi: 10.1186/s12870-014-0193-7
Chai, Y., Li, A., Wai, S. C., Song, C., Zhao, Y., Duan, Y., et al. (2020). Cuticular wax composition changes of 10 apple cultivars during postharvest storage. Food Chem. 324:126903. doi: 10.1016/j.foodchem.2020.126903
Chun, O. K., Kim, D. O., Smith, N., Schroeder, D., Han, J. T., and Lee, C. Y. (2005). Daily consumption of phenolics and total antioxidant capacity from fruit and vegetables in the American diet. J. Sci. Food Agric. 85, 1715–1724. doi: 10.1002/jsfa.2176
de Oliveira Raphaelli, C., dos Santos Pereira, E., Camargo, T. M., Vinholes, J., Rombaldi, C. V., Vizzotto, M., et al. (2019). Apple phenolic extracts strongly inhibit α-glucosidase activity. Plant Foods Hum. Nutri. 74, 430–435. doi: 10.1007/s11130-019-00757-3
Denver, S., and Jensen, J. D. (2014). Consumer preferences for organically and locally produced apples. Food Qual. Prefer. 31, 129–134. doi: 10.1016/j.foodqual.2013.08.014
Du, L., Song, J., Palmer, L. C., Fillmore, S., and Zhang, Z. (2017). Quantitative proteomic changes in development of superficial scald disorder and its response to diphenylamine and 1-MCP treatments in apple fruit. Postharvest Biol. Technol. 123, 33–50. doi: 10.1016/j.postharvbio.2016.08.005
Endrizzi, I., Torri, L., Corollaro, M. L., Dematt,è, M. L., Aprea, E., Charles, M., et al. (2015). A conjoint study on apple acceptability: sensory characteristics and nutritional information. Food Qual. Prefer. 40, 39–48. doi: 10.1016/j.foodqual.2014.08.007
Francini, A., and Sebastiani, L. (2013). Phenolic compounds in apple (Malus x domestica Borkh.): compounds characterization and stability during postharvest and after processing. Antioxidants 2, 181–193. doi: 10.3390/antiox2030181
Garrido Assis, O. B., and de Britto, D. (2011). Evaluation of the antifungal properties of chitosan coating on cut apples using a non-invasive image analysis technique. Polym. Int. 60, 932–936. doi: 10.1002/pi.3039
Gong, Y., Song, J., Palmer, L. C., Vinqvist-Tymchuk, M., Fillmore, S., Toivonen, P., et al. (2021). Tracking the development of the superficial scald disorder and effects of treatments with diphenylamine and 1-MCP using an untargeted metabolomic approach in apple fruit. Food Chem. Mol. Sci. 21:100022. doi: 10.1016/j.fochms.2021.100022
Hodgson, J. M., Prince, R. L., Woodman, R. J., Bondonno, C. P., Ivey, K. L., Bondonno, N., et al. (2016). Apple intake is inversely associated with all-cause and disease-specific mortality in elderly women. Br. J. Nutrit. 115, 860–867. doi: 10.1017/S0007114515005231
Hua, C., Zhao, J., Wang, H., Chen, F., Meng, H., Chen, L., et al. (2018). Apple polyphenol relieves hypoxia-induced pulmonary arterial hypertension via pulmonary endothelium protection and smooth muscle relaxation: In vivo and in vitro studies. Biomed. Pharmacotherap. 107, 937–944. doi: 10.1016/j.biopha.2018.08.080
Hyson, D. A. (2011). A comprehensive review of apples and apple components and their relationship to human health. Adv. Nutri. 2, 408–420. doi: 10.3945/an.111.000513
Iñigo-Nuñez, S., Herreros, M. A., Encinas, T., and Gonzalez-Bulnes, A. (2010). Estimated daily intake of pesticides and xenoestrogenic exposure by fruit consumption in the female population from a Mediterranean country (Spain). Food Control 21, 471–477. doi: 10.1016/j.foodcont.2009.07.009
Jakobek, L., Ištuk, J., Buljeta, I., Voća, S., Žlabur, J. Š., and Babojelić, M. S. (2020). Traditional, indigenous apple varieties, a fruit with potential for beneficial effects: their quality traits and bioactive polyphenol contents. Foods 9:52. doi: 10.3390/foods9010052
Jha, S. N., Rai, D. R., and Shrama, R. (2012). Physico-chemical quality parameters and overall quality index of apple during storage. J. Food Sci. Technol. 49, 594–600. doi: 10.1007/s13197-011-0415-z
Karagiannis, E., Tanou, G., Scossa, F., Samiotaki, M., Michailidis, M., Manioudaki, M., et al. (2020). Systems-based approaches to unravel networks and individual elements involved in apple superficial scald. Front. Plant Sci. 11:8. doi: 10.3389/fpls.2020.00008
Kraśniewska, K., Gniewosz, M., Synowiec, A., Przyby,ł, J. L., Baczek, K., and Weglarz, Z. (2014). The use of pullulan coating enriched with plant extracts from Satureja hortensis L. to maintain pepper and apple quality and safety. Postharvest Biol. Technol. 90, 63–72. doi: 10.1016/j.postharvbio.2013.12.010
Kwon, Y. I. I., Vattem, D. A., and Shetty, K. (2006). Evaluation of clonal herbs of Lamiaceae species for management of diabetes and hypertension. Asia Pac. J. Clin. Nutr. 15:107.
Li, D., Sun, L., Yang, Y., Wang, Z., Yang, X., Zhao, T., et al. (2019). Young apple polyphenols postpone starch digestion in vitro and in vivo. J. Funct. Foods 56, 127–135. doi: 10.1016/j.jff.2019.03.009
Li, H., Wang, Y., Liu, F., Yang, Y., Wu, Z., Cai, H., et al. (2015). Effects of chitosan on control of postharvest blue mold decay of apple fruit and the possible mechanisms involved. Sci. Hortic. 186, 77–83. doi: 10.1016/j.scienta.2015.02.014
Li, L., Song, W., Shen, C., Dong, Q., Wang, Y., and Zuo, S. (2020). Active packaging film containing oregano essential oil microcapsules and their application for strawberry preservation. J. Food Process. Preserv. 44:e14799 doi: 10.1111/jfpp.14799
Lu, X. G., Ma, Y. P., and Liu, X. H. (2012). Effects of maturity and 1-MCP treatment on postharvest quality and antioxidant properties of ‘Fuji'apples during long-term cold storage. Horticult. Environ. Biotechnol. 53, 378–386. doi: 10.1007/s13580-012-0102-7
Lurie, S., and Watkins, C. B. (2012). Superficial scald, its etiology and control. Postharvest Biol. Technol. 65, 44–60. doi: 10.1016/j.postharvbio.2011.11.001
McCue, P., Kwon, Y. I., and Shetty, K. (2005). Anti-amylase, anti-glucosidase and anti-angiotensin i-converting enzyme potential of selected foods. J. Food Biochem. 29, 278–294. doi: 10.1111/j.1745-4514.2005.00020.x
McCue, P. P., and Shetty, K. (2004). Inhibitory effects of rosmarinic acid extracts on porcine pancreatic amylase in vitro. Asia Pac. J. Clin. Nutr. 13:1.
Moggia, C., León, M. M., Pereira, M., Yuri, J. A., and Lobos, G. A. (2010). Effect of DPA and 1-MCP on chemical compounds related to superficial scald of Granny Smith apples. Spanish J. Agricult. Res. 1, 178–187. doi: 10.5424/sjar/2010081-1157
Musacchi, S., and Serra, S. (2018). Apple fruit quality: overview on pre-harvest factors. Sci. Hortic. 234, 409–430. doi: 10.1016/j.scienta.2017.12.057
Oboh, G., Ademosun, A. O., Ayeni, P. O., Omojokun, O. S., and Bello, F. (2015). Comparative effect of quercetin and rutin on α-amylase, α-glucosidase, and some pro-oxidant-induced lipid peroxidation in rat pancreas. Comp. Clin. Path. 24, 1103–1110. doi: 10.1007/s00580-014-2040-5
Porat, R., Lichter, A., Terry, L. A., Harker, R., and Buzby, J. (2018). Postharvest losses of fruit and vegetables during retail and in consumers' homes: quantifications, causes, and means of prevention. Postharvest Biol. Technol. 139, 135–149. doi: 10.1016/j.postharvbio.2017.11.019
Qi, H., Hu, W., Jiang, A., Tian, M., and Li, Y. (2011). Extending shelf-life of fresh-cut ‘Fuji'apples with chitosan-coatings. Innov. Food Sci. Emerg. Technol. 12, 62–66. doi: 10.1016/j.ifset.2010.11.001
Rana, S., and Bhushan, S. (2016). Apple phenolics as nutraceuticals: assessment, analysis and application. J. Food Sci. Technol. 53, 1727–1738. doi: 10.1007/s13197-015-2093-8
Sabban-Amin, R., Feygenberg, O., Belausov, E., and Pesis, E. (2011). Low oxygen and 1-MCP pretreatments delay superficial scald development by reducing reactive oxygen species (ROS) accumulation in stored ‘Granny Smith' apples. Postharvest Biol. Technol. 62, 295–304. doi: 10.1016/j.postharvbio.2011.06.016
Sarkar, D., Ankolekar, C., Greene, D., and Shetty, K. (2018). Natural preservatives for superficial scald reduction and enhancement of protective phenolic-linked antioxidant responses in apple during post-harvest storage. J. Food Sci. Technol. 55, 1767–1780. doi: 10.1007/s13197-018-3090-5
Schiavano, G. F., De Santi, M., Brandi, G., Fanelli, M., Bucchini, A., Giamperi, L., et al. (2015). Inhibition of breast cancer cell proliferation and in vitro tumorigenesis by a new red apple cultivar. PLoS ONE 10:e0135840. doi: 10.1371/journal.pone.0135840
Shao, X. F., Tu, K., Tu, S., and Tu, J. (2012). A combination of heat treatment and chitosan coating delays ripening and reduces decay in “Gala” apple fruit. J. Food Qual. 35, 83–92. doi: 10.1111/j.1745-4557.2011.00429.x
Shetty, K., Curtis, O. F., Levin, R. E., Witkowsky, R., and Ang, W. (1995). Prevention of Vitrification Aßociated with in vitro Shoot Culture of Oregano (Origanum vulgare) by Pseudomonas spp. J. Plant Physiol. 147, 447–451. doi: 10.1016/S0176-1617(11)82181-4
Sultanbawa, Y. (2014). Plant extracts as natural antimicrobials in food preservation. Microbial Food Safety Preserv. Techniq. 17, 373–382. doi: 10.1201/b17465-23
USDA (2020). Fresh Apples, Grapes, and Pears: World Markets and Trade. Foreign Agriculture Service, United States Department of Agriculture. Available online at: https://apps.fas.usda.gov/psdonline/circulars/fruit.pdf (accessed March 2, 2021).
Vinson, J. A., Su, X., Zubik, L., and Bose, P. (2001). Phenol antioxidant quantity and quality in foods: fruits. J. Agric. Food Chem. 49, 5315–5321. doi: 10.1021/jf0009293
Keywords: antioxidant, antihyperglycemia, phenolic bioactives, postharvest preservation, stored apples
Citation: Ankolekar C, Sarkar D, Greene D and Shetty K (2021) Using Biological Elicitation to Improve Type 2 Diabetes Targeted Food Quality of Stored Apple. Front. Sustain. Food Syst. 5:709384. doi: 10.3389/fsufs.2021.709384
Received: 13 May 2021; Accepted: 12 October 2021;
Published: 05 November 2021.
Edited by:
Ana Sanches Silva, Instituto Nacional Investigaciao Agraria e Veterinaria (INIAV), PortugalReviewed by:
Robert David Hall, Wageningen University and Research, NetherlandsRebogile Mphahlele, Agricultural Research Council of South Africa (ARC-SA), South Africa
Copyright © 2021 Ankolekar, Sarkar, Greene and Shetty. This is an open-access article distributed under the terms of the Creative Commons Attribution License (CC BY). The use, distribution or reproduction in other forums is permitted, provided the original author(s) and the copyright owner(s) are credited and that the original publication in this journal is cited, in accordance with accepted academic practice. No use, distribution or reproduction is permitted which does not comply with these terms.
*Correspondence: Kalidas Shetty, a2FsaWRhcy5zaGV0dHkmI3gwMDA0MDtuZHN1LmVkdQ==