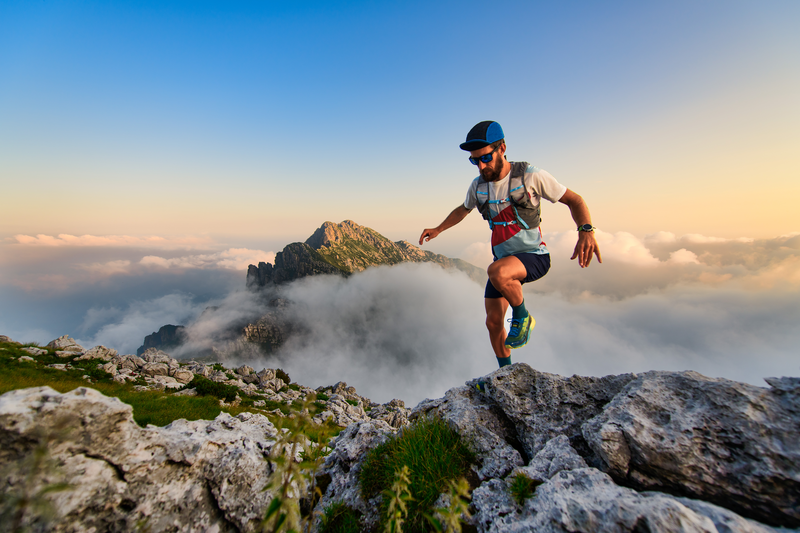
95% of researchers rate our articles as excellent or good
Learn more about the work of our research integrity team to safeguard the quality of each article we publish.
Find out more
MINI REVIEW article
Front. Sustain. Food Syst. , 23 July 2021
Sec. Climate-Smart Food Systems
Volume 5 - 2021 | https://doi.org/10.3389/fsufs.2021.692137
This article is part of the Research Topic Climate-Smart Agriculture: Productivity, Efficiency and Sustainability View all 4 articles
Well-managed legume-based food systems are uniquely positioned to curtail the existential challenge posed by climate change through the significant contribution that legumes can make toward limiting Green House Gas (GHG) emissions. This potential is enabled by the specific functional attributes offered only by legumes, which deliver multiple co-benefits through improved ecosystem functions, including reduced farmland biodiversity loss, and better human-health and -nutrition provisioning. These three critical societal challenges are referred to collectively here as the “climate-biodiversity-nutrition nexus.” Despite the unparalleled potential of the provisions offered by legumes, this diverse crop group remains characterized as underutilized throughout Europe, and in many regions world-wide. This commentary highlights that integrated, diverse, legume-based, regenerative agricultural practices should be allied with more-concerted action on ex-farm gate factors at appropriate bioregional scales. Also, that this can be achieved whilst optimizing production, safeguarding food-security, and minimizing additional land-use requirements. To help avoid forfeiting the benefits of legume cultivation for system function, a specific and practical methodological and decision-aid framework is offered. This is based upon the identification and management of sustainable-development indicators for legume-based value chains, to help manage the key facilitative capacities and dependencies. Solving the wicked problems of the climate-biodiversity-nutrition nexus demands complex solutions and multiple benefits and this legume-focus must be allied with more-concerted policy action, including improved facilitation of the catalytic provisions provided by collaborative capacity builders—to ensure that the knowledge networks are established, that there is unhindered information flow, and that new transformative value-chain capacities and business models are established.
The biggest challenge facing humanity today is how best to rapidly implement those changes which will allow adaptation to, and mitigation of, climate change whilst redressing the loss of biodiversity and ecosystem services (Intergovernmental Science-Policy Platform on Biodiversity and Ecosystem Services (IPBES), 2019). The recommendations of the Intergovernmental Panel on Climate Change are clear (Hoegh-Guldberg et al., 2018) and embodied in the “Paris Agreement” (United Nations, 2015), which aims to keep average global temperature rise to below 2°C, and preferably below 1.5°C. Toward these goals, and at current rates of decarbonisation among the various main industry sectors, agriculture remains historically recalcitrant to lowering its greenhouse gas (GHG) footprint (Wollenberg et al., 2016). Even if fossil fuel emissions were immediately halted, current trends in global agriculture and food production would prevent achievement of the 1.5°C target (Clark et al., 2020). However, the agricultural sector (and land-use more widely), is well-placed to support the EC policy (Green Deal) commitment to “net-zero” emissions by 2050 (EC, 2019). Particularly since the technological solutions to remove and store CO2 (and other greenhouse gases) are unlikely to become operational at a significant scale soon (Sanna et al., 2014).
The agriculture-based contribution to “net-zero” demands the uptake of low-carbon farming measures and among these, legumes have a central role to play (Luscher et al., 2014; Oliveira et al., 2021). The journey to net-zero should include greater focus on the delivery of adaptative measures to improve geochemical-cycles or -flows, and ecosystem functions via control of key climate change “drivers,” and especially sustainable nutrient solutions through more efficient use and management of reactive-nitrogen resources (Steffen et al., 2015; Ascott et al., 2017; Breitburg et al., 2018; Folke et al., 2021). In this reactive-nitrogen context, legume crops can meet their own nitrogen needs for growth via “biological nitrogen fixation” (BNF) (Peoples et al., 1995), and do not necessarily require the addition of synthetic nitrogenous fertilizer. BNF by legumes also reduces synthetic nitrogen input requirement for subsequent, or companion, non-legume crops. Deployed in well-managed cropped systems, legumes have significantly lower emissions of N2O than would be associated with crops receiving synthetic nitrogen fertilizers (Rochette and Janzen, 2005; Rees et al., 2013). In such well-integrated cropped systems, legumes offer the greatest carbon abatement potential relative to other strategies: legume-based crop rotations and grassland farming systems > precision agriculture of legumes > optimized soil pH > intercropping > nitrification inhibitors > improved crop varieties (Eory et al., 2020). Due to their wide diversity of types, legumes may be integrated into cropped systems and ex-farmgate value systems in many ways (Supplementary Figure 1). Additionally, high levels of legume inclusion need not compromise yields, since it has been shown that maximum productivity and minimum synthetic fertilizer use can be achieved at 50% legume cover (crop residence over rotation-time) (Iannetta et al., 2016). This coverage can be defined as an equal balance between non-legumes and grain-/forage-legumes over the course of the crop rotation (Supplementary Figure 2), particularly where various forms of intercrop-based interventions are deployed (Brooker et al., 2014).
More-sustainable bioregionally produced, legume-based food- and feed-value chains, as allowed by the biogeographical and socio-economic constraints, must be realized to help circumvent any negative impacts of global-trade. Since, in the last few decades Europe (and other major regions of the world) have increased the arable land area used for more commercially rewarding cereal production, at the expense of land area sown with legumes (Watson et al., 2017). Yet, Europe also expanded livestock and poultry production (i.e., increased feed demand) during the same period following increased demand for animal protein Food and Agriculture Organization of the United Nations (FAO) statistics (FAOSTAT, 2019). The European Commission (EC) “Feed Protein Balance sheet” (EC, 2020), highlights that the EC is self-sufficient in grass-based protein forages. and while the legume component of such forages needs to be increased, the low domestic production of high-protein legume grains has been encouraged by large-scale imports of inexpensive (tariff free) grains, especially of soya, from other countries (Kuhlman et al., 2014). By 2010, Europe was importing almost 90% of its soya demand from the USA, Brazil, and Argentina (CAPRI Dataset, 2020). This has led to wide-scale tropical deforestation and carbon emissions in some countries (Bonini et al., 2018). Thus, developing European sufficiency in grain legume supply could achieve GHG mitigation in Europe, and South America. Equally, it should also be acknowledged that grain legume production by smallholder producers in less industrialized, or more-agrarian based economies, is also critically important, and may also need to be intensified (Nassary et al., 2019; Kebede, 2020). The best routes to such intensification requires careful consideration to ensure that ecosystem functions and services in already industrialized and industrializing food systems allow mutual benefits (c.f. Wiggins, 2009). Allied to this, it may also be the case that trade protection policies need deployed to help protect the nutritional and economic well-being of smallholders in less-industrialized regions (Brooks and Matthews, 2015).
The existential challenges posed by the impacts of climate change constitute what should be recognized as “wicked problems” (Churchman, 1967). That is, challenges which comprise complex interdependencies, which should be addressed using solutions that also help solve other critical negative impacts upon ecosystem functions. In this “multi-functional solution context,” and if wisely deployed and facilitated, legumes offer an intervention which can deliver multiple co-benefits including reduced loss of farmland biodiversity, improved environmental sustainability (Stagnari et al., 2017), and better human-health and -nutrition (Foyer et al., 2016).
Intensification of food production systems, and polarization of crop choices, have accelerated the loss of biodiversity, functional, and genetic (not simply at the species-level) from farmland habitats resulting in the degradation of the system functions on which sustainable and resilient production depends (e.g., decomposition and nutrient dynamics, detoxification, pest suppression, pollination) (Hawes et al., 2005). This decline in regulating and supporting functions results in further reliance on chemical intervention to maintain crop productivity (Palomo-Campesino et al., 2018), exacerbating the impacts of climate change by inappropriate habitat conversion and agrochemical (particularly pesticide) use (Hallmann et al., 2017). Traditional organic systems seek to reverse these trends by replacing agrochemicals with biologically based substitutes (Röös et al., 2018) which, although they may benefit local biodiversity (Bengtsson et al., 2005), may not necessarily have a significant impact on system sustainability—since they still tend to rely heavily on external inputs, plowing, and livestock. Integrated crop production systems based on regenerative agricultural practices utilize diversity for enhanced resilience, thereby reducing reliance on external inputs to maintain productivity (Hawes et al., 2021). In these systems, incorporating a greater diversity of crop types, including legumes and other underutilized crops, enables an increase in production efficiency through facilitation and complementation mechanisms to deliver multiple functional and resource-use benefits over more traditional cropping systems (Lin, 2011). Enhanced biodiversity associated with diversified legume-based crop rotations may also help facilitate resilience to the impacts of stochastic-weather conditions and shifting pest pressures that are linked to climate change (Lin, 2011). Ultimately, legume-based rotations can directly contribute to climate change mitigation since such plant-protein crops have a higher input efficiency, energy conversion, lower environmental impact, and protein provisions compared to livestock production (Clark and Tilman, 2017; Leinonen et al., 2020). It has been long understood that well-integrated, diversified crop systems, incorporating a range of crop functional types offers greater potential than monoculture cropping for pest and disease control through reduced apparency of specialist crop pests to their host and dilution of total resource available (Root, 1973). However, we acknowledge that the potential of such integrated systems is reliant only upon the development and greater uptake of integrated pest management (IPM) measures—which must be effective under the increasingly stochastic weather patterns associated with climate change (Clement et al., 2000; Sharma et al., 2010).
To support the increasing demand for food and feed, it is predicted that agricultural productivity must be increased by 60% between 2012 and 2050. Climate change jeopardizes this nutritional provision 2-fold: by affecting crop productivity (and food availability) and indirectly by decreasing the nutritional quality of plant-based foods and feeds (Soares et al., 2019a). This could exacerbate the existing challenges concerning dietary deficiencies and depletion of agronomic resources. Consider: 20% of EU deaths are attributable to unhealthy diets that are otherwise preventable and current food systems are failing to guide citizens, and especially those who are most vulnerable, toward sustainable, and healthy diets that are available, affordable, appealing, and aspirational. In this context, legumes have a major role to play (Abarca-Gómez et al., 2017). One of the best-known nutrients to be affected by climate change, particularly elevated CO2, is protein (Soares et al., 2019b). Dried legumes grains are excellent sources of protein/amino acids, fatty acids, fibers, carbohydrates, and phytochemicals, such as polyphenols (including flavonoids, and catechins) (Carbas et al., 2020). Dried grains of pulses also contain carbohydrates which promote lowered glycaemic index, so avoiding peaks in blood glucose levels that are a contributory factor to Type-2 diabetes—a major diet-related global-health issue and socioeconomically crippling health-care-cost burden (American Diabetes Association, 2013; Seuring et al., 2015). Grain legume consumption has also implicated in a reduced level of mortality (8% reduction) among the long-lived elderly, and regardless of ethnicity (Darmadi-Blackberry et al., 2004). Such nutritional health benefits are not restricted to provisions for humans (Vasconcelos et al., 2020), since as already indicated above, these can extend to improved ecosystem functions from provisions to above- and below-ground biodiversity (Altieri, 1999), and may be extended to farm animal health too via nutritional and non-nutritional capacities (Dixon and Sumner, 2003).
It is in these three critical contexts, the climate-biodiversity-nutrition nexus, that an EU-funded project structure (www.true-project.eu) was conceived and designed around a specific holistic framework (Figure 1) based on the Three Pillars of Sustainability (Passet, 1979). This aspirational structure leads one naturally to identify those accounting and functional indicators which may be effective to help define and better-manage food- and feed-systems. For example, the FAO has identified 17 Sustainable Development Goals (SDGs) and 167 sustainable development indicators (SDIs) (FAO, 2021), which are mostly operationalized in bioregional and international decision-making. However, SDGs and SDIs developed for the national and international contexts are not operational overs shorter value chains, bioregional scales, and are devoid of any specific reference to levels of legume-inclusive food- and feed-value chains. This is critical, since it is the implementation of good agronomic practices and business governance at bioregional scales which will determine the improved management of key resources (energy, carbon, nitrogen, and phosphorus) necessary to safeguard water quality, optimize soil function, and facilitate the necessary levels of crop diversification to minimize biodiversity loss, whilst also delivering optimized nutrition in an equitable manner.
Figure 1. The focal structure and ambition of the EU-funded TRUE-Project has adapted a functional perspective of the Three Pillars of Sustainability (economic, environmental, and social) and with respect to the UN Sustainable Development Goals. The TRUE-Project identifies focal indicators for each Pillar which are built into the TRUE “Pathfinder” Decision Support System [work package WP 8], and value chain sustainability assessment tool. This schematic diagram is adapted from Figure 3 of Vasconcelos et al. (2020), to highlight where the TRUE-WPs reside relative to the “Pillar-WPs” (5, 6, and 7). Furthermore, the structure highlights the pivotal role of policy and good-governance to ensure safeguarding environmental function and nutritional qualities which also ensure good-health and well-being—since these core elements should be harmonized with a more-facilitative economic framework.
The potential of whole (cropped) system-interventions and -function indicators for cropped systems at more practical field-, farm-, and regional-scales has been demonstrated (Hawes et al., 2019, 2021). Field- and farm-scale system function indicators have been allied to the evolution of approaches for whole system accounting, from farm-to-fork. These include forms of Life Cycle Assessment (LCA) which seek to move beyond environmental footprints based solely on emissions and resource consumption per unit of a commoditized (food) product, to include system functions as important co-products delivered by farm systems (Zhang et al., 2010a,b). Such an approach can help to broaden sustainability-accounting measures provided by LCA, to functional parameters that can be applied at bioregional-scales from the field to bioregion (e.g., Patouillard et al., 2016). The utility of bioregionalized food system approaches has also been recently demonstrated via place-based short-value chains which compensate for the limitations of the global food system manifested during the COVID-19 pandemic (Laborde et al., 2020; Rivington et al., 2021).
In these contexts, the history of policy effort from within the EC has been strongly directed toward increased production of grain legumes within Europe and such effort was initiated with the launch of the European Soya Declaration (2017), which has informed development of the ECs “Plant Protein Plan” (PPP) (EC, 2018). However, the “Plant Protein Plan” has been perceived by some stakeholder groups as an “animal feed self-sufficiency plan,” encouraging misuse of food-land for continued protein over-consumption (Westhoek et al., 2011)—with significant negative health, as well as environmental, impacts (Walker et al., 2005). Consequently, the climate change-biodiversity-health nexus has also informed formulation of the “Planetary Health Diet” (Willett et al., 2019) among others, and which all agree that consumption of fruits, vegetables, nuts, and legumes by humans should increase (2-fold), while red meat and sugar consumption must reduce (by half)—a dietary shift pattern which has also been advocated by the Intergovernmental Panel on Climate Change (IPCC) (Shukla et al., 2019). Here again we highlight that legume-based food systems would lower food-security risk due to the significant land-use sparing can be accommodated from even moderate (10%) offsetting of meat consumption supported by legume-derived protein (Searchinger et al., 2019; Table 6-2, p. 81).
Additional follow-up recommendations to the original Plant Protein Plan highlighted that efforts should focus on the development of infrastructure for processing plant proteins for food (Clément et al., 2018). In line with the EC recommendations, national plant protein plans are being developed by member states, and while some approaches simply seek to design and implement strategies to establish greater levels of plant-protein self-sufficiency, these do not necessarily recommend definitive targets with respect to domestic bioregionalized value chains (production and consumption) of legume protein. Others which aim to establish protein plans as one component a foundation for “sustainable food systems” more generally, and these approaches should set legumes apart from other crops based on their functional potentials. However, some approaches recognize legumes only as another protein crop alongside other non-nitrogen crops i.e., ignoring the critical functional benefits offered by legumes such as biological nitrogen fixation, GHG mitigation, soil-carbon sequestration, biodiversity functions, food-culture, and complex provision of nutritional and non-nutritional dietary components.
Historically, policy-led food system transformation has been mainly realized via efforts focused on the production system as a main point of entry, such as increasing yields and crop diversification. While such an entry-approach has obvious value, there appears to be insufficient focus of policy on ex-farm gate and market “facilitation” and “pull” factors (Vasconcelos et al., 2020; Balázs et al., 2021). Allied to this, the confused policy framework (Balázs et al., 2021), and role of specific actors in the ex-farm gate sector are also neglected or ignored. As such, “integrated practices” must not be restricted to the cropped system but extended across the full value chain. System function indictors such as gender equity and social justice also emerge as features which should be allied to greater legume-use and food-system resilience (Schipanski et al., 2016).
Consider the simple schematic shown in Figure 2 (developed from Hamann et al., 2018), which shows that value-chain “pinch-points” may be perceived as risks or lock-ins. However, the same pinch-points may also be viewed as an opportunity for positive transformative change toward more sustainable and healthy diets. For example, perhaps encouraging the development of grain legume processing capacities (dehulling, milling, fractionation) among seed aggregators which serve as major processors for key and emerging markets. Though this may also involve justifying the use of those raw materials in their existing product range. Nevertheless, while capacities for plant-based food increase in response to rising consumer demand and commercial opportunity, where these openings are legume-based, there is no expectation that even a proportion of the legume crops must be grown bioregionally. Nor does this preclude the possibility that such plant-based products present high GHGs costs or/and poor nutrition risks (through ultra-processing). A key question for consumers and concerned value-chain stakeholders including legume-based feed processors, food manufacturers, wholesalers, retailers, and especially policy makers, might be: to what extent does my consumption, product, or raw-material choice improve the function of production ecosystems and the sustainability of the value chains, bioregionally, and globally? The success of any resultant initiatives to increase production and consumption of bioregionally grown legumes is likely to be governed by the ability to recruit and mentor a diverse array of “collaborative capacity builders” (c.f. Weber and Khademian, 2008). Such individuals will be essential to help establish and ensure the necessary business-to-business knowledge-networks, -flows, -capacities, and transformative governance structures, to help solve the wicked problems posed by the climate-biodiversity-nutrition nexus.
Figure 2. Adapted from the TRUE-Project WP4 Deliverable of Hamann et al. (2018), the schematic value chain pattern shown here is commonly associated with modern large-scale industrialized feed- and food-based value chains. It highlights “pushing” and “pulling” segments, and specific “key stakeholder” groups, and downstream stakeholder-interfaces to help drive positive transformative food-system change. While the number of “Key Stakeholders” is relatively low at specific value-chain segments (or, “pinch-points”) and may reinforce risks and impacts of current lock-ins, the same “pinch-points” also offer focal groups of value chain control and transformation toward new and more-sustainable norms.
This process was led and directed by PI (first and corresponding author), and the foundation determined in close collaboration and direction with CH, RV, and RR (second, penultimate, and final authors, respectively). The order of remaining authors reflects the strategic order of TRUE-Project work package (WP) leaders and co-leaders (WP 1-8). All authors contributed to conceptualization, writing, literature and methodology reviews, manuscript-editing, and funding acquisition.
Open-Access Deliverables and peer-reviewed publications relating to this perspective may be found at www.true-project.eu (and forth-coming, the www.legumeinnovationnetwork.eu), which received funding from the European Union's Horizon 2020 Research and Innovation Programme under Grant Agreement number 727973. PI is also supported by: www.plant-teams.eu and www.tomres.eu, which has also received funding EU Horizon 2020 under Grant Agreement numbers 727284 and 727929, respectively. The James Hutton Institute and SRUC are supported by the Rural & Environment Science & Analytical Services (RESAS), a division of the Scottish Government. MV acknowledges scientific support from the Fundação Ciência e Tecnologia (Portugal) project, UIDB/50016/2020. EK acknowledges the support of the János Bolyai Research Scholarship of the Hungarian Academy of Sciences.
The authors declare that the research was conducted in the absence of any commercial or financial relationships that could be construed as a potential conflict of interest.
All claims expressed in this article are solely those of the authors and do not necessarily represent those of their affiliated organizations, or those of the publisher, the editors and the reviewers. Any product that may be evaluated in this article, or claim that may be made by its manufacturer, is not guaranteed or endorsed by the publisher.
The Supplementary Material for this article can be found online at: https://www.frontiersin.org/articles/10.3389/fsufs.2021.692137/full#supplementary-material
Supplementary Figure 1. This flow chart depicts in simple terms the main legume types and their basic utilities.
Supplementary Figure 2. The grain- and forage-legume proportion as a fraction of the total legume proportion (grain and forage) across all the legume-supported rotations assessed by Iannetta et al. (2016), and available from the EU-funded project Legume Futures (Framework Programme) Data Repository held at the James Hutton Institute. The data highlights a relationship between relative uptake of grain- and forage-legume types.
Abarca-Gómez, L., Abdeen, Z. A., Hamid, Z. A., Abu-Rmeileh, N. M., Acosta-Cazares, B., Acuin, C., et al. (2017). Worldwide trends in body-mass index, underweight, overweight, and obesity from 1975 to 2016: a pooled analysis of 2416 population-based measurement studies in 128·9 million children, adolescents, and adults. Lancet 390, 2627–2642. doi: 10.1016/S0140-6736(17)32129-3
Altieri, M. A. (1999). “The ecological role of biodiversity in agroecosystems,” in Invertebrate Biodiversity as Bioindicators of Sustainable Landscapes (Amsterdam: Elsevier), 19–31. doi: 10.1016/B978-0-444-50019-9.50005-4
American Diabetes Association (2013). Economic costs of diabetes in the US in 2012. Diabetes Care 36, 1033–1046. doi: 10.2337/dc12-2625
Ascott, M. J., Gooddy, D. C., Wang, L., Stuart, M. E., Lewis, M. A., Ward, R. S., et al. (2017). Global patterns of nitrate storage in the vadose zone. Nat. Commun. 8:1416. doi: 10.1038/s41467-017-01321-w
Balázs, B., Kelemen, E., Centofanti, T., Vasconcelos, M. W., and Iannetta, P. P. (2021). Integrated policy analysis to identify transformation paths to more-sustainable legume-based food and feed value-chains in Europe. Agroecol. Sustain. Food Syst. 45, 931–953. doi: 10.1080/21683565.2021.1884165
Bengtsson, J., Ahnstrom, J., and Weibull, A.-C. (2005). The effects of organic agriculture on biodiversity and abundance: a meta-analysis. J. Appl. Ecol. 42, 261–269. doi: 10.1111/j.1365-2664.2005.01005.x
Bonini, I., Marimon-Junior, B. H., Matricardi, E., Phillips, O., Petter, F., Oliveira, B., et al. (2018). Collapse of ecosystem carbon stocks due to forest conversion to soybean plantations at the Amazon-Cerrado transition. For. Ecol. Manage. 414, 64–73. doi: 10.1016/j.foreco.2018.01.038
Breitburg, D., Levin, L. A., Oschlies, A., Grégoire, M., Chavez, F. P., Conley, D. J., et al. (2018). Declining oxygen in the global ocean and coastal waters. Science 359:7240. doi: 10.1126/science.aam7240
Brooker, R. W., Bennett, A. E., Cong, W.-F., Daniell, T. J., George, T. S., Hallett, P. D., et al. (2014). Improving intercropping: a synthesis of research in agronomy, plant physiology and ecology. N. Phytol. 206, 107–117. doi: 10.1111/nph.13132
Brooks, J., and Matthews, A. (2015). “Trade dimensions of food security,” in OECD Food, Agriculture and Fisheries Papers, No. 77. Paris: OECD Publishing.
CAPRI Dataset (2020). CAPRI Modelling System. Available online at: https://www.capri-model.org/dokuwiki/doku.php?id=capri:concept:market (accessed March 31, 2021).
Carbas, B., Machado, N., Oppolzer, D., Ferreira, L., Brites, C., Rosa, E. A., et al. (2020). Comparison of near-infrared (NIR) and mid-infrared (MIR) spectroscopy for the determination of nutritional and antinutritional parameters in common beans. Food Chem. 306:125509. doi: 10.1016/j.foodchem.2019.125509
Clark, M., and Tilman, D. (2017). Comparative analysis of environmental impacts of agricultural production systems agricultural input efficiency and food choice. Environ. Res. Lett. 12:064016. doi: 10.1088/1748-9326/aa6cd5
Clark, M. A., Domingo, N. G. G., Colgan, K., Thakrar, S. K., Tilman, D., Lynch, J., et al. (2020). Global food system emissions could preclude achieving the 1.5° and 2°C climate change targets. Science 370, 705–708. doi: 10.1126/science.aba7357
Clement, S. L., Wightman, J. A., Hardie, D. C., Bailey, P., Baker, G., and McDonald, G. (2000). “Opportunities for integrated management of insect pests of grain legumes,” in ‘Linking Research and Marketing Opportunities for Pulses in the 21st Century’. Vol 34. ed R. Knight (Dordrecht: Springer), 467–480. doi: 10.1007/978-94-011-4385-1_43
Clément, T., Joya, R., Bresson, C., and Clément, C. (2018). Market Developments and Policy Evaluation Aspects of the Plant Protein Sector in the EU. Brussels: Agrosynergie EEIG for the European Commission.
Darmadi-Blackberry, I., Wahlqvist, M. L., Kouris-Blazos, A., Steen, B., Lukito, W., Horie, Y., et al. (2004). Legumes: the most important dietary predictor of survival in older people of different ethnicities. Asia Pac. J. Clin. Nutr. 13, 217–220. Available online at: http://citeseerx.ist.psu.edu/viewdoc/download?doi=10.1.1.538.8279&rep=rep1&type=pdf
Dixon, R. A., and Sumner, L. W. (2003). Legume natural products: understanding and manipulating complex pathways for human and animal health. Plant Physiol. 131, 878–885. doi: 10.1104/pp.102.017319
EC (2018). On the Development of Plant Proteins in the European Union. A Report From the Commission to the Council and the European Parliament.
EC (2019). The European Green Deal. Brussels, 1–24. Available online at: https://eur-lex.europa.eu/resource.html?uri=cellar:b828d165-1c22-11ea-8c1f-01aa75ed71a1.0002.02/DOC_1&format=PDF (accessed June 29, 2021).
EC (2020). Commission Publishes EU Feed Protein Balance Sheet for 2019/20. European Commission (europa.eu). Available online at. https://ec.europa.eu/info/news/commission-publishes-eu-feed-protein-balance-sheet-2019-20-2020-jul-01_en (accessed June 29, 2021).
Eory, V., Topp, K., Rees, B., Leinonen, I., and Maire, J. (2020). Marginal Abatement Cost Curve for Scottish Agriculture. Scotland's Rural College. Available online at: https://era.ed.ac.uk/handle/1842/37470 (accessed June 29, 2021).
European Soya Declaration (2017). Enhancing Soya and Other Legumes Cultivation. Available online at: https://www.donausoja.org/fileadmin/user_upload/Activity/Media/European_Soya_signed_declaration.pdf
FAO (2021). Sustainable Development Goals. Available online at: http://www.fao.org/sustainable-development-goals/en/ (accessed June 29, 2021).
FAOSTAT (2019). Compare Livestock Primary Production Data 1961-2019. Available online at: http://www.fao.org/faostat/en/#compare (accessed March 31, 2021).
Folke, C., Polasky, S., Rockström, J., Galaz, V., Westley, F., Lamont, M., et al. (2021). Our future in the Anthropocene biosphere. Ambio 50, 834–869. doi: 10.1007/s13280-021-01544-8
Foyer, C. H., Lam, H. M., Nguyen, H. T., Siddique, K. H., Varshney, R. K., Colmer, T. D., et al. (2016). Neglecting legumes has compromised human health and sustainable food production. Nat. Plants 2:16112. doi: 10.1038/nplants.2016.112
Hallmann, C. A., Sorg, M., Jongejans, E., Siepel, H., Hofland, N., Schwan, H., et al. (2017). More than 75 percent decline over 27 years in total flying insect biomass in protected areas. PLoS ONE 12:e0185809. doi: 10.1371/journal.pone.0185809
Hamann, K., Tran, F., Varandas, E., Balázs, B., Kelemen, E., and Iannetta, P. P. M. (2018). Report on Public and Private Procurement (D4.4) for the EU-H2020 funded project, ‘TRansition paths to sUstainable legume-based systems in Europe’ (TRUE), under Grant Agreement Number 727973. doi: 10.21820/23987073.2018.6.85
Hawes, C., Begg, G. S., Squire, G. R., and Iannetta, P. P. M. (2005). Individuals as the basic accounting unit in studies of ecosystem function: functional diversity in shepherd's purse, Capsella. Oikos 109, 521–534. doi: 10.1111/j.0030-1299.2005.13853.x
Hawes, C., Iannetta, P. P. M., and Squire, G. R. (2021). Agroecological practices for whole-system sustainability. CAB Rev. 16:5. doi: 10.1079/PAVSNNR202116005
Hawes, C., Young, M., Banks, G., Begg, G., Christie, A., Iannetta, P., et al. (2019). Whole-systems analysis of environmental and economic sustainability in arable cropping systems: a case study. Agronomy 9:438. doi: 10.3390/agronomy9080438
Hoegh-Guldberg, O., Jacob, D., Bindi, M., Brown, S., Camilloni, I., Diedhiou, A., et al. (2018). Impacts of 1.5 C Global Warming on Natural and Human Systems. Global Warming of 1.5 oC. An IPCC Special Report. Geneva: IPCC Secretariat.
Iannetta, P. P. M., Young, M., Bachinger, J., Bergkvist, G., Lopez-Bellido, R. J., Doltra, J., et al. (2016). A comparative nitrogen balance and productivity analysis of legume and non-legume supported cropping systems: the potential role of biological nitrogen fixation. Front. Plant Sci. 7:1700. doi: 10.3389/fpls.2016.01700
Intergovernmental Science-Policy Platform on Biodiversity and Ecosystem Services (IPBES) (2019). Global Assessment Report on Biodiversity and Ecosystem Services of the Intergovernmental Science-Policy Platform on Biodiversity and Ecosystem Services, eds E. S. Brondizio, J. Settele, S. Díaz, and H. T. Ngo (Bonn: IPBES secretariat).
Kebede, E. (2020). Grain legumes production and productivity in Ethiopian smallholder agricultural system, contribution to livelihoods and the way forward. Cogent Food Agric. 6:1722353. doi: 10.1080/23311932.2020.1722353
Kuhlman, T., Helming, J., and Oudendag, D. (2014). “Policy impacts on legume-based agriculture at EU level,” in Paper Presented at the EAAE 2014 Congress ‘Agri-Food and Rural Innovation for Healthier Societies’ (Ljubljana).
Laborde, D., Martin, W., Swinnen, J., and Vos, R. (2020). COVID-19 risks to global food security. Science 369, 500–502. doi: 10.1126/science.abc4765
Leinonen, I., Iannetta, P. P. M., MacLeod, M., Rees, R. M., Russell, W., Watson, C., et al. (2020). Regional land use efficiency and nutritional quality of protein production. Global Food Security 26:100386. doi: 10.1016/j.gfs.2020.100386
Lin, B. B. (2011). Resilience in agriculture through crop diversification: adaptive management for environmental change. BioScience 61, 83–193. doi: 10.1525/bio.2011.61.3.4
Luscher, A., Mueller-Harvey, I., Soussana, J. F., Rees, R. M.1, and Peyraud, J.L. (2014). Potential of legume-based grassland-livestock systems in Europe. Grass Forage Sci. 69, 206–228. doi: 10.1111/gfs.12124
Nassary, E. K., Baijukya, F., and Ndakidemi, P. A. (2019). Sustainable intensification of grain legumes optimizes food security on smallholder farms: a review. Int. J. Agric. Biol. 1–17. doi: 10.17957/ijab/15.1254
Oliveira, M, Castro, C, Coutinho, J, and Trindade, H. (2021). Grain legume-based cropping systems can mitigate greenhouse gas emissions from cereal under Mediterranean conditions. Agric. Ecosyst. Environ. 313, 107406. doi: 10.1016/j.agee.2021.107406
Palomo-Campesino, S., Gonzalez, J. A., and Garcia-Llorente, M. (2018). Exploring the connections between agroecological practices and ecosystem services: a systematic literature review. Sustainability 10:4339. doi: 10.3390/su10124339
Patouillard, L., Bulle, C., and Margni, M. (2016). Ready-to-use and advanced methodologies to prioritise the regionalisation effort in LCA. Matériaux Tech. 104:105. doi: 10.1051/mattech/2016002
Peoples, M. B., Herridge, D. F., and Ladha, J. K. (1995). “Biological nitrogen fixation: An efficient source of nitrogen for sustainable agricultural production?,” in ‘Management of Biological Nitrogen Fixation for the Development of More Productive and Sustainable Agricultural Systems’ - Developments in Plant and Soil Sciences, eds J. K. Ladha and M. B. Peoples (Dordrecht: Springer), 3–28.
Rees, R. M., Augustin, J., Alberti, G., Ball, B. C., Boeckx, P., Cantarel, A., et al. (2013). Nitrous oxide emissions from European agriculture–an analysis of variability and drivers of emissions from field experiments. Biogeosciences 10, 2671–2682. doi: 10.5194/bg-10-2671-2013
Rivington, M., King, R., Duckett, D., Iannetta, P., Benton, T. G., Burgess, P. J., et al. (2021). UK food and nutrition security during and after the COVID-19 pandemic. Nutr. Bull. 46, 88–97. doi: 10.1111/nbu.12485
Rochette, P., and Janzen, H. (2005). Towards a revised coefficient for estimating N2O emissions from legumes. Nutrient Cycl. Agroecosyst. 73, 171–179. doi: 10.1007/s10705-005-0357-9
Röös, E., Mie, A., Wivstad, M., Salomon, E., Johansson, B., Gunnarsson, S., et al. (2018). Risks and opportunities of increasing yields in organic farming. a review. Agron. Sustain. Dev. 38:14. doi: 10.1007/s13593-018-0489-3
Root, R. B. (1973). Organization of a plant-arthropod association in simple and diverse habitats: the fauna of collards (Brassica oleracea). Ecol. Monogr. 43, 95–124. doi: 10.2307/1942161
Sanna, A., Uibu, M., Caramanna, G., Kuusik, R., and Maroto-Valer, M. M. (2014). A review of mineral carbonation technologies to sequester CO2. Chem. Soc. Rev. 43, 8049–8080. doi: 10.1039/C4CS00035H
Schipanski, M. E., MacDonald, G. K., Rosenzweig, S., Chappell, M. J., Bennett, E. M., Kerr, R. B., et al. (2016). Realizing resilient food systems. BioScience 66, 600–610. doi: 10.1093/biosci/biw052
Searchinger, T., Waite, R., Hanson, C., Ranganathan, J., Dumas, P., Matthews, E., et al. (2019). Creating a Sustainable Food Future: A Menu of Solutions to Feed Nearly 10 Billion People by 2050. Final Report. Washington, DC: World Resources Institute.
Seuring, T., Archangelidi, O., and Suhrcke, M. (2015). The economic costs of type 2 diabetes: a global systematic review. Pharmacoeconomics 33, 811–831. doi: 10.1007/s40273-015-0268-9
Sharma, H. C., Srivastava, C. P., Durairaj, C., and Gowda, C. L. L. (2010). “Pest management in grain legumes and climate change,” in ‘Climate Change and Management of Cool Season Grain Legume Crops’ (Dordrecht: Springer), 115–139. doi: 10.1007/978-90-481-3709-1_7
Shukla, P. R., Skea, J., Calvo Buendia, E., Masson-Delmotte, V., Pörtner, H. O., Roberts, D. C., et al. (2019). Climate Change and Land: an IPCC Special Report on Climate Change, Desertification, Land Degradation, Sustainable Land Management, Food Security, and Greenhouse Gas Fluxes in Terrestrial Ecosystems. Available online at: https://spiral.imperial.ac.uk/bitstream/10044/1/76618/2/SRCCL-Full-Report-Compiled-191128.pdf (accessed June 29, 2021).
Soares, J., Deuchande, T., Valente, L. M., Pintado, M., and Vasconcelos, M. W. (2019b). Growth and nutritional responses of bean and soybean genotypes to elevated CO2 in a controlled environment. Plants 8:465. doi: 10.3390/plants8110465
Soares, J. C., Santos, C. S., Carvalho, S. M., Pintado, M. M., and Vasconcelos, M. W. (2019a). Preserving the nutritional quality of crop plants under a changing climate: importance and strategies. Plant Soil 443, 1–26. doi: 10.1007/s11104-019-04229-0
Stagnari, F., Maggio, A., Galieni, A., and Pisante, M. (2017). Multiple benefits of legumes for agriculture sustainability: an overview. Chem. Biol. Technol. Agric. 4, 1–13. doi: 10.1186/s40538-016-0085-1
Steffen, W., Broadgate, W., Deutsch, L., Gaffney, O., and Ludwig, C. (2015). The trajectory of the Anthropocene: the great acceleration. Anthropocene Rev. 2, 81–98. doi: 10.1177/2053019614564785
United Nations (2015). Paris Agreement. In, ‘Report of the Conference of the Parties to the United Nations Framework Convention on Climate Change’. United Nations Treaty Collection, 1–27. Available online at: https://unfccc.int/sites/default/files/english_paris_agreement.pdf (accessed June 29, 2021).
Vasconcelos, M., Gomes, A., Pinto, E., Ferreira, H., Vieira, E., Pimenta, A., et al. (2020). “The push-, pull- and enabling -capacities necessary for legume grain inclusion into sustainable agri-food systems and healthy diets. Chapter 1,” in ‘Hidden Hunger and the Transformation of Food Systems. How to Combat the Double Burden of Malnutrition?’, ed H. K. Biesalski (Basel: Karger), 121. doi: 10.1159/000507498
Walker, P., Rhubart-Berg, P., McKenzie, S., Kelling, K., and Lawrence, R. S. (2005). Public health implications of meat production and consumption. Public Health Nutr. 8, 348–356. doi: 10.1079/PHN2005727
Watson, C. A., Reckling, M., Preissel, S., Bachinger, J., Bergkvist, G., Kuhlman, T., et al. (2017). Grain legume production and use in European agricultural systems. Adv. Agron. 144, 235–303. doi: 10.1016/bs.agron.2017.03.003
Weber, E. P., and Khademian, A. M. (2008). Wicked problems, knowledge challenges, and collaborative capacity builders in network settings. Public Adm. Rev. 68, 334–349. doi: 10.1111/j.1540-6210.2007.00866.x
Westhoek, H. J., Rood, G. A., van den Berg, M., Janse, J. H., Nijdam, D. S., Reudink, M. A., et al. (2011). The protein puzzle: the consumption and production of meat, dairy and fish in the European Union. Europ. J. Nutr. Food Safety. 1, 123–144. Available online at: https://www.journalejnfs.com/index.php/EJNFS/article/view/30006
Wiggins, S. (2009). Can the Smallholder Model Deliver Poverty Reduction and Food Security. Future Agricultures, Working Paper 8. Available online at: https://opendocs.ids.ac.uk/opendocs/handle/20.500.12413/2338 (accessed June 29, 2021).
Willett, W., Rockström, J., Loken, B., Springmann, M., Lang, T., Vermeulen, S., et al. (2019). Food in the Anthropocene: the EAT–Lancet Commission on healthy diets from sustainable food systems. Lancet 393, 447–492. doi: 10.1016/S0140-6736(18)31788-4
Wollenberg, E., Richards, M., Smith, P., Havlík, P., Obersteiner, M., Tubiello, F. N., et al. (2016). Reducing emissions from agriculture to meet the 2 C target. Glob. Chang. Biol. 22, 3859–3864. doi: 10.1111/gcb.13340
Zhang, Y. I., Baral, A., and Bakshi, B. R. (2010b). Accounting for ecosystem services in life cycle assessment, part II: toward an ecologically based LCA. Environ. Sci. Technol. 44, 2624–2631. doi: 10.1021/es900548a
Keywords: climate change, biodiversity, nutrition, legumes (Fabaceae), value chain, food system
Citation: Iannetta PPM, Hawes C, Begg GS, Maaß H, Ntatsi G, Savvas D, Vasconcelos M, Hamann K, Williams M, Styles D, Toma L, Shrestha S, Balázs B, Kelemen E, Debeljak M, Trajanov A, Vickers R and Rees RM (2021) A Multifunctional Solution for Wicked Problems: Value-Chain Wide Facilitation of Legumes Cultivated at Bioregional Scales Is Necessary to Address the Climate-Biodiversity-Nutrition Nexus. Front. Sustain. Food Syst. 5:692137. doi: 10.3389/fsufs.2021.692137
Received: 07 April 2021; Accepted: 17 June 2021;
Published: 23 July 2021.
Edited by:
Sanzidur Rahman, Shandong University of Finance and Economics, ChinaReviewed by:
Aditya Pratap, Indian Institute of Pulses Research (ICAR), IndiaCopyright © 2021 Iannetta, Hawes, Begg, Maaß, Ntatsi, Savvas, Vasconcelos, Hamann, Williams, Styles, Toma, Shrestha, Balázs, Kelemen, Debeljak, Trajanov, Vickers and Rees. This is an open-access article distributed under the terms of the Creative Commons Attribution License (CC BY). The use, distribution or reproduction in other forums is permitted, provided the original author(s) and the copyright owner(s) are credited and that the original publication in this journal is cited, in accordance with accepted academic practice. No use, distribution or reproduction is permitted which does not comply with these terms.
*Correspondence: Pietro P. M. Iannetta, cGV0ZS5pYW5uZXR0YUBodXR0b24uYWMudWs=
Disclaimer: All claims expressed in this article are solely those of the authors and do not necessarily represent those of their affiliated organizations, or those of the publisher, the editors and the reviewers. Any product that may be evaluated in this article or claim that may be made by its manufacturer is not guaranteed or endorsed by the publisher.
Research integrity at Frontiers
Learn more about the work of our research integrity team to safeguard the quality of each article we publish.