- 1World Agroforestry (ICRAF) West and Central Africa Regional Office Sc/Center for International Forestry Research (CIFOR), Ouagadougou, Burkina Faso
- 2World Agroforestry (ICRAF), ICRAF-West and Central Africa Office, Bamako, Mali
- 3Institut de l'Environnement et de Recherches Agricoles, Ouagadougou, Burkina Faso
- 4International Union for Conservation of Nature, Central and West Africa Program (IUCN-PACO), Ouagadougou, Burkina Faso
- 5CGIAR Research Program on Climate Change, Agriculture and Food Security (CCAFS), International Crops Research Institute for the Semi-Arid Tropics (ICRISAT), Bamako, Mali
- 6AGRHYMET Regional Center, Boulevard de l'Université Abdou Moumouni, Niamey, Niger
- 7CSIR-Savanna Agricultural Research Institute (CSIR-SARI), Tamale, Ghana
- 8Institut Sénégalais de Recherches Agricoles (ISRA)-Centre National de Recherches Forestières (CNRF), Dakar, Senegal
- 9Institut National de la Recherche Agronomique du Niger (INRAN), Niamey, Niger
- 10Institut d'Economie Rurale (IER), Bamako, Mali
- 11World Agroforestry (ICRAF), Nairobi, Kenya
Climate change and variability are significant challenges for the environment and food security worldwide. Development strategies focusing simultaneously on adaptive farming, productivity, and reducing greenhouse gas (GHG) emissions-known as climate-smart agriculture (CSA) strategies-are key to responding to these challenges. For almost a decade, within the framework of Climate Change, Agriculture and Food Security (CCAFS), World Agroforestry (ICRAF), and its partners have been using Participatory Action Research (PAR) to fully engage key stakeholders in co-creating such CSA development strategies. This includes the testing of Agricultural Research for Development (AR4D) CSA scalability options. The multidisciplinary teams include the National Research and Extension Systems (NARES), national meteorological services (NMS), non-profit organizations (NGOs), and local radio programs, among others. The CCAFS-West Africa Program, World Agroforestry-West and Central Africa (ICRAF-WCA), International Union for Conservation of Nature (IUCN), University of Reading, and Centre Régional de Formation et d'Application en Agro-météorologie et Hydrologie Opérationnelle (AGRHYMET) provide technical backstopping to the national teams. Climate information (CI) was used as an entry point to inform the development of CSA technologies and practices within Climate-Smart Villages (CSV). This groundwork has led to a greater understanding of three critical factors for successful CSV implementation: (1) Building strong partnerships to co-design and develop agricultural systems that improve ecosystem and population resilience, (2) Key stakeholders (researchers, farmers, development agents, and students) capacity strengthening through vocational and academic training, and (3) Using CI for livelihood planning at all scales. These three factors support more effective identification and testing of agricultural technologies and practices addressing climate variability and change at plot, community, and landscape levels. This paper discusses the PAR-CSA methodology and parameters for evaluation, including biophysical and social change. Keys to success, including communication, knowledge sharing tools, and scalability are also discussed. Finally, future opportunities for improvement are presented, including knowledge product development, CSA policy and investment planning, capacity building, further engagement of the private sector, and additional research on existing practices and tools.
Introduction
Climate naturally varies on multiple timescales; yet the scientific community has now broadly concluded that human activities are the overwhelming cause of long-term changes (Cook et al., 2016; Chao and Feng, 2018; Oreskes, 2018; Trenberth, 2018). Relatively small net increases in temperature exist due to natural factors like energy changes from the sun and cooling effects of volcanic eruptions. However, human activities affect radiative forcing, which is the difference between sunlight absorbed by the Earth and energy radiated back to space, including changes in greenhouse gases (GHGs), small air-borne particles like aerosols, and the reflectivity of the Earth's surface. Both factors contribute to global warming due to the positive amplification of earth-atmosphere-ocean system feedback processes (Wuebbles et al., 2017). Human activities related to global food demand further exacerbate these concerns in light of rising population and changing diets (Tilman et al., 2011; Alexandratos and Bruinsma, 2012; Bodirsky et al., 2015). If current trends continue, by 2050 agricultural production will need to increase by more than 50% relative to 2005 to meet food demand (Alexandratos and Bruinsma, 2012; Hunter et al., 2017).
The West African region has both humid and dryland areas that are severely impacted by climate change. About 70% of the population predominantly depend on rainfed agriculture, making climate change a direct threat to progress toward several UN Sustainable Development Goals (SDGs), most notably Zero Hunger (SDG 1) (Kotir, 2011; Connolly-Boutin and Smit, 2016; Serdeczny et al., 2017; Diedhiou et al., 2018). In addition, models predict that temperature increases within West Africa will be higher than global mean temperature increases in both the 1.5 and 2°C average increase scenarios. This will imply more frequent, prolonged, and severe heatwaves for the entire region (Sultan and Gaetani, 2016; Diedhiou et al., 2018; Sultan et al., 2019), particularly the Sahel. Meanwhile, the Guinea Coast will experience mild changes in total precipitation with increased periods of heavy rainfall (Diedhiou et al., 2018), resulting in increased frequency of droughts and floods, as well as a later start, earlier end, and shorter length of growing seasons (Dayamba et al., 2018; Clarkson et al., 2019; Mechiche-Alami and Abdi, 2020). In terms of agriculture, these changes in regional climates will bring water scarcity, crop yield reduction or failure, and ultimately, greater food insecurity (Klutse et al., 2016; Taylor et al., 2017; Parkes et al., 2018). Although the extent of crop losses remains uncertain, models show yields reductions by up to 41% in +1.5°C average global temperature increase scenario (Sultan et al., 2019; Mechiche-Alami and Abdi, 2020).
In addition to climate constraint, the majority of the region's soils have fertility limitations despite the diversity of its agroecosystems (Jalloh et al., 2012). The main conditions include acidity, unavailability of micronutrients, low carbon content (Jayne et al., 2014), and consequently low cation exchange and water holding capacities (Bationo et al., 2006; Jayne et al., 2014). Thus, the use of unsustainable agricultural practices quickly leads to soil and land degradation (Costantini et al., 2016; Fossey et al., 2020) to the extent that applying mineral fertilizers alone becomes non-viable (Pieri, 1989; Bationo et al., 2006). These factors further contribute to chronic food and nutritional insecurity in West Africa (Pomati and Nandy, 2019). Over 77% of the population of the Economic Community of West African States (ECOWAS) is employed by the food system economy (Allen et al., 2018). Climate change adaptation that focuses on agriculture thus informs both ecosystem restoration, improved livelihoods, and regional food and nutritional security is needed. The need to have more climate-friendly approaches goes beyond the resilience of people and ecosystems; it also aims to reduce GHG emissions in alignment with the Paris Agreement. In this context, regional stakeholders have partnered to develop CSA options using locally adapted participatory methods. This work has occurred under the Participatory Action Research (PAR) efforts of the Climate Change, Agriculture, and Food Security (CCAFS) program of CGIAR (Bayala et al., 2016, 2017; Zougmoré et al., 2016; Dayamba et al., 2018; Partey et al., 2018).
West Africa like the other four regions of CCAFS program in the world has tested and validated CSA practices in the five CCAFS benchmark sites in five countries (Burkina Faso, Ghana, Mali, Niger, and Senegal) as well as in scaling up countries all together represented on Figure 1 using climate-smart village (CSV) approach. Climate-smart village concept is an agricultural research for development (AR4D) approach that robustly tests technological and institutional options for dealing with climatic variability and climate change using participatory methods. It generates evidence of what climate-smart agricultural (CSA) options work best, where, why, and how, and use this evidence to draw out lessons for decision making of different stakeholders at various scales (Bayala et al., 2016; Aggarwal et al., 2018). Climate-smart agriculture aims to increase sustainable agricultural production by adapting to and building resilience to climate variability and change while reducing GHGs wherever possible (FAO, 2013). For almost a decade, within the frame of CCAFS program, World Agroforestry (ICRAF) and its partners are developing agricultural management practices and technologies that will support farmers' CSA capacity (ICRAF, 2016; Bayala et al., 2018a; Bonilla-Findji et al., 2018). This work embraces a multifunctional landscape with integrated farming systems that provide buffer functions at several ecological and socio-economical scales. The goal is to reduce human and ecosystem risk to climate change by an iterative participatory approach. The work is conducted across stakeholders and along the learn by doing action research chain to develop appropriate policy regulations that enable long-term stakeholder CSA investments. The present paper reports the stakeholders' experiences in CSA deployment in West Africa (ICRAF, 2016; Bayala et al., 2018b; Bonilla-Findji et al., 2018).
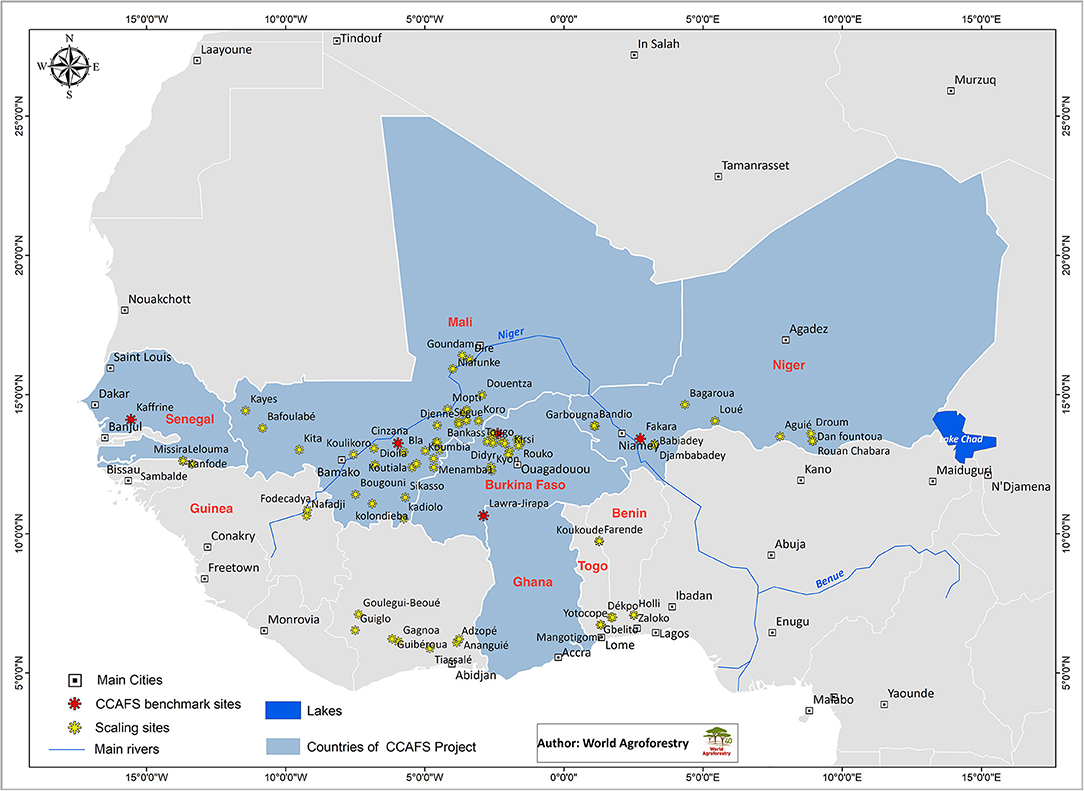
Figure 1. West African Climate-Smart Villages (CSV) Agricultural Research for Development (AR4D) and scaling up sites.
Methodological Approach Developed in West Africa for Climate-Smart Village Implementation
National Meteorological Services Data Production Capacity Building
Farmers can effectively use downscaled seasonal forecasts accompanied by local historical climate data when supported by a structured participatory process (Dinku et al., 2018a). However, West African countries do not have the robust climate information (CI) necessary to guide climate adaptation actions (Hansen et al., 2011). Few weather stations exist and the limited data they produce is not edited, well-stored, or widely used (Traore et al., 2014). This step aimed to bolster national capacity to produce good quality, locally relevant CI with broad coverage.
National Meteorological Service (NMS) staff were trained to analyze historical climate records (using Instat/R-Instat) and predict crop yield using SARAA-O/H (Traore et al., 2011; Vintrou et al., 2014; Sultan et al., 2019). Where data gaps constrained access to high-quality local climate records, NMS staff were trained to use the Enhancing National Climate Services (ENACTS) approach. This overcomes data gaps by blending NMS station data with satellite and other proxy data to produce moderately high-resolution historical gridded data (Traore et al., 2014; Dinku et al., 2018a,b). Additionally, trainees learned how to conduct an inventory and to prioritize potential climate-smart options based on three key criteria: the three pillars of CSA (production, adaptation, and GHG mitigation); a cost-benefit analysis; and degree of scalability (Table 2; Bayala et al., 2018a; M'Bo et al., 2019). The prioritization exercise eliminated options that did not fit farmers' needs and highlighted farmer-tested options (Bonilla-Findji et al., 2018; Table 1). This testing process involved CI-based planning using seasonal forecasts long time before the onset of the cropping season and making tactical adjustments during cropping seasons through short-term forecasts (Dayamba et al., 2018; Clarkson et al., 2019). Finally, they graphically formatted the data in probabilistic terms to be easily understood by people across the literacy spectrum (Dinku et al., 2018a).

Table 1. Number of practices inventoried, prioritized, and ranked in the five benchmark sites of CCAFS in West Africa: Yatenga in Burkina Faso, Lawra Jirapa in Ghana, Cinzana in Mali, Fakara in Niger, and Kaffrine in Senegal.
The analysis of local climate seasonality, trends, and variability provided insights into farmer and value chain actors' risk, as well as whether perceived changes in agricultural performance are driven by climate or environmental changes such as soil degradation (Dinku et al., 2018a). The resulting graphs were critical elements of the Participatory Integrated Climate Services for Agriculture (PICSA) trainings. Participatory Integrated Climate Services for Agriculture is an approach that uses historical climate records, participatory decision-making tools and seasonal climate forecasts to help farmers identify and better plan livelihood options suited to their own circumstances and climate conditions (Dorward et al., 2015).
Participatory Planning for Adaptation to Climate Change
Participatory Action Research uses past, present, and future CI to choose, test, and validate climate smart options for crops and livestock research and social innovations. In our case, four types of CI were used to inform the CSA selection and testing for the CSVs:
• Climate profiles to identify potential climate-smart technologies and practices;
• Climate historical records to match production choices with local climate features and individual circumstances;
• Seasonal and short-term forecasts to adjust operational plans and test for climate variability—for example: practices such as early/late planting or the use of an early maturing variety (Ghana only);
• Climate analogs to learn from similar climate sites (Senegal only).
The participatory planning process was based on the TOP-SECAC (Trousse à Outils de Planification et Suivi-Evaluation des Capacités d'Adaptation au Changement Climatique) toolkit developed by the International Union for Conservation of Nature (IUCN) (Somda et al., 2014). The process in this step involves establishing a PAR platform for Climate-Smart Agriculture (PAR-CSA) actors, identifying technological climate adaptation options for on-farm testing, and participatory evaluation and validation, and helps translate the program's common objectives into contextualized actions in each of the benchmark sites in five countries: Burkina Faso, Ghana, Mali, Niger, and Senegal (Bayala et al., 2016; Sanogo et al., 2016). In preparation for the PAR, National Agricultural Research Systems (NARSs) scientists were trained in PAR to test and validate CSA options (Bayala et al., 2016, 2020). Farmers, extension staff, and NGO staff were also trained to use CI to plan activities (Dayamba et al., 2018; Clarkson et al., 2019).
Next, rural communities collaborated to create visions of the desired CSVs and how they would deal with the effects of climate change and related hazards. Participating farmers visited villages with a climate comparable (climate analog sites) to that of their predicted climate, enabling direct observation of production systems and exchanges with locals to offer ideas of potential adaptation approaches. Thus, the climate analog sites were also used to strengthen local actors' capacity to further analyze possible climate changes in their environments and plan for the desired future. The initial outputs were shared with regional rural development stakeholders to fine-tune the model and identify strategic actors. This also helped establish partnerships between researchers, extension services, non-governmental organizations (NGOs), private-sector actors, policy makers, and communities (Somda et al., 2016) founded on developing agricultural systems that promote vegetative cover and land restoration through sustainable intensification.
Ground Testing for Evidence of Climate Smart Agriculture Effectiveness
The selected CSA technologies and practices were then tested. Tested options were comprised of a combination of:
• minimum tillage, crop rotation, organic, and inorganic fertilizer use (micro-dosing);
• land reclamation and water conservation techniques (Zaï, half-moons, earth, or stone bunds);
• restoration and species diversification (assisted natural tree regeneration, also known as Farmer-Managed Natural Regeneration or FMNR);
• protected areas or plots to regenerate vegetative cover and tree planting (wood, fruits, nuts, vegetables, and fertilization);
• crop diversification (sesame, cowpea, sorghum, hibiscus, and okra);
• short-cycle and drought-tolerant varieties (sorghum, millet, cowpea, and groundnut);
• bio-fortified varieties (cereals and orange-flesh potatoes); and
• new energy options that use Jatropha curcas-based farming systems with cereal crops.
In most cases, successfully deployed activities were based on existing techniques or innovations in the community that were adjusted to fit the new context. The exception was Ghana, where land reclamation techniques were new to the communities involved. In this country, there was a tendency to screen a large number of techniques across integrated soil fertility management techniques (crop rotation, manure and inorganic fertilizer application), new soil and water conservation practices (ridges and tied ridges, stone lines, etc.), and new crop varieties (maize, soybean, and cowpea). Emphasis was placed on interventions that were likely to be more beneficial to women and resource-poor individuals, including nutritional education e.g., soybean preparation, village savings and loans groups, and income generating activities for short-term wins. In other countries, activities that promote women's involvement include gardening, tree propagation (grafting), fruit tree planting, tree products processing, value added post-harvest handling, dry season supplementary activities, and nutrition and health information.
Progress was participatorily evaluated at the middle and end of each cropping season via field visits and community meetings. The results were discussed at the end of each rainy season in annual review and planning meetings at both community and regional levels to make adjustments when and where needed. As a result, the list of options narrowed significantly (Tables 1, 2).
Monitoring and Evaluation
All participatory evaluation processes were supported by rigorous monitoring and evaluation (M&E) (Bonilla-Findji et al., 2018; Partey et al., 2018). Monitoring and evaluation (M&E) was designed and implemented in a participatory manner, so adaptation and mitigation activities are sustained by “adaptive behavior” leading to increased food security. Behavior change from program participation was monitored at farmer and community levels using the Most Significant Changes technique (Davies and Dart, 2005) integrated into a step-by-step toolkit for planning and M&E of climate change adaptation (Somda et al., 2011). Through storytelling, significant changes enacted by individual farmers and by gender-differentiated groups were gathered and analyzed. Changes were substantiated and used to learn about newly initiated behaviors and any remaining constraints farmers face in maintaining these new behaviors.
Major Achievements and Lessons Learnt
Increased Multi-Functional Capacities of Stakeholders to Develop Climate-Smart Village
Through this work, climate information services (CIS) have increasingly become a key entry point to guide farmers' decisions and selections of crops, varieties, agro-sylvo-pastoral systems, technologies, production area, degree of intensification, production timelines, and investment levels (Sanogo et al., 2016). Climate information services are also more available and accessible by farmers thanks to national meteorology offices, mobile phone text messaging, rural radio broadcasts, and the various PICSA trainings.
Capacity building to bolster stakeholders' understanding of local climate data and evidence-based decision making (Dorward et al., 2015; Dayamba et al., 2018; Clarkson et al., 2019; ICRAF, 2019) was an ongoing component of this work. A total of 18 PICSA trainings, 4 CSA trainings, 8 data collection trainings, and 2 M&E trainings directly reached 630 extension agents, researchers, NGO staff, NMS staff, and NARS staff. Stakeholders were trained to generate and use quality historical CI at the relevant scale; assess the climate-smart potential of projects and programs; PAR; crop yield prediction modeling; CIS delivery; and leverage participatory M&E methods (Bayala et al., 2016; Somda et al., 2020). These trainings effectively developed local expertise to help sustain regional climate-smart action, thus building a foundation for CSA mainstreaming in future projects or programs (Ouédraogo et al., 2019; Bayala et al., 2020). As a result, there is evidence of CI use (Dayamba et al., 2018; Clarkson et al., 2019) and even willingness to pay for access (Ouédraogo et al., 2018). In addition, farmers received training through demonstration plots, farms-of-the-future, farmer field schools, field days for farmer-to-farmer learning, traditional annual farming festivals, local radio programming, mobile phones, etc. (Bayala et al., 2016).
Peer-to-peer conversation and collaborative learning methods within and between communities are powerful participatory ways to strengthen existing social learning approaches for scaling CSA (Somda et al., 2020). Through our M&E processes, the IUCN social learning approach team captured gender-differentiated social learning methods, institutions, and sociocultural events (Somda et al., 2020). The prediction of scalable production needs by administrative unit (sub-national, national, and regional) through the use SARRA-O/H models was meant to enabling management to plan for risks associated with the prospective volume of production (Traore et al., 2014). Finally, the co-developed CSA options have contributed to the compendium of CSA technologies developed by the CCAFS program for scalable CSA (CCAFS/ICRAF, 2020; Figure 2). These proven climate-smart technologies serve to design evidence-based effective CSA profiles and investment plans to catalyze their broad adoption at both national and regional scales (Figure 3).
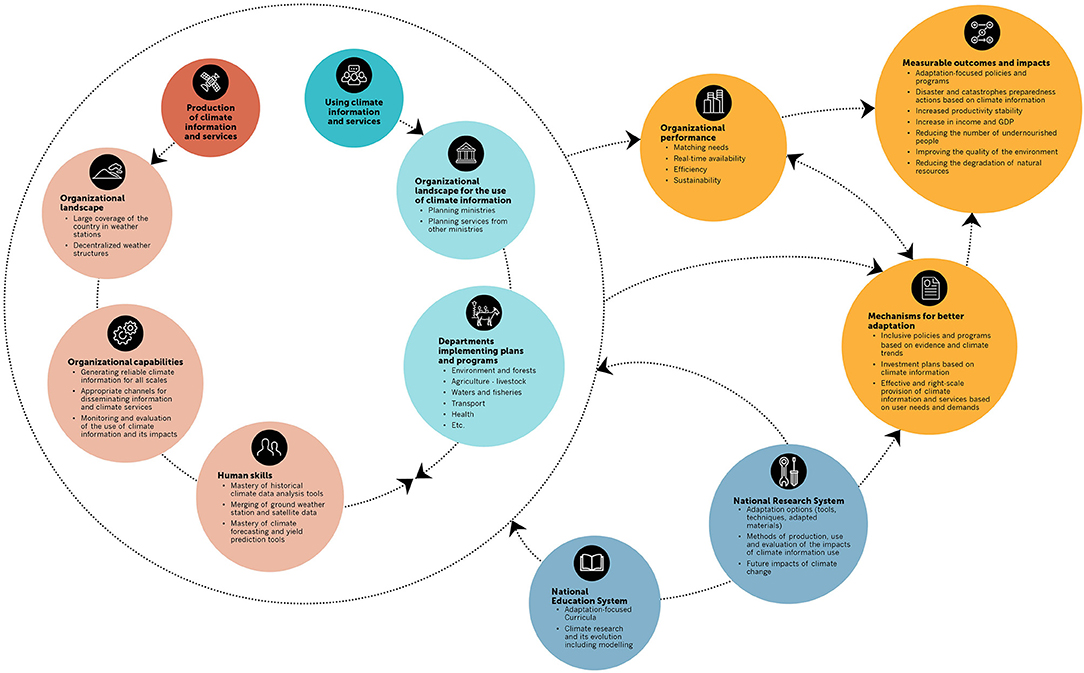
Figure 3. Suggested institutional setup for adapted climate information and advisory services delivery.
Rethinking Institutional Arrangements to Support Climate-Smart Village
There is a clear need for institutional support on CI delivery. Issues exist surrounding organizational landscape, organizational capacities, and human resources (Figure 3). Expanded weather station networks and human capital development to provide advisory services on time and at the relevant scale for planning or data-based decision making would represent significant improvements. However, skepticism around the accuracy of CI is a further obstacle to its use in decision making at all levels. In order to address this, CI must be available, and stakeholders must know how to apply the information. Effectively designed policies and investment plans to assess impacts can improve user confidence. Finally, national education and research systems must embrace climate issues with adapted curricula and production systems (Figure 3).
Strong partnership is crucial to effective implementation of PAR-CSA at the national level. Our experience suggests that the engagement of 10–12 national partners is indispensable (Bayala et al., 2016). Partnerships at the regional level are equally vital, including coordination with CCAFS programs, and CGIAR centers such as ICRAF, and other international and regional organizations like IUCN, and AGRHYMET, respectively. Such partnerships enable broad changes in knowledge, attitudes, and practices (KAP) (Somda et al., 2011). Finally, connecting PAR-CSA achievements to national policies through a science-policy dialogue is key. Some progress has already been achieved in our pilot areas in this regard, and much remains to be done (Partey et al., 2018; Raile et al., 2019; Zougmoré et al., 2019).
Balancing the Number of Trial Replicates in the Climate Smart Agriculture Participatory Action Research
Throughout implementation, a concerted effort to connect activities with national adaptation and mitigation priorities is vital. In our experience, one major challenge has been capturing a breadth of farmer experiences in sampling replicates while controlling costs (Figure 4). In addition, time constraints and a lack of perceived benefits mean farmers tend to execute activities individually on their farms rather participating collectively. This is partly due to the fact collective actions on communal lands are associated with governance issues, including land and tree tenures (Bayala et al., 2016).
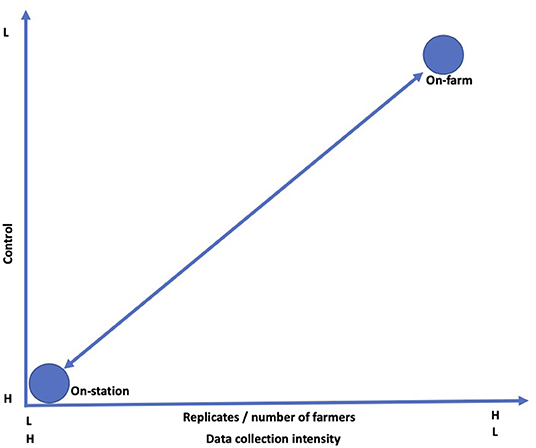
Figure 4. A balance between cost and data collection must be considered for on-farm trial replicates for PAR-CSA in West Africa. High costs (green star) and low data intensity (red star) are to be avoided. A balance between the two must be struck (blue line) (H, High; L, Low) (Source: Bayala et al., 2016).
Knowledge and Communication in Climate Smart Agriculture
Two main categories of barriers to CSA adoption were identified. The first category is about access to inputs/knowledge in broad terms: limited access to information, knowledge and new skills on CSA options, limited availability, and access to inputs and equipment, all together leading to poor technical capacity (Ouédraogo et al., 2018). The second category relates to the institutional, cultural, policy, and regulatory environments as well as governance. The last category has implications about moving from plot or household levels to landscape scale as well as on power relations.
To address these barriers, knowledge sharing and communication are crucial to effective CSA. A strong knowledge bank, such as Evidence for Resilient Agriculture (ERA; available at https://era.ccafs.cgiar.org/) may be crucial to supporting risk-informed adaptation planning while successes and lessons learnt inform efficiency in future work. Outreach, meanwhile, helps leverage investments. The high upfront costs of CSA make funding an integral part of scalability. Establishing clear links from local needs to national or regional strategic directives can help bring funding to the local level. Technical competency through capacity building is also indispensable to ensure that available funding is utilized in evidence-based programming at the appropriate scale. Communication may be improved by: (1) strengthening the communication capacity of national programming teams; (2) making further use of information communication technology (ICT), including local communication channels and context specific program information dissemination (Etwire et al., 2017). This work has generated 32 publications, including peer-reviewed articles, manuals, occasional papers, info notes, a special issue, and more.
In some cases, these publications have ultimately informed scalable CSA projects. For example, evidence from our work at the regional level catalyzed the European Union-funded Projet d'Appui à la Résilience Climatique pour un Développement Agricole Durable (PARC-DAD) and the World Bank-funded Projet d'Appui à l'Agriculture Sensible aux Risques Climatiques (PASEC). Our results also helped bring about the UTZ/Rainforest Alliance-funded Consultancy in view of developing a training curriculum on climate-smart agriculture for small cocoa farmers in Côte d'Ivoire. Further, the Conseil Ouest et Centre Africain pour la Recherche et le Développement Agricole (CORAF), funded under the West Africa Agricultural Productivity Program (WAAPP), a project on Capacitating Stakeholders in Using Climate Information for Enhanced Resilience in the Agricultural Sector in West Africa (CaSCIERA-TA). Based on the CaSCIERA-TA experience, the Benin team has expanded its work to other parts of the country and Burkina Faso's Meteo service has developed its independent project on Strengthening national capacities for Early Warning System (EWS) Service Delivery in Burkina Faso. Similar scaling up initiatives are ongoing at both national and regional levels across the region.
Policy support is needed for such initiatives to spread at lager scale (Raile et al., 2019), and in many cases a clear link between field action achievements and the national policy is lacking. Synthesizing the results of in-field PAR in a format that speaks to policymakers can help ameliorate this issue. We found that multi-stakeholder national science-policy dialogue platforms on CSA at the regional level helped filling this gap. These dialogue platforms used scientific evidence to create awareness of climate change impacts on agriculture and to advocate for CSA development plans (Zougmoré et al., 2016; Partey et al., 2018). Compendia of national agricultural policies as well as a plan, program, or strategy for each country were developed based on desk reviews and meetings with decision-makers. Similarly, catalogs of CSA options were produced that help farmers adapt to climate change and variability. These two actions targeted relevant development planning for climate change and CSA mainstreaming toward agricultural policy or investment initiatives (Zougmoré et al., 2019).
Discussion
The need to adapt agriculture to climate change and mitigate its effects in West Africa is apparent (Parkes et al., 2018; Sultan et al., 2019). This effort should focus on crop suitability areas and CSA management practices (Schroth et al., 2016; Egbebiyi et al., 2019; M'Bo et al., 2019; Mechiche-Alami and Abdi, 2020). Climate-smart agriculture is not a silver bullet; rather, what constitutes CSA for any given area is anchored in a thorough analysis of the unique socioeconomic and biophysical vulnerabilities of that region, including gender-responsive solutions (Carr et al., 2020; Gumucio et al., 2020). Identification of potential practices followed by community co-testing for best fit to their situations and needs enhances the success of CSA (Bayala et al., 2016; M'Bo et al., 2019).
The most promising CSA technologies and practices in West Africa that emerged from testing include agroforestry (forest management and natural resources); soil and water conservation technologies (Zaï, half-moon, tie and contour ridges, and conservation agriculture); improved crop varieties; and CIS (Sanou et al., 2016; Bayala et al., 2017; Buah et al., 2017; Etwire et al., 2017; Nkegbe et al., 2017; Ouédraogo et al., 2017b, 2018; Sanogo et al., 2017; Traore et al., 2017; Partey et al., 2018). New practices have also come to light, such as bio-fortified crop varieties, water basins for supplemental irrigation, Sahelian bocage, cocoa-agroforestry, integrated tree-crop-livestock systems, high-yielding and drought-tolerant seeds, agriculture insurance, and the use of CI for farm planning and other livelihood activities (Savadogo et al., 2011; Terre Verte, 2011; Zougmoré et al., 2014, 2016; CIAT, BFS/USAID, 2016; Zongo, 2016; FAO/ICRISAT/CIAT, 2018). Both the old and new practices constitute opportunities for farmers and production systems to adapt to and/or mitigate climate-related risks (Partey et al., 2018).
Based on the various crops, practices, and products targeted in the portfolios of each country (Bayala et al., 2016, 2018a, 2020; ICRAF, 2016; Andrieu et al., 2017), it is evident that on-farm diversification has been a clear focus for farmers to adapt to climate variability and change. Diversification aims to produce food for consumption and for income generation, with a focus on income generating activities for women, including market gardening, non-timber forest product processing, and raising small ruminants and poultry (Bayala et al., 2016, 2017). These diversified farm systems act as a safety net for farmers against both market pressures and climate risks (Ouédraogo et al., 2017a; van Zonneveld et al., 2020).
Regional CSA alliances in West Africa, such as the West African Alliance for Climate-Smart Agriculture (WAACSA) launched in Bamako, Mali in June 2015, could help identify capacity building needs that can be addressed within the alliance (Bayala et al., 2020). These regional alliances could also support rural development ministries in developing national and/or sub-national CSA profiles and investment plans. This has already started in some countries in the region like Cote d'Ivoire, Senegal, and others (CIAT, BFS/USAID, 2016; FAO/ICRISAT/CIAT, 2018). The WAACSA specifically intends to integrate adaptation measures within a sustainable agricultural system (ECOWAS, 2017; Mechiche-Alami and Abdi, 2020). Adding regional coordination to this agenda would also support the many transboundary agricultural and climate issues that require coordinated regional action.
Despite real advances, serious barriers to implementing CSA in the region remain. Current weather station networks for generating accurate information at scale are restricted (Hansen et al., 2011; Traore et al., 2014), and there is a lack of global reporting networks integrated with satellite observation to generate accurate CI at scale and on time based on the context-specific needs of the end users (Malhi et al., 2013; Hansen et al., 2019). There is also a need for appropriate packaging of the technologies that underpin CSA practices for wider on-farm adoption and for managing the perceived trade-offs between farmers and policymakers. Additional research on the biophysical adaptation of CSA practices in marginal agroecological systems and the institutional support to scale CSA from farm to landscape and country levels are also key. Further barriers include insufficient staff capacity for relevant CI advisory delivery, limited theoretical knowledge, and a lack of supportive policy and financing; the private sector has an important role in some of these areas, although their involvement generally remains low (Etwire et al., 2017). Bankable business cases and a conducive policy environment, particularly in early CSA investment plans, could help resolve this bottleneck (FAO/ICRISAT/CIAT, 2018; Raile et al., 2019). The development and implementation of effective risk-sharing mechanisms would further support integrating CSA into existing policy frameworks (Campbell et al., 2014; Zougmoré et al., 2014; Bayala et al., 2017; Partey et al., 2018).
Participatory Action Research being inclusive, it lays the ground to consider the interests of all categories of actors at the community level. By so doing, it helps addressing the barriers around the institutional environment, the policy, the governance, and the power relations for more equity at local level even though this still needs to be connected with upper subnational and national levels (Partey et al., 2018; Zougmoré et al. 2018; Eriksen et al., 2019). Participatory Action Research is also helping a lot in the peer-to-peer connection paving the way for more active social learning that our team has embraced as scaling up mechanism (Somda et al., 2020). Finally, the social learning associated with the participation in field actions, learning by doing, constitutes the best way for capacitating actors with new skills while taking into consideration their local knowledge in a co-design process (Bayala et al., 2016).
Scalability is a critical area of improvement toward implementing CSA. In the present experience, scalability was addressed through a combination of approaches that included KAP changes, social learning, and mainstreaming principles into ongoing projects and programs (Ouédraogo et al., 2019; Bayala et al., 2020; Somda et al., 2020). This program went beyond pilot sites by expanding to scaling sites while sustaining KAP changes to improve numerous farmers' livelihoods (Koerner et al., 2020). Capacity building of key stakeholders through PAR in NARES, formal trainings, and evaluation of the climate-smart potential of ongoing projects or programs proved key to sustainable scaling (Ouédraogo et al., 2019; Bayala et al., 2020). Partnership, engagement, and the views of end users were equally important to scaling. This high degree of engagement and learning led some participants to develop their own projects and to expand these approaches and principles in other communities. Theories of change often omit or ignore vital intermediate steps needed to produce the desired results, including the time required for social learning and the impacts on people's lives (Somda et al., 2020). This has led to an attribution gap, particularly for changes in the environment for which we still have yet to develop suitable approaches for evaluation.
Anticipating and managing year-to-year production fluctuations require yield prediction tools; SARRA-O/H models have been developed to serve that cause (Traore et al., 2014). By combining satellite and field experiment data, these models allow extrapolation at a regional scale. Combining MODIS NDVI and LST variables to simulate SARRA-O/H has produced yield estimations close to official agricultural statistics for 11 years (2000–2011) in Niger (Leroux et al., 2016). This is an area that deserves further efforts.
Our results demonstrate the instrumentality of national science-policy dialogue platforms in mainstreaming CSA into agricultural development strategies, plans, and policies (Robinson and Crane, 2016). Institutionalized, multi-way platforms with credible communication products would enable countries to bring heightened stability to their agricultural sector and national food security while also reducing GHG emissions in accordance with each country's Nationally Determined Contributions (NDCs) (Zougmoré et al., 2019). The generation of evidence-based information on climate impacts and CSA practices would help effect this change.
Conclusion
Testing AR4D CSA in West Africa using PAR proved to deliver tangible outputs at the technical level by linking new techniques to their appropriate uses. Context-specific agricultural technologies and practices were tested and proven adaptable to both climatic and socioecological circumstances. Scalable actions were undertaken based on these findings and used in additional regional projects and programs. There are several favorable parameters for CSA expansion, including, but not limited to, engaging youth, experienced stakeholders, and CSA alliances in the region. These factors could help accelerate large-scale promotion and adoption of CSA practices for both West Africa's dry and humid areas.
Author Contributions
Design of the approach: JB, CK-D, SD, JS, AA, MO, and RZ. Coordinating the implementation of activities at country level: SD, AB, JB, DS, AT, and KT. Regional Supervision: JB, CK-D, SD, JS, MO, AC, RZ, and TR. Manuscript draft: JB. Final manuscript: JB, CK-D, AC, and TR. All authors contributed to the article and approved the submitted version.
Funding
The work reported in the paper has been supported by the following projects: Building Resilient Agro-sylvo-pastoral Systems in West Africa through Participatory Action Research (BRAS-PAR), Partnerships for Scaling Climate-Smart Agriculture (P4S) Phase II and Capacitating African Stakeholders with Climate Advisories and Insurance Development I & II. This work was funded by the CGIAR Research Program on Climate Change, Agriculture and Food Security (CCAFS), which is a strategic partnership of CGIAR and Future Earth, led by the International Center for Tropical Agriculture (CIAT). The Program is carried out with funding by CGIAR Fund Donors, the Danish International Development Agency (DANIDA), the Australian Government (ACIAR), Irish Aid, Environment Canada, the Ministry of Foreign Affairs for the Netherlands, the Swiss Agency for Development and Cooperation (SDC), Instituto de Investigação Científica Tropical (IICT), UK Aid, the Government of Russia, the European Union (EU), the New Zealand Ministry of Foreign Affairs and Trade, with technical support from the International Fund for Agricultural Development (IFAD).
Conflict of Interest
The authors declare that the research was conducted in the absence of any commercial or financial relationships that could be construed as a potential conflict of interest.
Acknowledgments
Colleagues from national research institutions—Institut de l'Environnement et de Recherches Agricoles (INERA) of Burkina Faso, Institut National de la Recherche Agronomique (INRAN) of Niger, Institut Sénégalais de Recherche Agricole (ISRA) of Senegal, and Savanna Agricultural Research Institute (SARI) of Ghana, Institut d'Economie Rurale (IER) of Mali—also generously shared their documents and data with us. Colleagues who have produced the illustrations are acknowledged.
References
Aggarwal, P. K., Jarvis, A, Campbell, B. M, Zougmoré, R. B., Khatri-Chhetri, A., Vermeulen, S. J., et al. (2018). The climate-smart village approach: framework of an integrative strategy for scaling up adaptation options in agriculture. Ecol. Soc. 23:14. doi: 10.5751/ES-09844-230114
Alexandratos, N., and Bruinsma, J. (2012). World Agriculture Towards 2030/2050: The 2012 Revision. ESA Working Paper No. 12-03. Rome: Food and Agriculture Organization of the United Nations. Available online at: http://www.fao.org/docrep/016/ap106e/ap106e.pdf (accessed November 6, 2013).
Allen, T., Heinrigs, P., and Heo, I. (2018). Agriculture, Food and Jobs in West Africa. West African Papers, N°14. Paris: OECD Publishing.
Andrieu, N., Sogoba, B., Zougmoré, R., Howland, F., Samake, O., Bonilla-Findji, O., et al. (2017). Prioritizing investments for climate-smart agriculture: lessons learned from Mali. Agric. Syst. 154, 13–24. doi: 10.1016/j.agsy.2017.02.008
Bationo, A., Hartemink, A., Lungu, O., Naimi, M., Okoth, P., Smaling, E., et al. (2006). African soils: their productivity and profitability of fertilizer use, in Background Paper Prepared for the African Fertilizer Summit, Abuja, Nigeria - June 9–13, 2006 (Abuja).
Bayala, J., Dayamba, S. D., Ayantunde, A. A., Somda, J., Ky-Dembele, C., Bationo, B. A., et al. (2018a). Methodological Guide: Community Participatory Inventory and Prioritization of Climate Smart Crop-Livestock-Agroforestry Technologies/Practices. ICRAF Technical Manual. Nairobi: World Agroforestry Centre.
Bayala, J., Dayamba, S. D., Ky-Dembele, C., Savadogo, P., and Arinloye, D. A. (2018b). Manuelle de Vulgarisation sur L'agriculture Intelligente Face au Climat. Manuel Technique ICRAF. Nairobi: World Agroforestry Centre.
Bayala, J., Dayamba, S. D., Lamien, N., Zougmoré, R. B., Agali, A., Ky-Dembélé, C., et al. (2020). Capacitating Stakeholders to Using Climate Information in West Africa: Achievements and Lessons Learned from the WAAPP-Funded CaSCIERA-TA Project. CCAFS Info Note. Wageningen: CGIAR Research Program on Climate Change, Agriculture and Food Security (CCAFS).
Bayala, J., Zougmoré, R., Dayamba, S. D., and Olivier, A. (2017). Climate-smart agriculture technologies in West Africa: learning from the ground research for development experiences. Agric. Food Secur. 6:40. doi: 10.1186/s40066-017-0117-5
Bayala, J., Zougmoré, R., Ky-Dembele, C., Bationo, B. A., Buah, S., Sanogo, D., et al. (2016). Towards Developing Scalable Climate-Smart Village Models: Approach and Lessons Learnt From Pilot Research in West Africa. ICRAF Occasional Paper No. 25. Nairobi: World Agroforestry Centre doi: 10.5716/WP16051.PDF
Bodirsky, B. L., Rolinski, S., Biewald, A., Weindl, I., Popp, A., and Lotze-Campen, H. (2015). Global food demand scenarios for the 21st century. PLoS ONE 10:e0139201. doi: 10.1371/journal.pone.0139201
Bonilla-Findji, O., Ouédraogo, M., Partey, S. T., Dayamba, S. D., Bayala, J., and Zougmoré, R. (2018). West Africa Climate-Smart Villages AR4D Sites: 2017 Inventory. Wageningen: CGIAR Research Program on Climate Change, Agriculture and Food Security (CCAFS). Available online at: www.ccafs.cgiar.org
Buah, S. S. J., Ibrahim, H., Derigubah, M., Kuzie, M., Segtaa, J. V., Bayala, J., et al. (2017). Tillage and fertilizer effect on maize and soybean yields in the Guinea Savanna Zone of Ghana. Agric. Food Secur. 6:17. doi: 10.1186/s40066-017-0094-8
Campbell, B. M., Thornton, P., Zougmoré, R., van Asten, P., and Lipper, L. (2014). Sustainable intensification: what is its role in climate smart agriculture? Curr. Opin. Environ. Sustain. 8, 39–43. doi: 10.1016/j.cosust.2014.07.002
Carr, E. R., Goble, R., Rosko, H. M., Vaughan, C., and Hansen, J. (2020). Identifying climate information services users and their needs in Sub-Saharan Africa: a review and learning agenda. Clim. Dev. 12, 23–41. doi: 10.1080/17565529.2019.1596061
CCAFS/ICRAF (2020). Evidence for Resilient Agriculture (ERA) - Data on the Performance of Agricultural Technologies Accessible for Development Decisions. Available online at: http://era.ccafs.cgiar.org
Chao, Q., and Feng, A. (2018). Scientific basis of climate change and its response. GEI 1, 420–427. doi: 10.14171/j.2096-5117.gei.0.2018.04.002
CIAT, BFS/USAID. (2016). Climate-Smart Agriculture in Senegal. CSA Country Profiles for Africa Series. International Center for Tropical Agriculture (CIAT), Bureau for Food Security, United States Agency for International Development (BFS/USAID), Washington, DC, p. 20.
Clarkson, G., Dorward, P., Osbahr, H., Torgbor, F., and Kankam-Boadu, I. (2019). An investigation of the effects of PICSA on smallholder farmers' decision-making and livelihoods when implemented at large scale - the case of Northern Ghana. Clim. Serv. 14, 1–14. doi: 10.1016/j.cliser.2019.02.002
Connolly-Boutin, L., and Smit, B. (2016). Climate change, food security, and livelihoods in sub-Saharan Africa. Reg. Environ. Change 16, 385–399. doi: 10.1007/s10113-015-0761-x
Cook, J., Oreskes, N., Doran, P. T., Anderegg, W. R. L., Verheggen, B., Maibach, E. D., et al. (2016). Consensus on consensus: a synthesis of consensus estimates on human-caused global warming. Environ. Res. Lett. 11:048002. doi: 10.1088/1748-9326/11/4/048002
Costantini, E. A. C., Branquinho, C., Nunes, A., Schwilch, G., Stavi, I., Valdecantos, A., et al. (2016). Soil indicators to assess the effectiveness of restoration strategies in dryland ecosystems. Solid Earth 7, 397–414. doi: 10.5194/se-7-397-2016
Davies, R., and Dart, J. (2005). The “Most Significant Change” (MSC) Technique. A Guide to Its Use. Available online at: http://www.mande.co.uk/docs/MSCGuide.htm
Dayamba, S. D., Ky-Dembele, C., Bayala, J., Dorward, P., Clarkson, D., Sanogo, D., et al. (2018). Assessment of the use of Participatory Integrated Climate Services for Agriculture (PICSA) approach by farmers to manage climate risk in Mali and Senegal. Clim. Serv. 12, 27–35. doi: 10.1016/j.cliser.2018.07.003
Diedhiou, A., Bichet, A., Wartenburger, R., Seneviratne, S. I., Rowell, D. P., Sylla, M. B., et al. (2018). Changes in climate extremes over West and Central Africa at 1.5°C and 2°C global warming. Environ. Res. Lett. 13:065020. doi: 10.1088/1748-9326/aac3e5
Dinku, T., Hansen, J., Rose, A., Damen, B., and Sheinkman, M. (2018a). Enhancing National Climate Services (ENACTS) Approach to Support Climate Resilience in Agriculture. Improving Availability, Access, and Use of Historical Climate Information. InfoNote.
Dinku, T., Thomson, M. C., Cousin, R., del Corral, J., Ceccato, P., Hansen, J., et al. (2018b). Enhancing National Climate Services (ENACTS) for development in Africa. Clim. Dev. 10, 664–672. doi: 10.1080/17565529.2017.1405784
Dorward, P., Clarkson, G., and Stern, R. (2015). Participatory Integrated Climate Services for Agriculture (PICSA): Field Manual. Reading: Walker Institute, University of Reading.
ECOWAS (2017). 2025 Strategic Policy Framework. Abuja: ECOWAS Department of Agriculture, Environment and Water Resources.
Egbebiyi, T. S., Crespo, O., and Lennard, C. (2019). Defining crop-climate departure in West Africa: improved understanding of the timing of future changes in crop suitability. Climate 7:101. doi: 10.3390/cli7090101
Eriksen, S. H., Cramer, L. K., Vetrhus, I., and Thornton, P. (2019). Can Climate Interventions Open Up Space for Transformation? Examining the Case of Climate-Smart Agriculture (CSA) in Uganda. Front. Sustain. Food Syst. 3:111. doi: 10.3389/fsufs.2019.00111
Etwire, P. M., Buah, S., Ouédraogo, M., Zougmoré, R., Partey, S. T., Martey, E., et al. (2017). An assessment of mobile phone-based dissemination of weather and market information in the Upper West Region of Ghana. Agric. Food Secur. 6:8. doi: 10.1186/s40066-016-0088-y
FAO (2013). Climate-Smart Agriculture Sourcebook.. Rome: Food and Agriculture Organization of the United Nations.
FAO/ICRISAT/CIAT (2018). Climate-Smart Agriculture in Côte d'Ivoire. CSA Country Profiles for Africa Series. International Center for Tropical Agriculture (CIAT); International Crops Research Institute for the Semi-Arid Tropics (ICRISAT); Food and Agriculture Organization of the United Nations (FAO). Rome, Italy. p. 23.
Fossey, M., Angers, D., Bustany, C., Cudennec, C., Durand, P., Gascuel-Odoux, C., et al. (2020). A framework to consider soil ecosystem services in territorial planning. Front. Environ. Sci. 8:28. doi: 10.3389/fenvs.2020.00028
Gumucio, T., Hansen, J., Huyer, S., and van Huysen, T. (2020). Gender-responsive rural climate services: a review of the literature. Clim. Dev. 12, 241–254. doi: 10.1080/17565529.2019.1613216
Hansen, J. W., Mason, S. J., Sun, L., and Tall, A. (2011). Review of seasonal climate forecasting for agriculture in sub-saharan Africa. Expl. Agric. 47, 205–240. doi: 10.1017/S0014479710000876
Hansen, J. W., Vaughan, C., Kagabo, D. M., Dinku, T., Carr, E. R., Körner, J., et al. (2019). Climate services can support african farmers' context-specific adaptation needs at scale. Front. Sustain. Food Syst. 3:21. doi: 10.3389/fsufs.2019.00021
Hunter, M. C., Smith, R. G., Schipanski, M. E., Atwood, L. W., and Mortensen, D. A. (2017). Agriculture in 2050: recalibrating targets for sustainable intensification. BioScience 67, 386–391. doi: 10.1093/biosci/bix010
ICRAF (2016). Promising Climate-Smart Crop-Livestock-Agroforestry Technologies/Practices in CCAFS Benchmark Sites in West-Africa: Inventory and Prioritization Workshops Report. A Synthesis made by ICRAF-WCA Sahel Based on Countries' Reports. Bamako: ICRAF.
ICRAF (2019). Delivering Climate Information to Rural Households: Forecasts and Capacity Building. CSA@ICRAF Brief.
Jalloh, A., Roy-Macauley, H., and Sereme, P. (2012). Major agro-ecosystems of West and Central Africa: brief description, species richness, management, environmental limitations and concerns. Agric. Ecosyst. Environ. 157, 5–16. doi: 10.1016/j.agee.2011.11.019
Jayne, T. S., Chamberlin, J., and Headey, D. D. (2014). Land pressures, the evolution of farming systems, and development strategies in Africa: a synthesis. Food Policy 48, 1–17. doi: 10.1016/j.foodpol.2014.05.014
Klutse, N. A. B., Sylla, M., Diallo, I., Sarr, A., Dosio, A., Diedhiou, A., et al. (2016). Daily characteristics of West African summer monsoon precipitation in CORDEX simulations. Theor. Appl. Climatol. 123, 369–386. doi: 10.1007/s00704-014-1352-3
Koerner, J., Theissen, A. H., Loboguerrero, A. M., and Campbell, B. (2020). The Scaling Mindset – Shifting from Problems to Solutions. Insights from the Review of CCAFS Scaling Activities, 2019. CCAFS Working Paper no. 300. Wageningen: CGIAR Research Program on Climate Change, Agriculture and Food Security (CCAFS).
Kotir, J. H. (2011). Climate change and variability in Sub-Saharan Africa: a review of current and future trends and impacts on agriculture and food security. Environ. Dev. Sustain. 13, 587–605. doi: 10.1007/s10668-010-9278-0
Leroux, L., Baron, C., Zoungrana, B., Traoré, S. B., Lo Seen, D., and Bégué, A. (2016). Crop monitoring using vegetation and thermal indices for yield estimates: case study of a rainfed cereal in semi-arid West Africa. IEEE J. Sel. Top. Appl. Earth Obs. Remote Sens. 9, 347–362. doi: 10.1109/JSTARS.2015.2501343
Malhi, Y., Adu-Bredu, S., Asare, R. A., Lewis, S. L., and Mayaux, P. (2013). African rainforests: past, present and future. Philos. Trans. R. Soc. B. 368:20120312. doi: 10.1098/rstb.2012.0312
M'Bo, K. A., Kouassi, A., Amani, K., Degrande, A., Bayala, J., and Kouame, C. (2019). Recommandations Adaptées au Climat pour les Régions Cacaoyères de la Côte d'Ivoire. Etude en vue de Développer un Programme de Formation sur les Meilleures Pratiques en Matière de Production Cacaoyère Tenant Compte du Climat en Côte d'Ivoire. Rapport, World Agroforestry, ICRAF-West and Central Africa and Rainforest Alliance, Abidjan, Côte d'Ivoire. P. 81.
Mechiche-Alami, A., and Abdi, A. M. (2020). Agricultural productivity in relation to climate and cropland management in West Africa. Sci. Rep. 10:3393. doi: 10.1038/s41598-020-59943-y
Nkegbe, P. K., Abu, B. M., and Issahaku, H. (2017). Food security in Savannah Accelerated Development Authority Zone of Ghana: an ordered probit with household hunger scale approach. Agric. Food Secur. 6:35. doi: 10.1186/s40066-017-0111-y
Oreskes, N. (2018). The scientific consensus on climate change: how do we know we're not wrong?, in Climate Modelling, eds Lloyd, A., and Winsberg, E., (Cham: Palgrave Macmillan), 31–64. doi: 10.1007/978-3-319-65058-6_2
Ouédraogo, M., Barry, S., Zougmoré, R. B., Partey, S. T., and Somé, L. (2018) Farmers' Willingness to Pay for Climate Information Services: evidence from Cowpea Sesame Producers in Northern Burkina Faso. Sustainability 10:611. doi: 10.3390/su10030611.
Ouédraogo, M., Partey, S. T., Zougmoré, R. B., Nuyor, A. B., Zakari, S., and Traoré, K. B. (2018). Uptake of Climate-Smart Agriculture in West Africa: What can we learn from Climate-Smart Villages of Ghana, Mali and Niger? CCAFS Info Note. Bamako, Mali: CGIAR Research Program on Climate Change, Agriculture and Food Security (CCAFS). Availalble online at: https://hdl.handle.net/10568/93351
Ouédraogo, M., Zougmoré, R., Moussa, S. A., Partey, T. S., Thornton, K. P., Kristjanson, P., et al (2017a) Markets climate are driving rapid change in farming practices in Savannah West Africa. Reg. Environ. Chang. 17, 437–449. doi: 10.1007/s10113-016-1029-9.
Ouédraogo, M., Zougmoré, R. B., Houessionon, P., Gnangle, G., Nadjiam, D., Diaby, M. F., et al. (2019). Assessing the Climate-Smartness of the West Africa Agricultural Productivity Programme (WAAPP): What can we learn from Benin, Guinea, Niger, Togo and Chad projects? Findings from participatory assessments of the climate-smartness of the World Bank funded “West Africa Agricultural Productivity Programme (WAAPP)”.
Ouédraogo, P., Bationo, B. A., Sanou, J., Traore, S., Barry, S., Dayamba, S. D., et al. (2017b). Uses and vulnerability of ligneous species exploited by local population of northern Burkina Faso in their adaptation strategies to changing environments. Agric. Food Secur. 6:15 doi: 10.1186/s40066-017-0090-z
Parkes, B., Defrance, D., Sultan, B., Ciais, P., and Wang, X. H. (2018). Projected changes in crop yield mean and variability over West Africa in a world 1.5K warmer than the pre-industrial era. Earth Syst. Dynam. 9, 119–134. doi: 10.5194/esd-9-119-2018
Partey, S. T., Zougmoré, R. B., Ouédraogo, M., and Campbell, B. M. (2018). Developing climate-smart agriculture to face climate variability in West Africa: challenges and lessons learnt. J. Clean. Prod. 187, 285–295. doi: 10.1016/j.jclepro.2018.03.199
Pieri, C. (1989). Fertilité des Terres de Savannes. Bilan de Trente Ans de Recherche et de Développement Agricoles au Sud du Sahara. Ministère de la Coopération. Paris: CIRAD/IRAT.
Pomati, M., and Nandy, S. (2019). Assessing progress towards SDG2: trends and patterns of multiple malnutrition in young children under 5 in West and Central Africa. Child. Ind. Res. 13, 1847–1873. doi: 10.1007/s12187-019-09671-1
Raile, E. R., Young, L. M., Sarr, A., Mbaye, S., Raile, A. N. W., Wooldridge, L., et al. (2019). “Political will and public will for climate-smart agriculture in Senegal: opportunities for agricultural transformation”. J. Agribusiness Dev. Emerg. Econ. 9, 44–62. doi: 10.1108/JADEE-01-2018-0003
Robinson, L. W., and Crane, T. A. (2016). Conceptual Framework for Analysing Science-Policy Interactions for Improved Climate Policy. ILRI Project Report. Nairobi: International Livestock Research Institute (ILRI).
Sanogo, D., Dayamba, D. S., Ouédraogo, M., Zougmoré, R., Bayala, J., Ndiaye, O., et al. (2016). Climate-Smart Village Models: What Research and Insights from Current Implementation in Daga Birame CSV in Senegal? Case Study of Daga-Birame CSV for CCAFS ISP11/6.1.2 - Senegal.
Sanogo, D., Ndour, B. Y., Sall, M., Toure, K., Diop, M., Camara, B. A., et al. (2017). Participatory diagnosis and development of climate change adaptive capacity in the groundnut Basin of Senegal: building a climate-smart village model. Agric. Food Secur 6:13. doi: 10.1186/s40066-017-0091-y
Sanou, J., Bationo, B. A., Barry, S., Nabie, L. D., Bayala, J., Zougmoré, R., et al. (2016). Combining soil fertilization, cropping systems and improved varieties to minimize climate risks on farming productivity in northern region of Burkina Faso. Agric. Food Secur. 5:20. doi: 10.1186/s40066-016-0067-3
Savadogo, M., Somda, J., Seynou, O., Zabré, S., and Nianogo, A. J. (2011). Catalogue des Bonnes Pratiques D'adaptation aux Risques Climatiques au Burkina Faso. Ouagadougou: UICN Burkina Faso. p. 52.
Schroth, G., Läderach, P., Martinez-Valle, A., Bunn, C., and Jassogne, L. (2016). Vulnerability to climate change of cocoa in West Africa: patterns, opportunities and limits to adaptation. Sci. Total Environ. 556, 231–241. doi: 10.1016/j.scitotenv.2016.03.024
Serdeczny, O., Adams, S., Baarsch, F., Coumou, D., Robinson, A., Hare, W., et al. (2017). Climate change impacts in sub-Saharan Africa: from physical changes to their social repercussions. Reg. Environ. Change 17, 1585–1600. doi: 10.1007/s10113-015-0910-2
Somda, J., Faye, A., and N'Djafa Ouaga, H. (2011). Handbook and User Guide of the Toolkit for Planning, Monitoring and Evaluation of Climate Change Adaptive Capacities. Niamey: AGRHYMET Regional Centre. p. 84.
Somda, J., Ouédraogo, R., Buah, S., Barry, S., Bationo, B. A., Bayala, J., et al (2020). Scaling Climate-Smart Agriculture: Linking Participatory Action Research to Social Learning. CCAFS Blog. Available online at: https://ccafs.cgiar.org/research-highlight/scaling-climate-smart-agriculture-linking-participatory-action-research-social#.Xtkqbi2cbyu
Somda, J., Sawadogo, I., Savadogo, M., Zougmoré, R., Bationo, B. A., Moussa, A. S., et al (2014). Participatory Vulnerability Assessment and Planning of Adaptation to Climate Change in the Yatenga, Burkina Faso. CGIAR Challenge Program on Climate Change, Agriculture and Food Security. Available online at: www.ccafs.cgiar.org
Somda, J., Zougmoré, R., Sawadogo, I., Bationo, B. A., and Partey, T. S. (2016). Setting Partnership for Climate Change Adaptation in the CCAFS Yatenga Site, Burkina Faso: Analysis of Gaps and Opportunities. CCAFS Working Paper no. 176. Copenhagen, Denmark: CGIAR Research Program on Climate Change, Agriculture and Food Security (CCAFS). Available online at: www.ccafs.cgiar.org
Sultan, B., Defrance, D., and Lizumi, T. (2019). Evidence of crop production losses in West Africa due to historical global warming in two crop models. Sci. Rep. 9:12834. doi: 10.1038/s41598-019-49167-0
Sultan, B., and Gaetani, M. (2016). Agriculture in West Africa in the twenty-first century: climate change and impacts scenarios, and potential for adaptation. Front. Plant Sci. 7:1262. doi: 10.3389/fpls.2016.01262
Taylor, C., Belušić, D., Guichard, F., Parker, D. J., Vischel, T., Bock, O., et al. (2017). Frequency of extreme Sahelian storms tripled since 1982 in satellite observations. Nature 544, 475–478. doi: 10.1038/nature22069
Terre Verte (2011). Réalisation d'un Périmètre Bocager au Sahel – Cahier Technique. Manuel Technique. Ouagadougou, Burkina Faso.
Tilman, D., Balzer, C., Hill, J., and Befort, B. L. (2011). Global food demand and the sustainable intensification of agriculture. Proc. Natl. Acad. Sci. U.S.A. 108, 20260–20264. doi: 10.1073/pnas.1116437108
Traore, K., Sidibe, D. K., Coulibaly, H., and Bayala, J. (2017). Optimizing yield of improved varieties of millet and sorghum under highly variable rainfall conditions using contour ridges in Cinzana, Mali. Agric. Food Secur. 6:11. doi: 10.1186/s40066-016-0086-0
Traore, S. B., Alhassane, A., Muller, B., Kouressy, M., Some, L., Sultan, B., et al. (2011). Characterizing and modeling the diversity of cropping situations under climatic constraints in West Africa. Atmos. Sci. Lett. 12, 89–95. doi: 10.1002/asl.295
Traore, S. B., Ali, A., Tinni, S. H., Samake, M., Garba, I., Maigari, I., et al. (2014). AGRHYMET: a drought monitoring and capacity building center in the West Africa Region. Weather. Clim. Extremes 3, 22–30. doi: 10.1016/j.wace.2014.03.008
Trenberth, K. E. (2018). Climate change caused by human activities is happening and it already has major consequences. J. Energy Nat. Resour. Law 36, 463–481. doi: 10.1080/02646811.2018.1450895
van Zonneveld, M., Turmel, M.-S., and Hellin, J. (2020). Decision-making to diversify farm systems for climate change adaptation. Front. Sustain. Food Syst. 4:32. doi: 10.3389/fsufs.2020.00032
Vintrou, E., Bégué, A., Baron, C., Saad, A., Seen, D. L., and Traoré, S. B. (2014). A comparative study on satellite- and model-based crop phenology in West Africa. Remote Sens. 6, 1367–1389. doi: 10.3390/rs6021367
Wuebbles, D. J., Easterling, D. R., Hayhoe, K., Knutson, T., Kopp, R. E., and Kossin, J. P. (2017), Our globally changing climate, in Climate Science Special Report: Fourth National Climate Assessment, Vol. I, eds. Wuebbles, D. J., Fahey, D. W., Hibbard, K. A., Dokken, D. J., Stewart, B. C., and Maycock, T. K., (Washington, DC: U.S. Global Change Research Program), 35–72. doi: 10.7930/J08S4N35.
Zongo, B. (2016). Stratégies Innovantes D'adaptation à la Variabilité et au Changement Climatiques au Sahel: Cas de L'irrigation de Complément et de L'information Climatique Dans les Exploitations Agricoles du Burkina Faso. Thèse en Cotutelle en Sciences Agronomiques et Ingénierie Biologique de l'Université de Liège, Gembloux Agro-Bio Tech (ULg - GxABT) en Belgique et Science et Technologie de l'Eau, de l'Energie et de l'Environnement de l'Institut International d'Ingénierie de l'Eau et de l'Environnement (2iE) de Ouagadougou au Burkina Faso.
Zougmoré, R., Jalloh, A., and Tioro, A. (2014). Climate-smart soil water and nutrient management options in semiarid West Africa: a review of evidence and analysis of stone bunds and Zaï techniques. Agric. Food Secur. 3:16. doi: 10.1186/2048-7010-3-16
Zougmoré, R., Partey, S., Ouédraogo, M., Omitoyin, B., Thomas, T., Ayantunde, A., et al. (2016). Toward climate-smart agriculture in West Africa: a review of climate change impacts, adaptation strategies and policy developments for the livestock, fishery and crop production sectors. Agric. Food Secur. 5:26. doi: 10.1186/s40066-016-0075-3
Zougmoré, R.B., Partey, S.T., Ouédraogo, M., Torquebiau, E., and Campbell, B.M. (2018). Facing climate variability in Sub-Saharan Africa: Analysis of climatesmart agriculture opportunities to manage climate-related risks. Cahi. Agric. 27:34001. doi: 10.1051/cagri/2018019
Zougmoré, R. B., Partey, S. T., Totin, E., Ouédraogo, M., Thornton, P., Karbo, N., et al. (2019). Science-policy interfaces for sustainable climate-smart agriculture uptake: lessons learnt from national science-policy dialogue platforms in West Africa. J. Agric. Sustain. 17, 367–382. doi: 10.1080/14735903.2019.1670934
Keywords: adaptation, ecosystem, farming system, mitigation, livelihood, resilience
Citation: Bayala J, Ky-Dembele C, Dayamba SD, Somda J, Ouédraogo M, Diakite A, Chabi A, Alhassane A, Bationo AB, Buah SSJ, Sanogo D, Tougiani A, Traore K, Zougmoré RB and Rosenstock TS (2021) Multi-Actors' Co-Implementation of Climate-Smart Village Approach in West Africa: Achievements and Lessons Learnt. Front. Sustain. Food Syst. 5:637007. doi: 10.3389/fsufs.2021.637007
Received: 02 December 2020; Accepted: 24 March 2021;
Published: 29 April 2021.
Edited by:
Sanzidur Rahman, Shandong University of Finance and Economics, ChinaReviewed by:
Pramod Aggarwal, CGIAR Research Program on Climate Change, Agriculture and Food Security (CCAFS), IndiaArun Khatri-Chhetri, CGIAR Research Program on Climate Change, Agriculture and Food Security (CCAFS), Netherlands
Copyright © 2021 Bayala, Ky-Dembele, Dayamba, Somda, Ouédraogo, Diakite, Chabi, Alhassane, Bationo, Buah, Sanogo, Tougiani, Traore, Zougmoré and Rosenstock. This is an open-access article distributed under the terms of the Creative Commons Attribution License (CC BY). The use, distribution or reproduction in other forums is permitted, provided the original author(s) and the copyright owner(s) are credited and that the original publication in this journal is cited, in accordance with accepted academic practice. No use, distribution or reproduction is permitted which does not comply with these terms.
*Correspondence: J. Bayala, ai5iYXlhbGFAY2dpYXIub3Jn