- 1African Centre for Crop Improvement (ACCI), University of KwaZulu-Natal, Pietermaritzburg, South Africa
- 2Department of Biochemistry, Microbiology and Biotechnology, School of Molecular and Life Sciences, University of Limpopo, Polokwane, South Africa
- 3Towoomba Research Station, Crop Research Division, Limpopo Department of Agriculture and Rural Development, Bela-Bela, South Africa
Bottle gourd [Lagenaria siceraria (Molina) Standl.] is an important multi-purpose cucurbit crop grown for its leaf, fruit, and seed. It is widely cultivated and used for human consumption in sub-Saharan Africa (SSA) providing vital human nutrition and serving as food security crop. There is wide genetic variation among bottle gourd genetic resources in Africa for diverse qualitative and quantitative attributes for effective variety design, product development, and marketing. However, the crop is under- researched and -utilized, and improved varieties are yet to be developed and commercialized in the region. Therefore, the objective of this review is to provide the progress on bottle gourd genetic improvement and genetic analysis targeting agronomic and horticultural attributes, nutritional composition, biotic, and abiotic stress tolerance to guide current and future cultivar development, germplasm access, and conservation in SSA. The first section of the paper presents progress on breeding of bottle gourd for horticultural traits, agronomic performance, nutritional and anti-nutritional composition, and biotic and abiotic stress tolerance. This is followed by important highlights on key genetic resources of cultivated and wild bottle gourd for demand driven breeding. Lastly, the review summaries advances in bottle gourd genomics, genetic engineering and genome editing. Information presented in this paper should aid bottle gourd breeders and agronomists to develop and deploy new generation and promising varieties with farmer- and market -preferred attributes.
Introduction
Bottle gourd [Lagenaria siceraria (Molina) Standl.] is widely cultivated in sub-Saharan Africa (SSA) for multiple uses (Morimoto et al., 2005; Abdin et al., 2014; Gürcan et al., 2015; Mashilo et al., 2015). It is grown for its young and succulent leaves and young fruit which are consumed as cooked vegetable (Morimoto and Mvere, 2004; Hart, 2011; Mashilo et al., 2017a,b,c). The leaves contain various micro-and macro-elements, and phytochemical compounds beneficial to human health. Therefore, genotypes with high leaf and fruit yields are preferred for vegetable production. Sun-dried or fresh leaves are sold at local and regional markets scoping the development of value-added products and economic gains from bottle gourd enterprises. Further, fresh and dried vines are often used as livestock fodder supplement during dry spells where natural pastures are scarce. Therefore, vine development and branching capacity are key attributes for developing genotypes suited for fodder production.
Young and immature fruits of bottle gourd are highly preferred in semi-urban and urban areas as fruit vegetable. In many parts of South Africa including in KwaZulu-Natal, Gauteng and Limpopo provinces, young bottle gourd fruits with varied fruit shapes are sold at retail stores with prices ranging from 1 to 3 USD per kilogram. The dried fruit of bottle gourd has a hard shell which is ideal for making custom-made containers for decoration and other household uses. For instance, a dried fruit with neck length varying between 8 and 15 cm is valued for making containers to serve traditional beer or water. Also, designer decorative materials are prepared from dried fruits which are widely used in celebration of traditional ceremonies. Small to large oval fruits with thick rind (i.e., 2 cm) and fruit neck length of ~3 cm are used to prepare decorations and containers. Immature and ripening seeds are consumed with young fruit. The seeds are rich sources of protein, amino acids, and essential micro-and-macro elements with health-promoting benefits (Ojiako and Igwe, 2007; Said et al., 2014; Sithole et al., 2015). The number of seeds per fruit is highly variable amongst bottle gourd genotypes (Morimoto et al., 2005) providing opportunities for genotype selection with high seed yield potential. Dried seeds of bottle gourd are mainly used as roasted snack.
In SSA, bottle gourd is grown predominantly by smallholder farmers using genetically diverse landrace varieties (Mashilo et al., 2016c). There is wide genetic variation among bottle gourd genetic resources in Africa for selection and ideotype breeding. Commercial varieties are yet to be developed and deployed in the region due to a lack of dedicated genetic improvement programs of the crop. As such bottle gourd is categorized as an under-utilized crop in SSA where its production is mostly practiced by small-scale farmers under low input farming systems. Also, commercial uses of the crop (e.g., as a rootstock for watermelon production) is not known in the region limiting the development of bottle gourd as functional food and commercial crop. There are no improved cultivars released in the region for food, feed, value-adding, and for rootstock in watermelon production.
There is need for concerted and collaborative research efforts on bottle gourd among plant breeders, agronomists, geneticists in the region and internationally. This will enable knowledge and germplasm sharing and innovative research to design, develop, and release promising and well-adapted bottle gourd cultivars. The next generation of bottle gourd cultivars should encompass product profiles including quality and leave quantity, fruit, fodder, seed, nutritional compositions to serve varied value chains, and the food and feed industry. Therefore, the aim of this paper is to provide progress on bottle gourd genetic improvement and genetic analysis targeting agronomic and horticultural attributes, nutritional composition, biotic and abiotic stress tolerance to guide current and future cultivar development, germplasm access, and conservation in SSA and globally.
Progress in Breeding of Bottle Gourd
Fruit Qualitative Traits
Considerable genetic variability exists in bottle gourd genetic resources for fruit horticultural traits (Decker-Walters et al., 2001; Morimoto et al., 2005, 2006; Sivaraj and Pandravada, 2005; Mashilo et al., 2015, 2016b, 2017b) useful for strategic breeding and cultivar development. The crop show variation for fruit shape, size, length, color, and texture (Figure 1) (Morimoto et al., 2005; Sivaraj and Pandravada, 2005; Yetişir et al., 2008; Xu et al., 2014; Mashilo et al., 2015). Fruit shape is an important trait that determines usability of the crop either for food or decorative purposes. Bottle gourd fruits vary in shape such as club-shaped, globular, bottle shaped, flate, pear shaped cylindrical, elongated straight, pyriform, round (oblate), elongated curved, and oval (Sivaraj and Pandravada, 2005; Achigan-Dako et al., 2008; Yetişir et al., 2008; Mashilo et al., 2015; Kaylan et al., 2016). Fruit shape can therefore serve as a selection marker in improvement programs. There is high genetic variability for fruit shape which may provide opportunities for improving this character. Further, fruit shape is a key market-preferred trait. Therefore, knowledge on the underlying gene action conditioning fruit shape is important for developing unique and attractive fruits to increase market opportunities. Oval and pear fruit shapes in bottle gourd are controlled by a combination of one dominant and one recessive genes. Round fruit shape is controlled by two recessive genes, whereas dominant genes control the expression of long fruit shape (Kushwaha and Ram, 1996).
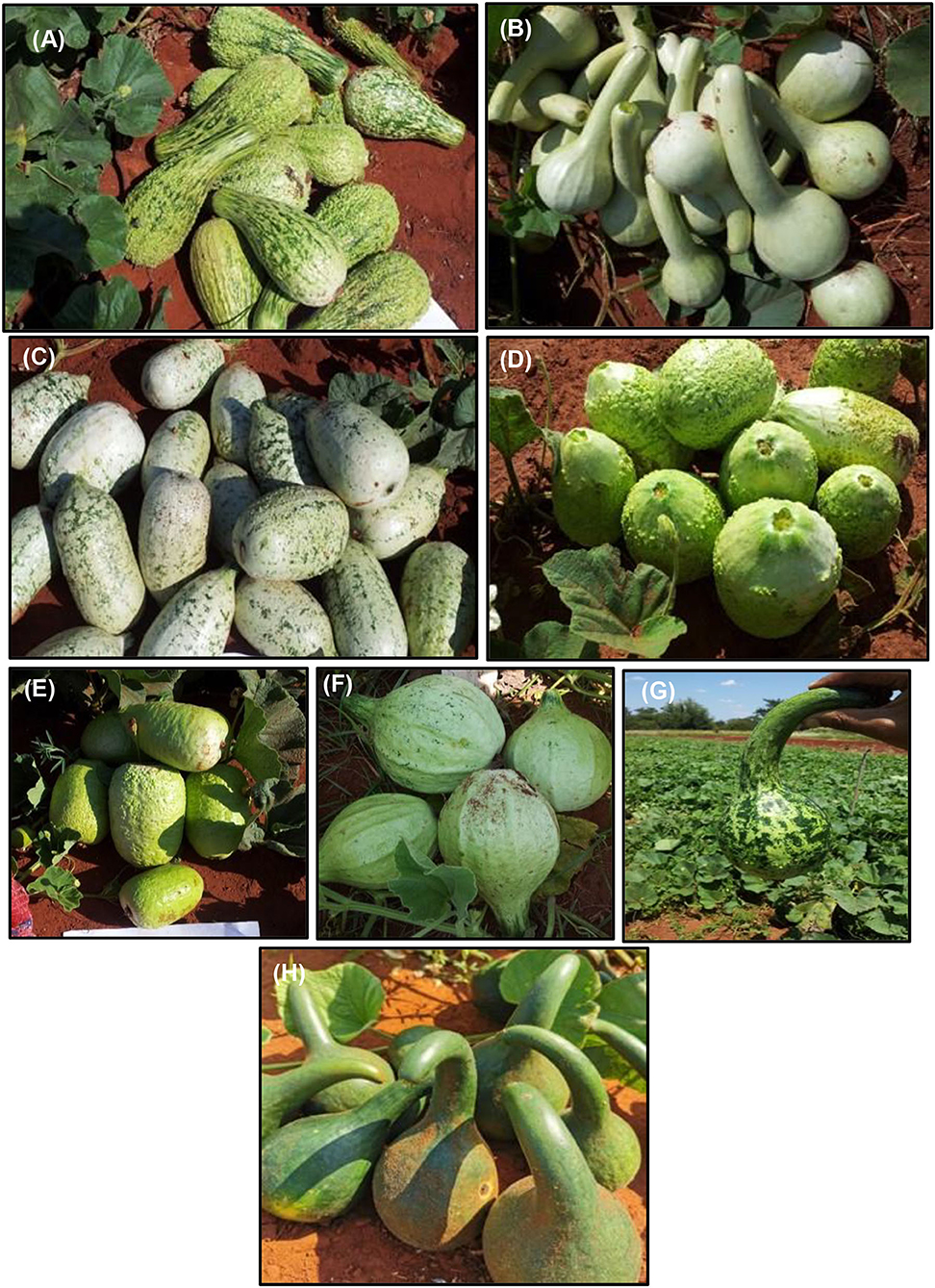
Figure 1. Fruit morphotypes of bottle gourd accessions cultivated in the Limpopo Province of South Africa. The bottle gourd accesions are denoted as BG series. (A) BG-67, verrucose fruit texture and club-shaped fruit used for food, (B) BG-78, light-green fruit color and long neck used for making containers and to serving traditional sorghum-brewed beer, (C) BG-70, white-green fruit color grown for food, (D) BG-79, cultivated for its edible fruit, (E) BG-100, commercially grown and sold in various retail outlets in KwaZulu-Natal and Gauteng provinces of South Africa, (F) BG-80, light-green fruit color, small-neck, and corrugated skin texture, (G) BG-27, dark and light green fruit color and slightly curved fruit neck, and (H) BG-31, dark green fruit color and curved fruit neck. The accessions were originally collected from various farmers' fields in the Limpopo Province of South Africa where cultivated forms of bottle gourd are prevalent. The collections are maintained by the Limpopo Department of Agriculture and Rural Development (LDARD). The codes are allocated by LDARD. The collections were cross-checked and compared with Lagenaria specimens maintained by the Agricultural Research Council—Herbarium Unit (South Africa). All collections were confirmed to be bottle gourd. (Photos provided by J. Mashilo).
The fruit neck varies considerably in shape between different genotypes (Morimoto et al., 2005; Yetişir et al., 2008; Gürcan et al., 2015; Mashilo et al., 2015). In addition, fruit neck length is highly variable in bottle gourd serving as useful trait for developing bottle gourd genotypes for ornamental purposes. Fruit neck is controlled by two genes with complementary gene action in bottle gourd (Amangoua et al., 2019). Fruit color is an important trait for developing visually attractive fruits to increase the market value. Bottle gourd fruit are characterized by smooth or “corrugated” texture or a combination of these traits (Montes-Hernandez and Eguiarte, 2002; Mladenović et al., 2012). The immense variation in fruit horticultural traits offers breeding and market opportunities. To develop “new” genotypes of bottle gourd, crosses can be made between cultivars expressing the traits of interest (e.g., neck shape, neck length, fruit shape, and color). This could be followed by selection of progenies showing the trait of interest in the F2 generation to develop desirable individuals.
Agronomic Performance
Extensive phenotypic variation exists for agronomic traits in bottle gourd (Decker-Walters et al., 2001; Morimoto and Mvere, 2004; Morimoto et al., 2005; Sivaraj and Pandravada, 2005; Mladenović et al., 2012; Mashilo et al., 2015, 2017c). Trait variation may be attributed to long agricultural history, adaptation to diverse agro-ecological conditions, and selection by famers for desirable agronomic attributes and ethnobotanical utilization (Montes-Hernandez and Eguiarte, 2002). Traits including flowering time, plant height, number of main and lateral branches per plant, fruit weight, and number of fruit per plant show high levels of variation in bottle gourd (Mashilo et al., 2015, 2017c). These traits can be exploited in pre-breeding and breeding programs for cultivar development and deployment.
Variation in fruit shape and size in bottle gourd has a direct relationship with fruit yield. Several studies reported that direct selection for agronomic traits including number of female flowers per plant, number of branches per plant, number of fruits per plant, fruit width and plant height can increase fruit yield potential in bottle gourd (Narayan et al., 1996; Behera et al., 2015; Yao et al., 2015). For direct improvement of fruit yield, selection for high number of female flowers is desirable due to high correlations between these two traits (Dey et al., 2006; Mashilo et al., 2016b). To achieve this, bottle gourd with high female flowering capacity can be crossed followed by selections and advancement of progenies expressing high number of females flowers and fruit yield (Arora et al., 1982; Cramer and Wehner, 2000). To improve seed yield, fruit weight, number of seeds per fruit and hundred seed weight may serve as important attributes for hybrid development (Yao et al., 2015). Further, characters such as number of branches, plant length, number of fruit per plant, fruit weight, number of seed per fruit, hundred seed weight, sex ratio, number of male and female flowers and seed yield are highly variable and heritable (Koffi et al., 2009; Yao et al., 2015; Janaranjani et al., 2016). Hence selection for these trait enhances genetic advancement through hybrid breeding.
Identification of parental genotypes with suitable agronomic and horticultural attributes is useful to develop high-performing hybrids. Various studies have identified genetically unrelated and promising genotypes possessing useful traits for hybrid breeding (Xu et al., 2011; Mashilo et al., 2015, 2017b, 2018; Yildiz et al., 2015). Hybrid vigor has been reported for important characters such as flowering time of female flowers, fruit weight (Dubey and Ram, 2007), hundred seed weight (Dubey and Ram, 2007; Janaranjani et al., 2016), days to 1st male flowering (Dubey and Ram, 2007; Janaranjani et al., 2016), sex ratio (i.e., female: male flowers), and number seeds per fruit (Dubey and Maurya, 2007; Dubey and Ram, 2007; Janaranjani et al., 2016). Analysis of combining ability is widely used in breeding programmes to identify superior parents for cultivar development and to identify progenies with traits of interest for genetic advancement (Acquaah, 2007). General combining ability (GCA) measures the breeding value of parental genotypes and is associated with additive genetic action. Specific combining ability (SCA) measures the performance of a progeny and is associated with non-additive gene action (i.e., dominance and epistasis (Falconer and Mackay, 1996). Both additive and non-additive gene action are reported to control inheritance of agronomic/horticultural attributes in bottle gourd (Quamruzzaman et al., 2020). For example, analysis of gene action for agronomic traits revealed that the SCA effect was greater than the GCA effect with GCA/SCA ratio of <1. This suggested the predominance of non-additive gene action over additive gene action influencing traits such as vine length, days to first male flower anthesis, days to first female flower anthesis, sex ratio, fruit length and width, fruit flesh thickness, number of seed per fruit, hundred seed weight, and fruit yield per plant (Janaranjani et al., 2016). Therefore, improvement of agronomic traits governed by non-additive genes may be targeted through hybridization among desirable parents, which may induce high SCA effects in the progenies. Therefore, heterosis breeding is well-suited to exploit improvement of traits expressing non-additive gene action (Singh, 2005; Janaranjani et al., 2016). Heterosis for desirable traits such as earliness, number of fruits and fruit yield have been reported in bottle gourd (Singh, 2005). Exploitation heterosis in bottle gourd is achievable due to its high cross-pollinating nature allowing recombination of favorable genes that may contribute to yield improvement (Morimoto et al., 2004).
In India, several high-yielding varieties have been developed by exploiting heterosis followed by mass selection from segregating populations (Sarao et al., 2014; Behera et al., 2015). Similar, hybrid breeding has been explored in various cucurbit crops including bitter gourd (Momordica charantia L.), cucumber (Cucumis sativus L.), squash (Cucurbita maxima L.), melon (Cucumis melo L.), and watermelon (Citrullus lanatus L.) (Rhodes and Zhang, 2000; Robinson, 2000; Kohli and Vikram, 2005; Al-Mamun et al., 2016). On the contrary, a higher magnitude of GCA effect compared to SCA effect were recorded for fruit yield indicating the predominance of additive gene action controlling this trait in bottle gourd (Quamruzzaman et al., 2019, 2020). Therefore, recurrent selection would also be effective for yield improvement after crossing of parental genotypes. In SSA where bottle gourd is widely grown as food and fodder crop, exploitation of hybrid vigor is feasible in this crop, however, this has not been explored and the crop remains underutilized and unexploited in breeding programmes. As a result, there are no commercial varieties available or released for production to serve the diverse value chains of the crop in the region. Hybrid varieties of bottle gourd may play a vital role in satisfying the interest of producers and consumers. The identification and utilization of the most heterotic crosses is important for hybrid breeding. Therefore, a well-planned and dynamic bottle gourd breeding programme is needed in SSA using the available genetic resources to meet the required demand for production, value-adding, rootstock development and commercialization.
Nutritional and Anti-nutritional Compositions
In SSA and Asia, bottle gourd leaves are used as cooked leaf vegetable. The immature fruit and seed are boiled and consumed as tender vegetable (Morimoto and Mvere, 2004). The leaves contains high levels of phosphorus (P), calcium (Ca), magnesium (Mg), zinc (Zn), copper (Cu), iron (Fe) and manganese (Mn) (Modgil et al., 2004; Hassan et al., 2008; Sithole et al., 2015). Potassium and Ca are the abundant mineral elements in bottle gourd leaves (Sithole et al., 2015). The fruit is vital source of amino acids notably aspartic acid (19.84–143.59 μg/g), threonine (143.72–1593.36 μg/g), serine (3.67–149.62 μg/g), glutamic acid (25.14–431.55 μg/g), alanine (11.39–222.74 μg/g), valine (7.93–126.06 μg/g), leucine acid (9.00–148.30 μg/g), phenylalanine (14.19–177.84 μg/g), lysine (6.18–160.97 μg/g), and arginine (16.15–212.42 μg/g) (Wu et al., 2017a). Bottle gourd seed contains crude protein with a value of 35% and crude lipid of 39%, essential amino acids (i.e., isoleucine, leucine, lysine, methionine, cysteine, phenylalanine, tyrosine, threonine, valine, alanine, arginine, aspartic acid, glutamic acid, glycine, histidine, proline and serine), with glutamic acid (14.3 g/100 g), leucine acid (7.66 g/100 g) and aspartic acid (9.11 g/100 g) being the predominant amino acids (Hassan et al., 2008). Ogunbusola et al. (2010) reported glutamic acid content of 139–168 mg/g protein, aspartic acid (89.0–116 mg/g protein), leucine (65.8 mg/g protein), arginine (58.6 mg/g protein), and lysine (56.2 mg/g protein) in bottle gourd seed flour. The amino acids and protein content of bottle gourd seed is as a cheap protein source adequate to meet the recommended daily allowance required for children and adults and can be augmented in cereal-based diets (Ogunbusola et al., 2010). The seeds also contain crude fat content of 47.8 g/100 g, crude protein (35.0 g/100 g), and carbohydrates (7.3 g/100 g), potassium 204.97 mg/100 g), and magnesium (33.16 mg/100 g) (Ogunbusola, 2018). Macro- and micro-nutrients and amino acid profiles present in the edible plant parts can be targeted in genetic improvement programmes for developing bio-fortified bottle gourd genotypes. Therefore, it is important to understand relationships between macro- and micro-nutrients and amino acid compositions in bottle gourd to develop breeding strategies aimed to improve nutritional profiles of the crop.
The seed also has significant amount of oil reaching up to 35%. The oil is used in the food and pharmaceutical industries. Bottle gourd oil has medicinal property for treating skin infections (Said et al., 2014). The total fatty acid content of bottle gourd seed is about 39.22% with linoleic acid (63.32%) and palmatic acid (21.36%) being the most abundant (El-dengawy et al., 2001). The nutritional value of bottle gourd may be affected by the presence of unfavorable anti-nutrients. Bottle gourd seeds contain trace amounts of tannic acid content of 1.70 mg/100 g, phytin phosphorus (0.82 mg/100 g), phytic acid (2.88 mg/100 g), and oxalate (1.58 mg/g) (Ogunbusola, 2018). Linkages of essential nutrients and with anti-nutrients can reduce bioavailability and digestibility of essential mineral elements. However, the amount of anti-nutrients in bottle gourd seed is relatively low and could further be reduced through cross breeding and selection.
The leaves and fruits are rich sources of bio-active compounds such as cucurbitacins (Attar and Ghane, 2018, 2019; Chanda et al., 2019) which have various health benefits such as antioxidant, antidiabetic, anti-tumor, anti-proliferrative and anti-microbial properties (Marzouk et al., 2012; Ma et al., 2014; Ku et al., 2017; Attar and Ghane, 2019). The contents of cucubitacins type E and I have been quantified in leaves (Mashilo et al., 2018; Chanda et al., 2019). The concentration of cucurbitacin I was 0.04%w/w in mature fruit (Chanda et al., 2019). Cucurbitacin I contents of 1.00, 6.34, and 1.61 mg/g were reported in the fruit epicarp, fruit mesocarp, and seeds of wild bottle gourd, in that order (Attar and Ghane, 2018). In sweet domesticated bottle gourd, very low concentration of cucurbitacin E (0.05 mg/g) were reported, whereas cucurbitacin I ranging from 0.12 to 14 mg/g were reported (Mashilo et al., 2018). Very high concentration of cucurbitacins cause bitterness and poisoning (Puri et al., 2011; Ho et al., 2014). For example, cucurbitacin E content varying from 2 to 12.5 mg/kg is reportedly toxic to humans. Cucurbitacin C content of 1 and 2 mg/kg is reported to be toxic (Yuan et al., 2019). Hence there is a need for a strategic breeding approach to develop varieties with desirable cucurbitacin contents to reduce phyto-toxicity and to enhance the use of bottle gourd fruit for medicinal purposes. To develop bottle gourd genotypes with enhanced nutritional and phytochemical compositions, germplasm profiling for micro-and-macro nutrients, amino acids, oil and fatty acids contents, anti-nutrients, and phytochemical compounds is required. This will ascertain the levels of genotypic variation for nutritional and phyto-compounds. Association studies between nutritional, anti-nutrients, and phyto-compounds are required to develop an effective breeding programme to improve selection efficiency combing the desired traits. Planned crossing between desirable genotypes followed by assessment of gene action governing inheritance of nutritional and anti-nutritional traits in the parents and progenies is vital. Appropriate selection strategies will then be used for selection and genetic advancement of promising lines for further evaluation, release, and commercialization.
Biotic Stress Tolerance
Bottle gourd is moderately resistant to a number of viral and fungal caused diseases such as papaya ringspot, Fusarium wilt, powdery mildew, cercospora leaf spot and tobacco mosaic virus (Yetişir and Sari, 2003; Yetişir et al., 2007; Kousik et al., 2008), cucumber mosaic virus (CMV), papaya ringspot virus (PRSV-P), watermelon mosaic virus 2 (WMV-2), and yellow mosaic virus (ZYMV) (Provvidenti, 1981, 1995; Provvidenti and Gonsalves, 1984; Ling and Levi, 2007). Owing to its desirable biotic stress resistance, bottle gourd is one of the four commercial rootstocks widely used in watermelon production (Yetişir and Sari, 2003; Cohen et al., 2007; Kousik et al., 2008; Keinath and Hassell, 2014) to improve resistance to soil-borne pathogens, improve fruit yield and quality of grafted watermelon (Yetişir et al., 2008; Çandir et al., 2013). For example, sweet watermelon (cv Crimson Tide) grafted onto bottle gourd rootstocks namely FR Gold, Emphasis, 216 and Skopje bottle had increased fruit yield by 8.98, 9.64, 8.20, and 13.26 kg/m2 compared to non-grafted plants (6.43 kg/m2) (Yetişir et al., 2003). Sweet watermelon (cv Suprit) grafted onto bottle gourd rootstocks namely: Arka Bahar and Pusa Samriddhi recorded high fruit yield at 4.1 and 4.2 kg, respectively compared with un-grafted watermelon plants (3.63 kg) (Pal et al., 2020). Grafted sweet watermelon (cv Crimson Tide) onto bottle gourd rootstock designated as 01-16, 07-06, 09-01, 31-08, 31-09, 33-02, 33-45, 46-03, and Macis had increased the total sugar content being >9% compared to non-grafted plants (~8%) (Çandir et al., 2013). Further, increased contents of total soluble solids to titratable acidity ratio, juice pH, citric acid, organic acid, lycopene, and carotenoid were reported on sweet watermelon grafted onto bottle gourd rootstock compared to non-grafted control (Çandir et al., 2013). Flesh firmness of 9.41 kg cm−2 was reported for sweet watermelon (cv Zaojia 8424) grafted onto bottle gourd rootstock (cv Jingxinzhen 1) compared to non-grafted plants (6.64 kg cm−2) (Huang et al., 2016). Transcriptome analysis of grafted watermelon onto bottle gourd rootstock revealed activation of genes involved in the increase of fruit size and rind toughness affirming the beneficial impact of bottle gourd as rootstock for improving fruit quality of grafted watermelon (Garcia-Lonazo et al., 2020). Rootstock: scion graft combinations and compatibility of genetically diverse bottle gourd and sweet watermelon genetic resources are yet to be determined for improving fruit yield and quality of grafted watermelon for commercial production. Some bottle gourd rootstocks currently used for grafted watermelon in various countries such as the USA and China include Coloso, Emphasis, Macis, Skopje, Fr Gold, Jingxinzhen no.1, WMXP3938 and WMXP3945 (Yetişir et al., 2003; Thies et al., 2010; Keinath and Hassell, 2014; Zhong et al., 2018).
Several bottle gourd genotypes resistant to powdery mildew race 1 (Kousik et al., 2008) such as PI271353, PI 271357, and PI 271359 possess moderate to high resistance to Zucchini yellow mosaic virus (Provvidenti and Gonsalves, 1984; Ling and Levi, 2007). PI 271353 was selected for its resistance against a number of viruses such as cucumber mosaic virus, squash mosaic virus, and watermelon mosaic virus (Rai et al., 2008). Some varieties such as Pusa Naveen, Pusa Santushti, Pusa Samridhi, and Pusa Sandesh reportedly had high level of resistance to cercospora leaf spot (Maheshwari et al., 2015). From these reports there is higher possibility of developing highly resistant gourd rootstocks/hybrids for watermelon production. Potential bottle gourd genotypes have been identified which may serve as useful for rootstocks commercial production of grated watermelon (Yetişir et al., 2007; Kousik et al., 2008; Keinath and Hassell, 2014). These include accessions 33-15, 31-08, 31-09, 48-07, 07-04, 07-06, 07-42, 09-01, 35-01, and 07-06 identified in Turkey (Yetişir et al., 2007; Yetişir and Karaca, 2018) and plant introductions PI271353, PI271357, PI381840, PI273663, PI271353, PI271357, PI381840, PI273663, USVL1-8, USVL5-5, USVL351-PMR, and USVL482-PMR identified in the USA (Kousik et al., 2008, 2018; Ling and Levi, 2013). Development of bottle gourd genotypes with multiple disease resistance could result in highly-adapted and highly performing bottle gourd genotypes for production or use as rootstocks. For example, bottle gourd genotype PI 280633 originally collected from South Africa show complete resistance to ZYMV (Ling and Levi, 2007). Furthermore, genotypes such as Emphasis, Macis, PI 27353, USVL1-8, and USVL5-5 with resistance to powdery mildew, water melon mosaic virus, and zucchini yellow mosaic virus are useful germplasm for developing rootstocks with multiple disease tolerance. Bottle gourd hybrids such as FR-Ganggeon and FR-Sinsegye developed from crosses between FRD 22 × 963381 and FRD 2010 × 963385-2 show high levels of resistance to Fusarium wilt (Huh et al., 2012). Further, some hybrids developed in India display high levels of resistance against Fusarium wilt (Dhillon et al., 2016). To develop superior bottle gourd rootstock for watermelon production, the rooting characteristics of the cultivated accessions requires rigorous evaluations. Accessions with high deep rooting system and vining ability expressing high fruit potential can be selected for further evaluations. This is followed by grafting on widely grown watermelon cultivars followed by evaluations for yield potential and fruit quality assessment from rootstock: scion combinations. Potential accessions can then be recommended as rootstocks for commercialization and production of watermelon in SSA.
Abiotic Stress Tolerance
Bottle gourd exhibit some level of drought tolerance compared to other cucurbits (Samadia, 2002; Quamruzzaman et al., 2009; Mashilo et al., 2017a). Furthermore, the crop exhibits high salinity tolerance (Nisini et al., 2002; Yetişir and Sari, 2003; Colla et al., 2005; Yang et al., 2015; Yetişir et al., 2016) and hence widely used as rootstock for watermelon. Several drought tolerant landrace varieties have been identified (Mashilo et al., 2016a, 2017a, 2018). Also, genotypes including L28-16-3, LS28-17-2, Macis, Argentario, and Chaofeng Kangshengwang were reported to be salt tolerant (Yang et al., 2012, 2013, 2015; Yetişir and Karaca, 2018). Strategic crosses among drought tolerant landrace accessions of bottle gourd may enhance the level of drought tolerance and development of well-adapted hybrids.
Understanding of the physiological basis of drought tolerance in bottle gourd can aid effective screening and identification of novel genetic resources for breeding. Secondary metabolites such as cucurbitacins are produced in response to drought and heat stress and may also be important indicators of stress tolerance (Balkema-Boomstra et al., 2003; Mashilo et al., 2018). However, information is scanty supporting their role in conferring drought tolerance in cucurbit crops. Mashilo et al. (2018) reported increased levels of cucurbitacin E and I in response to water stress. The authors suggested that the accumulation of cucurbitacins may be a potential physiological marker for identification and selection of bottle gourd genotypes for drought tolerance breeding. Therefore, understanding the role of cucurbitacins for abiotic stress tolerance may aid as a novel selection/breeding criterion for abiotic stress tolerance breeding in bottle gourd or related cucurbit crops. Profiling of diverse cucurbitacins should be carried out involving a broader bottle gourd genetic pool to identify novel genetic resources that can be used as mapping populations to unravel molecular mechanisms and identification of genes regulating cucurbitacin biosynthesis in bottle gourd.
Genetic Resources of Cultivated and Wild Bottle Gourd
The genus Lagenaria comprises of the cultivated bottle gourd and five wild species including: L. breviflora (Benth.) Roberty, L. abyssinica (Hook f.) Jeffrey, L. rufa (Gilg.) Jeffrey, L. sphaerica (Sonder) Naudin, and L. guineensis (G. Don) Jeffrey (Whitaker, 1971; Jeffrey, 1976). Germplasm collection of the cultivated bottle gourd are available in several genebanks such in the USA, Africa (i.e., South Africa, Zimbabwe, and Kenya), Asia (i.e., China and Japan), and Turkey (Morimoto et al., 2005; Kousik et al., 2008; Yetişir et al., 2008; Xu et al., 2014). For wild species, a large set of collections of L. sphaerica and few collections of L. abyssinica and L. breviflora were reported in Kenya (Morimoto et al., 2005). There is generally limited data available regarding germplasm status of wild Lagenaria species in genebanks. Morphologically, the cultivated bottle gourd show extensive variation compared to its wild relatives for quantitative fruit traits (Morimoto et al., 2005). Reportedly, intra-specific variations exists between L. siceraria and L. sphaerica, L. abyssinica and L. breviflora (Decker-Walters et al., 2001; Morimoto et al., 2006). Within the genotypes of L. sphaerica, there also exists extensive genotypic variation (Morimoto et al., 2006). However, there is inconclusive evidence on phylogenic relationships among genotypes of L. abyssinica and L. breviflora (Morimoto et al., 2006) and this is yet to be resolved using diagnostic molecular markers. Further, there is limited data on the extent of introgression between the cultivated and wild species of bottle gourd. Wild species are a useful genetic resource for introgressing useful genes for biotic and abiotic stress tolerance. For example, hybrids derived between cowpea [Vigna unguiculata (L.) Walp.] and its wild relatives V. umbellata and V. exilis showed enhanced drought tolerance (Takahashi et al., 2015). Inter-specific hybrids of the cultivated eggplant (Solanum melongena) with wild species S.insanum displayed higher levels of drought tolerance (Kouassi et al., 2021). For effective use of wild Lagenaria species for breeding, there is need for comparison based on qualitative and quantitative traits using relatively large germplasm set to determine the levels of variation for these traits. Also, heat and drought tolerance, and diseases resistance studies on wild species of Lagenaria are required for genotype identification and selection. This information is critical to develop breeding strategies incorporating wild relatives in breeding programmes. The domesticated bottle gourd genotypes are non-bitter, whereas wild forms are very bitter (Sivaraj and Pandravada, 2005; Morimoto et al., 2006). Fruit bitterness is associated with two RAPD markers suggesting that bitter genotypes of wild species are genetically distinctive from non-bitter cultivated types (Morimoto et al., 2006). Bitterness in the wild species is caused by accumulation of cucurbitacin I (Attar and Ghane, 2018). The low levels of cucurbitacins in domesticated bottle gourd suggest that growers could have selected for non-bitterness through domestication and unconscious selection (Qi et al., 2013). As a result, wild species of bottle gourd may be useful sources of phytochemical compounds especially cucurbitacins for pharmaceutical applications. We propose comparative analysis of cucurbitacins profiling between cultivated and wild bottle gourd species to determine the worth of wild species as sources of phytochemical compounds. Also, there is scant information on nutritional compositions of wild bottle gourd species and its worthwhile for breeding for nutritionally-enhanced genotypes in the cultivated bottle gourd. Comparative analysis of macro-and micro nutrients, amino acids, oil, and fatty acids contents between the cultivated and wild species is required to guide breeding approaches.
Advances in Bottle Gourd Genomics
Marker-assisted breeding is a complementary tool to phenotyping allowing accelerated cultivar development and deployment. Molecular markers such as randomly amplified polymorphic DNA (RAPD), simple sequence repeat (SSR), sequence-related amplified polymorphism (SRAP), inter-simple sequence repeat (ISSR), and single nucleotide polymorphism (SNP) markers have been used to assess genetic variability in bottle gourd and identified genetically distant genotypes for cultivar development (Provvidenti and Gonsalves, 1984; Singh et al., 2010; Xu et al., 2011; Abdin et al., 2014; Srivastava et al., 2014; Bhawna et al., 2015; Gürcan et al., 2015; Mashilo et al., 2016c). Among molecular markers deployed for genetic analysis of bottle gourd genetic resources, SSR markers show high degree of polymorphism and are highly discriminative of bottle gourd germplasm (Xu et al., 2011; Sarao et al., 2014; Bhawna et al., 2015; Mashilo et al., 2017c). Limited SSR markers have been developed for genetic differentiation of bottle gourd genetic resources. Development of SSR markers will more genomic coverage are useful to facilitate rapid screening of large bottle gourd populations including wild species for identification and selection of genetically unrelated and complementary genotypes for hybrid breeding. The marker data will complement genotype selection based on agronomic, horticultural and nutritional attributes, and biotic and abiotic stress tolerance for effective breeding.
Sequence databases that allows for high quality genome assemblies are available for bottle gourd (Xu et al., 2011; Wang et al., 2018). This include the GourdBase, RNA-Seq, UniProt, SNAP, AUGUSTUS, PASA2 pipe-line, Pfam, MARCOIL, and cucurbit genomic databases (Xu et al., 2011; Wu et al., 2017b; Wang et al., 2018). GourdBase consist of more than 27,500 genes, over 6,000 molecular markers, fruit shape phenotypes for over 100 genetically diverse bottle gourd accessions and 56 quantitative trait loci (QLTs) controlling various traits such as umami taste, fruit shape and bitterness (Wang et al., 2018). Transcriptome sequencing data determined regulation of fruit size in bottle gourd revealing a total of 1,250 differentially expressed genes (DEG) of which 422 genes were upregulated and 828 genes were downregulated. The genes were associated with various physiological functions including cell wall metabolism, phytohormones, cell cycle, and cell division (Zhang H. et al., 2020). These genomic resources present opportunities for bottle gourd genetic analysis and improvement to accelerate breeding of novel genotypes for diverse markets and consumers. Further, the available genomic resource will aid dissection of QTLs linked to agronomic and horticultural traits and nutritional attributes, and resistance to biotic and abiotic resistance to facilitate breeding and cultivar deployment.
To date, there is little information regarding QTLs controlling qualitative and quantitative traits, biotic and abiotic stress resistance in bottle gourd. Two QTLs namely QTL QBt.1 and QTL QBt.2 were reported to regulate bitter taste in bottle gourd (Wu et al., 2019). Thirty-seven genes conditioning plant growth and development have been identified in bottle gourd. The genes encode plant-specific transcription factors. Most of these genes were located in the nucleus, chloroplast, and mitochondria (Sidhu et al., 2020). In other cucurbit crops such as bitter gourd and Cucurbita pepo, QTLs have been mapped for different traits such as sex ratio (i.e., QTLs qSR14a-F3, qSR14b-F3 and qSR14b-F3), sex expression (i.e., QTL gy/fffn/ffn), node at first pistillate flower appearance (i.e., QTL qNPF9-F3 and qNPF14-F2), fruit epidermal structure (i.e., QTL fwa and Wr), immature fruit color (i.e., w/L/H°), and days to first pistillate flower appearance (i.e., QTL qDPF14-F3) (Montero-Pau et al., 2017; Cui et al., 2018; Rao et al., 2018). In addition, QTLs associated with fruit yield per plant (QTL ypp5.1), number of fruits per plant (QTLs fpp5.1 and ypp5.1), fruit weight (QTLs fw4.1, fw5.1, fw6.1, and fw12.1), first female flower node (QTL ffn9.1), female flower ratio (QTL ffr5.1 and ffr4.1) and fruit length (fl1.1) were mapped in bitter gourd (Wang and Xiang, 2013). QTLs with positive correlation with fruit shape (QTL IFSh_3, IFSh_12, MFSh_4, and MFSh_5), fruit length (QTLs MFLe_3 MFLe_12, MFLe_6, MFLe_9, IPeLe_10, IPeLe_16, and MPeLe_14), fruit color (QTLs ILRCo_4, ILRCo_10, ILRCo_1, ibRCo_4, ibRCo_3, ibRCo_12, laRCo_10, laRCo_3, MLRCo_4, MLRCo_1, and MLRCo_2) were reported in Cucurbita pepo and other cucurbits (Montero-Pau et al., 2017). Two QTLs associated with flowering time and four QTLs linked to pollen fertility (qPF1.1, qPF1.2, qPF3, and qPF7) were mapped in hybrids developed between Luffa acutangula (L.) Roxb. and L.cylindrica (L.) Roem. (Wu et al., 2016). To develop QTLs in bottle gourd, genetic linkage maps using an F2 population derived from desirable parental genotypes can be generated. This is followed by QTL mapping to dissect genomic regions associated with desired traits. This approach is widely undertaken in other cucurbit crops for QTL mapping and marker-assisted breeding (Wu et al., 2016; Cui et al., 2018) and provides opportunities for gene mapping in bottle gourd.
Heat and drought stress are major production constraints to bottle gourd production in arid and semi-arid environments. Seven heat-tolerant quantitative trait loci (qHT1.1, qHT2.1, qHT2.2, qHT5.1, qHT6.1, qHT7.1, and qHT8.1) were reported in bottle gourd. QTL qHT2.1 was identified with major genetic effect linked to drought tolerance (Song et al., 2020). SNP markers namely SNP 2, SNP 16, and SNP 31 were associated with heat tolerance (Song et al., 2020) which are useful genomic resources for marker-assisted selection for heat tolerance in bottle gourd. Plant diseases are a major constraint in bottle gourd production (Mashilo et al., 2017b). High quality bottle gourd genome sequence facilitated mapping of a dominant monogenic locus (Prs) that conferred resistance to papaya-ringspot virus (Wu et al., 2017c). Several multiple genes such as LsWD, LsGAPDH, LsH3, LsP4H, and LsCYP associated with resistance to cucumber green mottle mosaic virus affecting bottle gourd were mapped (Zhang et al., 2018). Recently, a gene designated HG_GLEAN_10011803 was identified and thought to be the major gene conferring resistance to Fusarium wilt in bottle gourd (Yanwei et al., 2021). High-density genetic maps have been developed employing various molecular markers including ISSR, SSR and SNPs (Xu et al., 2011, 2014; Bhawna et al., 2015). This allowed marker–trait associations using genome wide association (GWAS) such as conditioning free glutamate content (Wu et al., 2017a). In melon and cucumber, GWAS was successfully employed to identify genomic regions associated with Fusarium wilt resistance (Joobeur et al., 2004; Zhang et al., 2014). Bottle gourd genotypes possessing resistance to economically important diseases such as zucchini yellow mosaic virus, water melon mosaic virus, squash mosaic virus, tomato ringspot virus, tobacco mosaic virus, cucumber green mottle mosaic virus and powdery mildew have been identified (Provvidenti, 1981, 1995; Kousik et al., 2008; Ling and Levi, 2013). These are useful sources to identify candidate genes linked to disease resistance. However, QTL controlling disease resistance remains under-studied thus limiting marker-assisted breeding for biotic stress resistance of bottle gourd. Further, molecular markers linked to economically important diseases of bottle gourd remains limited for marker-assisted breeding. We propose GWAS to identify SNPs and genomic regions associated with resistance to economically important diseases in bottle gourd. This will aid to design of closely associated molecular markers linked to disease resistance for effective germplasm characterization and breeding. For development of molecular markers, next-generation sequencing (NGS) technologies have been widely used in cucurbit crops such as watermelon, bitter gourd and bottle gourd to generate large amounts of sequence information for marker development (Ren et al., 2012; Zhu et al., 2016; Wu et al., 2019). NGS technology can also be applied in bottle gourd for marker development associated with disease resistance. Generally, NGS may assist in development of genomic tools for bottle gourd breeding to facilitative cultivar development with desirable traits for consumers and various industries. Cytogenetic analysis of under-researched crops such as bottle gourd is useful to achieve successful crossing, and for conventional and genomic selection programs to improve economic traits. Achigan-Dako et al. (2008) reported genome size differences reaching up to 9.5% within the cultivated genotypes of L. siceraria with diploid nuclear DNA value (2C) varying from 0.702 to 0.759 picograms. Generally, there is dearth of information on genome size and chromosomal variation in cultivated and wild species of bottle gourd.
Genetic Engineering and Genome Editing
Genetic engineering is the technique of inserting new genetic information into existing cells in order to improve the expression of traits of interest. Agrobacterium tumefaciens-mediated transformation provided opportunities for incorporating useful traits in bottle gourd (Han et al., 2004, 2005; Cho et al., 2017). An Arabidopsis CBF3/DREB1A gene which confers cold acclimation was successfully transferred into bottle gourd using Agrobacterium-mediated transformation (Cho et al., 2017). These resulted in cold resistant transgenic plants compared to non-transgenic plants. The CBF3/DREB1A gene in useful for developing bottle gourd genotypes for rootstock purposes for grafting watermelon to enhance tolerance to cold temperatures. Bottle gourd expressing high levels of drought tolerance was developed incorporating the Arabidopsis AVP1 gene that encodes a vacuolar H+-pyrophosphatase. After 10 days of re-watering, wild-type plants showed minimal growth while the AVP1-expressing plants resumed rapid growth. Bottle gourd genotype expressing the AVP1-gene showed better recovery after 12 days of drought stress and rewatering displaying longer primary roots and more robust root systems compared to wild bottle gourd genotype (Park et al., 2014). Also, transgenic bottle gourd expressing the Arabidopsis H+-pyrophosphatase AVP1 gene showed improved salt tolerance and maintained higher relative water content under salt stress condition (Park et al., 2014). To improve the performance of bottle gourd as a rootstock, genetic engineering using Arabidopsis Ca2+/H+ exchanger sCAX2B resulted in enhanced Ca2+ substrate specificity and decreased Mn2+ transport capability. This resulted in watermelon/transgenic bottle gourd (scion/rootstock) combinations with higher osmotic pressure and more soluble solids suggesting that the sCAX2B gene improve watermelon fruit quality by effective translocation of nutrients (Han et al., 2009). Watermelon plants grafted onto transgenic bottle gourd rootstock expressing the Arabidopsis H+-pyrophosphatase AVP1 gene demonstrated high levels of salt tolerance than those grafted onto wild-type bottle gourd rootstock (Han et al., 2015). These studies, demonstrated that genetic engineering by incorporating CBF3/DREB1A and AVP1 genes can result in bottle gourd genotypes with improved abiotic stress tolerance and agronomic performance. Success of genetic transformation depends on a reliable tissue culture regeneration system, gene construct(s), suitable vector(s) for transformation and efficient procedures to introduce desired genes into target plants. Bottle gourd cotyledons are the widely used plant tissue for genetic transformation (Han et al., 2004, 2005; Han et al., 2015).
For improving biotic stress tolerance, genetic engineering has potential for developing disease and inspect pest resistant genotypes. Transgenic watermelon lines expressing artificial microRNAs that target Cucumber Mosaic Virus (CMV) 2a/2b genes developed via Agrobacterium mediated-transformation displayed resistance to CMV infection (Liu et al., 2016). A watermelon rootstock named “Gongdae” resistant to Cucumber Green Mottle Mosaic Virus (CGMMV) was developed using Agrobacterium mediated cDNA encoding the CGMMV coat protein gene (CGMMV-CP) (Park et al., 2005). Resistance to fungal disease (i.e., powdery mildew), virus diseases (i.e., cucumber green mottle mosaic, watermelon virus diseases) and insect pests (i.e., aphids and thrips) is yet to be achieved in bottle gourd through genetic engineering.
Genome editing offers great potential to develop agronomically and nutritionally superior genotypes with biotic and abiotic stress tolerance. CRISPR/Cas9 is the widely used genome editing platform allowing for substantial improvement of economic traits. In watermelon, CRISPR/Cas9 system was used to perform genome editing of ClPDS, a phytoene desaturase gene in watermelon which confers the albino phenotype. Transgenic watermelon plants harbored the ClPDS mutations and showed the albino phenotype, indicating that CRISPR/Cas9 offers effective genome editing efficiency to develop transgenic watermelon lines with desired traits (Tian et al., 2017). CRISPR/Cas9 system was successfully employed to knockout the Clpsk1 gene, encoding the PSK precursor, to confer enhanced Fusarium oxysporum f.sp. niveum (FON) resistance in watermelon (Zhang M. et al., 2020). Development of Zucchini yellow mosaic virus and Papaya ring spot mosaic virus-W resistant cucumber lines was developed using Cas9/subgenomic RNA (sgRNA) technology to disrupt the function of the recessive eIF4E (eukaryotic translation initiation factor 4E) gene (Chandrasekaran et al., 2016). Genetic modification is an effective approach for creating genetic variation for desired traits for bottle gourd improvement, however this approach has not been widely adopted. Lack of efficient protocols for transformation, effective gene delivery, selection of transformed cells, propagation, and regeneration methods are among the technical challenges limiting genetic modification in bottle gourd (Song et al., 2019).
Conclusions
There is extensive genetic variation of bottle gourd in Africa for diverse qualitative and quantitative horticultural attributes for variety design, product development, and marketing. However, bottle gourd is under- researched and –utilized crop in sub-Saharan Africa. Improved varieties are yet to be developed and commercialized in the region to serve the diverse human needs and for the market place. The present review summarized progress on bottle gourd breeding, genetic resources, and advances in bottle gourd genomics, genetic engineering and genome editing to guide cultivar development. There is need for collaborative research on bottle gourd involving plant breeders, agronomists, geneticists, and food scientists in the region and internationally for knowledge and germplasm sharing and innovative product development. The next generation of bottle gourd cultivars should encompass product profiles including quality and quantity leaves, fruit, fodder, seed, and nutritional compositions to serve varied value chains and the food and feed industry.
Author Contributions
All authors listed have made a substantial, direct and intellectual contribution to the work, and approved it for publication.
Funding
The University of KwaZulu-Natal (UKZN), University of Limpopo (UL), and the Limpopo Department of Agriculture and Rural Development are acknowledged for financial support of this study.
Conflict of Interest
The authors declare that the research was conducted in the absence of any commercial or financial relationships that could be construed as a potential conflict of interest.
References
Abdin, B. M., Arya, L., Sureja, D. S. A. K., and Verma, M. (2014). Population structure and genetic diversity in bottle gourd [Lagenaria siceraria (Mol.) Standl.] germplasm from India assessed by ISSR markers. Plant Syst. Evol. 300, 767–773. doi: 10.1007/s00606-014-1000-5
Achigan-Dako, E. G., Fuchs, J., Ahanchede, A., and Blattner, F. R. (2008). Flow cytometric analysis in Lagenaria siceraria (Cucurbitaceae) indicates correlation of genome size with usage types and growing elevation. Plant Syst. Evol. 276, 9–19. doi: 10.1007/s00606-008-0075-2
Al-Mamun, M. H., Rashid, M. H., Uddin, M. N., Islam, M. R., and Asaduzzaman, M. (2016). Heterosis studies in bitter gourd. Int. J. Veg. Sci. 22, 442–450. doi: 10.1080/19315260.2015.1072613
Amangoua, N. F., Koffi, K. K., Baudoin, J. P., and Zoro Bi, I. A. (2019). Inheritance of fruit neck, rind and seed coat hardness, and seed coat colour in bottle gourd. SA J. Plant. Soil. 36, 57–64. doi: 10.1080/02571862.2018.1484191
Arora, S. K., Pandita, M. L., and Sidhu, A. S. (1982). Effect of maleic hydrazide on vegetative growth, flowering and fruiting of bottle gourd. Sci. Hortic. 17, 211–215. doi: 10.1016/0304-4238(82)90042-5
Attar, U. A., and Ghane, S. G. (2018). Optimized extraction of anti-cancer compound – cucurbitacin I and LC-MS identification of major metabolites from wild bottle gourd (Lagenaria siceraria (Molina) Standl.). SA J. Bot. 119, 181–187. doi: 10.1016/j.sajb.2018.09.006
Attar, U. A., and Ghane, S. G. (2019). In vitro antioxidant, antidiabetic, antiacetylcholine asterase, anticancer activities and RP-HPLC analysis of phenolics from the wild bottle gourd (Lagenaria siceraria (Molina) Standl.). SA J. Bot. 125, 360–370. doi: 10.1016/j.sajb.2019.08.004
Balkema-Boomstra, A. G., Zijlstra, S., Verstappen, F. W. A., Inggamer, H., Mercke, P. E., and Jongsma, M. A. (2003). Role of Cucurbitacin C in resistance to spider mite (Tetranychus urticae) in cucumber (Cucumis sativus L.). J. Chem. Ecol. 29, 225–235. doi: 10.1023/A:1021945101308
Behera, T., Sirohi, P., Pal, A., and Singh, I. (2015). ‘Pusa Santushti’: a new high yielding bottle gourd variety. Int. J. Veg. Sci. 21, 323–328. doi: 10.1080/19315260.2013.876565
Bhawna, M. Z., Abdin, L., Arya, L., and Verma, M. (2015). Transferability of cucumber microsatellite markers used for phylogenetic analysis and population structure study in bottle gourd (Lagenaria siceraria (Mol.) Standl.). Appl. Biochem. Biotechnol. 175, 2206–2223. doi: 10.1007/s12010-014-1395-z
Çandir, E., Yetişir, H., Karaca, F., and Üstün, D. (2013). Phytochemical characteristics of grafted watermelon on different bottle gourds (Lagenaria siceraria) collected from the Mediterranean region of Turkey. Turkish J. Agric. For. 37, 443–456. doi: 10.3906/tar-1207-21
Chanda, J., Biswas, S., Kar, A., and Muherjee, P. K. (2019). Determination of cucurbitacin E in some selected herbs of ayurvedic importance through RP-HPLC. J. Ayurveda Integrative Med. 34, 43–56. doi: 10.1016/j.jaim.2019.01.002
Chandrasekaran, J., Brumin, M., Wolf, D., Leibman, D., Klap, C., Pearlsman, M., et al. (2016), Development of broad virus resistance in non-transgenic cucumber using CRISPR/Cas9 technology. Mol. Plant. Pathol. 17, 1140–1153. doi: 10.1111/mpp.12375
Cho, S. H., Joung, Y. H., Karna, S., Lee, H. E., Kim, J. H., Kim, J. H., et al. (2017). The development of cold resistance rootstock using Agrobacterium-mediated transformation of Arabidopsis CBF3/DREB1A in bottle gourd (Lageneraria siceraria Standl.). Sci. Hortic. 214, 141–146. doi: 10.1016/j.scienta.2016.11.017
Cohen, R., Burger, Y., and Horev, C. (2007). Introducing grafted cucurbits to modern agriculture: the Israeli experience. Plant Sci. 91, 916–923. doi: 10.1094/PDIS-91-8-0916
Colla, G., Fanasca, S., Cardarelli, M., Rouphael, Y., Saccard, F., Graifenberg, A., et al. (2005). Evaluation of salt tolerance in rootstocks of Cucurbitaceae. Acta Hort. 697, 469–474. doi: 10.17660/ActaHortic.2005.697.59
Cramer, C. S., and Wehner, T. C. (2000). Path analysis of the correlation between fruit number and plant traits of cucumber populations. HortScience 35, 708–711. doi: 10.21273/HORTSCI.35.4.708
Cui, J., Luo, S., Niu, Y., Haung, R., Wen, Q., Su, J., et al. (2018). A RAD-based genetic map for anchoring scaffold sequences and identifying QTLs in bitter gourd (Momordica charantia). Front. Plant Sci. 9:477. doi: 10.3389/fpls.2018.00477
Decker-Walters, D., Staub, J., López-Sesè, A., and Nakata, E. (2001). Diversity in landraces and cultivars of bottle gourd (Lagenaria siceraria; Cucurbitacaea) as assessed by random amplified polymorphic DNA. Genet. Resour. Crop Evol. 48, 369–380. doi: 10.1023/A:1012079323399
Dey, S. S., Singh, A. K., Chandel, D., and Behera, T. K. (2006). Genetic diversity of bitter gourd (Momordica charantia L.) genotypes revealed by RAPD markers and agronomic traits. Sci. Hort. 109, 21–28. doi: 10.1016/j.scienta.2006.03.006
Dhillon, N. P. S., Sanguansil, S., Singh, S. P., Masud, M. A. T., Kumar, P., Bharathi, L. K., et al. (2016). “Gourds: bitter, bottle, wax, snake, sponge and ridge,” in Genetics and Genomics of the Cucurbitaceae, eds R. Grumet, N. Katzir, and J. Garcia-Mas (Cham: Springer Intl Pub AG). pp 155–172. doi: 10.1007/7397_2016_24
Dubey, H. K., and Ram, H. H. (2007). Genetics of seed yield and its components in bottle gourd [Lagenaria siceraria (Molina) Standl.]. Cucurbits Genet. Coop. Rep. 30, 64–70.
Dubey, S. K., and Maurya, I. B. (2007). Combining ability for characters related to yield and earliness in bottle gourd [Lagenaria siceraria (Mol.) Standl.]. Ind. J. Agric. Res. 41, 59–62.
El-dengawy, R. A., Khalifa, A., and Ramadan, B. R. (2001). Use of bottle gourd (Lagenaria siceraria) seeds in production of Tahina. Egyptian J. Food Sci. 29, 1–11.
Falconer, D. S., and Mackay, T. F. C. (1996). Introduction to Quantitative Genetics, 4th Edn. Harlow, DC: Pearson Prentice Hall.
Garcia-Lonazo, M., Dutta, S. K., Natarajan, P., Tomason, Y. R., Lopez, C., Katam, R., et al. (2020). Transcriptome changes in reciprocal grafts involving watermelon and bottle gourd reveal molecular mechanisms involved in increase of the fruit size, rind toughness and soluble solids. Plant Mol. Biol. 102, 213–223. doi: 10.1007/s11103-019-00942-7
Gürcan, K., Say, A., and Yetişir, H. (2015). A study of genetic diversity in bottle gourd [Lagenaria siceraria (Molina) Standl.] population, and implication for the historical origins on bottle gourds in Turkey. Genet. Resour. Crop Evol. 62, 321–333. doi: 10.1007/s10722-015-0224-8
Han, J. S., Kim, C. K., Park, S. H., Hirschi, K. D., and Mok, I. (2005). Agrobacterium-mediated transformation of bottle gourd (Lagenaria siceraria Standl.). Plant. Cell Rep. 23, 692–698. doi: 10.1007/s00299-004-0874-z
Han, J. S., Oh, D. G., Mok, I. G., Park, H. G., and Kim, C.K. (2004). Efficient plant regeneration from cotyledon explants of bottle gourd (Lagenaria siceraria Standl.) Plant. Cell. Rep. 23, 291–296. doi: 10.1007/s00299-004-0846-3
Han, J. S., Park, K. I., Jeon, S. M., Park, S., Naing, A. H., and Kim, C. K. (2015), Assessments of salt tolerance in a bottle gourd line expressing the Arabidopsis H+-pyrophosphatase AVP1 gene in a watermelon plant grafted onto a transgenic bottle gourd rootstock. Plant Breed. 134, 233–238. doi: 10.1111/pbr.12253
Han, J. S., Park, S., and Shigaki, T. (2009). Improved watermelon quality using bottle gourd rootstock expressing a Ca2+/H+ antiporter. Mol. Breed. 24, 201–211. doi: 10.1007/s11032-009-9284-9
Hart, T. G. B. (2011). The significance of African vegetables in ensuring food security for South Africa's rural poor. Agric. Human Val. 28, 321–333. doi: 10.1007/s10460-010-9256-z
Hassan, L. G., Sani, N. A., Dangoggo, S. M., and Ladan, M. J. (2008). Nutritional value of bottle gourd (Lagenaria siceraria) seeds. Global J. Pure Appl. Sci. 14, 301–306. doi: 10.4314/gjpas.v14i3.16812
Ho, C. H., Ho, M. G., Ho, S., and Ho, H. H. (2014). Bitter bottle gourd (Lagenaria siceraria) toxicity. J. Emerg. Med. 46, 772–775. doi: 10.1016/j.jemermed.2013.08.106
Huang, Y., Zhao, L., Kong, Q., Fie, C., Mengliang, N., Xie, J., et al. (2016). Comprehensive mineral nutrition analysis of watermelon grafted onto two different rootstocks. Hortic. Plant J. 2, 105–113. doi: 10.1016/j.hpj.2016.06.003
Huh, Y. C., Lee, W. M., Ko, H. C., Park, D. K., and Lee, H. J. (2012). “Development of Fusarium resistant F1 hybrids of bottle gourd (Lagenaria siceraria Standl.) for watermelon rootstock,” in Proceedings of the Xth EUCARPIA Meeting on Genetics and Breeding of Cucurbitaceae. Antalya (Turkey), 74–80.
Janaranjani, K. G., Kanthaswamy, V., and Kumar, R. (2016). Heterosis, combining ability, and character association in bottle gourd for yield attributes. Int. J. Veg. Sci. 22, 490–515. doi: 10.1080/19315260.2015.1084967
Jeffrey, C. (1976). “Cucurbitaceae,” in Flora of Tropical East Africa. Crown Agents for Oversea Governments and Administrations, eds E. Milne-Redhead and R. M. Polhill (London: Crown Agents), 1–157.
Joobeur, T., King, J. J., Nolin, S. J., Thomas, C. E., and Dean, R. A. (2004). The Fusarium wilt resistance locus Fom-2 of melon contains a single resistance gene with complex features. Plant J. 39, 283–297. doi: 10.1111/j.1365-313X.2004.02134.x
Kaylan, R. A. O., Kaylan, B. S., Tomar, B., Singh, B., and Aher, B. (2016). Morphological characterization of parental lines and cultivated genotypes of bottle gourd (Lagenaria siceraria). Ind. J. Agric. Sci. 86, 65–70.
Keinath, A. P., and Hassell, R. L. (2014). Control of Fusarium wilt of watermelon by drafting onto bottle gourd or interspecific hybrid squash despite colonization of rootstocks by Fusarium. Plant Dis. 98, 255–266. doi: 10.1094/PDIS-01-13-0100-RE
Koffi, K. K., Anzera, G. K., Malice, M., Dje, Y., Bertin, P., Baudoin, J. P., et al. (2009). Morphological and allozyme variation in a collection of Lagenaria siceraria (Molina) Standl. from Cote D'Ivoire. Biotechnol. Agron. Soc. Environ. 13, 257–270.
Kohli, U. K., and Vikram, A. (2005). Hybrid cucumber. J. New Seeds. 6, 375–380. doi: 10.1300/J153v06n04_04
Kouassi, A. B., Kouassi, K. B. A., Sylla, Z., Plazas, M., Fonseka, R. M., Kouassi, A., et al. (2021). Genetic parameters of drought tolerance for agromorphological traits in eggplant, wild relatives, and interspecific hybrids. Crop Sci. 61, 55–68. doi: 10.1002/csc2.20250
Kousik, C. S., Ikerd, J. L., Mandal, M. K., Adkins, S., Webster, C. G., and Turechek, W. W. (2018). Powdery mildew–resistant bottle gourd germplasm lines: USVL351-PMR and USVL482-PMR. HortScience 53, 1224–1227. doi: 10.21273/HORTSCI13067-18
Kousik, C. S., Levi, A., Ling, K., and Wechter, W. P. (2008). Potential sources of resistance to Cucurbit powdery mildew in U.S. plant introductions of bottle gourd. HortScience 43, 1359–1364. doi: 10.21273/HORTSCI.43.5.1359
Ku, J. M., Hong, S. H., Kim, H. I., Lim, Y. S., Lee, S. J., Kim, M., et al. (2017). Cucurbitacin D exhibits its anti-cancer effect in human breast cancer cells by inhibiting stats and ATk signaling. Eur. J. Inflam. 16, 1–9. doi: 10.1177/1721727X17751809
Kushwaha, M. L., and Ram, H. H. (1996). Vr, Wr grphical analysis in bottle gourd. Veg. Sci. 23, 162–165.
Ling, K. S., and Levi, A. (2007). Sources of resistance to zucchini yellow mosaic virus in Lagenaria siceraria germplasm. HortScience 42, 1124–1126. doi: 10.21273/HORTSCI.42.5.1124
Ling, K. S., and Levi, A. (2013). Development and field evaluation of multiple virus-resistance bottle gourd (Lagenaria siceraria). Plant Dis. 97, 1057–1062. doi: 10.1094/PDIS-07-12-0639-RE
Liu, L., Gu, Q., Ijaz, R., Zhang, J., and Ye, Z. (2016). Generation of transgenic watermelon resistance to Cucumber mosaic virus facilitated by an effective Agrobacterium-mediated transformation method. Sci. Hortic. 205, 32–38. doi: 10.1016/j.scienta.2016.04.013
Ma, J., Zi Jiang, Y., Shi, H., Mi, C., Li, J., Xing Nan, J., et al. (2014). Cucurbitacin B inhibits the translational expression of hypoxia-inducible factor-1α. Eur. J. Pharmac. 723, 46–54. doi: 10.1016/j.ejphar.2013.12.005
Maheshwari, S. K., Choudhary, B. R., Singh, D., Sharma, B. D., and Sharma, S. K. (2015). Evaluation of resistance in different varieties/genotypes of bottle gourd (Lagenaria siceraria) against Cercospora leaf spot under field conditions. Ind. J. Agric. Sci. 85, 1269–1272.
Marzouk, B., Mahjoub, M. A., Bouraoui, A., Fenina, A., Auri, M., and Marzouk, Z. (2012). Anti-inflammatory and analgesic activities of a new cucurbitacin isolated from Citrullus colocynthis seeds. Med. Chem. Res. 22, 3984–3990. doi: 10.1007/s00044-012-0406-2
Mashilo, J., Odindo, A., Shimelis, H., Musenge, P., Tesfay, S. Z., and Magwaza, L. S. (2018). Photosynthetic response of bottle gourd [Lagenaria siceraria (Molina) Standl.] to drought stress; relationship between cucurbitacins accumulation and drought tolerance. Sci. Hort. 231, 133–143. doi: 10.1016/j.scienta.2017.12.027
Mashilo, J., Shimelis, H., and Odindo, A. (2015). Genetic diversity of bottle gourd (Lagenaria siceraria (Molina) Standl.) landraces of South Africa assessed by morphological traits and simple sequence repeat markers. SA J. Plant Soil. 33, 113–124. doi: 10.1080/02571862.2015.1090024
Mashilo, J., Shimelis, H., and Odindo, A. (2016a). Yield-based selection indices for drought tolerance evaluation in selected bottle gourd [Lagenaria siceraria (Molina) Standl.] landraces. Acta Agric. Scand. Section B – Soil and Plant Sci. 67, 43–50. doi: 10.1080/09064710.2016.1215518
Mashilo, J., Shimelis, H., and Odindo, A. (2016b). Correlation and path coefficient analysis of qualitative and quantitative traits in selected bottle gourd landraces. Acta Agric. Scand. Sec. B – Soil Plant Sci. 66, 558–569. doi: 10.1080/09064710.2016.1197965
Mashilo, J., Shimelis, H., and Odindo, A. (2017a). Drought tolerance of selected bottle gourd [Lagenaria siceraria (Molina) Standl.] landraces assessed by leaf gas exchange and photosynthetic efficiency. Plant Physiol. Biochem. 120, 75–87. doi: 10.1016/j.plaphy.2017.09.022
Mashilo, J., Shimelis, H., and Odindo, A. (2017b). Phenotypic and genotypic characterization of bottle gourd [Lagenaria siceraria (Molina) Standl.] and implications for breeding: a review. Sci. Hort. 222, 136–144. doi: 10.1016/j.scienta.2017.05.020
Mashilo, J., Shimelis, H., Odindo, A., and Amelework, B. (2016c). Genetic diversity of South African bottle gourd [Lagenaria siceraria (Molina) Standl.] landraces revealed by simple sequence repeat markers. Sci. Hort. 51, 120–126. doi: 10.21273/HORTSCI.51.2.120
Mashilo, J., Shimelis, H., Odindo, A., and Amelework, B. (2017c). Genetic differentiation of bottle gourd [Lagenaria siceraria (Molina) Standl.] landraces assessed by fruit qualitative traits and simple sequence repeat markers. Sci. Hort. 216, 1–11. doi: 10.1016/j.scienta.2016.12.022
Mladenović, E., Berenji, J., Ognjanov, V., Ljubojević, M., and Cukanović, J. (2012). Genetic variability of bottle gourd Lagenaria siceraria (Mol.) Standley and its morphological characterization by multivariate analysis. Arch. Biol. Sci 64:573–583. doi: 10.2298/ABS1202573M
Modgil, M., Modgil, R., and Kumar, R. (2004). Carbohydrate and mineral content of Chyote (Sechium edule) and bottle gourd (Lagenaria Siceraria). J. Human Ecol. 15, 157–159. doi: 10.1080/09709274.2004.11905683
Montero-Pau, J., Blanca, J., Esteras, C., Martìnez-Pèrez, E. M., Gómez, P., Monforte, A. J., et al. (2017). An SNP-based saturated genetic map and QTL analysis of fruit-related traits in Zucchini using genotyping-by-sequencing. BMC Genomics 11, 86–94. doi: 10.1186/s12864-016-3439-y
Montes-Hernandez, S., and Eguiarte, L. E. (2002). Genetic structure and indirect estimates of gene flow in three taxa of Cucurbita (Cucurbitaceae) in western Mexico. Amer. J. Bot. 89, 1156–1163. doi: 10.3732/ajb.89.7.1156
Morimoto, Y., Gikungu, M., and Maundu, P. (2004). Pollinators of bottle gourd (Lagenaria siceraria) observed in Kenya. Int. J. Trop. Insect Sci 24, 79–86. doi: 10.1079/IJT20046
Morimoto, Y., Maundu, P., Fujimaki, H., and Morishima, H. (2005). Diversity of landraces of the white-flowered gourd (Lagenaria siceraria) and its wild relatives in Kenya: fruit and seed morphology. Genet. Resour. Crop Evol. 52, 737–747. doi: 10.1007/s10722-004-6119-8
Morimoto, Y., Maundu, P., and Kawase, M. (2006). RAPD polymorphism of the white-flowered gourd (Lagenaria siceraria (Molina) Standl. landraces and its wild relatives in Kenya. Genet. Resour. Crop Evol. 53:963–974. doi: 10.1007/s10722-004-7070-4
Morimoto, Y., and Mvere, B. (2004). “Lagenaria siceraria,” in Vegetables Plant Resources of Tropical Africa 2, eds G. J. H. Grubben and O. A. Denton (Wageningen/Leiden: Backhuys Publishers/CTA), 353–358.
Narayan, R., Singh, S. P., Sharma, D. K., and Rastogi, K. B. (1996). Genetic variability and selection parameters in bottle gourd. Ind. J. Hort. 53, 53–58.
Nisini, T. P., Colla, G., Granati, E., Temperini, O., Crinó, P., and Saccardo, F. (2002). Rootstock resistance to Fusarium wilt and effect on fruit yield and quality of two muskmelon cultivars. Sci. Hort. 93, 281–288. doi: 10.1016/S0304-4238(01)00335-1
Ogunbusola, E. M. (2018). Nutritional and antinutritional composition of calabash and bottle gourd seed flours (var Lagenaria siceraria). J. Culinary Sci. Technol. 16, 326–335. doi: 10.1080/15428052.2017.1390518
Ogunbusola, M. E., Fagbemi, T. N., and Osundahunsi, O. F. (2010). Amino acid composition of Lagenaria siceraria seed flour and protein fractions. J. Food Sci. Technol. 47, 656–661. doi: 10.1007/s13197-010-0111-4
Ojiako, O. A., and Igwe, C. U. (2007). Nutritional and anti-nutritional compositions of Cleome rutidosperma, Lagenaria siceraria, and Cucurbita maxima seeds from Nigeria. J. Med. Food. 10, 735–738. doi: 10.1089/jmf.2007.625
Pal, S., Rao, S. E., Hebbar, S. S., Sriram, S., Pitchaimuthu, M., and Rao, K. V. (2020). Assessment of Fusarium wilt resistant Citrullus sp. rootstocks for yield and quality traits of grafted watermelon. Sci. Hort. 272:109497. doi: 10.1016/j.scienta.2020.109497
Park, M., Han, J., and Ahn, Y. (2014). Ectopic expression of Arabidopsis H+-pyrophosphatase AVP1 enhances drought resistance in bottle gourd (Lagenaria siceraria Standl.). Plant. Cell. Tiss. Organ Cult. 118, 383–389. doi: 10.1007/s11240-014-0490-8
Park, S., Lee, J., and Jegal, S. (2005). Transgenic watermelon rootstock resistant to CGMMV (cucumber green mottle mosaic virus) infection. Plant. Cell. Rep. 24, 350–356. doi: 10.1007/s00299-005-0946-8
Provvidenti, R. (1981). Sources of resistance to viruses in Lagenaria siceraria. Cucurbit Genet. Coop. Rep. 4, 38–39.
Provvidenti, R. (1995). A multi-viral resistant cultivar of bottle gourd (Lagenaria siceraria) from Taiwan. Cucurbit Genet. Coop. Rep. 18, 65–67.
Provvidenti, R., and Gonsalves, D. (1984). Occurrence of zucchini yellow mosaic virus in Cucurbits from Connecticut, New York, Florida and California. Plant Dis. 68, 443–446. doi: 10.1094/PD-69-443
Puri, R., Sud, R., Khaliq, A., Kumar, M., and Jain, S. (2011). Gastrointestinal toxicity due to bitter bottle gourd (Lagenaria siceraria) –a report of 15 cases. Ind. J. Gastroenterol. 30, 233–236. doi: 10.1007/s12664-011-0110-z
Qi, J., Liu, X., Shen, D., Miao, H., Xie, B., Li, X., et al. (2013). A genomic variation map provides insights into the genetic basis of cucumber domestication and diversity. Nature Genet. 45, 1510–1515. doi: 10.1038/ng.2801
Quamruzzaman, A., Salim, M., Akhter, L., Mazed, K., and Chowdhury, M. (2019). Genetic architecture of yield in bottle gourd (Lagenaria siceraria (Mol.) Standl.). Agric. Sci. 10, 567–576. doi: 10.4236/as.2019.104045
Quamruzzaman, A., Salim, M., Akhter, L., Rahman, M., and Chowdhury, M. (2020). Heterosis, combining ability and gene action for yield in bottle gourd. Amer. J. Plant Sci. 11, 642–652. doi: 10.4236/ajps.2020.115048
Quamruzzaman, A. K. M., Rashid, M. A., Masud, M. A. T., and Uddin, M. N. (2009). Heterosis in bottle gourd. J. Agric. Res. 3, 465–472. doi: 10.3329/bjar.v34i3.3973
Rai, M., Pandey, S., and Kumar, S. (2008). “Cucurbit research in India: a retrospect,” in Proceedings of the IXth Eucarpia Meeting on Genetics and Breeding of Cucurbitaceae, (Avignon) 285–294.
Rao, P. G., Behera, T. K., Gaikwad, A. B., Munshi, A. D., Jat, G. S., and Boopalakrishnan, G. (2018). Mapping and QTL analysis of gynoecy and earliness in bitter gourd (Momordica charantia L.) using genotyping-by-sequencing (GBS) technology. Front. Plant Sci. 9:1555. doi: 10.3389/fpls.2018.01555
Ren, Y., Zhao, H., Kou, Q., Jiang, J., Guo, S., Zhang, H., et al. (2012). A high resolution genetic map anchoring scaffolds of the sequenced watermelon genome. PLoS ONE 7:e29453. doi: 10.1371/journal.pone.0029453
Rhodes, B., and Zhang, X. (2000). Hybrid seed production in watermelon. J. New Seeds. 1, 69–88. doi: 10.1300/J153v01n03_03
Robinson, R. W. (2000). Rationale and methods for producing hybrid cucurbit seed. J. New Seeds. 1, 1–47. doi: 10.1300/J153v01n03_01
Said, P. P., Pradhan, R. C., and Rai, B. N. (2014). A green separation of Lagenaria siceraria seed oil. Ind. Crops Prod. 52, 796–800. doi: 10.1016/j.indcrop.2013.12.009
Samadia, D. K. (2002). Performance of bottle gourd genotype under hot arid environment. Ind. J. Hort. 59, 167–170.
Sarao, N. K., Pathak, M., Kaur, N., and Kaur, K. (2014). Microsatellite-based DNA fingerprinting and genetic diversity of bottle gourd genotypes. Plant Genetic Resour. 12, 1–4. doi: 10.1017/S1479262113000385
Sidhu, N. S., Pruthi, G., Singh, S., Bishnoi, R., and Deepak, S. (2020). Genome-wide identification and analysis of GRAS transcription factors in the bottle gourd genome. Sci. Rep. 10:14338. doi: 10.1038/s41598-020-71240-2
Singh, D. K., Padiyar, S., Choudhary, H., Tiwari, A., and Ram, H. H. (2010). Utilization of novel genotype of bottle gourd for identification of hybrid seed with RAPD marker. Acta Hort. 871, 491–498. doi: 10.17660/ActaHortic.2010.871.68
Sithole, J. N., Modi, A. T., and Pillay, K. (2015). An assessment of minerals and protein contents in selected South African bottle gourd landraces [Lagenaria siceraria (Mol.) Standl.]. J. Human Ecol. 51, 279–286. doi: 10.1080/09709274.2015.11906923
Sivaraj, N., and Pandravada, S. R. (2005). Morphological diversity for fruit characters in bottle gourd germplasm from tribal pockets of Telangana region of Andhra Pradesh, India. Asian Agricultural-Hist. 9, 305–310.
Song, G., Prieto, H., and Orbovic, V. (2019). Agrobacterium-mediated transformation of tree fruit crops: methods, progress, and challenges. Front. Plant Sci. 10:226. doi: 10.3389/fpls.2019.00226
Song, H., Huang, Y., and Gu, B. (2020). QTL-Seq identifies quantitative trait loci of relative electrical conductivity associated with heat tolerance in bottle gourd (Lagenaria siceraria). PLoS ONE 15:e0227663. doi: 10.1371/journal.pone.0227663
Srivastava, D., Khan, N. A., Shamim, M. D., Yadav, P., Pandey, P., and Singh, K. N. (2014). Assessment of the genetic diversity in bottle gourd (Lagenaria siceraria [Molina] Standl.) using SDS-PAGE and RAPD markers. Nat. Acad. Sci. Lett. 37, 155–161. doi: 10.1007/s40009-013-0207-2
Takahashi, Y., Iseki, K., Kitazawa, K., Muto, C., Somta, P., Irie, K., et al. (2015). A homoploid hybrid between wild Vigna species found in a limestone Karst. Front. Plant Sci. 6:1050. doi: 10.3389/fpls.2015.01050
Thies, J. A., Ariss, J. J., Hassell, R. L., Olson, S., Kousik, C. S., and Levi, A. (2010). Grafting for management of southern root-knot nematode, Meloidogyne incognita, in watermelon. Plant Dis. 94, 1195–1199. doi: 10.1094/PDIS-09-09-0640
Tian, S., Jiang, L., Gao, Q., Zhang, J., Zong, M., Zhang, H., et al. (2017). Efficient CRISPR/Cas9-based gene knockout in watermelon. Plant Cell. Rep. 36, 399–406. doi: 10.1007/s00299-016-2089-5
Wang, Y., Xu, P., Wu, X., Wang, B., Huang, Y., Hu, Y., et al. (2018). Gourdbase: a genome-cantered multi-omics database for the bottle gourd (Lagenaria siceraria), an economically important cucurbit crop. Sci. Rep. 10, 1038–1044. doi: 10.1038/s41598-018-22007-3
Wang, Z., and Xiang, C. (2013). Genetic mapping of QTLs for horticulture traits in a F2-3 population of bitter gourd (Momordica charantia L.). Euphytica. 193, 235–250. doi: 10.1007/s10681-013-0932-0
Whitaker, T. W. (1971). “Endemism and pre-columbian migration of the bottle gourd, Lagenaria siceraria (Mol.) Standl,” in Man Across the Sea, eds J. C. Kelley and C. W. Pennington (Austin, TX: University of Texas Press), 320–337.
Wu, H., He, X., Gong, H., Luo, S., Li, M., Chen, J., et al. (2016). Genetic linkage map construction and QTL analysis of two interspecific reproductive isolation traits in sponge gourd. Front. Plant Sci. 7:980. doi: 10.3389/fpls.2016.00980
Wu, S., Shamimuzzaman, M. D., Su, H., Salse, J., Sui, X., Wilder, A., et al. (2017c). The bottle gourd genome provides insights into Cucurbitaceae evolution and facilitates mapping of a Papaya ring-spot virus resistant locus. Plant J. 92, 963–975. doi: 10.1111/tpj.13722
Wu, X., Wu, X., Wang, Y., Wang, B., Lu, Z., Xu, P., et al. (2019). Molecular genetic mapping of two complementary genes underpinning fruit bitterness in bottle gourd [Lagenaria siceraria (Mol,) Standl.]. Front. Plant Sci. 10:1493. doi: 10.3389/fpls.2019.01493
Wu, X., Xu, P., Wu, X., Wang, B., Lu, Z., and Li, G. (2017a). Genome-wide association analysis of free glutamate content, a key factor conferring umami taste in the bottle gourd [Lagenaria siceraria (Mol.) Standl.]. Sci. Hortic. 225, 795–801. doi: 10.1016/j.scienta.2017.08.015
Wu, X., Xu, P., Wu, X., Wang, B., Lu, Z., and Li, G. (2017b). Development of insertion and deletion markers for bottle gourd based on restriction site-associated DNA sequencing data. Hortic. Plant J. 3, 13–16. doi: 10.1016/j.hpj.2017.01.011
Xu, P., Wu, X., Wang, B., Liu, Y., Ehlers, J. D., Wang, S., et al. (2011). Partial sequencing of the bottle gourd genome reveals markers useful for phylogenetic analysis and breeding. BMC Genomics 12:467. doi: 10.1186/1471-2164-12-467
Xu, P., Xu, S., Wu, X., Tao, Y., Wang, B., Wang, S., et al. (2014). Population genomic analyses from low-coverage RAD-Seq data: a case study on the non-model cucurbit bottle gourd. Plant J. 77, 430–442. doi: 10.1111/tpj.12370
Yang, Y., Lu, X., Yan, X., Lin, B., Sun, J., Guo, S., et al. (2013). Bottle gourd rootstock-grafting affects nitrogen metabolism in NaCl-stressed watermelon leaves and enhances short-term salt tolerance. J. Plant Physiol. 170, 653–661. doi: 10.1016/j.jplph.2012.12.013
Yang, Y., Wang, L., Tian, J., Sun, J., He, L., Guo, S., et al. (2012). Proteomic study participating the enhancement of growth and salt tolerance of bottle gourd rootstock-grafted watermelon seedlings. Plant Physiol. Biochem. 58, 54–65. doi: 10.1016/j.plaphy.2012.05.026
Yang, Y., Yu, L., Wang, L., and Guo, S. (2015). Bottle gourd rootstock-grafting promotes photosynthesis by regulating the stomatal and non-stomatal performance in leaves of watermelon seedlings under NaCl stress. J. Plant Physiol. 187, 50–58. doi: 10.1016/j.jplph.2015.07.013
Yanwei, L., Ying, W., Xinyi, W., Jian, W., Xiaohua, W., Baogen, W., et al. (2021). Novel genomic regions of Fusarium wilt resistance in bottle gourd [Lagenaria siceraria (Mol.) Standl.] discovered in genome-wide association study. Front. Plant Sci. 12:650157. doi: 10.3389/fpls.2021.650157
Yao, K. A. G., Koffi, K. K., Ondo-Azi, S. A., Baudoin, J. P., and Zoro, B. I. A. (2015). Seed yield component identification and analysis for exploiting recombinative heterosis in bottle gourd. Int. J. Veg. Sci. 21, 441–453. doi: 10.1080/19315260.2014.895791
Yetişir, H., Denli, N., Ata, A., Ula,ş, A., Pinar, H., Kirnak, H., et al. (2016). Determination of salt tolerance potential of Turkish bottle gourd (Lagenaria siceraria) germplasm. Turkish J. Agric. Forestry. 31, 381–388.
Yetişir, H., and Karaca, F. (2018). Assessment of rooting capacity and rootstock potential of some Turkish bottle gourd (Lagenaria siceraria) accessions used as rootstocks for watermelon [Citrullus lanatus (Thunb.) Matsum. Nakai]. Asian Res. J. Agric. 9, 1–10. doi: 10.9734/ARJA/2018/41653
Yetişir, H., Kurt, S., Sari, N., and Tok, F. M. (2007). Rootstock potential of Turkish Lagenaria siceraria germplasm for watermelon: plant growth graft compatibility, and resistance to Fusarium. Turkish J. Agric. Forestry. 31, 381–388.
Yetişir, H., Sakar, M., and Serçe, S. (2008). Collection and morphological characterization of Lagenaria siceraria germplasm from Mediterranean region of Turkey. Genet. Resour. Crop Evol. 55, 1257–1266. doi: 10.1007/s10722-008-9325-y
Yetişir, H., and Sari, N. (2003). Effect of different rootstock on plant growth, yield and quality of watermelon. Austr. J. Exp. Agric. 43, 1269–1274. doi: 10.1071/EA02095
Yetişir, H., Sari, N., and Yücel, S. (2003). Rootstock resistance to Fusarium wilt and effect of watermelon fruit yield and quality. Phytoparasitica. 31, 163–169. doi: 10.1007/BF02980786
Yildiz, M., Cuevas, H. E., Sensory, S., Erdinc, C., and Baloch, F. S. (2015). Transferability of cucurbita SSR markers for genetic diversity assessment of Turkish bottle gourd (Lagenaria siceraria) genetic resources. Biochem. Syst. Ecol. 59, 45–53. doi: 10.1016/j.bse.2015.01.006
Yuan, R. Q., Qian, L., Yun, W. J., Cui, X. H., Lv, G. X., Tang, W. Q., et al. (2019). Cucurbitacins extracted from Cucumis melo L. (CuEC) exert a hypotensive effect via regulating vascular tone. Hypertension Res. 42, 1152–1161. doi: 10.1038/s41440-019-0258-y
Zhang, C., Zheng, H., Wu, X., Xu, H., Han, K., Peng, J., et al. (2018). Genomic-wide identification of new reference genes for RT-qPCR normalization in CGMMV-infected Lagenaria siceraria. Front. Plant Sci. 11:177. doi: 10.3389/fpls.2020.00177
Zhang, H., Tan, J., Zhang, M., Huang, S., and Chen, X. (2020). Comparative transcriptomic analysis of two bottle gourd accessions differing in fruit size. Genes 11:359. doi: 10.3390/genes11040359
Zhang, M., Liu, Q., Yang, X., Xu, J., Liu, G., Yao, X. , et al. (2020). CRISPR/Cas9-mediated mutagenesis of Clpsk1 in watermelon to confer resistance to Fusarium oxysporum f.sp. niveum. Plant Cell Rep. 39, 589–595. doi: 10.1007/s00299-020-02516-0
Zhang, S. P., Miao, H., Yang, Y. H., Xie, B. Y., Wang, Y., and Gu, X. F. (2014). A major quantitative trait locus conferring resistance to Fusarium wilt was detected in cucumber by using recombinant inbred lines. Mol. Breed. 34, 1805–1815. doi: 10.1007/s11032-014-0140-1
Zhong, Y., Chen, C., Nawaz, M. A., Jiao, Y., Zheng, Z., Shi, X., et al. (2018). Using rootstock to increase watermelon fruit yield and quality at low potassium supply: a comprehensive analysis from agronomic, physiological and transcriptional perspective. Sci. Hort. 241, 144–151. doi: 10.1016/j.scienta.2018.06.091
Keywords: abiotic stress, agronomic traits, biotic stress, bottle gourd, horticultural traits, watermelon
Citation: Mkhize P, Mashilo J and Shimelis H (2021) Progress on Genetic Improvement and Analysis of Bottle Gourd [Lagenaria siceraria (Molina) Standl.] for Agronomic Traits, Nutrient Compositions, and Stress Tolerance: A Review. Front. Sustain. Food Syst. 5:683635. doi: 10.3389/fsufs.2021.683635
Received: 21 March 2021; Accepted: 15 June 2021;
Published: 19 July 2021.
Edited by:
Matteo Balderacchi, Independent Researcher, Piacenza, ItalyReviewed by:
Biplab Bhowmick, Scottish Church College, IndiaHanno Schaefer, Technical University of Munich, Germany
Copyright © 2021 Mkhize, Mashilo and Shimelis. This is an open-access article distributed under the terms of the Creative Commons Attribution License (CC BY). The use, distribution or reproduction in other forums is permitted, provided the original author(s) and the copyright owner(s) are credited and that the original publication in this journal is cited, in accordance with accepted academic practice. No use, distribution or reproduction is permitted which does not comply with these terms.
*Correspondence: Jacob Mashilo, amFjb2JtYXNoaWxvQHlhaG9vLmNvbQ==