- 1Division of Plant Biotechnology, Entomology Research Institute, Loyola College, Chennai, India
- 2Department of Biosciences, Rajagiri College of Social Sciences, Cochin, India
- 3Xavier Research Foundation St. Xavier's College, Tirunelveli, India
Finger millet plays a vital role in the food and nutritional security of many people in developing countries particularly in Asia and Africa. It is a staple food for poor people in many regions of Asian (India, China, Nepal, and Sri Lanka etc.) and African (South Africa, Ethiopia, Kenya, Uganda, and Nigeria etc.) countries. Finger millet contains nutrient rich components such as dietary fibers, minerals, vitamins, and phytochemicals that include phenolic compounds with several potential health benefits. Calcium (Ca) is an important macronutrient for healthy life of plants, humans and animals. It plays an indispensable role in structure and signaling and its deficiency causes low bone density, osteoporosis, colon cancer etc. Finger millet grains contain exceptionally higher amount of Ca (>300 mg/100 g) when compared to other major cereals. Ca transporter and sensor family genes are involved in the uptake, transport and accumulation of Ca. Understanding the molecular mechanisms of Ca transporter and sensor family genes is important for growth, development and seed fortification in finger millet. Expression analysis of Ca transporter and sensor family genes has been carried out in various tissues of finger millet. Only a very little research work has been done to understand the Ca accumulation in the grains of finger millet. In this review, we discuss the nutritional importance and health benefits of finger millet. We discuss the studies on Ca sensor, accumulation and transport genes that help to improve the grains of finger millet with special reference to Ca. Improved Ca content in finger millet may help to alleviate the Ca deficiency throughout the world particularly in the semi-arid tropics of Asia and Africa.
Introduction
Food security remains a major challenge especially in less developed countries. Orphan crops like cereals, legumes, vegetables and tubers play a vital role in the food security and livelihood of resource poor farmers and consumers in the developing countries of Asia and Africa (Tadele, 2019). Cereals including rice, wheat, maize, sorghum and millets are rich sources of nutrients for humans and animals in these countries. Finger millet (Eleusine coracana) is a nutrient rich crop. Finger millet is being used as food (grains) in developing countries and as animal feed (straw) in developed countries indicating that it is considered as a poor man's food (Ceasar et al., 2018; Wambi et al., 2020). It is a major crop in the arid and semiarid regions of developing countries of Asia and Africa (Ceasar et al., 2018; Krishna et al., 2018, 2020). Among the various millets, finger millet ranks fourth on a global scale of production next to sorghum (Sorghum bicolor), pearl millet [Cenchrus americanus (new name), Pennisetum glaucum (old name)] and foxtail millet (Setaria italica) (Upadhyaya et al., 2007; Maharajan et al., 2019). Millets are also nutritionally superior to rice and wheat since their grains contain high amounts of vitamins, iron, carbohydrate, calcium (Ca), potassium, zinc, phosphorus, magnesium, and essential amino acids (Saleh et al., 2013).
Ca is required for a number of basic regulatory functions such as transmission of nerve impulses, contraction and relaxation of muscles, blood coagulation cascade, activation of enzymes, stimulation of hormonal secretion and so on in human body and so on (Pravina et al., 2013). It also helps in the protection from cancers including colorectal, ovarian, breast, and prostate cancers (Goodman et al., 2002; Gao et al., 2005; Lin et al., 2007). Intake of Ca is important for development of fetal skeleton, increasing birth weight prenatal hypertension and avoidance of pre-clamsis in pregnant women (Chan et al., 2006; Puranik et al., 2017). Around the world, 3.5 billion people are affected by Ca deficiency, with ~90% of people affected in Africa and Asia (Kumssa et al., 2015). Ca deficiency is a serious health problem both in the developing countries of Asia and Africa (Sharma et al., 2017). Human diets with low levels of Ca has been linked to diseases such as osteoporosis and indirectly to rickets (through Vitamin D) (Heaney, 1993; Chan et al., 2006; Pettifor, 2008). Such diets with low Ca levels is prevalent among 80% of elderly population and are consequently affected by osteoporosis worldwide (Bhatia, 2008; Pettifor, 2008). Elderly population is mostly affected by Ca deficiency predominantly in the form of osteoporosis and osteopenia (Puranik et al., 2017). The onset of bone decalcification and demineralization leads to reductions in bone mass causing the osteoporosis among above 50 years old men and menopausal women (Michaelsson et al., 2005). In particular, 80% of the women suffer from osteoporosis (Sharma et al., 2017). Therefore, osteoporosis is a major problem in developing countries (Sozen et al., 2017). The World Health Organization (WHO) considered that osteoporosis as the next main public healthcare concern globally, after cardiovascular diseases afflicting almost 75 million people in Europe, the United States of America and Japan. Hypocalcaemia disease occurs in human body when Ca levels are low in the blood (Fong and Khan, 2012). Milk as well as dairy products are one of the major sources of Ca in human diets. If people consume 1 L of milk per day they will get the recommended amount 1000–1300 mg of Ca (Thorning et al., 2016). Most poor people living in the developing countries of Asia and Africa cannot afford to buy and consume 1 L of milk per day (Puranik et al., 2017). These people consume <500 mL of milk per day. Unavailability of recommended amount of milk among poor population and lactose intolerance lead to Ca deficiency (Curry, 2013).
Plants are the cheap, convenient and alternative source of dietary Ca. People frequently consume cereal based food products which are low in Ca. Major cereal crops may not provide adequate amount of Ca for low income people. Finger millet contains higher amount of Ca compared to the other major cereals (Figure 1). For example, the Ca content in finger millet (344 mg/100 g) is almost 10-fold higher than wheat (Triticum aestivum) (41 mg/100 g), maize (Zea mays) (26 mg/100 g) and rice (Oryza sativa) (33 mg/100 g) and three times higher than milk (Kumar et al., 2016). So finger millet is an example of Ca rich crop in developing countries of tropical and subtropical regions. Finger millet grains also contain higher content of minerals such as phosphorus, iron and manganese compared to other cereals (Kumar et al., 2016). The seeds can be stored for more than 5 years without insect damage that makes it a valuable crop for famine hit areas (Latha et al., 2005). Crops such as rice and wheat provide food security, but finger millet provides nutritional security to the world (Devi et al., 2014; Ceasar et al., 2018).
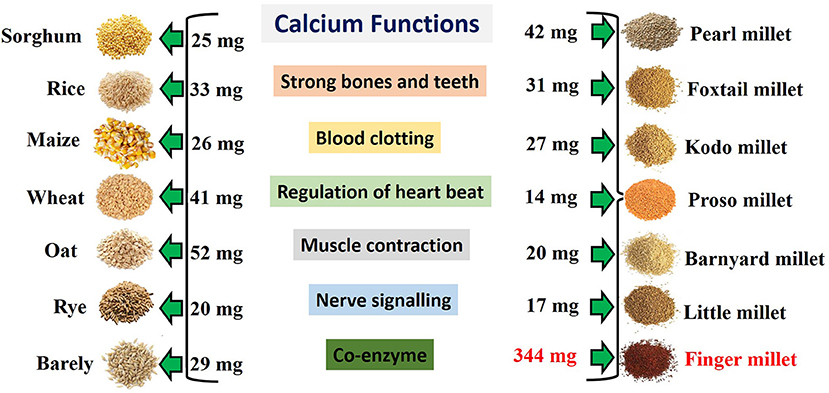
Figure 1. Calcium (Ca) content of various cereals. The Ca content of finger millet and other major cereals and small millets are presented for strengthening the human health. The given Ca content is expressed per 100 g of seeds for all cereals including finger millet. Ca content data of all cereals were collected from Shobana et al. (2013), Saleh et al. (2013), and McKevith (2004).
Plants uptake Ca by root and translocate to the aerial parts of plants including fruits and seeds by symplastic and apoplastic pathways (Kumar et al., 2014; Mirza et al., 2014). In finger millet, Ca is present in aleurone layer followed by seed coat and embryo (Nath et al., 2013). The Ca transporters are actively involved in the uptake and transport of Ca in the cells, while Ca sensors are involved in the regulation of Ca transporters. Only a few Ca transporters have been identified before the releasing of draft genome sequence in finger millet. A few molecular marker-based studies have been conducted to identify the quantitative trait loci (QTL) for Ca traits in finger millet. In this review, we describe the nutritional importance and health benefits of finger millet. We have provided the detailed information on Ca sensor, accumulation and transport genes and identification of QTL related to Ca traits in finger millet.
Nutritional Importance and Health Benefits of Finger Millet
Finger millets serve as a major food in resource-poor countries of Asia and Africa by providing 75% of total calorie intake next to fine cereal grains (Singh et al., 2019) and gluten-free cereal products (Chandrasekara et al., 2012; Saleh et al., 2013). It is nutritionally superior to rice and wheat due to high amount of dietary fibers, iron, zinc, Ca, phosphorus, potassium, vitamin B, and essential amino acids (Parameswaran and Sadasivam, 1994; Ceasar and Ignacimuthu, 2011). Finger millet also possesses more lysine, threonine, and valine than other millets (Ravindran, 1991; Sripriya et al., 1997). It has anti-nutrients including phytates, polyphenols, tannins, trypsin inhibitory factors and dietary fibers. Finger millet's dietary fiber and polyphenols offer various health benefits like antidiabetic, antioxidant, hypocholesterolaemic, antimicrobial, delayed nutrient absorption, increased fecal bulk, lowering of blood lipids effects (Devi et al., 2014). Over 50 phenolic compounds belonging to several classes such as phenolic acids and their derivatives, dehydrodiferulates and dehydrotriferulates, flavan-3-ol monomers and dimers, flavonols, flavones, and flavanonols were identified in finger millet (Saleh et al., 2013).
Finger millet's phenolic compounds like gallic, protocatechuic, p-hydroxy benzoic, p-coumaric, vanillic, syringic, ferulic, trans-cinnamic acids, and quercetin exhibit major antidiabetic and antioxidant properties and also inhibit cataract effectively (Saleh et al., 2013). Finger millet yields less glucose than the polished rice which is good for diabetic patients (Wang et al., 2018). Consumption of finger millet based diets resulted in significantly lower plasma glucose levels due to its higher fiber content and antinutritional factors than rice and wheat (Kumari and Sumathi, 2002). It also has beneficial effect on nerve growth factor production and wound healing properties at early diabetic condition (Rajasekaran et al., 2004). The grains and various fractions of finger millet have the potentials to prevent and treat diabetics (Saleh et al., 2013; Devi et al., 2014; Kam et al., 2016). Globally, most countries face high and increasing rates of cardiovascular diseases due to obesity, smoking, unhealthy diet, and lack of physical activity. This reduce plasma triglycerides in hyperlipidemic rats and prevent cardiovascular disease (Lee et al., 2010). The antioxidants and phenolics rich finger millet grains can also contribute to improve health, to protect against aging and metabolic syndrome (Bravo, 1998). Seed coat extracts of finger millet showed antimicrobial activity against Bacillus cereus and Aspergillus flavus (Radhajeyalakshmi et al., 2003).
Identification of QTL for Ca Traits in Finger Millet
Molecular markers are one of the most crucial diagnostic tools for plant breeding programmes. It is used to analyse genomes and enables the study of the genetic structure and traits of crop genomes. These markers are used to identify the specific genes responsible for better growth, development and resistance to abiotic and biotic stresses. The microsatellite markers have been used to identify the agronomically important traits such as grain yield, quality, disease resistance, drought resistance, nutritional quality, nutritional use-efficiency, etc (Maharajan et al., 2018). The availability of simple sequence repeats (SSR) markers in finger millet is limited compare to those for other cereal crops (Ceasar et al., 2018; Krishna et al., 2018). Only few QTL associated with Ca traits have been reported in finger millet. For example, 23 anchored SSR markers were used to identify nine QTL associated with Ca content in 113 genotypes of finger millet by association mapping (Kumar et al., 2015b). Recently, Yadav et al. (2020) identified two QTL (UGEP78 and UGEP60) for grain calcium content in 238 genotypes of finger millet through association mapping using 85 SSR markers. They also identified two genotypes with the highest grain calcium content [GPHCPB45 (452.8 mg) and (IE 2957 (447 mg)] among the 238 genotypes. This type of study is needed to detect high Ca content genotypes from more than 28,000 accessions of finger millet. The detection of genotypes with high Ca content may help to eradicate Ca deficiency around the world, particularly in Asian and African countries. Only association mapping populations were used for QTL studies so far in finger millet. There is a crucial need to develop the linkage maps for finger millet for the identification of QTL for the agronomically important traits including Ca. High-resolution research on the identification of QTL in finger millet for agronomically important trait is essential for molecular breeding and finger millet improvement. The advanced backcross QTL (AB-QTL) methods are also helpful for a variety development (Kumari et al., 2020). Methods of AB-QTL analysis have been further used to identify and transfer the valuable QTL from un-adapted to cultivated germplasm in a single process (Wang and Chee, 2010; Bhanu et al., 2017). Back cross population (BC2 and BC3) are used for detection of QTL in this method. If worked effectively, AB- QTL analysis can prove to be a potential method to exploit unadapted and exotic germplasm for the quantitative trait improvement of a number of crop plants including finger millet. The released finger millet draft genome provides excellent opportunities to identify expressed sequence tag derived SSR markers for crop improvement (Hittalmani et al., 2017; Ceasar et al., 2018; Pandian and Ramesh, 2019).
Genome-Wide Association Studies for the Enrichment of Nutritive Value in Finger Millet
The introduction of next-genome sequencing (NGS) technology helps for rapid and accurate detection of the genetic basis of phenotypic variation in crops. Due to its significant contribution to genome analysis, it can aid in understanding the genome organization of crops. NGS technology is useful to identify the genetic variability across the genomes of individuals and identify their genotype-phenotype associations in the huge germplasm of the crops. Therefore, genome sequencing plays a vital role in GWAS for crop improvement through a genome-assisted breeding (GAB) program. A very little effort was paid on GWAS in finger millet and only one report is available till date. Six nutritional traits such as iron, zinc, Ca, magnesium, potassium, and sodium were evaluated through GWAS using 190 finger millet genotypes and 418 single nucleotide polymorphism (SNP) linked with nutritional traits were identified (Swati et al., 2020). Out of 418 SNP, 18 SNP markers showed homology with candidate genes having putative functions in binding, remobilization/transport of metal ions in finger millet (Swati et al., 2020). This study provides the insight to genotypic variation of the phenotypes for the selection of the best breeding materials to improve grain nutritional content and nutrient use-efficiency in finger millet. Researchers need to pay more attention to GWAS of finger millet for improving their nutritive traits which may contribute to human health in the future. Furthermore, genotyping by sequencing (GBS) technology generated SNP markers are used to analyze the agro-morphological traits of 113 finger millet accessions and identify consistent SNP markers linked to grain yield and its component traits (Sharma et al., 2018). The identified SNP was associated with the candidate genes of rice and foxtail millet, which were responsible for flowering, maturity, and grain yield (Sharma et al., 2018). In another recent report, Tiwari et al. (2020) identified QTL and genes for seed protein content, days to maturity and grain yield in finger millet using 2977 SNPs by GBS technology. Among these, five SNPs were associated with seed protein content and grain yield and three for days to maturity. They have identified four candidate genes responsible for seed protein content using SNPs through in-silico analysis (Tiwari et al., 2020). Notably, aspartyl protease has been found to be the most promising candidate gene for seed protein content of finger millet. The plant breeders and molecular biologists need to focus more on GWAS in finger millet since it would be a key tool of GAB for finger millet improvement in the future.
Transporters and Sensors for Ca Accumulation in Finger Millet
The Ca is taken up by roots from the soil solution in a divalent cation (Ca2+) form (White and Broadley, 2003). The primary roles of the Ca are maintaining chemical balance in the soil, reducing soil salinity, activating the plant growth regulating enzymes and improving the water penetration and disease resistance in plants. Ca also improves the absorption of the other nutrients by roots and their translocation within the plant (Hepler, 2005). Ca transporters are classified into three types viz. Ca2+ channel, Ca2+ ATPase, and Ca2+ cation exchanger/antiporter (CAX) (Sze et al., 2000; Demidchik et al., 2018). These transporters export Ca2+ to the apoplast and into the cellular organelles specifically to the endoplasmic reticulum, golgi/endosome/pre-vacuolar compartments, and plastids/vacuoles (Karley and White, 2009). Plants absorb Ca by roots from the soil solution, which reaches the shoots through the xylem stream.
Two contrasting finger millet genotypes (GP-1; low Ca containing and GP-45; high Ca containing) were used to identify the expression pattern of CAX1, two pore channel (TPC1), calmodulin (CaM), Ca2+ATPase, and CaM dependent protein kinase (CaMK1 and 2) genes in vegetative tissues and various stages of developing spike in finger millet (Mirza et al., 2014). This study laid the foundation for the identification of Ca transporter genes in finger millet and same genotypes were also utilized in the following studies. Same two genotypes were used to identify two CaM independent protein kinases (CDPK3 and 13), four Calciuneurin B-like protein (CBL)-interacting protein kinases (CIPK2, 4, 10, and 14), one CaM, one TPC1, two CAXs (CAX1 and 3) and four ATPases (CaATPase, CaATPase1, 6 and 9) transporters in developing spikes of finger millet (Kumar et al., 2015a). CIPK10, CDPK13, CIPK4, CaATPase, TPC1 and CAX3 and CaM, CDPK3, CIPK2, CaATPase6, 9, CAX1, and 3 were expressed in developing spikes of GP-45 at S3 and S4 stages respectively (Figure 2). CDPK3, CaM, CIPK14, CaATPase1, TPC1, and CAX1 were expressed in the developing spikes of GP-1 at S4 stage. Among these, expression level of CIPK14 and CaATPase1 were higher in GP-1 at S4 stage compared to other genes (Kumar et al., 2015a). This basic study revealed the spatial and temporal expression of these genes. Therefore, further study is required to characterize both the genes in GP-1 which may help to improve the low Ca containing genotype. The above results show that most of the Ca transport and accumulation genes were expressed in high Ca genotype compared to the low Ca genotype.
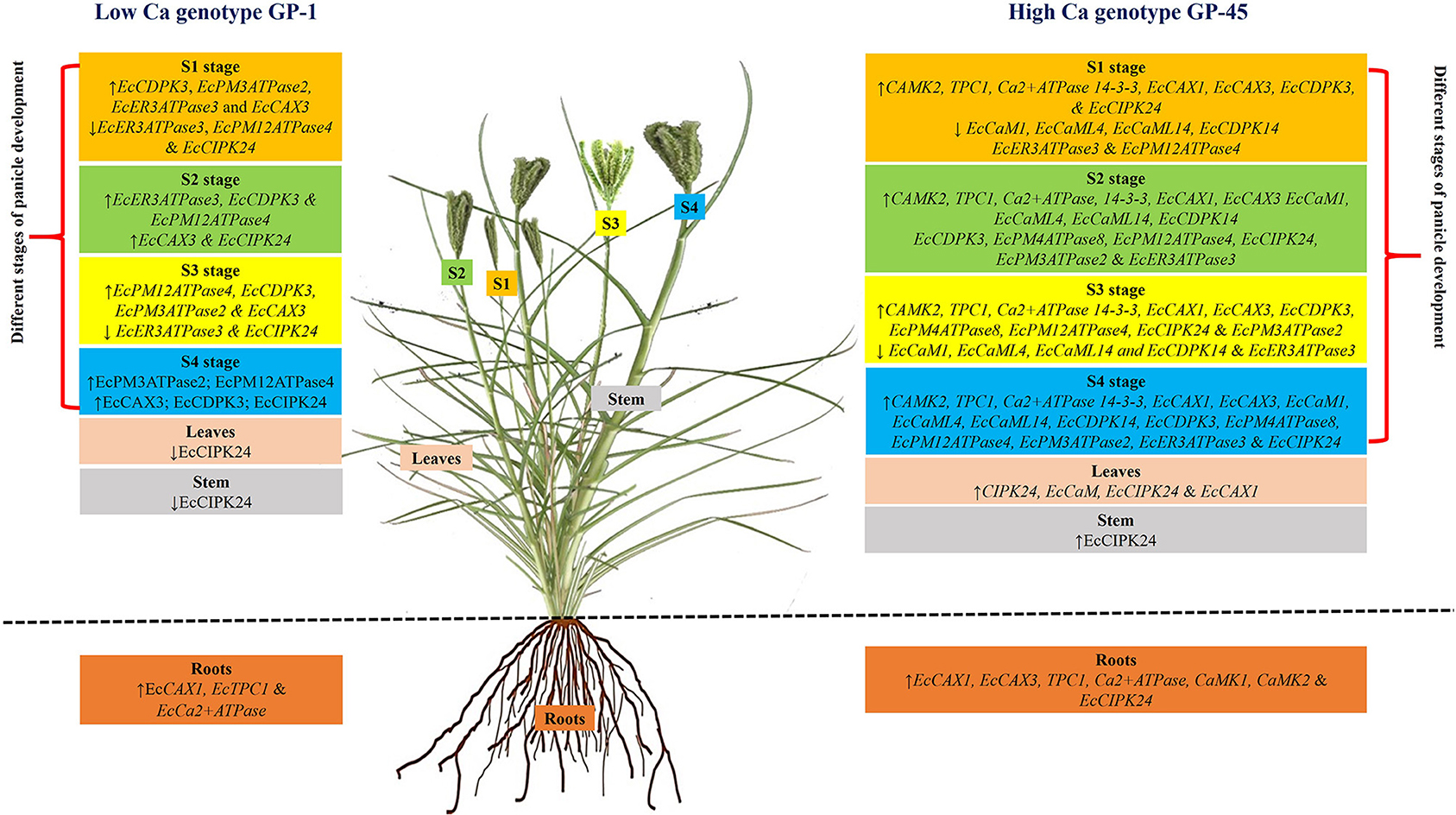
Figure 2. Comparisons between genes related to calcium (Ca) uptake, transport, and accumulation identified in low Ca containing (GP-1) and high Ca (GP-45) containing genotypes. The Ca uptake, transport and accumulation related genes were identified in root, leaves, stems at vegetative stage and developing spikes at various stages of [S1 (booting), S2 (anthesis), S3 (grain ripening), and S4 (maturation)] of finger millet. Stages S1–S4 are marked with a rectangular shape and filled in orange, green, yellow, and blue, respectively. More number of Ca uptake, transport and accumulation genes were expressed in high Ca containing genotype as compared to the low Ca containing genotype.
Likewise, 19 Ca transporter genes, which includes 11 Ca2+ATPases, seven Ca exchangers and one Ca2+ channel genes were identified in GP-1 and GP-45 through RNA-seq (Singh et al., 2015). RNA-seq analysis revealed that nine (EcVCax3, EcER3ATPase3, EcPM12ATPase4, EcPM8ATPase, EcPM5ATPase4, EcPM4ATPase8, EcPM3ATPaseB, EcPM3ATPase2, and EcPM2ATPase1) and three (EcCaX1b, EcCL1pore, and EcPM12ATPase4) genes were highly expressed in GP-45 and GP-1 genotypes, respectively. The EcPM4ATPase8 was highly expressed in developing spike of GP-45 at S2 and S3 stages under 20 mM and 5 mM Ca concentrations, respectively. Ca accumulation in seed is a polygenic trait and Ca2+ transporters (especially EcCAX3) might play very important role in seed Ca accumulation (Singh et al., 2015). This study reported first time an extensive list of Ca2+sensing and transport related genes in finger millet. However, functions of these gene have not yet been validated by knock-out and knock-in studies. Another study shows that Ca sensor gene CIPK (EcCIPK24) was highly expressed in root, shoot, leaves and developing spike of GP-45 compared to GP-1 (Figure 2) (Chinchole et al., 2017). CIPK activates the CAX protein by interaction with CBL proteins. Docking analysis for EcCIPK24 protein of the both genotypes with CBL proteins (EcCBL4 and 10) were performed by Chinchole et al. (2017). The docking study proposed that EcCBL4 has a strong binding affinity with EcCIPK24 and might play a significant role in the accumulation of Ca in seeds (Chinchole et al., 2017). These predictions reveal the functions of the key Ca sensor gene in finger millet and further functional characterization of this gene may help to understand the specific role in finger millet Ca accumulation.
In another study, CAX1, CAX3, CaM, CBL4, CBL10, and CIPK24 genes were identified in roots, stem, leaves and developing spike of GP-1 and GP-45 (Figure 2) (Kokane et al., 2018). Most of the genes were highly expressed in roots (CAX1 and CAX3), leaves (CIPK24), and stems (CIPK24, CaM, and CAX1) of GP-45 genotype compared to GP-1. Much of these works were carried out before the availability of draft genome of finger millet. So the full length gene sequences were not used in designing the primers and other molecular studies. Draft genomes of finger millet were released in 2017 by two groups (Hittalmani et al., 2017; Hatakeyama et al., 2018). Many reviewers have suggested that draft genomes of finger millet will help to improve the finger millet growth and yield through various molecular studies under both biotic and abiotic stress (Ceasar et al., 2018; Vetriventhan et al., 2020; Wambi et al., 2020). Hittalmani et al. (2017) identified 330 Ca transporter and accumulation related genes (28 CaM ATPase, 145 CaMK1, 125 CaMK2, 29 CAX1, and 3 TPC1 genes) through transcriptome analysis. The identified genes will help in exploring more finger millet genotypes for Ca uptake, translocation and accumulation studies in future.
Apart from preliminary studies mentioned above, Ca transport and accumulation related genes have not yet been studied extensively. Overexpression of Ca-signaling transporter genes is directly proportional to increased levels of Ca (Demidchik et al., 2018). For example, overexpression of CAX1 transporter in Arabidopsis thaliana (Hirschi, 2001) and rice (Kim et al., 2005) enhanced the Ca levels in seeds of both the plants. Ca is acquired by root from the soil solution in free ionic form. Once Ca enters inside the root epidermal cells, it can move both apoplastically and symplastically to the cortex and then to the stele (Kumar et al., 2015c). Members of Ca sensors, exchangers and transporters are involved in Ca uptake, transport and accumulation at cellular levels in plants. Seed consists of maternal and filial tissues with no direct vascular connection between them (Wolswinkel et al., 1992). Ca is deposited in seed during its development which is mediated by specialized Ca transporters. It gets pumped toward the apoplast from seed coat, where it is absorbed by aleurone and then in endodermal cell (Sharma et al., 2017). Therefore, further studies to characterize the Ca sensors, exchangers and transporters may help to improve the finger millet growth and especially to accumulate Ca in seeds. When compared to research on Ca transporter family genes in finger millet to that of A. thaliana and rice, very little research has been carried out. Further study on Ca transporters' structure, function, affinity, and other molecular studies will throw more light on the complex function of Ca transporters in Ca uptake, transport, remobilization, and utilization in finger millet. Future high-resolution study on various aspects of Ca transporters in finger millet and the future development of new finger millet varieties with high Ca content will surely aid in enhancing nutritional security of the poor in the developing world.
Conclusion and Future Prospects
Crop improvement has been one of the major priority areas of research in agriculture. Ca deficiency is a widespread problem across the globe, however, very little effort has been made to understand the mechanisms Ca accumulation in finger millet seeds. It may be due the reason that finger millet is being grown and consumed by less developed regions in the world. Further study on the post-transcriptional and post-translational regulation of Ca transporters needs to be fine-tuned to exploit them in finger millet production to meet the ever increasing global population and food demand. Recently, the clustered regularly interspaced short palindromic repeats (CRISPR)/CRISPR associated protein 9 (Cas9) gene-editing tool is projected to play a vital role in crop improvement. CRISPR/Cas9 tool could be applied to Ca transporter genes in future to dissect and improve the Ca accumulation in seeds of finger millet. Finger millet genotype with high Ca content will help to reduce Ca deficiency worldwide.
Bio-fortification is a food based approach to overcome the nutrient starvation by delivering nutrient dense crops at the door steps of poor populations. Bio-fortified crops, particularly finger millet contain high amount of proteins, essential amino acids, minerals, and vitamins which can help to reduce hidden hunger in the most vulnerable populations in the world. Finger millet is called as a super cereal because it is the richest source of Ca among all the cereals. Farmers who grow bio-fortified crops including finger millet can easily access the nutritious foods with minimal investments. Hence the incorporation of naturally Ca-rich cereals like finger millet into global bio-enrichment programs can be a good starting point to alleviate Ca malnutrition.
Author Contributions
TM, TA, and SA conceptualized, wrote, edited, and updated the manuscript. SA and SI contributed critically in revising and improving the manuscript for publication. All authors contributed to the article and approved the submitted version.
Funding
The research in our lab is currently funded by the Department of Biotechnology, Government of India (No: BT/PR21321/GET/119/76/2016).
Conflict of Interest
The authors declare that the research was conducted in the absence of any commercial or financial relationships that could be construed as a potential conflict of interest.
Publisher's Note
All claims expressed in this article are solely those of the authors and do not necessarily represent those of their affiliated organizations, or those of the publisher, the editors and the reviewers. Any product that may be evaluated in this article, or claim that may be made by its manufacturer, is not guaranteed or endorsed by the publisher.
Abbreviations
Ca, Calcium; CaM, calmodulin; CaMK, CaM dependent protein kinase; CAX, Ca2+ cation exchanger/antiporter; CBL, Calciuneurin B-like protein; CDPK, CaM independent protein kinases; CIPK, Calciuneurin B-like protein (CBL)-interacting protein kinases; DAS, days after sowing; QTL, quantitative trait loci; SNP, single nucleotide polymorphism; TPC1, two pore channel.
References
Bhanu, A. N., Yugandhar, G., and Singh, M. N. (2017). Advanced backcross QTL method: a brief overview. Trends Biosci.10, 20–25.
Bravo, L. (1998). Polyphenols: chemistry, dietary sources, metabolism, and nutritional significance. Nutr. Rev. 56, 317–333. doi: 10.1111/j.1753-4887.1998.tb01670.x
Ceasar, S., Maharajan, T., Ajeesh Krishna, T., Ramakrishnan, M., Victor Roch, G., Satish, L., et al. (2018). Finger millet [Eleusine coracana (L.) Gaertn.] improvement: current status and future interventions of whole genome sequence. Front. Plant Sci. 9:1054. doi: 10.3389/fpls.2018.01054
Ceasar, S. A., and Ignacimuthu, S. (2011). Agrobacterium-mediated transformation of finger millet (Eleusine coracana (L.) Gaertn.) using shoot apex explants. Plant Cell Rep. 30, 1759–1770. doi: 10.1007/s00299-011-1084-0
Chan, G. M., McElligott, K., McNaught, T., and Gill, G. (2006). Effects of dietary calcium intervention on adolescent mothers and newborns: a randomized controlled trial. Obstet. Gynecol. 108, 565–571. doi: 10.1097/01.AOG.0000231721.42823.9e
Chandrasekara, A., Naczk, M., and Shahidi, F. (2012). Effect of processing on the antioxidant activity of millet grains. Food Chem. 133, 1–9. doi: 10.1016/j.foodchem.2011.09.043
Chinchole, M., Pathak, R. K., Singh, U. M., and Kumar, A. (2017). Molecular characterization of EcCIPK 24 gene of finger millet (Eleusine coracana) for investigating its regulatory role in calcium transport. 3 Biotech. 7, 1–10. doi: 10.1007/s13205-017-0874-7
Demidchik, V., Shabala, S., Isayenkov, S., Cuin, T. A., and Pottosin, I. (2018). Calcium transport across plant membranes: mechanisms and functions. New Phytol. 220, 49–69. doi: 10.1111/nph.15266
Devi, P. B., Vijayabharathi, R., Sathyabama, S., Malleshi, N. G., and Priyadarisini, V. B. (2014). Health benefits of finger millet (Eleusine coracana L.) polyphenols and dietary fiber: a review. J. Food Sci. Tech. 51, 1021–1040. doi: 10.1007/s13197-011-0584-9
Fong, J., and Khan, A. (2012). Hypocalcemia: updates in diagnosis and management for primary care. Can. Fam. Physician. 58, 158–162.
Gao, X., LaValley, M. P., and Tucker, K. L. (2005). Prospective studies of dairy product and calcium intakes and prostate cancer risk: a meta-analysis. J. Natl. Cancer Inst. 97, 1768–1777. doi: 10.1093/jnci/dji402
Goodman, M. T., Wu, A. H., Tung, K. H., McDuffie, K., Kolonel, L. N., Nomura, A. M., et al. (2002). Association of dairy products, lactose, and calcium with the risk of ovarian cancer. Am. J. Epidemiol. 156, 148–157. doi: 10.1093/aje/kwf022
Hatakeyama, M., Aluri, S., Balachadran, M. T., Sivarajan, S. R., Patrignani, A., Grüter, S., et al. (2018). Multiple hybrid de novo genome assembly of finger millet, an orphan allotetraploid crop. DNA Res. 25, 39–47. doi: 10.1093/dnares/dsx036
Heaney, R. P. (1993). Nutritional factors in osteoporosis. Annu. Rev. Nutr. 13, 287–316. doi: 10.1146/annurev.nu.13.070193.001443
Hepler, P. K. (2005). Calcium: a central regulator of plant growth and development. Plant Cell 17, 2142–2155. doi: 10.1105/tpc.105.032508
Hirschi, K. (2001). Vacuolar H+/Ca2+ transport: who's directing the traffic? Trends Plant Sci. 6, 100–104. doi: 10.1016/S1360-1385(00)01863-X
Hittalmani, S., Mahesh, H., Shirke, M. D., Biradar, H., Uday, G., Aruna, Y., et al. (2017). Genome and transcriptome sequence of finger millet (Eleusine coracana (L.) Gaertn.) provides insights into drought tolerance and nutraceutical properties. BMC Genomics 18:465. doi: 10.1186/s12864-017-3850-z
Kam, J., Puranik, S., Yadav, R., Manwaring, H. R., Pierre, S., Srivastava, R. K., et al. (2016). Dietary interventions for type 2 diabetes: how millet comes to help. Front. Plant Sci. 7:1454. doi: 10.3389/fpls.2016.01454
Karley, A. J., and White, P. J. (2009). Moving cationic minerals to edible tissues: potassium, magnesium, calcium. Curr. Opin. Plant Biol. 12, 291–298. doi: 10.1016/j.pbi.2009.04.013
Kim, K. M., Park, Y. H., Kim, C. K., Hirschi, K., and Sohn, J. K. (2005). Development of transgenic rice plants overexpressing the Arabidopsis H+/Ca 2+ antiporter CAX 1 gene. Plant Cell Rep. 23, 678–682. doi: 10.1007/s00299-004-0861-4
Kokane, S. B., Pathak, R. K., Singh, M., and Kumar, A. (2018). The role of tripartite interaction of calcium sensors and transporters in the accumulation of calcium in finger millet grain. Biol. Plant. 62, 325–334. doi: 10.1007/s10535-018-0776-5
Krishna, T. A., Maharajan, T., David, R. H. A., Ramakrishnan, M., Ceasar, S. A., Duraipandiyan, V., et al. (2018). Microsatellite markers of finger millet (Eleusine coracana (L.) Gaertn) and foxtail millet (Setaria italica (L.) Beauv) provide resources for cross-genome transferability and genetic diversity analyses in other millets. Biocatal. Agric. Biotechnol. 16, 493–501. doi: 10.1016/j.bcab.2018.09.009
Krishna, T. A., Maharajan, T., Roch, G. V., Ramakrishnan, M., Ceasar, S. A., and Ignacimuthu, S. (2020). Hybridization and hybrid detection through molecular markers in finger millet [Eleusine coracana (L.) Gaertn.]. J. Crop Improv. 34, 335–355. doi: 10.1080/15427528.2019.1709596
Kumar, A., Gaur, V. S., Goel, A., and Gupta, A. K. (2015a). De novo assembly and characterization of developing spikes transcriptome of finger millet (Eleusine coracana): a minor crop having nutraceutical properties. Plant Mol. Biol. Rep. 33, 905–922. doi: 10.1007/s11105-014-0802-5
Kumar, A., Metwal, M., Kaur, S., Gupta, A. K., Puranik, S., Singh, S., et al. (2016). Nutraceutical value of finger millet [Eleusine coracana (L.) Gaertn.], and their improvement using omics approaches. Front. Plant Sci. 7:934. doi: 10.3389/fpls.2016.00934
Kumar, A., Mirza, N., Charan, T., Sharma, N., and Gaur, V. S. (2014). Isolation, characterization and immunolocalization of a seed dominant CaM from finger millet (Eleusine coracana L. Gaertn.) for studying its functional role in differential accumulation of calcium in developing grains. Appl. Biochem. Biotechnol. 172, 2955–2973. doi: 10.1007/s12010-013-0714-0
Kumar, A., Singh, U. M., Manohar, M., and Gaur, V. S. (2015c). Calcium transport from source to sink: understanding the mechanism (s) of acquisition, translocation, and accumulation for crop biofortification. Acta Physiol. Plant 37:1722. doi: 10.1007/s11738-014-1722-6
Kumar, A., Yadav, S., Panwar, P., Gaur, V., and Sood, S. (2015b). Identification of anchored simple sequence repeat markers associated with calcium content in finger millet (Eleusine coracana). Proc. Natl. Acad. Sci. India Sect. B. Biol. Sci. 85, 311–317. doi: 10.1007/s40011-013-0296-1
Kumari, P. L., and Sumathi, S. (2002). Effect of consumption of finger millet on hyperglycemia in non-insulin dependent diabetes mellitus (NIDDM) subjects. Plant. Foods. Hum. Nutr. 57, 205–213. doi: 10.1023/A:1021805028738
Kumari, V., Gowda, M., and Yeri, S. (2020). Utilization of advanced backcross population derived from synthetic amphidiploid for dissecting resistance to late leaf spot in peanut (Arachis hypogaea L.). Trop. Plant Biol. 13, 50–61. doi: 10.1007/s12042-019-09246-y
Kumssa, D. B., Joy, E. J., Ander, E. L., Watts, M. J., Young, S. D., Walker, S., et al. (2015). Dietary calcium and zinc deficiency risks are decreasing but remain prevalent. Sci. Rep. 5, 1–11. doi: 10.1038/srep10974
Latha, A. M., Rao, K. V., and Reddy, V. D. (2005). Production of transgenic plants resistant to leaf blast disease in finger millet (Eleusine coracana (L.) Gaertn.). Plant Sci. 169, 657–667. doi: 10.1016/j.plantsci.2005.05.009
Lee, S. H., Chung, I. M., Cha, Y. S., and Park, Y. (2010). Millet consumption decreased serum concentration of triglyceride and C-reactive protein but not oxidative status in hyperlipidemic rats. Nutr. Res. 30, 290–296. doi: 10.1016/j.nutres.2010.04.007
Lin, J., Manson, J. E., Lee, I. M., Cook, N. R., Buring, J. E., and Zhang, S. M. (2007). Intakes of calcium and vitamin D and breast cancer risk in women. Arch. Intern. Med. 167, 1050–1059. doi: 10.1001/archinte.167.10.1050
Maharajan, T., Ceasar, S. A., Ajeesh krishna, T. P., Ramakrishnan, M., Duraipandiyan, V., Naif Abdulla, A. D., et al. (2018). Utilization of molecular markers for improving the phosphorus efficiency in crop plants. Plant Breed. 137, 10–26. doi: 10.1111/pbr.12537
Maharajan, T., Ceasar, S. A., Krishna, T. P. A., and Ignacimuthu, S. (2019). Phosphate supply influenced the growth, yield, and expression of PHT1 family phosphate transporters in seven millets. Planta 250, 1433–1448. doi: 10.1007/s00425-019-03237-9
McKevith, B. (2004). Nutritional aspects of cereals. Nutr. Bull. 29, 111–142. doi: 10.1111/j.1467-3010.2004.00418.x
Michaelsson, K., Melhus, H., Ferm, H., Ahlbom, A., and Pedersen, N. L. (2005). Genetic liability to fractures in the elderly. Arch. Intern. Med. 165, 1825–1830. doi: 10.1001/archinte.165.16.1825
Mirza, N., Taj, G., Arora, S., and Kumar, A. (2014). Transcriptional expression analysis of genes involved in regulation of calcium translocation and storage in finger millet (Eleusine coracana L. Gartn.). Gene 550, 171–179. doi: 10.1016/j.gene.2014.08.005
Nath, M., Roy, P., Shukla, A., and Kumar, A. (2013). Spatial distribution and accumulation of calcium in different tissues, developing spikes and seeds of finger millet genotypes. J. Plant Nutr. 36, 539–550. doi: 10.1080/01904167.2012.748072
Pandian, S., and Ramesh, M. (2019). Decoding of finger millet genome: a milestone of millet genomics. Signal Transduct. Insights 8, 1–3. doi: 10.1177/1178643418820541
Parameswaran, K. P., and Sadasivam, S. (1994). Changes in the carbohydrates and nitrogenous components during germination of proso millet, Panicum miliaceum. Plant Foods Hum. Nutr. 45, 97–102. doi: 10.1007/BF01088466
Pettifor, J. M. (2008). Vitamin D and/or calcium deficiency rickets in infants and children: a global perspective. Indian J. Med. Res. 127, 245–249.
Pravina, P., Sayaji, D., and Avinash, M. (2013). Calcium and its role in human body. Int. J. Res. Pharm. Biomed. Sci. 4, 659–668.
Puranik, S., Kam, J., Sahu, P. P., Yadav, R., Srivastava, R. K., Ojulong, H., et al. (2017). Harnessing finger millet to combat calcium deficiency in humans: challenges and prospects. Front. Plant Sci. 8:1311. doi: 10.3389/fpls.2017.01311
Radhajeyalakshmi, R., Yamunarani, K., Seetharaman, K., and Velazhahan, R. (2003). Existence of thaumatin-like proteins (TLPs) in seeds of cereals. Acta Phytopathol. Hung. 38, 251–257. doi: 10.1556/APhyt.38.2003.3-4.5
Rajasekaran, N., Nithya, M., Rose, C., and Chandra, T. (2004). The effect of finger millet feeding on the early responses during the process of wound healing in diabetic rats. Biochim. Biophys. Acta BBA Mol. Basis Dis. 1689, 190–201. doi: 10.1016/j.bbadis.2004.03.004
Ravindran, G. (1991). Studies on millets: proximate composition, mineral composition, and phytate and oxalate contents. Food Chem. 39, 99–107. doi: 10.1016/0308-8146(91)90088-6
Saleh, A. S., Zhang, Q., Chen, J., and Shen, Q. (2013). Millet grains: nutritional quality, processing, and potential health benefits. Compr. Rev. Food Sci. Food Saf. 12, 281–295. doi: 10.1111/1541-4337.12012
Sharma, D., Jamra, G., Singh, U. M., Sood, S., and Kumar, A. (2017). Calcium biofortification: three pronged molecular approaches for dissecting complex trait of calcium nutrition in finger millet (Eleusine coracana) for devising strategies of enrichment of food crops. Front. Plant Sci. 7:2028. doi: 10.3389/fpls.2016.02028
Sharma, D., Tiwari, A., Sood, S., Jamra, G., Singh, N., Meher, P. K., et al. (2018). Genome wide association mapping of agro-morphological traits among a diverse collection of finger millet (Eleusine coracana L.) genotypes using SNP markers. PLoS ONE 13:e0199444. doi: 10.1371/journal.pone.0199444
Shobana, S., Krishnaswamy, K., Sudha, V., Malleshi, N. G., Anjana, R. M., Palaniappan, L., et al. (2013). Finger millet (Ragi, Eleusine coracana L.): a review of its nutritional properties, processing, and plausible health benefits. Adv. Food Nutr. Res. 69, 1–39. doi: 10.1016/B978-0-12-410540-9.00001-6
Singh, R. B., Khan, S., Chauhan, A. K., Singh, M., Jaglan, P., Yadav, P., et al. (2019). “Millets as functional food, a gift from Asia to Western World,” in The Role of Functional Food Security in Global Health, eds R. B. Singh, R. R. Watson, and T. Takahashi (Amsterdam: Academic Press; Elsevier), 457–468. doi: 10.1016/C2016-0-04169-4
Singh, U. M., Metwal, M., Singh, M., Taj, G., and Kumar, A. (2015). Identification and characterization of calcium transporter gene family in finger millet in relation to grain calcium content. Gene 566, 37–46. doi: 10.1016/j.gene.2015.04.021
Sozen, T., Ozisik, L., and Basaran, N. C. (2017). An overview and management of osteoporosis. Eur. J. Rheumatol. 4:46. doi: 10.5152/eurjrheum.2016.048
Sripriya, G., Antony, U., and Chandra, T. (1997). Changes in carbohydrate, free amino acids, organic acids, phytate and HCl extractability of minerals during germination and fermentation of finger millet (Eleusine coracana). Food Chem. 58, 345–350. doi: 10.1016/S0308-8146(96)00206-3
Swati, P., Sahu, P. P., Beynon, S., Srivastava, R. K., Sehgal, D., Ojulong, H., et al. (2020). Genome-wide association mapping and comparative genomics identifies genomic regions governing grain nutritional traits in finger millet (Eleusine coracana L. Gaertn.). Plants People Planet 2, 649–662. doi: 10.1002/ppp3.10120
Sze, H., Liang, F., Hwang, I., Curran, A. C., and Harper, J. F. (2000). Diversity and regulation of plant Ca2+ pumps: insights from expression in yeast. Annu. Rev. Plant Biol. 51, 433–462. doi: 10.1146/annurev.arplant.51.1.433
Tadele, Z. (2019). Orphan crops: their importance and the urgency of improvement. Planta 250, 677–694. doi: 10.1007/s00425-019-03210-6
Thorning, T. K., Raben, A., Tholstrup, T., Soedamah-Muthu, S. S., Givens, I., and Astrup, A. (2016). Milk and dairy products: good or bad for human health? an assessment of the totality of scientific evidence. Food Nutr. Res. 60:32527. doi: 10.3402/fnr.v60.32527
Tiwari, A., Sharma, D., Sood, S., Jaiswal, J. P., Pachauri, S. P., Ramteke, P. W., et al. (2020). Genome-wide association mapping for seed protein content in finger millet (Eleusine coracana) global collection through genotyping by sequencing. J. Cereal Sci. 91:102888. doi: 10.1016/j.jcs.2019.102888
Upadhyaya, H., Gowda, C., and Reddy, V. G. (2007). Morphological diversity in finger millet germplasm introduced from Southern and Eastern Africa. J. SAT Agri. Res. 3, 1–3.
Vetriventhan, M., Azevedo, V. C., Upadhyaya, H., Nirmalakumari, A., Kane-Potaka, J., Anitha, S., et al. (2020). Genetic and genomic resources, and breeding for accelerating improvement of small millets: current status and future interventions. Nucleus 63, 217–239. doi: 10.1007/s13237-020-00322-3
Wambi, W., Otienno, G., Tumwesigye, W., and Mulumba, J. (2020). Genetic and genomic resources for finger millet improvement: opportunities for advancing climate-smart agriculture. J. Crop Improv. 35, 204–233. doi: 10.1080/15427528.2020.1808133
Wang, B., and Chee, P. W. (2010). Application of advanced backcross QTL analysis in crop improvement. J. Plant Breed. Crop Sci. 2, 221–232.
Wang, J., Vanga, S., Saxena, R., Orsat, V., and Raghavan, V. (2018). Effect of climate change on the yield of cereal crops: a review. Climate 6:41. doi: 10.3390/cli6020041
White, P. J., and Broadley, M. R. (2003). Calcium in plants. Ann. Bot. 92, 487–511. doi: 10.1093/aob/mcg164
Wolswinkel, P., Ammerlaan, A., and Koerselman Kooij, J. (1992). Effect of the osmotic environment on K+ and Mg2+ release from the seed coat and cotyledons of developing seeds of Vicia faba and Pisum sativum. Evidence for a stimulation of efflux from the vacuole at high cell turgor. J. Exp. Bot. 43, 681–693. doi: 10.1093/jxb/43.5.681
Keywords: calcium deficiency, calcium transporters, finger millet, genome-wide association studies, health benefits, quantitative trait loci
Citation: Maharajan T, Antony Ceasar S, Ajeesh Krishna TP and Ignacimuthu S (2021) Finger Millet [Eleusine coracana (L.) Gaertn]: An Orphan Crop With a Potential to Alleviate the Calcium Deficiency in the Semi-arid Tropics of Asia and Africa. Front. Sustain. Food Syst. 5:684447. doi: 10.3389/fsufs.2021.684447
Received: 23 March 2021; Accepted: 29 June 2021;
Published: 26 July 2021.
Edited by:
E. O. Deedi Sogbohossou, Wageningen University and Research, NetherlandsReviewed by:
Umakanta Sarker, Bangabandhu Sheikh Mujibur Rahman Agricultural University, BangladeshSagar Maitra, Sagar Maitra, India
Vikram Singh Gaur, Jawaharlal Nehru Agricultural University, India
Salej Sood, Indian Council of Agricultural Research (ICAR), India
Copyright © 2021 Maharajan, Antony Ceasar, Ajeesh Krishna and Ignacimuthu. This is an open-access article distributed under the terms of the Creative Commons Attribution License (CC BY). The use, distribution or reproduction in other forums is permitted, provided the original author(s) and the copyright owner(s) are credited and that the original publication in this journal is cited, in accordance with accepted academic practice. No use, distribution or reproduction is permitted which does not comply with these terms.
*Correspondence: Stanislaus Antony Ceasar, antony_sm2003@yahoo.co.in