- 1Graduate School of Horticulture, Chiba University, Matsudo, Chiba, Japan
- 2Research Group of Postharvest Technology, Mae Fah Luang University, Chiang Rai, Thailand
- 3School of Agro-Industry, Mae Fah Luang University, Chiang Rai, Thailand
Green mold caused by Penicillium digitatum is an important factor limiting the shelf life of mandarin fruit. In this study, the effect of ultraviolet-C (UV-C) irradiation on cellular structure, endogenous jasmonic acid (JA), and development of P. digitatum in satsuma mandarin fruit was investigated. UV-C treatments included 0 (untreated control), 3, and 10 kJ m−2 or the exposure time of 0, 1.18, and 4.52 min, respectively. The UV-C dose of 10 kJ m−2 significantly reduced the development of P. digitatum both in vitro and in vivo, resulting in the maintenance of the cellular structure of the albedo tissue. The production of malondialdehyde (MDA) was decreased upon UV-C treatment of 10 kJ m−2. The concentration of JA increased in the treatment of 10 kJ m−2 compared to the treatment of 3 kJ m−2 and the control. UV-C irradiation increased total phenolic and total flavonoid concentrations and DPPH radical scavenging capacity. These results suggest that UV-C at 10 kJ m−2 has a potential to control green mold caused by P. digitatum, maintain cellular structure, stimulate the accumulation of JA, and induce biochemical compounds in satsuma mandarin.
Introduction
Citrus fruit is one of the largest commodities consumed worldwide (Lu et al., 2020). Satsuma mandarin (Citrus unshiu) is one of the most popular fruit for fresh consumption at present because it is seedless, is rich in bioactive compounds, and contributes to nutrition and energy sources for health (Gao et al., 2018). However, this citrus fruit is highly susceptible to postharvest decay caused by various pathogens such as Botrytis cinerea, Penicillium italicum, and Penicillium digitatum. In general, green mold decay caused by the pathogenic fungus P. digitatum is the major cause of postharvest losses in mandarin fruit. The fungus causes a soft water-soaked peel that is later covered with white mycelia of the fungus (Chen and Peng, 2016).
Generally, fungicides have been used to suppress decay in citrus before harvest (Yamaga et al., 2016). However, the frequent use of some fungicides has led to adverse effects on human health and the environment, as well as the development of fungicide-resistant pathogens. For example, P. digitatum was resistant to thiabendazole due to a mutation of β-tubulin gene correlating to amino acid 200 (Sánchez-Torres and Tuset, 2011). Recently, several studies have used ultraviolet-C (UV-C) irradiation to control microbial infections in fruits and vegetables (Mohamed et al., 2017; Sripong et al., 2019). For example, irradiation with UV-C reduced gray mold development caused by B. cinerea in strawberry fruit (Fragaria ananassa) (Jin et al., 2017), and regulated gene expression related to lipid oxidation and cell wall degradation in tomato fruit (Lycopersicon esculentum) (Liu et al., 2011). Moreover, in fresh produce exposed to UV-C irradiation, antioxidant capacity such as total phenolic and total flavonoid concentrations were stimulated (Park and Kim, 2015).
Jasmonic acid (JA), an endogenous plant hormone, is usually produced in plants in response to various stressful situations (Yang et al., 2019a). Studies have reported that JA influence the direct and indirect response to trigger signaling molecules that then activate the plant stress tolerance (Zhang et al., 2009; Margherita et al., 2018). This hormone plays a role in increasing the production of secondary metabolites and defense strategies against pathogens in plants (Shikano et al., 2018).
Therefore, the objective of this study was to investigate the effect of UV-C irradiation on cellular structure, concentrations of JA and bioactive compounds, antioxidant capacity, and green mold development in satsuma mandarin during storage.
Materials and Methods
Suppression of Fungal Development Caused by Penicillium digitatum: In vitro Study
A-7-day-old pure culture of P. digitatum (Registration No. MAFF 242809) was used in this study. The mycelial disk, 6 mm in diameter, was placed on the center of potato dextrose agar (PDA) in a Petri dish. The dishes were uncovered just before being placed in the UV-C chamber and placed 25 cm away from the UV-C sources with the critical peak emission at 253 nm (GL-20, Toshiba, Japan). Three groups of 10 plates were exposed to 3 UV-C doses of 0 (control), 3, and 10 kJ m−2 or the exposure time of 0, 1.18, and 4.52 min, respectively. After UV-C treatment, the plates were covered and then incubated at 25°C. The effect of UV-C treatments on the development of P. digitatum was examined at 3, 4, and 5 days after treatment (DAT), which was calculated from the longest length and the greatest width perpendicular to the length of the mycelium using a transparent ruler.
Inhibition of Penicillium digitatum in Satsuma Mandarin Fruit
“Aoshima unshu” satsuma mandarin (C. unshiu) fruit at commercial maturity stage were harvested in late December from the orchard in Shizuoka Prefecture, Japan. Prior to treatment, fruits were disinfected by immersion in 150 ppm sodium hypochlorite (NaOCl) for 1 min, rinsed thoroughly in tap water, and drained overnight at 25°C. Satsuma mandarin fruits were divided into three groups (90 fruits per treatment). Sufficient spores from a pure culture of P. digitatum were harvested using 25 ml of sterilized distilled water containing 0.5% agar and then adjusted to a final spore concentration of 1.2 × 106 spores ml−1.
For inoculation, a wound of 5-mm diameter and 3-mm depth was made between the vertical and horizontal parts at the equator of the fruit using a sterilized scalpel (Suktawee et al., 2019). The 20 ml of spore suspension was inoculated into the center of the wound of each fruit using a micropipette and allowed to air-drain for pathogen settlement. Two groups of artificially infected fruits were irradiated with UV-C at different irradiation doses of 3 and 10 kJ m−2, and the non-irradiated group was set as untreated control. All fruits were stored at 25°C and 95% relative humidity. Lesion diameters of green mold infestation (15 fruits per treatment) were measured and calculated by taking the mean of the vertical and horizontal of each lesion with a digital vernier caliper at 3, 4, and 5 DAT.
Effect of UV-C Irradiation on the Mycelial Density of Albedo
Fruit peel before and after inoculation (5DAT) were cut into 0.5 cm3 and immediately coated with liquid nitrogen. The sample was observed under a scanning electron microscope (SEM) (SU1510; Hitachi, Japan). The images were then illustrated at the accelerating power of 30.0 kV with the magnification of 100 μm.
Effect of UV-C Irradiation on Lipid Peroxidation
Lipid peroxidation was determined as production of malondialdehyde (MDA) equivalents using a modified method of Phonyiam et al. (2016). The extracted sample (500 μl) was mixed with 0.5% of thiobarbituric acid (TBA) in 10% of trichloroacetic acid (TCA) (1.5 ml). The mixed solution was incubated at 85°C for 30 min. Then, the cooled mixture was measured with a spectrophotometer (U-2910; Hitachi, Japan) at the absorbance of 532 nm. A non-specific turbidity was measured at the absorbance of 600 nm.
Effect of UV-C Irradiation on JA Concentration
The JA concentration was analyzed according to the method of Kondo et al. (2005) with modifications. Fresh peel sample (1 g) was homogenized in 1 M citric acid (250 μl), sodium chloride (NaCl) (5 ml), and diethyl ether containing 0.005% BHT (10 ml). The sample mixture was then mixed with ibuprofen (500 μl) and used as an internal standard. The diethyl ether fraction was collected after centrifugation at 15,000 g for 10 min at 4°C. Then, the qualification of JA was evaluated by RP-18 octadecylsilyl column (5 μm, 2 mm I.D. ×150 mm; Kanto Chemical, Tokyo, Japan). The JA concentration was calculated from the ratio of the peak area for m/z 206/161 (ibuprofen) and expressed as nanomole per 1 kg of fruit weight (nmol kg−1 FW).
Effect of UV-C Irradiation on Bioactive Compounds and Antioxidant Capacity
Peel sample (3 g of fresh weight) was homogenized in 20 ml of 80% methanol. The homogenized samples were then centrifuged at 15,000 g for 10 min at 4°C. Total phenolic concentrations in peel were analyzed using a spectrophotometer (U-2910; Hitachi, Japan) according to the Folin–Ciocalteu assay of Sheng et al. (2018) with slight modifications. The 0.25 ml of the crude extract was mixed with 1.25 ml of 10% Folin–Ciocalteu reagent and 1 ml of 7.5% sodium carbonate (Na2CO3). The mixture was incubated for 1 h at ambient condition, and the total phenolic concentration was measured at the absorbance of 765 nm and expressed as milligram of gallic acid equivalent (GAE) per 1 kg of fruit weight (mg GAE kg−1 FW). Total flavonoid concentration was measured by the method modified from Sheng et al. (2018). The sample (250 μl) was mixed with 75 μl of 10% aluminum chloride (AlCl3), 75 μl of 5% sodium nitrite (NaNO2), and 1 ml of deionized water. After 5 min, 1 ml of 4% sodium hydroxide (NaOH) was added to the mixture and incubated for 10 min at ambient temperature. The reaction mixture was evaluated at the absorbance of 510 nm. Total flavonoid concentration in the peel sample was expressed as mg catechin equivalent (CAE) per 1 kg of fruit weight (mg CAE kg−1 FW). The DPPH radical scavenging capacity was determined according to the method described by Phonyiam et al. (2016) with some modifications. The sample extract (500 μl: 9 replicates per treatment) was mixed with 0.1 mM of DPPH-methanolic solution (1.5 ml) and the mixture was incubated. After incubation at 25°C for 30 min, DPPH radical scavenging capacity was monitored at the absorbance of 517 nm and expressed as micromole Trolox equivalent (TE) 1 kg of fruit weight (μmol TE kg−1 FW).
Statistical Analysis
Triplicate determinations were analyzed for the entire duration of the experimental period using the least significant difference (LSD) test at the significance level of P ≤ 0.05 (SPSS 16; IBM, Armonk, NY). Data were expressed as the mean ± standard error (SE).
Results
Green Mold Development
Mycelial growth of P. digitatum was significantly lower in the UV-C treatments than that in the control (Figure 1A). At 5 DAT, mycelium diameters were 3.80 ± 0.04, 4.08 ± 0.03, and 4.40 ± 0.08 cm in the UV-C treatments of 10, 3, and 0 kJ m−2 (control), respectively. This indicates that UV-C irradiation suppresses in vitro growth of P. digitatum. Similarly, mandarin fruit treated with 10 kJ m−2 showed the smallest lesion diameter (1.82 ± 0.06 cm) compared to those treated with 3 kJ m−2 (2.97 ± 0.06 cm) and the controls (5.13 ± 0.00 cm) (Figure 1B). The effect of UV-C irradiation on green mold development on mandarin is shown in Figure 1C. Furthermore, UV-C at a dose of 10 kJ m−2 significantly promoted the suppression of mycelial density while maintaining the albedo tissue of cellular structure on 5 DAT compared to other treatments (Figure 2).
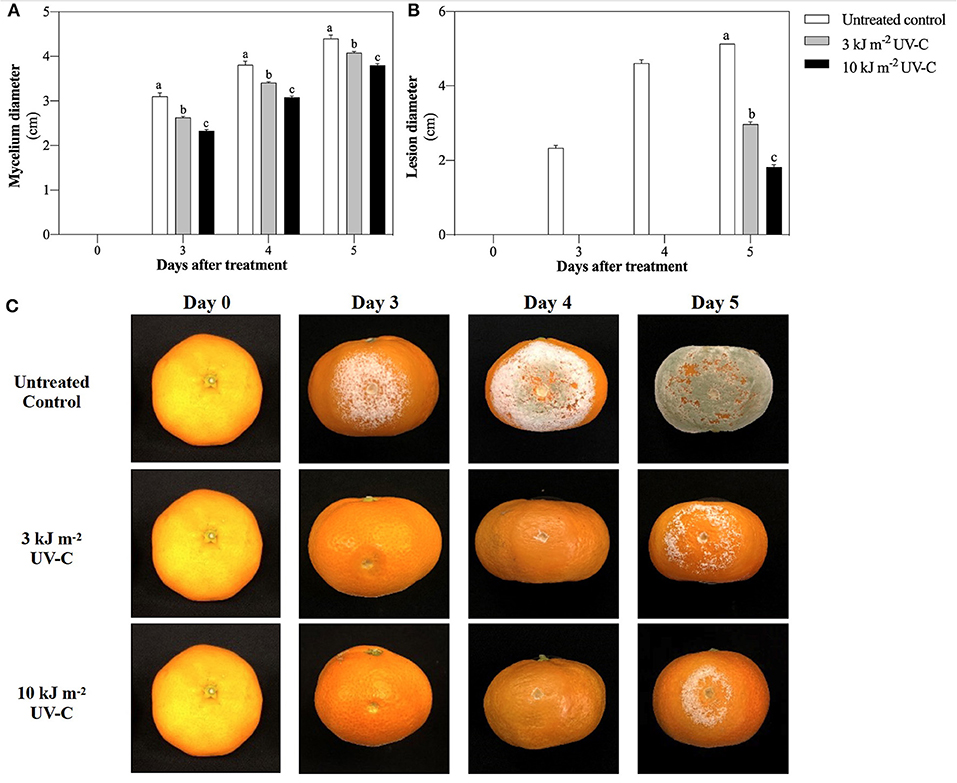
Figure 1. Effect of UV-C treatment on suppression of P. digitatum growth in vitro study (A), on inhibition of green mold decay development in satsuma mandarin fruit (B), and visual appearance of inoculcated satsuma mandarin fruit after exposure to UV-C in untreated control, 3 kJ m−2, and 10 kJ m−2 UV-C groups (C). The different letters indicate significant differences at P ≤ 0.05 by LSD test at each day with the mean ± SE of three replications.
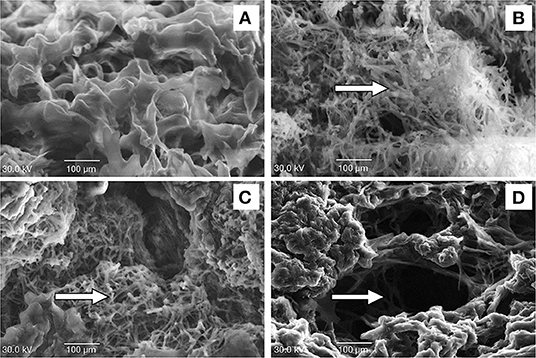
Figure 2. Effect of UV-C treatment on cellular structure of albedo tissue in satsuma mandarin fruit; before treatment (A), untreated control (B), 3 kJ m−2 (C), and 10 kJ m−2 UV-C (D) groups. The arrows indicate mycelium and spores of P. digitatum.
Lipid Peroxidation
MDA concentrations in all treatments increased slightly from 3 DAT to 4 DAT and then decreased at 5 DAT (Figure 3). At 4 DAT, all UV-C doses inhibited MDA concentrations, as indicated by the significantly lower MDA concentrations in the UV-C treatments (22.90 ± 1.02 and 27.47 ± 0.50 mmol kg−1 FW for 10 and 3 kJ m−2, respectively) compared with the untreated control (39.87 ± 1.58 mmol kg−1 FW). This result suggests that the strongest suppression of MDA was achieved at the UV-C dose of 10 kJ m−2.
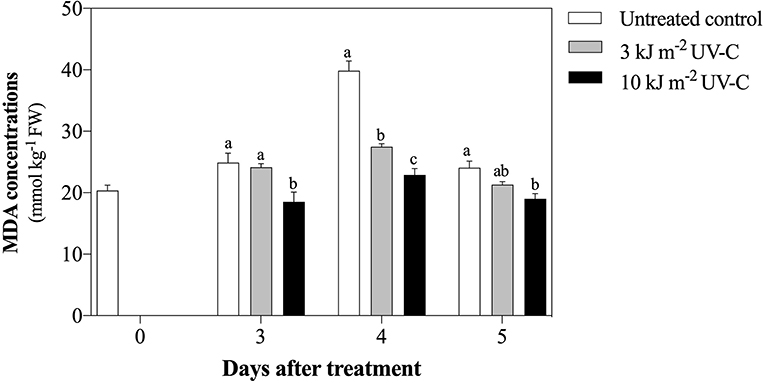
Figure 3. Effect of UV-C treatment on change of malondialdehyde concentration in satsuma mandarin fruit in untreated control, 3 kJ m−2, and 10 kJ m−2 UV-C groups. The different letters indicate significant differences at P ≤ 0.05 by LSD test at each day with the mean ± SE of three replications.
JA Concentration
UV-C treatments significantly enhanced JA accumulation in mandarin fruit (Figure 4). In 4 DAT, JA concentration was increased in the UV-C treatment with 10 kJ m−2 (338.33 ± 6.49 nmol kg−1 FW), while in the treatment with 3 kJ m−2, it was 198.37 ± 14.76 nmol kg−1 FW. Among the treatments, the lowest JA concentration was observed in the untreated control.
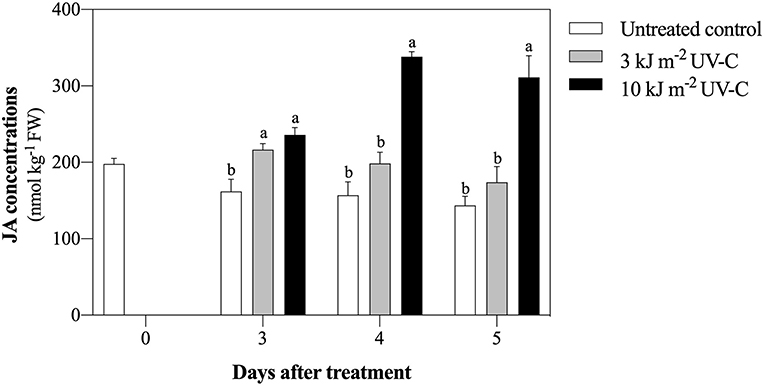
Figure 4. Effect of UV-C treatment on jasmonic acid concentration in satsuma mandarin fruit in untreated control, 3 kJ m−2, and 10 kJ m−2 UV-C groups. The different letters indicate significant differences at P ≤ 0.05 by LSD test at each day with the mean ± SE of three replications.
Bioactive Compounds and Antioxidant Capacity
The total phenolic concentration of fruits irradiated with UV-C increased significantly. The total phenolic concentration in inoculated fruit after treatment with UV-C at 3 and 10 kJ m−2 was 2,539.29 ± 37.11 and 2,502.70 ± 32.20 mg GAE kg−1 FW, respectively, while the total phenolic concentration in the untreated control was 2,294.42 ± 20.40 mg GAE kg−1 FW (Figure 5A).
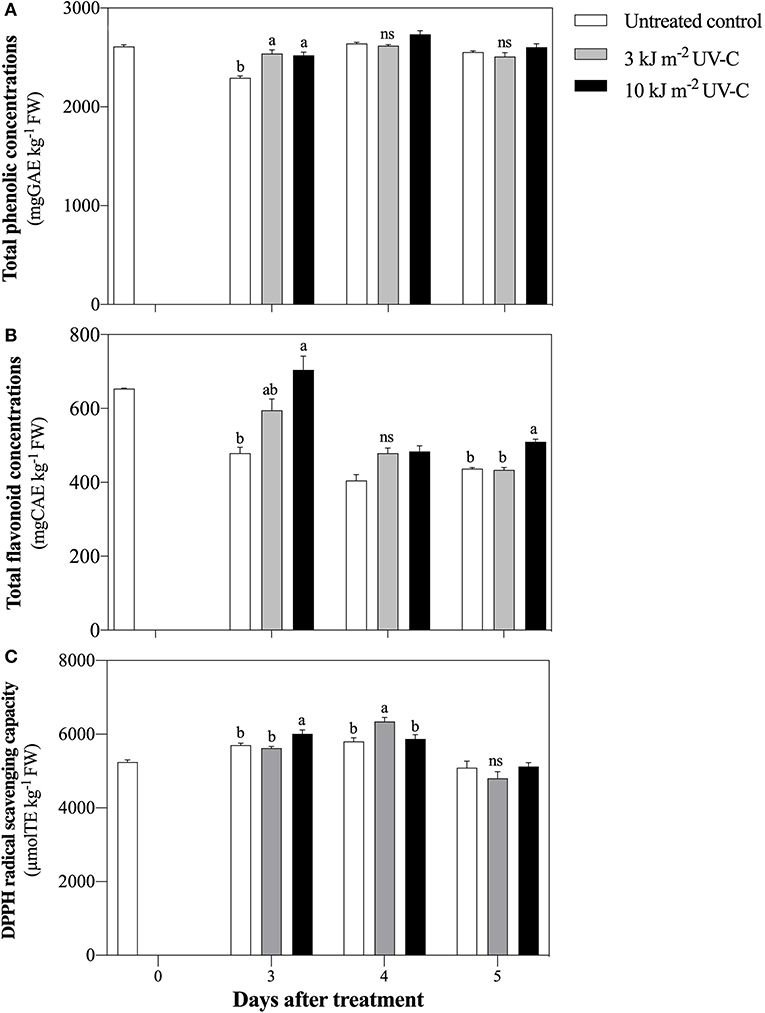
Figure 5. Effect of UV-C treatment on change of total phenolic concentrations (A), total flavonoids concentrations (B), and DPPH radical scavenging capacity (C) in satsuma mandarin fruit in untreated control, 3 kJ m−2 UV-C, and 10 kJ m−2 UV-C groups. The different letters indicate significant differences at P ≤ 0.05 by LSD test at each day with the mean ± SE of three replications.
Total flavonoid concentration increased in all treatments at 3 DAT and then decreased until the end of the storage period (Figure 5B). At 5 DAT, the total flavonoid concentration was significantly higher in the UV-C treatment with 10 kJ m−2 (509.99 ± 7.16 mg CAE kg−1 FW) compared with the treatment with 3 kJ m−2 (433.65 ± 6.90 mg CAE kg−1 FW) and the untreated control (437.00 ± 3.14 mg CAE kg−1 FW).
At 3 DAT, DPPH scavenging capacity increased significantly in fruit treated with 10 kJ m−2 UV-C treatments compared with the other treatments (Figure 5C). Moreover, the highest DPPH scavenging capacity was obtained in the 3 kJ m−2 UV-C treatment at 4 DAT (6,343.97 ± 113.86 μmol TE kg−1 FW), while the DPPH values in the 10 kJ m−2 treatment and the untreated control were 5,870.30 ± 116.49 μmol TE kg−1 FW and 5,804.98 ± 95.68 μmol TE kg−1 FW, respectively.
Discussion
UV-C radiation has been demonstrated to inhibit Escherichia coli in apple (Malus domestica) (Graça et al., 2013), Guignardia citricarpa in orange (Citrus sinensis) (Canale et al., 2011), and Colletotrichum musae in banana (Musa acuminate) (Bokhari et al., 2013). In addition, Oliveira et al. (2016) and Jin et al. (2017) reported that UV-C induces disease resistance response and promotes pathogen-related genes such as cinnamoyl CoA reductase 1 allele (CCR-1 allele), chitinase 2 (CHI2), and phenylalanine ammonia lyase 6 (PAL6) in strawberry fruit. In this study, the growth of P. digitatum, the causal agent of green mold disease, was significantly lower than that of the untreated control both in vitro and in vivo upon UV-C treatment of 10 kJ m−2. This suggests that UV-C treatment may have a direct effect on the fungus and an indirect effect by inducing defense mechanism against the pathogen infection in satsuma mandarin fruit.
The cell wall is the primary mode of entry of pathogen into plant tissue. The alteration of cell structure barrier could influence the colonization of pathogen (Cantu et al., 2008). Our study indicated that treatment with UV-C at 10 kJ m−2 greatly reduced fungal mycelia and maintained the cellular structure of the albedo tissue in infected satsuma mandarin fruit. This result is in agreement with a previous study that showed that high dose of UV-C may have induced the plasmolysis process of epidermal cells, resulting in the strengthening of cell wall structure (Charles et al., 2008). However, UV-C at 3 kJ m−2 and the untreated control at 5 DAT showed intense mycelia of P. digitatum. Therefore, the result suggests that 10 kJ m−2 UV-C induces disease resistance by improving the structural barrier of the cell wall, resulting in inhibition of green mold infestation. On the other hand, MDA is the by-product of the lipid peroxidation process that occurs when reactive oxygen species (ROS) are exceeded in plant cells. This primary injury has been associated with withdrawal of electrons from membrane lipids, leading to membrane structure breakdown and high production of MDA (He et al., 2019). Pongprasert et al. (2011) confirmed that UV-C decreases MDA production in banana peel. In satsuma mandarin, 10 kJ m−2 UV-C treatment reduced MDA concentrations after inoculation with P. digitatum. The significant decrease in lipid peroxidation formation was probably due to UV-C irradiation.
Pathogenic infection can be reduced by JA application in some crops such as sugar beet root (Beta vulgaris), grape (Vitis vinifera), and naranjilla (Solanum quitoense) (Fugate et al., 2012; Wang et al., 2015; Avila et al., 2019). Interestingly, Xu et al. (2016) demonstrated that UV-C application promoted the activation of JA signaling molecules and biosynthetic pathway in Arabidopsis thaliana. In satsuma mandarin, an increase in endogenous JA concentrations was observed upon UV-C treatment of 10 kJ m−2. This suggests that the reduction of green mold infestation achieved in the UV-C treatments may be due to the accumulation of JA.
The total phenolic compounds act as the main precursor to stimulate antioxidant properties and increase disease resistance of various plants (Yoruk and Marshall, 2003). UV-C irradiation increases phenolic compounds by enhancing the activity of phenylalanine ammonia lyase (PAL), thereby participating in the phenolic biosynthetic pathway (Bhat et al., 2007). For example, Pataro et al. (2015) reported the increase in total phenolic concentrations in tomato fruit exposed to UV-C irradiation. However, in this study, UV-C treatments were found to increase the total phenolic concentration only on the first day of the storage period. This result is in agreement with Sripong et al. (2019) who found that UV-C-irradiated mangosteen (Garcinia mangostana) fruit increased the defense against Lasiodiplodia theobromae, which corresponded with the induction of total phenolic accumulation. Both 3 and 10 kJ m−2 UV-C irradiation also increased total flavonoid concentration compared to the untreated control. Shen et al. (2013) and Yang et al. (2019b) reported that UV-C treatment strongly promoted flavonoid concentration and therefore upregulated the expression levels of flavonol synthase (FLS), leucoanthocyanidin reductase (LAR), and anthocyanidin reductase (ANR) genes in the flavonoid pathway in satsuma mandarin and blueberry (Vaccinium corymbosum) fruit.
All UV-C doses induced total antioxidant activity as measured by DPPH radical scavenging capacity in satsuma mandarin. As reported in previous studies, UV-C irradiation increased DPPH radical scavenging capacity in grape and papaya (Carica papaya) as well as total phenolic and flavonoid concentrations (Maurer et al., 2017; Sheng et al., 2018). Nevertheless, a decrease in total antioxidant activity in all treatments during the final storage period may be influenced by the depletion of total phenolic and flavonoid concentrations. Our results showed that UV-C application activated total phenolic and total flavonoid concentrations and increased the ability to scavenge DPPH radicals; this promoted the plant defense mechanism against P. digitatum infection in satsuma mandarin fruit. From our study, promising UV-C irradiation with pathogen defense mechanism associated with bioactive compounds on fruit quality requires further studies.
Conclusion
UV-C irradiation at a dose of 10 kJ m−2 effectively maintained the integrity of the membrane structure by reducing lipid peroxidation and increasing JA accumulation with the induction of bioactive compounds and antioxidant potential of DPPH radical scavenging capacity. These results contributed to the inhibition of green mold infestation caused by P. digitatum in satsuma mandarin fruit. As shown in this study, UV-C irradiation has a very good potential to be considered as a safe alternative treatment for postharvest control of green mold diseases in citrus.
Data Availability Statement
The raw data supporting the conclusions of this article will be made available by the authors, without undue reservation.
Author Contributions
OP designed and conducted the experiment, analyzed the data, prepared the figures, and wrote the first draft of the manuscript. HO supported the material used in the experiment. MN critically reviewed and corrected the English. SK and SS conceived, supervised, and critically reviewed the manuscript. All authors contributed to the manuscript revision, read, and approved the submitted version.
Conflict of Interest
The authors declare that the research was conducted in the absence of any commercial or financial relationships that could be construed as a potential conflict of interest.
Acknowledgments
The authors are grateful to the Ministry of Education, Culture, Sports, Science, and Technology (MEXT) and Mae Fah Luang University for a grant.
References
Avila, A. C., Ochoa, J., Proano, K., and Martinez, M. C. (2019). Jasmonic acid and nitric oxide protects naranjilla (Solanum quitoense) against infection by Fusarium oxysporum f. sp. quitoense by eliciting plant defense responses. Physiol. Mol. Plant Pathol. 106, 129–136. doi: 10.1016/j.pmpp.2019.01.002
Bhat, R., Sridhar, K. R., and Tomita-Yokotani, K. (2007). Effect of ionizing radiation on antinutritional features of velvet bean seeds (Mucuna pruriens). Food Chem. 103, 860–866. doi: 10.1016/j.foodchem.2006.09.037
Bokhari, N. A., Siddiqui, I., Parveen, K., Siddique, I., Rizwana, H., and Soliman, D. A. W. (2013). Management of anthracnose of banana by UV irradiation. J. Anim. Plant Sci. 23, 1211–1214.
Canale, M., Benato, E., Cia, P., Haddad, M., and Pascholati, S. (2011). In vitro effect of UV-C irradiation on Guignardia citricarpa and on postharvest control of citrus black spot. Trop. Plant Pathol. 36, 356–361. doi: 10.1590/S1982-56762011000600003
Cantu, D., Vicente, A. R., Labavitch, J. M., Bennett, A. B., and Powell, A. L. T. (2008). Strangers in the matrix: plant cell walls and pathogen susceptibility. Trends Plant Sci. 13, 610–617. doi: 10.1016/j.tplants.2008.09.002
Charles, M. T., Goulet, A., and Arul, J. (2008). Physiological basis of UV-C induced resistance to Botrytis cinerea in tomato fruit: IV. Biochemical modification of structural barriers. Postharvest Biol. Technol. 47, 41–53. doi: 10.1016/j.postharvbio.2007.05.019
Chen, P. S., and Peng, Y. H. (2016). Inhibition of Penicillium digitatum and Citrus Green mold by volatile compounds produced by Enterobacter cloacae. J. Plant Pathol. Microbiol. 07:339. doi: 10.4172/2157-7471.1000339
Fugate, K. K., Ferrareze, J. P., Bolton, M. D., Deckard, E. L., and Campbell, L. G. (2012). Postharvest jasmonic acid treatment of sugarbeet roots reduces rot due to Botrytis cinerea, Penicillium claviforme, and Phoma betae. Postharvest Biol. Technol. 65, 1–4. doi: 10.1016/j.postharvbio.2011.10.005
Gao, J., Wu, B., Gao, L. X., Liu, H. R., Zhang, B., Sun, C. D., et al. (2018). Glycosidically bound volatiles as affected by ripening stages of Satsuma mandarin fruit. Food Chem. 240, 1097–1105. doi: 10.1016/j.foodchem.2017.07.085
Graça, A., Salazar, M., Quintas, C., and Nunes, C. (2013). Low dose UV-C illumination as an eco-innovative disinfection system on minimally processed apples. Postharvest Biol. Technol. 85, 1–7. doi: 10.1016/j.postharvbio.2013.04.013
He, Y., Li, Z., Tan, F., Liu, H., Zhu, M., Yang, H., et al. (2019). Fatty acid metabolic flux and lipid peroxidation homeostasis maintain the biomembrane stability. Food Chem. 292, 314–324. doi: 10.1016/j.foodchem.2019.04.009
Jin, P., Wang, H., Zhang, Y., Huang, Y., Wang, L., and Zheng, Y. (2017). UV-C enhances resistance against gray mold decay caused by Botrytis cinerea in strawberry fruit. Sci. Hort. 225, 106–111. doi: 10.1016/j.scienta.2017.06.062
Kondo, S., Monrudee, K., and Sirichai, K. (2005). Preharvest antioxidant activities of tropical fruit and the effect of low temperature storage on antioxidants and jasmonates. Postharvest Biol. Technol. 36, 309–318. doi: 10.1016/j.postharvbio.2005.02.003
Liu, C., Cai, L., Han, X., and Ying, T. (2011). Temporary effect of postharvest UV-C irradiation on gene expression profile in tomato fruit. Gene. 486, 56–64. doi: 10.1016/j.gene.2011.07.001
Lu, Y., Li, D., Li, L., Belwal, T., Xu, Y., Lin, X., et al. (2020). Effects of elevated CO2 on pigment metabolism of postharvest mandarin fruit for degreening. Food Chem. 318:126462. doi: 10.1016/j.foodchem.2020.126462
Margherita, M., Milena, P., Roberto, F., Luigi, Z., Marco, S., and Fabio, M. (2018). Methyl jasmonate and ozone affect the antioxidant system and the quality of wine grape during postharvest partial dehydration. Food Res. Int. 112, 369–377. doi: 10.1016/j.foodres.2018.06.061
Maurer, L. H., Bersch, A. M., Santos, R. O., Trindade, S. C., Costa, E. L., Peres, M. M., et al. (2017). Postharvest UV-C irradiation stimulates the non-enzymatic and enzymatic antioxidant system of ‘Isabel' hybrid grapes (Vitis labrusca × Vitis vinifera L.). Food Res. Int. 102, 738–747. doi: 10.1016/j.foodres.2017.09.053
Mohamed, S. N. T., Ding, P., Kadir, J., and Hasanah, M. G. (2017). Potential of UVC germicidal irradiation in suppressing crown rot disease, retaining postharvest quality and antioxidant capacity of Musa AAA “Berangan” during fruit ripening. Food Sci. Nutr. 5, 967–980. doi: 10.1002/fsn3.482
Oliveira, I. R., Crizel, G. R., Severo, J., Renard, C. M. G. C., Chaves, F. C., and Rombaldi, C. V. (2016). Preharvest UV-C radiation influences physiological, biochemical, and transcriptional changes in strawberry cv. Camarosa. Plant Physiol. Biochem. 108, 391–399. doi: 10.1016/j.plaphy.2016.08.012
Park, M. H., and Kim, J. G. (2015). Low-dose UV-C irradiation reduces the microbial population and preserves antioxidant levels in peeled garlic (Allium sativum L.) during storage. Postharvest Biol. Technol.100, 109–112. doi: 10.1016/j.postharvbio.2014.09.013
Pataro, G., Sinik, M., Capitoli, M. M., Dons,ì, G., and Ferrari, G. (2015). The influence of post harvest UV-C and pulsed light treatments on quality and antioxidant properties of tomato fruits during storage. Innov. Food Sci. Emerg. Technol. 30, 103–111. doi: 10.1016/j.ifset.2015.06.003
Phonyiam, O., Kongsuwan, A., and Setha, S. (2016). Effect of short-term anoxic treatment on internal browning and antioxidant ability in pineapple cv. Phulae. Int. Food Res. J. 23, 521–527.
Pongprasert, N., Sekozawa, Y., Sugaya, S., and Gemma, H. (2011). A novel postharvest UV-C treatment to reduce chilling injury (membrane damage, browning and chlorophyll degradation) in banana peel. Sci. Hort. 130, 73–77. doi: 10.1016/j.scienta.2011.06.006
Sánchez-Torres, P., and Tuset, J. J. (2011). Molecular insights into fungicide resistance in sensitive and resistant Penicillium digitatum strains infecting citrus. Postharvest Biol. Technol. 59, 159–165. doi: 10.1016/j.postharvbio.2010.08.017
Shen, Y., Sun, Y., Qiao, L., Chen, J., Liu, D., and Ye, X. (2013). Effect of UV-C treatments on phenolic compounds and antioxidant capacity of minimally processed Satsuma mandarin during refrigerated storage. Postharvest Biol. Technol. 76, 50–57. doi: 10.1016/j.postharvbio.2012.09.006
Sheng, K., Zheng, H., Shui, S., Yan, L., Liu, C., and Zheng, L. (2018). Comparison of postharvest UV-B and UV-C treatments on table grape: changes in phenolic compounds and their transcription of biosynthetic genes during storage. Postharvest Biol. Technol. 138, 74–81. doi: 10.1016/j.postharvbio.2018.01.002
Shikano, I., McCarthy, E., Hayes-Plazolles, N., Slavicek, J. M., and Hoover, K. (2018). Jasmonic acid-induced plant defenses delay caterpillar developmental resistance to a baculovirus: slow-growth, high-mortality hypothesis in plant-insect-pathogen interactions. J. Invertebr. Pathol. 158, 16–23. doi: 10.1016/j.jip.2018.09.001
Sripong, K., Jitareerat, P., and Uthairatanakij, A. (2019). UV irradiation induces resistance against fruit rot disease and improves the quality of harvested mangosteen. Postharvest Biol. Technol. 149, 187–194. doi: 10.1016/j.postharvbio.2018.12.001
Suktawee, S., Shishido, M., Wang, S., Saito, T., Okawa, K., Ohara, H., et al. (2019). n-Propyl dihydrojasmonates influence ethylene signal transduction in infected apple fruit by Botrytis cinerea. Hortic. J. 88, 41–49. doi: 10.2503/hortj.UTD-001
Wang, S., Takahashi, H., Saito, T., Okawa, K., Ohara, H., Shishido, M., et al. (2015). Jasmonate application influences endogenous abscisic acid, jasmonic acid and aroma volatiles in grapes infected by a pathogen (Glomerella cingulata). Sci. Hortic.192, 166–172. doi: 10.1016/j.scienta.2015.06.001
Xu, W., Wang, T., Xu, S., Li, F., Deng, C., Wu, L., et al. (2016). UV-C-Induced alleviation of transcriptional gene silencing through plant-plant communication: Key roles of jasmonic acid and salicylic acid pathways. Mutat. Res. 790, 56–67. doi: 10.1016/j.mrfmmm.2016.04.003
Yamaga, I., Kuniga, T., Aoki, S., Kato, M., and Kobayashi, Y. (2016). Effect of ultraviolet-B irradiation on disease development caused by Penicillium italicum in satsuma mandarin Fruit. Hort. J. 85:86–91. doi: 10.2503/hortj.MI-074
Yang, J., Duan, G., Li, C., Liu, L., Han, G., Zhang, Y., et al. (2019a). The Crosstalks between jasmonic acid and other plant hormone signaling highlight the involvement of jasmonic acid as a core component in plant response to biotic and abiotic stresses. Front. Plant Sci. 10:1349. doi: 10.3389/fpls.2019.01349
Yang, J., Shi, W., Li, B., Bai, Y., and Hou, Z. (2019b). Preharvest and postharvest UV radiation affected flavonoid metabolism and antioxidant capacity differently in developing blueberries (Vaccinium corymbosum L.). Food Chem. 301:125248. doi: 10.1016/j.foodchem.2019.125248
Yoruk, R., and Marshall, M. (2003). Physicochemical properties and function of plant polyphenol oxidase: a review. J. Food Biochem. 27, 361–422. doi: 10.1111/j.1745-4514.2003.tb00289.x
Keywords: green mold, cellular structure, jasmonic acid, mandarin fruit, UV-C radiation
Citation: Phonyiam O, Ohara H, Kondo S, Naradisorn M and Setha S (2021) Postharvest UV-C Irradiation Influenced Cellular Structure, Jasmonic Acid Accumulation, and Resistance Against Green Mold Decay in Satsuma Mandarin Fruit (Citrus unshiu). Front. Sustain. Food Syst. 5:684434. doi: 10.3389/fsufs.2021.684434
Received: 23 March 2021; Accepted: 18 May 2021;
Published: 24 June 2021.
Edited by:
Veronica Calado, Federal University of Rio de Janeiro, BrazilReviewed by:
Asanda Mditshwa, University of KwaZulu-Natal, South AfricaPaloma Sánchez-Torres, Institute of Agrochemistry and Food Technology (IATA), Spain
Lise Korsten, University of Pretoria, South Africa
Copyright © 2021 Phonyiam, Ohara, Kondo, Naradisorn and Setha. This is an open-access article distributed under the terms of the Creative Commons Attribution License (CC BY). The use, distribution or reproduction in other forums is permitted, provided the original author(s) and the copyright owner(s) are credited and that the original publication in this journal is cited, in accordance with accepted academic practice. No use, distribution or reproduction is permitted which does not comply with these terms.
*Correspondence: Sutthiwal Setha, c3V0dGhpd2FsLnNldCYjeDAwMDQwO21mdS5hYy50aA==