- Department of Agronomy, Universidade Federal de Viçosa, Viçosa, Brazil
Nitrogen, the most demanded nutrient by coffee plants, has a rate of recovery from the soil of about 50%. Because of that high doses of nitrogenous fertilizers are used to reach high production, and consequently high amounts of N are lost to the environment. Knowing the kinetic parameters of nitrate (NO3−) absorption over the fruit development cycle is important as a mean of achieve more adjusted fertilizer doses and better recovery rates for the N applied as fertilizers. This study aimed determining the kinetic parameters of NO3− absorption in different development stages of fruits from adult coffee plants. The kinetic parameters Vmax and Km were determined in a low production year, at the pinhead (PH), rapid expansion (RE), grain filling (GF), and maturation (MT) stages. One month before each kinetics assay, lateral roots of eight plants were excavated and wrapped into non-woven fabrics grow cylinders filled in with vermiculite to produce absorbent roots. On the assay day, the roots were washed and immersed into a container with 1 L of 90 μmol L−1 NO3− solution. Sampling began one and a half hours after that, and was taken every hour over 7 h. Data on NO3− depletion were used to calculate the absorption kinetic parameters Vmax and Km. In a low production year the Vmax ranged from 0.14 to 0.72 μmol g−1 h−1 in a root fresh matter basis and Km from 6.47 to 50.31 μmol L−1. The Vmax values were highest at the PH and MT stages; the lowest absorption rate was recorded at GF and Km was lowest at RE. As at the RE stage of fruits Vmax shows a positive correlation with grain production, adequate nitrogen availability must be ensured before this phase to not to affect coming coffee production.
Introduction
Nitrogen (N) is an important nutrient for plant growth and development. This nutrient participates of several components of the photosynthetic apparatus and enzymes involved in CO2 fixation, determining biomass production, and thereby is considered an important factor in crop productivity. Among the available forms of N, nitrate is absorbed in greatest amounts by plants. NO3− uptake can range from 0.1 to 5.0 mmol L−1 in cultivated soils (Bose and Srivastava, 2001). Nitrate (NO3−) is also the N form most absorbed by coffee roots (Miller et al., 2007; Reis et al., 2007). However, due to losses in the soil by volatilization and leaching, the recovery efficiency of nitrogen fertilizers is low, between 40 and 50% of the dose applied. The loss of N applied as urea (three top dressings a year) was reported to be 31.2% in coffee orchards located at the Lavras region, in Brazil (Dominghetti et al., 2016). In addition to the economic impact, these losses cause negative environmental impact due to gaseous N volatilization and contamination of waterways by leached NO3−.
Absorption mechanisms enable plants to adjust to environmental fluctuations in the concentrations of nutrients and their different ionic forms. In the case of N, transport proteins in the plasma membranes, which have different affinities for both nitrate and ammonium, mediate the absorption of nitrate and ammonium by roots. Plants developed a refined absorption system for nitrate with high-affinity transport system (HATS) that operate in low external concentrations (<0.5 mmol L−1) of the ion, whereas low-affinity system operate in high concentrations (>0.5 mmol L−1), thus ensuring the absorption of nitrate according to the ion availability in the substrate (Marschner, 2012; Wang et al., 2012).
Difficulties in quantifying N fractions in the soil have limited the nutrient recommendation based on estimates of this availability by chemical analysis and hindered reliable predictions of their effective availability to plants. Therefore, kinetic parameters of N uptake may provide information on the ability of plants to acquire NO3− at a given concentration in the substrate. Kinetic parameters also allow the plant to serve as a sensitive indicator of the fertilizer required to meet the nutritional demands, which can increase the agronomic efficiency of nitrogen fertilizers. In this sense, recent works have measured the kinetic absorption parameters as a mean of selecting rootstocks of peach, grapevine and pear more efficient in the absorption of nitrate-nitrogen and ammonium-nitrogen (Paula et al., 2018; Kulmann et al., 2020; Sete et al., 2020). Relatively to coffee-plant the estimated kinetic parameters of NO3− uptake in 9.5 month-old plants of eight coffee varieties grown in growth chambers were in average 3.47 μmol g−1 h−1 for Vmax and 236.3 μmol L−1 for Km. The varieties showed significant differences among them. “Catuaí Amarelo” presented high Vmax and low Km without or with water stress, meanwhile Mundo Novo although presenting low Vmax and low Km did not suffer alterations in these parameters when under water stress (Martinez et al., 2020).
Kinetic parameters have been traditionally estimated using hydroponic systems because of the ease of collecting root samples and depletion solution without major methodological complexities. However, few references are found on studies conducted with adult plants, and in field conditions the lack of information is even greater, despite these estimates could be more realistic.
Another important consideration is that root morphological characteristics like diameter, length, surface and volume showed to be highly related to kinetic parameters of N absorption (Kulmann et al., 2020), and these root characteristics are known for changing during coffee berry development (Magalhães, 2021).
When cultivated at full sun Coffea arabica presents biennial production cycle as a result of abundant flowering and of the drain force of developing grains (Cannell, 1985). In a high fruit production year the load of fruits impairs the vegetative growth of productive branches, in which the fruiting would occur in the following year, but this reduced vegetative growth is preceded by death of thin absorbent roots (Rena and Maestri, 1987). This way, a relationship between production and root growth parameters is expected. In fact Silva et al. (2020) verified positive correlation between Coffea canephora production and root length, surface and volume.
Considering these features of adult coffee plants, as an approach of the field conditions Pinto (2016) used a drip system to estimate the kinetic parameters of NO3− absorption at different phenological phases of coffee fruit development using 5-year-old trees of variety Catuaí Vermelho IAC 99 cultivated in vases containing sand, in a greenhouse. The author found that Km was highest at the stage of the first rapid expansion, intermediate at the pre-flowering and flowering-to-pinhead, and lowest at grain filling. Vmax was lowest at grain filling compared with the other stages.
The objective of this study was to estimate the kinetic parameters of NO3− absorption in 5 years old coffee plants at different phenological phases of fruit development, using methodology developed for field conditions.
Materials and Methods
Experimental Conditions
The study was carried out in the coffee experimental plantation of the Federal University of Viçosa (20° 45' 58” S latitude; 42° 52' 06” W; 676 m altitude). The soil of the area is classified as Red-Yellow Latosol (Jacomine, 2009). The climate of the region is of the type Cwa, according to the Köppen classification, hot and humid with dry cold winter and minimum temperatures below 10°C. Figure 1 shows the temperature and relative air humidity variations over the experimental period.
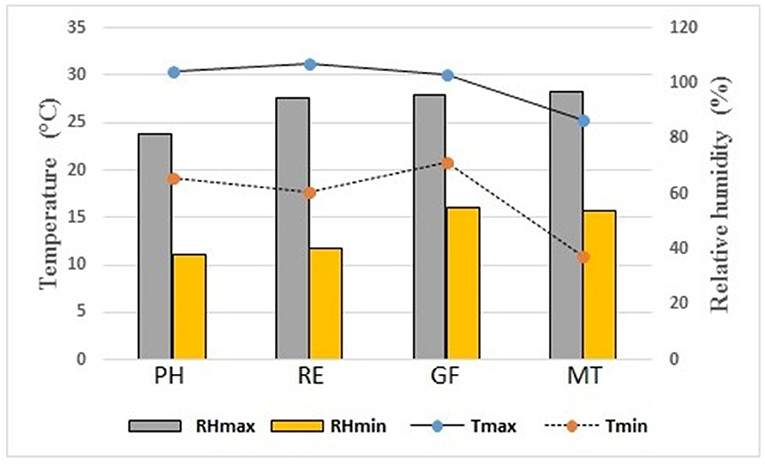
Figure 1. Daily relative humidity (RH), and daily temperature (T) over the absorption kinetic assays.
Five-year-old coffee trees of cultivar Catuaí Vermelho IAC 99, in the low production year of the biennial cycle, formed the stand in this study. The coffee was planted at the spacing 2 × 1 m in the rainfed system. The crop has been maintained with usual management practices, liming and fertilization according to Ribeiro et al. (1999), since planting. During the experimental phase, the plants were kept free from crop-weed competition through mechanical weeding. Pest and disease management was carried out according to the technical recommendations for coffee cultivation.
The experimental plot comprised 10 rows with 75 plants each, totaling 1,500 m2. Table 1 shows the results of the soil chemical analysis at the beginning of the experiment. The analysis was done according to the methods described by Defelipo and Ribeiro (1997) and Alvarez et al. (2000).
Before the beginning of the crop season, in August 2017, liming was carried out with 1.65 t/ha of dolomitic limestone (CaO 30% and MgO 10%) with 77% TRNP, according to Ribeiro et al. (1999). Fertilizers were applied at the outside edge of the crown by applying 100, 35, and 45 g/plant of ammonium sulfate, simple superphosphate and potassium chloride in early November, late December 2017 and, mid-February 2018. One cycle of irrigation was applied immediately after fertilization.
Nitrate Depletion Assays
At the end of the previous crop cycle, in July 2017, 32 trees were randomly selected for homogeneity in size, number of plagiotropic branches, leaves, and vigor. These plants were labeled to be used in four depletion assays that were conducted in the fruit phenological development stages pinhead (PH), rapid expansion (RE), grain filling (GF), and maturity (MT). Approximately 1 month before each sampling, 8 trees among the 32 selected were picked at random. Lateral roots near the trunk, in the 0–10 cm soil layer under the crown, were exposed by careful digging trenches (50 cm long, 20 cm wide and 20 cm deep). When the lateral root was reached, it was wrapped into a NWF (non-woven fabric), forming a root growth chamber (8 cm diameter and 30 cm long) filled with vermiculite.
The root growth chamber consisted of a 15 × 30 cm NWF rectangle placed under the root selected, which was surrounded by vermiculite, then wrapped in the shape of a cylinder, and tied in place with plastic string. The distal end of the root was cut at the base of the cylinder, while the proximal end remained attached to the plant. The trench was filled with the excavated soil surrounding the cylinder to support the root. The growth chamber remained below the soil surface until the depletion assays were performed. Afterwards, the soil surrounding the growth chamber was removed to expose the newly developed absorbent roots. The roots were placed into a plastic tray, washed with deionized water, and placed into urine-like collection bags filled with 1 L of the depletion solution attached to a portable aerator. The bags had an aperture on the top to introduce the roots. The apparatus was isolated from the underlying soil by polyethylene film to avoid contamination of the depletion solution (Figure 2).
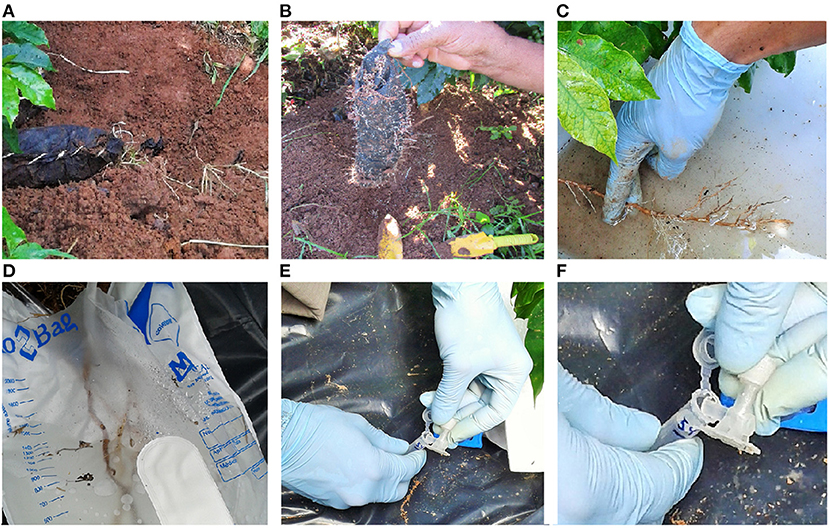
Figure 2. Exhaustion assay description: (A) Excavation, lateral permanent root identification, and packaging in substrate; (B,C) Withdrawal of substrate and washing of fine roots; (D) Packing of fine roots in a bag containing exhaustion solution; (E,F) Exhaustion solution sampling.
The depletion solution contained 90 μmol L−1 of NO3− in the form of KNO3. Absorption took 90 min to reach the steady state. Then, every hour, about 2 mL of the depletion solution were collected through the drain tap at the bottom of the bag, for 7 h (between 9:30 am and 4:30 pm). The samples were transferred into a previously weighed Eppendorf tube and quickly placed in a box with ice. At the end of the process, the final volume of the depletion solution was measured to determine the volume lost by transpiration. Fine roots (Ø ≤ 2.00 mm) were cut from the selected lateral root, washed, separated from the thick roots, and weighed in an analytical balance to determine fresh matter.
The main flowering of the coffee plants occurred on 10/9/2017 and the samplings were carried out on 11/16/17, 12/10/17, 03/14/18, and 5/11/18 at the PH, RE, GF, and MT stages, according to the duration of the phenological phases determined by Laviola et al. (2009) in this region. We choose sunny days to perform the four assays. The total radiation over the canopy during each assay was of 18,587.70; 22,032.00; 15,919.10, and 13,169.98 kJ/m2, respectively.
In the laboratory, the Eppendorf tubes were re-weighed to determine the exact volume of each sample and the nitrate concentrations in the aliquots were measured. The nitrate concentrations in the depletion solution were determined by the colorimetric method as described by Doane and Horwath (2003), using a Biochrom EZ 400 READ molecular absorption spectrophotometer, in the 540 nm wavelength.
Kinetic Parameters Determination
The volumes of depletion solution available to the plant at the sampling times were calculated using an electronic spreadsheet, taking into account the amounts removed by the samplings and the transpiration estimated. The water loss by transpiration was considered constant over the sampling period of 7 h.
The methodology of Claassen and Barber (1974) was used to calculate the kinetic parameters of absorption. The method relates the amount of the nutrient (obtained by multiplying the concentration by the volume of the depletion solution available to the plant at each sampling) to the sampling time. For each plant assayed, a function nutrient quantity × time was obtained, which was the basis for estimating the kinetic parameters. The mathematical-graphical method described by Ruiz (1985) was used to calculate Vmax and Km. The method divides the aforementioned function into a linear segment for calculating Vmax and another asymptotic segment for calculating Km. Vmax was estimated considering the fresh matter weight of absorbent roots, expressed as μmol g−1 h−1.
The values obtained for Vmax, Km were inserted in to the Michaelis -Menten equation to provide better explanation of the nitrate absorption process in RE, GF, and MT fruit development phases:
Where:
v0 = instantaneous velocity of NO3− absorption;
Vmax = maximum NO3− absorption velocity;
C = NO3− external concentration;
Km= NO3− concentration at which ½ Vmax is reached.
Fine Root Production per Plant and Bean Production per Plant
The production of absorbent roots by each plant was estimated at the GF and MT stages. For the amount of absorbent roots per tree, soil samples of known volume were collected from the 0 to 30 cm layer with a 7.5 cm diameter soil probe. Samples were collected from three sampling points inside the canopy projection area of each assayed tree. The samples were removed from the probe, placed in trays, and roots were separated, washed and weighed. The weight of roots with diameter bellow 2 mm present in the soil volume sampled was then extrapolated to the volume of the cylinder estimated by the average crown diameter to the 30 cm depth, in which about 90% of the absorbent roots of the coffee tree are concentrated (Inforzato and Junqueira, 1963, Motta et al., 2006; Ronchi et al., 2015; Silva et al., 2020; Magalhães, 2021).
After complete maturity, coffee cherries of each assayed plant were picked and weighed to determine their fresh matter weight.
Statistical Analysis
Although we have had assayed 32 plants, eight in each phenological stage of fruit development, not all results fit to a expected biological trend for nitrate absorption. For the exhaustion assays we had suitable results to two plants in the PH stage, four plants to the RE and MT stages and five plants to the GF stage. The kinetic parameter data were presented as descriptive statistics (mean ± standard deviation). In addition, Pearson's correlation coefficients were calculated between the kinetic parameters Vmax, Km, fresh weigh of roots, and fruit production.
Results
Kinetic Parameters of Nitrate Absorption
The Vmax kinetic parameter varied between 0.143 and 0.724 μmol g−1 h−1 and the Km varied between 6.476 and 50.316 μmol L−1. Both of them varied according to the phenological phases of fruit development (Table 2). The mean confidence interval at the maturity stage shows that Vmax was significantly higher to those at the rapid expansion and bean filling stages; however, it was statistically similar to the result observed at the pinhead stage.
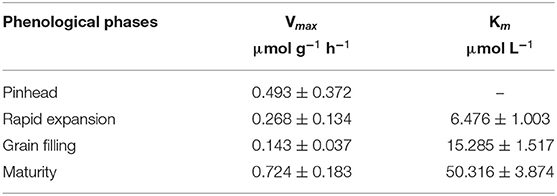
Table 2. Nitrate absorption kinetic parameters: maximum absorption velocity (Vmax) and Michaelis-Menten constant (Km) in four coffee-plant phenological phases.
It was not possible to determine Km for the pinhead stage in the depletion assays performed, because in this phase the depletion adjusted to a linear model. The values of Km for the other stages increased with the year round and the succession of the phases of fruit development. The lowest values were achieved at the rapid expansion stage and the highest values at maturation stage (Table 2).
The Michaelis -Menten equation applied to these data, showed that nitrate uptake exhibited saturation kinetics, as expected for the range of low external concentrations (Figure 3). The absorption rate up to the concentration of 20 μmol L−1 was higher at the RE stage than at the stages of GF and MT and was achieved with the lowest nitrate external concentrations due to its lowest Km value. Above 20 μmol L−1, the absorption rate was the highest at the maturation stage due to its highest Vmax value, despite its highest Km. At the GF stage, the nitrate absorption rate was the lowest among the stages evaluated, which was influenced to a greater extent by the lowest Vmax (Figure 3).
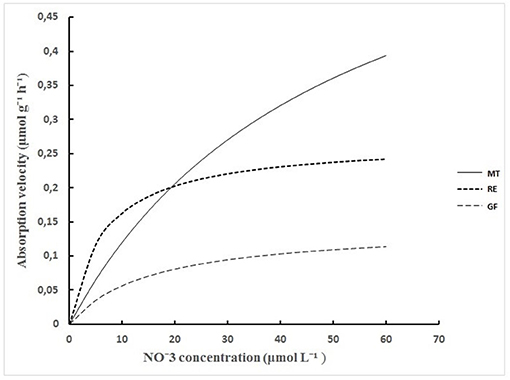
Figure 3. Nitrate absorption velocity by adult coffee trees (μmol g−1 h−1) as a variable of its concentration in the external solution (μmol L−1) in three phenological phases of fruit development: RE (rapid expansion), GF (grain filing), and MT (maturation).
Correlations Between Kinetic Parameters, Production of Fine Roots and Beans
In this study, the root system fresh matter of plants was evaluated at the GF and MT stages. The GF stage was selected because it shows great competition between fruits and vegetative organs for photoassimilates (Valarini et al., 2005; Laviola et al., 2006). Maturity was selected because of the lowering of the photoassimilates demand by fruits in this stage, allowing the recovery of root growth. At the GF stage, the plants presented, on average, four times less fresh matter of fine roots than in the MT stage (Figure 4).
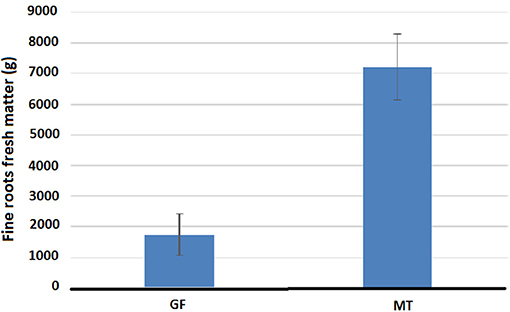
Figure 4. Fresh matter of fine roots of 5-year old coffee-trees in the phenological phase of grain filing and maturation of fruits.
The average cherry production was 10.51 t/ha, corresponding to 1.65 t/ha of coffee beans with moisture content of 11%. It is of note that coffee has a biennial production cycle and that during the study the crop was in a year of low production and without irrigation, which lead to relatively low average yields.
The fruit production of the trees (Prod) used in the depletion assays was correlated with the NO3− absorption velocity during the fruit rapid expansion stage (0.95 Pearson correlation coefficient). On the other hand, the Vmax of NO3− absorption obtained at the GF and MT showed a weak negative correlation with production. Vmax decreased at these later stages, suggesting a slightly lower NO3− uptake capacity of the most productive plants at bean filling. The correlation between Vmax and fresh root mass was also negative. A positive correlation, although not very high (0.57), was found between the fresh matter of fine roots at grain filling and production. Vmax, at maturity, had a negative correlation with low degree of association with Prod. Vmax at the grain filling stage had a negative correlation with root fresh matter. In contrast to the response at the GF stage, root fresh matter at the MT stage had a negative correlation with Prod (Table 3).
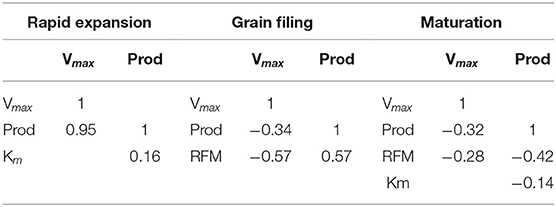
Table 3. Pearson's correlation coefficients between fruit production, root fresh matter and kinetic parameters.
Discussion
Kinetic Parameters of Nitrate Absorption
The range of values of the kinetic parameters observed in field conditions, between 0.143 and 0.724 μmol g−1 h−1 for Vmax and between 6.476 and 50.316 μmol L−1 for Km (Table 2) agrees with the results obtained by Pinto (2016) in greenhouse conditions. This author have worked with 60-month-old coffee plants of the same variety under no water stress, in a drip hydroponic system with sand substrate, and found values of Vmax, ranging between 0.34 and 0.83 μmol g−1 h−1. For the Km, this author found values between 120.48 and 264.98 μmol L−1, which were considerably higher than the results of the present work. These differences are likely to be due to different experimental conditions, especially the nitrate concentration in the depletion solution, the fresh matter of fine roots, and the environmental conditions.
The confidence interval of the mean at the maturity stage (Table 2) shows that Vmax was significantly higher in this phase than those at the rapid expansion and bean filling stages; however, it was statistically similar to the result observed at the pinhead stage. Epstein and Bloom (2006) state that Vmax is defined by the multiplication of the number of absorption sites (transporter proteins) per root unit and its operation velocity, whereas Li et al. (2013) state that Vmax refers to the maximum capacity of the root system to absorb a particular nutrient when all transporters are saturated, indicating maximum transport rate. It was seen, therefore, contrary to what was expected based on the demands of the fruit and contrary to expectations that the maximum capacity of nitrate uptake occurs at the pinhead and maturity stages, either by increasing the number of the transporters or by increasing its operation velocity.
In agreement to our results Neto et al. (2014) found that the NO3− leaf content increased from the pre-anthesis to the pinhead stage with a subsequent drop at the grain filling stage. This drop is attributed to N remobilization to support fruit growth. However, the authors observed an increase in leaf NO3− levels at the beginning of fruit ripening and high Nitrate Reductase activity at pre-anthesis. Similarly, Carelli et al. (1989) found high Nitrate Reductase activity at pre-anthesis and at the end of fruit development, indicating the intense metabolism and demand for N at these stages. Carvajal et al. (1969) and Reis et al. (2009) also found in mature coffee plants higher levels of nitrate absorption at pre-anthesis and at the beginning of fruit ripening.
Our observation of the highest Vmax values at the pinhead and maturity stages is quite consistent with the foliar concentrations and patterns of the Nitrate reductase activity found in the studies mentioned in the previous paragraph. This suggests that Vmax responded to the nitrogen demands at these stages leading to greater or lower nitrate uptake and that the mechanisms operating the absorption manage to modulate this parameter as a function of the different nutrient demands signalized by the phenological phases. Additionally, Laviola et al. (2006) observed a reduction in the leaf N content of three varieties of Coffea arabica between the phases of flowering and suspended growth, followed by a small recovery in this phase and later decline during the grain-filling/maturity. Prezotti and Bragança (2013), on the other hand, found that nitrogen contents in both the leaf and the root of Coffea canephora decreased with time after flowering, indicating occurrence of nitrogen remobilization during fruit development and suggesting a greatest uptake in the phases that precede those with the greatest demand. Covre et al. (2018) studied the accumulation of macronutrients along the development of fruits of Coffea canephora and the concomitant variation in their contents in the leaves adjacent to them. These authors also verified a recover of leaf N content in the maturation phase in both, irrigated and non-irrigated plants.
Fernandes and Sousa (2006) mention that the control of nitrogen uptake has been related to the nitrogen status in the plant, showing that high levels of reduced forms of nitrogen (NO2−, NH4+ and free amino acids) lead to reduced uptake, via feedback control, indicating that the levels of reduced compounds in the plant are related to the nitrate absorption dynamics. Ivashikina and Sokolov (1997) found that the supply of reduced nitrogen compounds (ammonium and the amino acid glutamine) decreased the activity of nitrate reductase and increased the Km values for nitrate. Thus, the status of reduced nitrogen compounds in the plant can contribute to the regulation of the nitrate uptake kinetic parameters.
Unfortunately it was not possible to determine Km for the pinhead stage because, in this case, the depletion of NO3− over the time adjusted to a linear model. It is worth to note that in this early stage of fruit development the raining season was only beginning, after a long period of dry season. Because of that, few new fine roots had developed in the lateral roots selected and wrapped into vermiculite cylinders. The values of Km for the other stages increased with the successive phases of fruit development, with the lowest values been verified at the rapid expansion stage and the highest values at maturation stage. Km is defined as the solution concentration at which half of Vmax is reached and indicates the affinity of the transporters for a particular form of the nutrient, thus the lower the Km values, the greater the transporters' affinity with the ion and the greater its transport toward the interior of the root cells. These results show that the greatest transporter's affinity for nitrate occurred at the rapid expansion stage, but there was a reduction at the grain filling stage and even a greater reduction at the maturity stage. In view of that we can hypothesize that the highest Vmax at pinhead and bean filling is caused by the greater number of transporters at these stages, since its turnover was lower, as indicate by the Km values observed.
The Michaelis-Menten equation was used for a better interpretation of our data. The equation is adapted to the kinetics of nutrient uptake, and describes the absorption rate of a solute as a function of its external concentration considering Vmax and Km. The nitrate uptake by plants exhibited saturation kinetics as expected, in the range of low external concentrations (Figure 3). The absorption rate up to the concentration of 20 μmol L−1 was higher at the RE stage than at the stages of GF and MT and was achieved with the lowest concentrations due to its lowest Km value. This indicates that the nitrate uptake was more efficient in low concentrations. Above 20 μmol L−1, the absorption rate was the highest at the maturation stage due to its highest Vmax value, despite its highest Km, indicating that, in this case, probably a greater number of transporters contributed to a greater absorption, compensating for the lower affinity of the transporter for nitrate.
At the GF stage, the nitrate uptake rate was the lowest among the stages evaluated, which was influenced to a greater extent by the lowest Vmax, indicating that at this stage the number of carriers or their operating velocity was low (Figure 3). In agreement to these results, Pinto (2016) working with coffee plants of the same variety and age cultivated in hydroponic sand culture also verified the lowest Vmax at GF stage.
Correlations Between Kinetic Parameters, Production of Fine Roots and Beans
In addition to the kinetic parameters, nutrient absorption also depends on the morphological characteristics of the roots, length, diameter, surface area, and their distribution in the soil profile. A greater root system length indicates a greater volume of soil explored by the roots, with greater potential for nutrient absorption, in which the surface area and length are the most relevant characteristics for nutrient uptake efficiency (Zonta et al., 2006).
In this study, the root system fresh matter of plants was evaluated at the GF and MT stages. The GF stage was selected because it shows great competition between fruits and vegetative organs for photoassimilates (Valarini et al., 2005; Laviola et al., 2006). At the GF stage, the maximum daily rates of carbohydrate accumulation, especially starch, are achieved in the fruits (Laviola et al., 2007), which sometimes, according to plant production, results in great level of death of absorbent roots. Maturity stage was selected because of the low carbohydrate demand by fruits, which can allow the recovery of root growth.
At the GF stage, the plants presented, on average, four times less fresh matter of fine roots than in the MT stage, which could be associated with root death caused by the high demand of the fruits for carbohydrates and nutrients due to its strong sink activity (Figure 4). Mengel and Barber (1974) suggested that fruit development may affect root growth, since after pollination, in corn, root density in the topsoil layer decreased. Nutman (1933) reported that a heavy fruit load in the coffee tree severely reduced the supply of carbohydrates to the roots leading to their death. The greater amount of fine roots at the maturity stage (near the end of the rainy summer time) may indicate the recovery of root growth, which contributes to meet the nutrient demands at this stage, or otherwise, to give support to accumulate reserves for the growth of the next production cycle. In this regard, DaMatta et al. (1999) found that the nitrogen supply during the dry and cold autumn-winter, after harvest, in which the coffee plants stop growing, provided an increase in the nitrogen concentration and the activity of Nitrate Reductase in the leaf in the next active growth phase, which begins with flowering in the spring. The authors found that the nitrogen compounds accumulated in the root were reallocated to shoots, which could support higher growth rates. Therefore, these functional relationships would depend on a greater amount of fine absorbent roots at the maturity stage, which together with a higher Vmax, would increase the reserves of nitrogen compounds for further use in the new crop cycle.
In the same orchard used in this study, Magalhães (2021) studied the variation in root length, surface, volume and fresh matter over the phenological cycle of coffee fruit production during 2 years, one of low production and the other of high production. He observed that in the year of high production length, area, volume and fresh matter of thin roots (with <2 mm diameter) located in the first 20 cm soil layer decreased from rapid expansion up to maturation, while in the year of low production the reverse occurred. Such finding shows that the previous year production will determine in some extent the amount or the growth rate of roots available for nutrient absorption in the beginning of the subsequent cycle. This evidences that in addition to the kinetic parameters we need considering the fluctuations that occur in the amount of absorbent roots throughout the stages of fruit development in order to modeling properly nitrogen fertilization doses at each stage of fruit development and year of the biennial cycle of production. Anyway, the results obtained in this study corroborate the observation of Laviola et al. (2006) that the availability of N must be adequate already in the phase of rapid expansion of the fruits, and the first N fertilization need to be done before that.
The average cherry production was 10.51 t/ha, corresponding to 1.65 t/ha of coffee beans with moisture content of 11%. It is of note that the experiment was done in a year of low production and without irrigation, which leads to reduction in the average yields.
The fruit production of each tree (Prod) was correlated with the Vmax of NO3− uptake during the rapid expansion stage (0.95 Pearson correlation coefficient, Table 3). According to Laviola et al. (2009), in this same region, the RE stage has the highest daily rates of DM and N accumulation in the fruits. This indicates that at the RE stage there was accumulation of nitrogen or nitrogen compounds in the plant, which afterwards, via reallocation, contributed to complete the fruit growth.
On the other hand, the Vmax of NO3− uptake obtained at the GF and MT showed a weak negative correlation with production (Table 3). Vmax decreased at these stages, suggesting a slightly lower NO3− uptake capacity of the most productive plants at bean filling, which would indicate a negative feedback of N uptake in plants that had high uptake at the rapid expansion stage. The correlation between Vmax and fresh root mass was also negative, indicating a possible compensatory effect of increased uptake velocity as the amount of roots decreases, which seems consistent in the sense of maintaining a stable absorption rate in the face of a decrease in acquisition tissue. Edwards and Barber (1976) observed that decreasing the proportion of corn roots exposed to nitrogen-containing nutrient solution increased N uptake rate per root unit, but not enough to compensate the deleterious effect of the root length reduction.
A positive correlation, although not very high (0.57), was found between the fresh matter of fine roots at grain filling and production. This may indicate that a larger amount of roots at this stage would have little influence on fruit production, which corroborates the hypothesis that the N uptake during the rapid expansion meets the demand during the later fruit growth. In fact, Vmax at this stage was numerically lower than at the other stages.
The nitrate Vmax, at maturation, had a negative correlation with low degree of association with Prod (Table 3) despite showing the highest value among the studied phenological phases, indicating that, as expected, at this stage nitrate Vmax has little influence on the current coffee production. Similar to what occurred at the GF stage, at the MT phase nitrate Vmax had a negative correlation with root fresh matter, possibly indicating that increase in the amount of roots has a regulatory effect on nitrate Vmax as already mentioned. In contrast to the response at the GF stage, root fresh matter at the MT stage had a negative correlation with Prod, which reinforces that, at this stage, a larger amount of fine roots apt to absorb nitrate has no influence on the current fruit production.
Conclusions
Coffee-trees nitrate absorption rates are dependent on the phenological phases of fruit development, showing that the absorption mechanisms can modulate the kinetic parameters according to the different demands at each fruit development stage.
In low productivity years the coffee-tree nitrate Vmax ranges from 0.14 to 0.72 μmol g−1 h−1 in a root fresh matter basis and the Km from 6.47 to 50.31 μmol L−1.
In low productivity years the nitrate Vmax of coffee-tree is highest at the pinhead and maturation phenological phases and lowest at the grain filling phenological phase. The lowest Km occurs in the rapid fruit expansion.
At the rapid expansion stage of fruits Vmax shows a positive correlation with grain production, so before this stage, adequate nitrogen availability in the soil must be ensured so as not to affect coming coffee production.
Data Availability Statement
The original contributions presented in the study are included in the article/supplementary materials, further inquiries can be directed to the corresponding author/s.
Author Contributions
CA has planned and performed the experiment, he has also done the statistical analysis, discussed the data and wrote the first draft of the paper. HM has planned and advised the experimental activities, discussed the results and wrote the final version of the paper. RS has helped in planning the experiment and discussing the results. All authors contributed to the article and approved the submitted version.
Funding
The authors thank FAPEMIG (Pronex - CAG APQ 00594-14), CAPES (Finance code 001) and CNPq for the financial support to this study.
Conflict of Interest
The authors declare that the research was conducted in the absence of any commercial or financial relationships that could be construed as a potential conflict of interest.
References
Alvarez, V., Novais, R., Dias, L., and Oliveira, J. (2000). Determinação e uso do fósforo remanescente. Bol. Inf. Soc. Bras. Ci. Solo. 25, 27–32.
Bose, B., and Srivastava, H. (2001). Absorption and accumulation of nitrate in plants: influence of environmental factors. Indian J. Exp. Biol. 39, 101–110.
Cannell, M. G. R. (1985). “Physiology of the coffee crop,” in Coffee: Botany, Biochemistry and Production of Beans and Beverage, eds M. N. Clifford and K. C. Willson (London: Croom Helm), 108–134.
Carelli, M., Fahl, J. I., and Magalhães, C. (1989). Assimilação de nitrato durante desenvolvimento reprodutivo de plantas de café. Rev. Bras. Ci. Solo 13, 59–64.
Carvajal, J. F., Acevedo, A., and Lopez, C. A. (1969). Nutrient uptake by the coffee tree during a yearly cycle. Turrialba 19, 13–20.
Claassen, N., and Barber, S. (1974). A method for characterizing the relation between nutrient concentration and flux into roots of intact plants. Plant Physiol. 54, 564–568. doi: 10.1104/pp.54.4.564
Covre, A. M., Partelli, F. L., Bonomo, R., Tomaz, M. A., and Ramalho, J. C. (2018). Impacts of water availability on macronutrients in fruit and leaves of conilon coffee. Pesq. Agropec. Bras. 53, 1025–1037. doi: 10.1590/s0100-204x2018000900006
DaMatta, F., Amaral, J., and Rena, A. (1999). Growth periodicity in trees of Coffea arabica L. in relation to nitrogen supply and nitrate reductase activity. Field Crops Res. 60, 223–229. doi: 10.1016/S0378-4290(98)00127-0
Defelipo, B. V., and Ribeiro, A. C. (1997). Análise química do solo (metodologia). Viçosa: Universidade Federal (Boletim de Extensão no. 29).
Doane, T., and Horwath, W. (2003). Spectrophotometric determination of nitrate with a single reagent. Anal. Lett. 36, 2713–2722. doi: 10.1081/AL-120024647
Dominghetti, A. W., Guelfi, D. R., Guimarães, R. J., Caputo, A. L. C., Spehar, C. R., and Faquin, V. (2016). Nitrogen loss by volatilization of nitrogen fertilizers applied to coffee orchard. Ciênc. Agrotec. 40, 173–183. doi: 10.1590/1413-70542016402029615
Edwards, J., and Barber, S. (1976). Phosphorus uptake rate of soybean roots as influenced by plant age, root trimming, and solution P concentration. Agron. J. 68, 973–975. doi: 10.2134/agronj1976.00021962006800060033x
Epstein, E., and Bloom, A. (2006). Nutrição mineral de plantas: princípios e perspectivas. Londrina: Planta.
Fernandes, M., and Sousa, S. (2006). “Absorção de nutrientes,” in Nutrição mineral de plantas, ed M. S. Fernandes (Viçosa: Sociedade Brasileira de Ciência do Solo), 115–152.
Inforzato, R., and Junqueira, A. (1963). Estudo comparativo do Sistema radicular dos cafeeiros Boubon Amarelo e Mundo Novo. Bragantia 22, 741–750. doi: 10.1590/S0006-87051963000100067
Ivashikina, V., and Sokolov, A. (1997). Regulation of nitrate uptake and distribution in maize seedlings by nitrate, nitrite, ammonium and glutamate. Plant Sci. 123, 29–37. doi: 10.1016/S0168-9452(96)04566-9
Jacomine, P. K. T. (2009). A nova classificação brasileira de solos. Na. Acad. Pernamb. Ciênc. Agron. 5/6, 161–79.
Kulmann, M. S., Sete, P. B., de Paula, B. V., Stefanello, L. O., Schwalbert, R., Schwalbert, R. A., et al. (2020). Kinetic parameters govern of the uptake of nitrogen forms in ‘Paulsen' and ‘Magnolia' grapevine rootstocks. Sci. Hort. 264:109174. doi: 10.1016/j.scienta.2020.109174
Laviola, B. G., Martinez, H. E. P., Salomão, L. C. C., Cruz, C. D., Mendonça, S. M., and Neto, A. P. (2007). Alocação de fotoassimilados em folhas e frutos de cafeeiro cultivado em duas altitudes. Pesq. Agropec. Bras. 42, 1521–1530. doi: 10.1590/S0100-204X2007001100002
Laviola, B. G., Martinez, H. E. P., Souza, R. B., and Alvarez, V. V. H. (2006). Dinâmica de N e K em folhas, flores e frutos de cafeeiro arábico. Biosci. J. 22, 33–47. Available online at: http://www.seer.ufu.br/index.php/biosciencejournal/article/view/6372
Laviola, B. G., Souza, R. B., Martinez, H. E. P., Salomão, L. C. C., and Cruz, C. D. (2009). Macronutrient accumulation in coffee fruits at Brazilian Zona Da Mata conditions. J. Plant Nutr. 32, 980–995. doi: 10.1080/01904160902872164
Li, S. X. X., Wang, Z. H. H., and Stewart, B. A. (2013). Responses of crop plants to ammonium and nitrate N. Adv. Agron. 118, 205–397. doi: 10.1016/B978-0-12-405942-9.00005-0
Magalhães, W. B. (2021). Sistema radicular e suas interações com o desenvolvimento e nutrição do cafeeiro (Dissertation/master's thesis). Viçosa, MG: Universidade Federal de Viçosa.
Martinez, H. E. P., Souza, B. P., de Caixeta, E. T., Carvalho, F. P., and Clemente, J. M. (2020). Water deficit changes nitrate uptake and expression of some nitrogen related genes in coffee-plants (Coffea arabica L.). Sci. Hortic. 267:109254. doi: 10.1016/j.scienta.2020.109254
Mengel, D., and Barber, S. (1974). Rate of nutrient uptake per unit of corn root under field conditions. Agron. J. 66, 399–402. doi: 10.2134/agronj1974.00021962006600030019x
Miller, J., Fan, X., Orsel, M., Smith, S., and Wells, D. (2007). Nitrate transport and signaling. J. Exp. Bot. 58, 2297–2306. doi: 10.1093/jxb/erm066
Motta, A. C. V., Nick, J. A., Yorinori, G. T., and Serrat, B. M. (2006). Distribuição horizontal e vertical da fertilidade do solo e das raízes de cafeeiro (Coffea arabica L.) cultivar Catuaí. Acta Sci. Agron. 28, 455-463 doi: 10.4025/actasciagron.v28i4.758
Neto, A. P., Favarin, J. L., and Reis, A. R. (2014). Nitrogen metabolism in coffee plants in response to nitrogen supply by fertigation. Theor. Exp. Plant Physiol. 27, 41–50. doi: 10.1007/s40626-014-0030-2
Nutman, F. J. (1933). The root system of Coffea arabica L. I. Root systems in typical soils of British East Africa. Empire J. Exptl. Agric. 1, 271–284.
Paula, B. V., Marques, A. C. R., Rodrigues, L. A. T., de Souza, R. O. S., de Kulmann, M. S., Kaminski, J., et al. (2018). Morphological and kinetic parameters of the uptake of nitrogen forms in clonal peach rootstocks. Sci. Hortic. 239, 205–209. doi: 10.1016/j.scienta.2018.05.038
Pinto, W. S. (2016). Parâmetros cinéticos da absorção de nitrato e fotossíntese em cafeeiros adultos sob estresse hídrico (Dissertation/master's thesis). Viçosa, MG: Universidade Federal de Viçosa.
Prezotti, L. C., and Bragança, S. M. (2013). Acúmulo de massa seca, N, P e K em diferentes materiais genéticos de café Conilon. Coffee Sci. 8, 284–294. Available online at: http://www.coffeescience.ufla.br/index.php/Coffeescience/article/view/435
Reis, A., Favarin, J. L., and Malavolta, E. (2009). Nitrate reductase and glutamine synthetase activity in coffee leaves during fruit development. Rev. Bras. Ci. Solo 33, 315–324. doi: 10.1590/S0100-06832009000200009
Reis, A., Furlani Júnior, E., and Haga, K. (2007). Atividade da redutase do nitrato em folhas de cafeeiro em função da adubação nitrogenada. Acta. Sci. Agron. 29, 269–276. doi: 10.4025/actasciagron.v29i2.270
Rena, A. B., and Maestri, M. (1987). “Ecofisiologia do Cafeeiro,” in Ecofisiologia da Produção Agrícola, eds P. R. C. Castro, S. O. Ferreira, and T. Yamada (Piracicaba: Potafos), 119–147.
Ribeiro, A., Guimarães, G., and Alvarez, V. (1999). Recomendações para o uso de corretivos e fertilizantes em Minas Gerais: 5.a. aproximação. Viçosa: Comissão de Fertilidade do Solo do Estado de Minas Gerais.
Ronchi, C. P., Sousa Júnior, J. M. D., Ameida, W. L. D., Souza, D. S., Silva, N. O., Oliveira, L. B. D., et al. (2015). Morfologia radicular de cultivares de café arábica submetidas a diferentes arranjos espaciais. Pesq. Agropec. Bras. 50, 187–195. doi: 10.1590/S0100-204X2015000300001
Ruiz, H. A. (1985). Estimativa dos Parâmetros Cinéticos Km e Vmax por uma aproximação gráfico matemática. Rev. Cer. 32, 79–84.
Sete, P. B., Paula, B. V., de Souza Kulmann, M. S., de Rossi, A., Rozane, D. E., Hindersmann, J., et al. (2020). Kinetic parameters related to nitrogen uptake efficiency of pear trees (Pyrus communis). Sci. Hort. 272:109530. doi: 10.1016/j.scienta.2020.109530
Silva, L. O. E., Schmidt, R., Valani, G. P., Ferreira, A., Ribeiro-Barros, A. I., and Partelli, F. L. (2020). Root trait variability in Coffea canephora genotypes and its relation to plant height and crop yield. Agronomy 10:1394. doi: 10.3390/agronomy10091394
Valarini, V., Bataglia, O. C., and Fazuoli, L. C. (2005). Macronutrientes em folhas e frutos de cultivares de café arábica de porte baixo. Bragantia 64, 661–672. doi: 10.1590/S0006-87052005000400016
Wang, X., Bian, Y., Cheng, K., Zou, H., Sun, S. S-M., and He, J-X. (2012). Comprehensive differential proteomic study of nitrate deprivation in arabidopsis reveals complex regulatory networks of plant nitrogen responses. J. Proteome Res. 11, 2301–2315. doi: 10.1021/pr2010764
Keywords: Vmax, Km, phenological stages, absorption rate, nitrogen
Citation: Avellaneda Bohórquez CA, Martinez HEP and Santos RHS (2021) Kinetic Parameters of Nitrate Absorption by Adult Coffee Trees. Front. Sustain. Food Syst. 5:677580. doi: 10.3389/fsufs.2021.677580
Received: 08 March 2021; Accepted: 20 May 2021;
Published: 21 June 2021.
Edited by:
Everlon Cid Rigobelo, São Paulo State University, BrazilReviewed by:
Roberta Mendes, São Paulo State University, BrazilFábio Luiz Partelli, Federal University of Espirito Santo, Brazil
Copyright © 2021 Avellaneda Bohórquez, Martinez and Santos. This is an open-access article distributed under the terms of the Creative Commons Attribution License (CC BY). The use, distribution or reproduction in other forums is permitted, provided the original author(s) and the copyright owner(s) are credited and that the original publication in this journal is cited, in accordance with accepted academic practice. No use, distribution or reproduction is permitted which does not comply with these terms.
*Correspondence: Herminia Emilia Prieto Martinez, herminia@ufv.br