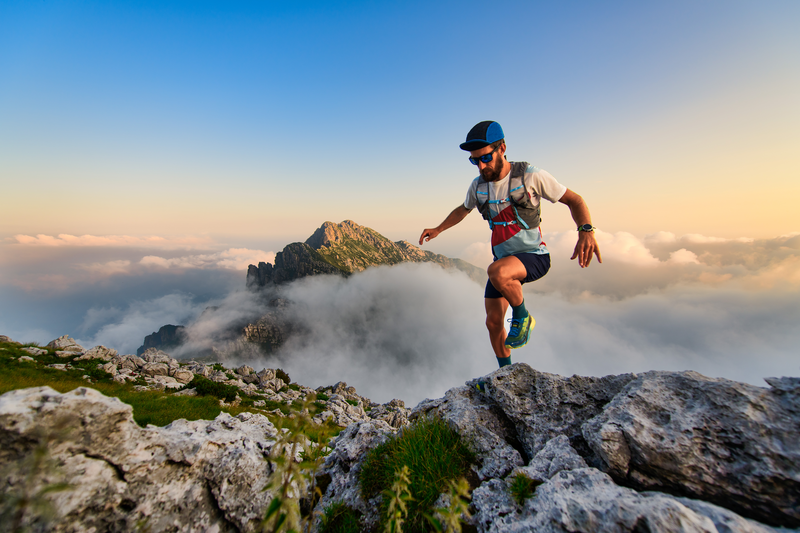
94% of researchers rate our articles as excellent or good
Learn more about the work of our research integrity team to safeguard the quality of each article we publish.
Find out more
REVIEW article
Front. Sustain. Food Syst. , 14 October 2021
Sec. Climate-Smart Food Systems
Volume 5 - 2021 | https://doi.org/10.3389/fsufs.2021.657936
This article is part of the Research Topic Greenhouse Gas Emissions and Emissions Mitigation from Agricultural and Horticultural Production Systems View all 10 articles
Cattle production systems are an important source of greenhouse gases (GHG) emitted to the atmosphere. Animal manure and managed soils are the most important sources of emissions from livestock after enteric methane. It is estimated that the N2O and CH4 produced in grasslands and manure management systems can contribute up to 25% of the emissions generated at the farm level, and therefore it is important to identify strategies to reduce the fluxes of these gases, especially in grazing systems where mitigation strategies have received less attention. This review describes the main factors that affect the emission of GHG from manure in bovine systems and the main strategies for their mitigation with emphasis on grazing production systems. The emissions of N2O and CH4 are highly variable and depend on multiple factors, which makes it difficult to use strategies that mitigate both gases simultaneously. We found that strategies such as the optimization of the diet, the implementation of silvopastoral systems and other practices with the capacity to improve soil quality and cover, and the use of nitrogen fixing plants are among the practices with more potential to reduce emissions from manure and at the same time contribute to increase carbon capture and improve food production. These strategies can be implemented to reduce the emissions of both gases and, depending on the method used and the production system, the reductions can reach up to 50% of CH4 or N2O emissions from manure according to different studies. However, many research gaps should be addressed in order to obtain such reductions at a larger scale.
Greenhouse gas (GHG) concentrations in the world have increased rapidly since pre-industrial times due to human activities, with negative effects on the climate(IPCC (Intergovernmental Panel on Climate Change), 2013). Methane (CH4) concentrations have doubled while nitrous oxide (N2O) concentrations in the atmosphere are 20% higher than pre-industrial levels (IPCC (Intergovernmental Panel on Climate Change), 2013). Agriculture is considered one of the main sources of CH4 and N2O, two high warming potential gases. Within the agricultural sector, animal production contributes 14.5% of human-induced emissions (Gerber et al., 2013) and produces ~37 and 65% of global emissions of CH4 and N2O, respectively (Steinfeld and Wassenaar, 2007).
Within livestock, cattle production systems can be broadly classified into confined, mixed -in which cattle can be in-house during part of the day or the year, and grassland-based systems (Seré and Steinfeld, 1995). In confined and semiconfined systems, manure can be stored and processed to be disposed in the field, whereas in grazing systems, manure is deposited directly on pastures and is degraded under environmental and grazing conditions (Uchida et al., 2011). Manure (feces and urine) managed and deposited on grasslands and pastures is the second largest source of GHG emissions after enteric methane and is responsible for ~7% of agricultural emissions of CH4 and N2O worldwide (Aguirre-Villegas and Larson, 2017).
Nitrous oxide is the third most abundant GHG and accounts for 6% of all radiative forcing (Myhre et al., 2013). Despite its low concentration in the atmosphere compared to CH4 and CO2, N2O has a significant effect on global warming, as it has a lifespan of ~120 years and 265 times higher radiative potential than CO2 (IPCC (Intergovernmental Panel on Climate Change), 2014; U. S. E. P. A and United States Environmental Protection U. S. E. P. A. United States Environmental Protection Agency, 2021). In addition, it contributes significantly to the depletion of stratospheric ozone (Myhre et al., 2013).
Grasslands around the world emit about 2.2 Tg of N2O–N, 74% of which comes from anthropogenic sources (Dangal et al., 2019). Deposition of animal feces and urine is the biggest source of N2O emissions per year in grasslands (54%), followed by manure application (13%), and nitrogen fertilizers (7%) (Dangal et al., 2019). Nitrification and denitrification are the main responsible mechanisms for the production of N2O in soils, although nitrification-denitrification, codenitrification and chemodenitrification can also lead to the formation of N2O given a microbial community and suitable environmental conditions (Hallin et al., 2018). Regarding methane, ruminant manure is responsible for the emissions of 109 million tons of this GHG to the atmosphere per year, of which 86% comes from cattle. Three main factors affect the amount of CH4 emitted by manure: the type of storage, the climate, and the composition of manure (Opio et al., 2013). While most of CH4 emissions from manure occur during storage under anaerobic conditions, in tropical regions manure can also be a generator of a considerable amount of emissions of this gas at the grassland level (Montes et al., 2013; Cai et al., 2017).
However, it is important to mention that although these gases play an important role in global warming, as they are predominantly flow pollutant gasses, they differ in their impact from CO2 that is a stock pollutant with a very long-term persistence in the atmosphere and consequently with a greater cumulative effect on the climate (Lynch et al., 2021). In addition, grasslands around the world also hold a large mitigation potential for building and conserving soil carbon and could capture as much as 0.5 Pg C per year to 1 m depth, as they cover ~52.5 million km2 equivalent to 40.5% of the land area (Gerber et al., 2013; Lorenz and Lal, 2018).
This article reviews the magnitude of typical N2O and CH4 emissions from manure, analyses the factors affecting them, and discuss potential mitigation strategies in bovine production systems, with an emphasis on tropical and subtropical regions where grazing systems are predominant.
Ruminants are poor nitrogen converters, because only 5–30% of ingested nitrogen are up taken by the animal and the remaining 70–95% are excreted via feces and urine (Luo et al., 2010). Therefore, nitrogen loads in animal excreta, often exceed plant demands and are vulnerable to losses via gaseous emissions and leaching (Selbie et al., 2015). This is more critical as the proportion of nitrogen in animal urine has increased with increasing nitrogen intake; although it has remained relatively constant in feces (Jarvis et al., 1995). According to Jaimes and Correa (2016) the efficiency in the use of N by lactating cows varies between 8.96 and 27.82% in Colombia, which, according to the number of animals per unit area can generate the application of up to 374 kg of N/ha/yr from manure (Correa et al., 2012). Likewise, Rivera et al. (2018) found that cows excreted 72% of ingested nitrogen in tropical dairy systems, generating the deposition of 46.8 kg N/animal/yr from manure and 42.9 kg from urine when the diet had on average 14% crude protein (CP). For this reason, improving the efficiency in the use of this nutrient by ruminants may be a viable alternative not only to increase animal productivity but also to reduce GHG emissions by reducing N excretion. It must be noted however that in extensive grazing and pastoralist systems cattle can be undernourished, and the scarce nutrients can be used more effectively by animals (Manzano and White, 2019).
Grazed pastures are systems with a wide range of environmental and management conditions that can result in the emission of N2O (Wecking, 2021). A large proportion of total farm N2O emissions in grazing systems often occurs from relatively small areas (Luo et al., 2017). These sites can be located where animals congregate (feeding bins, water troughs and gateways), occur after additional irrigation or result from soil compaction due to trampling and in the soil underneath excreta patches (Roesch et al., 2019). The magnitude of N2O emissions depends on the interplay between prevailing soil microclimate, microbial activity, plant composition, biomass, and excreta composition, that in turn is defined by animal type and feed intake (Wecking, 2021). All these factors can alter the spatial heterogeneity of soil respiration and, hence, cause impact also on resulting N2O emissions (Shi et al., 2019). Nitrous oxide emissions from a single application of cattle urine and feces can be as high as 16.8 kg N2O–N/ha/yr and 5.57 kg N2O–N/ha/yr, respectively (Luo et al., 2018). A meta-analysis by López-Aizpún et al. (2019) showed that, when reporting urine derived N2O emissions, it was important to account for differences in animal diet, sex and breed, in addition to urine composition and nitrogen loads.
The two main processes that generate N2O: nitrification and denitrification, are strongly influenced by climate and soil factors (Chen et al., 2008). The production of N2O depends on the availability of substrates for both processes, i.e., for nitrification and for denitrification (Zaman et al., 2007). The most important factors are the presence of oxygen, temperature, pH, humidity, salinity, and soil management; in the case of denitrification, it also depends on the carbon available for heterotrophic processes (Dalal et al., 2003). In addition, these factors are regulated by climate, vegetation, chemical, and physical properties of soil (apparent density, organic C, pH, and clay content), and agricultural management practices (Uchida et al., 2011). Each of these factors is discussed in more detail below.
The process of nitrification was first described by Schloesing and Muentz in 1877. Nine years later, Gayon and Dupetit discovered denitrification (Elmerich and Newton, 2007). An overarching framework capturing the production and consumption of N2O and NO by nitrification and denitrification within a conceptual model was published by Firestone and Davidson (1989) and has been acknowledged as the “hole in the pipe model” (Wecking, 2021). However, recent research suggests that a range of other biotic and abiotic pathways might also lead to the emission of N2O e.g., heterotrophic nitrification, nitrifier denitrification, chemodenitrification, coupled nitrification-denitrification, co-denitrification, and anaerobic NH3 oxidation– apart from potential other yet still undiscovered processes in the nitrogen-cycling network (Kuypers et al., 2018). Figure 1 shows the pathways of microbial driven nitrogen transformations in excreta patches in grassland ecosystems.
Figure 1. Pathways of microbial driven nitrogen transformations in excreta patches in grassland ecosystems (Cai et al., 2017). The N2O can also be produced by nitrifier denitrification, chemodenitrification and dissimilatory nitrate reduction to ammonium. The SOM and LON are soil organic matter and labile organic nitrogen, respectively.
Irrespective of the underlying process, most nitrification and denitrification pathways in soil lead to the net emission of N2O (Myrold, 2005). Only under certain conditions denitrifier activity, and to some extent that of nitrifiers, stimulate the uptake of atmospheric N2O. Soils are more likely to act as a sink for N2O when the soil mineral nitrogen is low and when high soil moisture or other factors prevent microbial access to alternative oxygen (O2) sources (Philippot et al., 2009).
Among the factors affecting N2O emissions, the availability of C and N is critical, particularly when these elements are in labile organic form (van Groenigen et al., 2005). There is a linear relationship between the N input, either by fertilizer or by manure, and the emission of N2O in agricultural areas (Dobbie et al., 1999); although, according to Zebarth et al. (2008), this relationship can also be exponential. In relation to soil properties, the moisture content is perhaps the variable with greatest influence on N2O emissions (Saggar et al., 2004). Saturation values of 60–70% moisture promote the generation of N2O since they limit the O2 diffusion, resulting in denitrification processes (Saggar et al., 2004). Nitrous oxide emissions are therefore higher in wet soils and after dry conditions, N2O production begins immediately after applying water to the soils (Chirinda et al., 2019).
Temperature is another factor influencing the level of emissions. When soil water content is close to the maximum retention capacity, N2O emissions respond to temperature changes (Machefert et al., 2002). However, some studies have shown no significant relationships between emission and temperature variations mainly due to the higher influence of moisture content in the flow of gases (Singurindy et al., 2009). Soil type can also influence N2O emissions, mainly through their effect on drainage level and moisture content (Luo et al., 2010). Poorly drained soils have higher N2O emissions than well-drained soils (Oenema et al., 2007). Poor drainage and changes in the physical properties of soil such as compaction can affect transformations from N to N2O because they affect the soil oxygen diffusion (Oenema et al., 1997) and can increase N gaseous losses (van der Meer, 2008).
Animal grazing with its trampling can favor this condition compared with grasslands without animal occupation. Compaction can also be increased when grazing takes place during winter and/or when there is inadequate management of animal stocking rates as this causes loss of structure and drastic decrease of porous space (Luo et al., 2010). pH can also affect the mechanisms that control N2O emissions. A study of denitrifying enzymes found a link between soil pH and soil emission rate (van der Weerden et al., 1999), as denitrification processes decrease as soil pH tends to acidity. According to Dalal et al. (2003), the optimal pH for nitrification activity is 7, while for denitrification the optimal pH is 7.0–8.0.
Most studies comparing N2O–N emission factors of feces and urine under grazing conditions suggest higher values for urine (van Groenigen et al., 2005; López-Aizpún et al., 2020). However, authors such as Sherlock et al. (2003) have reported similar values for both components, while Wachendorf et al. (2008) found higher emissions of N2O–N in livestock feces, suggesting the need of disaggregating emission factors based on the type of excreta (Luo et al., 2010; López-Aizpún et al., 2020). According to Meng et al. (2014) and Sordi et al. (2014), the fraction of N lost as N2O–N in the urine is greater than that of feces because only a relatively small fraction of N in manure is in an unstable condition and this depends on the diet of the animals.
According to the Intergovernmental Panel on Climate Change (IPCC) guidelines, it is estimated that the generation of N2O by manure deposited in the grasslands corresponds to 2% of the total excreted N (IPCC (Intergovernmental Panel on Climate Change), 2006). However, some studies have found that this value may be considerably lower, to such a point that by 2019 this same body changed that value to 0.4% to estimate direct emissions and another 0.27% for indirect emissions by leaching and volatilization (IPCC (Intergovernmental Panel on Climate Change)., 2019). In a study carried out in New Zealand, Luo et al. (2008) reported emission factors (EF) for urine applications between 0.2 and 1.59%, depending on the season. This variability in EF highlights the importance of determining country-specific or climate region emissions. This variability can be caused by multiple factors such as: (i) moisture, carbon content, pH and soil structure; (ii) environmental conditions such as temperature and rain (due to its influence on soil moisture); (iii) quantity and availability of nutrients in soil and excreta; (iv) plant species present; and (v) soil management (Oertel et al., 2016; López-Aizpún et al., 2020).
Countries like New Zealand have advanced in disaggregating EF by type of livestock (bovines and sheep), type of excreta (feces and urine) and climate (wet and dry). In this country, EF for urine and feces are 1 and 0.25% respectively, and both have been implemented in the national inventory of agricultural greenhouse gases (van der Weerden et al., 2020). New Zealand values, applied to all major classes of livestock (sheep, cattle, and deer), are similar to those found by Chadwick et al. (2018) in studies in the United Kingdom (average urine and feces of 0.69 and 0.19%, respectively) and Ireland where EF of 1.18 and 0.31% have been found for these two emission sources, respectively (Krol et al., 2016). Similarly, Rivera et al. (2018) found EF between 1.37 and 1.77% for feces, and between 0.3 and 3.47% for urine in tropical conditions of Colombia, which are higher than those reported by Sordi et al. (2014) in Brazil who found values from 0.19 to 0.33% and 0.12 to 0.19% for urine and feces, respectively. Figure 2 represents the final distribution of N in urine and feces, showing higher amounts of N leached and higher amounts of NH3 in urine than in feces, which could lead to higher emissions in urine patches.
Figure 2. Fate of nitrogen losses from cattle urine and feces patches distinguished into a leaching (blue), gaseous (orange) and plant uptake (green) component. DON stands for dissolved organic nitrogen. The size of the circles can be put into perspective compared to the two large black circles that reference a patch nitrogen load of 1,000 g. Figure adapted from Cai and Akiyama (2016) and Wecking (2021).
The production of CH4 occurs via the microbial degradation of the proteins, organic acids, carbohydrates, and soluble lipids present in excreta (Khan et al., 1997). According to the IPCC-Tier 1 (2006), 1 kg of CH4 is emitted from dung annually per adult head of cattle in grazing systems, but according to others reports these values may be lower (0.45–0.67 kg/animal/day), and can be highly variable (IPCC (Intergovernmental Panel on Climate Change)., 2019).
In the soil, CH4 is produced under anaerobic conditions by methanogens and is converted to CO2 by methanotrophs under both aerobic and anaerobic conditions, and the net CH4 flux in the soil-atmosphere system represents the balance between these two microbial processes (Le Mer and Roger, 2001). The impact of excreta deposition on CH4 emission thus mainly depends on its relative impact on CH4 production and CH4 oxidation activities in the patch (Cai et al., 2017).
Around 15–30% of total global CH4 emissions could be derived from soil source (Yanan et al., 2018). Ruminant manure is responsible for the emissions of 109 million tons of this GHG to the atmosphere per year, of which 86% comes from cattle. Three main factors affect the amount of CH4 emitted by manure: the type of treatment, the climate, and the composition of manure (Opio et al., 2013). While most of CH4 emissions from manure occur during storage under anaerobic conditions, in tropical regions manure can also be a generator of a considerable amount of emissions of this gas at the grassland level (Montes et al., 2013; Cai et al., 2017).
Even though pastures can emit CH4, under certain conditions, upland soils including those covered by grasslands are also an important sink for atmospheric CH4 as they can oxidize it at a faster rate than croplands (between 3 and 6 kg of CH4/ha/yr), although at a slower rate than uncultivated soils (Boeckx and Van Cleemput, 2001). This is caused by the oxidative activity of methanotrophs and ammonium oxidizing bacteria (Shukla et al., 2013). The oxidative capacity is nevertheless affected, among other factors, by water content and inorganic N in the soil, by the use of inorganic fertilizers and by the released during urine urea hydrolysis (Le Mer and Roger, 2001; Saari et al., 2004). During the rainy season CH4 emissions rise due to increased anaerobic conditions caused by water saturation but, when soil moisture is reduced due to decreased rainfall, these are replaced by oxidative processes with predominance of aerobic bacteria that generate negative methane flows and act as CH4 sinks (Visscher et al., 2007).
As for N2O, microbial processes that determine methane emissions into the atmosphere in grazing systems are conditioned by soil factors such as redox potential, pH, temperature, organic carbon and nitrogen content (Towprayoon et al., 2005). These factors can affect the proliferation of some soil microorganisms and, in turn, promote or limit bacterial metabolism through its impact on synthesis and enzymatic activity. The process of methanogenesis is regulated by the concentration of O2, the content of organic matter as a substrate, and the factors that determine its redox potential (Conrad, 1996). Organic matter is the main input for triggering methane production processes. The increase of available organic matter, and its subsequent decomposition in soils under anaerobic conditions, stimulates methanogenesis by providing a substrate for the production of acetate and hydrogen and causing soil reducing conditions (Sass et al., 1991).
In pastures, most of the organic matter comes from plants through leaves senescence and root decomposition, and the transformation and deposit by animal excreta (Waschütza et al., 1992). For this reason, forage species and their physiological status can also influence methane emissions (Kerdchoechuen, 2005). The strictly anaerobic methanogens, mainly Methanobacteria, Methanococci and Methanopyri, are sensitive to changes in soil water content (Malyan et al., 2016). Methanogenic activity may be stimulated by urine deposition that create anaerobic conditions. Furthermore, increased soil pH resulting from hydrolysis of urine urea and decreased redox potential may also favor methanogenic activities (Le Mer and Roger, 2001). Emissions of CH4 from excreta deposited by animals on pastures range from 7 to 27% of total emissions by ruminants (Kreuzer and Hindrichsen, 2006). However, these emissions may become less significant depending on environmental conditions and manure management (Oenema et al., 2007). When anaerobic conditions occur, ruminant manure in pastures release significant amounts of CH4 (Misselbrook et al., 2001).
In an evaluation of methane fluxes of an intensive silvopastoral system (iSPS) with high density of Leucaena, an intensive pasture monoculture system and a secondary dry forest, Rivera et al. (2018) found that both the forest and the iSPS had negative CH4 flows of −0.56 and −0.02 kg of CH4/ha/yr respectively, probably due to the biodiversity of microorganisms found in their soils (Vallejo et al., 2010). Negative flows of CH4 were also found during the dry season in three pasture systems in the north of Colombia (Espinosa-Carvajal et al., 2020). Methane flows are also influenced by the grass species and fertilization level. Pastrana et al. (2011) in a study evaluating three accessions of Brachiaria humidicola (Rendle) Schweickerdt found that methane emissions were increased from sink (−23.6 μg m2/h) when nitrogen fertilization was zero, to emit 107.9 μg m2/h with application of 150 kg of N/ha/yr and 59.7 μg m2/h with 300 kg of N/ha/yr. CH4 emissions were also affected by the B. humidicola accession.
CH4 emissions from excreta deposited by animals on pastures range from 7 to 27% of total emissions by ruminants (Kreuzer and Hindrichsen, 2006). However, these emissions may become less significant depending on environmental conditions and manure management (Oenema et al., 2007). According to recent IPCC estimates, 0.49 ± 0.43 g of CH4 per kg of dry matter (DM) of manure can be generated on average (IPCC (Intergovernmental Panel on Climate Change)., 2019). In addition, urine deposited directly in the grasslands increases emissions of this gas by up to 100 times compared to grasslands without urine patches (Oertel et al., 2016). The diversity of conditions that change CH4 emissions has generated great dispersion in the results (Andueza et al., 2017), as there are reports of up to 1 g of CH4 per kg of DM of manure generated, value that can be up to five times higher when manure is incorrectly stored under anaerobic conditions (Sneath et al., 2006). According to Chadwick (2005) manure emissions can range from 0.4 to 9.7% of the total C content deposited by manure.
Finally, Life Cycle Analysis (LCA) studies have identified that manure emissions (CH4 and N2O) can be considerable under different production conditions. For example, Rivera et al. (2014), who evaluated the LCA in two dairy systems in Colombia, found that manure emissions accounted for 30% as CO2-eq of the total emitted on the farm and 22% of the total emitted throughout the LCA. In another study in dairy production systems, Rivera et al. (2016) found that manure emissions accounted for 6.5% of all farm-level emissions. According to this study, emission levels from manure depend mainly on the amount of excreted N given by the consumption of this nutrient in the diet, by the type of manure management, and by the rainfall regimen and use (or not) of irrigation in the grasslands.
Opportunities to reduce N2O and CH4 emissions from livestock manure are diverse and can be addressed to different parts of the animal production cycle to control the production and emission of these two gases (Montes et al., 2013). Given the different environmental and metabolic conditions that influence N2O and CH4 flows from soil and manure, it is difficult to implement efficient mitigation alternatives that target both gases simultaneously (Montes et al., 2013). However, as presented in Table 1; Figure 3, measures related to improving production efficiency and improving soil protection with adequate cover and introduction of trees can contribute simultaneously to reduce emissions of both gases and, in addition, improve carbon sequestration.
Table 1. Mitigation alternatives and potential for reducing CH4 and N2O emissions in grazing systems.
Figure 3. Mitigation strategies classification, limitations and potential for reducing CH4 and N2O emissions in grazing systems.
The success of mitigation measures can be estimated based on the optimal conditions under which nitrification and denitrification processes occur, in the case of N2O and from the dry matter degradation processes of manure for CH4. Since CH4 is produced under anaerobic conditions, while N2O production requires sufficient oxygen levels, some practices that reduce CH4 production tend to increase N2O emissions. Table 1 presents a list of mitigation alternatives with their potential impact and limitations.
According to de Klein and Eckard (2008) and Wecking (2021), strategies to mitigate N2O emissions from grazing systems should include two complementary approaches: (1) to manage the sources of nitrogen uptake at the animal level, and (2) to control N2O production in situ through soil and pastoral management. Beukes et al. (2010) determined that measures such as improved animal genetics, reduction of nitrogen fertilization, and improved grazing management had the potential to decrease GHG emissions from pasture-based systems by 27–32%. Future mitigation should ideally be focused on finding combined strategies that avoid any offsetting effect of the desired mitigation benefits and also, help to improve the efficiency of nitrogen cycling through the soil-plant-animal system while, at the same time, reducing emissions (Cai et al., 2017).
The first approach includes improving management and feeding practices, supplying nutrients, in particular protein sources, according to animal requirements, and increasing animal productivity and nitrogen efficiency per kilogram of animal product through breeding and genetic manipulation (de Klein and Eckard, 2008; Wecking, 2021). For the second approach, mitigation strategies addressed at soil and grazing management are manifold and include managing the intensity and timing of grazing events (van der Weerden et al., 2018), increasing pasture productivity and soil carbon storage (Whitehead et al., 2018), and improving nutrient, fertilizer, and manure management (Kim and Giltrap, 2017). Removing animals from pasture areas can reduce treading damage, prevent leaching and gaseous losses of N, and thus preserve soil conditions (Luo et al., 2017). However, negative side effects of stand-off pads can be the accumulation of manure and reduced production that might outweigh the mitigation benefits. van der Weerden et al. (2018) showed that controlled grazing was beneficial on poorly drained soils where it contributed to reducing N2O emissions, whereas the approach was not suitable on imperfectly-drained pasture.
Nitrous oxide and CH4 emissions differ between intensive and non-intensive systems. Intensive systems can emit more GHG because they have a higher stocking rate, offer commercial feed, use fertilizers and irrigation. However, under tropical and subtropical conditions extensive systems are predominant; non-intensively managed pastures occupy 66% of the total grassland area around the world (Klein Goldewijk et al., 2017). Mitigation strategies must be in accordance with the type of system to achieve cost-effective reductions under grazing conditions. The main mitigation strategies are presented in more detail below:
The most promising options for reducing GHG emissions at the livestock management level include improving animal production through dietary changes. Nitrogen (N) excretion rates, which affect N2O emissions from manure, are based on dry matter consumption (DMC) and its N content (Vergé et al., 2012). Therefore, dietary manipulation to optimize protein consumption, and thus improve the efficiency of N utilization, is one of the most effective measures to reduce emissions from manure (Novak and Fiorelli, 2010). The more nitrogen used by an animal, the less will be excreted; it is recommended that an adjusted amount of nutrients be offered in the diet to meet the animal's requirements, thus, avoiding increased excretion (Schils et al., 2008). This condition can occur in both high-supply N systems such as dairy production, as well as in tropical systems where the N supply in the feed is reduced. According to Klausner et al. (1998), in dairy systems, feeding of lactating cows with rations based on their production decreases the excretion of N by up to 34%. According to these authors, the reduction occurs by optimizing microbial fermentation in rumen which significantly improves the use of N.
Since urine is the main source of volatile N emissions, manipulating the N excretion pathway becomes an important N2O and NH3 mitigation tool. Urea is the main nitrogenous component of ruminant urine reaching 60–80% of total urinary N in high-production dairy cows (Montes et al., 2013) and decreasing proportionally as dietary CP decreases (Colmenero and Broderick, 2006). In low-protein diets, ureic N may drop to 46–53% of total urinary N (Hristov et al., 2011; Lee et al., 2012). For this reason, decreasing the concentration of CP in the diet is an effective method to mitigate N emissions from manure (Hristov et al., 2013). Similarly, emissions of manure deposited in the soil are reduced because low CP diets generate manure with a slower N mineralization rate (Powell et al., 2011). Optimizing N supply to animals can achieve between 12 and 21% less N excretion and 15–33% less N volatilization losses in livestock fed according to the physiological status of the animals (Erickson and Klopfenstein, 2010).
de Klein and Eckard (2008) concluded that N2O reduction should be part of an integrated approach to improving efficiency in the use of N in animal production systems. According to these authors, current technologies could offer up to 50% reduction in N2O emissions from a confinement system, but only up to 15% of a grazing system. In intensively operated pastoral systems, supplementation of cattle with N-low foods such as maize or silage, which generally reduce the concentration of N in the diet, can reduce urinary N losses and, consequently, NH3 and N2O emissions in manure and soil by 8–36% (de Klein and Monaghan, 2011).
Also, plants species such as Lolium perenne, Trifolium repens, and Plantago lanceolata, that may exhibit diuretic properties have the potential to reduce the urinary-N loading in individual urine patches by increasing the urination frequency of grazing animals (de Klein et al., 2019; des Roseaux et al., 2020). Although the increased urination frequency results in greater coverage of urine-affected soil, total paddock-scale N2O emissions from urine patches are not likely to be higher if the total amount of urinary-N excreted remains the same. In fact, they could be lower if the N2O emission factor reduces with N loading rate (de Klein et al., 2019).
Diet manipulation can also reduce CH4 emissions from manure. In a study by Lombardi et al. (2021) the supplementation of grazing beef steers with maize grain lowered CH4 emissions of dung from 4.0 to 1.7 g CH4-C/m2. Dung from supplemented animals had higher N, starch, and DM content, which resulted in lower CH4 emissions compared with dung from non-supplemented animals. Results from this study indicated that the initial water content may control the CH4 emissions, since rainfall events after dung crusting did not increase the CH4 fluxes from dung patches (Zhu et al., 2018). On the other hand, these authors state that supplementation with maize grain can thus have dual benefits in cattle production, through maximizing body weight gain in grazing steers (18% more) by improving the efficiency of utilization of nutrients (dietary N in particular) and through decreasing the total amount and/or the intensity of GHG emissions. In addition, the improvement in N utilization efficiency may reduce N2O emissions from urine deposition (Cai et al., 2017).
This mitigation route can be used especially in grazing systems with daily rotation, where there is a greater control in feeding. In these systems animals are usually supplemented during milking or at certain times of the year (for example in summer or winter, according to the area). Also, the manipulation of the diet can be done to certain groups of animals that may demand more nutrients or simply by using pastures with various forage species that can be supplemented or whose nutrient supply is in accordance with the physiological state of the animal. This could be applied to both intensive and non-intensive systems (use of species that favor an adequate energy:protein balance).
Although diet supplementation might be difficult under very extensive systems, it is possible in more managed systems such as those under rotational grazing.
Maintaining a more diverse environment with healthy soils and good pasture cover is another strategy that can help reduce emissions. Chirinda et al. (2019) found lower urine patch emission factors in seven locations in South America (0.42 vs. 0.18%) when the grasslands had greater plant cover compared to areas with poor cover or degraded pastures. The results indicate that, under rainy conditions, adequate plant cover, through good pasture management, helps reduce urine-induced N2O emissions. According to these authors, higher emissions in low-covered soils are due to grass degradation that can stimulate or restrict N losses. For example, low plant cover can reduce N sinks for deposited excreta and therefore increase N vulnerability and loss through microbial soil and leaching processes (Chirinda et al., 2019). However, low plant cover may also be associated with fewer exuded plant roots that decrease microbial activity and N2O emissions (Henry et al., 2008).
Improved soil cover and pasture management also contributes to maintain or increase soil organic matter (SOM) (Aryal et al., 2018), which plays a critical role in determining the N2O emission response to urea deposition (Clough et al., 2020). Increasing SOM increases cation exchange capacity, reducing the soil solution concentration that in turn, reduces soil solution NH3 and associated inhibition of oxidation, thus reducing urea-derived N2O emissions (Breuillin-Sessoms et al., 2017). Soil buffer capacity increases with increasing soil organic matter alleviating increases in soil pH following urea deposition and associated solubilization of organic matter, and the formation of dissolved organic C and N (Breuillin-Sessoms et al., 2017).
On the other hand, excessive grazing without time for grass recovery increases the risk of soil compaction, an indicator of grass degradation. Compaction reduces soil porosity and pore continuity, decreases aeration, restricts plant growth, and increases soil N2O emissions in urine patches (van Groenigen et al., 2005). In addition, soil acidification, which could also be an indicator of pasture degradation, has been shown to increase N2O emissions as acidic conditions generally reduce plant growth and inhibit the activity of the enzyme N2O reductase, which is responsible for transforming N2O into dinitrogen (N2) (Robinson et al., 2014).
The incorporation of shrubs and trees in pastures in the called silvopastoral systems (SPS) can also contribute to reduce emissions by improving soil cover and health and by increasing the quality of the diet (Chará et al. 2019). These systems have demonstrated effects on the physical, chemical and microbiological properties of the soil both by the provision of shade and higher amount of heterogeneous biomass that is deposited on the soil in the form of leaves, branches, fruits, and exudates and by improving the root microbiome allowing the modification of microorganism populations in the soil that can regulate nitrification and oxidation processes (Vallejo et al., 2010, 2012). Silvopastoral systems can also contribute to increase SOM (Aryal et al., 2018) which contributes to reduce GHG flows as mentioned previously. Cubillos et al. (2016) found in a study including SPS of different ages, that these arrangements have significantly lower potential for ammonia nitrification compared to monoculture systems (between 15 and 20%), similar to those observed in forest patches, which is why N2O flows are expected to be reduced under these systems. According to these authors, in SPSs ammonia oxidizing bacteria and archaea limit the rate in nitrification and the resulting N2O emissions could be reduced.
On the other hand, such systems can modify emissions in feces by the presence of dung beetles that limit the interactions of manure with mineral soil, restricting substrates for nitrification and denitrification processes (Slade et al., 2016). Studies in different regions of Colombia have shown that SPSs have greater abundance, diversity, and activity of beetles than treeless pastures (Giraldo et al., 2011; Montoya-Molina et al., 2016). According to Slade et al. (2016) the presence of beetles in livestock systems reduces N2O emissions by 14.7% and CH4 emissions by 17%.
Another effect of these systems is the increased N partitioning into dung relative to urine as this has shown to reduce N2O emissions from pastures, since the emission factor for dung is lower than that for urine (Luo et al. 2018). Feeding animals condensed tannin (CT)-rich diets can also increase N partitioning into dung (Carulla et al., 2005). The inclusion of CT either as a dietary supplement or in forages fed to ruminants reduced urinary-N excretion, increased the amount of N excreted in the dung and improved N retention in the animal (Misselbrook et al., 2005). Since N2O emissions are traditionally higher in urine, this may be a mitigation pathway. Silvopastoral systems use forage species such as Leucaena leucocephala, Tithonia diversifolia, and Gliricidia sepium, which contain significant amounts of tannins in their leaves and stems (Barahona et al., 2006; Rivera et al., 2021). Rivera et al. (2018) compared an iSPS and a traditional system and found reductions in emission factors (N2O) of 23% and 10 times less for feces and urine, respectively.
Finally, plant morphological factors that can affect soil N cycling and N2O emissions include the effect of the root system on a plant's ability to access water and nutrients, and plant canopy-effects on the dispersion of urine voided by grazing animals (de Klein et al., 2019). When roots are present, root morphology can affect soil structure and hydrology, both of which influence conditions that govern the reduction of N2O to N2 and diffusion of gases to the soil surface (Chapuis-lardy et al., 2007). Root morphology can also affect plant N uptake and thus availability for soil nitrification and denitrification processes (Abalos et al., 2014). These authors found that combining two grasses, L. perenne and Poa trivialis, which is a high fertility responsive grass like L. perenne, produced the greatest amount of biomass and the lowest N2O emissions. They suggested this was due to the complementarity of the root foraging strategies of these two species, where P. trivialis may access N hotspots not previously emptied by L. perenne. This combination, together with its very high total root biomass, is thought to increase mineral N uptake, thereby lowering soil nitrate content and subsequent N2O emissions (Abalos et al., 2014).
Plant species diversity and interactions can also influence N uptake and N2O emissions (Niklaus et al. 2016). Increasing plant species richness from 1 to 16 grassland species has been found to reduce N2O emissions in the absence of N fertilizer (Niklaus et al., 2016), due to more efficient soil inorganic N uptake. However, this pattern was not observed when the diversity included a large proportion of legumes (de Klein et al., 2019).
Although pasture species with increasing root mass or rooting depth have greater ability to take up N, the winter-activity of pasture species such as Lolium multiflorum Lam.—i.e., the ability of roots to take up N under cooler conditions—appeared to be more important than specific root architecture (e.g., deep roots) for reducing N leaching losses (Woods et al., 2016). Woods et al. (2016) found that winter-active Italian ryegrass had the greatest N uptake and lowest N leaching, whereas the opposite was found for the tap-rooted L. perenne. The high N leaching losses from M. sativa in this study were attributed to poor winter herbage growth and the limited depth of the lysimeters (0.7 m) used for this deep rooting species.
Biological nitrogen fixation (BNF) in association with forage legumes provides an alternative N source for grazing systems (Li et al., 2013). In tropical and subtropical conditions, legumes such as Desmodium ovalifolium, Leucaena leucocephala, Centrosema pubescens, Stylosanthes guianensis, Cajanus cajan, among others, can fix between 120 and 150 kg N/ha/yr. For SPS with Leucaena leucocephala planted in rows in Australia, nitrogen fixation rates range between 36 and 61.9 kg/ha/y (Radrizzani et al., 2011; Conrad et al., 2018) while for an iSPS with high density of L. leucocephala in Mexico ranged between 77.1 and 80 kg N/ha over a period of 100 days (Sarabia-Salgado et al., 2020). This fixed N becomes available slowly over time to the grass in pastures after its release into soil via exudates from living legume roots, by mineralization of senesced legume tissues and in excreta after consumption by grazing animals (Ledgard et al., 2009), generating lower losses of N that can be converted into N2O (Schmeer et al., 2014). Rising costs of fertilizer N and environmental regulations governing stocking densities and fertilizer N use on farms is increasing interest in the use of legumes in pastures. A review by Andrews et al. (2007) concluded that herbage and milk production from legumes-based pastures (perennial ryegrass with 20% white clover (Trifolium pratense) in herbage DM on an annual basis) are likely to be similar to that from a perennial ryegrass pasture receiving annual input of 200 kg/ha of N fertilizer and around 70% of that obtained with perennial ryegrass receiving an annual input of 350–400 kg/ha of fertilizer.
The N2O emissions induced by the growth of legume crops/forages may be estimated solely as a function of the above-ground and below-ground N inputs from crop residues (Li et al., 2013). Accordingly, N2O emissions from legume-based grasslands are much lower than fertilized grasslands. For example, Ruzjerez et al. (1994) reported up to five-fold more N2O emission from heavily N fertilized grasslands than from their legume-based counterparts in New Zealand. A data synthesis indicates that the average soil N2O emissions from field-grown legumes, N fertilized grass pastures and crops, and unfertilized soils are 1.29, 3.22, and 1.20 kg N/ha/yr, respectively (Li et al., 2013).
Within soil GHG emission mitigation strategies, especially for N2O flows, the use of nitrification inhibition grass species (BNI) is an option to reduce gas production (Byrnes et al., 2017; Teutscherova et al., 2019). According to Byrnes et al. (2017) and Beeckman et al. (2018) the use of nitrification inhibitors, whether synthetic or plant-based (biological nitrification inhibitors -BNI) reduce soil nitrification rates and therefore leachate and N2O emissions. In addition, this reduction in the nitrification rate contributes to increase the efficiency in the use of N in the system (Yang et al., 2016).
Species such as Brachiaria humidicola (Byrnes et al., 2017), Sorghum bicolor, Oryza sativa and Triticum aestivum have demonstrated their ability to decrease N2O flows (Subbarao et al., 2009; Zhu et al., 2012). Byrnes et al. (2017) reported that in urine patches with B. humidicola cv. Tully, N2O emissions were 60% lower than in B. hybrid cv. Mulato (32 vs. 80 mg N2O–N m2, respectively). These authors also found that the high content of had a positive relationship (p < 0.05) with the number of copies of the amoA gene of ammonia oxidizing archaea and bacteria at sites with higher emissions. Studies regulating soil N dynamics associated with plant and microorganism interaction such as BNI and, more recently, inhibition of biological denitrification (BDI), have increased, as they represent an ecological, sustainable, and cost-effective strategy compared to the use of synthetic inhibitors (Subbarao et al., 2017).
On the other hand, root exudates can also affect the availability of soil mineral N, as C in these exudates may temporarily increase microbial immobilization of N (Fisk et al., 2015). Carbon from root exudates may thus reduce N2O emissions derived from urine deposition due to immobilization of urine-N. Indeed, a number of studies have demonstrated that excess N in urine patches does not immediately become available for nitrification and denitrification (Bol et al., 2004). Instead, it can be immobilized into organic matter or fixed on clay particles.
Biochar is a carbonaceous material produced during the thermal decomposition of different materials (wood, plant litter, crop residues, animal manure or waste products) under low-oxygen conditions (Cai et al., 2017). Biochar may inhibit nitrification by compounds such as α-pinene and ethylene and constrain denitrification through physical and biochemical regulations, including improved soil aeration, increased sorption of substrates for denitrification, and increased soil pH to facilitate complete denitrification to N2 (Cayuela et al., 2014). A meta-analysis reports a 54% reduction of N2O emissions (with a confidence interval from −60 to −48%) following biochar addition to agricultural soils (Cayuela et al., 2014).
Biochar can also decrease CH4 emission or increase CH4 oxidation via increasing soil aeration and reducing soil bulk density, but some compounds contained in biochar may also inhibit the activity of methanotrophs and increase CH4 emission (van Zwieten et al., 2010).
Since increased pH can enhance the activity of N2O reductase, lime application should be able to reduce N2O emissions. Liming has also been shown to enhance nitrification (Khan et al., 2011). Therefore, the effect of liming on N2O emission depends on its net effect on N2O reductase and nitrification. Generally, liming can increase N2 emission and reduce the ratio of N2O to N2 for emission from urine patches, but there are contradictory results about its effect on N2O emission (McMillan et al., 2016). In addition, owing to the decreased toxicity as pH increases, liming has shown to increase soil CH4 oxidation and reduce CH4 emission; this effect might be more pronounced in acid soils (Kunhikrishnan et al., 2016). However, it should be noted that even though liming has merit for lowering N2O emissions, the increased nitrification after lime application may also potentially enhance a risk of N2O production from denitrification when the soils become anaerobic, due to the possible accumulation of the resultant from nitrification (Barton et al., 2013).
Given the many uncertainties of the effect of liming on N2O emission, caution should be exercised in using liming as an option for mitigating N2O from excreta patches, and the effect of liming on the emission of CO2, CH4, and NH3 from excreta patches should also be considered (Cai et al., 2017).
Since N2O emission from excreta patches mainly results from nitrification and denitrification, thus any inhibitor that can suppress these two processes could be used to mitigate N2O emissions from excreta patches Cai et al. (2017). Nitrification inhibitors (NIs) such as dicyandiamide (DCD), 3, 4-dimethylpyrazole phosphate (DMPP), nitrapyrin, and pyrazole derivatives (PD), can inhibit the conversion of to , decrease production and the subsequent denitrification (Barneze et al., 2015). Additionally, Urease inhibitors (UIs) can slow down the conversion of urea to and NH3 and decrease the availability of for nitrification (Cai et al., 2017). The efficacy of NIs in reducing N2O emission varies widely depending on the application rate, timing and method (Cai et al., 2017). Application of DCD significantly decreases N2O emissions from cattle urine (by 4.24 ± 1.10 kg N/ha) and cattle feces (by 0.66 ± 0.61 kg N/ha) patches (Cai et al., 2017). However, NIs may decrease CH4 oxidation (or increase CH4 emission) by the inhibitory effect of accumulating and possibly directly affect methane monooxygenase (MMO), presumably due to the close structural relationship between ammonia monooxygenase (AMO) and MMO activities (Le Mer and Roger, 2001; Hatch et al., 2005). Therefore, the effect of NIs on CH4 emission from both urine and feces patches needs to be further studied under a wide range of conditions, since uncertainties about the differential effect of urine and feces deposition on CH4 emission are large (Cai et al., 2017).
Although this mitigation pathway is effective, its applicability is difficult under grazing conditions, since these are systems where the excretion of urine and feces is dispersed; also, the costs could be high and may not outweigh the benefits.
According to Adhikari et al. (2021) in intensively managed dairy pastures, urine deposited by cattle during grazing covers relatively small proportion of the total grazed area. Maire et al. (2018) reported that urine patches represent only about 6–12% of the grazed area in a single dairy cow grazing event. Therefore, there is a large potential for limiting the risk of NIs entry into these systems if these compounds can be targeted directly to urine patches, avoiding the need for their application across often large areas of pasture unaffected by urine deposition during grazing. Most N2O emissions from a urine patch occur within the first few days to weeks of its deposition, depending on soil and climatic conditions (Selbie et al., 2015). Technologies are therefore needed that can identify and treat urine patches shortly after deposition to inform optimum mitigation options for emission reductions (Marsden et al., 2017). Approaches to identifying urine patches include visually monitoring cows in the field, automated monitoring using electromagnetic induction, electrical conductivity measurements and optical sensing (Misselbrook et al., 2016), ground-based sensing and airborne technologies, such as remotely piloted areal systems (RPAS), LiDAR and satellites using hyperspectral and near infra-red imaging, and temperature sensors (Dennis et al., 2013). The application rates for DCD, DMPP and nitrapyrin in previous field experiments involving urine ranged from 5 to 80, 1 to 5, and 1 to 10 kg/ha, respectively (Adhikari et al., 2021).
According to IPCC (Intergovernmental Panel on Climate Change). (2019), in bovine systems in tropical and subtropical areas only 4 to 15% of manure receives some type of management. Considering the manure handling chain, mitigation options involve adequate handling, storage, and application, especially in those systems where animals are kept in confinement permanently or during part of the day or the year. According to Montes et al. (2013) there are many options for mitigating emissions of N2O and CH4 during storage. For CH4 mitigation, during solid manure storage, composting can be an efficient mitigation option, if it is properly managed. Samer (2015) found that adding straw to solid manure reduces CH4 emissions, but under certain conditions, it could increase N2O emissions.
In general, the most effective methods are anaerobic digestion and composting, which in turn have the advantage of generating products that replace fossil fuels and chemical fertilizers. Generally speaking, to mitigate N2O emissions from manure deposits, the following are the best methods: (i) maintain anaerobic deposits (e.g., compact and covered); (ii) adopt a liquid manure system compared to a deep-bed system (although it has the drawback of increased water use); and (iii) add straw to immobilize ammonium. On the other hand, to mitigate CH4 emissions, the following are the best methods: (i) anaerobic digestion (Chadwick et al., 2011); (ii) water removal in manure (opposite to N2O mitigation); (iii) minimize the volume of liquid manure stored during the summer months; (iv) cooling; and (v) aeration of solid manure and composting heaps. According to these recommendations, apart from anaerobic digestion, there are no options to tackle both gases simultaneously, but there are some general strategies to reduce GHG emissions by manure management. Although some of the strategies proposed are efficient in reducing emissions, their actual mitigation potential must be evaluated against possible tradeoffs as they may rely on high use of electricity, fossil fuels, labor or water, or reduce productivity. For example, cooling, constant washing of excreta or the use of specialized structures, could increase costs or increase emissions of other gases such as CO2, or generate other negative environmental impacts such as eutrophication and acidification.
Integrated production systems such as silvopastoral systems are strategic to reduce emissions of both CH4 and N2O through the reduction in the use of external inputs (i.e., fertilizer and feed supplements), soil protection and improvement of its structure and aeration, and efficient use of nutrients in the production process. An efficient production system that provides nutrients to animals according to their requirements not only contributes to reducing emissions but also allows for more efficient production. Although the reduction of emissions for integrated systems can be as high as 50%, the uptake of these alternatives is still very low and many research gaps remain to make these reductions more generalized.
With regard to mitigation practices, it is important to note that these may result in an “emission exchange” or increase in the flows of some GHGs. Therefore, due to numerous interactions, mitigation practices should not be evaluated in isolation but as a component of the bovine production system (Montes et al., 2013). Optimizing the animal diet to improve the efficiency of N use, balance N input with production level and maintaining fiber digestibility while reducing enteric fermentation of CH4, are important steps to reduce N2O and CH4 emissions from manure. In addition, the use of BNI fodder, as well as providing adequate soil cover offered in well-managed systems, are strategies alternatives to reducing N2O emissions (Figure 3).
Finally, it is important to mention that some strategies, if applied separately, have different limitations and drawbacks as mentioned in Table 1. For this reason, it is important to advance in studies focused on evaluating the economic impact of mitigation strategies, and to determine their impact on animal and food production. It is also important to work on the estimation of CH4 emission factors and to evaluate mitigation strategies for this gas that has received less attention compared to N2O.
JR and JC were involved in the conceptual development of the article. JR led the general construction of the texts and the literature review. JC contributed to manuscript revision and proposed some mitigation strategies. All authors contributed to the article and approved the submitted version.
Project 18_III_106_COL_A_sustainable production strategies. This project is part of the International Climate Initiative (IKI). The Federal Ministry of Environment, Nature Protection and Nuclear Safety (BMU) of Germany supports this initiative based on a decision of the German Bundestag.
The authors declare that the research was conducted in the absence of any commercial or financial relationships that could be construed as a potential conflict of interest.
All claims expressed in this article are solely those of the authors and do not necessarily represent those of their affiliated organizations, or those of the publisher, the editors and the reviewers. Any product that may be evaluated in this article, or claim that may be made by its manufacturer, is not guaranteed or endorsed by the publisher.
The authors acknowledge the support of Minciencias and the Fund for Science, Technology and innovation Francisco José de Caldas to CIPAV (Contract 80740-006-2020).
Abalos, D., De Deyn, G. B., Kuyper, T. W., and van Groenigen, J. W. (2014). Plant species identity surpasses species richness as a key driver of N2O emissions from grassland. Glob. Change Biol. 20, 265–275. doi: 10.1111/gcb.12350
Adhikari, K. P., Chibuike, G., Saggar, S., Simon, P. L., Luo, J., and de Klein, C. A. M. (2021). Management and implications of using nitrification inhibitors to reduce nitrous oxide emissions from urine patches on grazed pasture soils—a review. Sci. Total Environ. 791:148099. doi: 10.1016/j.scitotenv.2021.148099
Aguirre-Villegas, H. A., and Larson, R. A. (2017). Evaluating greenhouse gas emissions from dairy manure management practices using survey data and lifecycle tools. J. Clean. Prod. 143, 169–179. doi: 10.1016/j.jclepro.2016.12.133
Andrews, M., Scholefield, D., Abberton, M. T., McKenzie, B. A., Hodge, S., and Raven, J. A. (2007). Use of white clover as an alternative to nitrogen fertiliser for dairy pastures in nitrate vulnerable zones in the UK: productivity, environmental impact and economic considerations. Ann. Appl. Biol. 151, 11–23. doi: 10.1111/j.1744-7348.2007.00137.x
Andueza, D., Picard, F., Dozias, D., and Aufrère, J. (2017). Fecal near-infrared reflectance spectroscopy prediction of the feed value of temperate forages for ruminants and some parameters of the chemical composition of feces: efficiency of four calibration strategies. Appl. Spectrosc. 71, 2164–2176. doi: 10.1177/0003702817712740
Aryal, D. R., Gómez-González, R. R., Hernández-Nuriasmú, R., and Morales-Ruíz, R. (2018). Carbon stocks and tree diversity in scattered tree silvopastoral systems in Chiapas, Mexico. Agroforest Syst. 93, 213–227. doi: 10.1007/s10457-018-0310-y
Barahona, R., Sánchez, S., Lascano, C. E., Owen, E., Morris, P., and Theodorou, M. K. (2006). Effect of condensed tannins from tropical legumes on the activity of fibrolytic enzymes from the rumen fungus Neocallimastyx hurleyensis. Enzyme Microb. Tech. 39, 281–288. doi: 10.1016/j.enzmictec.2005.10.011
Barneze, A. S., Minet, E. P., Cerri, C. C., and Misselbrook, T. (2015). The effect of nitrification inhibitors on nitrous oxide emissions from cattle urine depositions to grassland under summer conditions in the UK. Chemosphere 119, 122–129. doi: 10.1016/j.chemosphere.2014.06.002
Barton, L., Gleeson, D. B., Maccarone, L. D., Zuniga, L. P., and Murphy, D. V. (2013). Is liming soil a strategy for mitigating nitrous oxide emissions from semi-arid soils? Soil Biol. Biochem. 62, 28–35. doi: 10.1016/j.soilbio.2013.02.014
Beeckman, F., Motte, H., and Beeckman, T. (2018). Nitrification in agricultural soils: impacts, actors and mitigation. Curr. Opin. Biotech. 50, 166–173. doi: 10.1016/j.copbio.2018.01.014
Beukes, P. C., Gregorini, P., Romera, A. J., Levy, G., and Waghorn, G. C. (2010). Improving production efficiency as a strategy to mitigate greenhouse gas emissions on pastoral dairy farms in New Zealand. Agric. Ecosyst. Environ. 136, 358–365. doi: 10.1016/j.agee.2009.08.008
Boeckx, P., and Van Cleemput, O. (2001). Estimates of N2O and CH4 fluxes from agricultural lands in various regions in Europe. Nutr. Cycling Agroecosyst. 60, 35–47. doi: 10.1023/A:1012604032377
Bol, R., Petersen, S., Christofides, C., Dittert, K., and Hansen, M. N. (2004). Short-term N2O, CO2, NH3 fluxes, and N/C transfers in a Danish grass-clover pasture after simulated urine deposition in autumn. J. Soil Sci. Plant Nutr. 167, 568–576. doi: 10.1002/jpln.200321334
Breuillin-Sessoms, F., Venterea, R. T., Sadowsky, M. J., Coulter, J. A., Clough, T. J., and Wang, P. (2017). Nitrification gene ratio and free ammonia explain nitrite and nitrous oxide production in urea-amended soils. Soil Biol. Biochem. 111, 143–153. doi: 10.1016/j.soilbio.2017.04.007
Byrnes, R. C., Núñez, J., Arenas, L., Rao, I., Trujillo, C., Alvarez, C., et al. (2017). Biological nitrification inhibition by Brachiaria grasses mitigates soil nitrous oxide emissions from bovine urine patches. Soil Biol. Biochem. 107, 156–163. doi: 10.1016/j.soilbio.2016.12.029
Cai, Y., and Akiyama, H. (2016). Nitrogen loss factors of nitrogen trace gas emissions and leaching from excreta patches in grassland ecosystems: a summary of available data. Sci. Total Environ. 572, 185–195. doi: 10.1016/j.scitotenv.2016.07.222
Cai, Y. J., Chang, S. X., and Cheng, Y. (2017). Greenhouse gas emissions from excreta patches of grazing animals and their mitigation strategies. Earth-Sci. Rev. 171, 44–57. doi: 10.1016/j.earscirev.2017.05.013
Carulla, J. E., Kreuzer, M., Machmüller, A., and Hess, H. D. (2005). Supplementation of Acacia mearnsii tannins decreases methanogenesis and urinary nitrogen in forage-fed sheep. Aust. J. Agric. Res. 56, 961–970. doi: 10.1071/AR05022
Cayuela, M. L., van Zwieten, L., Singh, B. P., Jeffery, S., Roig, A., and Sanchez-Monedero, M. A. (2014). Biochar's role in mitigating soil nitrous oxide emissions, a review and metaanalysis. Agric. Ecosyst. Environ. 191, 5–16. doi: 10.1016/j.agee.2013.10.009
Chadwick, D. (2005). Emissions of ammonia, nitrous oxide and methane from cattle manure heaps: effect of compaction and covering. Atmos. Environ. 39, 787–799. doi: 10.1016/j.atmosenv.2004.10.012
Chadwick, D., Sommer, S., Thorman, R., Fangueiro, D., Cardenas, L., Amon, B., et al. (2011). Manure management: implications for greenhouse gas emissions. Anim. Feed Sci. Technol. 166, 514–531. doi: 10.1016/j.anifeedsci.2011.04.036
Chadwick, D. R., Cardenas, L. M., Dhanoa, M. S., Donovan, N., Misselbrook, T., Williams, J. R., et al. (2018). The contribution of cattle urine and dung to nitrous oxide emissions: quantification of country specific emission factors and implications for national inventories. Sci. Total Environ. 635, 607–617. doi: 10.1016/j.scitotenv.2018.04.152
Chapuis-lardy, L., Wrage, N., Metay, A., Chotte, J. L., and Bernoux, M. (2007). Soils, a sink for N2O? a review. Glob. Change Biol. 13, 1–17. doi: 10.1111/j.1365-2486.2006.01280.x
Chen, D., Li, Y., Grace, P., and Mosier, A. R. (2008). N2O emissions from agricultural lands: a synthesis of simulation approaches. Plant Soil 309, 169–189. doi: 10.1007/s11104-008-9634-0
Chirinda, N., Loaiza, S., Arenas, L., Ruiz, V., Faverín, C., Alvarez, C., et al. (2019). Adequate vegetative cover decreases nitrous oxide emissions from cattle urine deposited in grazed pastures under rainy season conditions. Sci. Rep. 9:908. doi: 10.1038/s41598-018-37453-2
Clough, T. J., Rochette, P., Thomas, S. M., Pihlatie, M., Christiansen, J. R., and Thorman, R. (2020). Global Research Alliance N2O chamber methodology guidelines: Design considerations. J. Environ. Qual. 49, 1081–1091. doi: 10.1002/jeq2.20117
Colmenero, J. J., and Broderick, G. A. (2006). Effect of dietary crude protein concentration on milk production and nitrogen utilization in lactating dairy cows. Int. J. Dairy Sci. 89, 1704–1712. doi: 10.3168/jds.S0022-0302(06)72238-X
Conrad, K., Dalal, R. C., Fujinuma, R., and Menzies, N. W. (2018). Soil organic carbon and nitrogen sequestration and turnover in aggregates under subtropical Leucaena-grass pastures. Soil Res. 56, 632–647. doi: 10.1071/SR18016
Conrad, R. (1996). Soil microorganisms as controllers of atmospheric trace gases (H2, CO, CH4, OCS, N2O, and NO). Clin. Microbiol. Rev. 60, 609–640. doi: 10.1128/MMBR.60.4.609-640.1996
Correa, H. J., Rodríguez, Y. G., Pabón, Y. G., and Carulla, J. E. (2012). Efecto de la oferta de pasto kikuyo (Pennisetum clandestinum) sobre la producción, la calidad de la leche y el balance de nitrógeno en vacas Holstein. Livestock Research for Rural Development. Available online at: http://www.lrrd.org/lrrd24/11/corr24204.htm. (accessed January 11, 2021].
Cubillos, A. M., Vallejo, V. E., Arbeli, Z., Terán, W., Dick, R. P., Molina, C. H., et al. (2016). Effect of the conversion of conventional pasture to intensive silvopastoral systems on edaphic bacterial and ammonia oxidizer communities in Colombia. Eur. J. Soil Biol. 72, 42–50. doi: 10.1016/j.ejsobi.2015.12.003
Dalal, R. C., Wang, W., Robertson, G. P., and Parton, W. J. (2003). Nitrous oxide emission from Australian agricultural lands and mitigation options: a review. Aust. J. Soil Res. 41, 165–195. doi: 10.1071/SR02064
Dangal, S. R. S., Tian, H., Xu, R., Chang, J., Canadell, J. G., Ciais, P., et al. (2019). Global nitrous oxide emissions from pasturelands and rangelands: magnitude, spatiotemporal patterns, and attribution. Glob. Biogeochem Cy. 33, 200–222. doi: 10.1029/2018GB006091
de Klein, C. A. M., and Eckard, R. J. (2008). Targeted technologies for nitrous oxide abatement from animal agriculture. Aust. J. Exp. Agric. 48,14–20. doi: 10.1071/EA07217
de Klein, C. A. M., and Monaghan, R. M. (2011). The effect of farm and catchment management on nitrogen transformations and N2O losses from pastoral systems—can we offset the effects of future intensification? Curr. Opin. Environ. Sustain. 3, 396–406. doi: 10.1016/j.cosust.2011.08.002
de Klein, C. A. M., van der Weerden, T. J., Luo, J., Cameron, K. C., and Di, H. J. (2019). A review of plant options for mitigating nitrous oxide emissions from pasture-based systems. J. Agric. Res. 63, 29–43. doi: 10.1080/00288233.2019.1614073
Dennis, S. J., Moir, J. L., Cameron, K. C., Edwards, G. R., and Di, H. J. (2013). Measuring excreta patch distribution in grazed pasture through low-cost image analysis. Grass Forage Sci. 68, 378–385. doi: 10.1111/gfs.12000
des Roseaux, M. D., Shi, S., Duff, A. M., Brennan, F. P., Condron, L., Finn, J. A., et al. (2020). Impacts of pasture species and ruminant urine on N2O emissions and nitrogen transforming microbial communities in soil mesocosms. New Zealand J. Agric. Res. 18:880. doi: 10.1080/00288233.2020.1848880
Di, H. J., and Cameron, K. C. (2012). How does the application of different nitrification inhibitors affect nitrous oxide emissions and nitrate leaching from cow urine in grazed pastures? Soil Use Manage. 28, 54–61. doi: 10.1111/j.1475-2743.2011.00373.x
Dobbie, K. E., McTaggart, I. P., and Smith, K. A. (1999). Nitrous oxide emissions from intensive agricultural systems: variations between crops and seasons, key driving variables, and mean emission factors. J. Geophys. Res. Atmos. 104, 26891–26899. doi: 10.1029/1999JD900378
Elmerich, C., and Newton, W. E. (2007). Associative and Endophytic Nitrogen-fixing Bacteria and Cyanobacterial Associations, Heidelberg: Springers.
Erickson, G., and Klopfenstein, T. (2010). Nutritional and management methods to decrease nitrogen losses from beef feedlots. J. Anim. Sci. 88, E172–E180. doi: 10.2527/jas.2009-2358
Espinosa-Carvajal, M., Contreras-Santos, J. L., Cadena-Torres, J., Martínez-Atencia, J., Jaramillo-Barrios, C. I., and Hurtado, M. (2020). Flujos de metano en suelos con coberturas de pastos en el norte de Colombia. Agronomía Mesoamericana 31, 291–309. doi: 10.15517/am.v31i2.38387
Firestone, M. K., and Davidson, E. A. (1989). “Microbiological basis of NO and N2O production and consumption in soil,” in Exchange of Trace Gases between Terrestrial Ecosystems and the Atmosphere, eds M. O. Andreae and D. S. Schmimmel (Chichester: John Wiley and Sons Ltd), 7–21.
Fisk, L. M., Barton, L., Jones, D. L., Glanville, H. C., and Murphy, D. V. (2015). Root exudate carbon mitigates nitrogen loss in a semi-arid soil. Soil Biol. Biochem. 88, 380–389. doi: 10.1016/j.soilbio.2015.06.011
Gerber, P. J., Steinfeld, H., Henderson, B., Mottet, A., Opio, C., Dijkman, J., et al (2013). Hacer frente al cambio climático a través de la ganadería—Evaluación global de las emisiones y las oportunidades de mitigación. FAO: Roma. http://www.fao.org/3/i3437s/i3437s.pdf. (accessed January 11, 2021).
Giraldo, C., Escobar, F., Char,á, J., and Calle, Z. (2011). The adoption of silvopastoral systems promotes the recovery of ecological processes regulated by dung beetles in the Colombian Andes. Insect. Conserv. Diver. 4, 115–122. doi: 10.1111/j.1752-4598.2010.00112.x
Hallin, S., Philippot, L., Löffler, F. E., Sanford, R. A., and Jones, C. M. (2018). Genomics and ecology of novel N2O-reducing microorganisms. Trends. Microbiol. 26, 43–55. doi: 10.1016/j.tim.2017.07.003
Hatch, D., Trindade, H., Cardenas, L., Carneiro, J., Hawkins, J., Scholefield, D., et al. (2005). Laboratory study of the effects of two nitrification inhibitors on greenhouse gas emissions from a slurry-treated arable soil, impact of diurnal temperature cycle. Biol. Fertil. Soils 41, 225–232. doi: 10.1007/s00374-005-0836-9
Henry, S., Texier, S., and Hallet, S. (2008). Disentangling the rhizosphere effect on nitrate reducers and denitrifiers: insight into the role of root exudates. Environ. Microbiol. 10, 3082–3092. doi: 10.1111/j.1462-2920.2008.01599.x
Hristov, A. N., Domitrovich, C., Wachter, A., Cassidy, T., Lee, C., Shingfield, K. J., et al. (2011). Effect of replacing solvent-extracted canola meal with high-oil traditional canola, high-oleic acid canola, or high-erucic acid rapeseed meals on rumen fermentation, digestibility, milk production, and milk fatty acid composition in lactating dairy cows. Int. J. Dairy Sci. 94, 4057–4074. doi: 10.3168/jds.2011-4283
Hristov, A. N., Oh, J., Lee, C., Meinen, R., Montes, F., Ott, T., et al. (2013). “Mitigation of greenhouse gas emissions in livestock production—a review of technical options for non-CO2 emissions,” in FAO Animal Production and Health paper no. 177, eds P. Gerber, B. Henderson, and H. Makkar (Rome: FAO). Available online at: http://www.fao.org/3/i3288e/i3288e.pdf. (Accessed January 11, 2021).
IPCC (Intergovernmental Panel on Climate Change) (2006). IPCC Guideline for National Greenhouse Inventories. Intergovernmental Panel on Climate Change IPCC. France, Paris (IPCC/OECD/IEA). Available online at: https://www.ipcc-nggip.iges.or.jp/public/2006gl/index.html. (accessed January 11, 2021).
IPCC (Intergovernmental Panel on Climate Change) (2013). “Summary for Policymakers,” in Climate Change 2013: The Physical Science Basis. Contribution of Working Group I to the Fifth Assessment Report of the Intergovernmental Panel on Climate Change, eds T.F., Stocker et al., (New York, NY: Cambridge University Press). Available online at:https://www.ipcc.ch/site/assets/uploads/2018/03/WG1AR5_SummaryVolume_FINAL.pdf. (accessed January 11, 2021).
IPCC (Intergovernmental Panel on Climate Change) (2014). “AR5. Climate Change 2014: Mitigation,” in Contribution of Working Group III to the Fifth Assessment Report of the Intergovernmental Panel on Climate Change eds O., Edenhofer et al. (New York, NY: Cambridge University Press). Available online at: https://www.ipcc.ch/report/ar5/wg3/ (accessed January 11, 2021).
IPCC (Intergovernmental Panel on Climate Change). (2019). “Volume 4. Agriculture, Forestry and Other Land Use National Greenhouse Gas Inventories Programme,” in Guidelines for National Greenhouse Gas Inventories 2019 Refinement to the 2006, eds D. Blain., F. Agus., M. A. Alfaro., and H. Vreuls (IGES) Available online at: https://www.ipcc-nggip.iges.or.jp/public/2019rf/vol4.html. (accessed January 11, 2021).
Jaimes, L., and Correa, H. J. (2016). Balance de nitrógeno, fósforo y potasio en vacas Holstein pastando praderas de kikuyo (Cenchrus clandestinus) en el norte de Antioquia. Rev. CES Med. Zootec. 11, 18–41. doi: 10.21615/cesmvz.11.2.2
Jarvis, S. C., Scholefield, D., and Pain, B. F. (1995). “Nitrogen cycling in grazing systems,” in Nitrogen Fertilization in the Environment, ed Bacon, P. (New York, NY: Dekker Inc).
Kerdchoechuen, O. (2005). Methane emission in four rice varieties as related to sugars and organic acids of roots and root exudates and biomass yield. Agric. Ecosyst. Environ. 108, 155–163. doi: 10.1016/j.agee.2005.01.004
Khan, R. Z., Muller, C., and Sommer, S. G. (1997). Micrometeorological mass balance technique for measuring CH4 emission from stored cattle slurry. Biol. Fertil. Soils 24, 442–444. doi: 10.1007/s003740050270
Khan, S., Clough, T. J., Goh, K. M., and Sherlock, R. R. (2011). Influence of soil pH on NOx and N2O emissions from bovine urine applied to soil columns. N. Z. J. Agric. Res. 54, 285–301. doi: 10.1080/00288233.2011.607831
Kim, D. G., and Giltrap, D. (2017). Determining optimum nitrogen input rate and optimum yield-scaled nitrous oxide emissions: Theory, field observations, usage, and limitations. Agric. Ecosyst. Environ. 247, 371–378. doi: 10.1016/j.agee.2017.07.003
Klausner, S. D., Fox, D. G., Rasmussen, C. N., Pitt, R. E., Tylutki, T. P., Wright, P. E., et al. (1998). Improving dairy farm sustainability I: An approach to animal and crop nutrient management planning. J. Prod. Agric. 11, 225–233. doi: 10.2134/jpa1998.0225
Klein Goldewijk, K., Beusen, A., Doelman, J., and Stehfest, E. (2017). Anthropogenic land use estimates for the Holocene; HYDE 3.2. Earth Syst. Sci. Data. 9, 927–953. doi: 10.5194/essd-9-927-2017
Kreuzer, M., and Hindrichsen, I. K. (2006). Methane mitigation in ruminants by dietary means: the role of their methane emission from manure. Int. Congr. 1293, 199–208. doi: 10.1016/j.ics.2006.01.015
Krol, D. J., Carolan, R., Minet, E., McGeough, K. L., Watson, C. J., Forrestal, P. J., et al. (2016). Improving and disaggregating N2O emission factors for ruminant excreta on temperate pasture soils. Sci. Total Environ. 568, 327–338. doi: 10.1016/j.scitotenv.2016.06.016
Kunhikrishnan, A., Thangarajan, R., Bolan, N. S., Xu, Y., Mandal, S., Gleeson, D. B., et al. (2016). Functional relationships of soil acidification, liming, and greenhouse gas flux. Adv. Agron. 139, 1–71. doi: 10.1016/bs.agron.2016.05.001
Kuypers, M. M. M., Marchant, H. K., and Kartal, B. (2018). The microbial nitrogen-cycling network. Nat. Rev. Microbiol. 16, 263–276. doi: 10.1038/nrmicro.2018.9
Le Mer, J., and Roger, P. (2001). Production, oxidation, emission and consumption of methane by soils: a review. Eur. J. Soil Biol. 37, 25–50. doi: 10.1016/S1164-5563(01)01067-6
Ledgard, S., Schils, R., Eriksen, J., and Luo, J. (2009). Environmental impacts of grazed clover/grass pastures. Ir. J. Agric. Food Res. 48, 209–226. doi: 10.jstor.org/stable/20720369
Lee, C., Hristov, A. N., Cassidy, T. W., and Heyler, K. (2012). Nitrogen isotope fractionation and origin of ammonia nitrogen volatilized from cattle manure in simulated storage. Atmosphere 2, 256–270. doi: 10.3390/atmos2030256
Li, D., Watson, C. J., Yan, M. J., Lalor, S., Rafique, R., Hyde, B., et al. (2013). A review of nitrous oxide mitigation by farm nitrogen management in temperate grassland-based agriculture. J. Environ. Manage 128, 893–903. doi: 10.1016/j.jenvman.2013.06.026
Lombardi, B., Alvarado, P. I., Ricci, P., Guzmán, S. A., Gonda, H. L., and Juliarena, M. P. (2021). Methane and nitrous oxide emissions from dung patches deposited by grazing cattle supplemented with maize grain. Anim. Feed Sci. Technol. 279:115029. doi: 10.1016/j.anifeedsci.2021.115029
López-Aizpún, M., Horrocks, C. A., Charteris, A. F., Marsden, K. A., Ciganda, V. S., Evans, J. R., et al. (2020). Meta-analysis of global livestock urine-derived nitrous oxide emissions from agricultural soils. Glob. Change Biol. 26, 2002–2013. doi: 10.1111/gcb.15012
Lorenz, K., and Lal, R. (2018). “Carbon sequestration in grassland soils”. in Carbon Sequestration in Agricultural Ecosystems, ed K. Lorenz and R. Lal (Switzerland: Springer), 175–209.
Luo, J., Balvert, S. F., Wise, B., Welten, B., Ledgard, S. F., de Klein, C. A. M., et al. (2018). Using alternative forage species to reduce emissions of the greenhouse gas nitrous oxide from cattle urine deposited onto soil. Sci. Total Environ. 18, 1271–1280. doi: 10.1016/j.scitotenv.2017.08.186
Luo, J., de Klein, C. A. M., Ledgard, S. F., and Saggar, S. (2010). Management options to reduce nitrous oxide emissions from intensively grazed pastures: a review. Agric. Ecosyst. Environ. 136, 282–282. doi: 10.1016/j.agee.2009.12.003
Luo, J., Lindsey, S. B., and Ledgard, S. F. (2008). Nitrous oxide emissions from animal urine application on a New Zealand pasture. Biol. Fertil. Soils. 44, 463–470. doi: 10.1007/s00374-007-0228-4
Luo, J., Wyatt, J., van der Weerden, T. J., Thomas, S. M., de Klein, C. A. M., Li, Y., et al. (2017). Potential hotspot areas of nitrous oxide emissions from grazed pastoral dairy farm systems. Adv. Agron. 145, 205–268. doi: 10.1016/bs.agron.2017.05.006
Lynch, J., Cain, M., Frame, D., and Pierrehumbert, R. (2021). Agriculture's contribution to climate change and role in mitigation is distinct from predominantly fossil CO2-Emitting Sectors. Front. Sustain. Food Syst. 4:518039. doi: 10.3389/fsufs.2020.518039
Machefert, S. E., Dise, N. B., Goulding, K. W. T., and Whitehead, P. G. (2002). Nitrous oxide emission from a range of land uses across Europe. Hydrol. Earth Syst. Sci. Discuss. 6, 325–337. hal-00304665. doi: 10.5194/hess-6-325-2002
Maire, J., Gibson-Poole, S., Cowan, N., Reay, D. S., Richards, K. G., Skiba, U., et al. (2018). Identifying urine patches on intensively managed grassland using aerial imagery captured from remotely piloted aircraft systems. Front. Sustain. Food Syst. 2:10. doi: 10.3389/fsufs.2018.00010
Malyan, S. K., Bhatia, A., Kumar, A., Gupta, D. K., Singh, R., Kumar, S. S., et al. (2016). Methane production, oxidation and mitigation: a mechanistic understanding and comprehensive evaluation of influencing factors. Sci. Total Environ. 572, 874–896. doi: 10.1016/j.scitotenv.2016.07.182
Manzano, P., and White, S. (2019). Intensifying pastoralism may not reduce greenhouse gas emissions: wildlife-dominated landscape scenarios as a baseline in life-cycle analysis. Clim. Res. 7, 91–97. doi: 10.3354/cr01555
Marsden, K. A., Jones, D. L., and Chadwick, D. R. (2017). DMPP is ineffective at mitigating N2O emissions from sheep urine patches in a UK grassland under summer conditions. Agric. Ecosyst. Environ. 246, 1–11. doi: 10.1016/j.agee.2017.05.017
McMillan, A. M. S., Pal, P., Phillips, R. L., Palmada, T., Berben, P. H., Jha, N., et al. (2016). Can pH amendments in grazed pastures help reduce N2O emissions from denitrification? the effects of liming and urine addition on the completion of denitrification in fluvial and volcanic soils. Soil Biol. Biochem. 93, 90–104. doi: 10.1016/j.soilbio.2015.10.013
Meng, Q., Sun, Y., Zhao, J., Zhou, L., Ma, X., Zhou, M., et al. (2014). Distribution of carbon and nitrogen in waterstable aggregates and soil stability under long-term manure application in solonetzic soils of the Songnen plain, northeast China. J. Soils Sediments. 14, 1041–1049. doi: 10.1007/s11368-014-0859-7
Misselbrook, T., Fleming, H., Camp, V., Umstatter, C., Duthie, C. A., Nicoll, L., et al. (2016). Automated monitoring of urination events from grazing cattle. Agric. Ecosyst. Environ. 230, 191–198. doi: 10.1016/j.agee.2016.06.006
Misselbrook, T. H., Powell, J. M., Broderick, G. A., and Grabber, J. H. (2005). Dietary manipulation in dairy cattle: laboratory experiments to assess the influence on ammonia emissions. Int. J. Dairy Sci. 88, 1765–1777. doi: 10.3168/jds.S0022-0302(05)72851-4
Misselbrook, T. H., Webb, J., Chadwick, D. R., Ellis, S., and Pain, B. F. (2001). Gaseous emissions from outdoor concrete yards used by livestock. Atmos. Environ. 35, 5331–5338. doi: 10.1016/S1352-2310(01)00289-8
Montes, F., Meinen, R., Dell, C., Rotz, A., Hristov, A. N., Oh, J., et al. (2013). Special topics-Mitigation of methane and nitrous oxide emissions from animal operations: II. a review of manure management mitigation options. J. Anim. Sci. 91, 5070–5094. doi: 10.2527/jas.2013-6584
Montoya-Molina, S., Giraldo-Echeverri, C., Montoya-Lerma, J., Char,á, J., Escobar, F., and Calle, Z. (2016). Land sharing vs. land sparing in the dry Caribbean lowlands: a dung beetles' perspective. Appl. Soil. Ecol. 98, 204–212. doi: 10.1016/j.apsoil.2015.10.017
Myhre, G., Shindell, D., Bréon, F. M., Collins, W., Fuglestvedt, J., Huang, J., et al. (2013). “Anthropogenic and natural radiative forcing,” in Climate Change 2013: The Physical Science Basis. Contribution of Working Group I to the Fifth Assessment Report of the Intergovernmental Panel on Climate Change, ed T.F. Stocker et al. (Cambridge: Cambridge University Press), 659–740.
Myrold, D. D. (2005). “Transformations of Nitrogen,” in Principles and applications of soil microbiology. ed D. M. Sylvia et al. (Pearson Education LTD, Singapore, Canada, Japan, Australia, North Asia, Mexico, Malaysia), 333–372.
Nguyen, Q. V., Wu, D., Kong, X., Bol, R., Petersen, S. O., Jensen, L. S., et al. (2017). Effects of cattle slurry and nitrification inhibitor application on spatial soil O2 dynamics and N2O production pathways. Soil Biol. Biochem. 114, 200–209. doi: 10.1016/j.soilbio.2017.07.012
Niklaus, P. A., Le Roux, X., Poly, F., Buchmann, N., Scherer-Lorenzen, M., Weigelt, A., et al. (2016). Plant species diversity affects soil–atmosphere fluxes of methane and nitrous oxide. Oecologia 181, 919–930. doi: 10.1007/s00442-016-3611-8
Novak, S. M., and Fiorelli, J. L. (2010). Greenhouse gases and ammonia emissions from organic mixed crop-dairy systems: a critical review of mitigation options. Agron. Sustain. Dev. 30, 215–236. doi: 10.1051/agro/2009031
Oenema, O., Oudendag, D., and Velthof, G. L. (2007). Nutrient losses from manure management in the European Union. Livest. Sci. 112, 261–272. doi: 10.1016/j.livsci.2007.09.007
Oenema, O., Velthof, G. L., Yamulki, S., and Jarvis, S. C. (1997). Nitrous oxide emissions from grazed grassland. Soil Use Manage. 13, 288–295. doi: 10.1111/j.1475-2743.1997.tb00600.x
Oertel, C., Matschullat, J., Zurba, K., Zimmermann, K., and Erasmi, S. (2016). Greenhouse gas emissions from soils—a review. Geochem 76, 327–352. doi: 10.1016/j.chemer.2016.04.002
Opio, C., Gerber, P., Mottet, A., Falcucci, A., Tempio, G., MacLeod, M., et al. (2013). Greenhouse gas emissions from ruminant supply chains—A global life cycle assessment. Food and Agriculture Organization of the United Nations (FAO), Rome: FAO.
Pastrana, I., Reza, S., Espinosa, M., Suárez, E., and Díaz, E. (2011). Efecto de la fertilización nitrogenada en la dinámica del óxido nitroso y metano en Brachiaria humidicola (Rendle) Schweickerdt. Cienc. Tecnol. Agropecuaria. 12, 134–142. doi: 10.21930/rcta.vol12_num2_art:223
Philippot, L., Hallin, S., Börjesson, G., and Baggs, E. M. (2009). Biochemical cycling in the rhizosphere having an impact on global change. Plant Soil 321, 1-2, 61–81. doi: 10.1007/s11104-008-9796-9
Powell, J. M., Aguerre, M. J., and Wattiaux, M. A. (2011). Dietary crude protein and tannin impact dairy manure chemistry and ammonia emissions from incubated soils. J. Environ. Qual. 40, 1767–1774. doi: 10.2134/jeq2011.0085
Radrizzani, A., Shelton, H. M., Dalzell, S. A., and Kirchhof, G. (2011). Soil organic carbon and total nitrogen under Leucaena leucocephala pastures in Queensland. Crop Pasture Sci. 62, 337–345. doi: 10.1071/CP10115
Rivera, J., Char,á, J., Arango, J., and Barahona, R. (2021). Effect of different genotypes of Tithonia diversifolia on fermentation of feed mixtures with Urochloa brizantha cv. Marandú. Crop Past. Sci. 21:1102. doi: 10.1071/CP21102
Rivera, J., Char,á, J., and Barahona, R. (2016). Análisis de ciclo de vida para la producción de leche bovina en un sistema silvopastoril intensivo y un sistema convencional en Colombia. Trop. Subtrop. Agroecosystems. 19, 237–251. doi: 10.1007/redalyc.org/articulo.oa?id=93949148007.
Rivera, J. E., Char,á, J., and Barahona, R. (2018). CH4, CO2 and N2O emissions from grasslands and bovine excreta in two intensive tropical dairy production systems. Agrofor. Syst. 93, 915–928 doi: 10.1007/s10457-018-0187-9
Robinson, A., Di, H. J., Cameron, K. C., Podolyan, A., and He, J. Z. (2014). The effect of soil pH and dicyandiamide (DCD) on N2O emissions and ammonia oxidizer abundance in a stimulated grazed pasture soil. J. Soils Sedim. 14, 1434–1444. doi: 10.1007/s11368-014-0888-2
Roesch, A., Weisskopf, P., Oberholzer, H., Valsangiacomo, A., and Nemecek, T. (2019). An approach for describing the effects of grazing on soil quality in Life-Cycle assessment. Sustainability 11, 4870. doi: 10.3390/su11184870
Ruzjerez, B. E., White, R. E., and Ball, P. R. (1994). Long-term measurement of denitrification in three contrasting pastures grazed by sheep. Soil Biol Biochem 26, 29–39. doi: 10.1016/0038-0717(94)90192-9
Saari, A., Rinnan, R., and Martikainen, P. J. (2004). Methane oxidation in boreal forest soils: kinetics and sensitivity to pH and ammonium. Soil Biol. Biochem. 36, 1037–1046. doi: 10.1016/j.soilbio.2004.01.018
Saggar, S., Andrew, R. M., Tate, K. R., Hedley, C. B., Rodda, N. J., and Townsend, J. A. (2004). Modelling nitrous oxide emissions from New Zealand dairy grazed pastures. Nutr. Cycling Agroecosyst. 68, 243–255. doi: 10.1023/B:FRES.0000019463.92440.a3
Samer, M. (2015). “GHG Emission from Livestock Manure and Its Mitigation Strategies,” in Climate Change Impact on Livestock: Adaptation and Mitigation, ed V. Sejian, J. Gaughan, L. Baumgard and C. Prasad. (New York, NY: Springer), 321–346. doi: 10.1007/978-81-322-2265-1_20
Sarabia-Salgado, L., Solorio-Sánchez, F., Ramírez-Avilés, L., Rodrigues, B. J., Ku-Vera, J., Aguilar-Pérez, C., et al. (2020). Increase in Milk Yield from Cows through Improvement of Forage Production Using the N2-Fixing Legume Leucaena leucocephala in a Silvopastoral System. Animals 10:734. doi: 10.3390/ani10040734
Sass, R. L., Fisher, F. M., Harcombe, P. A., and Turner, F. T. (1991). Mitigation of methane emission from rice fields: Possible adverse effects of incorporated rice straw. Global Biogeochem Cy 5, 275–287. doi: 10.1029/91GB01304
Schils, R. L. M., van Groenigen, J. W., Velthof, G. L., and Kuikman, P. J. (2008). Nitrous oxide emissions from multiple combined applications of fertiliser and cattle slurry to grassland. Plant Soil 310, 89–101. doi: 10.1007/s11104-008-9632-2
Schmeer, M., Loges, R., Dittert, R., Senbayram, M., Horn, R., and Taube, F. (2014). Legume-based forage production systems reduce nitrous oxide emissions. Soil Tillage Res. 143, 17–25. doi: 10.1016/j.still.2014.05.001
Selbie, D. R., Buckthought, L. E., and Shepherd, M. A. (2015). Chapter four—the challenge of the urine patch for managing nitrogen in grazed pasture systems. Adv. Agron 129, 229–292. doi: 10.1016/bs.agron.2014.09.004
Seré, C., and Steinfeld, H. (1995). World livestock production systems: current status, issues and trends. FAO Animal Production and Health Paper 127. Rome: FAO. Available online at: http://www.fao.org/3/w0027e/w0027e.pdf. (accessed July 22, 2021).
Sherlock, R. R., de Klein, C. A. M., and L,i, Z. (2003). Determination of the N2O and CH4 emission factor from animal excreta, following a summer application in 3 regions of New Zealand. Report for MAF Policy. Available online at: https://www.mpi.govt.nz/document-vault/2950. (accessed January 11, 2021).
Shi, B., Xu, W., Zhu, Y. G., Wang, C., Loik, M. E., and Sun, W. (2019). Heterogeneity of grassland soil respiration: antagonistic effects of grazing and nitrogen addition. Agric. For. Meteorol. 268, 215–223. doi: 10.1016/j.agrformet.2019.01.028
Shukla, P. N., Pandey, K. D., and Mishra, V. K. (2013). Environmental determinants of soil methane oxidation and methanotrophs. Crit. Rev. Environ. Sci. Technol. 43, 1945–2011. doi: 10.1080/10643389.2012.672053
Singurindy, O., Molodovskaya, M., Richards, B. K., and Steenhuis, T. S. (2009). Nitrous oxide emission at low temperatures from manure-amended soils under corn (Zea mays L.). Agric. Ecosyst. Environ. 132, 74–81. doi: 10.1016/j.agee.2009.03.001
Slade, E., Riutta, T., Roslin, T., and Tuosismo, H. (2016). The role of dung beetles in reducing greenhouse gas emissions from cattle farming. Sci. Rep. 6:18140. doi: 10.1038/srep18140
Sneath, R. W., Beline, F., Hilhorst, M. A., and Peu, P. (2006). Monitoring GHG from manure stores on organic and conventional dairy farms. Agric. Ecosyst. Environ. 112, 122–128. doi: 10.1016/j.agee.2005.08.020
Sordi, A., Dieckow, J., Bayer, C., Albuquerque, M. A., Piva, J. T., Zanatta, J. A., et al. (2014). Nitrous oxide emission factors for urine anddung patches in a subtropical Brazilian pastureland. Agric. Ecosyst. Environ. 90, 94–103. doi: 10.1016/j.agee.2013.09.004
Steinfeld, H., and Wassenaar, T. (2007). The role of livestock production on carbon and nitrogen cycles. Annu. Rev. Environ. Resour. 32, 271–294. doi: 10.1146/annurev.energy.32.041806.143508
Subbarao, G., Arango, J., Masahiro, K., Hooper, A. M., Yoshihashi, T., Ando, Y., et al. (2017). Genetic mitigation strategies to tackle agricultural GHG emissions: the case for biological nitrification inhibition technology. Plant Sci. 262, 165–168. doi: 10.1016/j.plantsci.2017.05.004
Subbarao, G., Nakahara, K., Hurtado, M., Ono, H., Moreta, D., Salcedo, A. F., et al. (2009). Evidence for biological nitrification inhibition in Brachiaria pastures. PNAS 106, 17302–17307. doi: 10.1073/pnas.0903694106
Teutscherova, N., Vazquez, E., Arango, J., Arevalo, A., Benito, M., and Pulleman, M. (2019). Native arbuscular mycorrhizal fungi increase the abundance of ammonia-oxidizing bacteria, but suppress nitrous oxide emissions shortly after urea application. Geoderma 338, 493–501. doi: 10.1016/j.geoderma.2018.09.023
Towprayoon, S., Smakgahn, K., and Poonkaew, S. (2005). Mitigation of methane and nitrous oxide emissions from drained irrigated rice fields. Chemosphere 59, 1547–1556. doi: 10.1016/j.chemosphere.2005.02.009
Uchida, Y., Clough, T. J., Kelliher, F. M., Hunt, J. E., and Sherlock, R. R. (2011). Effects of bovine urine, plants and temperature on N2O and CO2 emissions from a sub-tropical soil. Plant Soil 345, 171–186. doi: 10.1007/s11104-011-0769-z
U. S. E. P. A. United States Environmental Protection Agency (2021). Climate Indicators Explorer. Atmospheric Concentrations of Greenhouse Gases. Available online at: https://edap.epa.gov/public/extensions/CCIDataViewer/CCIDataViewer.html#greenhouse-gasesandatm-conc-ghg. (accessed July 7, 2021).
Vallejo, V. E., Averly, Z., Terán, W., Lorenz, N., Dick, R. P., and Roldán, F. (2012). Effect of land management and Prosopis juliflora (Sw.) DC trees on soil microbial community and enzymatic activities in intensive silvopastoral systems in Colombia. Agric. Ecosyst. Environ. 150, 139–148. doi: 10.1016/j.agee.2012.01.022
Vallejo, V. E., Roldán, F., and Dick, R. P. (2010). Soil enzymatic activities and microbial biomass in an integrated agroforestry chronosequence compared to monoculture and a native forest of Colombia. Biol. Fertil. Soils 46, 577–587. doi: 10.1007/s00374-010-0466-8
van der Meer, H. G. (2008). Optimising manure management for GHG outcomes. Aust. J. Exp. Agric. 48, 38–45. doi: 10.1071/EA07214
van der Weerden, T., Beukes, P., de Klein, C. A. M., Hutchinson, K., Farrell, L., Stormink, T., et al. (2018). The effects of system changes in grazed dairy farmlet trials on greenhouse gas emissions. Animals 8:234. doi: 10.3390/ani8120234
van der Weerden, T. J., Noble, A. N., Luo, J., de Klein, C. A. M., Saggar, S., Giltrap, D., et al. (2020). Meta-analysis of New Zealand's nitrous oxide emission factors for ruminant excreta supports disaggregation based on excreta form, livestock type and slope class. Sci. Total Environ. 732:139235. doi: 10.1016/j.scitotenv.2020.139235
van der Weerden, T. J., Sherlock, R. R., Williams, P. H., and Cameron, K. C. (1999). Nitrous oxide emissions and methane oxidation by soil following cultivation of two different leguminous pastures. Biol. Fertil. Soils. 30, 52–60. doi: 10.1007/s003740050587
van Groenigen, J. W., Kuikman, P. J., de Groot, W. J. M., and Velthof, G. L. (2005). Nitrous oxide emissions from urine-treated soil as influenced by urine composition and soil physical conditions. Soil Biol. Biochem. 37, 463–473. doi: 10.1016/j.soilbio.2004.08.009
van Zwieten, L., Kimber, S., Morris, S., Downie, A., Berger, E., Rust, J., et al. (2010). Influence of biochars on flux of N2O and CO2 from ferrosol. Aust. J. Soil Res. 48, 555–568. doi: 10.1071/SR10004
VanderZaag, A. C., Jayasundara, S., and Wagner-Riddle, C. (2011). Strategies to mitigate nitrous oxide emissions from land applied manure. Anim. Feed Sci. Technol. 167, 464–479. doi: 10.1016/j.anifeedsci.2011.04.034
Vergé, X. P. C., Dyer, J. A., Worth, D. E., Smith, W. N., Desjardins, R. L., and McConkey, B. G. (2012). A greenhouse gas and soil carbon model for estimating the carbon footprint of livestock production in Canada. Animals 2, 437–454. doi: 10.3390/ani2030437
Visscher, A., Boeckx, P., and Cleemput, O. (2007). “Artificial methane sinks,” in Greenhouse gas sinks. eds D.S. Reay, C.N. Hewitt, K.A. Smith and J. Grace (Wallingford: CAB International), 184–200.
Wachendorf, C., Lampe, C., Taube, F., and Dittert, K. (2008). Nitrous oxide emissions and dynamics of soil nitrogen under 15N-labeled cow urine and dung patches on a sandy grassland soil. J. Soil Sci. Plant Nutr. 171, 171–180. doi: 10.1002/jpln.200625217
Waschütza, S., Hofmann, N., Niemann, E. G., and Fendrik, I. (1992). Investigations on root exudates of Korean rice. Symbiosis 13, 181–189.
Wecking, A. R. (2021). Paddock Scale Nitrous Oxide Emissions from Intensively Grazed Pasture: Quantification and Mitigation. [dissertation/PhD's thesis]. [Auckland (New Zealand)]: The University of Waikato.
Whitehead, D., Schipper, L. A., Pronger, J., Moinet, G. Y. K., Mudge, P. L., Calvelo Pereira, R., et al. (2018). Management practices to reduce losses or increase soil carbon stocks in temperate grazed grasslands: New Zealand as a case study. Agric. Ecosyst. Environ. 265, 432–443. doi: 10.1016/j.agee.2018.06.022
Woods, R. R., Cameron, K. C., Edwards, G. R., Di, H. J., Clough, T. J., and Nicholson, F. (2016). Effects of forage type and gibberellic acid on nitrate leaching losses. Soil Use Manag. 32, 565–572. doi: 10.1111/sum.12297
Yanan, X., Yang, S.-H., Xu, J., Ding, J., Sun, X., and Jiang, Z. (2018). Effect of biochar amendment on methane emissions from paddy field under water-saving irrigation. Sustainability 10:1371. doi: 10.3390/su10051371
Yang, M., Fang, Y., Sun, D., and Shi, Y. (2016). Efficiency of two nitrification inhibitors (dicyandiamide and 3, 4-dimethypyrazole phosphate) on soil nitrogen transformations and plant productivity: a meta-analysis. Sci. Rep. 6:22075. doi: 10.1038/srep22075
Zaman, M., Nguyen, M. L., Matheson, F., Blennerhassett, J. D., and Quin, B. F. (2007). Can soil amendments (zeolite or lime) shift the balance between nitrous oxide and dinitrogen emissions from pasture and wetland soils receiving urine or urea-N? Aust. J. Soil Res. 45, 543–553. doi: 10.1071/SR07034
Zebarth, B. J., Rochette, P., and Burton, D. L. (2008). N2O emissions from spring barley production as influenced by fertilizer nitrogen rate. Can. J. Soil Sci. 88, 197–120. doi: 10.4141/CJSS06006
Zhu, Y., Merbold, L., Pelster, D., Diaz-Pines, E., Wanyama, G. N., and Butterbach-Bahl, K. (2018). Effect of dung quantity and quality on greenhouse gas fluxes from tropical pastures in Kenya. Glob. Biogeochem. Cycles 32, 1589–1604. doi: 10.1029/2018GB005949
Keywords: global warming, climate change, nutrient excretion, nitrification, mitigation, nitrogen losses
Citation: Rivera JE and Chará J (2021) CH4 and N2O Emissions From Cattle Excreta: A Review of Main Drivers and Mitigation Strategies in Grazing Systems. Front. Sustain. Food Syst. 5:657936. doi: 10.3389/fsufs.2021.657936
Received: 24 January 2021; Accepted: 16 September 2021;
Published: 14 October 2021.
Edited by:
Dietmar Schwarz, Leibniz Institute of Vegetable and Ornamental Crops, GermanyReviewed by:
Marta Andrea Alfaro, Instituto de Investigaciones Agropecuarias, ChileCopyright © 2021 Rivera and Chará. This is an open-access article distributed under the terms of the Creative Commons Attribution License (CC BY). The use, distribution or reproduction in other forums is permitted, provided the original author(s) and the copyright owner(s) are credited and that the original publication in this journal is cited, in accordance with accepted academic practice. No use, distribution or reproduction is permitted which does not comply with these terms.
*Correspondence: Julián Esteban Rivera, amVyaXZlcmFAZnVuLmNpcGF2Lm9yZy5jbw==
Disclaimer: All claims expressed in this article are solely those of the authors and do not necessarily represent those of their affiliated organizations, or those of the publisher, the editors and the reviewers. Any product that may be evaluated in this article or claim that may be made by its manufacturer is not guaranteed or endorsed by the publisher.
Research integrity at Frontiers
Learn more about the work of our research integrity team to safeguard the quality of each article we publish.