- 1The Alliance of Bioversity International-CIAT, Crops for Nutrition and Health, Tropical Forages Program, Cali, Colombia
- 2The Colombian Agricultural Research Corporation, Villavicencio, Colombia
In many parts of the foothills of the Orinoquía region of Colombia, cattle production takes place on poorly drained soils. The region is dominated by extensive grazing systems of Brachiaira humidicola cv. Humidicola, a grass with high adaptation potential under temporal waterlogging conditions. Inadequate management practices and low soil fertility result in degradation, however, with important negative effects on pasture productivity and the quality and provision of (soil) ecosystem services–a situation that is likely to worsen in the near future due to climate change. Against this background, AGROSAVIA (Corporación Colombiana de Investigación Agropecuaria) selected Arachis pintoi CIAT 22160 cv. Centauro (Centauro) as a promising alternative for the sustainable intensification of livestock production and rehabilitation of degraded areas. This study assesses dual-purpose milk production in the foothills of the Colombian Orinoquía from an economic perspective. We compare two production systems: the Centauro–Brachiaira humidicola cv. Humidicola association (new system) and Brachiaira humidicola cv. Humidicola as a monoculture (traditional system). We used cashflow and risk assessment models to estimate economic indicators. The projections for economic returns consider changes in forage characteristics under regional climate change scenarios RCP (2.6, 8.5). The LIFE-SIM model was used to simulate dairy production. Results show that the inclusion of Centauro has the potential to increase animal productivity and profitability under different market scenarios. The impact of climatic variables on forage production is considerable in both climate change scenarios. Both total area and potential distribution of Centauro could change, and biomass production could decline. Brachiaira humidicola cv. Humidicola showed better persistence due to higher nitrogen levels in soil when grown in association with Centauro. The legume also provides a number of ecosystem services, such as improving soil structure and composition, and also contributes to reducing greenhouse gas emissions. This helps to improve the adaptation and mitigation capacity of the system.
Introduction
Context of Improved Forages in the Orinoquía
In many parts of the foothills of the Orinoquía region of Colombia, cattle production takes place on poorly drained soils. Consequently, extensive grazing systems characterize the region and Brachiaira humidicola cv. Humidicola is the most common feed option to be found. It was introduced in the 1970s in order to improve the production of the region, due to its high adaptation potential under temporal waterlogging conditions and good forage production (ICA, 1987). As a result of inappropriate management practices and low soil fertility in the region, however, most of these pastures are, today, in some state of degradation (Rincón et al., 2018). This has led to a significant reduction in pasture productivity, as well as negative effects on the quality and provision of soil ecosystem services (Fonte et al., 2014; Galdino et al., 2015), generating important economic and ecological implications for the region. Cattle and dairy production are considered to be one of the main sources of greenhouse gas emissions, derived from the digestion process of the animals (methane and nitrous oxide emissions), the use of nitrogen fertilizers, as well as the expansion of productive areas through deforestation or the invasion of protected areas (CIAT CORMACARENA, 2018). The cattle sector is therefore considered one of the key sectors for interventions with great potential for climate change mitigation. In this sense, achieving intensive livestock farming in a sustainable way has become one of the main approaches for sector development in the Orinoquía.
In short, this means more efficient cattle farms which consider, protect and sustainably use existing water and environmental resources, allowing for a reduction of greenhouse gas emissions and deforestation levels. This focus is particularly important for the Orinoquía, which is recognized for its strategic environmental importance with ecosystems of high conversation value, such as natural savannas, flooded forests, humid forests, foothills, estuaries and wetlands. The need to achieve more efficient cattle farming without affecting natural ecosystems is enshrined in the most recent Regional Climate Change Plan for the Orinoquía (CIAT CORMACARENA, 2018), which is aligned with the national approaches defined in the Strategic Plan for the Colombian Cattle Sector from 2019 (FEDEGAN, 2018)1.
This context is likely to aggravate under a climate change scenario that would accelerate soil degradation processes (Olsson et al., 2019), especially when combined with unsustainable land use or poor management practices (Sattler et al., 2018). According to the climate projections for the Orinoquía to 2100, annual precipitation will decrease and maximum temperatures will increase, leading to periods of more extreme heat and heat stress (IDEAM, 2015). These forecasts would affect livestock production mainly through (i) changes in biomass production and quality of forages, which translates into a decrease in milk and meat production, and (ii) heat stress in animals, which leads to significant losses in production, growth, development and reproduction (CIAT CORMACARENA, 2018). Furthermore, not only projected mean changes can have an impact, but also changes in the variability and strength of extreme weather events, leading to significant consequences for livestock production (e.g., increased frequency of heat stress, drought events and floods; Thornton et al., 2009). The effect of these climatic phenomena is also reflected at the macroeconomic level via prices, because when faced with climatic events, food prices tend to vary, generating transitory inflationary pressures (Melo et al., 2017). As a result of reductions in precipitation levels caused by the El Niño phenomenon, for example, a reduction in agricultural supply is generated, which in turn leads to temporary price increases (Melo et al., 2017).
Apart from the climatic impacts on local production systems, the increasing demand for animal source food (OECD/FAO, 2020) creates pressure on livestock producers to extend production areas. In the case of the Orinoquía, this can cause increasing rates of deforestation and a penetration of important local ecosystems (such as native savannas), leading to irreversible changes within, and losses of, local ecosystems, biodiversity and cultural heritage, aggravating climate change even further.
In this sense, there is an increasingly pressing need to implement sustainable production systems with greater capacity for adaptation and mitigation to climate change, systems that contribute to maintaining, improving and protecting local ecosystems. One of the most promising alternatives to achieve the previous objectives, as well as to restore degraded areas, is the use of forage legumes in livestock systems (Fisher et al., 1994; Shelton et al., 2005; Murgueitio et al., 2011; Schultze-Kraft et al., 2018). Their high protein content improves nutritional values and the efficiency of animal feed, which in turn reduces enteric methane emissions (Dickie et al., 2014). Legumes also contribute Nitrogen (N) to the soil through symbiotic N fixation that improves both soil fertility and forage persistence (Rao et al., 2014; Villegas et al., 2020). According to Fisher et al. (1994), the association of deep-rooted grasses with nitrogen-fixing legumes has three important effects, namely (i) increased nutrient cycling, (ii) improved animal production, and (iii) increased soil biological activity, and thus play a key role in restoration, stabilizing the global carbon cycle and reducing greenhouse gas emissions. In addition to that, they provide many other ecosystem services, such as improved soil structure, water infiltration, increased carbon accumulation, favored biological activity, and contributions to weed control and soil conservation (Jensen et al., 2012; Schultze-Kraft et al., 2018).
As part of the research efforts to identify forage legumes adapted to the specific conditions of temporary water saturation in the Orinoquía foothills, AGROSAVIA started evaluating 22 promising legumes in 2013. After 3 years of agronomic evaluations, Arachis pintoi CIAT 22160 cv. Centauro (Centauro) was selected as the most promising material for release. It presents desirable characteristics in both productive terms (e.g., good nutritional quality, less weed presence, greater foliar area, absence of pests and diseases) and environmental terms (e.g., better soil coverage and, consequently, less susceptibility to soil erosion) and has high potential for integration in silvo-pastoral systems (shade tolerance) (Rincón et al., 2020). These characteristics make Centauro a good alternative for the purposes of sustainable intensification and restoration of degraded pastures in the region. When it comes to new technologies, however, land-use and adoption decisions by the livestock producer are mainly based on the profitability promises that the technology can generate (Pannell et al., 2006). Profitability is a fundamental attribute to incentivize or generate adoption, information which, in many cases, is not available to the livestock producer or the extension agents supporting decision-making processes. Profitability is not, however, the only measure since other factors exist that contribute to incentivizing or discouraging the adoption of new technologies, such as cultural, behavioral or environmental factors.
Regarding economic studies on the inclusion of Arachis pintoi in livestock systems, limited advances have been made so far. Most of them were carried out by the International Center for Tropical Agriculture (CIAT) in Latin America more than two decades ago. These studies mainly dealt with measuring the effects on different economic indicators of the inclusion of Arachis pintoi CIAT 17434 in grazing systems. Rivas and Holmann (2000) evaluated changes that occurred between 1986 and 1997 in productive and economic indicators in farms in the Colombian Caquetá Department that were early adopters of the Arachis pintoi CIAT 17434 variety. According to their results, production levels of both meat and milk more than doubled with the inclusion of the legume, reflected in higher gross yields per hectare (6%) and animal (20%). Based on these results, the same authors carried out an ex-ante evaluation estimating an Internal Rate of Return (IRR) of between 19.3 and 21.1% resulting from the inclusion of the legume—which equates to an increase compared to the traditional production system (IRR = 12%). Evaluations in Costa Rica estimated a 30% reduction in production costs per kilogram of milk associated with the inclusion of Arachis pintoi and Cratylia (Peters et al., 2001). Also in Costa Rica, Jansen et al. (1997) estimated an IRR of 122% in a well-managed grass-legume association of Brachiaria brizantha and Arachis pintoi. For the Amazon region of Brazil, Valentim and Andrade (2005) estimated a gross profit per year of US$ 4,000 generated by the adoption of Arachis pintoi by ~1,000 cattle producers. According to our literature review, neither more recent economic analyses nor any quantitative risk assessments or climate change impact estimates were found for Arachis pintoi, nor the new CIAT 22160 Centauro variety. Our study therefore contributes to closing an important knowledge gap and provides updated information on the new Centauro variety, released in 2020, in order to facilitate dissemination and adoption processes for the actors involved (e.g., cattle producers, extension agents, development agencies or donors).
In this sense, the objective of our study is to evaluate the economic viability of milk production in a dual-purpose cattle system in the foothills region of the Colombian Orinoquía under a grass-legume association with Brachiaria humidicola cv. Humidicola and Arachis pintoi CIAT 22160 cv. Centauro (grass-legume association). We compare these results with a traditional production system under a Brachiaria humidicola cv. Humidicola monoculture (grass monoculture). In order to estimate economic indicators, we used a cashflow model and conducted a risk assessment using a Monte Carlo simulation model. The projection of economic returns is carried out considering changes in forage characteristics (dry matter production) for both production systems as a response to changes in projected climatic variables, according to the climate change scenarios for the Representative Concentration Pathways of the region (RCP 2.6 and 8.5; IDEAM, 2015). It also includes potential effects on price variations as a consequence of recurring climatic events (El Niño and La Niña). With this information, profitability indicators for each system (e.g., Net Present Value, Internal Rate of Return) are calculated and help in the identification of the treatment with better adaptability under climate change scenarios.
Research on Arachis pintoi in the Neotropics
Historical Review: Technical Evaluation Processes of Arachis pintoi in Colombia
The evaluation of Arachis genotypes in Colombia began in 1978 with the introduction of 45 accessions from germplasm collections in the U.S. [i.e., from the University of Florida and the United States Department of Agriculture (USDA)] by CIAT to its Carimagua Research Center in the Orinoquía region (Rincón et al., 1992). These accessions have been wild-collected since 1981 by USDA, EMBRAPA (Empresa Brasileira de Pesquisa Agropecuária) and CIAT (Valls and Pizarro, 1995). Arachis species are present in countries such as Brazil (more than 60 wild species), Bolivia (15), Paraguay (14), Argentina (6), and Uruguay (2) (Valls and Pizarro, 1995).
From 1987 to 1990, CIAT (in collaboration with other institutions) worked on the selection of Arachis pintoi germplasm with potential for adaptation to acid soils and to restore large areas of degraded pastures in the Colombian Caquetá Department. The grass-legume association of Arachis pintoi with various species of Brachiaria was identified as the most promising solution (Lascano et al., 2005), and after several years of research, the variety Arachis pintoi CIAT 17434 (perennial forage peanut) was released in 1992. The variety is characterized by its good adaptation to climate and soil conditions in the Colombia Orinoquía but also has some important limitations such as slow establishment, low forage production during the first 2 years and high defoliation rate in the dry season (Rincón, 2001). In an attempt to solve these problems, the evaluation of new Arachis pintoi accessions was taken up in 1994 in various South American countries (Colombia, Brazil, Ecuador, Peru and Bolivia). The accession CIAT 22160 was among the evaluated materials. This accession is native to Brazil, was found in the eastern Andes, between the Amazon and La Plata rivers, and was collected in 1992 by the researcher Wantuil Werneck and delivered to the CIAT gene bank by EMBRAPA (Brazil).
The first evaluations of this material were made in Brazil in 1994 together with another 49 Arachis pintoi accessions. CIAT 22160 stood out for presenting high persistence during the dry season (CIAT, 1994). In Colombia, the earliest evaluation records of CIAT 22160 were documented by Moreno et al. (1999), Cárdenas et al. (1999), and Peters et al. (2000) as part of a multilocational trial with several Arachis pintoi accessions. The objective was to find alternatives to Arachis pintoi CIAT 17434 (perennial forage peanut) with higher adaptability. The evaluated accessions were acquired by CIAT between 1993 and 1994 from EMBRAPA-CENARGEN (Brazil) and the National Institute of Agricultural Technology (INTA, Argentina) (CIAT, 1994). The experiments were established between 1994 and 1995 in different locations: (i) 39 accessions in a tropical dry forest (Moreno et al., 1999), (ii) 41 accessions in a very humid premontane forest (Cárdenas et al., 1999), and (iii) 61 accessions in a very humid forest ecosystem (Peters et al., 2000). Accession CIAT 22160 was identified as promising material for very humid tropical forests, with superior characteristics to the control variety (Arachis pintoi CIAT 17434), such as greater rooting, faster growth, higher dry matter production and a more efficient use of Phosphorus (CIAT, 2002). The first evaluation of CIAT 22160 in the Colombian Orinoquía was conducted by Rincón (2001) with 11 Arachis pintoi accessions (Arachis pintoi CIAT 17434 as control variety). Rincón (2001) established two experiments at the CORPOICA (now AGROSAVIA) research center La Libertad in the Meta Department on poorly drained soils, leading to a preselection of the three best performing accessions according to their agronomic performance: Arachis pintoi 22160, 18748, and 18744. All three accessions stood out for their high dry matter production (>1 ton/ha) and level of soil cover (>70%) (Rincón, 2001).
Evaluation of Arachis pintoi CIAT 22160 Under Temporary Flooding Conditions
In 2013, CORPOICA (now AGROSAVIA) started the evaluation of Arachis pintoi under temporary flooding conditions in the Orinoquía (Rincón and Pesca, 2017). Trials were established at the research center La Libertad in the Meta Department under medium drainage conditions and included 22 Arachis pintoi accessions. Each accession was established in plots with an area of 6 m2, in a complete random block design with three repetitions. From 2013 to 2014, a series of agronomic evaluations were carried out that led to the preselection of four accessions (22160, 18748, 18744, and 17434) which were then evaluated at the agronomic level during both the dry and rainy seasons. After another year of evaluation, the Arachis pintoi CIAT 22160 was selected for grazing trials as a result of its outstanding attributes of soil cover, persistence, competition with weeds, forage production and nutritional quality.
The grazing trials were carried out from February 2016 to April 2017 at the farm Los Arrayanes, and from October 2019 to February 2020 at the farm El Recreo, both located in the Orinoquía region and presenting temporary flooding conditions. The accession CIAT 22160 was established in August 2015 in both locations with vegetative material, in an area of 2000 m2 and in association with the grass Brachiaria humidicola cv. Humidicola (grass-legume association). Productivity results were compared with data obtained from a monoculture grazing trial with the grass Brachiaria humidicola cv. Humidicola (grass monoculture). Animal productivity was measured in lactating cows under a dual-purpose system. The animals were supplemented, in both treatments (grass monoculture and grass-legume association) with 8% mineralized salt at an amount of 80 g AU−1 d−1 throughout the year. Cut grass silage was supplied during the daily milking time at an amount of 2 kg/animal/d for ~180 days a year. In order to maintain pasture productivity levels, maintenance fertilization and weed control were performed once a year for both treatments. Diammonium Phosphate (DAP) (100 kg ha−1) and Sulgamac (100 kg ha−1) were applied for the grass-legume association. For the grass monoculture urea (100 kg ha−1) was added to DAP and Sulgamac. The results of these measurements (biomass production, nutritional quality and animal response) for each treatment are presented in Table 1, since our economic analysis is based on these technical parameters obtained in the study by Rincón et al. (2020), previously described.
General Characteristics of Arachis pintoi CIAT 22160 cv. Centauro
Arachis pintoi CIAT 22160 was identified by AGROSAVIA as a promising material to improve the quality of cattle feed in the Colombian Orinoquía, especially under poorly drained soil conditions (Rincón et al., 2020). It was released in 2020 under the common name Centauro (Arachis pintoi CIAT 22160 cv. Centauro), but its commercialization will only begin in 2022 in accordance with private sector seed production schedules. Centauro is a perennial herbaceous forage legume with prostrate growth. It has an average height of 20 cm and a leaf-stem ratio of 1.4 (60% leaves and 40% stems) (Rincón et al., 2020). Its flower is generally self-pollinated but can also be cross-pollinated by bees. The first flowers appear at a plant age of 14–55 days (Simpson et al., 1995). Centauro has a wide range of adaptation, from low acid soils to high fertility soils, with a soil texture ranging from sandy loam to clay loam and with good or poor drainage. In addition, it grows well in tropical regions from 0 to 1,800 m elevation and with an annual rainfall between 1,200 and 4,000 mm. It is characterized by a high biomass production and forage quality (Table 1), and its leaf crude protein levels vary between 15 and 18%. It adapts well in association with invasive Gramineae or in silvo-pastoral systems (good shade tolerance), has strong persistence, competes with weeds, and is tolerant to several pests and diseases. Its prostrate and invasive growth results in soil cover levels of >90%, favoring the reduction of soil compaction and erosion (Rincón et al., 2020).
Potential Ecosystem Services of Arachis pintoi CIAT 22160 cv. Centauro
The inclusion of Centauro in forage-based livestock systems has high potential regarding the provision of ecosystem services. In grazing systems, different studies have shown a high persistence of Arachis pintoi with positive effects on soil conservation and improving soil conditions (Rincón, 1999; CIAT, 2004; Castillo-Gallegos et al., 2005; Robertson, 2005; Valentim and Andrade, 2005). These positive effects are mainly associated with high production of seed below ground, a prostrate growth habit that invades bare soil, as well as tolerance to trampling and defoliation, protecting the arable soil layer and, therefore, avoiding degradation and erosion processes (Rincón, 1999; Rincón et al., 2020). Under animal grazing trials, grass-legume associations with Arachis pintoi have not shown signs of degradation after several years of grazing (e.g., Lascano, 1994; Rincón, 1999; Valentim and Andrade, 2005). Arachis pintoi has positive effects on the soil organic matter content and soil biodiversity (Rincón, 2001), improving the physical, chemical and biological soil conditions and avoiding erosion associated with overgrazing, but may reduce above ground biodiversity. Arachis pintoi can also improve the persistence of the associated grasses resulting from a symbiotic nitrogen fixation to the soil, which is then used by the grass (Villegas et al., 2020). For example, Dubeux et al. (2017) estimated a range of 123 to 280 kg ha−1 yr−1 of fixed nitrogen in six Arachis pintoi accessions, and Pereira et al. (2019) evaluated beef production in an associated system of Brachiaria brizhanta and Arachis pintoi (cv. Belomonte), estimating a minimum nitrogen fixation of 120 kg ha−1 yr−1. The higher contribution of nitrogen not only represents a strategy for the restoration of degraded pastures, but also contributes to reducing the use of nitrogen fertilizers and, therefore, to reducing nitrous oxide emissions. Other studies focused on estimating the effect of Arachis pintoi accessions on carbon levels and other elements in the soil. Nutrient uptake in Brachiaria humidicola monoculture pastures and in grass-legume associations of Brachiaria humidicola and Arachis pintoi in acid soils with low fertility was, for example, measured in the Orinoquía, showing that the inclusion of Arachis pintoi increased the nitrogen, calcium, potassium and phosphorus availability in the soil by 130, 133, 19, and 13%, respectively (CIAT, 1994). In evaluations in the Atlantic coast of Costa Rica and the humid forest of the Colombian Amazon, different grass-legume associations with Arachis pintoi showed statistically higher levels of carbon reserves in the soil than in the native forest (Amézquita et al., 2004). In the Orinoquía, the inclusion of Arachis pintoi in a Brachiaria humidicola pasture notably increased the amount of carbon in the soil (CIAT, 1994). Arachis pintoi also helps in reducing greenhouse gas emissions associated with ruminal fermentation processes (higher nutritional quality of the forage) and the application of nitrogen fertilizers, while contributing to the intensification of cattle systems through productivity and increases in animal carrying capacity (Rincón et al., 2020).
Materials and Methods
Discounted Cash Flow Model
The present study's economic analysis is based on a discounted cash flow model and the estimation of profitability indicators, such as Net Present Value (NPV) and Internal Rate of Return (IRR). These indicators are obtained assuming the most probable values of the model variables (associated with benefits and costs). The analysis is carried out by comparing the profitability indicators for the grass-legume association and the grass monoculture. The cash flow allows ordering and synthesizing the sequence of income, costs and investments associated with the evaluated technologies. The following cost categories were considered: total costs of establishment and maintenance of each treatment, opportunity costs of capital and operating costs (animal health, supplementation, permanent and occasional labor). The benefits are derived from milk production in a dual-purpose system, according to the animal response indicators obtained for each treatment (Table 1).
Model Assumptions
For the construction of the cash flow it is necessary to establish different economic and technical assumptions. The following sections provide detailed explanations for each of them.
Technical Assumptions
Given that productivity was measured only for daily milk production (see section Research on Arachis pintoi in the Neotropics), the other technical indicators are assumed to be the same for both treatments and were described by consulting average values reported for the study region: (i) 550 days calving interval; (ii) calf age of 9 months and weight of 150 kg at weaning; and (iii) lactation time of 8.5 months. AGROSAVIA researchers verified these indicators for the region.
Evaluation Horizon
The evaluation horizon is established according to the expected lifespan of a technology under evaluation. For the evaluation of the grass-legume association and the grass monoculture, a period of 10 years (2020–2029) was defined, which is in accordance with the productive lifespan for improved pastures (Riesco and Seré, 1985). It is, however, worthwhile mentioning that improved pastures can have a much longer productive lifespan if managed adequately (e.g., in terms of grazing and fertilization).
Discount Rate
The cost of financing is chosen as the discount rate according to the rural credit lines of FINAGRO (the Colombian Fund for the Financing of the Agricultural Sector). This financing cost is considered the opportunity cost of capital and is associated with a risk factor present in the activities of the rural sector. The following discount rate was therefore established: DTF (fixed-term deposit rate) + 5% effective annual interest rate. The projection of the discount rate in the corresponding periods was made following the DTF projections according to the Annual Report on Economic Projections Colombia 2020 (Bancolombia–Dirección de Investigaciones Económicas, 2020).
Permanent Labor
The required permanent labor is defined according to the weighting factors for labor established by FEDEGAN (2003). In a dual-purpose cattle system, 4.8 permanent jobs are needed for every 100 animals. The minimum salary for 2019 was used, including transportation assistance, contributions to social security, and social and parafiscal benefits, adding up to US$ 422 per month. For salary projections during the period of analysis (2020–2029), the following was assumed: Variation of the minimum salary (in %) = expected inflation (in %) + observed variation of workforce productivity (WP, in %). A WP of 1% is assumed, according to historical estimates from national statistics (DANE, 2020a).
Currency at Current Prices
Inflation is considered for estimating revenue and cost streams during the evaluation period. For revenues, the projection of the Consumer Price Index (CPI) estimated by Bancolombia–Dirección de Investigaciones Económicas (2020) for the period 2020-2023 was considered. For production costs, the Producer Price Index (PPI) estimated by DANE (2020b) was used.
Milk Price. Price information was obtained from the Milk Price Monitoring Unit (USP) for the predefined Region 2, where dual-purpose production systems predominate (MADR/USP, 2020). The prices were projected according to the CPI projections. Additionally, we included projections for the effect of extreme climatic events (El Niño and La Niña phenomena) on milk price variations. Abril et al. (2017) quantified climate impacts on food inflation in Colombia. According to their results, after the occurrence of an El Niño or La Niña phenomenon, food inflation increases significantly between four and 5 months later (increasingly when the intensity of the phenomenon is strong), and its response is asymmetric depending on the impacts and size of the shock. This directly affects the income received by producers and household purchasing power in Colombia. Regarding milk prices, variations have been >7% in the years with such climatic events, compared to variations of <1% in years without (DANE, 2020a,b). The variation margin of the CPI was assumed for the occurrence climatic phenomena as follows: (i) the spread of the CPI vs. the CPI of milk in 2018 was assumed as the spread that the price of milk would have against the national CPI for a scenario where no climatic phenomenon occurs; (ii) the spread of the CPI vs. the milk CPI of 2015–2016 was assumed as the spread that the price of milk would have against the national CPI for a scenario where a climatic phenomenon occurs; (iii) variations in the CPI of 7% with a climatic phenomenon and <1% without are considered (Figure 1); and (iv) both the CPI and CPI for milk were obtained from DANE (DANE, 2020b,c).
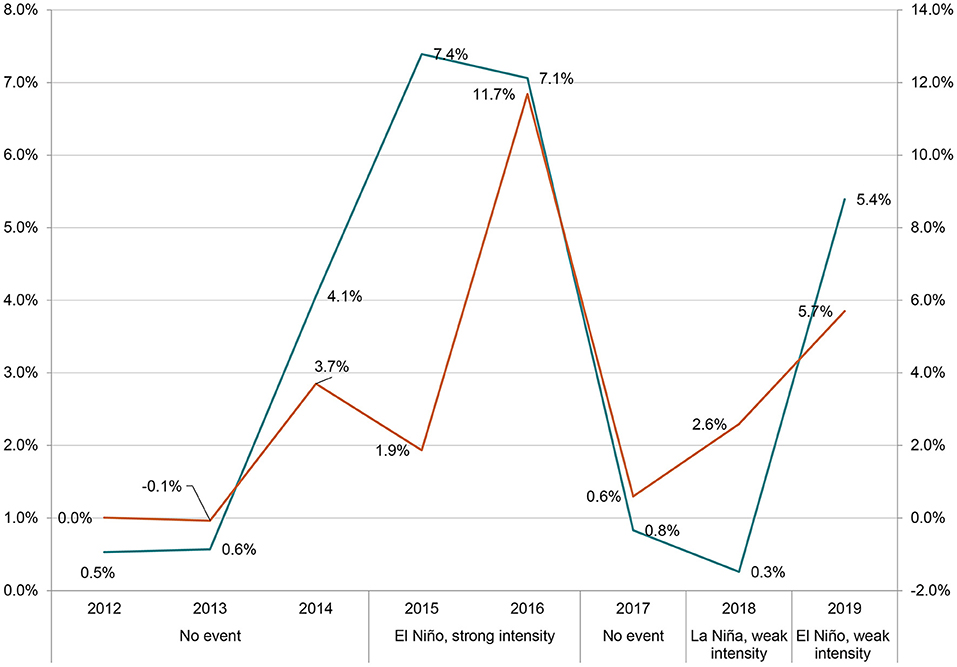
Figure 1. Consumer Price Index (CPI) and Producer Price Index (PPI) behavior of milk in the face of climatic events. Source: Own elaboration based on DANE (2020b,c).
Quantitative Risk Analysis
Risk is defined as the possibility that the real return on an investment is less than the expected return (Park, 2007). Profitability is therefore associated with the variability of revenue and cost streams, and these in turn depend on the randomness of the main variables of the investment project (e.g., yields, market prices). Rural investment projects involve particular risks, and their results depend on a broad set of variables which, in many cases, cannot be controlled by the investor/producer (e.g., climatic factors). In this sense, it is necessary to incorporate risk levels associated with the profitability indicators for each of the evaluated investment alternatives. For this purpose, we apply a Monte Carlo simulation model. The accuracy of simulation models depends on the quality of the input data. In this study, for example, the milk production data under each treatment was derived from on-farm measurements carried out during representative periods (i.e., rainy and dry seasons; section Evaluation of Arachis pintoi CIAT 22160 Under Temporary Flooding Conditions). We consider this data reliable, reflecting the distribution and real behavior of the variable observed by the technical team. Cost data and possible variations of its values were constructed with experts from AGROSAVIA according to the real conditions of cattle producers in the region in terms of prices and quantities used.
Monte Carlo Simulation Model
Monte Carlo simulation is a method in which a random sample of results is generated for a specific probability distribution (Park, 2007). This method allows potential investors or decision makers to see all the possible results and to evaluate the impact of risk on profitability indicators in investment projects. To perform the simulation, it is necessary to determine the random input variables (those that can have more than one possible value) and the possible range values for each. These variables are assigned a probability distribution, to later calculate the determined profitability indicators. Monte Carlo simulation was performed with the software @Risk (Paladise Corporation). For the evaluated treatments, 5,000 iterations were performed with a confidence level of 95%.
Decision Criteria
As decision criteria, the mean values and the variance of the profitability indicators resulting from the simulation are used: Net Present Value (NPV) and Internal Rate of Return (IRR) [Equations (1, 2)]. The use of the mean value criterion is based on the law of large numbers, which establishes that, if many repetitions of an experiment are carried out, the average result will tend toward the expected value (Park, 2007). The variance of the indicators determines the degree of spread or dispersion on both sides of the mean value (Park, 2007). That is, the lower the variance, the lower the variability (loss potential) associated with the indicators.
Where,
E (FCt): Expected value of the net profit flow for period t
Var (FCt): Net profit flow variance for period t.
r: Real discount rate
r*: Internal rate of return
t: Evaluation horizon of the project
The NPV at risk indicator (VaR) is also estimated and the probability of success of the evaluated investments is estimated (Prob NPV (mean) > 0). The VaR is defined as the maximum expected loss that the project could suffer from investment in a time interval and with a certain level of confidence (Park, 2007). The probability of success is defined as the proportion of positive results of all interactions (NPV > 0, the project is economically viable). A sensitivity analysis was performed using a tornado graph, which sensitizes each variable in order to measure its impact on the profitability indicators and to identify within the critical variables those with the greatest effects on the profitability indicators.
Simulated Variables
The study considers how sensitive the economic results are to changes in the main variables of the model. Table 1 shows the variables identified as risk variables and the distributions and parameters used for modeling them. For modeling the milk production variable for the grass-legume association and the grass monoculture, a distribution adjustment of the data was performed with @Risk.
Economic Evaluation Under Climate Change Scenarios RCP 2.6 and 8.5
Forage Production Under Climate Change Scenarios
The effect of climate change on livestock productivity was determined by comparing forage biomass production under a baseline (current) scenario with estimated levels under climate change scenarios. We used two climate change scenarios for the region: RCP 2.6 and RCP 8.5 (Armenta et al., 2015). To identify the main environmental factors that affect the productivity of the evaluated treatments, as well as the magnitude of the effect, an analysis of variance (ANOVA) was performed. The delta identified in both climate change scenarios was applied to each of these environmental factors, to estimate the monthly biomass production per hectare. This delta refers to the change in climatic variables between one scenario and another. It is important to note that the model is only considering changes in the climatic variables of the RCP, keeping constant the assumptions of pasture and soil management, level of technology, investment in labor and animal characteristics.
In addition to possible changes at the productive level of the forage species, the change in environmental conditions under climate change scenarios can alter the potential distribution of plant species. In other words, the species would tend to modify their distribution toward latitudes and altitudes different to those where they are currently found (Walther et al., 2005). To identify this possible effect, the maximum entropy model Maxent (version 3.4.1; Phillips et al., 2021) was used. The model makes it possible to estimate the extent of future environments and to determine, in the case of the legume Arachis, if and where conditions similar to the current environments exist.
The Maxent model requires two input streams: (i) points of presence (distribution) of the species throughout the world and, (ii) bioclimatic variables. The current distribution points of Arachis were downloaded from Global Biodiversity Information Facility (GBIF.org, 2020) with more than 600 points of presence which, after cleaning outliers and anomalous data, reached just over 300 total points. The second input of the Maxent model were the bioclimatic variables for RCP 2.6 and 8.5 obtained from Navarro-Racines et al. (2020). These variables represent annual trends (e.g., mean annual temperature and precipitation), seasonality (e.g., annual ranges of temperature and precipitation) and extreme or limiting environmental factors (e.g., temperature of the coldest and warmest month, precipitation of humidity).
Milk Production Under Climate Change Scenarios
Based on the forage production estimates under climate change scenarios (section Potential Ecosystem Services of Arachis pintoi CIAT 22160 cv. Centauro), milk production estimations were performed in the LIFE-SIM model (version Dairy 15.1), developed by the International Potato Center (CIP; León-Velarde et al., 2006). In this model, milk production estimations are based on the characteristics of the animals, forages and climatic conditions (temperature, humidity and wind speed) (León-Velarde et al., 2006). Tables 2, 3 and 4 present the information used in the model. The analysis did not consider episodes of heat stress in the animals, which could also affect milk production.
Economic Evaluation Under Climate Change Scenarios
Based on the results of milk production under the climate change scenarios (Table 7) and according to the methodology presented in sections Evaluation of Arachis pintoi CIAT 22160 Under Temporary Flooding Conditions and General Characteristics of Arachis pintoi CIAT 22160 cv. Centauro, the profitability indicators were estimated for both treatments. Figure 2 shows how the different models used in this study are interlinked (Maxent, LIFE-SIM and economic models).
Results
Discounted Cash Flow Model
Table 5 shows the average costs and revenues for the grass-legume association and the grass monoculture, respectively. The models include the variable costs and revenues associated with the establishment of each technology under a dual-purpose production system. The revenue results from the sale of raw milk and the sale of weaned calves (150 kg) every 550 days (calving interval). According to average daily milk production, the inclusion of Centauro in the system (grass-legume) allowed an increase in milk production per hectare by, on average, 52% when compared to grass in monoculture. Particularly during the months of minimal rainfall (dry season from January to March), grass-legume showed greater persistence and, consequently, a more stable milk production. The average production was 2,373 l ha−1 yr−1 for the grass-legume association and 1,560 l ha−1 yr−1 for the grass monoculture, respectively. This is equivalent to a gross income from raw milk sales of US$ 822 and US$ 518, respectively, representing a 58% increase for the grass-legume association.
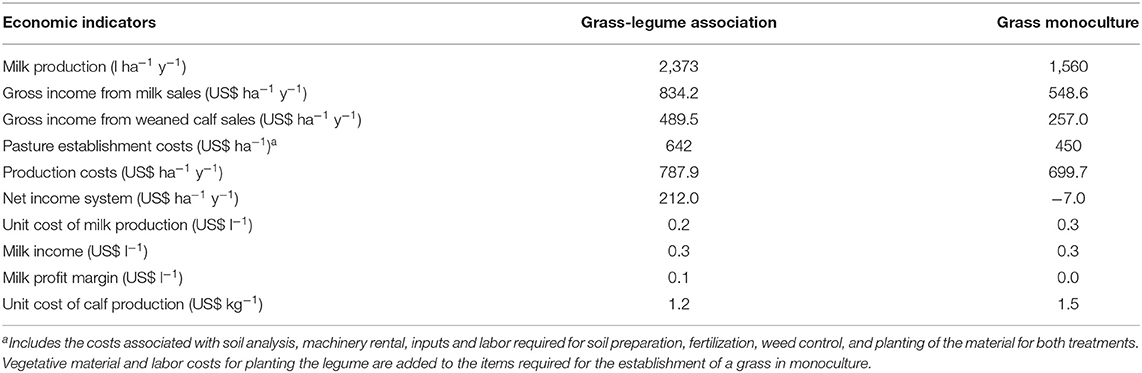
Table 5. Summary of main costs and revenues for the grass-legume association and the grass monoculture.
Regarding production costs, labor (63%) makes up the largest share, followed by inputs for pastures (21%), supplements (8.5%), drugs (1.2%), and other costs (5%). The unit production cost of milk is US$ 0.23 for the grass-legume association and US$ 0.31 for the grass monoculture, respectively, representing 35% lower costs for the grass-legume association. The average net profit for the year is US$ 212 for the grass-legume association and US$ −6.95 for the grass monoculture. Under these assumptions, production under the grass monoculture is unprofitable, a consequence of the low productive indicators associated with this alternative. It is important, however, to highlight the social connotation of dual-purpose systems in the country: given the cash flow provided by the sale of raw milk, its high nutritional value and the relatively low barriers for getting involved in the business, it is still an attractive alternative for many producers, from which they derive the subsistence for their family. This exercise includes the required labor costs (permanent and occasional), valued at the minimum salary (plus benefits). These costs could, however, reflect the opportunity cost of family labor that, in many cases, is not accounted for within the cost structures, but rather represents part of the household income.
Profitability Indicators and Risk Analysis
The summary of the main financial indicators obtained from the Monte Carlo simulation is presented in Table 6 Under the assumptions used in the model and according to the indicator coefficient of variation (CV) and VaR, the inclusion of Centauro in the grass-legume association allows better economic and lower risk indicators to be obtained when compared with Brachiaria humidicola cv. Humidicola as a monoculture. The results indicate that the investment in the establishment of the grass-legume association is profitable, with an average NPV of US$ 121 and an IRR of 12.2%.
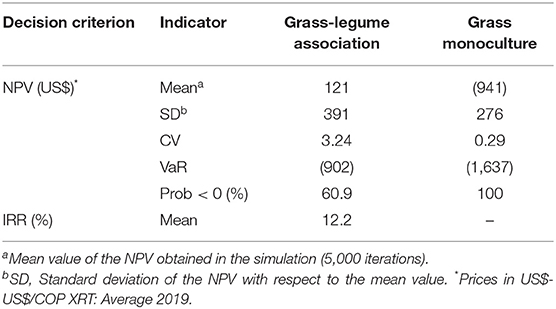
Table 6. Profitability indicators of the simulation model for the grass-legume association and the grass monoculture.
Regarding the probability of not obtaining financial feasibility, the results of the NPV probability distribution are presented in Figure 3 and reflect the amplitude of the variation for the NPV indicator. For the grass-legume association, in 60.9% of the scenarios generated during the simulation, an NPV > 0 was obtained, whereas for the grass monoculture, the investment was not profitable under any of the generated scenarios.
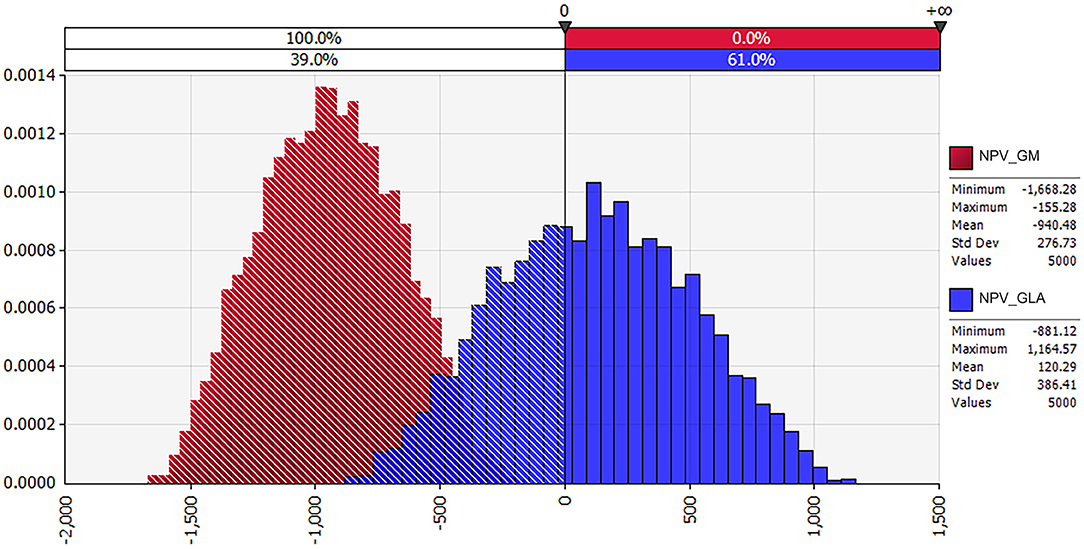
Figure 3. Probability density distributions for NPV for the grass-legume association and the grass monoculture. GLA, Grass-legume association; GM, Grass monoculture.
Sensitivity Analysis
Figure 4 shows the contribution of different input variables to the NPV variance as result of the simulation. These graphs represent the correlation that each input variable simulated in the model has with the NPV profitability indicator. As can be seen, the profitability of the treatments measured by the NPV indicator is highly sensitive to changes in the milk production variable for both treatments. The correlation between the NPV indicator and the milk production variable is positive, which means that changes in daily production affect the indicator by more than 90% for both treatments. This results from the high dependence on the income generated from milk production and the productivity levels in this system.
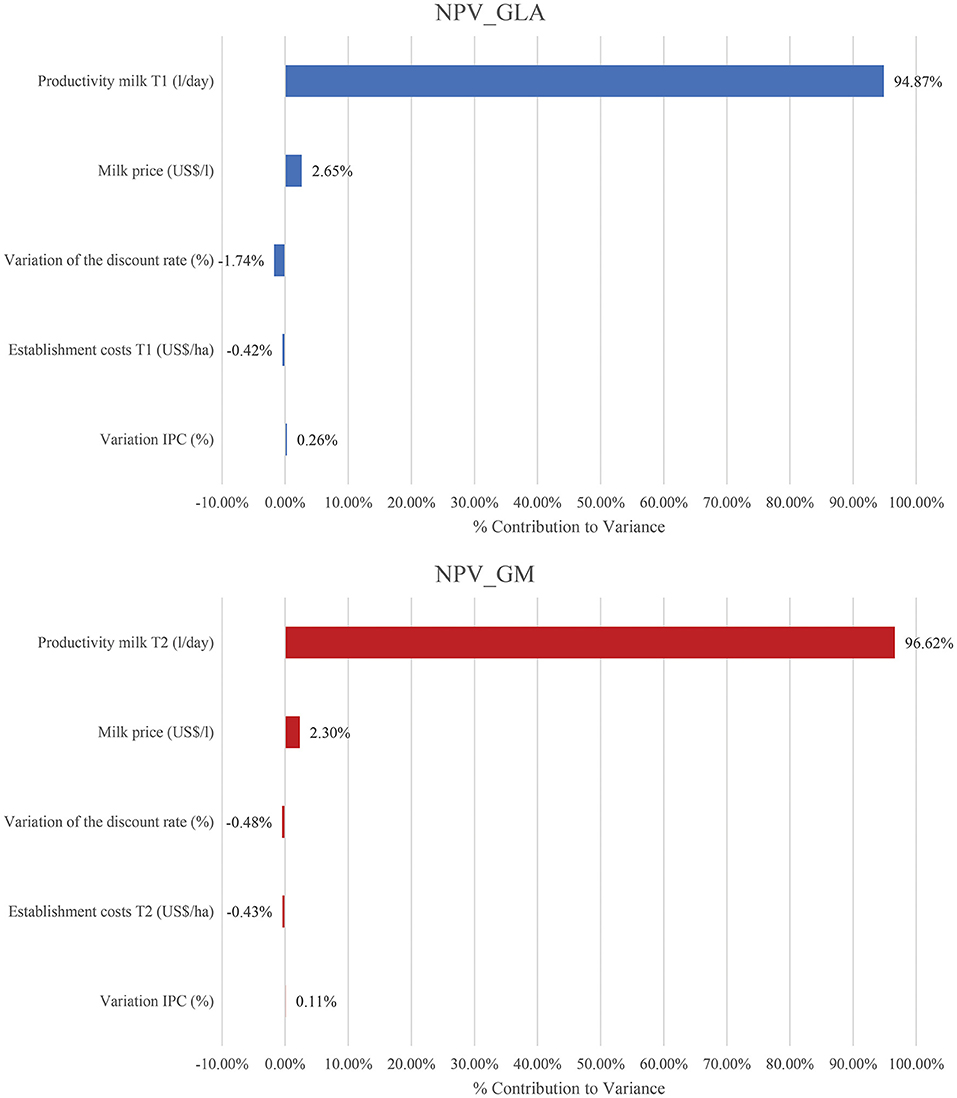
Figure 4. Contribution to the NPV variance for the grass-legume association and the grass monoculture. GLA, Grass-legume association; GM, Grass monoculture.
Economic Evaluation Under Climate Change Scenarios RCP 2.6 and 8.5
Forage Production Under Climate Change Scenarios
The estimates for dry matter production for the evaluated climate change scenarios are presented in Figure 5. According to the results, changes in the climatic variables in the RCP scenarios have notable impacts on the productivity of both treatments. For the grass-legume association, biomass production was reduced, on average, by 7.74 and 16.62% under RCP 2.5 and 8.5, respectively. For the grass monoculture, the reductions were 14.95 and 35.27% under RCP 2.5 and 8.5, respectively. The most influential environmental factors on productivity were average temperature and bio32 for the grass-legume association and precipitation73 and bio3 for the grass monoculture.
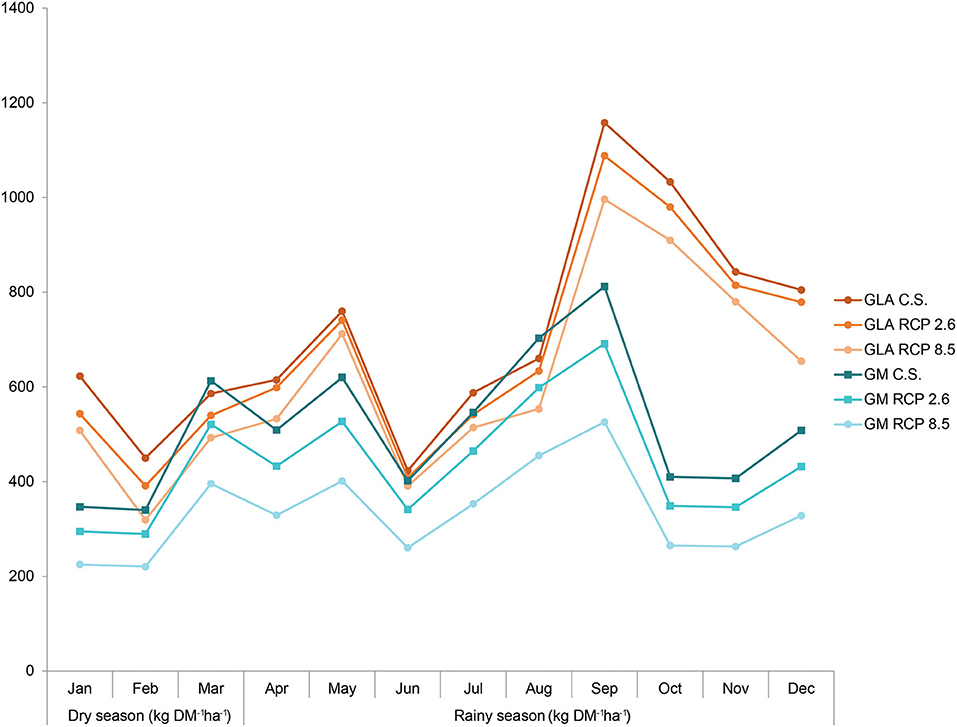
Figure 5. Dry Matter (DM) production under climate change scenarios (RCP 2.6 y 8.5) and Current Scenario (C.S). GLA, Grass-legume association; GM, Grass monoculture.
In addition to the possible changes at the productive level of Arachis, projected changes in environmental conditions under climate change scenarios can alter its potential distribution. According to the results of the Maxent model, under RCP 2.6 (until 2050) a shift of suitable areas for Arachis toward higher altitudes would occur (Figure 6), meaning a decrease in their suitability for lower altitudes like the foothills or savannas. Under RCP 8.5, in addition to the described shift of suitable areas, a total reduction of the potential area for Arachis would occur (Figure 6).
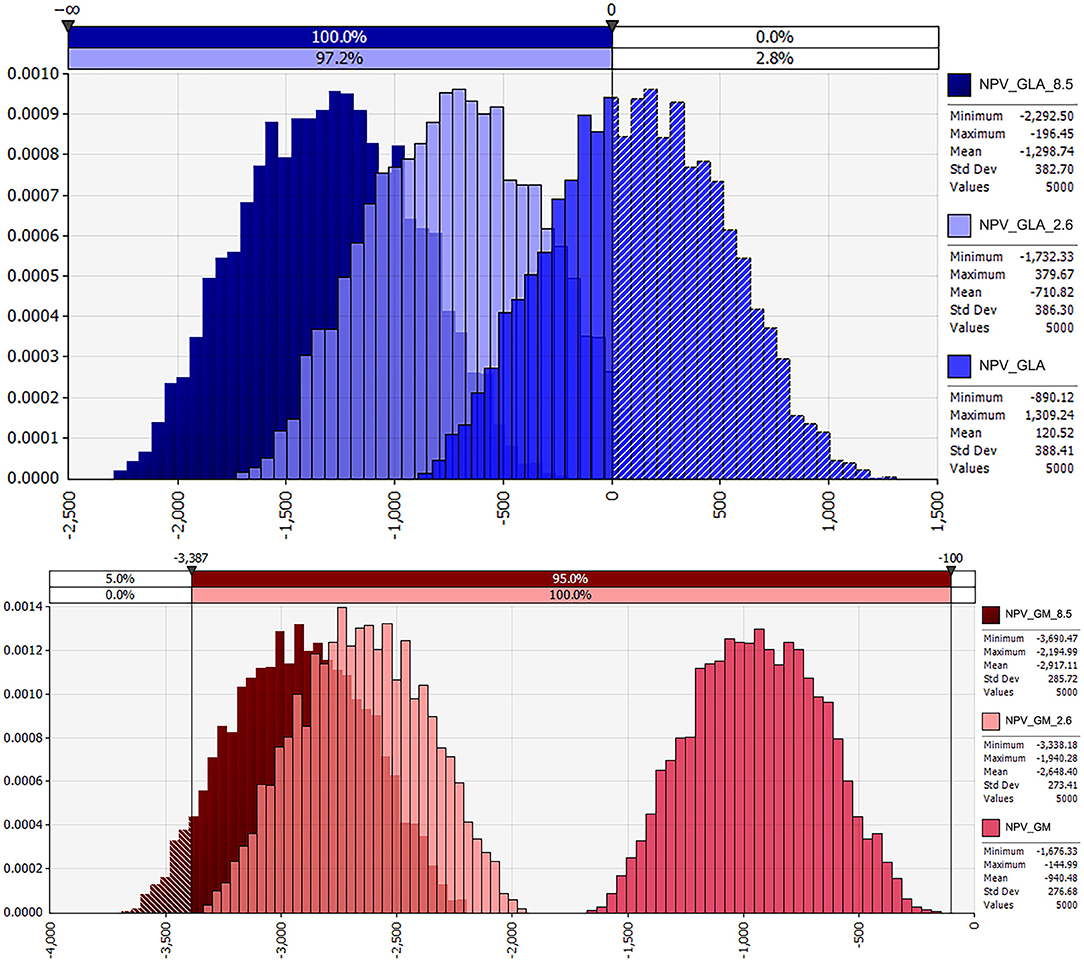
Figure 6. Estimated change in suitable areas for Centauro by 2050 under RCP 2.6 (left) and RCP 8.5 (right).
Milk Production Under Climate Change Scenarios
The effects of the climate change scenarios on forage biomass production translate into a strongly marked decrease in milk production for both treatments (Table 6). For the grass-legume association, during the first 3 months of the year (dry season) and compared to the current scenario, a decrease of close to 23% would occur under RCP 2.6 and 33% under RCP 8.5, respectively. These reductions are less marked during the rainy season but are still relevant for the low production volumes under this system. In the case of the grass monoculture, the change in climatic variables would cause a reduction of milk production during dry season of 63 and 67% for RCP 2.6 and RCP 8.5, respectively. During rainy season, the effect on productivity would be above 40% for both RCP scenarios.
Profitability Indicators Under Climate Change Scenarios
The reduced milk production under both climate change scenarios leads to a leftward shift of the distribution curves for the NPV indicator in both treatments, when compared to the current scenario (Figure 7). The net income is reduced by 60 and 90% for the grass-legume association, and 113 and 131% for the grass monoculture under the scenarios RCP 2.6 and RCP 8.5, respectively. Although including Centauro in the system has highly positive effects at the productive level and therefore on the economic performance, both climate change scenarios would affect the system in such a strong way that the investment in any of the treatments would not be profitable.
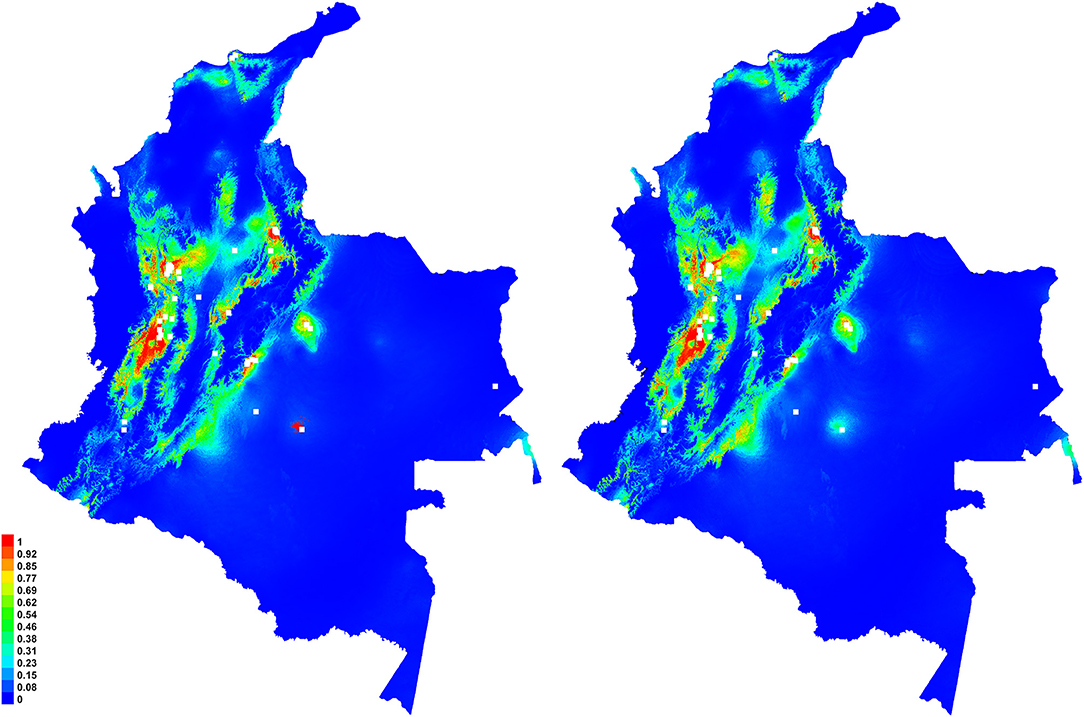
Figure 7. NPV probability distribution for the grass-legume association and the grass monoculture under climate change scenarios. GLA, Grass-legume association; GM, Grass monoculture.
Discussion
The inclusion of the legume Arachis pintoi CIAT 22160 cv. Centauro in a monoculture of Brachiaria humidicola cv. Humidicola allows an improvement in the technical parameters of the production system and results in better economic indicators. At the productive level, this association increased daily milk production by 14% on average and the animal stocking rate by 33% compared to the monoculture. This results from the higher crude protein content of the diet (39%), the higher dry matter production (14%) and the lower proportion of Acid Detergent Fiber that favors digestibility and, therefore, a better use of the available forage (Rincón et al., 2020). The higher milk production level in turn helps to improve the financial indicators of the association compared to the monoculture base scenario.
These results (i.e., the increased milk productivity by 52% and the related increased income from milk sales by 58%) are consistent with (and even surpass the results of) different studies that have evaluated the potential of Arachis pintoi accessions (mainly CIAT 17434) in integrated grass-legume systems for livestock production in the tropics. These studies highlight, in comparison with monoculture pastures, improvements in both forage quantity and quality, a strong compatibility with aggressive Brachiaria species, as well as higher meat and milk production levels and stocking rate (Peters et al., 2011; Crestani et al., 2013; Pereira et al., 2019; Boddey et al., 2020; Villegas et al., 2020). Other studies show average increases in milk production of 31% in Colombia (Rivas and Holmann, 2000), 7 and 11.4% in Costa Rica (Peters et al., 2001; Romero and González, 2004), and 20% in Peru (Lara and Reategui, 2004). In addition to milk yield increases, Romero and González (2004) found differences regarding the milk composition: both the milk protein (3.66 vs. 3.54%) and total solid contents (13.89 vs. 13.73%) were higher in a grass-legume association with Arachis pintoi than in a grass monoculture with Brachiaria. Regarding the animal stocking rate, the reported increases are between 33 and 50% in Colombia (1.5-2 AU ha−1 vs. 1 AU ha−1; Holmann, 2004), 29% in Brazil (2.26 vs. 1.6; Vasques et al., 2019), 50% in the Peruvian Amazon (4.13 vs. 2.07; Lara and Reategui, 2004), and 25% in Costa Rica (4.6 vs. 3.7; Romero and González, 2004). Other studies highlight successful cases of early adoption of Arachis accessions in livestock systems, e.g., in western Brazil (Valentim and Andrade, 2005), in the Colombian Caquetá Department (Lascano et al., 2005) and in northern Costa Rica (Wunscher et al., 2004), suggesting the relevance of, and the potential for dissemination, across different regions, for the technology evaluated in our study.
The improvements in the economic indicators resulting from the inclusion of Centauro are also associated with improvements in the risk indicators. The probability of obtaining economic loss was reduced from 100 to 39.1%, for example. The sensitivity analysis shows that the daily milk production variable has the highest impact on the economic performance indicators. The monoculture is more sensitive to small reductions in milk production as when associated with Centauro. Changes of just 1% in milk production lead, however, to changes in profitability indicators of more than 90% in both systems. Since different empirical studies have shown that risk factors (perception of risk about future returns from implementing a new technology, and level of risk aversion of the producer) are determining factors in technology adoption (e.g., Marra et al., 2003; van Winsen et al., 2014; Trujillo-Barrera et al., 2016), there is reason to believe that the lower risk levels resulting from the inclusion of Centauro in the cattle production system will enhance technology adoption.
The inclusion of different climate change scenarios (RCP 2.6 and RCP 8.5) in our models revealed the substantial impact that climatic variables have on forage production, both in terms of geographic distribution and available forage biomass. Until 2050, the available forage biomass would reduce in both systems, the grass monoculture and the grass-legume association. For the latter, however, reductions would be of a lower magnitude (maximum reduction of 16.6 vs. 35.3% for the monoculture). The highest losses are to be expected during dry season from January to March. These effects on forage productivity are the result of a combination of increased temperatures, variations in precipitation levels and atmospheric CO2 concentrations caused by climate change (Thornton et al., 2009; Rojas-Downing et al., 2017). Not only would forage productivity be affected if the favorable environmental conditions changed but also the potential distribution of Centauro and other Arachis varieties. Centauro would migrate to higher altitudes more favorable for its development and the overall potential area for distribution would decrease. This could pave the way for the arrival of new (invasive) species or native grasses with a better adaptation capacity to the conditions projected for 2050. Although in our model we only made projections for Centauro, the impacts of climate change in the Orinoquía would also affect the distribution, quantity and quality of other forage species by up to 60% according to estimates provided by CIAT CORMACARENA (2018), putting livestock production in a difficult position.
Although the modeled impacts from the climate change scenarios were relevant for both alternatives, the grass monoculture and the grass-legume association, the latter shows a better adaptation capacity. This can be attributed to the symbiotic effect between the legume and the grass associated with the contribution of nitrogen-fixing (Dubeux et al., 2017; Pereira et al., 2019; Villegas et al., 2020). The higher availability of N improves both the yields and persistence of the grass, and comes with the co-benefit of mitigating GHG emissions through reducing (i) methane emissions (as a result of an improved diet), and (ii) synthetic fertilizer use (resulting in lower N2O emissions). Simultaneously, Arachis has positive impacts on the physical and chemical properties of the soil, and contributes to increasing both soil microfauna and organic matter (Schultze-Kraft et al., 2018). Arachis accessions in particular, offer dense soil cover and, therefore, prevent soil erosion problems (Schultze-Kraft et al., 2018).
The effects of climate change on forage biomass production would lead to a strong decrease in milk production in both systems. The grass-legume would, however, be less affected (-19%) than the grass monoculture (−56%). This in turn would lead to significant economic losses in the dual-purpose production systems in the region. Given the low productivity levels and values of technical indicators in dual-purpose livestock systems, profitability margins are inherently sensitive to small changes in production levels. The effects of climatic variations on livestock production have been identified in the literature as one of the main impacts (e.g., Garnett, 2009; Thornton et al., 2009; Nardone et al., 2010; Henry et al., 2012). The severity or level of the impact varies significantly between regions, however (Rojas-Downing et al., 2017). For the Orinoquía region, CIAT CORMACARENA, 2018 predict that the impact of climate change would lead to significant losses in cattle live weight gains and dairy production, and lower birth and increased mortality rates. As mentioned in the methodology, our study only considers changes in dairy production as a result of the impacts of climate change on pasture productivity. Production could also be affected, however, by other possible effects not considered in our study, such as effects on health, growth and reproduction, water availability, and the distribution of pests and diseases (Garnett, 2009; Thornton et al., 2009; Rojas-Downing et al., 2017). In particular, periods of heat stress could become the main source of loss at the productive level in the livestock sector (Garnett, 2009; Nardone et al., 2010). This scenario does not, however, consider potential technological changes nor the inclusion of other (new) species better adapted to the predicted regional climate change scenarios. Likewise, our study does not include any potential benefits that might be derived from culled cows on the beef market.
Despite the benefits of including Arachis pintoi and other legumes in cattle systems, adoption levels remain low. Several studies have identified some of the factors that limit the adoption of Arachis accessions in countries such as Colombia and Costa Rica, including a lack of commercial seed availability, high establishment costs of planting material, limited technical information on the establishment and management of the material in pastures, a lack of promotion and little knowledge about its benefits (CIAT, 2004; Wunscher et al., 2004; Lascano et al., 2005). A particularly important issue is seed supply, which also continues to be a restriction in the dissemination and adoption processes for the new Centauro accession. In this sense, it is necessary to develop focused strategies, for example, artisanal seed production by cattle producers, as this could not only contribute to generating higher technology adoption levels. Focused strategies could also play an important role in providing additional income, assuring income diversification, and opening new business alternatives for young people and women. In the long run, this would strongly contribute to supporting both the rural economy and sustainable intensification processes in the region. In addition, the increased demand and adoption of the legume could generate interest from the private sector for seed production.
In addition to the above-mentioned limiting factors, there are structural conditions that could slow down or discourage sustainable intensification. The prevailing tradition of extensive production systems and low land prices, for example, make it more efficient to acquire more (new) land than to intensify existing land (White et al., 2001). In particular, in regions such as the Orinoquía, where land is relatively abundant and are prices low, producers continue to favor more extensive systems at the cost of deforestation processes. Even if the costs of implementing new technologies are below land prices, cattle producers may not reduce the area, since one of the main reasons for land expansion is to secure land ownership rights (Kaimowitz and Angelsen, 2008). This may be favored by speculation processes in land prices, where a high price generates additional incentives for extension, given the increase in the value of capital gains (Smith et al., 1997). These speculation processes could also, however, promote intensification if the amount of land that can be acquired is reduced, for example by regulations (Smith et al., 1997). Unfortunately, in most cases producers may not be willing to intensify until land is scarce and most forests are gone (Kaimowitz and Angelsen, 2008). Similarly, if producers have few alternatives to invest their savings other than cattle production, this can contribute to the expansion of pasture areas. This situation is further aggravated by the precarious controls on land tenure and the lack of monitoring and control regarding the expansion of the agricultural frontier. Positive advances have been documented in Costa Rica, where the agricultural frontier cannot be expanded any further as a result of the little remaining forest area and high land prices, forcing cattle producers to use their land more efficiently, e.g., through incorporating Arachis pintoi in their pastures and adopting Cratylia protein banks (White et al., 2001). The opposite was documented in Peru, where land is still abundant and cheap, and market access is limited. Producers failed to adopt legumes such as Arachis in Peru, given the higher level of investment required (White et al., 2001).
Intensification strategies in the Orinoquía have been a subject of debate, mainly in environmental terms, since the introduction improved forages (Brachiaira species) in the 1970s. On the one hand, different studies have reported positive impacts associated with intensification processes with improved forages, such as (i) lower incidence of degradation of native savannas in intensified areas with improved forages, since they reduce the pressure to produce animal feed in the native savannas (Smith et al., 1997); (ii) reduction of greenhouse gas emissions associated with burning native savannas (Smith et al., 1997), carried out to increase grassland and savanna productivity in the short term at the expense of eliminating vegetation cover and nutrient availability in the long-term (Peñuela et al., 2014); and (iii) reduction of nitrous oxide emissions, associated with Biological Nitrification Inhibition (BNI) in Brachiaria humidicola pastures (Subbarao et al., 2009, 2017; Moreta et al., 2014). On the other hand, however, negative effects have also been reported and include (i) the loss and degradation of native savannas and threats to biodiversity (decrease in bird, animal and fish species), with gallery forests being the ecosystems under the greatest threat in the most intensified areas (Smith et al., 1997); (ii) increases in deforestation levels to expand grazing areas with introduced forages and, therefore, compromising ecosystem stability and functions (e.g., altering microclimates and shifting the rates of consumption and supply of light, water and mineral nutrients), and increasing greenhouse gas emissions due to land-use changes (Williams and Baruch, 2000; Reid et al., 2010; Peñuela et al., 2014; CIAT CORMACARENA, 2018); (iii) a displacement of native species given the aggressive growth characteristics, invasive behavior and fire resistance of Brachiaria species, particularly Brachiaria humidicola in savannas and highlands (Peñuela et al., 2014); (iv) increased soil erosion processes (Peñuela et al., 2011); and (v) increased frequency and intensity of fires due to establishment and management processes and the large standing necro mass left by grasses of African origin (such as Brachiaria) at the end of the dry season that facilitate the combustion (Williams and Baruch, 2000). In the case of the new Centauro variety, although its introduction into livestock systems provides a strategy to restore degraded areas, improve productivity and provide ecosystem services, it could also be a technology that promotes deforestation processes and has negative impacts on protected ecosystems. The higher profitability associated with new technologies, such as Centauro, could, for example, lead producers to increase their herd size and hence their pasture area. Likewise, profitable technologies can also provide farmers with the additional capital they need to finance livestock expansion (Kaimowitz and Angelsen, 2008).
In this sense, diffusion and adoption processes of new technologies like Centauro must be accompanied by land use governance and management policies. These policies require a multidimensional approach that includes the development of coordinated land tenure security policies, specific economic incentives aimed at promoting sustainable intensification (e.g., special credit lines, conservation requirements to access benefits or credits), integrated planning and zoning of land use, protection of forests and ecosystems, and tracking and monitoring of land use change, particularly at the agricultural frontier. This also implies greater institutional coordination and coordination between national policies (e.g., related to land use, agriculture, rural development), partnerships between the public and private sectors, and local communities that increase the effectiveness of policies and other instruments. Brazil for example, has reported a notable reduction in deforestation rates in the Amazon region, which has been the result of a combination of multiple public and private mechanisms for the protection of forests (FAO, 2016). For example, the new Brazilian Forest Code (Federal Law No. 12,651/2012; Presidência da República, 2012) obliges rural land owners to submit data with geographic coordinates for the registration of private rural properties, certify their intention to comply environmental regulations, and in cases where this does not occur, land owners are subject to administrative, civil or criminal processes and charges. Commercial banks are required (in accordance with the Forest Code) to request rural land owners and holders to provide a registration certificate from the Rural Environmental Registry before granting loans for agricultural purposes. Zero deforestation agreements for livestock signed by major beef companies have helped in reducing deforestation in certain parts of Brazil (Gibbs et al., 2015) and the Brazil Green Bag Initiative (Presidência da República, 2012) is a conditional cash transfer program with a commitment to responsibly manage resources and conserve ecosystems (FAO, 2016).
Conclusions
The results of this study suggest that integrating the legume Arachis pintoi CIAT 22160 cv. Centauro in a Brachiaria humidicola cv. Humidicola monoculture has great potential to improve both productive and economic indicators in the dual-purpose cattle production system of the Orinoquía region. Not only that, Centauro also helps in generating important ecosystem services with positive effects on, for example, the quality and persistence of the associated grass (restoration of degraded pastures), the soil system and biodiversity. Centauro improves the system's resilience to climatic variations, which is especially important considering the rather pessimistic climate projections for the region. These attributes make the inclusion of Centauro in the production system a key alternative for sustainable intensification in the region, and thus also contributes to achieving other environmental objectives such as the liberation of areas for reforestation purposes or the protection of local ecosystems.
It is important to mention, however, that, despite their numerous environmental benefits (see section Potential Ecosystem Services of Arachis pintoi CIAT 22160 cv. Centauro) and because of their economic and social benefits, forage technologies that are selected for intensification purposes (even if sustainable such as Centauro), bear a risk of misuse in regions and contexts where neither grasses nor legumes should be planted. This could lead to results contrary to the objectives of sustainable intensification and could therefore negatively affect local landscapes with significant ecological consequences. In the case of the Orinoquía region, this includes for example the promotion of deforestation or the penetration of important local ecosystems (such as native flooded savannas). The Orinoquía has a high degree of vulnerability to changes generated by human actions, which include transformations of productive models that are ignorant of the natural cycles threatening the ecosystem balance. The continuous search for productivity increases has led to significant changes in the productive models of the region, including, for example, the introduction of improved pastures in floodable savannas (Peñuela et al., 2011). This situation is likely to worsen considering the imminent effects of climate change, and threatens the savanna ecosystem as it could become subject to desertification processes as a consequence of inadequate natural resource management (Peñuela et al., 2011). To avoid such unwanted consequences, effective technology diffusion approaches need to be applied (which include extension and training programs), involving institutions relevant to the region within a context that helps to close information and monitoring gaps. In the case of Centauro, the focus of such information efforts should be on the correct establishment and management of the legume, highlighting both potential economic benefits and environmental threats. This needs to go hand in hand with strong inter- and intra-institutional coordination, and the development of public policies and comprehensive monitoring and control mechanisms. National and regional multi-stakeholder platforms, such as the Colombian Roundtable for Sustainable Beef and Dairy (MGS) and its regional sub-roundtables, can fill some of the gaps—at least in short- to medium-term, e.g., through providing targeted information campaigns and trainings or developing indicators and frameworks for sustainable intensification of the sector. In the long term, however, and based on the abovementioned efforts of multi-stakeholder platforms, comprehensive public policies need to be developed, applied and monitored.
Accelerating climate change will also affect the Orinoquía region. Our study suggests that variations in the local climatic conditions would have significant impacts on the economic viability of the dual-purpose cattle systems of the region. It is necessary, therefore, to implement regional climate change adaptation and mitigation measures that include specific strategies for the local context. Among others, animal breeding strategies that improve cattle by crossing with rustic breeds, silvo-pastoral systems or scattered trees in pastures for heat stress reduction, and water harvesting for animal consumption, can significantly increase the adaptation potential of dual-purpose systems, particularly during dry seasons.
Data Availability Statement
The data analyzed in this study is subject to the following licenses/restrictions: Data was retrieved from another research project and is not publicly available. Requests to access these datasets should be directed to cy5idXJrYXJ0QGNnaWFyLm9yZw==.
Author Contributions
SB, KE, and DR: conceptualization and methodology. KE, DR, and ÁR: formal analysis. KE, DR, ÁR, and SB: writing the original draft, review, editing, and resources. SB: supervision, funding acquisition, and project administration. All authors contributed to the article and approved the submitted version.
Funding
This work was funded by the CGIAR Research Program on Livestock and by the Colombian Ministry of Agriculture and Rural Development (MADR). The funders had no role in the design of the study; in the collection, analyses, or interpretation of data; in the writing of the manuscript, or in the decision to publish the results.
Author Disclaimer
The views expressed in this document may not be taken as the official views of these organizations.
Conflict of Interest
The authors declare that the research was conducted in the absence of any commercial or financial relationships that could be construed as a potential conflict of interest.
Publisher's Note
All claims expressed in this article are solely those of the authors and do not necessarily represent those of their affiliated organizations, or those of the publisher, the editors and the reviewers. Any product that may be evaluated in this article, or claim that may be made by its manufacturer, is not guaranteed or endorsed by the publisher.
Acknowledgments
This work was carried out as part of the CGIAR Research Program on Livestock. We thank all donors who globally support our work through their contributions to the CGIAR System. CGIAR is a global research partnership for a food-secure future. Its science is carried out by 15 Research Centers in close collaboration with hundreds of partners across the globe. This work was conducted as part of the project Materiales forrajeros y estrategias de utilización y manejo, para mejorar la producción de Carne y leche en los sistemas ganaderos de la Orinoquía Colombiana, conducted under the cooperation agreement between MADR, AGROSAVIA (previously CORPOICA) and CIAT and funded by MADR. Additionally, this work was part of the project Evaluación multilocacional de nuevo germoplasma forrajero, conducted under the cooperation agreement between AGROSAVIA and CIAT under the macroproject Incremento de la oferta forrajera a través de la liberación de nuevos materiales y el desarrollo de estrategias integrales de manejo para aumentar la competitividad de la ganadería en Colombia, funded by MADR.
Footnotes
1. ^FEDEGAN: Federación Colombiana de Ganaderos, Colombian Cattle Federation.
2. ^bio3 is a percentage indicator that shows the variability of the diurnal temperature range with respect to the annual temperature range. Bio3 = (Bio2/Bio7)*100; Where: Bio2 = Mean Diurnal Range [Mean of monthly (max temp – min temp)] and Bio7 = Temperature Annual Range (Max Temperature of Warmest Month – Min Temperature of Coldest Month).
3. ^precipitation 7 is a continuous variable representing the amount of rainfall per m2 at a geographic point during the seventh month of the year (July).
References
Abril, D. S., Melo, L. F., and Parra, D. (2017). “Impactos de los fenómenos climáticos sobre el precio de los alimentos en Colombia,” in El desarrollo equitativo, competitivo y sostenible del sector agropecuario en Colombia, eds C. G. Cano, A. M. Iregui, M. T. Ramirez, and A. M. Tribin (Caracas: Banco de Desarrollo de América Latina), 315–347.
Amézquita, M. C., Ibrahim, M., Llanderal, T., Buurman, P., and Amézquita, E. (2004). Carbon sequestration in pastures, silvo-pastoral systems and forests in four regions of the latin American tropics. J. Sustain. For. 21, 31–49. doi: 10.1300/J091v21n01_02
Armenta, G., Dorado, J., Rodríguez, A., and Ruiz, J. (2015). Escenarios de cambio climático para precipitación y temperaturas en Colombia. Instituto de Hidrología, Meteorología y Estudios Ambientales de Colombia IDEAM. Available online at: http://documentacion.ideam.gov.co/cgi-bin/koha/opac-detail.pl?biblionumber=37489 (accessed January 15, 2021).
Bancolombia–Dirección de Investigaciones Económicas, Sectoriales y de Mercados. (2020). Actualización de proyecciones económicas para Colombia–segundo trimestre de 2020. Available online at: https://www.shorturl.at (accessed February 05, 2020).
Boddey, R. M., Casagrande, D. R., Homem, B. G. C., and Alves, B. J. R. (2020). Forage legumes in grass pastures in tropical Brazil and likely impacts on greenhouse gas emissions: a review. Grass Forage Sci. 75, 1–15. doi: 10.1111/gfs.12498
Cárdenas, E. A., Maass, B. L., Peters, M., and Franco, L. H. (1999). Evaluación de germoplasma nuevo de Arachis pintoi en Colombia. 2. Bosque muy húmedo–Premontano (Zona cafetera), Caldas. Past. Trop. 21, 42–59.
Castillo-Gallegos, E., Mannetje, L., and Aluja-Schunemann, A. (2005). Production and persistence of a native pasture-Arachis pintoi association in the humid tropics of Mexico. Trop. Grassl. 39. Available online at: https://www.tropicalgrasslands.info/index.php/tgft/pages/view/tropicalGrasslands
CIAT (1994). Programa de Forrajes Tropicales-Informe Anual 1994. Cali: Centro Internacional de Agricultura Tropical (CIAT). Available online at: https://cgspace.cgiar.org/handle/10568/69061?show=full (accessed April 21, 2021).
CIAT (2002). Tropical Grasses and Legumes: Optimizing genetic Diversity for Multipurpose Use (Project IP5). Cali: Centro Internacional de Agricultura Tropical (CIAT). Available online at: hdl.handle.net/10568/69061 (accessed September 14, 2021).
CIAT (2004). Tropical Grasses and Legumes: Optimizing genetic Diversity for Multipurpose Use (Project IP5). Annual Report 2004. Cali: Centro Internacional de Agricultura Tropical. Available online at: hdl.handle.net/10568/69061 (accessed September 14, 2021).
CIAT and CORMACARENA (2018). Plan regional integral de cambio climático para la Orinoquía. CIAT publicación No. 438. Cali: Centro Internacional de Agricultura Tropical (CIAT).
Crestani, S., Mendonça, H., Ribeiro, F. N., Frederico, M. M., Almeida, E. X., and Portela, F. A. (2013). Steers performance in dwarf elephant grass pastures alone or mixed with Arachis pintoi. Trop. Anim. Health Prod. 45, 1369–1374. doi: 10.1007/s11250-013-0371-x
DANE (2020a). Data from: Productividad Total de los Factores (PTF). Available online at: https://www.dane.gov.co/index.php/estadisticas-por-tema/cuentas-nacionales/productividad (accessed September 14, 2021).
DANE (2020b). Data from: Índice de precios al Productor (IPP). Available online at: https://www.dane.gov.co/index.php/estadisticas-por-tema/precios-y-costos/indice-de-precios-del-productor-ipp (accessed September 14, 2021).
DANE (2020c). Data from: Índice de precios al consumidor (IPC). Available online at: https://www.dane.gov.co/index.php/estadisticas-por-tema/precios-y-costos/indice-de-precios-al-consumidor-ipc/ipc-historico (accessed September 14, 2021).
Dickie, A., Streck, C., Roe, S., Zurek, M., Haupt, F., and Dolginow, A. (2014). Strategies for Mitigating Climate Change in Agriculture: Abridged Report. Climate Focus and California environmental Associates, Prepared With the Support of the Climate and Land Use Alliance. Available online at: https://www.climateandlandusealliance.org/reports/strategies-for-mitigating-climate-change-in-agriculture/ (accessed December 20, 2020).
Dubeux, J. C. B., Blount, A. R. S., Mackowiak, C., Santos, E. R. S., Pereira-Neto, J. D., Riveros, U., et al. (2017). Biological N2 fixation, belowground responses, and forage potential of rhizoma peanut cultivars. Crop Sci. 57, 1027–1038. doi: 10.2135/cropsci2016.09.0810
FAO (2016). El Estado de los bosques del mundo 2016. Roma: Los bosques y la agricultura: desafíos y oportunidades en relación con el uso de la tierra.
FEDEGAN (2003). Índice de costos ganaderos diciembre 2002–marzo 2003. Carta Fedegán Magazine No. 79. Bogotá: Federación Colombiana de Ganaderos, 17–32.
Fisher, M. J., Rao, I. M., Ayarza, M. A., Lascano, C. E., Sanz, J. I., Thomas, R. J., et al. (1994). Carbon storage by introduced deep-rooted grasses in the South American savannas. Nature 371, 236–238. doi: 10.1038/371236a0
Fonte, S. J., Nesper, M., Hegglin, D., Velásquez, J. E., Ramirez, B., Rao, I. M., et al. (2014). Pasture degradation impacts soil phosphorus storage via changes to aggregate-associated soil organic matter in highly weathered tropical soils. Soil Biol. Biochem. 68, 150–157. doi: 10.1016/j.soilbio.2013.09.025
Galdino, S., Sano, E. E., Andrade, R. G., Grego, C. R., Nogueira, S. F., Bragantini, C., et al. (2015). Large-scale modeling of soil erosion with rusle for conservationist planning of degraded cultivated Brazilian pastures. Land Degrad. Dev. 27, 773–784. doi: 10.1002/ldr.2414
Garnett, T. (2009). Livestock-related Greenhouse Gas Emissions: impacts and options for policy makers. Environ. Sci. Policy 12, 491–503. doi: 10.1016/j.envsci.2009.01.006
GBIF.org (2020). GBIF Occurrence. Available online at: https://doi.org/10.15468/dl.wvj4tj (accessed June 02, 2020).
Gibbs, H. K., Munger, J., L'Roe, J., Barreto, P., Pereira, R., Christie, M., et al. (2015). Did ranchers and slaughterhouses respond to zero deforestation agreements in the Brazilian Amazon? Conserv. Lett. 9, 32–42. doi: 10.1111/conl.12175
Henry, B., Charmley, E., Eckard, R., Gaughan, J. B., and Hegarty, R. (2012). Livestock production in a changing climate: adaptation and mitigation research in Australia. Crop Past. Sci. 63, 191–202. doi: 10.1071/CP11169
Holmann, F. (2004). “Potential benefits of new forage germplasm in dual-purpose cattle systems in the dry tropics,” in Feeding Systems With Forage Legumes to Intensify dairy Production in Latin America and the Caribbean-A Project Executed by the Tropileche Consortium, eds F. Holmann and C. Lascano (https://www.google.com/search?sxsrf=AOaemvIjDC_c2FIZ_-CAz1wW7vhQfNP8DA:1631316354048&q=Cali,+Colombia&stick=H4sIAAAAAAAAAOPgE-LUz9U3MDFPtyxWAjMNC4qL07SMMsqt9JPzc3JSk0sy8_P084vSE_MyqxJBnGKrjNTElMLSxKKS1KJihZz8ZLDwIlY-58ScTB0F5_yc_NykzMQdrIwA8gbAbWAAAAA&sa=X&ved=2ahUKEwir_bH-xvXyAhUbOisKHfaTDKsQmxMoAXoECC4QAw Cali: Centro Internacional de Agricultura Tropical-CIAT), 114–131.
ICA (1987). Pasto Llanero Brachiaria dictyoneura. Boletín técnico No. 151. Villavicencio: ICA. Available online at: http://ciat-library.ciat.cgiar.org/forrajes_tropicales/Released/Materiales/PastoLlaneroC.pdf (accessed December 15, 2020).
IDEAM PNUD, MADS, DNP, and Cancillería. (2015). Nuevos escenarios de cambio climático para Colombia 2011–2100 Herramientas Científicas para la Toma de Decisiones–Enfoque Nacional–Departamental: Tercera Comunicación Nacional de Cambio Climático. Bogotá: Instituto de Hidrología, Meteorología y Estudios Ambientales de Colombia (IDEAM).
Jansen, H. G. P., Ibrahim, M. A., Nieuwenhuyse, A., 't Mannetje, L., Joenje, M., and Abarca, S. (1997). The economics of improved pasture and silvopastoral technologies in the Atlantic zone of Costa Rica. Trop. Grassl. 31, 588–598.
Jensen, E. S., Peoples, M. B., Boddey, R. M., Gresshoff, P. M., Hauggard-Nielsen, H., et al. (2012). Legumes for mitigation of climate change and the provision of feedstock for biofuels and biorefineries. a review. Agron. Sustain. Dev. 32, 329–364. doi: 10.1007/s13593-011-0056-7
Kaimowitz, D., and Angelsen, A. (2008). Will livestock intensification help save latin America's tropical forests? J. Sustain. For. 27, 6–24. doi: 10.1080/10549810802225168
Lara, D., and Reategui, K. (2004). “Effect of associating Brachiaria brizantha with Arachis pintoi on milk yield,” in Feeding Systems With Forage Legumes to Intensify Dairy Production in Latin America and the Caribbean: A Project Executed by the Tropileche Consortium, eds. F. Holmann and C.E. Lascano (Cali: Centro Internacional de Agricultura Tropical, Tropileche Consortium; Addis Ababa: System-wide Livestock Programme; Nairobi: International Livestock Research Institute), 67–68.
Lascano, C. (1994). “Nutritive value and animal production of forage Arachis,” in Biology and Agronomy of Forage Arachis, eds P. C. Kerridge and B. Hardy (Cali: Centro Internacional de Agricultura Tropical (CIAT)), 109–121.
Lascano, C. E., Peters, M., and Holmann, F. (2005). Arachis pintoi in the humid tropics of Colombia: a forage legume success story. Trop. Grassl. 39:220. Available online at: https://www.tropicalgrasslands.info/index.php/tgft/pages/view/tropicalGrasslands (accessed April 17, 2021).
León-Velarde, C., Cañas, R., Quiroz, R., Osorio, J., Guerrero, J., and Pezo, D. (2006). LIFE-SIM: Livestock Feeding Strategies Simulation Models. Lima: International Potato Center (CIP). https://bit.ly/3nBVWVD (Accessed December, 12, 2020).
MADR/USP (2020). Precio pagado al productor Res 0017 de 2002. Available online at: http://uspleche.minagricultura.gov.co/ (accessed June 12, 2020).
Marra, M., Pannell, D. J., and Abadi Ghadim, A. (2003). The economics of risk, uncertainty and learning in the adoption of new agricultural technologies: where are we on the learning curve? Agric. Syst.75, 215–234. doi: 10.1016/S0308-521X(02)00066-5
Melo, S., Riveros, L., Romero, G., Álvarez, A., Diaz, C., and Calderón, S. (2017). Efectos económicos de futuras sequías en Colombia: Estimación a partir del Fenómeno El Niño 2015. Arch. Econ. 466, 1–34. Available online at: https://colaboracion.dnp.gov.co/CDT/Estudios%20Econmicos/466.pdf (accessed September 14, 2021).
Moreno, I. R., Maass, B. L., Peters, M., and Cárdenas, E. A. (1999). Evaluación de germoplasma nuevo de Arachis pintoi en Colombia. 1. Bosque seco tropical, Valle del Cauca. Past. Trop. 21:18–32. Available online at: https://www.tropicalgrasslands.info/index.php/tgft/pages/view/Pasturas
Moreta, D. E., Arango, J., Sotelo, M., Vergara, D., Rincón, A., Ishitani, M., et al. (2014). Biological nitrification inhibition (BNI) in Brachiaria pastures: a novel strategy to improve eco-efficiency of crop-livestock systems and to mitigate climate change. Trop. Grassl. 2, 88–91. doi: 10.17138/TGFT(2)88-91
Murgueitio, E., Calle, Z., Uribe, F., Calle, A., and Solorio, B. (2011). Native trees and shrubs for the productive rehabilitation of tropical cattle ranching lands. For. Ecol. Manage. 261, 1654–1663. doi: 10.1016/j.foreco.2010.09.027
Nardone, A., Ronchi, B., Lacetera, N., Ranieri, M. S., and Bernabucci, U. (2010). Effects of climate changes on animal production and sustainability of livestock systems. Livest. Sci. 130, 57–69. doi: 10.1016/j.livsci.2010.02.011
Navarro-Racines, C., Tarapues, J., Thornton, P., Jarvis, A., and Ramirez-Villegas, J. (2020). High-resolution and bias-corrected CMIP5 projections for climate change impact assessments. Sci. Data 7:7. doi: 10.1038/s41597-019-0343-8
Olsson, L., Barbosa, H., Bhadwal, S., Cowie, A., Delusca, K., Flores-Renteria, D., et al. (2019). “Land degradation,” in Climate Change and Land: An IPCC Special Report on Climate Change, Desertification, Land Degradation, Sustainable Land Management, Food Security, and Greenhouse Gas Fluxes in Terrestrial Ecosystems, eds P. R. Shukla, J. Skea, E. Calvo Buendia, V. Masson-Delmotte, H.-O. Pörtner, D. C. Roberts, P. Zhai, R. Slade, S. Connors, R. van Diemen, M. Ferrat, E. Haughey, S. Luz, S. Neogi, M. Pathak, J. Petzold, J. Portugal Pereira, P. Vyas, E. Huntley, K. Kissick, M. Belkacemi, J. Malley (Geneva: Intergovernmental Panel on Climate Change), 345–436. Available online at: https://www.ipcc.ch/site/assets/uploads/sites/4/2019/11/07_Chapter-4.pdf (accessed September 14, 2021).
Pannell, D. J., Marshall, G. R., Barr, N., Curtis, A., and Vanclay, F. W. R. (2006). Understanding and promoting adoption of conservation practices by rural landholders. Aust. J. Exp. Agric. 43, 1407–1424. doi: 10.1071/EA05037
Park, C. S. (2007). Contemporary Engineering Economics, 4th Edn. Upper Saddle River, NJ: Prentice Hall.
Peñuela, L., Fernández, A. P., Castro, F., and Ocampo, A. (2011). Uso y manejo de forrajes nativos en la sabana inundable de la Orinoquía. Convenio de cooperación interinstitucional entre The Nature Conservancy (TNC) y la Fundación Horizonte Verde (FHV) con el apoyo de la Fundación Biodiversidad de España y la Corporación Autónoma Regional de la Orinoquía (CorpOrinoquía) (Bogota, DC). 66.
Peñuela, L., Solano, C., Ardila, V., and Galán, S. (2014). Sabana inundable y ganadería, opción productiva de conservación en la Orinoquia. Bogotá: Asociación Red Colombiana de Reservas Naturales de la Sociedad Civil, Fundación Natura, World Wildlife Fund, The Nature Conservancy, y Parques Nacionales Naturales de Colombia.
Pereira, J. M., Rezende, C. P., Ferreira, A. M., Homem, B. G. C., Casagrande, D. R., Macedo, T. M., et al. (2019). Production of beef cattle grazing on Brachiaria brizantha (Marandu grass)—Arachis pintoi (forage peanut cv. Belomonte) mixtures exceeded that on grass monocultures fertilized with 120 kg N/ha. Grass Forage Sci. 75, 28–36. doi: 10.1111/gfs.12463
Peters, M., Franco, L., Schmidt, A., and Hincapié, B. (2011). Especies Forrajeras Multipropósito: Opciones para productores del trópico americano. Cali: Centro Internacional de Agricultura Tropical (CIAT); Bundesministerium für Wirtschaftliche Zusammenarbeit und Entwicklung (BMZ); Deutsche Gesellschaft für Technische Zusammenarbeit (GIZ).
Peters, M., Horne, P., Schmidt, A., Holmann, F., Kerridge, P. C., Tarawali, S. A., et al. (2001). The Role of Forages in Reducing Poverty and Degradation of Natural Resources in Tropical Production Systems. Agricultural Research and Extension Network. Network Paper No. 117. Available online at: https://cdn.odi.org/media/documents/5218.pdf (accessed September 14, 2021).
Peters, M., Maass, B. L., Franco, L. H., and Cárdenas, E. A. (2000). Evaluación de germoplasma nuevo de Arachis pintoi en Colombia. 3. Bosque muy húmedo tropical–Piedemonte amazónico, Caquetá. Past. Trop. 22, 2–28. Available online at: http://ciat-library.ciat.cgiar.org/Articulos_Ciat/PAST2221.pdf (accessed September 14, 2021).
Phillips, S.J., Dudík, M., and Schapire, R.E. (2021). Maxent software for modeling species niches and distributions (Version 3.4.1). Available online at: http://biodiversityinformatics.amnh.org/open_source/maxent/ (accessed October 02, 2020).
Presidência da República (2012). Lei N° 12.651, de 25 de maio de 2012. Dispõe sobre a proteção da vegetação nativa e dá outras providências. Presidência da República. Available online at: http://www.planalto.gov.br/ccivil_03/_ato2011-2014/2012/lei/L12651compilado.htm (accessed April 24, 2021).
Rao, I., Van der Hoek, R., Peters, M., and Castro, A. (2014). Tropical forage-based systems for climate-smart livestock production in Latin America. Rural 21, 12–15. Available online at: https://www.biopasos.com/biblioteca/74v%20rural2014_04-S12-15.pdf (accessed September 14, 2021).
Reid, R. S., Bedelian, C., Said, M. Y., Kruska, R. L., Mauricio, R. M., Castel, V., et al. (2010). “Global livestock impacts on biodiversity,” in Livestock in a Changing Landscape, Volume 1. Drivers, Consequences, and Responses, eds H. Steinfeld, H. A. Mooney, F. Schneider, L. E. Neville (Washington, DC: Island Press), 111–137. Available online at: https://hdl.handle.net/10568/2532 (accessed September 14, 2021).
Riesco, A., and Seré, C. (1985). “Análisis económico de resultados de las pruebas de pastoreo,” in Evaluación de pasturas con animales. Alternativas metodológicas. Memorias de una reunión celebrada en Perú 1-5 de octubre, 1984, eds C. Lascano and E. Pizarro (Lima: Red Internacional de Evaluación de Pastos Tropicales), 201–232.
Rincón, A. (1999). Maní forrajero (Arachis pintoi), la leguminosa para sistemas sostenibles de producción agropecuaria. Bogotá: Corporación Colombiana de Investigación Agropecuaria–Agrosavia. Available online at: http://hdl.handle.net/11348/4114 (accessed September 14, 2021).
Rincón, A. (2001). Potencial productivo de ecotipos de Arachis pintoi en el Piedemonte de los Llanos Orientales de Colombia. Past. Trop. 23, 19–24. Available online at: https://www.tropicalgrasslands.info/index.php/tgft/pages/view/Pasturas (accessed September 14, 2021).
Rincón, A., Bueno, G., Diaz, R. A., Burkart, S., and Enciso, K. (2020). Cultivar Centauro (Arachis pintoi CIAT 22160): leguminosa forrajera para sistemas de ganadería sostenible. Bogotá: Corporación Colombiana de Investigación Agropecuaria (Agrosavia).
Rincón, A., Cuesta, P. A., Pérez, R., Lascano, C. E., and Ferguson, J. (1992). Maní Forrajero Perenne (Arachis pintoi Krapovickas y Gregory) Una Alternativa para Ganaderos y Productores. Cali: Centro Internacional de Agricultura Tropical (CIAT). Available online at: http://ciat-library.ciat.cgiar.org/forrajes_tropicales/pdf/Leaflets/arachis_colombia.pdf (accessed September 14, 2021).
Rincón, A., Flórez, H., Ballesteros, H., and León, L. M. (2018). Efectos de la fertilización en la productividad de una pastura de Brachiaria humidicola cv. Llanero en el Piedemonte de los Llanos Orientales de Colombia. Trop. Grassl. 6, 158–168. doi: 10.17138/tgft(6)158-168
Rincón, A., and Pesca, A. (2017). Productividad animal de una leguminosa forrajera seleccionada para pastoreo en suelos con drenaje deficiente (Unpublished report). Bogotá: Corporación colombiana de investigación agropecuaria (Agrosavia).
Rivas, L., and Holmann, F. (2000). Early adoption of Arachis pintoi in the humid tropics: the case of dual-purpose livestock systems in Caquetá, Colombia. Livest. Res. Rural Dev. 12:23. Available online at: http://www.lrrd.org/lrrd12/3/riva123.htm (accessed September 14, 2021).
Robertson, A. D. (2005). “Forage Arachis in Nepal: a simple success,” in Proceedings of the XX International Grassland Congress: Offered papers (Dublin), 214. Available online at: https://www.tropicalgrasslands.info/public/journals/4/Historic/Tropical%20Grasslands%20Journal%20archive/PDFs/Vol_39_2005/Vol_39_04_2005_pp214_214.pdf (accessed September 14, 2021).
Rojas-Downing, M., Pouyan, A., Harrigan, T., and Woznicki, S. A. (2017). Climate change and livestock: impacts, adaptation, and mitigation. Clim. Risk Manag. 16, 145–163. doi: 10.1016/j.crm.2017.02.001
Romero, F., and González, J. (2004). “Effects of Brachiaria decumbens alone and associated with Arachis pintoi on milk production and milk components,” in Feeding systems with forage legumes to intensify dairy production in Latin America and the Caribbean-A project executed by the Tropileche Consortium, eds Holmann and Lascano (Cali: Centro Internacional de Agricultura Tropical), 5–11. Available online at: http://hdl.handle.net/10568/830 (accessed September 14, 2021).
Sattler, D., Seliger, R., Nehren, U., Naegeli, F., Soares da Silva, A., Raedig, C., et al. (2018). “Pasture degradation in south east brazil: status, drivers and options for sustainable land use under climate change,” in Climate Change Adaptation in Latin America, eds W. L. Filho and L. E. de Freitas (Cham: Springer), 3–17.
Schultze-Kraft, R., Rao, I. M., Peters, M., Clements, R. J., Bai, C., and Liu, G. (2018). Tropical forage legumes for environmental benefits: an overview. Trop. Grassl. 6, 1–14. doi: 10.17138/TGFT(6)1-14
Shelton, H. M., Franzel, S., and Peters, M. (2005). Adoption of tropical legume technology around the world: analysis of success. Trop. Grassl. 39, 198–209. Available online at: https://www.tropicalgrasslands.info/index.php/tgft/pages/view/tropicalGrasslands (accessed September 14, 2021).
Simpson, C. E., Valls, J. F. M., and Miles, J. W. (1995). “Biología reproductiva y potencial para la recombinación genética de Arachis,” in Biología y Agronomía de Especies Forrajeras de Arachis, ed P.C. Kerridge (Cali: Centro Internacional de Agricultura Tropical), 46–55. Available online at: https://hdl.handle.net/10568/54798
Smith, J., Cadavid, J. V., Rincón, A., and Vera, R. (1997). Land speculation and intensification at the frontier: a seeming paradox in the Colombian Savanna. Agric. Syst. 54, 501–520. doi: 10.1016/S0308-521X(96)00088-1
Subbarao, G., Nakahara, K., Hurtado, M., Ono, H., Moreta, D., Salcedo, A. F., et al. (2009). Evidence for biological nitrification inhibition in Brachiaria pastures. PNAS 106, 17302–17307. doi: 10.1073/pnas.0903694106
Subbarao, G. V., Arango, J., Masahiro, K., Hooper, A. M., Yoshihashi, T., Ando, Y., et al. (2017). Genetic mitigation strategies to tackle agricultural GHG emissions: The case for biological nitrification inhibition technology. Plant Sci. 262, 165–168. doi: 10.1016/j.plantsci.2017.05.004
Thornton, P. K., Van de Steeg, J., Notenbaert, A., and Herrrero, M. (2009). The impacts of climate change on livestock and livestock systems in developing countries: a review of what we know and what we need to know. Agric. Syst. 101, 113–127. doi: 10.1016/j.agsy.2009.05.002
Trujillo-Barrera, A., Pennings, J. M. E., and Hofenk, D. (2016). Understanding producers' motives for adopting sustainable practices: the role of expected rewards, risk perception and risk tolerance. Eur. Rev. Agric. Econ. 43, 359–382. doi: 10.1093/erae/jbv038
Valentim, J. F., and Andrade, C. M. S. (2005). Forage peanut (Arachis pintoi): a high yielding and high-quality tropical legume for sustainable cattle production systems in the western Brazilian Amazon. Trop. Grassl. 39:222. Available online at: https://www.tropicalgrasslands.info/index.php/tgft/pages/view/tropicalGrasslands (accessed September 14, 2021).
Valls, J. F. M., and Pizarro, E. A. (1995). “Recolección de germoplasma de Arachis silvestre,” in Biología y Agronomía de Especies Forrajeras de Arachis, ed P. C. Kerridge (Cali: Centro Internacional de Agricultura Tropical), 21–30. Available online at: https://hdl.handle.net/10568/54796 (accessed September 14, 2021).
van Winsen, F., de Mey, Y., Lauwers, L., Van Passel, S., Vancauteren, M., and Wauters, E. (2014). Determinants of risk behaviour: effects of perceived risks and risk attitude on farmer's adoption of risk management strategies. J. Risk Res. 19, 56–78. doi: 10.1080/13669877.2014.940597
Vasques, I. C. F., Souza, A. A., Morais, E. G., Benevenute, P. A. N., da Silva, L. C. M., Homem, B. G. C., et al. (2019). Improved management increases carrying capacity of Brazilian pastures. Agric. Ecosyst. Environ. 282, 30–39. doi: 10.1016/j.agee.2019.05.017
Villegas, D. M., Velasquez, J., Arango, J., Obregon, K., Rao, I. M., Rosas, G., et al. (2020). Urochloa grasses swap nitrogen source when grown in association with legumes in tropical pastures. Diversity 12:419. doi: 10.3390/d12110419
Walther, G., Beibner, S., and Burga, A. (2005). Trends in the upward shift of alpine plants. J. Veg. Sci. 16, 541–548. doi: 10.1111/j.1654-1103.2005.tb02394.x
White, D., Holmann, F., Fujisaki, S., Reategui, K., and Lascano, C. (2001). “Will intensifying pasture management in Latin America protect forests–or is it the other way around?” in Agricultural Technologies and Tropical Deforestation, eds A. Angelsen and D. Kaimowitz (Wallingford: CABI Publishing), 91–113.
Williams, D. G., and Baruch, Z. (2000). African grass invasion in the Americas: ecosystem consequences and the role of ecophysiology. Biol. Invasions 2, 123–140. doi: 10.1023/A:1010040524588
Keywords: climate change, forage legumes, adoption, economic evaluation, risk analysis (RA), land-use change (LUC)
Citation: Enciso Valencia KJ, Rincón Castillo Á, Ruden DA and Burkart S (2021) Risk Reduction and Productivity Increase Through Integrating Arachis pintoi in Cattle Production Systems in the Colombian Orinoquía. Front. Sustain. Food Syst. 5:666604. doi: 10.3389/fsufs.2021.666604
Received: 15 February 2021; Accepted: 06 September 2021;
Published: 04 October 2021.
Edited by:
Glenn Hyman, Independent Researcher, ColombiaReviewed by:
Alisher Mirzabaev, Center for Development Research (ZEF), GermanyAjit Singh, University of Nottingham Malaysia Campus, Malaysia
Copyright © 2021 Enciso Valencia, Rincón Castillo, Ruden and Burkart. This is an open-access article distributed under the terms of the Creative Commons Attribution License (CC BY). The use, distribution or reproduction in other forums is permitted, provided the original author(s) and the copyright owner(s) are credited and that the original publication in this journal is cited, in accordance with accepted academic practice. No use, distribution or reproduction is permitted which does not comply with these terms.
*Correspondence: Stefan Burkart, cy5idXJrYXJ0QGNnaWFyLm9yZw==