- 1Department of Animal, Dairy, and Veterinary Sciences, Utah State University, Logan, UT, United States
- 2Perdue Agribusiness, Salisbury, MD, United States
- 3Department of Animal Sciences, University of São Paulo, Piracicaba, Brazil
The use of grazing systems for milk production is widely used globally because it is a lower-cost feeding system. However, under tropical conditions, the energy content of pastures became is a limitation to improve animal performance and efficiency while reducing the environmental impact. The objective of our study was to evaluate the impact of supplying different dietary sources of energy to lactating dairy cows grazing tropical pastures on the recovery of human-edible (HE) nutrients in milk and the environmental impact. Two experiments were conducted simultaneously. In experiment 1, forty early lactating dairy cows were used in a randomized block design. In experiment 2, four late-lactating rumen-cannulated dairy cows were used in a 4 × 4 Latin Square design. All cows had free access to pasture and treatments were applied individually as a concentrate supplement. Treatments were flint corn grain-processing method either as fine ground (FGC) or steam-flaked (SFC) associated with Ca salts of palm fatty acids supplementation either not supplemented (CON) or supplemented (CSPO). We observed that feeding cows with SFC markedly reduced urinary nitrogen excretion by 43%, and improved milk nitrogen efficiency by 17% when compared with FGC. Additionally, we also observed that feeding supplemental fat improved milk nitrogen efficiency by 17% compared with cows receiving CON diets. A tendency for decreased methane (CH4) per unit of milk (−31%), CH4 per unit of milk energy output (−29%), and CH4 per unit of milk protein output (−31%) was observed when CSPO was fed compared with CON. Additionally, SFC diets increased HE recovery of indispensable amino acids by 7–9% when compared with FGC diets, whereas feeding supplemental fat improved HE recovery of indispensable amino acids by 17–19% compared with CON. Altogether, this study increased our understanding of how manipulating energy sources in the dairy cow diet under tropical grazing conditions can benefit HE nutrient recovery and reduce nutrient excretion.
Introduction
Based on the current food system, the United Nations Food and Agriculture Organization (FAO) estimates that there is a need to produce about 50% more food by 2050 to feed the increasing world population (FAO, 2018). This need to increase agricultural productivity and food security in a sustainable manner poses a huge challenge especially in developing countries (Altieri and Toledo, 2011). The environmental impact from agricultural activities can be reduced significantly by the implementation of practices that decrease emissions of carbon dioxide, methane, and nitrous oxide related to the production of crops and livestock (FAO, 2019). Therefore, improvements in the efficiency of resource-use will need to be achieved globally to meet the growing and changing food demand, while implementing strategies to minimize the environmental impact of food production activities.
Previous analysis suggested that net food production and calories availability could increase by shifting crop production away from activities such as livestock feed (Foley et al., 2011); but, the protein quality is a key concern for many developing nations. Milk is an important source of high quality protein in the human diet. The high nutritional quality of milk proteins originates from both its high level of indispensable amino acids, as well as, its high digestibility (Schaafsma, 2012). To our knowledge, the impact of altering animal nutrition strategies on the efficiency of recovery of human-edible (HE) nutrients in milk from cows grazing tropical pastures have not been determined yet.
Grasslands cover 26% of the world land area and are essential for world food production (FAO, 2010). The use of grazing systems for milk production in the dairy industry is widely used globally because of its lower-cost since forage is the cheapest source of nutrients in the diet (Bargo et al., 2003). Although the effect of nutritional strategies on the efficiency of animal production and environmental impact are relatively well-studied in cows grazing temperate pastures (Roche et al., 2017), much less research is available in cows grazing tropical pastures. Tropical pastures have a higher potential for forage yield accumulation (Santos et al., 2014) and recent rotational grazing strategies based on 95% light interception has considerably improved forage quality and animal performance compared with the traditional grazing approach (fixed days) (Congio et al., 2018). However, this grazing management strategy is not enough to meet the energy requirements of grazing dairy cows (Danes et al., 2013), mainly because tropical pastures have high fiber content and lower particle fragility, which negatively impacts animal feed intake and consequently performance (Moore and Mott, 1973; Allen, 1996). Therefore, the use of feeding systems combining grazing and the addition of high energy concentrates is required. Previous studies from our laboratory demonstrated that extensive processing of flint corn (e.g., steam-flaking) as well as the addition of rumen-protected in the diet of early-lactating dairy cows enhanced milk production and milk protein (Batistel et al., 2017; De Souza et al., 2017). However, the impact of these diets on the environment is not known.
Therefore, the objective of our study was to evaluate the associative impact of feeding dietary energy sources, corn-processing method or Ca salts of palm fatty acids, on the recovery of HE nutrients in milk as well as on nitrogen excretion and methane emission.
Materials and Methods
Two simultaneous experiments were carried out at the Dairy Teaching and Research Center of the University of São Paulo in Piracicaba, SP, Brazil. All procedures involving the animals were approved by the Ethical Committee for Animal Research of the University of São Paulo, Piracicaba.
Experiment 1
Experiment Description
Forty dairy cows (28 Holstein × Gir and 12 Jersey × Holstein) were used. At the beginning of the experiment, the cows had 494 ± 55 kg of body weight, 3.20 ± 0.12 body condition score, and milk yield of 19.2 ± 2.8 kg. Cows were blocked by breed, and cows within each block were balanced by parity and body condition score. Animals were assigned to four treatments arranged in a 2 × 2 factorial design and the dietary treatments were offered from week 3–16 postpartum. Treatments were flint corn grain processed either as fine ground (FGC) or steam-flaked (SFC; density of 360 g/L) associated with Ca salts of palm fatty acids supplementation either not supplemented (CON) or supplemented (CSPO; 400 g of CSPO cow/day, Nutri Gordura Lac, Nutricorp Inc., Araras, SP, Brazil). This level of fat supplementation was adopted to provide 2.0% of fatty acids of diet dry matter, assuming an intake of 17.5 kg/d and 85% of fatty acids in the Ca salts supplement (NRC, 2001). The treatments were added to a concentrate supplement (9 kg of dry matter/day), which was fed individually in two equal feedings at 400 and 1,600 h before milking. The ingredients and nutritional composition of the concentrate mixes are presented in Table 1.
Cows were managed as previously described (Batistel et al., 2017). Briefly, animals rotationally grazed elephant grass (Pennisetum purpureum L. Cameroon) and were allocated to a new paddock with 2,000 m2 every day, after evening milking, with free access to natural shade and freshwater. Elephant grass was managed in a rotational grazing system based on canopy height; the entry height was 103 cm, previously determined as the point for the canopy to intercept 95% of the incident light (Voltolini et al., 2010). The experimental group of cows grazed the canopy down to an average of 66 ± 3.2 cm. The nutritional composition of the forage is presented in Table 1.
Sample Collection and Analysis
Concentrate and forage samples were collected weekly and analyzed for dry matter, crude protein, neutral detergent fiber, acid detergent fiber, lignin, ash, starch, and fatty acids according to methods described by Batistel et al. (2017). Cows were milked twice a day at 600 and 1,800 h and milk yield was recorded daily. Milk samples from both milkings were collected once a week and preserved with a bronopol preservative pill. Milk samples were analyzed for fat, protein, lactose, and milk urea nitrogen using infrared procedures (Foss 4000; Foss North America, Eden Prairie, MN). Cows were weighed weekly after the morning milking. Body condition was scored weekly by two trained investigators on a 5-point scale, where 1 = thin and 5 = fat (Wildman et al., 1982).
Forage intake and total dry matter intake (DMI) were measured at weeks 6 and 14 postpartum from total fecal excretion and feed indigestibility. To determine fecal excretion, titanium dioxide was dosed twice a day (20 g/cow per day) after each milking for 15 days (De Souza et al., 2015). Fecal grab samples were collected after morning and afternoon milking from the last 5 days and then stored in at −20°C. Subsequently, samples were thawed, dried at 55°C in a forced-air oven, ground through a 1-mm screen (Wiley mill, Thomas Scientific), and composited by the cow. To determine fecal excretion, fecal samples were analyzed for titanium concentration according to Myers et al. (2004). To determine indigestibility, the indigestible neutral detergent fiber content (iNDF) of forage, concentrate, and fecal samples were determined according to Huhtanen et al. (1994). The iNDF contents of forage and concentrate were divided by the iNDF content in the feces of each cow to calculate the indigestibility of feeds for each animal. Total fecal excretion, fecal excretion coming from the concentrate (FEC), forage intake and total dry matter intake were calculated as follows: (1) total fecal excretion = Amount of titanium dioxide dosed (g/d)/concentration of titanium dioxide in feces (g/kg); (2) FEC = concentrate intake (kg/d) × concentrate indigestibility (%); (3) Forage intake = total fecal excretion (kg/d) – FEC (kg/d) / forage indigestibility; and (4) Total dry matter intake = concentrate intake (kg/d) + forage intake (kg/d).
Urine samples were collected from each cow at weeks 6 and 14 postpartum. Samples were acidified with H2SO4 (pH of urine <5) and stored at −20°C until further analysis. Urine samples were analyzed for creatinine, allantoin, and uric acid using an HPLC instrument (Pimpa et al., 2001) and for nitrogen by the micro-Kjeldahl procedure (AOAC, 1990). Total urine production was calculated by creatinine content of each sample, assuming that excretion of creatinine was constant at 0.213 mmol/kg of body weight (Chizzotti et al., 2008). Nitrogen intake was calculated from intakes of concentrate and pasture and their respective nitrogen contents. Urinary nitrogen excretion was calculated from total urine production times its nitrogen content. Apparent efficiency of nitrogen (assuming no retention or mobilization of body nitrogen) was calculated for each cow by dividing milk nitrogen output (milk crude protein/6.38) by total nitrogen intake. Fecal samples were analyzed for total nitrogen using a combustion assay (Leco FP2000 N Analyzer; Leco Instruments Inc., St. Joseph, MI). Fecal nitrogen excretion was calculated from fecal nitrogen output. Microbial synthesis in the rumen was estimated according to the method of Chen and Gomes (1992) from daily excretion of purine derivatives.
Blood samples were taken from a subset of 20 cows (five cows per treatment) at week 13 postpartum during three consecutive days. Samples were collected from a coccygeal artery, at ~400, 800, 1,200, and 1,800 h. Blood was refrigerated until centrifugation (2,400 × g, 4°C, 20 min), and the resulting plasma was stored at −20°C until analysis. Plasma samples were composited by cow and day and amino acid concentration was analyzed using a gas chromatograph coupled with a mass spectrometer (Shimadzu Corp, single quadrupole GCMS-QP2010; Kyoto, Japan).
Human-Edible Nutrient Recovery
We used the approach proposed by Takiya et al. (2019) to estimate HE nutrient recovery. Human-edible metabolizable energy and protein contents of diets were estimated based on sugar, starch, true protein, and fat concentrations in corn grain, soybean meal products, and palm oil-based fat supplement. The metabolizable energy content of feeds and milk was calculated based on Atwater calorie factors (Atwater and Bryant, 1900): 4 kcal/g of starch or sugar, 9 kcal/g of fat, and 4 kcal/g of protein. We calculated HE nutrient recovery in milk in two scenarios; the first considered corn, soybean meal, and palm oil-based fat supplement as HE feeds and the pasture as unedible (Pasture Scenario); the second was similar to the first scenario but we considered that the land used for pasture production could be used to grow soybeans and corn (Land Scenario). To estimate HE metabolizable energy and protein from the land scenario, we used a blend of corn and dehulled soybeans (82 and 18% on dry matter [DM] basis, respectively) suggesting a metabolizable energy:protein ratio similar to milk (21.8 kcal/g protein; 3.5% fat, 3.2% protein, and 4.8% lactose) in place of forage (elephant grass). Therefore, one hectare used for elephant grass production could be used to grow 0.70 ha of corn and 0.30 ha of soybeans. The annual yields (on DM basis) of corn (5,719 kg/ha), and soybeans (3,206 kg/ha), retrieved from CONAB 2018/2019 Annual Crop Brazilian Production Summary (CONAB, 2020), were used to determine the potential HE energy and protein displaced by pasture production. Pasture yield (DM basis) was calculated based on the yield of pasture leaves for elephant grass previously reported (De Souza et al., 2017). Soybean yield was discounted 7.3% (Perkins, 1995) to account for the inedibility of hulls.
To account for amino acid recovery, we used the same approach proposed by Takiya et al. (2019) estimating the HE digestible amino acid inputs and outputs using the feed total amino acid content and standardized ileal digestibility (SID) library. Recoveries of HE nutrients in milk were calculated as the ratio between nutrients secreted in milk and the corresponding HE nutrient intake by cows. Detailed calculations for HE nutrient displacement by forage, HE SID content of feeds, and HE nutrient recovery can be found in the Supplementary Material.
Statistical Analyses
Analysis of variance was performed using the Mixed procedure of SAS 9.4 (SAS Institute) in a model including the fixed effects of corn processing, fat supplementation, and their interaction as well the random effect of the block. The normality of the residuals was checked with normal probability and box plots and homogeneity of variances with plots of residuals vs. predicted values. The variance components matrix was the covariate structure used for repeated measures analysis because it resulted in the lowest Bayesian information criterion for most of the variables measured. Significances were determined at P ≤ 0.05 and tendencies at P ≤ 0.10.
Experiment 2
Experiment Description
This trial was conducted simultaneously with Experiment 1 to provide ruminal metabolic information. Four rumen-cannulated multiparous dairy cows, with 524 ± 16 kg of body weight, milk yield of 12.5 ± 3.1 kg, and 175 ± 18.1 days in milk were used in a 4 × 4 Latin Square design. Grazing management and concentrate feeding were the same as described in Experiment 1, except that each cow received 6.0 kg of concentrate per day (DM basis). This concentrate level was established to simulate the forage:concentrate ratio of the cows in Experiment 1. Each experimental period lasted 24 days; 14 days for adaptation and 10 days for sampling.
Sample Collection and Analysis
Cows were milked twice a day at 600 and 1,800 h and milk yield was recorded daily during the sampling period. Milk samples from both milkings were collected every two days and preserved with a bronopol preservative pill. Milk samples were analyzed for fat, protein, lactose, and milk urea nitrogen as previously described in Experiment 1.
Forage intake and total DMI were measured every period from total fecal excretion and feed indigestibility as previously described in Experiment 1. Titanium dioxide was dosed during the last 15 days of each period.
Ruminal fluid was collected from three locations in the ventral rumen on day 20 of each period. Samples were taken at 0 (relative to morning concentrate feeding), 2, 4, 6, 8, 12, 18, and 24 h, strained through two layers of cheesecloth, and pH was measured immediately using a glass electrode. Two 50-mL samples of ruminal fluid were then preserved by the addition of 1.0 mL of 50% H2SO4 and stored at −20°C. Ruminal fluid samples were thawed at room temperature and centrifuged (15,000 × g, 4°C, 30 min), and the supernatant was analyzed for volatile fatty acids by gas chromatography (Erwin et al., 1961) and ammoniacal nitrogen by the phenol-hypochlorite procedure (Chaney and Marbach, 1962). Methane was calculated using volatile fatty acid stoichiometry as previously reported (De Souza et al., 2020) according to the equations proposed by Williams et al. (2019).
Statistical Analyses
Analysis of variance was performed using the Mixed procedure of SAS 9.4 (SAS Institute) in a model including the fixed effects of corn processing, fat supplementation, and their interaction as well the random effect of period and cow. The normality of the residuals was checked with normal probability and box plots and homogeneity of variances with plots of residuals vs. predicted values. The variance components matrix was the covariate structure used for repeated measures analysis because it resulted in the lowest Bayesian information criterion for most of the variables measured. Significances were determined at P ≤ 0.05 and tendencies at P ≤ 0.10.
Results
Experiment 1
Production responses, nutrient digestibility, and milk fatty acid profile results were published previously (Batistel et al., 2017). No treatment differences were observed for nitrogen intake and the excretion of nitrogen in the feces (P > 0.10; Table 2). Cows fed SFC decreased excretion of nitrogen via urine compared with FGC (P < 0.01), whereas feeding CSPO did not affect it compared with CON (P = 0.56). Feeding SFC instead of FGC increased milk nitrogen output (P < 0.01) as well as supplementing CSPO also increased milk nitrogen output compared with CON (P < 0.01). Apparent nitrogen digestion was not affected by corn processing (P = 0.33; Figure 1A), but cows fed with CSPO had greater apparent nitrogen digestion compared with CON (P = 0.01). Cows fed SFC compared with FGC had greater efficiency of nitrogen utilization with lower urinary nitrogen (P < 0.01; Figure 1B) and greater milk protein output (P < 0.01; Figure 1C) per unit of nitrogen intake. Compared with FGC, SFC increased the arterial plasma concentration of all the amino acids measured (Ala, Arg, Asp, Cys, Glu, Gly, His, Ile, Leu, Lys, Met, Phe, Pro, Ser, Thr, Tyr, and Val; P < 0.01; Table 3), and the summation of indispensable and dispensable amino acids (P < 0.01). No effect of CSPO supplementation was observed for arterial plasma concentration of amino acids compared with CON (P > 0.10).
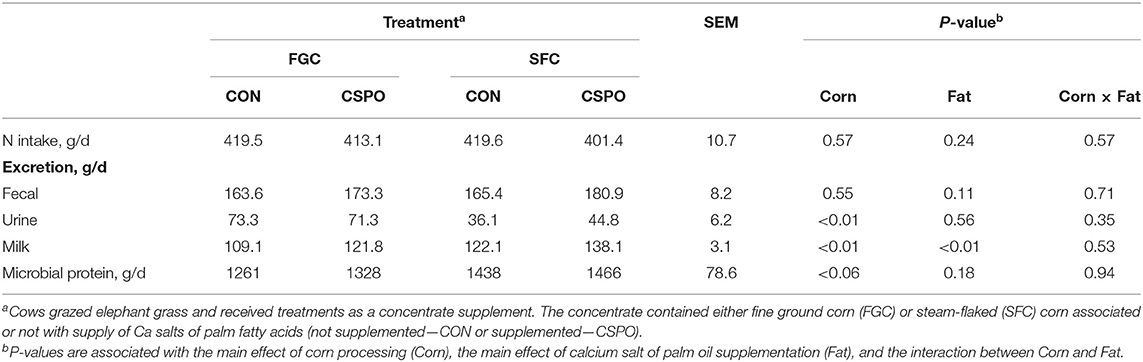
Table 2. Nitrogen (N) intake, nitrogen excretion, and rumen microbial protein of dairy cows receiving the treatment diets (Experiment 1).
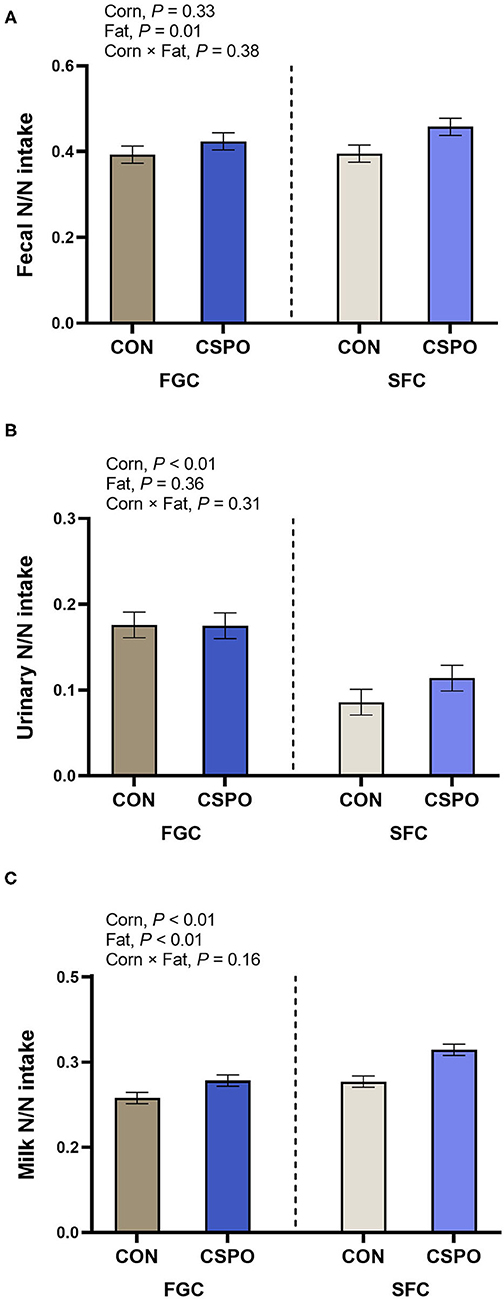
Figure 1. Apparent nitrogen digestion (A) and nitrogen excretion in urine (B) and milk (C) in cows that grazed elephant grass and received treatments as a concentrate supplement. The concentrate contained either fine ground corn (FGC) or steam-flaked (SFC) corn associated or not with supply of Ca salts of palm fatty acids (not supplemented—CON or supplemented—CSPO).
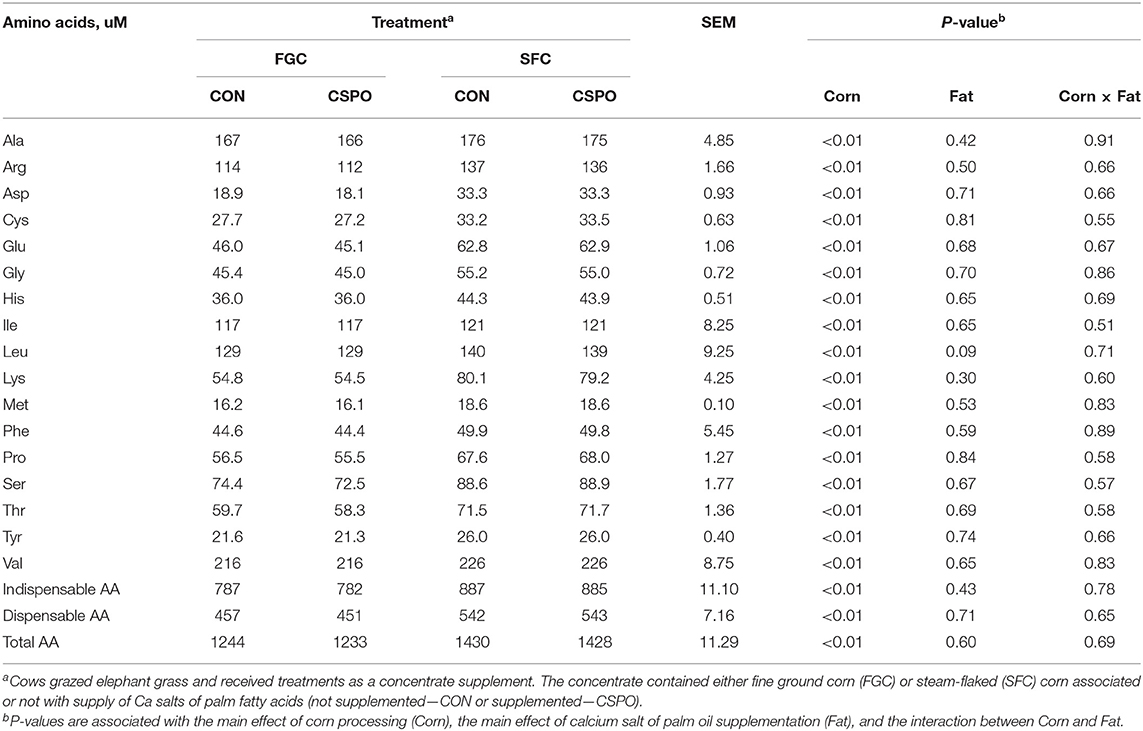
Table 3. Arterial plasma amino acid (AA) concentration of dairy cows receiving the treatment diets (Experiment 1).
We calculated HE energy and protein input considering two scenarios (Pasture and Land Use; Table 4). In the pasture scenario, we did not observe the effect of corn processing on energy and protein intake (P > 0.10), while feeding CSPO increased energy intake (P < 0.01). In the land scenario, we also did not observe the effect of corn processing on energy and protein intake (P > 0.10), while feeding CSPO tended to decrease HE protein intake compared with CON (P = 0.09). Cows fed SFC had greater milk energy (P = 0.01) and protein output compared with cows fed FGC (P < 0.01). Similarly, feeding CSPO increased milk energy (P < 0.01) and protein (P < 0.01) output compared with CON. In the pasture scenario, feeding cows with SFC increased the recovery of HE energy (P = 0.03; Figure 2A) and protein (P < 0.01; Figure 2B). Cows fed CSPO had greater recovery of HE protein (P < 0.01; Figure 2B), but no effect was observed on HE energy recovery (P = 0.22; Figure 2A). Recovery of HE energy and protein decrease in the land scenario compared with the pasture scenario (−30 and −34%, respectively). In the land scenario, cows fed with SFC increased the recovery of HE energy (P = 0.01; Figure 2C) and protein (P < 0.01; Figure 2D). Cows fed CSPO had greater recovery of HE energy (P < 0.01; Figure 2C) and HE protein (P < 0.01; Figure 2D). We also calculated the recovery of amino acids considering the pasture scenario. Cows fed with SFC had greater milk recovery of all amino acids evaluated (P < 0.05; Figure 3). Cows fed with CSPO had greater milk recovery of all amino acids evaluated (P < 0.01; Figure 3).
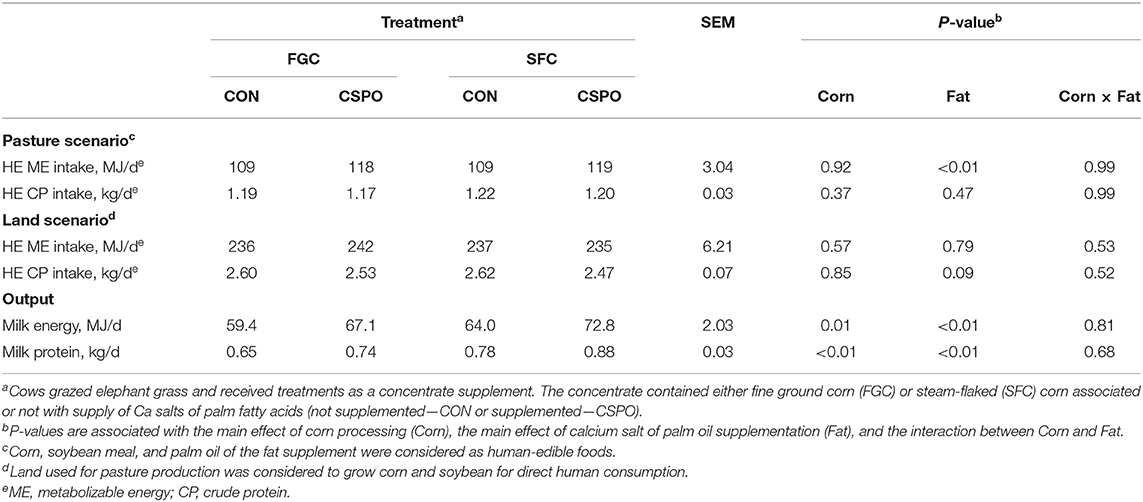
Table 4. Human-edible (HE) nutrient inputs and outputs of dairy cows receiving the treatment diets (Experiment 1).
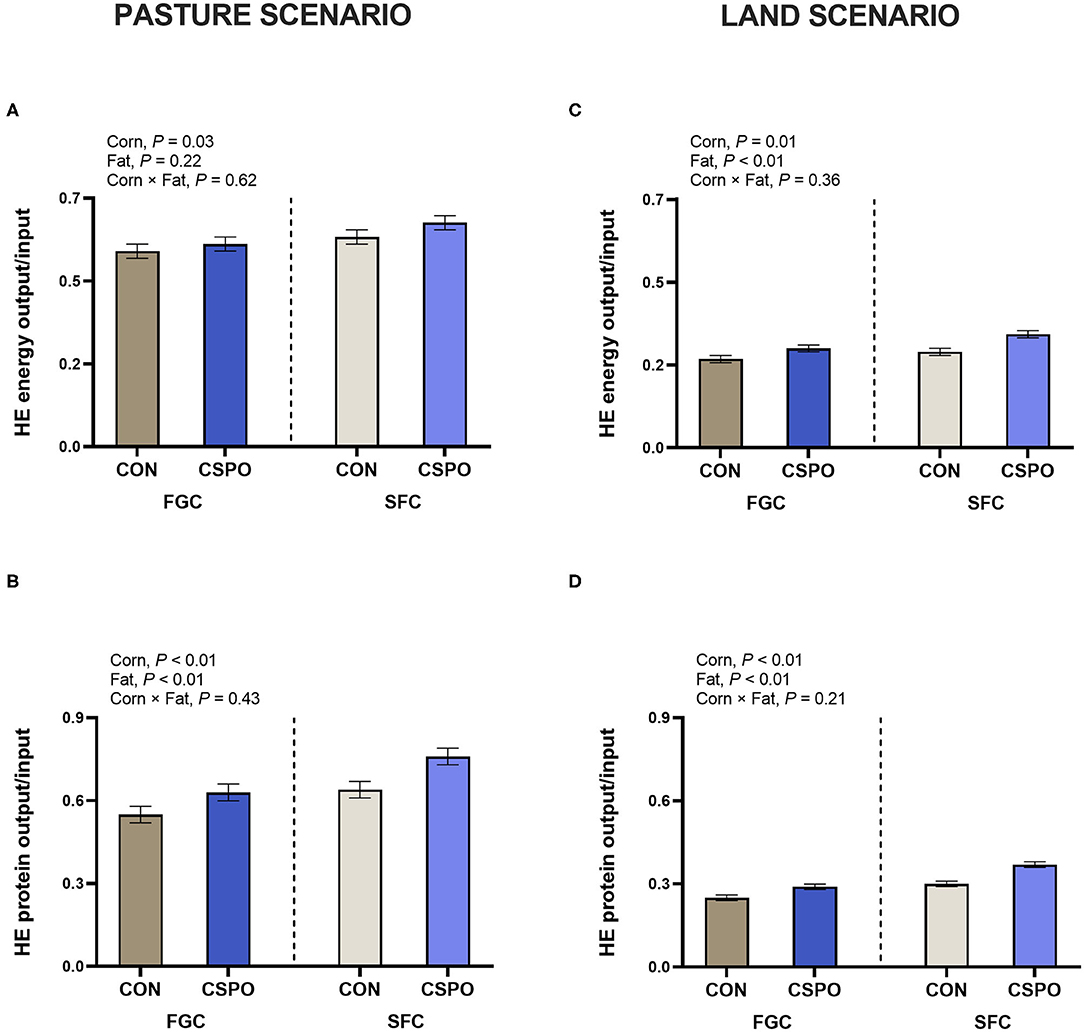
Figure 2. Human-edible (HE) metabolizable protein (bottom) and energy (top) conversion rate in cows that grazed elephant grass and received treatments as a concentrate supplement in a pasture scenario (A,B) or land scenario (C,D). The concentrate contained either fine ground corn (FGC) or steam-flaked (SFC) corn associated or not with supply of Ca salts of palm fatty acids (not supplemented—CON or supplemented—CSPO).
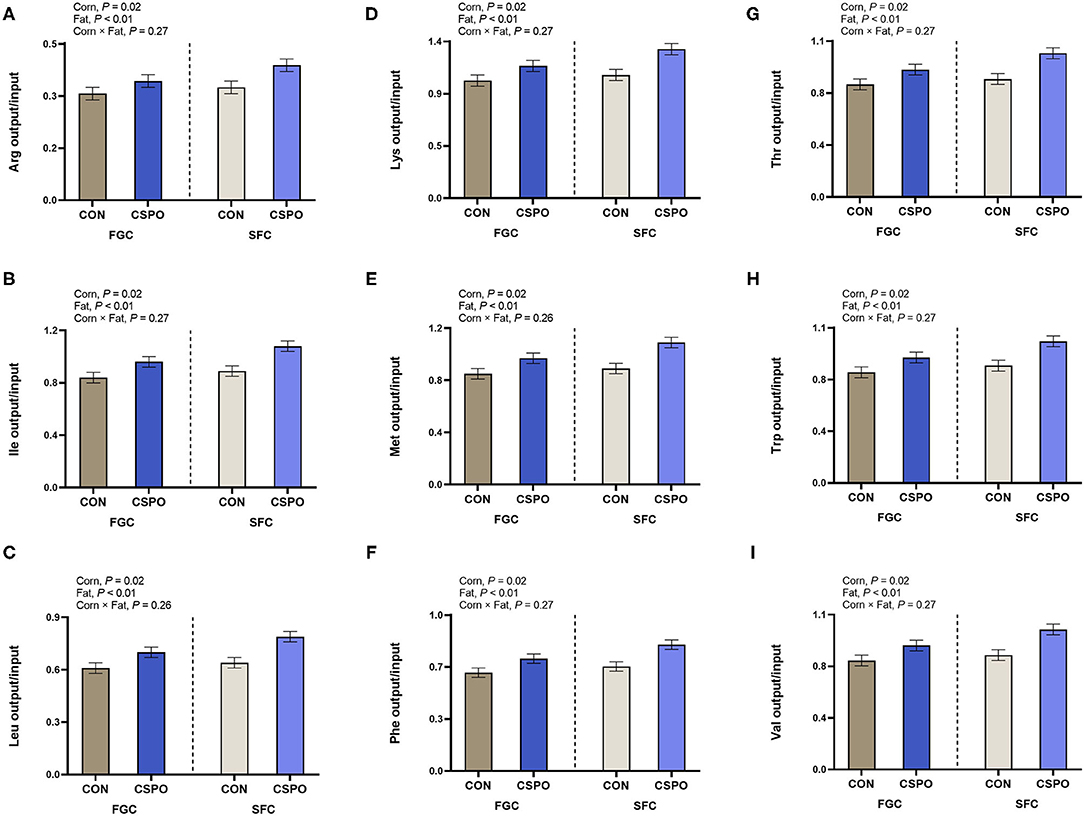
Figure 3. Milk recovery of indispensable amino acids (A–I) in cows that grazed elephant grass and received treatments as a concentrate supplement. The concentrate contained either fine ground corn (FGC) or steam-flaked (SFC) corn associated or not with supply of Ca salts of palm fatty acids (not supplemented—CON or supplemented—CSPO).
Experiment 2
No treatment differences were observed for feed intake (P > 0.10; Table 5). Cows fed with SFC had greater milk protein content (P = 0.01) and yield (P = 0.05) than cows receiving FGC. Feeding CSPO increased milk yield (P = 0.03) and fat yield (P = 0.03) compared with CON. Compared with CON, cows fed with CSPO tended to have greater milk protein yield (P = 0.08) and milk energy output (P = 0.06).
Cows fed with SFC had lower concentration of ammoniacal nitrogen (P = 0.01; Table 6) and isobutyrate (P = 0.01) than cows fed with FGC. Cows fed with SFC had greater total concentration of volatile fatty acids (P = 0.04), as well as concentration of acetate (P = 0.01), propionate (P = 0.01), butyrate (P = 0.02), and isovalerate (P < 0.01) compared with cows receiving FGC diets. The acetate/propionate ratio was lower in the cows that received SFC compared with FGC (P = 0.03). Valerate was not affected by corn processing (P = 0.39).
Feeding CSPO increased ammoniacal nitrogen (P = 0.01) compared with cows receiving CON. Feeding CSPO decreased total concentration of volatile fatty acids (P < 0.01), as well as concentration of acetate (P < 0.01), propionate (P = 0.03), butyrate (P = 0.01), and isovalerate (P = 0.03) compared with control. Valerate, isobutyrate, and acetate/propionate ratio were not affected by CSPO supply (P > 0.10).
We estimated methane output using volatile fatty acids stoichiometry (Figure 4). Feeding SFC decreased methane output in relation to feed intake (P = 0.01; Figure 4A) and tended to have lower methane output per unit of milk protein (P = 0.10; Figure 4D). Feeding CSPO tended to decrease methane output per unit of milk (P = 0.06; Figure 4B), methane output per unit of energy (P = 0.08; Figure 4C), and methane output per unit of protein (P = 0.09; Figure 4D).
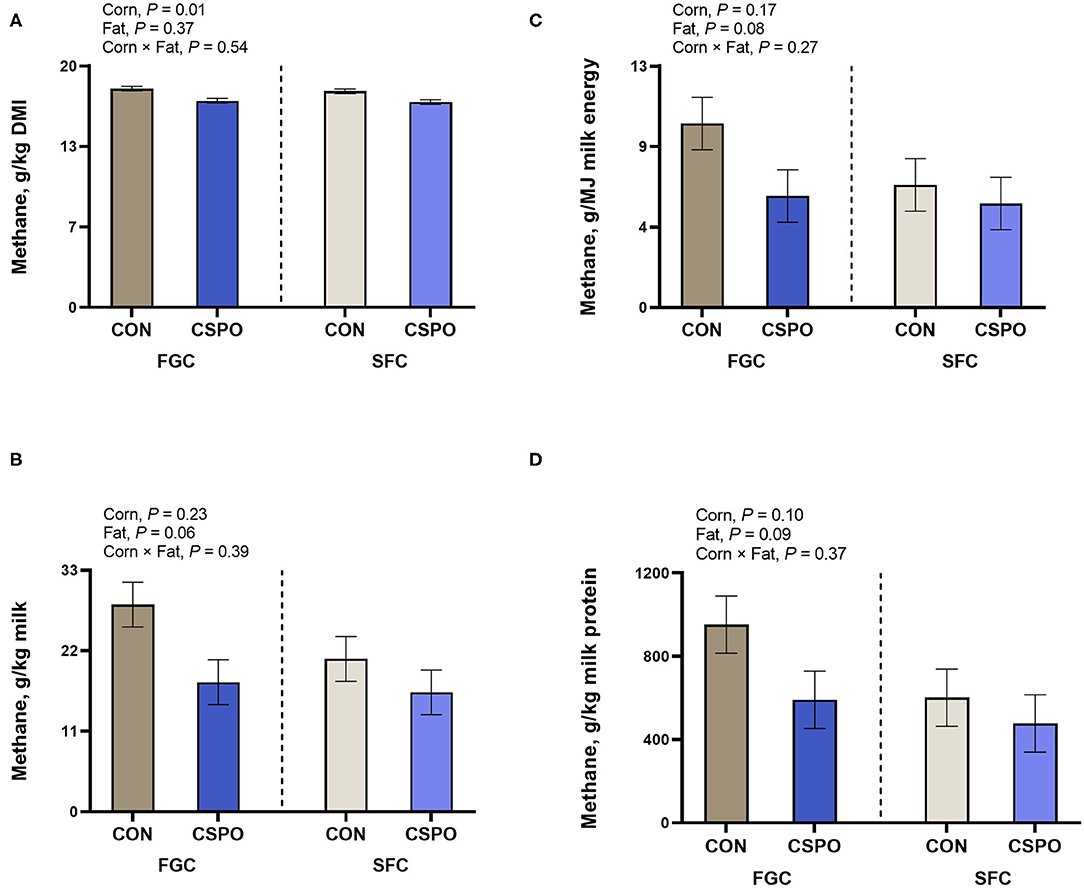
Figure 4. Estimated methane output (A–D) in cows that grazed elephant grass and received treatments as a concentrate supplement. The concentrate contained either fine ground corn (FGC) or steam-flaked (SFC) corn associated or not with supply of Ca salts of palm fatty acids (not supplemented – CON or supplemented – CSPO).
Discussion
The recent adoption of intensive management grazing practices has improved the nutritive value of tropical grasses (Da Silva et al., 2013) and animal performance (Congio et al., 2018). These management practices associated with pasture nitrogen fertilization have led to an increased level of crude protein in the forage ranging from 14 to 21% (Santos et al., 2014). According to the NRC model (NRC, 2001), for tropical forage with 62.2% total digestible nutrients, more than 14% of crude protein with 68% rumen degradable protein already leads to an excess supply of dietary protein for a dairy cow producing around 14 kg of milk daily exclusively from pasture. Importantly, the interaction between energy and protein metabolism occurs in lactating cows and influence nutrient transfer from feed into milk components, feed efficiency, and nutrient excretion. Since energy is most likely the major restriction to improve animal efficiency in pasture-based systems we evaluated whether altering energy sources in the diet through corn grain-processing method and fat supplementation affected the recovery of HE nutrient in the milk and the environmental impact (nitrogen excretion and methane emission) of dairy cows grazing on tropical pastures.
Nutritional management is an important tool to reduce the environmental impact of livestock systems and it has been the major focus of several disciplines. From the dietary nitrogen, the fraction not retained in animal tissues or secreted in milk is excreted in urine and feces, potentially contributing to water pollution, gaseous emissions, and small particulate matter formation in the atmosphere (Hristov, 2011; Cameron et al., 2013). In our study, we did not observe treatment differences in altering the corn processing method and fat supplementation for the amount of nitrogen excreted in feces indicating no differences in total nitrogen digestibility. Importantly, we observed that feeding cows with SFC markedly reduced urinary nitrogen excretion by 43% when compared with FGC. Similarly to our results, a study reported that nitrogen excretion was reduced by 30% and urinary nitrogen excretion by 45% when dietary crude protein content was lowered from 19 to 14% (Misselbrook et al., 2005). This reinforces that nitrogen utilization is not exclusively determined by the level of protein in the diet and that increasing the energy available to the rumen microbes can reduce nitrogen excretion. It is well-established that urinary nitrogen is much more labile and susceptible to fast leaching and volatilization losses than fecal nitrogen (Powell et al., 2017). Reducing urinary nitrogen through diet manipulation represents an important way to reduce nitrogen excretion and mitigate the environmental impact (Dijkstra et al., 2018). Therefore, our results indicate that altering the processing method of flint corn can be a useful strategy to reduce nitrogen excretion of cows under grazing conditions.
Ruminant animals are relatively inefficient utilizers of feed nitrogen. In research trials with dairy cows, milk nitrogen efficiency varied from 14 to 45% (average of 25 and 28% for North American and North European data, respectively) as reported in a meta-analysis by Huhtanen and Hristov (2009). In our study, we observed that feeding cows with SFC improved milk nitrogen efficiency by 17% compared with FGC. This increased in milk nitrogen efficiency is mostly related to the greater milk protein output (+ 16%) when cows received SFC compared with FGC (Batistel et al., 2017). Similarly, a summary of 19 lactation trials in North America has indicated that processing corn as a steam flake increased milk protein yield by 7.5% compared with rolled or ground corn (Theurer et al., 1999). However, these results reported by Theurer et al. (1999) were obtained in experiments conducted with dent corn grain, which is less vitreous than the flint corn grain typically grown and fed in several countries [e.g., Brazil, Colombia, and Northern Europe (Correa et al., 2002)]. Because vitreousness has been negatively correlated with ruminal starch availability, extensive processing of corn grain is expected to be of greater benefit with flint than with dent corn. The benefits of steam flaking on the digestion of corn are related to an increased surface area of grains and gelatinization of the starch due to the denaturation of the protein matrix (Mcallister et al., 2007). With that, not only total dietary starch digestibility increases but also a greater fraction of starch is digested in the rumen, which may favor microbial growth. This is supported by our Experiment 2, in which we observed that SFC increased the concentration of total volatile fatty acids and propionate while reduced the acetate:propionate ratio indicating greater production of gluconeogenic precursors. Furthermore, previous studies have reported that the synthesis of glucose precursors in the rumen from starch (Rius et al., 2010b; Cantalapiedra-Hijar et al., 2014b), and post ruminal glucose infusions (Nichols et al., 2019) can improve milk nitrogen efficiency. Thus, increases in milk protein output and milk nitrogen efficiency due to corn processing could be a result of (1) greater microbial synthesis (Oba and Allen, 2003) and a consequent increase in the post hepatic availability of amino acids (Cantalapiedra-Hijar et al., 2014a), or (2) effects of insulin on the activation cascade for milk protein synthesis (Arriola Apelo et al., 2014). Additionally, we also observed that feeding supplemental fat improved milk nitrogen efficiency by 17% compared with cows on CON diets. In agreement with our results, Nichols et al. (2018) reported that feeding supplemental fat enhanced milk nitrogen efficiency by 8% in dairy cows fed in a confinement system. Although fatty acids are not glucose precursors, supplying dietary long-chain fatty acids may increase their direct incorporation into milk fat reducing the glucose requirement in the mammary gland to synthesize de novo fatty acids (Rico et al., 2017). Thus, energy precursors can allow the use of available indispensable amino acids for milk protein synthesis by sparing them from gluconeogenesis or energy-yielding oxidative processes (Rius et al., 2010a). Importantly, while we observed increased milk nitrogen efficiency in response to SFC and supplemental fat, this was driven by increased milk nitrogen output and not by decreased nitrogen intake, which indicates that intensively grain processing and supplemental fat can be used to improve nitrogen utilization under grazing conditions when usually nitrogen intake is greater than animal needs.
In ruminants, the microbial fermentation produces short-chain fatty acids and microbial cells that are the major energy and amino acid sources nourishing the host. From the protein reaching the intestine, under normal conditions, over 50% can be originated from the microbial origin in dairy cows (NRC, 2001). Also, microbial protein is abundant in indispensable amino acids including lysine, leucine, and isoleucine (Sok et al., 2017). Therefore, improving the synthesis of microbial protein in ruminants not only guarantee a greater supply of protein, but also a supply of an excellent amino acid profile to be used by the animal. In our study, cows that received SFC tended to increase the synthesis of microbial protein in the rumen. Similarly, a previous meta-analysis indicated a greater flow of microbial protein to the duodenum of dairy cows when corn was processed as a steam-flake compared to dry rolled (Firkins et al., 2001). We also observed in Experiment 2 that ruminal concentration of ammoniacal nitrogen decreased with SFC compared with FGC reinforcing a greater utilization of nitrogen by the rumen microbes. The higher arterial plasma concentration of indispensable and dispensable amino acids in cows fed SFC compared with FGC reflects the synthesis of microbial protein in the rumen. In a previous study with cows in confinement, the authors observed that processing dent corn as steam flake did not change the plasma concentration of indispensable and dispensable amino acids, but SFC increased the mammary gland utilization of some indispensable amino acids (e.g., lysine, leucine) compared with rolled corn (Tagari et al., 2004). Altogether, our results suggest a benefit of extensively flint corn processing increasing microbial protein yield, the arterial plasma concentration of indispensable amino acids, and improvements in nitrogen utilization in grazing cows.
The livestock sector contributes with about 18% of the total global anthropogenic emissions of greenhouse gas emissions (Steinfeld et al., 2006) with enteric methane production being one of the main sources of emissions from dairy production systems and is among the main targets of mitigation practices for the dairy industry (Hristov et al., 2013). Therefore, mitigating enteric methane emissions is an approach for improving the sustainability and profitability of dairy production systems (Shetty et al., 2017). Importantly, one limitation of our study is that we estimated methane production using volatile fatty acid stichometry rather than directly measuring it. Using volatile fatty acid stichometry to estimate methane production has been widely used in previous studies because of the strong relationship between rumen fermentation parameters and methane (Cabezas-Garcia et al., 2017; Williams et al., 2019; De Souza et al., 2020). It is important to recognize uncertainty and variability in methane across different methods (Hristov et al., 2018); however, the method used still is feasible to compare differences across treatments. In our study, we did observe tendencies for a decrease in methane per unit of feed intake (−4%) and methane per unit of milk protein output (−30%) when comparing SFC with FGC diets. With beef cattle, feeding steers with diets varying corn grain from 56 to 73% of the diet decreased methane per unit of feed intake by 20% partially by decreasing feed intake by 4% when corn was processed as steam-flake compared to ground corn (Hales et al., 2012). Feed intake has been positively related to methane production (Ramin and Huhtanen, 2013), but in our study, we did not observe feed intake differences across treatments. Decreased methane emissions due to corn processing could be associated with differences in ruminal fermentation or a decreased ruminal pH which is potentially toxic to methanogenic bacteria (Van Kessel and Russell, 1996; Beauchemin and Mcginn, 2005). Also, we observed in our study changes in volatile fatty acids concentration that are likely indicative of changes in rumen fermentation patterns and/or microbial population. The concentration of acetate was decreased by 8% while the concentration of propionate increased by 14% when cows were fed SFC compared to FGC. In general, decreased methane production is associated with decreased rumen acetate proportion and increased propionate proportion (Johnson and Johnson, 1995); therefore, our results are in line with previous literature.
Furthermore, several studies have suggested that adding supplemental fat can decrease methane output (Hristov et al., 2013). A meta-analysis analyzed 27 studies and concluded that a 1% unit increase in dietary fat would decrease methane yield by 1 g/kg DMI in cattle and 2.6 g/kg in sheep (Grainger and Beauchemin, 2011). However, it is important to point out that in most studies feeding supplemental fat, a source of unsaturated fatty acid was fed. A negative association of unsaturated fatty acids and methane is expected since during biohydrogenation some H2 are used by rumen bacteria (Dijkstra et al., 2011). Also, feeding unsaturated fatty acids can decrease methane output because they usually are negatively associated with ruminal fiber digestion (Weld and Armentano, 2017) and feed intake (Rabiee et al., 2012). In our study, we fed a rumen-protected source of fatty acids with a fatty acid profile containing about 50% of saturated and unsaturated fatty acids. We observed a tendency for decreased methane per unit of milk (−31%), methane per unit of milk energy output (−29%), and methane per unit of milk protein output (−31%) when CSPO was fed compared with CON. Importantly, the source of fat that was fed in our study did not affect feed intake and fiber digestion (Batistel et al., 2017). When we fed CSPO in the diet, we replaced it with corn in the diet, and thus feeding supplemental fat decreased the intake of fermentable carbohydrates. Decreased intake of fermentable carbohydrates has been associated with a decrease in methane output (Cabezas-Garcia et al., 2017). Furthermore, most of the decrease in the ratio of methane per unit of milk, energy, and protein in our study is a result of the increased performance of cows receiving CSPO compared to CON.
Reducing the environmental impact of the livestock sector requires improving the efficiency of livestock production (Schader et al., 2015). Measuring efficiency is a broad terminology in livestock systems with the most common measurement in animal nutrition being the ratio of conversion of total feed into output. Recently, the concept of measuring efficiency considering edible feeds was introduced by Wilkinson (2011) to relate HE nutrient inputs and outputs in animal products. Although this concept has been recently applied to estimate HE energy and protein recovery in milk of cows fed in a confinement system (Ertl et al., 2015; Karlsson et al., 2018), some limitations to this approach have been proposed. Some concerns on Wilkinson's method includes (1) only estimates the proportion of crops that are potentially suitable for human consumption rather than considering the nutrient composition of each feedstuff; (2) do not consider that land used for growing forages may be used for growing crops that generate directly food consumed by humans; and (3) do not consider the quality and composition of output vs. input. Thus, Takiya et al. (2019) proposed an alternative approach that calculates the efficiency of HE feed utilization, considering energy, protein, and digestible amino acid content of feeds in cattle diets that could potentially be used for human consumption relative to the secretion of the respective nutrients in milk. To our knowledge, our study is the first to consider the concept of measuring the efficiency of HE nutrients under grazing conditions.
We used a similar approach as Takiya et al. (2019) to calculate the recovery of HE nutrients under two scenarios. Since land can be used to crop production, we calculated the recovery of HE nutrients using two scenarios. The pasture scenario considered corn, soybean meal, and palm oil-based fat supplement as HE feeds and the pasture as inedible; the land scenario considered that the land used for pasture production could be used to grow soybeans and corn. Under the pasture scenario, we observed that SFC increased the efficiency of recovery of HE energy by 9% and HE protein by 18% when compared with FGC. Also, feeding supplemental fat increased the recovery of HE protein by 17% when compared with CON diets. Similarly, Takiya et al. (2019) reported that feeding byproducts to cows fed in a confinement system increased HE recovery of energy by 13% in and 22% in terms of protein. Under the land scenario, we observed that SFC increased the efficiency of recovery of HE energy by 11% and HE protein by 21% when compared with FGC. Also, feeding supplemental fat increased the recovery of HE energy by 14% and protein by 20% when compared with CON diets. This suggests that regardless of method of calculation used for assessing HE nutrient recovery, both supplementing fat and corn processing were effective methods to improve the recovery of HE nutrients in milk under grazing conditions. When we considered the land used to grow the forage used for grazing that potentially could be used to grow corn and soybean to be used in human diets, the overall efficiency of recovery of HE energy dropped from 59 to 28% and protein from 64 to 30% (comparing pasture vs. land scenario, respectively). Corroborating with our results, Takiya et al. (2019) observed that HE energy recovery dropped from 96 to 60%, and 84 to 62% for HE protein recovery when they incorporated the HE opportunity cost of land used for alfalfa yield in their calculations. Previous analysis suggested that a combination of corn and soybeans would be the most effective way to feed people with limited land resources on a protein- and energy-equivalent basis to milk (Vandehaar, 1998). Importantly, we assumed that all land could be used to crop production instead of forage production, while in reality forage for grazing can be produced in non-arable land as well. Therefore, it is essential to consider the model assumptions and their applications in each case when comparing efficiency in livestock systems.
Protein quality is an important factor to consider for many developing countries where protein intake is limited when comparing the consumption of animal-based vs. plant-based protein (Ritchie et al., 2018). Because of ruminal fermentation, ruminants can convert non-protein nitrogen or protein that is inedible to human consumption with poor amino acid profile into a high-quality protein. Considering HE feed conversion efficiency, Ertl et al. (2016) suggested that the value of protein in animal products is 2.15 times higher than that of proteins in the potentially HE plant protein. In our study, to account for protein quality, we calculated the recovery of HE feed digestible amino acids in milk. HE recovery for the indispensable amino acids evaluated varied on average from 38 to 117%. Interestingly, we observed a greater rate of recovery of HE lysine (117%), methionine (95%), isoleucine (93%), and threonine (92%). Lysine is present in much lower than optimal proportions for human needs in grains, and similarly, the sulfur-containing amino acids (methionine and cysteine) are proportionally slightly lower in legumes than would be optimal for human needs (Mariotti and Gardner, 2019). Also, Ritchie et al. (2018) proposed that under the current food system the absence of animal protein (meat and milk) from human diets would generate a severe lysine deficiency. Furthermore, a recent analysis indicated that removing animals from US agriculture would not affect macronutrient outcomes but likely lead to deficiencies in supplies of several nutrients for the US population, including Ca, essential fatty acids, and vitamins A and B (White and Hall, 2017). Therefore, despite the model used to calculate the recovery of HE nutrients, most models indicate that, in general, recovery of HE essential nutrients (such as indispensable amino acids, vitamins) is critical when evaluating the efficiency of livestock feed systems. Additionally, in our trial, SFC diets increased HE recovery of indispensable amino acids by 7–9% when compared with FGC diets, whereas feeding supplemental fat improved HE recovery of indispensable amino acids by 17–19% compared with CON. Previous studies feeding byproducts have also indicated improved net production of several HE amino acids (histidine, isoleucine, leucine, and lysine) when replacing corn and soybean meal (Karlsson et al., 2018; Takiya et al., 2019). Notably, our results indicate that manipulating energy sources in dairy cow diet under grazing conditions can improve the efficiency of recovery of HE nutrients especially indispensable amino acids.
Although our analysis focused solely on the utility of dairy cattle in an efficient grazing system focusing primarily on arable land as a constraint, other aspects of sustainability should be considered in further analysis. For instance, despite the increase in performance and reduction of nutrient excretion demonstrated by altering corn processing and fat supplementation, the cost of these strategies and implementation may be a constraint in several regions. For instance, extra energy costs (e.g., transportation, electricity) should be expected to extensively process grain, and to produce rumen-protected fat supplements. As pointed out by Takiya et al. (2019) focusing on maximizing one narrow aspect of sustainability will likely harm sustainability in other ways. Altogether, this study increased our understanding of how manipulating energy sources in the dairy cow diet under grazing conditions can benefit HE nutrient recovery and reduce nutrient excretion.
Conclusion
In this study, we investigated for the very first time the impact of altering animal nutrition strategies on the efficiency of recovery of human-edible nutrients in milk from cows grazing tropical pastures. We observed that grazing dairy cows are relatively efficient at using human-edible foods to produce milk and particularly in generating a net increase in digestible indispensable amino acids. Furthermore, the manipulation of energy sources in the dairy cow diet under tropical grazing conditions can improve the efficiency of recovery of human-edible nutrients. In addition, we demonstrated that changing the supply of energy sources to grazing dairy cows by altering flint corn processing or feeding supplemental fat reduced nitrogen excretion of dairy systems. We estimated that methane output was reduced by altering flint corn processing or feeding supplemental fat, but further studies are needed to evaluate the impact of these nutrition strategies on real time methane emission in grazing systems.
Data Availability Statement
The original contributions presented in the study are included in the article/Supplementary Material, further inquiries can be directed to the corresponding author/s.
Ethics Statement
The animal study was reviewed and approved by Ethical Committee for Animal Research of the University of São Paulo, Piracicaba, SP, Brazil.
Author Contributions
FB, JS, and FS contributed to the overall design of the study. FB and JS carried the experiments, acquisition of data, analyses, and drafted and revised the first version of the manuscript. AV contributed to acquisition of data. All authors reviewed and approved the final manuscript.
Funding
This work was supported by the State of São Paulo Research Foundation (São Paulo, SP, Brazil) project 12/09535-1. Nutricorp (Araras, SP, Brazil) and Cargill (Campinas, SP, Brazil) partially supported the animal diets. This research was supported by the Utah Agricultural Experiment Station at Utah State University (Logan, UT), and approved as journal paper #9415.
Conflict of Interest
JS was employed by the company Perdue Agribusiness.
The remaining authors declare that the research was conducted in the absence of any commercial or financial relationships that could be construed as a potential conflict of interest.
Acknowledgments
Authors would like to thank the employees of the Department of Animal Sciences of the University of São Paulo and also the internship students Márcio Rezende Ribeiro Soares, Mateus Mendes Vilanova e Silva, Caroline Salles de Miranda Motta, Pâmela Domingues de Andrade, and Evangelina Miqueo (all from The University of São Paulo) for their assistance with the fieldwork.
Supplementary Material
The Supplementary Material for this article can be found online at: https://www.frontiersin.org/articles/10.3389/fsufs.2021.642265/full#supplementary-material
References
Allen, M. (1996). Physical constraints on voluntary intake of forages by ruminants. J. Animal Sci. 74, 3063–3075. doi: 10.2527/1996.74123063x
Altieri, M. A., and Toledo, V. M. (2011). The agroecological revolution in Latin America: rescuing nature, ensuring food sovereignty and empowering peasants. J. Peasant Stud. 38, 587–612. doi: 10.1080/03066150.2011.582947
Arriola Apelo, S. I., Knapp, J. R., and Hanigan, M. D. (2014). Invited review: current representation and future trends of predicting amino acid utilization in the lactating dairy cow. J. Dairy Sci. 97, 4000–4017. doi: 10.3168/jds.2013-7392
Atwater, W. O., and Bryant, A. P. (1900). “The availability and fuel values of food materials,” in Anual Report (Storrs, CT: Agriculture Experiment Station).
Bargo, F., Muller, L. D., Kolver, E. S., and Delahoy, J. E. (2003). Invited Review: production and digestion of supplemented dairy cows on pasture. J. Dairy Sci. 86, 1–42. doi: 10.3168/jds.S0022-0302(03)73581-4
Batistel, F., De Souza, J., and Santos, F. a.P. (2017). Corn grain-processing method interacts with calcium salts of palm fatty acids supplementation on milk production and energy balance of early-lactation cows grazing tropical pasture. J. Dairy Sci. 100, 5343–5357. doi: 10.3168/jds.2016-12503
Beauchemin, K. A., and Mcginn, S. M. (2005). Methane emissions from feedlot cattle fed barley or corn diets1. J. Anim. Sci. 83, 653–661. doi: 10.2527/2005.833653x
Cabezas-Garcia, E. H., Krizsan, S. J., Shingfield, K. J., and Huhtanen, P. (2017). Between-cow variation in digestion and rumen fermentation variables associated with methane production. J. Dairy Sci. 100, 4409–4424. doi: 10.3168/jds.2016-12206
Cameron, K. C., Di, H. J., and Moir, J. L. (2013). Nitrogen losses from the soil/plant system: a review. Annal. Appl. Biol. 162, 145–173. doi: 10.1111/aab.12014
Cantalapiedra-Hijar, G., Lemosquet, S., Rodriguez-Lopez, J. M., Messad, F., and Ortigues-Marty, I. (2014a). Diets rich in starch increase the posthepatic availability of amino acids in dairy cows fed diets at low and normal protein levels. J. Dairy Sci. 97, 5151–5166. doi: 10.3168/jds.2014-8019
Cantalapiedra-Hijar, G., Peyraud, J.L., Lemosquet, S., Molina-Alcaide, E., Boudra, H., Nozière, P., et al. (2014b). Dietary carbohydrate composition modifies the milk N efficiency in late lactation cows fed low crude protein diets. Animal 8, 275–285. doi: 10.1017/S1751731113002012
Chaney, A. L., and Marbach, E. P. (1962). Modified reagents for determination of urea and ammonia. Clin. Chem. 8, 130–132. doi: 10.1093/clinchem/8.2.130
Chen, X., and Gomes, M. J. (1992). “Estimation of microbial protein supply to sheep and cattle based on urinary excretion of purine derivates - An overview of the technical details,” in International Feed Resources Unit, ed R. R. Institute (Aberdeen: University of Aberdeen).
Chizzotti, M. L., Valadares Filho, S. D. C., Valadares, R. F. D., Chizzotti, F. H. M., and Tedeschi, L. O. (2008). Determination of creatinine excretion and evaluation of spot urine sampling in Holstein cattle. Livestock Sci. 113, 218–225. doi: 10.1016/j.livsci.2007.03.013
CONAB (2020). Acompanhamento da Safra Brasileira de Graos. 9. Available online at: https://www.conab.gov.br/ (accessed October 6, 2020).
Congio, G.F.S., Batalha, C.D.A., Chiavegato, M.B., Berndt, A., Oliveira, P.P.A., Frighetto, R.T.S., et al. (2018). Strategic grazing management towards sustainable intensification at tropical pasture-based dairy systems. Sci. Total Environ. 636, 872–880. doi: 10.1016/j.scitotenv.2018.04.301
Correa, C. E. S., Shaver, R. D., Pereira, M. N., Lauer, J. G., and Kohn, K. (2002). Relationship between corn vitreousness and ruminal in situ starch degradability. J. Dairy Sci. 85, 3008–3012. doi: 10.3168/jds.S0022-0302(02)74386-5
Da Silva, S.C., Gimenes, F.M.A., Sarmento, D.O.L., Sbrissia, A.F., Oliveira, D.E., Hernadez-Garay, A., et al. (2013). Grazing behaviour, herbage intake and animal performance of beef cattle heifers on marandu palisade grass subjected to intensities of continuous stocking management. J. Agri. Sci. 151, 727–739. doi: 10.1017/S0021859612000858
Danes, M. a. C., Chagas, L. J., Pedroso, A. M., and Santos, F. a. P. (2013). Effect of protein supplementation on milk production and metabolism of dairy cows grazing tropical grass. J. Dairy Sci. 96, 407–419. doi: 10.3168/jds.2012-5607
De Souza, J., Batistel, F., and Santos, F. a. P. (2017). Effect of sources of calcium salts of fatty acids on production, nutrient digestibility, energy balance, and carryover effects of early lactation grazing dairy cows. J. Dairy Sci. 100, 1072–1085. doi: 10.3168/jds.2016-11636
De Souza, J., Batistel, F., Welter, K. C., Silva, M. M., Costa, D. F., and Portela Santos, F. A. (2015). Evaluation of external markers to estimate fecal excretion, intake, and digestibility in dairy cows. Trop. Anim. Health Prod. 47, 265–268. doi: 10.1007/s11250-014-0674-6
De Souza, J., Leskinen, H., Lock, A. L., Shingfield, K. J., and Huhtanen, P. (2020). Between-cow variation in milk fatty acids associated with methane production. PLoS ONE 15:e0235357. doi: 10.1371/journal.pone.0235357
Dijkstra, J., Bannink, A., Bosma, P. M., Lantinga, E. A., and Reijs, J. W. (2018). Modeling the effect of nutritional strategies for dairy cows on the composition of excreta nitrogen. Front. Sustain. Food Syst. 2:63. doi: 10.3389/fsufs.2018.00063
Dijkstra, J., Van Zijderveld, S. M., Apajalahti, J. A., Bannink, A., Gerrits, W. J. J., Newbold, J. R., et al. (2011). Relationships between methane production and milk fatty acid profiles in dairy cattle. Anim. Feed Sci. Technol. 166–167, 590–595. doi: 10.1016/j.anifeedsci.2011.04.042
Ertl, P., Knaus, W., and Zollitsch, W. (2016). An approach to including protein quality when assessing the net contribution of livestock to human food supply. Animal 10, 1883–1889. doi: 10.1017/S1751731116000902
Ertl, P., Zebeli, Q., Zollitsch, W., and Knaus, W. (2015). Feeding of by-products completely replaced cereals and pulses in dairy cows and enhanced edible feed conversion ratio. J. Dairy Sci. 98, 1225–1233. doi: 10.3168/jds.2014-8810
Erwin, E. S., Marco, G. J., and Emery, E. M. (1961). Volatile fatty acid analyses of blood and rumen fluid by gas chromatography. J. Dairy Sci. 44, 1768–1771. doi: 10.3168/jds.S0022-0302(61)89956-6
FAO (2010). Challenges and Opportunities for Carbon Sequestration in Grassland Systems. Rome: Food and Agriculture Organization of the United Nations.
FAO (2018). The Future of Food and Agriculture: Alternative Pathways to 2050. Rome: Food and Agriculture Organization of the United Nations.
FAO (2019). “Chapter 10 - context for sustainable intensification of agriculture,” in Sustainable Food and Agriculture, eds C. Campanhola and S. Pandey (Rome: Academic Press), 171–172. doi: 10.1016/B978-0-12-812134-4.00010-8
Firkins, J. L., Eastridge, M. L., St-Pierre, N. R., and Noftsger, S. M. (2001). Effects of grain variability and processing on starch utilization by lactating dairy cattle. J. Anim. Sci. 79, E218–E238. doi: 10.2527/jas2001.79E-SupplE218x
Foley, J. A., Ramankutty, N., Brauman, K. A., Cassidy, E. S., Gerber, J. S., Johnston, M., et al. (2011). Solutions for a cultivated planet. Nature 478, 337–342. doi: 10.1038/nature10452
Grainger, C., and Beauchemin, K. A. (2011). Can enteric methane emissions from ruminants be lowered without lowering their production? Anim. Feed Sci. Technol. 166–167, 308–320. doi: 10.1016/j.anifeedsci.2011.04.021
Hales, K. E., Cole, N. A., and Macdonald, J. C. (2012). Effects of corn processing method and dietary inclusion of wet distillers grains with solubles on energy metabolism, carbon–nitrogen balance, and methane emissions of cattle1,2. J. Anim. Sci. 90, 3174–3185. doi: 10.2527/jas.2011-4441
Hristov, A. N. (2011). Technical note: Contribution of ammonia emitted from livestock to atmospheric fine particulate matter (PM 2.5) in the United States. J. Dairy Sci. 94, 3130–3136. doi: 10.3168/jds.2010-3681
Hristov, A. N., Kebreab, E., Niu, M., Oh, J., Bannink, A., Bayat, A. R., et al. (2018). Symposium review: Uncertainties in enteric methane inventories, measurement techniques, and prediction models. J. Dairy Sci. 101, 6655–6674. doi: 10.3168/jds.2017-13536
Hristov, A. N., Oh, J., Firkins, J. L., Dijkstra, J., Kebreab, E., Waghorn, G., et al. (2013). Special topics–Mitigation of methane and nitrous oxide emissions from animal operations: I. A review of enteric methane mitigation options. J. Anim. Sci. 91, 5045–5069. doi: 10.2527/jas.2013-6583
Huhtanen, P., and Hristov, A. N. (2009). A meta-analysis of the effects of dietary protein concentration and degradability on milk protein yield and milk N efficiency in dairy cows. J. Dairy Sci. 92, 3222–3232. doi: 10.3168/jds.2008-1352
Huhtanen, P., Kaustell, K., and Jaakkola, S. (1994). The use of internal markers to predict total digestibility and duodenal flow of nutrients in cattle given six different diets. Anim. Feed Sci. Technol. 48, 211–227. doi: 10.1016/0377-8401(94)90173-2
Johnson, K. A., and Johnson, D. E. (1995). Methane emissions from cattle. J. Anim. Sci. 73, 2483–2492. doi: 10.2527/1995.7382483x
Karlsson, J., Spörndly, R., Lindberg, M., and Holtenius, K. (2018). Replacing human-edible feed ingredients with by-products increases net food production efficiency in dairy cows. J. Dairy Sci. 101, 7146–7155. doi: 10.3168/jds.2017-14209
Mariotti, F., and Gardner, C. D. (2019). Dietary protein and amino acids in vegetarian diets-a review. Nutrients 11:2661. doi: 10.3390/nu11112661
Mcallister, T. A., Gibb, D. J., Beauchemin, K. A., and Wang, Y. (2007). “Starch type, structure and ruminal digestion,” in Cattle Grain Processing Symposium (Tulsa, OK), 30–41.
Misselbrook, T. H., Powell, J. M., Broderick, G. A., and Grabber, J. H. (2005). Dietary manipulation in dairy cattle: laboratory experiments to assess the influence on ammonia emissions. J. Dairy Sci. 88, 1765–1777. doi: 10.3168/jds.S0022-0302(05)72851-4
Moore, J. E., and Mott, G. O. (1973). “Structural inhibitors of quality in tropical grasses,” in Anti-Quality Components of Forages, ed A. G. Matches (Madison, WI: CSSA).
Myers, W. D., Ludden, P. A., Nayigihugu, V., and Hess, B. W. (2004). Technical note: a procedure for the preparation and quantitative analysis of samples for titanium dioxide. J. Anim. Sci. 82, 179–183. doi: 10.2527/2004.821179x
Nichols, K., Bannink, A., Pacheco, S., Van Valenberg, H. J., Dijkstra, J., and Van Laar, H. (2018). Feed and nitrogen efficiency are affected differently but milk lactose production is stimulated equally when isoenergetic protein and fat is supplemented in lactating dairy cow diets. J. Dairy Sci. 101, 7857–7870. doi: 10.3168/jds.2017-14276
Nichols, K., Dijkstra, J., Van Laar, H., Pacheco, S., Van Valenberg, H. J., and Bannink, A. (2019). Energy and nitrogen partitioning in dairy cows at low or high metabolizable protein levels is affected differently by postrumen glucogenic and lipogenic substrates. J. Dairy Sci. 102, 395–412. doi: 10.3168/jds.2018-15249
Oba, M., and Allen, M. S. (2003). Effects of diet fermentability on efficiency of microbial nitrogen production in lactating dairy cows. J. Dairy Sci. 86, 195–207. doi: 10.3168/jds.S0022-0302(03)73600-5
Perkins, E. G. (1995). “Chapter 2 - composition of soybeans and soybean products,” in Practical Handbook of Soybean Processing and Utilization, ed D. R. Erickson (Urbana, IL: AOCS Press), 9–28. doi: 10.1016/B978-0-935315-63-9.50006-1
Pimpa, O., Liang, J. B., Jelan, Z. A., and Abdullah, N. (2001). Urinary excretion of duodenal purine derivatives in Kedah-Kelantan cattle. Anim. Feed Sci. Technol. 92, 203–214. doi: 10.1016/S0377-8401(01)00259-0
Powell, J. M., Barros, T., Danes, M., Aguerre, M., Wattiaux, M., and Reed, K. (2017). Nitrogen use efficiencies to frow, feed, and recycle manure from the major diet components fed to dairy cows in the USA. Agri. Ecosyst. Environ. 239, 274–282. doi: 10.1016/j.agee.2017.01.023
Rabiee, A. R., Breinhild, K., Scott, W., Golder, H. M., Block, E., and Lean, I. J. (2012). Effect of fat additions to diets of dairy cattle on milk production and components: a meta-analysis and meta-regression. J. Dairy Sci. 95, 3225–3247. doi: 10.3168/jds.2011-4895
Ramin, M., and Huhtanen, P. (2013). Development of equations for predicting methane emissions from ruminants. J. Dairy Sci. 96, 2476–2493. doi: 10.3168/jds.2012-6095
Rico, J. E., De Souza, J., Allen, M. S., and Lock, A. L. (2017). Nutrient digestibility and milk production responses to increasing levels of palmitic acid supplementation vary in cows receiving diets with or without whole cottonseed. J. Anim. Sci. 95, 436–446. doi: 10.2527/jas.2016.1089
Ritchie, H., Reay, D. S., and Higgins, P. (2018). Beyond calories: a holistic assessment of the global food system. Front. Sustain. Food Syst. 2:57. doi: 10.3389/fsufs.2018.00057
Rius, A. G., Appuhamy, J. a. D. R. N., Cyriac, J., Kirovski, D., Becvar, O., Escobar, J., et al. (2010a). Regulation of protein synthesis in mammary glands of lactating dairy cows by starch and amino acids. J. Dairy Sci. 93, 3114–3127. doi: 10.3168/jds.2009-2743
Rius, A. G., Mcgilliard, M. L., Umberger, C. A., and Hanigan, M. D. (2010b). Interactions of energy and predicted metabolizable protein in determining nitrogen efficiency in the lactating dairy cow. J. Dairy Sci. 93, 2034–2043. doi: 10.3168/jds.2008-1777
Roche, J. R., Berry, D. P., Bryant, A. M., Burke, C. R., Butler, S. T., Dillon, P. G., et al. (2017). A 100-Year Review: a century of change in temperate grazing dairy systems. J. Dairy Sci. 100, 10189–10233. doi: 10.3168/jds.2017-13182
Santos, F. a. P., Dorea, J. R. R., De Souza, J., Batistel, F., and Costa, D. F. (2014). “Forage management and methods to improve nutrient intake in grazing cattle,” in 25th Annual Florida Ruminant Nutrition Symposium (Gainesville, FL), 144–164.
Schaafsma, G. (2012). Advantages and limitations of the protein digestibility-corrected amino acid score (PDCAAS) as a method for evaluating protein quality in human diets. Br. J. Nutr. 108(Suppl.2), S333–S336. doi: 10.1017/S0007114512002541
Schader, C., Muller, A., Scialabba, N.E.-H., Hecht, J., Isensee, A., Erb, K.-H., et al. (2015). Impacts of feeding less food-competing feedstuffs to livestock on global food system sustainability. J. Royal Soc. Interface 12:20150891. doi: 10.1098/rsif.2015.0891
Shetty, N., Difford, G., Lassen, J., Lovendahl, P., and Buitenhuis, A. J. (2017). Predicting methane emissions of lactating Danish Holstein cows using Fourier transform mid-infrared spectroscopy of milk. J. Dairy Sci. 100, 9052–9060. doi: 10.3168/jds.2017-13014
Sok, M., Ouellet, D. R., Firkins, J. L., Pellerin, D., and Lapierre, H. (2017). Amino acid composition of rumen bacteria and protozoa in cattle. J. Dairy Sci. 100, 5241–5249. doi: 10.3168/jds.2016-12447
Steinfeld, H., Gerber, P. J., Wassenaar, T., Castel, V., Rosales, M., and De Haan, C. (2006). “Livestock's long shadow – Environmental issues and options,” in Livestock's Role in Climate Change and Air Pollution (Rome: F.a.a.O.O.T.U. Nations), 79–124.
Tagari, H., Webb, K., Theurer, B., Huber, T., Deyoung, D., Cuneo, P., et al. (2004). Portal drained visceral flux, hepatic metabolism, and mammary uptake of free and peptide-bound amino acids and milk amino acid output in dairy cows fed diets containing corn grain steam flaked at 360 or steam rolled at 490g/L. J. Dairy Sci. 87, 413–430. doi: 10.3168/jds.S0022-0302(04)73181-1
Takiya, C. S., Ylioja, C. M., Bennett, A., Davidson, M. J., Sudbeck, M., Wickersham, T. A., et al. (2019). Feeding dairy cows with “leftovers” and the variation in recovery of human-edible nutrients in milk. Front. Sustain. Food Syst. 3:114. doi: 10.3389/fsufs.2019.00114
Theurer, C. B., Huber, J. T., Delgado-Elorduy, A., and Wanderley, R. (1999). Invited review: summary of steam-flaking corn or sorghum grain for lactating dairy cows. J. Dairy Sci. 82, 1950–1959. doi: 10.3168/jds.S0022-0302(99)75431-7
Van Kessel, J. a. S., and Russell, J.B. (1996). The effect of pH on ruminal methanogenesis. FEMS Microbiol. Ecol. 20, 205–210. doi: 10.1111/j.1574-6941.1996.tb00319.x
Vandehaar, M. J. (1998). Efficiency of nutrient use and relationship to profitability on dairy farms. J. Dairy Sci. 81, 272–282. doi: 10.3168/jds.S0022-0302(98)75576-6
Voltolini, T. V., Santos, F. a. P., Martinez, J. C., Imaizumi, H., Clarindo, R. L., and Penati, M. A. (2010). Milk production and composition of dairy cows grazing elephant grass under two grazing intervals. Braz. Anim. Sci. 39, 121–127. doi: 10.1590/S1516-35982010000100016
Weld, K. A., and Armentano, L. E. (2017). The effects of adding fat to diets of lactating dairy cows on total-tract neutral detergent fiber digestibility: a meta-analysis. J. Dairy Sci. 100, 1766–1779. doi: 10.3168/jds.2016-11500
White, R. R., and Hall, M. B. (2017). Nutritional and greenhouse gas impacts of removing animals from US agriculture. Proc. Natl. Acad. Sci. U. S. A. 114:E10301. doi: 10.1073/pnas.1707322114
Wildman, E. E., Jones, G. M., Wagner, P. E., Boman, R. L., Troutt, H. F., and Lesh, T. N. (1982). A dairy cow body condition scoring system and its rlationship to selected production characteristics. J. Dairy. Sci. 65, 495–501. doi: 10.3168/jds.S0022-0302(82)82223-6
Wilkinson, J. M. (2011). Re-defining efficiency of feed use by livestock. Animal 5, 1014–1022. doi: 10.1017/S175173111100005X
Keywords: corn processing, fat supplementation, nitrogen excretion, methane, steam-flaked corn
Citation: Batistel F, de Souza J, Vaz Pires A and Santos FAP (2021) Feeding Grazing Dairy Cows With Different Energy Sources on Recovery of Human-Edible Nutrients in Milk and Environmental Impact. Front. Sustain. Food Syst. 5:642265. doi: 10.3389/fsufs.2021.642265
Received: 15 December 2020; Accepted: 24 February 2021;
Published: 19 March 2021.
Edited by:
Kathleen L. Hefferon, Cornell University, United StatesReviewed by:
Chayon Goswami, Bangladesh Agricultural University, BangladeshSapna Langyan, National Bureau of Plant Genetic Resources (ICAR), India
Copyright © 2021 Batistel, de Souza, Vaz Pires and Santos. This is an open-access article distributed under the terms of the Creative Commons Attribution License (CC BY). The use, distribution or reproduction in other forums is permitted, provided the original author(s) and the copyright owner(s) are credited and that the original publication in this journal is cited, in accordance with accepted academic practice. No use, distribution or reproduction is permitted which does not comply with these terms.
*Correspondence: Fernanda Batistel, fernanda.batistel@usu.edu; Flávio Augusto Portela Santos, fapsantos@usp.edu