- Faculty of Renewable Natural Resources, Kwame Nkrumah University of Science and Technology, Kumasi, Ghana
Increasing demand on water resources, reduced land water availability, and concerns over food security have spurred the evolution of many innovative and complex food production. An aquaponic system is a productive, innovative, and sustainable fish and vegetable production system that is revolutionizing agriculture in the face of drought, soil fertility losses, and climate change. Aquaponics, as an advanced aquaculture-agriculture system, is expected to improve food security in developing countries. However, as an emerging technology, there is very limited information on the system in Africa. Questions about the ecological and socio-economic sustainability of aquaponics are answered in this comprehensive review. This review considers aquaponics projects in Africa, categorizes the technology by evidences of their effectiveness, fish and plant yields, and juxtaposes the technology within best-use practices to make recommendations that will inform evidence-based policymaking. It also maps the present spatial adoption of the technology in sub-Saharan Africa and highlights the system's contribution to improving food security on the continent. Egypt and South Africa are countries where aquaponics is emerging and being adopted at faster rates and contributing to food security. In West Africa, significantly lower net-discounted benefit-cost ratios were realized when aquaponics systems were constructed using imported materials compared to using locally available materials. Despite aquaponics systems generally having higher start-up costs currently, its potential to be economically viable when undertaken with local materials is very high.
Introduction
One of the greatest challenges facing the world is how to meet the nutritional needs of a growing human population that is projected to hit 10 billion by 2050. To meet the additional food demands imposed by the nearly 30% population increase, global food production has to increase by as much as 50% (FAO, 2017). Food production will, however, be challenged by factors such as climate change, pollution and degradation of arable lands (Goddek et al., 2019a). According to the projections of Bajželj et al. (2014), despite the development of high yielding crop varieties and enhanced food production methods, current food production trends will not meet the projected global food demand by 2050. The situation will further be exacerbated by reductions in agricultural lands. Between 1970 and 2013, global agricultural lands have decreased by more than 50% (Goddek et al., 2019a). By the end of the 21st century, climate change alone is projected to account to for up to 18% of arable land losses in Africa (Zhang and Cai, 2011) which will negatively affect the
continent's already dire food insecurity situation. These challenges to food production require innovation in food production systems, methods and practices, given that a billion people are already chronically malnourished (Godfray et al., 2010).
Aquaponics is a sustainable food production system that uses circular economy concepts and a biomimetic natural system to minimize input and waste. It is an industrious mechanism that incorporates impeccably with the sustainable growth of intensive agriculture (Tyson et al., 2011; Vermeulen and Kamstra, 2012; Joly et al., 2015). Aquaponics combines two primarily productive systems: recirculating aquaculture system (RAS) and hydroponic cultivation. Recirculating aquaculture involves the farming of fish and crustaceans in a tank, while hydroponic cultivation involves the cultivation of vegetables in a medium other than soil. Aquaponics emerges as a key technology with potential to transform agriculture and enhance food security in the wake of climate change, particularly in arid regions (Conijn et al., 2018).
Aquaponics in one form or another has been practiced for centuries in several countries but the technology still remains less popular compared to traditional food production methods and is largely practiced on small scales by individuals (Junge et al., 2017). The technology is, however, rapidly transforming from a largely backyard technology into industrial-scale production due to practical improvements in design and practice which have significantly increased both fish and crop output capacities and production efficiencies (Bernstein, 2011). Improvements in design and functions have transformed aquaponic systems beyond a water-reuse innovation into an efficient energy and wastewater recycling system (Goddek et al., 2019b). The technology is usually proffered as a solution for efficiently using marginal lands in urban areas for food production. Although the technology has been recommended as a means of addressing some of the food insecurity and nutrition-related challenges in Africa, its adoption across the continent is still very low. This chapter focuses on the adoption of aquaponics technology and reviews published information to highlight the potential of the technology in contributing to food security issues.
Aquaponics Technology in Africa
Aquaponics systems are fairly new to countries in Africa and as expected, there are a few published information (including gray literature) on the subject obtained through online search on Google Scholar and Scopus and also by an international survey conducted in 2014 where only a single response each was received from Ghana and South Africa (Love et al., 2015). The bubble plot for the number of aquaponic publications in different African countries is shown in Figure 1. A total of 82 publications on aquaponics were found from 15 African countries. Egypt, South Africa and Kenya (23, 20, and 14 publications, respectively) are the countries that appear to have widely adopted the technology in Africa. Except for Nigeria that had nine publications on aquaponics, the remaining countries had between 1 and 3 publications. The low adoption of aquaponic technology can be linked to low penetration of innovative fish production systems such as recirculating aquaculture systems that are an integral part of aquaponic systems. Adoption rates (based on the number of publications found) appear to correlate with the scale and development of aquaculture in the different countries. Egypt, Nigeria, Kenya and South Africa are major contributors to the continent's aquaculture production. Water scarcity could also be driving widespread adoption of aquaponics in an arid country like Egypt. In arid regions, circulation of water between the RAS and hydroponics units can result in a remarkable water re-use efficiency rate of 95–99% (Dalsgaard et al., 2013). The following subsections extensively review the adoption of aquaponics on a sub-regional basis across Africa. Although the review covers African sub-regions, the narrative in some instances may be skewed toward the countries that have wide-scale adoption of aquaponics.
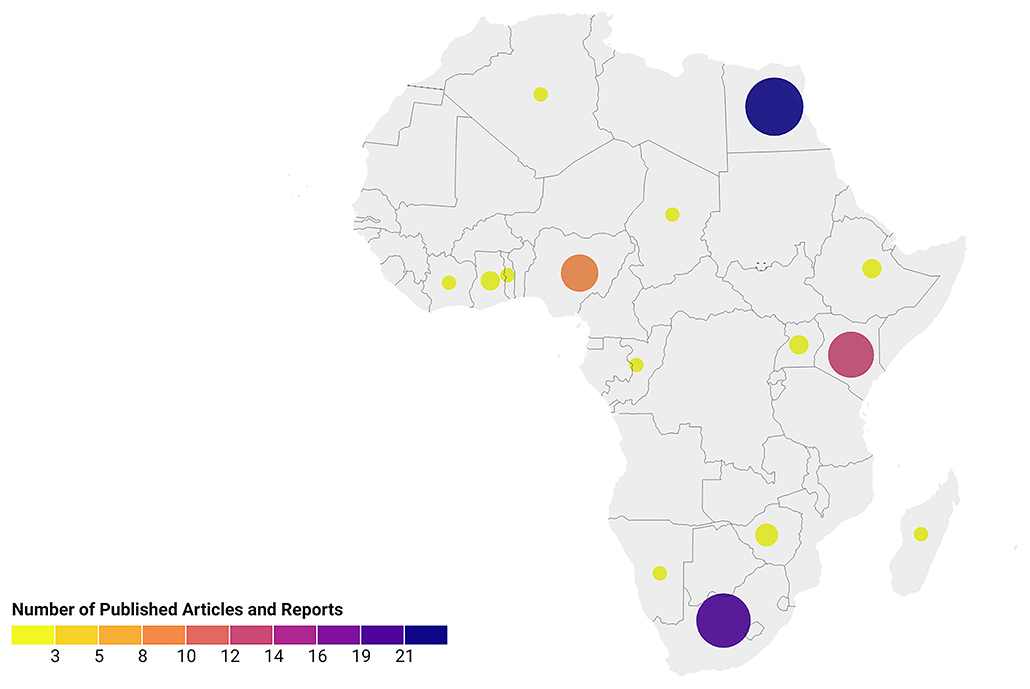
Figure 1. Bubble plot showing the number of aquaponic publications in different African countries (bubble color and size indicate the number of studies in each highlighted country).
North Africa
Water scarcity and food insecurity, combined with human population growth, pose serious challenges to North Africa's agricultural sector. Egypt's population is expected to increase from around 80 million today to around 97 million in 2025 (UN Population data), and current per capita water supply is expected to drop by 40% by 2025 (http://www.fao.org/nr/water/aquastat). According to McCarl et al. (2015), the effect of climate change on Egyptian agriculture might be very devastating. Most of the publications on aquaponics in the regions have thus touted the expansion of the technology as critical to address water scarcity issues (Essa et al., 2008; Soethoudt et al., 2016; El-Essawy et al., 2019). As opposed to traditional agriculture, aquaponics has higher economic potential and less negative effects on groundwater resources (El-Essawy et al., 2019). Commercial aquaponics units that have been coupled with greenhouses to improve crop production have been established in Egypt to grow Nile tilapia (Oreochromis niloticus) and olives. The feedbacks on the quality and sizes of fish produced in aquaponic units in Egypt are overwhelmingly positive and the reputation of the technology being a clean process has resulted in it rapidly gaining traction in the retail and wholesale markets (El-Essawy et al., 2019). Comparative studies on vegetable yield in the two commonly used aquaponics systems in Egypt, the deep-water culture (DWC) and sand-bed systems indicate that yields are about 30% higher in the DWC systems although this system uses lower water reuse efficiency (Salem, 2019).
While aquaponics has higher capital and operating costs than traditional agriculture in the short term, it is more profitable in the long run and saves more than 90% of the water wasted by conventional farming techniques in Dalsgaard et al., 2013 and El-Essawy et al., 2019. Profits from aquaponics systems after deducting operational expenditures are also about 30-fold higher than conventional agriculture due to the more efficient use of space and the additional income from the fish (El-Essawy et al., 2019). There is ongoing research to develop and build affordable aquaponics systems that use locally available recyclable materials to lower capital costs. Intermediate bulk containers (1,000 L capacity) are commonly used as fish culture and water treatment units in most of the aquaponics systems in Egypt as a cost-saving measure. While aquaponics has more economic and environmental benefits than drawbacks, there are technical and social limitations in addition to the high start-up investments that must be considered for it to be widely adopted in the arid regions of North Africa. According to Soethoudt et al. (2016), the development of aquaponics in Egypt is constrained by limited experience, technical expertise and scalability issues. Given the high start-up costs, it has been recommended that more research efforts should be targeted at developing units that can easily be adopted by small farms for the production of fish and crops that constitute a significant portion of local diets such as tomatoes (Soethoudt et al., 2016).
Southern Africa
The idea of aquaponics may be useful to southern African countries that have limited agricultural production resources (water and fertile croplands), high urbanization rate and exponentially increasing urban poverty (Mchunu et al., 2018). In South Africa, aquaponics has been viewed as having the potential to rehabilitate degraded coal mining sites (Botha, 2014). Another factor that has encouraged the adoption of aquaponics in South Africa is the near-collapse of freshwater pond aquaculture because outside environmental conditions do not allow cultured fish to independently establish economically viable populations (Swap et al., 2002). Water temperatures often fall below the tolerable minima required by the common aquaculture species on the continent including the Nile tilapia (Van der Waal, 2000). For year-round fish production, aquaponics installation in Southern Africa will require temperature control mechanisms or adjustments to efficiently operate. Heating costs in aquaculture generally contribute significantly to production costs.
Aquaponics is, however, an emerging but rapidly evolving practice in South Africa (Love et al., 2014, 2015; Mchunu et al., 2018). Fish stocking densities usually range from 15 to 19 kgm−3, which is low and suggests small-scale or subsistence nature of most of the systems (Sace and Fitzsimmons, 2013). The wide-scale adoption of these small units is, however, very critical in addressing some of the food security issues in the country. Magazines and news articles on aquaponics in South Africa are very common and highlight the potential of the technology on hobby, small-scale and commercial levels. Commercial aquaponics in South Africa has an intensive outlook and usually adopts fish stocking densities between 60 and 200 kgm−3 in 5,000 m3 tanks (FAO, 2014). The increased interest in aquaponics could also be because of the recent drought, food safety concerns, land reforms and increasing population size (Van der Waal, 2000; Faber et al., 2011; Mabhaudhi et al., 2013; Mchunu et al., 2018).
Most aquaponics operations started as aquaculture farms and then evolved to aquaponics (El-Essawy et al., 2019) and this is true for most of the South Africa establishments. Commercial aquaponics practitioners in South Africa have very good access to vegetable and fish markets (Mchunu et al., 2018). Vegetable are, however, harvested at higher frequencies of every 1–3 months compared to fish which are usually harvested at periods >6 months (Love et al., 2014, 2015). The plant component of most aquaponic system in South Africa comprise mostly salad greens, lettuce, basil, herbs, pepper, cucumber, beans and peas, tomatoes, carrots flowers and ornamental plants. While fruity vegetables have a higher economic value and return, most farmers raised leafy vegetables (salad greens, lettuce, basal, and herbs). Because of their low agronomic requirements, leafy vegetables use fewer nutrients than fruity vegetables (Rakocy et al., 2004) and grow fast when all nutrients are supplied (FAO, 2014). Moreover, leafy vegetables can be raised in higher density (up to 30 plants m−2) than fruity vegetables, which usually grown at a maximum density of 8 plants m−2 (USAID, 2013; FAO, 2014). These plants are grown in growth medium be, nutrient film technique and deep water culture production systems. Fish sales from aquaponics are quite popular because of perceived higher product quality, growing food insecurity and dietary lifestyle changes (Faber et al., 2011; Mchunu et al., 2019). Species of tilapia and trout are the commonly raised fish in typical South African aquaponic systems (Mchunu et al., 2018). Other less popular fish include catfishes, bass, bluegill, and some ornamental species. The Nile tilapia is the most dominant species cultured in South African aquaponics (FAO, 2014; Love et al., 2014, 2015). Tilapia grows well in a recirculating tank culture with higher tolerance to fluctuating water conditions such as pH, temperature, oxygen, and dissolved solids; tilapia can tolerate a wide range of water temperatures (9–42.5°C), dissolved oxygen as low as 0.1 mgL−1, and unionized ammonia concentration of 2.4 mgL−1 (D'Amato et al., 2007). The lower percentages for other fish species can be attributed to limiting environmental conditions in South Africa. Fish stocking densities commonly range between 15 and 19 kgm−3 although in some intensive systems densities can be >50 kgm−3 (Mchunu et al., 2018).
The nationwide survey of aquaponics users in South Africa by Mchunu et al. (2018) revealed that practitioner experiences ranged from 1 to 10 years and highlighted aquaponics as a new technology in the country. Most systems were self-constructed by the operators, sometimes with extension service assistance from the Department of Agriculture. Growth medium beds are the dominant method of crop production in South Africa (Mchunu et al., 2018) because it does not require setting up independent biofilters to remove excess nutrients from the water as the bed itself acts as a biofilter (Hu et al., 2015). Nutrient film techniques and the deep-water culture systems usually require independent biofiltration systems to facilitate nitrification, which add to the installation costs. Gravel media are the dominant method of crop production, as it is easily accessible and readily available compared to other media (Sikawa and Yakupitiyage, 2010). The flood and drain system is a cheap, simple, and easy to use method to return dissolved nutrients to the rearing tank, while giving plants enough time to take up the nutrients (FAO, 2014).
Feasibility studies have been conducted in Namibia to assess the viability of setting up aquaponics systems to enhance food security, sustainability, income generation, and as an educational resource (Rego et al., 2020). About 430,000 Namibians are reported to be food insecure as almost 70% of food is imported from South Africa (FAO, 2020). In this Namibian case, aquaponics is one of the most efficient way to combat food security (Rego et al., 2020). Aquaponic and hydroponic systems use water effectively to provide sustainable agriculture. Besides food security, aquaponics will be a sustainable agricultural system in Namibia during droughts (Rego et al., 2020). The trial runoff the prototype system indicated that tilapia and koi are the best suited fish species for aquaponics development in Namibia (Rego et al., 2020). Rego et al. (2020) found out that aquaponics would have a compound annual growth rate of 12.5% in Namibia. The study concluded that aquaponics is a lucrative agricultural system to invest in due to short- and long-term profit margins and potential local market expansions.
Facing a growing human population, inefficient traditional food production methods and unreliable rainfall, Zimbabwe is considering aquaponics as a viable means to avert famine (Marimbona and Mushiri, 2019). To offset the problem of frequent power outages and ensure continuous running of water and air pumps, a prototype aquaponics system powered by a standalone solar photovoltaic (PV) system has been tested in Zimbabwe. A 1.6 kW solar PV array produced enough output to run a combined electrical load of 293.2 W from a water pump, an aerator and electronics devices. The system used an Arduino based system to automatically monitor the temporal changes in pH, temperature and water flow velocity. The system was designed to be scalable and easy to set up in different localities.
West Africa
Literature on aquaponics in West Africa is limited compared to the Northern and Southern regions of Africa. Although internet search produces information on aquaponics from four West African countries, only a few of the publications contained enough information to be included in the review. The Sustainable Aquaponics for Nutritional and Food Security in Urban Sub-Saharan Africa (SANFU), a small-scale pilot project in Lagos, Nigeria, conducted an experiment to collect data on key variables to bring a small-scale aquaponics system to a productive and economically feasible level (Benjamin et al., 2020). This information had been up until this project been largely lacking for the sub-region. The prototype SANFU aquaponics system that was set up using relatively expensive foreign sourced components can yield about 28 kg of fish and 3 kg of vegetables per annum with a nitrogen outflow of 48.5 g. This corresponds to a rather unfavorable net discounted benefit-cost rate (DBCR) of 0.08 over a 20-year period. A similar system was setup constructed using locally sourced components had a significantly higher DBCR of 1.12. However, it is anticipated that the yield from the fish harvested can be 10 times the experimental yield if optimum real-life stocking and planting densities are considered (Benjamin et al., 2020). The SANFU study highlights the possibility of using locally available materials and increasing the chances of low-income individuals to set up small-scale aquaponic systems and contribute to food and nutrition security.
In Ghana, the implementation of aquaponic systems is slow and reports on aquaponics is very scarce. The most notable aquaponics project in Ghana was a collaborative project between a Ghanaian and Brazilian research institute, which was aimed at increasing smallholder food production through implementation of water conserving aquaponics-based food systems ensuring all-year-round food production for enhanced nutrition to the smallholder farmer (Frimpong et al., 2017). Aquaponics system may be configured to be fully recirculating or decoupled systems, and in the case of the project in Ghana, it was a decoupled system. Decoupled aquaponic are designed to culture the fish and plants as separate units whereby water is used to culture fish and the effluents supplied to the plants without circulating the water back to the fish (Karimanzira et al., 2017). In the Ghana trial, effluents from the fish production was administered to maize plots. This resulted in a maize yield of 2.3 t/ha (Table 1), which is higher than the maize yield range for Ghana of 1.5–1.7 t/ha (Ragasa et al., 2013). Decoupled designs allow more flexibility in customizing and optimizing the water chemistry in the effluents from the fish culture before supply to plants through supplementation of low or absent nutrients (Goddek et al., 2019b). Adding digesters to decoupled aquaponics systems can facilitate the microbial conversion of phosphorus in fish waste into orthophosphates that can be utilized by plants, with high recovery rates (Goddek et al., 2019b).
East Africa
From literature, it is apparent that aquaponics is a recent concept in East Africa. Most of the East African aquaponics trials are concentrated in Kenya. Adoption of new farming technologies that can increase climate resilience has been proposed as a way to increase food security in East Africa, particularly for subsistence farmers (Bryan et al., 2013). In Kenya, productivity of main crops such as maize is declining due to infestation of army worms, land degradation, unpredictable weather events such as prolonged dry conditions and continuous splitting of land between inheritors (Henze and Ulrichs, 2015). Food security in the 21st century requires new, innovative and sustainable food production systems that can increase crop yields using limited land and water resources with little impact on the environment and biodiversity (Pearson, 2007).
To offset the high cost of fish feed which can account for up to 70% of fish production costs (Obirikorang et al., 2020), the black soldier fly larvae (BSFL) has been experimentally included in fish feeds as substitutes for the expensive fishmeal for use in aquaponics systems in Ethiopia (Koop, 2016). Black soldier fly feeds could be suitable for use in aquaponics systems based on positive effects in optimizing fish and plant growth (Koop, 2016). van Gorcum et al. (2019) conducted an exploratory study into the demand side of the Kenyan market and consumer perceptions for aquaponically produced food products in Nairobi. Majority of the respondents in that study were willing to pay more for aquaponics products mainly because they perceived them to be fresher, healthier and free of pesticides. Based on the results of the market survey there appears to be a potential for marketing aquaponics products and the adoption of the system could represent an approach to bypass seasonal production issues due to Kenya's erratic climatic conditions. Besides market value and customer acceptance, the plants used in aquaponics are chosen based on their abilities to sufficiently recover nutrients for growth. Sweet wormwood, pigweed and pumpkin in the hydroponic component of aquaponics systems for the intensive production of Nile tilapia are able to take up nearly 74% of nitrate from the effluents from the fish production units (Gichana et al., 2018).
The challenges to establishing aquaponics projects in East Africa include lack of electricity in many rural areas, cost and access to fish feed which is the one important and most expensive inputs into the system, high set-up cost; which hinders many farmers from adopting aquaponics as most do not have access to agricultural loans. The lack of expertise and long-term planning as well as challenges related to changing customary practices undermine diffusion and adoption of new methods, tools and technologies (van Gorcum et al., 2019).
Food Security Issues in Africa
The United Nation's 2030 development agenda outlines 17 Sustainable Development Goals (SDGs) including SDG 2 which aims to address all forms of hunger and nutritional insecurity issues over the next decade (United Nations, 2015). Although significant progress has been made, food insecurity is still a key issue globally and over 1 billion people suffer from starvation, undernutrition and malnutrition. A disproportionate number of the people experiencing food insecurity are natives of developing countries. Whilst some African countries made significant gains in Millennium Development Goal targets such as “ending extreme poverty and hunger” and “reducing by half the proportion of people who suffer from hunger” by 2015, the large continental picture is patchy and the overall progress slow (FAO, 2015). Food insecurity reached catastrophic dimensions in some areas of Africa, particularly in the Horn of Africa and southern Madagascar (Sasson, 2012), and in some central African countries, the number of undernourished people has more than doubled since 1990 due to the synergistic effect of population growth, political instability and civil wars (FAO, 2015). West Africa has reduced the number of undernourished people by 60% since 1990 and is highlighted as one of the most successful sub-regions south of the Sahara (Hall et al., 2017). Eastern and Southern Africa also made significant progress in reducing the number of people facing food insecurity (FAO, 2015).
Food insecurity transcends insufficient food production, availability, and intake and also relates to the quality and nutritional composition of the food. Fish has often been promoted in many nutritional campaigns as a “rich food for poor people” (Beveridge et al., 2013), and plays an important role in improving Africa's food security and nutritional status. The potential of fish in improving the food security and nutritional status of rural poor, particularly of women and young children is well highlighted (Aiga et al., 2009; Longley et al., 2014; Thilsted et al., 2016; Bennett et al., 2018; Akuffo et al., 2020). Sensitization interventions tailored toward caregiver utilization of food sources like fish to improve child malnutrition has also positively affected fish consumption patterns (Bandoh et al., 2018). Africa's human population is the fastest growing in the world, with the population projected to reach 2.4 billion in 2050 from the present count of 1.1 billion (United Nations, 2015). This directly translates into more than half of the projected global population increase between now and 2050 being born in Africa. Africa's role in achieving SDG 2 is key to mitigating global food insecurity and several governments and sub-regional unions have made commitments to increase agricultural budgets and invest in technical solutions, high yielding crop varieties and sustainable agriculture (Sasson, 2012).
Contribution of Aquaponics to Food Security in Africa
The current discussions on food security and sustainable food production have highlighted the “water-energy-food nexus” approach as key to analyzing and managing the interactions among global resource systems (Scott et al., 2015). The nexus approach acknowledges the interconnectedness of land, water, energy, capital and labor and their associated drivers (Joyce et al., 2019). An important element of the food security agenda in most African countries is to adopt a practice or programme that directly supports food insecure people to achieve some level of food self-sufficiency, particularly nutrition security. Mchunu et al. (2017) highlights the immense potential of aquaponics systems in ensuring food security in many areas in Africa through the provision of fish and vegetables. Fish is very important to human nutrition and health and is projected to play an essential role in the food security and nutrition discussions (Beveridge et al., 2013). The role of cultured fish in ensuring food security discussion is quite recent, and has become crucial in the face of stagnated outputs from natural waters. Even in small quantities, fish can improve the nutritional profiles of human diets by contributing essential amino acids which are often deficient in plant ingredients (FAO, 2014). The healthy macro- and micronutrient profiles of fish highlight its important role in improving malnutrition, especially among children, pregnant and lactating women (Simler et al., 2005; Béné et al., 2015). Many African nations are promoting fish culture as the answer to some of their current and future food production challenges (Robaina et al., 2019). However, the expansion of fish production through conventional earthen ponds and floating cages is constrained by several factors including limited land and water spaces, reduced water availability and concerns over environmental impact (Badiola et al., 2012). Production system diversification with particular emphasis on intensive recirculating systems including aquaponics are thus vital to the increasing fish production to meet the growing human population (Thilsted et al., 2016). Aquaponics systems have generally been projected as an innovative food system that effectively manages the food-water-energy-nexus and exceeds traditional paradigms and can resolve some of the complexities arising from sustainability and food security issues (Werner et al., 2015).
Food insecurity issues in urban areas have been projected to become worsen in Africa (König et al., 2016) leading to significant deficits in the food supply infrastructure and the creations of what has been aptly termed “food deserts” (Beaulac et al., 2009). Aquaponics implemented either as commercial enterprise or as community intervention can enhance local food production capacities (König et al., 2016). The technology is presently an emerging but rapidly growing one in Africa which is well-suited to alleviate some of the negative consequences of climate change and population growth, particularly in urban centers (Robaina et al., 2019). In the Western Cape of South Africa, aquaponics systems have been set up in urban landscapes in response to the increasing levels of food insecurity (Milliken and Stander, 2019). Poor households have been assisted to set up different scales of backyard aquaponics systems to augment food self-sufficiency and incomes. Some have in addition to growing fish and crops, added the production and sale of fingerlings and seedlings. According to Milliken and Stander (2019), increasing popularity of urban food production through aquaponics as a way to reconnect urban residents with food production improved the food self-sufficiency of these low-income communities. In space-limited urban areas, food security can be enhanced by adopting more efficient growing technologies such as vertical farming (Khandaker and Kotzen, 2018).
The drier and water-scarce regions of Africa, particularly those with limited arable lands can benefit from aquaponics given to meet food self-sufficiency while reducing environmental footprints. As part of the measures to meet the millennium development goal of eradicating extreme poverty and hunger and of ensuring environmental stability in Egypt, the country targeted aquaculture and aquaponics as viable options (United Nations, 2015). The significance of fish in Egypt's food security discussions typically correlates with increasing demands for dietary animal protein (Zwirn, 2002). Aquaponics technology has been piloted and implemented as part of the country's solution to reducing the volumes of food imports. The increased promotion of sustainable food production technologies in Egypt and other North African countries is partly as a result of the social and political unrest the region faced following periods of food insecurity and increasing food prices. To dampen the effects of fluctuating world food prices and its impacts on citizens, particularly the impacts felt by the rural poor of that country, national agencies in charge of fisheries and aquaculture have piloted small-scale aquaponics systems in rural areas and report of favorable uptake of the technology (Goada et al., 2015). There have been several private establishments in Egypt offering scalable aquaponics models aimed at improving household food self-sufficiencies. Large-scale aquaponics farms have also been established across the country, especially in the regions with pervasive water problems and limited availability of arable land. Although presently the continent-wide adoption rate of aquaponics is low, there is a high potential for increased uptake of the technology if the installation components are sourced from local materials (Benjamin et al., 2020). While most of the narrative in this section highlights the contribution of aquaponics to food security, it should be seen as a complementary technology to conventional aquaculture and agriculture since it is presently well-suited for the culture of a few fish species and has not been optimally proven to be able to support the growing of most key staple foods.
Climate Change, Land and Water Resource Issues
In addition to population growth, climate change is projected to significantly affect food security in Africa over the coming decades and there is robust evidence suggesting that climate change impacts will be more severe in developing countries (Tschakert, 2007). Sub-Saharan Africa is predicted to be worst affected by climate change due to the already elevated air temperatures, reliance on rain-fed agriculture and fragile local economies (Niasse et al., 2004). About 600 million people in sub-Saharan Africa will be predisposed to food insecurity and malnutrition by 2060 because 60–90 million hectares of the land is expected to experience intense drought (Sasson, 2012). Farmers have increasingly made efforts to adapt to climate change through shifting planting seasons and planting draught-resistant crop varieties but the current trends in yield improvements will not match the projected global food demand by 2050, suggesting a necessary expansion of food production areas (Bajželj et al., 2014). Expansion in agricultural land, however, appears to be impossible due to the widespread land degradation and other environmental problems. Between 1970 and 2013, the availability of agricultural land globally has decreased by more than 50% (World Bank, 2018).
Climate change is also expected to impact water availability in Sub-Saharan Africa and this will affect water-dependent livelihoods such as aquaculture. Aquaculture is the fastest growing animal sector in the world and has been identified as an important sector to successfully address the challenges to global food security arising out of the increasing human population and climate change (National Research Council of the National Academies, 2015). However, aquaculture is likely to be affected by climate change, particularly temperature increments. Unlike other farmed animals, all cultured fish species for human consumption are poikilothermic. Consequently, any increase and/or decrease of the temperature of fish habitats could have a significant influence on general metabolism and hence the rate of growth and total production.
Increased frequency and severity of droughts, floods and extreme weather events are expected to affect water availability, food security, health, infrastructure and thus overall development. Water stress leading to decreased water availability in major rivers and lakes in Asia and Africa as reported by IPCC (2007) can significantly affect both cage and pond-based aquaculture by reducing water availability and/or retention times. The predicted increases in the occurrence of extreme weather events can also lead to significant economic losses resulting from damaged fish cages and fish escapes. Due to high poverty levels, the reliance on rain or natural water bodies as water sources and the lack of access to technology and improved aquaculture practices, these impacts are likely to be severely felt in the major aquaculture regions of Africa. This coupled with conventional food production systems facing limitations for further expansion due to lack of space, reduced water availability and heightened concerns over environmental impact (Goddek et al., 2019a), has led to progressive adoption of controlled food production systems. In this context, aquaponics as a food production system that recycles nutrient and waste can address food security issues, particularly for arid regions or areas with poor agricultural soils (Goddek and Körner, 2019). As a hybrid technology, aquaponics can also mitigate some of the effects of climate change on food production.
Conclusion
There is no simple solution to ensuring food security, but technological innovations in food production systems can directly support food insecure people to achieve some level of food self-sufficiency, particularly nutrition security. With current food production goals no longer aimed at simply maximizing productivity but optimizing outputs across different production systems, aquaponics technology holds an immense potential in ensuring food security in many parts of Africa. Household and commercial aquaponics establishments in Egypt and South Africa have been directly linked with ensuring food security in rural and urban settings. The continent-wide adoption of aquaponics positively correlates with the level of aquaculture output. Egypt, Nigeria, Kenya and South Africa who are major contributors to the continent's aquaculture in Africa are also countries leading in adoption of aquaponics. As the continent's population continues to increase and the threats of food insecurity heightens, aquaponics represents a promising technology for producing both high-quality fish protein and vegetables in ways that utilize substantially less land, less energy and less water while also minimizing chemical and fertilizer inputs that are used in conventional food production. It is, however, imperative that more research is directed at developing low-cost aquaponics systems that can easily be adopted by low- and middle-income Africans.
Author Contributions
The paper was initiated KO, BG, and WS. KO wrote the first version, provided by inputs from WS, BG, GA, and WA. All authors provided valuable inputs participated in discussions and reviewed the final draft and read and approved the final manuscript.
Conflict of Interest
The authors declare that the research was conducted in the absence of any commercial or financial relationships that could be construed as a potential conflict of interest.
Publisher's Note
All claims expressed in this article are solely those of the authors and do not necessarily represent those of their affiliated organizations, or those of the publisher, the editors and the reviewers. Any product that may be evaluated in this article, or claim that may be made by its manufacturer, is not guaranteed or endorsed by the publisher.
References
Aiga, H., Matsuoka, S., Kuroiwa, C., and Yamamoto, S. (2009). Malnutrition among children in rural Malawian fish-farming households. Trans. R. Soc. Trop. Med. Hyg. 103, 827–833. doi: 10.1016/j.trstmh.2009.03.028
Akuffo, A. S., Quagrainie, K. K., and Obirikorang, K. A. (2020). Analysis of the determinants of fish consumption by households in Ghana. Aquacult. Econ. Manag. 24, 294–309. doi: 10.1080/13657305.2020.1723734
Badiola, M., Mendiola, D., and Bostock, J. (2012). Recirculating aquaculture systems (RAS) analysis: main issues on management and future challenges. Aquac. Eng. 51, 26–35. doi: 10.1016/j.aquaeng.2012.07.004
Bajželj, B., Richards, K. S., Allwood, J. M., Smith, P., Dennis, J. S., Curmi, E., et al. (2014). Importance of food-demand management for climate mitigation. Nat. Clim. Chang. 4, 924–929. doi: 10.1038/nclimate2353
Bandoh, D. A., Manu, A., and Kenu, E. (2018). Lacking in abundance: undernutrition in a Peri-urban fishing community in Coastal Ghana. BMC Nutrition 4:20. doi: 10.1186/s40795-018-0229-8
Beaulac, J., Kristjansson, E., and Cummins, S. (2009). A systematic review of food deserts, 1966-2007 Prev. Chronic Dis. 6:A105.
Béné, C., Barange, M., Subasinghe, R., Pinstrup-Andersen, P., Merino, G., Hemre, G.-I., et al. (2015). Feeding 9 billion by 2050 – putting fish back on the menu. Food. Sec. 7, 261–274. doi: 10.1007/s12571-015-0427-z
Benjamin, E. O., Buchenrieder, G. R., and Sauer, J. (2020). Economics of small-scale aquaponics system in West Africa: a SANFU case study. Aquacult. Econ. Manag 25, 53–69. doi: 10.1080/13657305.2020.1793823
Bennett, A., Patil, P., Kleisner, K., Doug, R., Virdin, J., and Basurto, X. (2018). Contribution of Fisheries to Food Security: Current Knowledge, Policy and Research. NI Report 18-02. Durham, NC: Duke University. Available online at: http://nicholasinstitute.duke.edu/publication (accessed April 16, 2021).
Bernstein, S. (2011). Aquaponic Gardening: A Step-by-Step Guide to Raising Vegetables and Fish Together. Gabriola Island: New Society Publishers.
Beveridge, M. C. M., Thilsted, S. H., Phillips, M. J., Metian, M., Troell, M., and Hall, S. J. (2013). Meeting the food and nutrition needs of the poor: The role of fish and the opportunities and challenges emerging from the rise of aquaculture. J. Fish Biol. 83, 1067–1084. doi: 10.1111/jfb.12187
Botha, I. (2014). Aquaponics as a Productive Rehabilitation Alternative in Mpumalanga Highveld Coalfields (Ph.D. thesis). Bloemfontein: University of the Free State.
Bryan, E., Ringler, C., Okoba, B., Roncoli, C., Silvestri, S., and Herrero, M. (2013). Adapting agriculture to climate change in Kenya: Household strategies and determinants. J. environ. Manag. 114, 26–35. doi: 10.1016/j.jenvman.2012.10.036
Conijn, J. G., Bindraban, P. S., Schröder, J. J., and Jongschaap, R. E. E. (2018). Can our global food system meet food demand within planetary boundaries? Agric. Ecosyst. Environ. 251, 244–256. doi: 10.1016/j.agee.2017.06.001
Dalsgaard, J., Lund, I., Thorarinsdottir, R., Drengstig, A., Arvonen, K., and Pedersen, P. B. (2013). Farming different species in RAS in Nordic countries: current status and future perspectives. Aquac. Eng. 53, 2–13. doi: 10.1016/j.aquaeng.2012.11.008
D'Amato, M. E., Esterhuyse, M. M., Van Der Waal, B. C. W., Brink, D., and Volckaert, F. A. M. (2007). Hybridization and phylogeography of the Mozambique tilapia Oreochromis mossambicus in southern Africa evidenced by mitochondrial and microsatellite DNA genotyping. Conserv. Genet. 8, 475–488. doi: 10.1007/s10592-006-9186-x
El-Essawy, H., Nasr, P., and Sewilam, H. (2019). Aquaponics: a sustainable alternative to conventional agriculture in Egypt – a pilot scale investigation. Environ. Sci. Pollut. Res. 26, 15872–15883. doi: 10.1007/s11356-019-04970-0
Essa, M. A., Goda, A. M. A. S., Hanafy, M. A., El-Shebly, A. A., Mohamed, R. A., and El-Ebiary, E. H. (2008). Small-scale fish culture: guiding models of aquaponics and net-enclosures fish farming in Egypt. Egypt. J. Aquat. Res. 34, 320–337.
Faber, M., Witten, C., and Drimie, S. (2011). Community-based agricultural interventions in the context of food and nutrition security in South Africa. S. Afr. J. Clin. Nutr. 24, 21–30. doi: 10.1080/16070658.2011.11734346
FAO (2014). Small-Scale Aquaponic Food Production. Fisheries and aquaculture technical paper No. 589. Rome: Food and Agriculture Organisation of the United Nations, 262.
FAO (2015). The State of Food Insecurity in the World 2015. Meeting the 2015 International Hunger Targets: Taking Stock of Uneven Progress. Rome: Food and Agriculture Organisation of the United Nations.
FAO (2017). The Future of Food and Agriculture: Trends and Challenges. Rome: Food and Agriculture Organisation of the United Nations.
FAO (2020). GIEWS - Global Information and Early Warning System: Country Briefs. Food and Agriculture Organisation of the United Nations. Available online at: http://www.fao.org/giews/countrybrief/country.jsp?code=NAM (accessed April 20, 2021).
Frimpong, F., Amponsah, S. K, Owusu Danquah, E., Agyeman, K., Oteng-Darko, P, Oppong, C. P., et al. (2017). Assessment of aquaponics-based food production system effluents on the performance of maize. Int. J. Agric. Res. Rev. 5, 615–627.
Gibellato, S. (2020). Feasibility study of an aquaponic plant in Korhogo (MSc thesis). Venice: Ca' Foscari University of Venice.
Gichana, Z., Waidbacher, H., Zollitsch, W., Drexler, S., and Liti, D. (2018). The potential of aquaponics as food production and nutrient recovery systems in Kenya, in Towards Productive, Sustainable and Resilient Global Agriculture and Food Systems, eds Horská, E., Kapsdorferová, Z., and Hallová, M. (Proceedings International Scientific Days), 1154–1165.
Goada, A. M. A.-S., Essa, M. A., Hassaan, M. S., and Sharawy, Z. (2015). Bio-economic features for aquaponic systems in Egypt. Turk. J. Fish. Aquat. Sci. 15, 531–538. doi: 10.4194/1303-2712-v15_2_40
Goddek, S., Joyce, A., Kotzen, B., and Dos-Santos, M. (2019a). Aquaponics and global food challenges, in Aquaponics Food Production Systems, eds S. Goddek, A. Joyce, B. Kotzen, and G. M. Burnell (Cham: Springer), 3–18.
Goddek, S., Joyce, A., Wuertz, S., Körner, O., Bläser, I., Reuter, M., et al. (2019b). Decoupled aquaponics systems, in Aquaponics Food Production Systems, eds S. Goddek, A. Joyce, B. Kotzen, and G. M. Burnell (Cham: Springer), 201–230.
Goddek, S., and Körner, O. (2019). A fully integrated simulation model of multi-loop aquaponics: a case study for system sizing in different environments. Agric. Syst. 171, 143–154. doi: 10.1016/j.agsy.2019.01.010
Godfray, H. C. J., Beddington, J. R., Crute, I. R., Haddad, L., Lawrence, D., Muir, J. F., et al. (2010). Food security: the challenge of feeding 9 billion people. Science 327, 812–818. doi: 10.1126/science.1185383
Hall, C., Dawson, T., Macdiarmid, J., Matthews, R., and Smith, P. (2017). The impact of population growth and climate change on food security in Africa: looking ahead to 2050. Int. J. Agric. Sustain. 15, 124–135. doi: 10.1080/14735903.2017.1293929
Henze, J., and Ulrichs, C. (2015). Aquaponics and its potential for food security in Kenya, in International Conference on Biodiversity for Food and Nutrition on Capacity Building, Nairobi, Kenya. 21–22.
Hu, Z., Lee, J. W., Chandran, K., Kim, S., Brotto, A. C., and Khanal, S. K. (2015). Effect of plant species on nitrogen recovery in aquaponics. Bioresour. Technol. 188, 92–98. doi: 10.1016/j.biortech.2015.01.013
IPCC (2007). Climate Change 2007: The Physical Science Basis. Contribution of Working Group I to the Fourth Assessment Report of the Intergovernmental Panel on Climate Change. Cambridge: Cambridge University Press.
Joly, A., Junge, R., and Bardocz, T. (2015). Aquaponics business in Europe: some legal obstacles and solutions. Ecocycles 1, 3–5. doi: 10.19040/ecocycles.v1i2.30
Joyce, A., Goddek, S., Kotzen, B., and Wuertz, S. (2019). Aquaponics: closing the cycle on limited water, land and nutrient resources, in Aquaponics Food Production Systems: Combined Aquaculture and Hydroponic Production Technologies for the Future, eds S. Goddek, A. Joyce, B. Kotzen, and G. M. Burnell (Springer International Publishing), 19–34.
Junge, R., König, B., Villarroel, M., Komives, T., and Jijakli, M. (2017). Strategic points in aquaponics. Water 9:182. doi: 10.3390/w9030182
Karimanzira, D., Keesman, K., Kloas, W., Baganz, D., and Rauschenbach, T. (2017). Efficient and economical way of operating a recirculation aquaculture system in an aquaponics farm. Aquacult. Econ. Manag. 21, 470–486. doi: 10.1080/13657305.2016.1259368
Khandaker, M., and Kotzen, B. (2018). The potential for combining living wall and vertical farming systems with aquaponics with special emphasis on substrates. Aquac Res 49, 1454–1468. doi: 10.1111/are.13601
König, B., Junge, R., Bittsanszky, A., Villarroel, M., and Komives, T. (2016). On the sustainability of aquaponics. Ecocycles 2, 26–32. doi: 10.19040/ecocycles.v2i1.50
Koop, M. (2016). Aquaponics and the Potential of BSFL Farming in Ethiopia (BSc. Thesis). Velp: Van Hall Larenstein University of Applied Sciences.
Longley, C., Thilsted, S. H., Beveridge, M., Cole, S., Nyirenda, D. B., Heck, S., et al. (2014). The Role of Fish in the First 1,000 Days in Zambia. Brighton, UK: Institute of Development Studies. Retrieved from: https://opendocs.ids.ac.uk/opendocs/handle/123456789/4384 (accessed April 25, 2021).
Love, D. C., Fry, J. P., Genello, L., Hill, E. S., Frederick, J. A., Li, X., et al. (2014). An international survey of aquaponics practitioners. PLoS ONE 9:e102662. doi: 10.1371/journal.pone.0102662
Love, D. C., Fry, J. P., Li, X., Hill, E. S., Genello, L., Semmens, K., et al. (2015). Commercial aquaponics production and profitability: findings from an international survey. Aquaculture 435, 67–74. doi: 10.1016/j.aquaculture.2014.09.023
Mabhaudhi, T., Modi, A. T., and Beletse, Y. G. (2013). Response of taro (Colocasia esculenta L. Schott) landraces to varying water regimes under a rainshelter. Agric. Water Manag. 121, 102–112. doi: 10.1016/j.agwat.2013.01.009
Marimbona, E., and Mushiri, T. (2019). Design of a sustainable automated solar powered aquaponic system – case of St Peter's Mbare Secondary School – Harare, Zimbabwe, in Proceedings of the 17th Industrial and Commercial Use of Energy (ICUE) Conference; 2019. Cape Town. Available online at: https://ssrn.com/abstract=3657051 (accessed April 15, 2021).
McCarl, B. A., Musumba, M., Smith, J. B., Kirshen, P., Jones, R., El-Ganzori, A., et al. (2015). Climate change vulnerability and adaptation strategies in Egypt's agricultural sector. Mitig. Adapt. Strateg. Glob. Chang. 20, 1097–1109. doi: 10.1007/s11027-013-9520-9
Mchunu, N., Lagerwall, G., and Senzanje, A. (2017). Food sovereignty for food security, aquaponics system as a potential method: a review. J. Aquac. Res. Dev. 497, 1–9. doi: 10.4172/2155-9546.1000497
Mchunu, N., Lagerwall, G., and Senzanje, A. (2018). Aquaponics in South Africa: results of a national survey. Aquac. Rep. 12, 12–19. doi: 10.1016/j.aqrep.2018.08.001
Mchunu, N., Lagerwall, G., and Senzanje, A. (2019). Aquaponics model specific to South African conditions. S. Afric. J. Agric. Ext. 47, 73–91. doi: 10.17159/2413-3221/2019/v47n1a491
Milliken, S., and Stander, H. (2019). Aquaponics and social enterprise, in Aquaponics Food Production Systems: Combined Aquaculture and Hydroponic Production Technologies for the Future, eds S. Goddek, A. Joyce, B. Kotzen, G. M. Burnell (Springer International Publishing), 19–34.
National Research Council of the National Academies (2015). Critical Role of Animal Science Research in Food Security and Sustainability. Washington, DC: NRC of the National Academies Press.
Niasse, M., Afoud, A., and Amani, A. (2004). Reducing West Africa's Vulnerability to Climate Impacts on Water Resources, Wetlands and Desertification: Elements of Regional Preparedness and Adaptation. Gland; Cambridge: International Union for Conservation of Nature.
Obirikorang, K. A., Gyamfi, S., Goode, M. E., Amisah, S., Edziyie, R. E., Quagrainie, K., et al. (2020). Effect of soybean meal diets on the growth performance, ammonia excretion rates, gut histology and feed cost of Nile tilapia (Oreochromis niloticus) fry. Aquacult. Res. 51, 3520–3532. doi: 10.1111/are.14689
Oladimeji, S. A., Okomoda, V. T., Olufeagba, S. O., Solomon, S. G., Abol-Munafi, A. B., Alabi, K. I., et al. (2020). Aquaponics production of catfish and pumpkin: comparison with conventional production systems. Food Sci. Nutr. 8, 2307–2315. doi: 10.1002/fsn3.1512
Pearson, C. J. (2007). Regenerative, semi-closed systems. A priority for twenty-first-century agriculture. Bioscience 57, 409–418. doi: 10.1641/B570506
Ragasa, C., Dankyi, A., Acheampong, P., Wiredu, A. N., Chapoto, A., Asamoah, M., et al. (2013). Patterns of Adoption of Improved Maize Technologies in Ghana. Ghana Strategy Support Program Working Paper 36. Accra: International Food Policy Research Institute.
Rakocy, J. E., Shultz, R. C., Bailey, D. S., and Thoman, E. S. (2004). Aquaponic integration of Tilapia and Basil: comparing a batch and staggered cropping system. Acta Hortic. 648, 63–69. doi: 10.17660/ActaHortic.2004.648.8
Rego, M. M., Strazdus, S., Merianos, N. E., and Antoniou, S. L. (2020). The Aquaponics Project: Improving Food Security in Namibia in the Face of Climate Change. BSc Project. Worcester, MA: Worcester Polytechnic Institute
Robaina, L., Pirhonen, J., Mente, E., Sánchez, J., and Goosen, N. (2019). Fish diets in aquaponics, in: Aquaponics Food Production Systems: Combined Aquaculture and Hydroponic Production Technologies for the Future, eds S. Goddek, A. Joyce, B. Kotzen, G. M. Burnell (Springer International Publishing), 19–34.
Sace, C. F., and Fitzsimmons, K. M. (2013). Vegetable production in a recirculating aquaponic system using Nile tilapia (Oreochromis niloticus) with and without freshwater prawn (Macrobrachium rosenbergii). Acad. J. Agric. Res. 1, 236–250.
Salem, L. (2019). Assessing Deep-Water Culture and Sand-Bed Aquaponics Systems for Lettuce (Lactuca sativa) Yield and Water Consumption (MSc. thesis). Cairo: American University of Cairo.
Sasson, A. (2012). Food security for Africa: an urgent global challenge. Agric Food Sec. 1, 1–16. doi: 10.1186/2048-7010-1-2
Scott, C. A., Kurian, M., and Wescoat, J. L. Jr. (2015). The water-energy-food nexus: enhancing adaptive capacity to complex global challenges, in Governing the Nexus, eds Kurian M., and Ardakanian R. (Springer, Cham), 15–38.
Sikawa, D. C., and Yakupitiyage, A. (2010). The hydroponic production of lettuce (Lactuca sativa L) by using hybrid catfish (Clarias macrocephalus × C. gariepinus) pond water: potentials and constraints. Agric. Water Manag. 97, 1317–1325. doi: 10.1016/j.agwat.2010.03.013
Simler, K. R., Jacobs, K. L., Mensah-Homiah, J., Randriamamonjy, J., Wiesmann, D., and Abdul-Razak, A. (2005). Food-Based Approaches to Reducing Micronutrient Malnutrition: An Impact Evaluation of the UNICEF ICBD Programme in Savelugu Nanton District of Northern Ghana. International Food Policy Research Institute (IFPRI) Discussion Paper Series. Washington, DC: International Food Policy Research Institute. Available online at: https://www.researchgate.net/publication/255981941 (accessed April 20, 2021)
Soethoudt, J. M., Hendricks, J., and Waldhauer, N. (2016). Opportunities Hydro-and Aquaponics in Egypt. Wageningen: Wageningen UR (University & Research Centre).
Swap, R. J., Annegarn, H. J., Suttles, J. T., Haywood, J., Helmlinger, M. C., Hely, C., et al. (2002). The Southern African Regional Science Initiative (SAFARI 2000): overview of the dry season field campaign. S. Afr. J. Sci. 98, 125–130. doi: 10.1029/2003JD003747
Thilsted, S. H., Thorne-Lyman, A., Webb, P., Bogard, J. R., Subasinghe, R., Phillips, M. J., et al. (2016). Sustaining healthy diets: THE role of capture fisheries and aquaculture for improving nutrition in the post-2015 era. Food Policy 61, 126–131. doi: 10.1016/j.foodpol.2016.02.005
Tschakert, P. (2007). Views from the vulnerable: understanding climatic and other stressors in the Sahel. Glob. Environ. Change 17, 381–396. doi: 10.1016/j.gloenvcha.2006.11.008
Tyson, R. V., Treadwell, D. D., and Simonne, E. H. (2011). Opportunities and challenges to sustainability in aquaponic systems. Hort Technol 21, 6–13. doi: 10.21273/HORTTECH.21.1.6
United Nations (2015). Transforming Our World: The 2030 Agenda for Sustainable Development, A/RES/70/1. Available online at: https://www.refworld.org/docid/57b6e3e44.html (accessed April 24, 2021).
USAID (2013) Sustainable Fisheries and Responsible Aquaculture: A Guide for USAID Staff and Partners. Rhode Island: University of Rhode Island/Coastal Resources Center. Available online at: https://www.crc.uri.edu/download/FishAquaGuide14Jun13Final.pdf (accessed April 22 2021).
van der Heijden, P. G. M., Farrag, F., and Blom-Zandstra, G. (2013). Bustan aquaponics: Egypt's first working commercial aquaponic farm. Aquacult. Europe Mag. 38, 25–27.
Van der Waal, B. C. W. (2000). Fish as a resource in a rural river catchment in the Northern Province, South Africa. Afr. J. Aquat. Sci. 25, 56–70. doi: 10.2989/160859100780177839
van Gorcum, B., Goddek, S., and Keesman, K. J. (2019). Gaining market insights for aquaponically produced vegetables in Kenya. Aquac. Int. 27, 1231–1237. doi: 10.1007/s10499-019-00379-1
Vermeulen, T., and Kamstra, A. (2012). The need for systems design for robust aquaponic systems in the urban environment. Int. Symp. Soilless Cult. 1004, 71–77. doi: 10.17660/ActaHortic.2013.1004.6
Werner, K., Groß, R., Baganz, B., Graupner, J., Monsees, H., Schmidt, U., et al. (2015). A new concept for aquaponic systems to improve sustainability, increase productivity, and reduce environmental impacts. Aquac. Environ. Interact. 2, 179–92. doi: 10.3354/aei00146
World Bank (2018). Agricultural Land (% of Land Area). Available online at: https://data.worldbank.org/indicator/ag.lnd.agri.zs (accessed April 19, 2021).
Zhang, X., and Cai, X. (2011). Climate change impacts on global agricultural land availability. Environ. Res. Lett. 6:014014. doi: 10.1088/1748-9326/6/1/014014
Keywords: agri-aquaculture, aquaculture, aquaponics, fish, plants, sustainable agriculture
Citation: Obirikorang KA, Sekey W, Gyampoh BA, Ashiagbor G and Asante W (2021) Aquaponics for Improved Food Security in Africa: A Review. Front. Sustain. Food Syst. 5:705549. doi: 10.3389/fsufs.2021.705549
Received: 05 May 2021; Accepted: 30 July 2021;
Published: 20 August 2021.
Edited by:
Felix Kwabena Donkor, University of South Africa, South AfricaReviewed by:
Therese Mwatitha Gondwe, Independent Researcher, Lilongwe, MalawiElliot Alhassan, University for Development Studies, Ghana
Copyright © 2021 Obirikorang, Sekey, Gyampoh, Ashiagbor and Asante. This is an open-access article distributed under the terms of the Creative Commons Attribution License (CC BY). The use, distribution or reproduction in other forums is permitted, provided the original author(s) and the copyright owner(s) are credited and that the original publication in this journal is cited, in accordance with accepted academic practice. No use, distribution or reproduction is permitted which does not comply with these terms.
*Correspondence: Kwasi Adu Obirikorang, a2FvYmlyaWtvcmFuZy5jYW5yQGtudXN0LmVkdS5naA==; cXVhc2lhZHVAZ21haWwuY29t