- 1Agroecology, Environment and Systems Group, Instituto de Investigaciones Forestales y Agropecuarias de Bariloche (IFAB), Instituto Nacional de Tecnología Agropecuaria-Consejo Nacional de Investigaciones Científicas y Técnicas (INTA-CONICET), San Carlos de Bariloche, Argentina
- 2Agroécologie et Intensification Durable (AïDA), Centre de coopération Internationale en Recherche Agronomique pour le Développement (CIRAD), Université de Montpellier, Montpellier, France
- 3Groningen Institute of Evolutionary Life Sciences, Groningen University, Groningen, Netherlands
Sustainability assessments to inform the design of multifunctional grazing landscapes need to look beyond greenhouse gas emissions to simultaneously embrace other social and environmental criteria. Here I briefly examine trade-offs and synergies between the productivity of graze-based livestock systems and the environment, and share a few generic guidelines to design pathways for the ecological intensification of livestock systems following agroecological principles. I draw from experience on livestock farming in the Rio de la Plata Grassland Biome of South America (Argentina, Uruguay, and Brazil). Livestock systems based on native grasslands in this region may have greater carbon footprints (13–29 kg CO2 eq. kg LW−1) than intensive grass-feedlot systems in the region (9–14 kg CO2 eq. kg LW−1) or the average range reported for OECD countries (c. 10–20 kg CO2 eq. kg LW−1) when calculated per unit product, but only 20% greater when expressed on an area basis. Yet they use less external energy (10x) or nitrogen inputs (5x) per kg live weight (LW) produced, provide ecosystem services of local and global importance, such as carbon storage, habitat protection for biodiversity, watershed regulation, clean water, food and textiles, livelihoods and local cultures, and provide better living conditions for grazing animals. Traditional graze-based systems are less economically attractive than intensive livestock or grain production and they are being replaced by such activities, with negative social and environmental consequences. An ecological intensification (EI) of graze-based livestock systems is urgently needed to ensure economic profits while minimising social-ecological trade-offs on multifunctional landscapes. Examples of such EI systems exist in the region that exhibit synergies between economic and environmental goals, but a broad and lasting transition towards sustainable multifunctional landscapes based on agroecological principles requires (co-)innovation at both technical and institutional levels.
Introduction
Discourses on global issues such as climate change, diet-related human health, deforestation, desertification, air and water pollution or biodiversity loss point to livestock production as one of their main causes (e.g., Opio et al., 2013; Herrero et al., 2015; FAO, 2018). Admittedly, simplified industrial livestock systems rely heavily on external inputs (feeds, fertilisers, pesticides), antibiotics, growth promotors, fossil fuels, etc., are vulnerable to diseases, to climatic variability, to price spikes, etc., host little biodiversity, impact negatively on the environment, compromise animal welfare, do not provide substantial amounts of rural jobs, often need governmental subsidies to be economically viable, and tend to generate ecosystem disservices more frequent than services. They require a profound redesign to become sustainable. Traditional livestock production systems based on native grasslands and woodlands, on the other hand, often provide ecosystem services that may compensate for the environmental damage that they cause (cf. Tittonell et al., 2020). Yet these systems are under threat do to their poor ability to compete with more profitable land uses, to institutional pressure to undergo intensification or “modernisation,” or to the ageing of traditional livestock keepers associated with the migration of the rural youth to urban areas (e.g., Novotny et al., 2020; Solano-Hernandez et al., 2020). An intensification based on agroecological principles is urgently needed in both industrial and traditional livestock systems to arrive at a third way strategy by which economic, social and environmental trade-offs are minimised, resulting in multifunctional, sustainable grazing landscapes.
An important element – but not the only one – that prevents the development and implementation of knowledge, technologies and institutional incentives to support a transition towards multifunctional grazing landscapes is a poorly informed debate around livestock environmental sustainability. Quantitative assessments of environmental impacts of livestock systems have focused chiefly on carbon footprints (e.g., Opio et al., 2013; Becoa et al., 2014), and less frequently on other aspects such as biodiversity, energy and nutrient efficiencies, watershed regulation or socio-cultural values. The use of simplifying environmental accounting methods, such as the life cycle assessment, has often led to conclude that intensive livestock systems such as feedlots or animal warehouses are more “sustainable” than grazing systems due to their lower CO2 emission rate per kg of produce (e.g., De Vries and De Boer, 2010). This is certainly a narrow view on what sustainability really means. But such understandings are also fuelled by the fact that the current ability and potential of grazing systems to provide ecosystem services of local and global importance, and the trade-offs with their environmental impacts, have been generally poorly studied (FAO, 2019). And even less frequent are studies that simultaneously assess the various economic, social and environmental performances of alternative livestock systems, or that document comprehensive processes of system redesign and transition. Sustainability assessments to inform the design of multifunctional grazing landscapes need to look beyond greenhouse gas emissions to simultaneously embrace other social and environmental criteria.
The ultimate goal are multifunctional landscapes on naturally heterogeneous grazing ecosystems, that foster ecosystem services (provision, support, regulation, and cultural) and minimise trade-offs with other environmental indicators, such as the carbon foot print. Here I briefly examine trade-offs and synergies between the productivity of graze-based livestock systems and environmental indicators associated with the UN Sustainable Development Goals (SDG). For conciseness, I present quantitative examples that do not deal directly with socio-cultural ecosystem services except marginally with revenue, as the goal of this paper is to expand the debate from CO2 to other environmental sustainability criteria. Then, I share a few guidelines that may contribute to designing pathways for the ecological intensification of livestock systems following agroecological principles, in order to overcome such trade-offs. I draw these ideas from ca. 20 years of experience in research – hence the frequent self-citation – and implementation of ecologically intensive farming in the Rio de la Plata Grassland Biome of South America, engaging with farmers and researchers in trajectories of learning and development. I conclude with a few generalizable messages that can inform and hopefully inspire the design of multifunctional grazing landscapes.
Trade-offs Between Productivity and the Environment
Trade-offs around livestock production are often presented as an “either-or” choice between consuming animal products vs. cooling the planet. Vegetarian diets and veganism are advocated as the solution to our environmental problems, especially global warming. One problem with this approach is that it neglects the various functions and services associated with livestock, from ecological to social and cultural (e.g., Hoffmann et al., 2014). This is particularly true for grazing livestock systems, which next to providing food and incomes contribute to nutrient cycling and circular farming, to protect habitats for biodiversity, to regulate water and carbon flows in ecosystems, to preserve traditional livelihoods and their cultural capital, and to transform inedible plant biomass into valuable food for humans. Yet, these are not arguments in favour of increasing meat consumption or that of any other animal product. Meat consumption needs to be re-dimensioned at global scale, drastically reduced, but also better distributed across regions (for example, recommended per capita meat consumption rates are in the order of 26 Kg year−1; yet current world average is 43 Kg year−1, average consumption in the US or Australia is above 120 Kg year−1, around 80 in the UK and western Europe, 48 Kg year−1 in China, from 10 to 20 Kg year−1 in Africa, and <5 Kg year−1 in India).
Grazing livestock may play a positive role in agroecosystems. Virtuous crop-livestock interactions are central to the design of sustainable landscapes through agroecology (e.g., Bonaudo et al., 2014). Unfortunately, the trade-offs and synergies between grazing livestock production and other ecosystem services have been poorly documented, at least in quantitative terms. Such is the case also for the sheep and cattle ranging systems in the Río de la Plata grasslands (RPG), which comprises vast areas of Argentina, Uruguay, and Brazil. These nearly 700,000 km2 of native grasslands and woodlands store more than 5% of the soil carbon of the continent, prevent soil erosion, and provide clean water to major cities in the region where up to 20 million people live, host respectively 800 and 200 endemic species of grasses and legumes, a wide diversity of bird and mammal species, host 65 million livestock heads and sustain the livelihood of c. 430,000 local family farmers. These traditional systems are however under threat due to the expansion of more profitable activities that produce for the export market (soya, maize, rice), thereby displacing family farmers and converting land from grasslands and woodlands into uniform monocultures with high environmental impact (Modernel et al., 2016). Narratives that associate traditional grazing livestock with global warming do not contribute to halt such a trend.
Graze-based livestock systems in this part of the world encompass a diversity of management systems that range from full cycle cattle (calving, backgrounding and finishing on the same farm) to more specialised cow-calf or finishing/fattening systems. Traditional family farms are more often associated with cow-calf/sheep rearing, generally on unfertilized native grasslands, whereas specialised fattening systems tend to be more entrepreneurial and rely on intensive feeding regimes (Ruggia et al., 2015). Options for intensification of meat production are often oriented towards increasing the efficiency of the later stages in the production cycle, those concerned with the backgrounding and finishing phases. The various phases of the production cycle may take place with the animals grazing on native grasslands or on sown pastures (or leys, often involving the use of fertilisers, soil correctors and sometimes irrigation), or confined in feedlots where they are fed cereals (Modernel et al., 2016). Picasso et al. (2018) analysed the productivity and environmental performance of systems varying in their intensity during backgrounding and finishing, from those that relied exclusively on native grasslands (Grass-Grass), to those that combined them with sown pastures (Grass-Past), that relied exclusively on sown pastures (Past-Past), or that fed concentrates during the finishing phase (Past-Feed) (Figure 1). Emission calculations were done using IPCC 2006 tier 2 equations, as described in Modernel et al. (2013), considering also the emissions associated with production and distribution of feeds and other inputs (fertilisers, seeds, herbicides). Soil carbon was assumed to remain constant, as recommended by the IPCC. When comparing the four systems, beef productivity increased respectively from 140 to 300 kg LW ha−1 (109% increase), whereas the carbon footprint measured in CO2 equivalents per kg LW consequently decreased by 59% in the same range as the systems became more productive – a dilution effect!
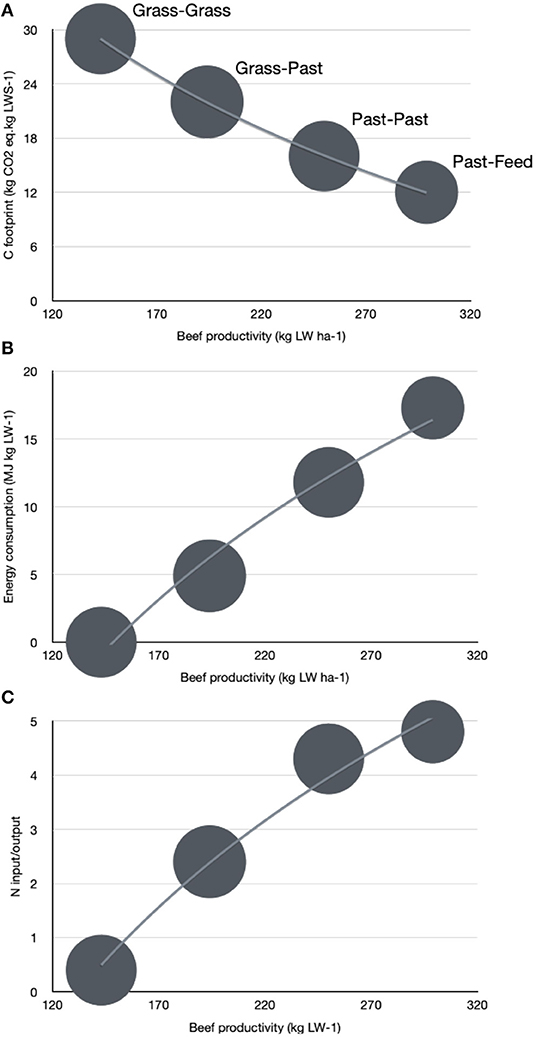
Figure 1. Calculations of environmental footprints of four representative beef production system types in Uruguay varying in their intensity during the backgrounding and finishing phases, using native grasslands (Grass), sown pastures (Past), or feedlots (Feed). Carbon footprint (A), energy consumption (B), and nitrogen inputs (C) are plotted against average beef productivity per hectare per year. The size of the circles represents the average CO2 emission rate per hectare per year. Grass-Grass indicates that both the backgrounding and finishing phases are done on native grasslands, Grass-Past indicates backgrounding on grasslands and finishing on pastures, and so on. The graphs were drawn using calculations presented by Picasso et al. (2018).
Dilution effects that result from expressing CO2 emission rates per kg of live weight (LW) are an artefact of the carbon footprint calculation and often used to portrait intensive livestock systems such as feedlots as being more sustainable than graze-based systems (e.g., McGinn et al., 2008; Capper, 2010). There are several reasons to explain this pattern. A cereal grain diet fed to cattle bypasses rumination and hence CH4 emissions are lower per kg of dry mater taken in by the animals. In addition, feedlot animals live generally shorter, they gain weight faster at the expense of their health (e.g., acidosis is common among grain-fed young steers), become “roundish” much earlier than grazing animals and are consequently slaughtered at a younger age, spending no more than 4 months on average in a feedlot. Since emission rates are calculated over the entire life span of an animal, shorter life spans have an additional dilution effect on the average emission rate per unit LW. When expressing emission rates per unit land instead of LW, systems that background and finish cattle exclusively on native grasslands without external inputs emitted on average 4,095 CO2 eq. ha−1, those that finished on pastures 4,330 CO2 eq. ha−1, those that used exclusively pastures 4,071 CO2 eq. ha−1, and those that finished in a feedlot 3,250 CO2 eq. ha−1 (emissions per unit land are represented by the size of the circles in Figure 1A). Thus, when the carbon footprint is calculated on an area basis – which makes perfect sense for a footprint – intensive beef production systems emit c. 20% less CO2 equivalents than traditional grazing systems on native grasslands, or than intensive grazing systems on sown pastures.
Fossil fuel energy consumption per kg LW produced, which is another environmental indicator associated with global warming, shows however critical increases as systems intensify in this way (Figure 1B). And, although more intensive livestock systems are also often portrayed as being more efficient in the use of external resources, the calculations of Picasso et al. (2018) show that the nitrogen input to output ratio becomes increasingly unfavourable as systems intensify relying increasingly on sown pastures and feedlots (Figure 1C). Intensive pasture and feedlot systems need five times more N than fully grazing systems per kg N exported as output. Yet although the average values for all these indicators fluctuates substantially between fully grazing to grazing plus feedlot systems (cf. Figure 1), they are still far from average values reported for intensive livestock systems worldwide. For example, Modernel et al. (2018) showed that beef systems in OECD countries are 52% more productive and emit 35% less (per unit LW!), but they use 500% more fossil fuel energy as compared with traditional grazing systems in the Rio de la Plata region.
But beyond these popular indicators to assess livestock sustainability, there are other impacts of intensification to be considered, which are beyond the scope of this paper, for example: Concentration of nutrient-rich dejections and more frequent use of pharmacological ingredients leads also to water pollution in the surrounding of feedlots with negative effect for human populations (e.g., Elorriaga et al., 2013). When the manure from such intensive operations is used to amend soils in fresh vegetable production there are high risks of contamination and antibiotic resistance building (Jechalke et al., 2014). Further, converting native grasslands and woodlands into fertilised pastures sown to exotic species such as ryegrass, or into annual cropping fields to produce the necessary feed grain, has also enormous consequences for soil, water and biodiversity conservation. Effects of land conversion and grazing management on biodiversity range from losses in abundance and richness of soil organisms (e.g., El Mujtar et al., 2019) or plant species diversity (e.g., Lezama et al., 2013; Pizzio et al., 2016; Herrero-Jáuregy and Oesterheld, 2018) to negative effects on amphibians, birds and mammals (e.g., Alkemade et al., 2013; Dias et al., 2014; Azpiroz and Blake, 2016; Schieltz and Rubenstein, 2016). Shifting from direct grazing to frequent mowing under intensive cut-and-carry feeding systems has also serious consequences for biodiversity, especially for ground nesting birds or the arthropods and worms they feed on (Kentie et al., 2016). Beyond global assessments on the effect of grazing management on soil carbon sequestration (e.g., Tanentzap and Coomes, 2012; Abdalla et al., 2018), studies in the Rio de la Plata region indicate that switching from native vegetation to sown pastures reduces soil carbon storage by more than 60% (Piñeiro et al., 2010), and water infiltration by almost 100% seriously affecting watershed regulation (floods, aquifer recharge) and soil erosion (e.g., Nosetto et al., 2012). Soils of native grasslands managed with high forage allowance (adjusted stocking rates over time-space) allow fast, resilient recovery of forage productivity after severe droughts (Modernel et al., 2019).
Next to biodiversity and the environment, animal welfare is also critically compromised as animals move from year-round grazing at sparse stocking rates on native vegetation to being stalled at high densities in a feedlot, where they receive antibiotics as growth promotors, have no access to grazing, stand on muddy soil and suffer confinement-related stress (e.g., Nielsen and Zhao, 2012; More et al., 2017). In Figure 2, un-managed livestock harvesting represents old traditional systems that have now virtually disappeared from the Rio de la Plata region, in which livestock were set free and gathered every year to “harvest” animals to be sold on the market (the system persists in part of the Patagonia or Andean drylands). This sort of natural welfare is not necessarily optimal for domestic animals, which require for example reproductive assistance, veterinary care, etc. Maximum welfare is achieved when animals are provided these plus also shelter, protection from predation, supplementary feed in times of drought or snow, clean and easily accessible water, organised mating and weaning around forage availability, etc. Productivity increases as animal welfare increases. Beyond a certain point, however, animal welfare is increasingly compromised in favour of livestock productivity, to the extreme of reducing it beyond cruelty levels when ruminants spend most of their life in a feedlot feeding on concentrates. Traditional yet stereotyped systems in the Rio de la Plata Grassland Biome are represented in Figure 2 by the range between points a, family systems, and b, entrepreneurial graze-based systems relying largely also on native grasslands. Figure 2 also indicates the scope for designing ecologically intensive grazing landscapes (see Towards Ecological Intensification: Design, Co-innovation, and System Transition section), represented by the green dashed line, which must aim to increase productivity while maintaining socially acceptable levels of animal welfare.
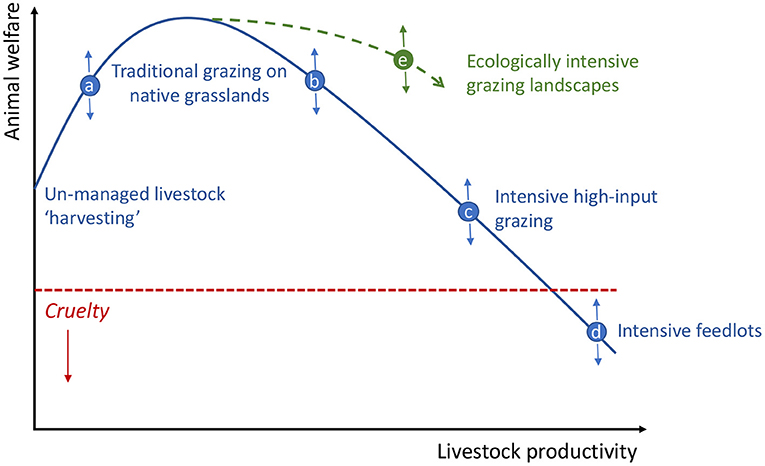
Figure 2. A representation of the relationship between (perceived) animal welfare and livestock productivity following the conceptual mode of McInerney (2004) to depict the various beef production systems that can be found in the Rio de la Plata Grassland Biome. Red dotted line represents a minimum welfare level below which we may speak of cruelty towards domestic animals. Points a and b indicate respectively the approximate situation of traditional family and entrepreneurial graze-based livestock systems in the region. Vertical arrows indicate variation in terms of animal welfare within each type of system. The green dashed line represents the scope of ecologically intensive razing landscapes in terms of increasing productivity while minimising the trade-offs with animal welfare.
Towards Ecological Intensification: Design, Co-innovation, and System Transition
In spite of the array of services they provide, from conservation of biodiversity or landscape regulating functions, to lifestyles and cultures, traditional grazing livestock systems are disappearing as being outcompeted by more profitable farming activities. Curtailing this trend requires measures to increase the profitability of family livestock keepers in the region. The ecological intensification of traditional grassland-based livestock systems has been proposed as a way to increase productivity while reducing costs and maintaining the provision of key ecosystem services (Albicette et al., 2017). But, what is ecological intensification? What is an ecologically intensive grazing landscape?
Definition and Design Principles
Ecological intensification relies on the knowledge and design principles of agroecology (cf. Tittonell, 2014). There are several definitions and interpretations of the term “intensification” and its qualifiers, but here I use the one adopted in Latin America by the PROCISUR (Programa Cooperativo para el Desarrollo Tecnológico Agroalimentario y Agroindustrial del Cono Sur: www.procisur.org.uy) in 2019: “Ecological intensification is a process of gradual improvement of the ecological efficiency of production systems through technological and institutional innovation, with the aim of using the natural functionalities offered by ecosystems to promote higher productivity with less environmental impact, maintain or improve the natural resource base, reduce dependence on non-renewable resources and favour adaptability, resilience and social equity” (Tittonell, 2018). This generic definition needs to be made specific for livestock systems, and in particular for grazing systems, i.e., their biophysical characteristics, socioeconomic context, management and business model, history and long-term strategy. Yet, I find it useful to derive a few general principles for the design of ecologically intensive landscapes. These principles are intended to be applicable regardless of the type of production system in question, and can be outlined as Tittonell (2020b):
1. Match: match the supply of resources with the requirements and demands of the production system (plant- or animal-based);
2. Fine-tune: regulate or correct those factors that impede or reduce the efficiency of the relationship between environmental supply and the system's requirements;
3. Reduce: reduce the dependence of the system on external inputs, particularly those obtained from non-renewable resources or that cause direct environmental impact;
4. Sustain: sustain the natural resource base and associated ecosystem services over time and increase the resilience of the system against exogenous disturbances.
These guidelines are subject to the objective(s) for which the landscape is designed or managed. Objectives may include production, conservation, regeneration or reproduction of natural capital or the productive resource base, provision of ecosystem services of local or global importance, diversification, investment, etc., or be of a cultural, ethical or affective nature. Hence there is not a single set of practises that could be universally applied to engage in an ecological intensification trajectory, as they will be objective- and context-specific. Table 1 offers however some examples of possible practises simply as illustration.
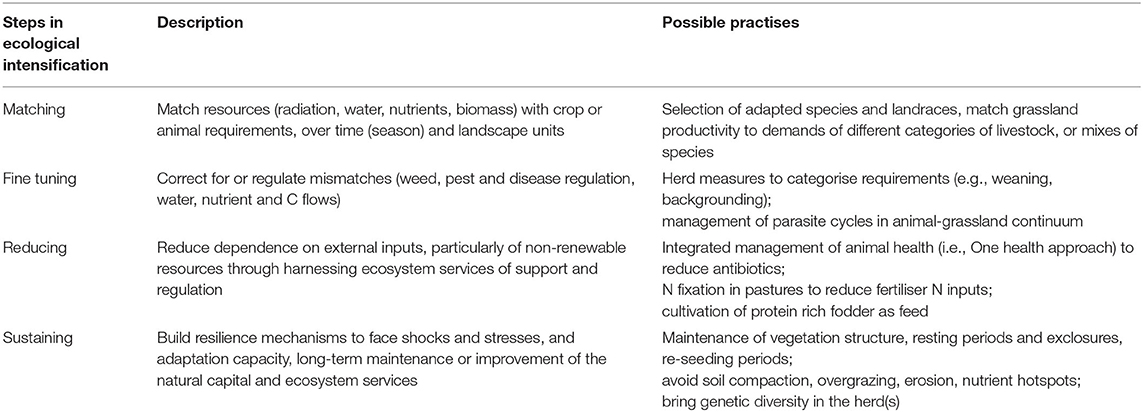
Table 1. Four steps proposed for the ecological intensification of grazing livestock systems and examples of possible management practises.
Examples of ecologically intensive management in practise were documented in the Rio de la Plata Grassland Biome by Modernel et al. (2018), who identified, through a combination of Pareto ranking and archetype analysis of graze-based livestock farms in Uruguay (n = 208), those that stood out in terms of economic and environmental performances (“Synergies” in Figure 3). The authors also identified archetype farms that exhibited good economic performance but less favourable environmental indicators (“Tradeoffs” in Figure 3), good environmental but poor economic performance (not shown in Figure 3), and poor performance on both criteria (“Lose-lose” in Figure 3). Beyond the implications of the absolute or comparative values of some of these indicators, this study revealed the existence of practical examples of ecologically intensive livestock farms in the region, positive deviants that can be used a benchmarks for learning and innovation, or to inform policy making (NB: P balances in Figure 3 are plotted in the opposite sense as compared with the original publication, since I consider P mining to be an acuter problem than P accumulation in the soils of the region). The experience in the Rio de la Plata region indicates that the ecological intensification of livestock on native vegetation, particularly the matching step proposed here, requires spatially explicit management of the heterogeneity of the grassland ecosystem (e.g., Soca et al., 2013; Trindade et al., 2016; Do Carmo et al., 2019). This, and the fact that sustainable landscapes are expected to be multifunctional, is why I prefer to speak of ecologically intensive grazing landscapes, and not just grazing systems. The term landscape includes not only the spatial but also the social, ecological and cultural aspects of the grazing system.
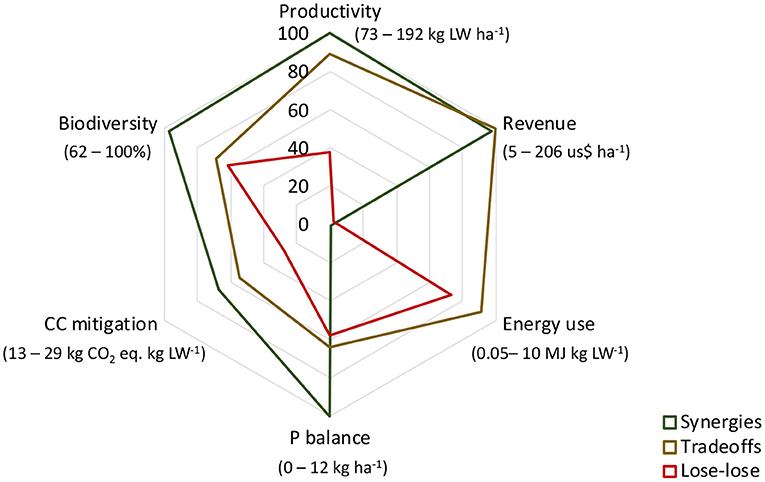
Figure 3. Economic and environmental performance of archetypal grazed-based livestock farms in Uruguay, Rio de la Plata Grassland Biome, derived from a survey of 280 cases by Modernel et al. (2018). The archetype analysis was based on a Pareto ranking of the sampled farms with respect to the various indicators (plus N balances, not shown in this graph). Four archetypes were found that represented win-win (synergies), win-lose (trade-offs), lose-win, and lose-lose situations in terms of these economic and environmental indicators, using local expert knowledge for benchmarking. Lose-win situations are not represented in this graph, as viable economic performances are a non-negotiable requisite to local stakeholders. Only 41 farms fitted these four archetypes of which only five farms stood out as win-win cases. Values between brackets indicate the range of average values for each archetype (min-max). Graphics built with data presented by Modernel et al. (2018).
Innovation Systems
The ecological intensification of graze-based livestock systems is highly knowledge intensive. Approaches that combine different types of knowledge through co-innovation processes are most successful at fostering ecological intensification (Tittonell et al., 2016). Since 2007, Rossing et al. (2021) crafted co-innovation as an approach for governance and management of ecological intensification processes, combining three elements: a complex adaptive systems perspective, social learning settings, and dynamic monitoring and evaluation. The experience collected so far shows that the combination of external technical assistance, co-construction of knowledge, and adapted process technologies are central to ecological intensification processes. Figure 4A illustrates the gains in livestock productivity that were achieved in General Lamadrid County, Argentina (Rio de la Plata Grassland Biome) during the period 1998–2010 by farmers who engaged in co-innovation platforms consisting of regional farmer networks (Pacín and Oesterheld, 2015). The lower the average productivity of the farms the greater the gains that were realised, of up to 300% greater beef productivity in some cases. Although access to knowledge-sharing platforms had a positive impact on forage productivity (ca. 30% increase on average – data not shown), the greatest part of the gain in beef productivity was explained by an increase in forage conversion rates (Figure 4B), which is a sensitive management-related indicator. Access to knowledge in this case was achieved through farmers' participation in regional innovation platforms known as Regional Consortiums for Agricultural Experimentation (CREA: www.crea.org.ar), a private network created in 1957, and a long-standing example of co-innovation in practise.
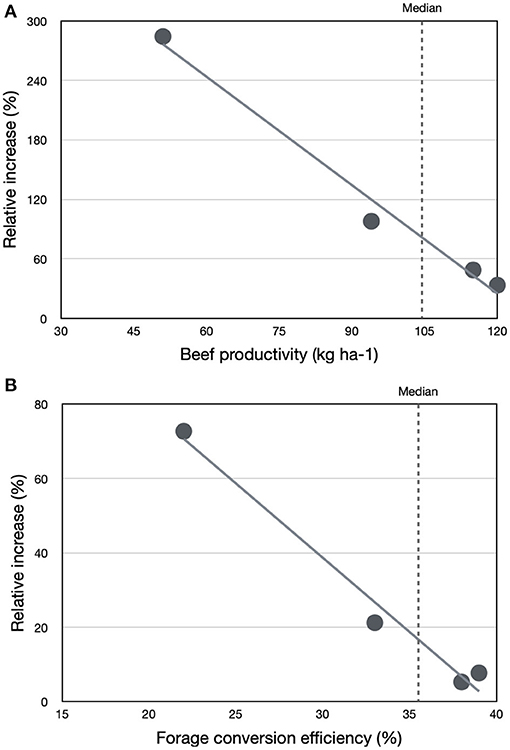
Figure 4. Room for improvement in terms of beef productivity (A) and forage conversion efficiency (B) when farmers engaged in regional innovation platforms, based on a 1998-2010 study at municipal level in General Lamadrid County, Buenos Aires, Argentina (Rio de la Plata Grassland Biome), using data from Pacín and Oesterheld (2015). Lamadrid County comprises 481,000 ha and had 437,000 heads of cattle at the time of this study, of which 37,000 were owned by farmers who participated in platforms. The four points shown in the figures correspond to years 2000/1, 2003/4, 2006/7, and 2009/10. Y-axis depicts the relative difference (%) between farms with and without technical assistance.
Agroecological Transition
The few examples presented here illustrate that there is room to improve the performance of grazing livestock systems, minimising production-environment trade-offs through design and management, to preserve biodiversity and propend to multifunctional landscapes. The ecological intensification of grazing landscapes is a special case of what is generally known as agroecological transition, or the necessary social-ecological reconfiguration of agroecosystems to produce following agroecological principles (Tittonell, 2020a). Agroecological transitions have been described in different ways but most definitions make implicit use of the Efficiency-Substitution-Redesign concept, which was probably coined by Hill and MacRae (1995) as the ESR-model, but frequently used by classical authors in agroecology such as Gliessman (2006). There is a narrow relationship between the phases of an agroecological transition and the various domains at which innovation is needed, such as technical and institutional innovation (cf. Tittonell, 2014). I try to explore this idea further with the diagrams of Figure 4, which represent (A) the domains for innovation as nested sets of constraints, and (B) the phases of an agroecological transitions following the ESR-model plus a governance phase. NB: None of these processes is linear or sequential! The use of “phases” is just the simplest way to explain it.
There are substantial gains to be achieved in both economic and environmental ecosystem services through proper management practises (e.g., Figures 3, 4), particularly considering heterogeneity management in patio-temporally diverse grazing landscapes. Adaptive, well-informed and spatially explicit management is a requisite to improve systems efficiencies (Figure 5). But this is not enough for ecological intensification. The ability of management practises to stir change towards sustainable, multi-functional landscapes is often constrained by the availability of the necessary technologies to sustain agroecologically intensive management. This is represented by the red set in Figure 5 (Technology constraints). Beyond technologies to support spatially explicit, precise management of heterogenous grazing landscapes, which are developing fast, there is a major gap in the realm of input technologies that constrains agroecological livestock intensification. For example, and although progress is underway, the design of biological solutions to replace traditional antibiotics or vermifuge treatments has still much ground to cover. Yet, even when bio-based technologies would be available for a complete input substitution, sustainable multifunctional management may be hampered by structural constraints in the production system that would require a thorough redesign. For example, systems that rely on single species of plants and animals, deployed over homogeneous landscapes (e.g., dairy cow-perennial ryegrass type of systems), offer narrow space for manoeuvring in terms of ecological intensification. These systems depend on inputs, whether synthetic of biological, because they are ecologically out of balance. As vastly discussed here, simplified industrial livestock systems would often require profound redesign measures to become sustainable (i.e., productive, stable, resilient, independent, reliable).
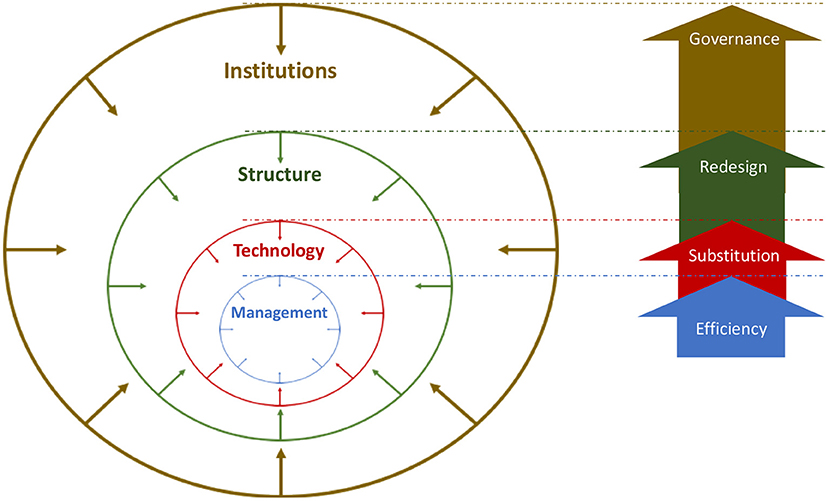
Figure 5. Innovation domains for the ecological intensification of livestock systems in multi-functional landscapes represented as sets of nested constraints (left), and four phases in agroecological transitions necessary to face the constraints imposed by each domain (right). The size of the sets and the length of the block arrows indicate the relative strength of the constraints to agroecological transitions.
But the extent at which innovation can take place in the redesign of current livestock system is constrained by their institutional context (Figure 5). By institutions, I mean markets, regulations, knowledge systems, principles and public or private organisations. For example, ecological intensification of pastoral systems cannot be regarded in isolation from the policy and legal environments in which pastoralist communities need to operate, especially when land tenure or access to natural resources are at stake (e.g., Dong, 2016). But on the other hand, consumers and value chains have a great power to stir change. Consider, for example, how the uprising “grassland beef” market has influenced producers to adopt regenerative, sustainable and externally monitored practises of native grassland management and biodiversity conservation in different parts of the world (Figure 6, Table 2, and further: e.g., USA: discover.grasslandbeef.com; Australia: grasslandsbeef.com.au; etc.). There are also different ways in which large food retailers by themselves can positively influence sustainability (e.g., Macfadyen et al., 2016). Finally, and although this exceeds the scope of the present manuscript, I find it relevant to end by stressing that moving beyond anecdotal cases towards successful, broad and lasting transitions to ecologically intensive grazing landscapes requires innovation, policy and action also in the realms of natural resource and food system governance.
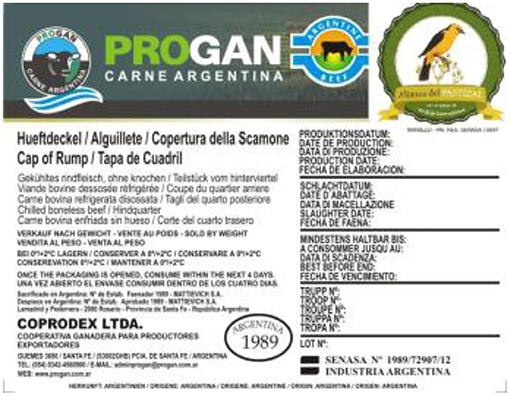
Figure 6. Commercial label of beef exported by a cooperative of livestock farmers in Argentina certified for birdlife-friendly grazing management practises by the Native Grasslands Alliance (Alianza del Pastizal). https://www.birdlife.org/grasslands-alliance/es.
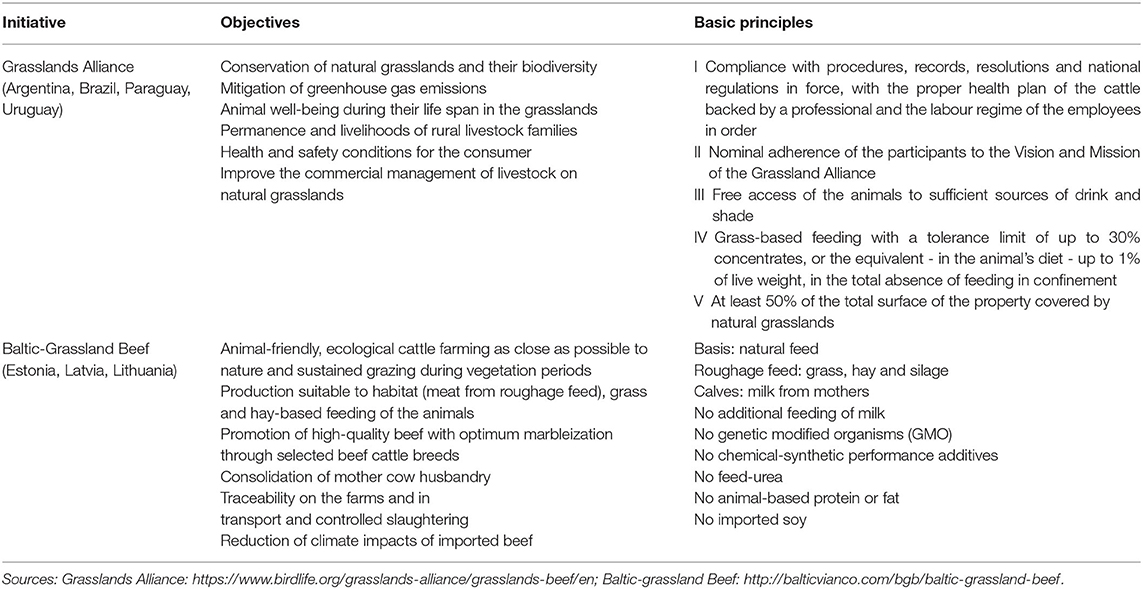
Table 2. Examples of grassland beef value chains in South America and Europe, outlining their objectives and basic principles.
Concluding Remarks
Designing truly sustainable, multifunctional grazing landscapes requires expanding our thinking and narratives beyond narrow discussions informed by greenhouse gas emissions or carbon footprint assessments. The contribution of livestock to global warming and the need to reduce our consumption of animal products are undeniable. Yet the positive roles that grazing livestock can play in ecologically intensive management systems must also be acknowledged, particularly when thinking about strategies to curtail the current trends of biodiversity loss. Since the area of nature reserves and conservancies represents barely 5% of the terrestrial surface area of the globe, it is obvious that biodiversity conservation has to take place mostly in production landscapes. Grazing landscapes offer habitat for many species of plants, animals and microorganisms, but such habitats may be disrupted rapidly, either under industrial intensification or through overgrazing and land degradation in more traditional systems. Livestock systems differ widely in their current productive and environmental performances, and the trade-offs with maintaining viable rural livelihoods need to be quantified within each specific socio-ecological context. Although not addressed in this manuscript, trade-offs between short-term productivity and social well-being are also conspicuous in the livestock sector.
Agroecology provides the knowledge and guiding principles to design ecologically intensive grazing landscapes that can contribute to reducing the various production-environment trade-offs examined here. Yet this knowledge is not enough to transform landscapes if it is not conveyed to farmers, adapted and co-constructed with them, disseminated beyond the farm gate through multi-actor innovation platforms. Although inspiring examples of ecologically intensive grazing exist, and their numbers are growing, broad and lasting transitions at scale require conducive policy environments that address not only the production but also the manufacturing, trading and consumption of animal products. Current industrial livestock farms serve an inequitable global food system that, next to falling short of providing food for all, promotes the irresponsible overconsumption of cheap, unhealthy and unsustainable animal products in certain parts of the world, contributing to an obesity epidemic that affects 1,300 million people worldwide. Thus, “Feeding the world” should no longer be used as a supposedly altruist argument in favour of intensifying livestock production in unsustainable ways.
Author Contributions
The author confirms being the sole contributor of this work and has approved it for publication.
Funding
Partial funding was obtained from the project Resilientes: Producción resiliente de alimentos en sistemas hortícolas-ganaderos de la Agricultura Familiar en regiones climáticamente vulnerables de Argentina y Colombia, EUROCLIMA+ (https://euroclimaplus.org/proyectos-alimentos-es-2/produccion-en-regiones-vulnerables), GIZ-EU - RFP2018027.
Conflict of Interest
The author declares that the research was conducted in the absence of any commercial or financial relationships that could be construed as a potential conflict of interest.
References
Abdalla, M., Hastings, A., Chadwick, D. R., Jones, D. L., Evans, C. D., Jones, M. B., et al. (2018). Agriculture, Ecosystems and Environment Critical review of the impacts of grazing intensity on soil organic carbon storage and other soil quality indicators in extensively managed grasslands. Agric. Ecosyst. Environ. 253, 62–81. doi: 10.1016/j.agee.2017.10.023
Albicette, M. M., Leoni, C., Ruggia, A., Scarlato, S., Albín, A., and Aguerre, V. (2017). A co-innovation approach in family-farming livestock systems in Rocha - Uruguay: three-year learning process. Outlook Agric. 46, 92–98. doi: 10.1177/0030727017707407
Alkemade, R., Reid, R. S., van den Berg, M., de Leeuw, J., and Jeuken, M. (2013). Assessing the impacts of livestock production on biodiversity in rangeland ecosystems. Proc. Nat. Acad. Sci. 110, 20900–20905. doi: 10.1073/pnas.1011013108
Azpiroz, A. B., and Blake, J. G. (2016). Associations of grassland birds with vegetation structure in the Northern Campos of Uruguay. Condor 118, 12–23. doi: 10.1650/CONDOR-15-49.1
Becoa, G., Astigarraga, L., and Picasso, V. D. (2014). Greenhouse gas emissions of beef cow- calf grazing systems in Uruguay. Sustain. Agric. Res. 3:89. doi: 10.5539/sar.v3n2p89
Bonaudo, T., Bendahan, A. B., Sabatier, R., Ryschawy, J., Bellon, S., Leger, F., et al. (2014). Agroecological principles for the redesign of integrated crop–livestock systems. Eur. J. Agron. 57, 43–51. doi: 10.1016/j.eja.2013.09.010
Capper, J. (2010). Is the grass always greener? Comparing the environmental impact of conventional, natural and grass-fed beef production systems. Animals 2, 127–143. doi: 10.3390/ani2020127
De Vries, M., and De Boer, I. J. M. (2010). Comparing environmental impacts for livestock products: a review of life cycle assessments. Livestock Sci. 128, 1–11. doi: 10.1016/j.livsci.2009.11.007
Dias, R. A., Bastazini, V. A., and Gianuca, A. T. (2014). Bird-habitat associations in coastal rangelands of southern Brazil. Iheringia. Série Zool. 104, 200–208. doi: 10.1590/1678-476620141042200208
Do Carmo, M. G., Cardozo, M., and Jaurena, P. S. (2019). Demonstrating control of forage allowance for beef cattle grazing Campos grassland in Uruguay to improve system productivity. Trop. Grasslands-Forrajes Tropicales 7, 35–47. doi: 10.17138/tgft(7)35-47
Dong, S. (2016). “Overview: pastoralism in the World,” in Building Resilience of Human-Natural Systems of Pastoralism in the Developing World, eds S. Dong, K. A. S. Kassam, J. F. Tourrand, and P. B. Boone (Cham: Springer), 1–37. doi: 10.1007/978-3-319-30732-9_1
El Mujtar, N., Muñoz, B., Prack Mc Cormick, M., Pulleman, P., and Tittonell, P. (2019). Role and management of soil biodiversity for food security and nutrition; where do we stand? Global Food Secur. 20, 132–144. doi: 10.1016/j.gfs.2019.01.007
Elorriaga, Y., Marino, D. J., Carriquiriborde, P., and Alicia, R. (2013). Screening of pharmaceuticals in surface water bodies of the Pampas region of Argentina. Int. J. Environ. Res. Public Health 6, 330–339. doi: 10.1504/IJENVH.2013.056974
FAO (2018). Shaping the Future of Livestock – Sustainably, Responsibly, Efficiently. The 10th Global Forum for Food and Agriculture (GFFA). Available online at http://www.fao.org/3/i8384en/I8384EN.pdf (accessed June 7, 2021).
Gliessman, S. R. (2006). Agroecology: Ecological Processes in Sustainable Agriculture, 2nd Edn. New York, NY: Lewis Publisher, Boca Raton.
Herrero, M., Wirsenius, S., Henderson, B., Rigolot, C., Thornton, P., Havlík, P., et al. (2015). Livestock and the environment: what have we learned in the past decade? Ann. Rev. Environ. Resour. 40, 177–202. doi: 10.1146/annurev-environ-031113-093503
Herrero-Jáuregy, C., and Oesterheld, M. (2018). Effects of grazing intensity on plant richness and diversity: a meta-analysis. Oikos 127, 757–766. doi: 10.1111/oik.04893
Hill, S. B., and MacRae, R. (1995). Conceptual frameworks for the transition from conventional to sustainable agriculture. J. Sustain. Agric. 7, 81–87. doi: 10.1300/J064v07n01_07
Hoffmann, I., From, T., and Boerma, D. (2014). Ecosystem Services Provided by Livestock Species and Breeds, With Special Consideration to the Contributions of Small-Scale Livestock Keepers and Pastoralists. Rome: Food and Agriculture Organization of the United Nations (FAO), 158.
Jechalke, S., Heuer, H., Siemens, J., Amelung, W., and Smalla, K. (2014). Fate and effects of veterinary antibiotics in soil. Trends Microbiol. 22, 536–545. doi: 10.1016/j.tim.2014.05.005
Kentie, R., Senner, N. R., Hooijmeijer, J. C. E. W., Márquez-Ferrando, R., Figuerola, J., Masero, J. A., et al. (2016). Estimating the size of the dutch 1900 breeding population of continental black-tailed godwits from 2007–2015 1901 using resighting data from spring staging sites. Ardea 104, 213–225. doi: 10.5253/arde.v104i3.a7
Lezama, F., Baeza, S., Altesor, A., Cesa, A., Chaneton, E. J., and Paruelo, J. M. (2013). Variation of grazing- induced vegetation changes across a large-scale productivity gradient. J. Veg. Sci. 25, 8–21. doi: 10.1111/jvs.12053
Macfadyen, S., Tylianakis, J. M., Letourneau, D. K., Benton, T. G., Tittonell, P., et al. (2016). The role of food retailers in improving resilience in global food supply. Global Food Secur. 7, 1–8. doi: 10.1016/j.gfs.2016.01.001
McGinn, D. M., Chen, D., Loh, Z., Hill, J., Beauchemin, K. A., and Denmead, O. T. (2008). Methane emissions from feedlot cattle in Australia and Canada Australian. J. Exp. Agric. 48, 183–185. doi: 10.1071/EA07204
McInerney, J. (2004). Animal Welfare, Economics and Policy. Report on a Study Undertaken for the Farm and Animal Health Economics Division of Defra, 75.
Modernel, P., Astigarraga, L., and Picaso, V. (2013). Global versus local environmental impacts of grazing and confined beef production systems. Environ. Res. Lett. 8:35052.
Modernel, P., Dogliotti, S., Alvarez, S., Corbeels, M., Picasso, V., Tittonell, P., et al. (2018). Identification of beef production farms in the Pampas and Campos area that stand out in economic and environmental performance. Ecol. Indicators 89, 755–770. doi: 10.1016/j.ecolind.2018.01.038
Modernel, P., Picasso, V., Do Carmo, M., Rossing, W. A. H., Corbeels, M., Soca, P., et al. (2019). Grazing management for more resilient mixed livestock farming systems on native grasslands of southern South America. Grass Forage Sci. 74, 1–14. doi: 10.1111/gfs.12445
Modernel, P., Rossing, W. A. H., Corbeels, M., Dogliotti, S., Picasso, V., and Tittonell, P. (2016). Land use change and ecosystem service provision in Pampas and Campos grasslands of southern South America. Environ. Res. Lett. 11:113002. doi: 10.1088/1748-9326/11/11/113002
More, S. J., Hanlon, A., Marchewka, J., and Boyle, L. (2017). Private animal health and welfare standards in quality assurance programmes: a review and proposed framework for critical evaluation. Vet. Rec. 180:612. doi: 10.1136/vr.104107
Nielsen, B. L., and Zhao, R. (2012). Farm animal welfare across borders: a vision for the future. Anim. Front. 2, 46–50. doi: 10.2527/af.2012-0048
Nosetto, M. D., Jobbágy, E. G., Brizuela, A. B., and Jackson, R. B. (2012). The hydrologic consequences of land cover change in central Argentina. Agric. Ecosyst. Environ. 154, 2–11. doi: 10.1016/j.agee.2011.01.008
Novotny, I. P., Fuentes-Ponce, M. H., Tittonell, P., Lopez-Ridaura, S., and Rossing, W. A. H. (2020). Back to the people: the role of community-based responses in shaping landscape trajectories in Oaxaca, Mexico. Land Use Policy 100:104912. doi: 10.1016/j.landusepol.2020.104912
Opio, C., Gerber, P., Mottet, A., Falculli, A., Tempio, G., MacLeod, M., et al. (2013). Greenhouse Gas Emissions From Ruminant Supply Chains- A Global Life Cycle Assessment. Rome: Food and Agriculture Organization of the United Nations (FAO), 214.
Pacín, P., and Oesterheld, M. (2015). Closing the technological gap of animal and crop production through technical assistance. Agric. Syst. 137, 101–107. doi: 10.1016/j.agsy.2015.04.007
Picasso, V., Schaefer, D. M., Modernel, P., and Astigarraga, L. (2018). Ecological intensification of beef grazing systems. Grassland resources for extensive farming systems in marginal lands. Grassl. Sci. Eur. 22, 218–220.
Piñeiro, G., Paruelo, J. M., Oesterheld, M., and Jobbágy, E. G. (2010). Pathways of grazing effects on soil organic carbon and nitrogen. Rangeland Ecol. Manage. 63, 109–119. doi: 10.2111/08-255.1
Pizzio, R., Herrero-Jauregui, C., Pizzio, M., and Oesterheld, M. (2016). Impact of stocking rate on species diversity and composition of a subtropical grassland in Argentina. Appl. Veg. Sci. 19, 454–461. doi: 10.1111/avsc.12229
Rossing, W. A. H., Albicette, M. M., Aguerre, V., Leoni, C., Ruggia, A., and Dogliotti, S. (2021). Crafting actionable knowledge on ecological intensification: lessons from co-innovation approaches in Uruguay and Europe. Agric. Syst. 190:103103. doi: 10.1016/j.agsy.2021.103103
Ruggia, A., Scarlato, S., Cardozo, G., Aguerre, V., Dogliotti, S., Rossing, W., et al. (2015). “Managing pasture-herd interactions in livestock family farm systems based on natural grasslands in Uruguay,” in 5th International Symposium for Farming Systems Design “Multi-Functional Farming Systems in a Changing World.”, eds G. Emmanuel and J. Wery (Montpellier: FSD5), 267–268.
Schieltz, J. M., and Rubenstein, D. I. (2016). Evidence based review: positive versus negative effects of livestock grazing on wildlife. What do we really know? Environ. Res. Lett. 11:113003. doi: 10.1088/1748-9326/11/11/113003
Soca, P., Espasandín, A. C., and Carriquiry, M. (2013). Efecto de la oferta de forraje y grupo genetico de las vacas sobre la productividad y sostenibilidad de la cría vacuna en campo natural. Instituto Nacional de Investigacian Agropecuaria, Serie FPTA-INIA No48, Montevideo, Uruguay.
Solano-Hernandez, A., Bruzzone, O., Groot, J., Laborda, L., Martínez, A., Tittonell, P., et al. (2020). Convergence between satellite information and farmers' perception of drought in rangelands of North-West Patagonia, Argentina. Land Use Policy 97:104726. doi: 10.1016/j.landusepol.2020.104726
Tanentzap, A. J., and Coomes, D. A. (2012). Carbon storage in terrestrial ecosystems: do browsing and grazing herbivores matter? Biol. Rev. 87, 72–94. doi: 10.1111/j.1469-185X.2011.00185.x
Tittonell (2014). Ecological intensification – sustainable by nature. Curr. Opin. Environ. Sustain. 8, 53–61. doi: 10.1016/j.cosust.2014.08.006
Tittonell, P. (2018). “Ch. 13 Ecological Intensification of Agriculture,” in Agriculture and Food Systems to 2050 Global Trends, Challenges and Opportunities, eds R. Serraj and P. Pingali (Hackensack, NJ: World Scientific), 437–472. doi: 10.1142/9789813278356_0013
Tittonell, P. (2020a). Assessing resilience and adaptability in agroecological transitions. Agric. Syst. 184:102862. doi: 10.1016/j.agsy.2020.102862
Tittonell, P. (2020b). “Intensificacion ecologica sostenible de la agricultura,” in Sistemas productivos sostenibles: bases y experiencias para repensar el modelo de producción agrícola y sus relación con la ganadería, ed E. H. Satorre (Ciudad Autónoma de Buenos Aires: Asociación Argentina de Consorcios Regionales de Experimentación Agrícola – AACREA), 334.
Tittonell, P., Klerkx, L., Baudron, F., Félix, G. F., Ruggia, A., van Apeldoorn, D., et al. (2016). Ecological intensification: local innovation to address global challenges. Sustain. Agric. Rev. 19, 1–34. doi: 10.1007/978-3-319-26777-7_1
Tittonell, P., Piaeiro, G., Garibaldi, L. A., Dogliotti, S., Olff, H., and Jobbagy, E. G. (2020). Agroecology in large scale farming: a research agenda. Front. Sustain. Food Syst. doi: 10.3389/fsufs.2020.584605
Keywords: agroecology, sustainability, livestock, trade-offs, environment, carbon footprint
Citation: Tittonell P (2021) Beyond CO2: Multiple Ecosystem Services From Ecologically Intensive Grazing Landscapes of South America. Front. Sustain. Food Syst. 5:664103. doi: 10.3389/fsufs.2021.664103
Received: 04 February 2021; Accepted: 14 May 2021;
Published: 22 June 2021.
Edited by:
Pablo Gregorini, Lincoln University, New ZealandReviewed by:
Ritodhi Chakraborty, Lincoln University, New ZealandGwen-Aelle Grelet, Manaaki Whenua Landcare Research, New Zealand
Copyright © 2021 Tittonell. This is an open-access article distributed under the terms of the Creative Commons Attribution License (CC BY). The use, distribution or reproduction in other forums is permitted, provided the original author(s) and the copyright owner(s) are credited and that the original publication in this journal is cited, in accordance with accepted academic practice. No use, distribution or reproduction is permitted which does not comply with these terms.
*Correspondence: Pablo Tittonell, tittonell.pablo@inta.gob.ar