- 1Department of Food and Nutrition, University of Helsinki, Helsinki, Finland
- 2Helsinki Institute of Sustainability Science, Faculty of Agriculture and Forestry, University of Helsinki, Helsinki, Finland
While the world population is steadily increasing, the capacity of Earth to renew its resources is continuously declining. Consequently, the bioresources required for food production are diminishing and new approaches are needed to feed the current and future global population. In the last decades, scientists have developed novel strategies to reduce food loss and waste, improve food production, and find new ingredients, design and build new food structures, and introduce digitalization in the food system. In this work, we provide a general overview on circular economy, alternative technologies for food production such as cellular agriculture, and new sources of ingredients like microalgae, insects, and wood-derived fibers. We present a summary of the whole process of food design using creative problem-solving that fosters food innovation, and digitalization in the food sector such as artificial intelligence, augmented and virtual reality, and blockchain technology. Finally, we briefly discuss the effect of COVID-19 on the food system. This review has been written for a broad audience, covering a wide spectrum and giving insights on the most recent advances in the food science and technology area, presenting examples from both academic and industrial sides, in terms of concepts, technologies, and tools which will possibly help the world to achieve food security in the next 30 years.
Introduction
The capacity of Earth to regenerate its own resources is continuously and drastically reducing due to the exponential growth of the human population (Ehrlich and Holdren, 1971; Henderson and Loreau, 2018). Over the last 50 years, the global human population has doubled, while the Earth overshoot day—the day on which humanity has exhausted the annual renewable bioresources of the Earth—has continuously become earlier, reaching its earliest date (July 29) in 2018 and 2019. Exceptionally, the Earth overshoot day was delayed to August 22 in 2020, due to the novel Coronavirus pandemic (Global Footprint Network, 2020a) (Figure 1). However, this delay is the result of a pandemic disease and it is not the consequence of any long-term planned strategy, which is still required to improve the sustainability of our society. Bioresources are necessary to feed people. However, the production, including loss and waste of food account for 26% of the human ecological footprint (Global Footprint Network, 2020b). This is due to low efficiency in food production coupled with non-optimal waste management. By taking action and promoting sustainable behavior in the entire food chain and among consumers, the Earth overshoot day could be delayed, preserving Earth's regenerative capacity (Moore et al., 2012).
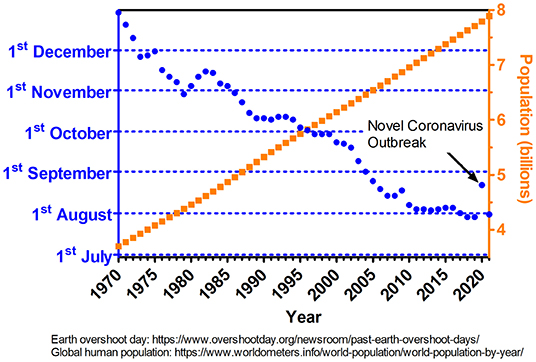
Figure 1. Earth overshoot day (blue) and global population (orange) evolution over the last 50 years.
By 2050, the population is expected to reach 9.7 billion and ensuring global food security will be a priority (Berners-Lee et al., 2018). The first step toward food security is the reduction of waste and loss of food. According to the Food and Agriculture Organization (FAO), ~1.3 billion tons of food are lost/wasted in the food chain from production to retail and by consumers annually (Wieben, 2017), which highlights the importance of the circular economy and consumer education. In addition, economic barriers should be addressed to give access to healthier and sustainable food to low-income consumers (Hirvonen et al., 2020). However, the reduction of waste and economic barriers is not enough to reach global food security. Indeed, to feed the world population of 2050, food production should increase by 70% (Floros et al., 2010). Additionally, diets should change and rely less on animal products, including more plant-, insect-, and microalgae-based products (van Huis and Oonincx, 2017; Caporgno and Mathys, 2018; Lynch et al., 2018). This change is necessary as animal-based diets are less sustainable comparatively due to their demand for more natural resources, resulting in more environmental degradation (Sabaté and Soret, 2014). Unfortunately, changing food production and consumption habits is not a straightforward process; it has to be efficient, sustainable, and economically feasible. New food products have to be nutritionally adequate, culturally and socially acceptable, economically accessible, as well as palatable. Moreover, new food products should aim to maintain or improve the health of consumers. Food science and technology can help address these problems by improving food production processes, including novel ingredients from more sustainable sources, and designing new highly-accepted food products.
However, the benefits of consuming novel and upgraded food products is not sufficient to obtain an effect on consumers. Indeed, the acceptability of, and demand for food varies around the world, based on, for example, geographic location, society structure, economy, personal income, religious constraints, and available technology. Food safety and nutritionally adequate foods (in terms of both macro- and micronutrients) are most important in low-income countries (Sasson, 2012; Bain et al., 2013), whereas medium- and high-income countries prioritize foods to reduce risk of chronic disease, and functional and environmentally friendly food (Azais-Braesco et al., 2009; Cencic and Chingwaru, 2010; Govindaraj, 2015). The concept of food has evolved from the amount of nutrients needed by a person to survive on a daily basis (Floros et al., 2010) to a tool to prevent nutrition-related diseases (e.g., non-communicable diseases: type 2 diabetes, coronary diseases, cancer, and obesity), and to improve human physical and mental well-being (Siró et al., 2008), and to slow/control aging (Rockenfeller and Madeo, 2010). Therefore, the development of new food products should consider the needs and demands of consumers. In spite of this, across countries, personal income can limit the access to sufficient food for survival, let alone new and improved food products that have extra benefits.
Coupled to this complex scenario, food demand is also constrained, and affected by human psychology (Wang et al., 2019). The naturally-occurring conservative and neophobic behavior of humans toward new food can lead to nutrition-related diseases due to poor dietary patterns already established during childhood (Perry et al., 2015) and can lead to acceptability problems related to food containing novel ingredients such as insects in Western countries (La Barbera et al., 2018). Additionally, the introduction in our diets of new food products obtained by means of novel technologies and ingredients from food waste and by-products can be undermined by low acceptability caused by human psychology (Bhatt et al., 2018; Cattaneo et al., 2018; Siegrist and Hartmann, 2020). Therefore, to increase the successful integration of the solutions discussed in this paper into the diet, consumer behavior has to be considered. Finally, it should not be forgotten that food consumption is also determined by pleasure rather than just being a merely mechanical process driven by the need for calories (Mela, 2006; Lowe and Butryn, 2007). The latter concept is particularly important when consumers are expected to change their eating habits. New food products developed using sustainable ingredients and processes should be designed to take in consideration sensorial attributes and psychological considerations, which will allow a straightforward transition to more sustainable diets.
The actions needed in the area of food to develop a sustainable society allowing the regeneration of Earth's bio-resources are several. They include changing our eating habits and dietary choices, reducing food waste and loss, preserving biodiversity, reducing the prevalence of food-related diseases, and balancing the distribution of food worldwide. To promote these actions, new ingredients and technologies are necessary (Table 1).
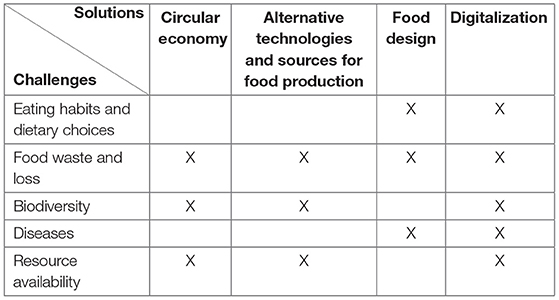
Table 1. Challenges/solutions matrix for the development of the food of the future using the most recent advances in food science and technology.
This review discusses the most recent advances in food science and technology that aim to ensure food security for the growing human population by developing the food of the future. We discuss (i) the circular economy, where food waste is valorized and enters back into the food production chain improving the sustainability of the food system and reduces Earth's biodiversity and resources loss; (ii) alternative technologies and sources for food production like cellular agriculture, algae, microalgae, insects, and wood-derived fibers, which use Earth's bioresources more efficiently; (iii) the design of food in terms of creative problem-solving that fosters food innovation allowing transition to more sustainable and nutritionally adequate diets without undermining their consumer acceptability; and (iv) digitalization in which artificial intelligence (AI), virtual reality (VR), and blockchain technology are used to better control and manage the food chain, and assist the development of novel ingredients and food, boosting the technological shift in the whole food system; (v) we also briefly discuss the effect of COVID-19 on the food supply chain, showing the need to develop a resilient food system.
Food Science and Technology Solutions for Global Food Security
The Circular Economy
The unsustainable practice of producing and consuming materials based on the linear (take-make-dispose) economic model calls for a shift toward innovative and sustainable approaches embodied in the principles of the circular economy (Jørgensen and Pedersen, 2018). In contrast to a linear economic model, where materials are produced linearly from a presumably infinite source of raw materials, the circular economy is based on closing the loop of materials and substances in the supply chain. In this model, the value of products, materials, and resources is preserved in the economy for as long as possible (Merli et al., 2018).
Integrated into the food system, the circular economy offers solutions to achieve global food sustainability by minimizing food loss and waste, promoting efficient use of natural resources and mitigating biodiversity loss (Jurgilevich et al., 2016), by retaining the resources within a loop, i.e., the resources are used in a cyclic process, reducing the demand for fresh raw materials in food production. This efficient use of natural resources for food in a circular economy, in turn, helps to rebuild biodiversity by preventing further conversion of natural habitats to agricultural land, which is one of the greatest contributors to biodiversity loss (Dudley and Alexander, 2017).
This measure is highlighted by the fact that an enormous amount of waste is generated at various stages of the food supply chain. Food loss and waste accounts for 30% of the food produced for human consumption globally, translating into an estimated economic loss of USD 1 trillion annually (FAO, 2019). Food loss and waste also takes its toll on the environment in relation to the emission of greenhouse gases associated with disposal of food waste in landfills, as well as in activities associated with the production of food such as agriculture, processing, manufacturing, transportation, storage, refrigeration, distribution, and retail (Papargyropoulou et al., 2014). The various steps in the food supply chain have an embedded greenhouse gas impact, which is exacerbated when food is wasted and lost.
Addressing the challenge of minimizing food loss and waste requires proper identification of what constitutes food loss and waste. The FAO defines food loss and waste as a decrease in the quantity or quality of food along the food supply chain (FAO, 2019). Food loss occurs along the food supply chain from harvest, slaughter, and up to, but not including, the retail level. Food waste, on the other hand, occurs at the retail and consumption level. From the FAO's definition, food that is converted for other uses such as animal feed, and inedible parts of foods, for example, bones, feathers, and peel, are not considered food loss or waste. The Waste and Resources Action Programme (Quested and Johnson, 2009), a charity based in the UK, has defined and categorized food waste as both avoidable and unavoidable. Avoidable food waste includes food that is still considered edible but was thrown away, such as vegetables or fruits that do not pass certain standards, leftover food, and damaged stock that has not been used. Unavoidable food waste arises from food preparation or production and includes those by-products that are not edible in normal circumstances, such as vegetable and fruit peels, bones, fat, and feathers. Despite the lack of consensus on the definition of food loss and waste, the reduction in food loss and waste points in one direction and that is securing global food sustainability.
In a circular food system, the strategies for reducing food waste vary with the type of waste (Figure 2). The best measure to reduce avoidable food waste is prevention, which can be integrated in the various stages of the food supply chain. Preventing overproduction, improving packaging and storage facilities, reducing food surplus by ensuring balanced food distribution, and educating consumers about proper meal planning, better understanding of best before dates, and buying food that may not pass quality control standards based on aesthetics are some preventive measures to reduce avoidable food waste (Papargyropoulou et al., 2014). For unavoidable food waste, reduction can be achieved by utilizing side-stream products as raw materials for the production of new food or non-food materials. The residual waste generated, both from the processing of avoidable and unavoidable food waste, can still be treated through composting, which returns nutrients back to the soil, and used for another cycle of food production (Jurgilevich et al., 2016). Indeed, in a circular food system, waste is ideally non-existent because it is used as a feedstock for another cycle, creating a system that mimics natural regeneration (Ellen MacArthur Foundation, 2019).
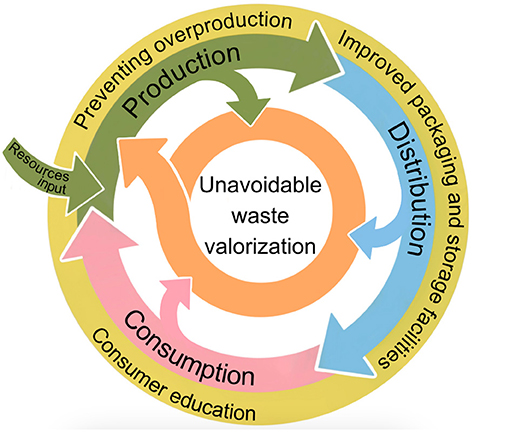
Figure 2. Strategies to reduce food waste in the food supply chain in a circular food system: prevention for avoidable food waste (yellow curve) and valorization for unavoidable food waste (orange curve).
The valorization of unavoidable food waste, which mostly includes by-products or side-stream materials from the food processing industries, has resulted in novel food technologies that harness the most out of food waste and add value to food waste. These novel food technologies serve as new routes to achieving a circular food system by converting food waste into new food ingredients or non-food materials. Several ongoing examples of side-stream valorization have been explored and some of the most recent technologies are presented herein and summarized in Table 2.
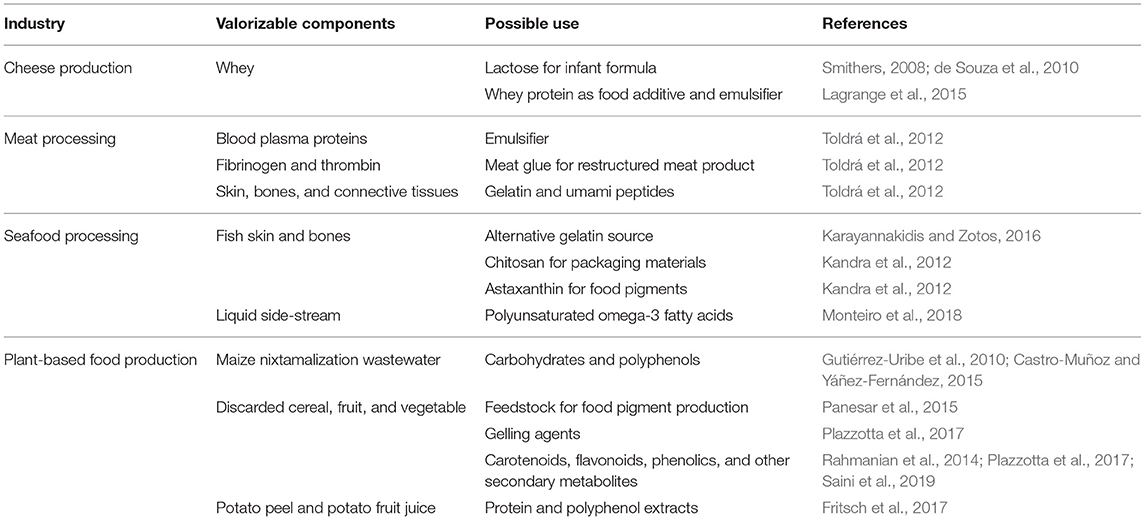
Table 2. Summary of potentially functional and nutritional food components from cheese production, meat processing, seafood processing, and plant-based food production by-products.
One of the most famous success stories of side-stream valorization is the processing of whey, the leftover liquid from cheese production. It is an environmental hazard when disposed of without treatment, having a high biological oxygen demand (BOD) value of >35,000 ppm as well as a high chemical oxygen demand (COD) value of >60,000 ppm (Smithers, 2008). These high BOD and COD values can be detrimental to aquatic life where the untreated whey is disposed of, reducing the available dissolved oxygen for fish and other aquatic animals. However, whey is loaded with both lactose and proteins, and therefore in the early days cheese producers sent their whey for use as pig feed, as still occurs in some areas today. As dairy science advanced, it was discovered that lactose and whey protein have great nutritional and technological potential. Lactose and its derivatives can be separated by various filtration and crystallization methods, which can then be used in infant formula or as a feedstock for glucose and galactose production (Smithers, 2008; de Souza et al., 2010). Whey protein has also gained popularity for use in sports performance nutrition and as an enhancer of the functional properties of food, and so has experienced a significant increase in demand, both as isolate and concentrate products (Lagrange et al., 2015).
The meat-processing industry produces various by-products that can also be further processed to obtain food ingredients. The plasma fraction of animal blood, which can easily be obtained by centrifugation, contains various plasma proteins, some of which can stabilize colloidal food systems, just like whey proteins. Others, like fibrinogen and thrombin, can act as meat glue and are therefore useful to make restructured meat product. Leftover skin, bones, and connective tissues can be processed to produce gelatin, an important gelling agent, as well as short peptides that impart an umami taste and are used in flavor enhancers. However, the use of non-muscle tissue from farm animals, especially from cows, would require strict toxicology assessment to ensure safety. There is a risk of spreading transmissible spongiform encephalopathy, a deadly disease caused by prion proteins which might spread to humans through the consumption of materials derived from non-meat tissues (Toldrá et al., 2012).
The by-products of the seafood industry also provide great opportunities for valorization, with several known products and many other yet to be discovered. Fish-derived gelatin from leftover fish skin and bones can be presented as a gelatin alternative for several religious groups, for whom cattle- and swine-derived gelatin products are unacceptable (Karayannakidis and Zotos, 2016). Rich in carotenoid and chitin, shells of common seafood such as crabs, lobster, and prawns can be further processed to extract functional ingredients. The extracted chitin from the shells can be treated to produce chitosan, a well-known biopolymer with the potential to be used as food packaging. One can also extract the red carotenoids present in the shells, most prominently astaxanthin, which can then be used as a nutritional and technological food additive (Kandra et al., 2012). The liquid side stream of the fish-canning industry also has potential as a source of bioactive lipids, such as polyunsaturated omega-3 fatty acids (Monteiro et al., 2018).
The increasing demand for plant-derived functional ingredients to cater for the vegetarian and vegan market can also be complemented with ingredients isolated from plant food processing side streams. Nixtamalization, the alkaline processing of maize, produces wastewater that is highly alkaline with a high COD of 10 200–20,000 ppm but is rich in carbohydrates and polyphenols (Gutiérrez-Uribe et al., 2010). Microfiltration and ultrafiltration methods are used to isolate enriched fractions of carbohydrates and polyphenols from nixtamalization wastewater, which can later be integrated into various subsequent processes (Castro-Muñoz and Yáñez-Fernández, 2015). Waste from the cereal, fruit, and vegetable industry can also be fermented by microbial means to produce various pigments for food production (Panesar et al., 2015). Pigment extraction can also be performed on the leftover waste of the fresh-cut salad industry, which includes leafy vegetables and fruits that are deemed to be too blemished to be sold to the customer. Aside from pigments, such waste can also be a source of natural gelling agents and bioactive compounds that can be refined for further use in the food industry (Plazzotta et al., 2017). Extraction of carotenoids, flavonoids, and phenolic compounds from fruits and vegetables waste as well as from wastewater (e.g., from olive mill) can be achieved using green technologies such as supercritical carbon dioxide, ultrasound, microwave, pulsed electric fields, enzymes, membrane techniques, and resin adsorption (Rahmanian et al., 2014; Saini et al., 2019). Additionally, waste from potato processing, such as potato peel and potato fruit juice (a by-product of potato starch production), can yield various polyphenols, alkaloids, and even protein extracts by using different refining methods (Fritsch et al., 2017).
In addition to food waste, there are also other, often unexpected, sources of food ingredients. For example, while wood cannot be considered part of the food industry by itself, the extraction of emulsifier from sawdust can serve as an example of how the waste of one industrial cycle can be used as a feedstock for another industrial cycle and in effect reduce the overall wasted material (Pitkänen et al., 2018). Straw from grain production, such as barley and wheat, can also be processed to extract oligosaccharides to be used as prebiotic additives into other food matrices (Huang et al., 2017; Alvarez et al., 2020). While young bamboo shoots have been commonly used in various Asian cuisines, older bamboo leaves can also act as a source of polyphenolic antioxidants, which can be used to fortify food with bioactive compounds (Ni et al., 2012; Nirmala et al., 2018).
Alternative Technologies and Sources for Food Production
To feed the growing population, the circular economy concept must be combined with increasing food production. However, food production has been impaired by depletion of resources, such as water and arable land, and by climate change. Projections indicate that 529,000 climate-related deaths will occur worldwide in 2050, corresponding with the predicted 3.2% reduction in global food availability (including fruits, vegetables, and red meat) caused by climate change (Springmann et al., 2016). Strategies to overcome food production issues have been developed and implemented that aim to improve agricultural productivity and resource use (vertical farming and genetic modification), increase and/or tailor the nutritional value of food (genetic engineering), produce new alternatives to food and/or food ingredients (cellular cultures, insects, algae, and dietary fibers), and protect biodiversity. Such solutions have been designed to supply current and future food demand by sustainably optimizing the use of natural resources and boosting the restructuration of the food industry models (Figure 3).
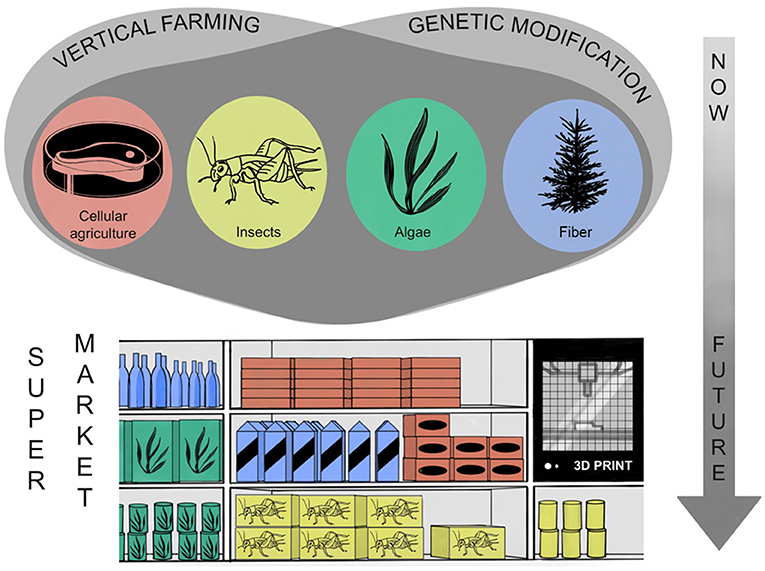
Figure 3. A view of future food based on current prospects for optimizing the use of novel techniques, food sources, and nutritional ingredients.
Cellular agriculture is an emerging field with the potential to increase food productivity locally using fewer resources and optimizing the use of land. Cellular agriculture has the potential to produce various types of food with a high content of protein, lipids, and fibers. This technique can be performed with minimal or no animal involvement following two routes: tissue engineering and fermentation (Stephens et al., 2018). In the tissue engineering process, cells collected from living animals are cultured using mechanical and enzymatic techniques to produce muscles to be consumed as food. In the case of the fermentation process, organic molecules are biofabricated by genetically modified bacteria, algae, or yeasts, eliminating the need for animal cells. The Solar Foods company uses the fermentation process to produce Solein, a single-cell pure protein (https://solarfoods.fi/solein/). This bioprocess combines the use of water, vitamins, nutrients, carbon dioxide (CO2) from air, and solar energy to grow microorganisms. After that, the protein is obtained in powder form and can be used as a food ingredient. Most of the production in cellular agriculture has been focused on animal-derived products such as beef, chicken, fish, lobster, and proteins for the production of milk and eggs (Post, 2014; Stephens et al., 2018). Compared with traditional meat, the production of cultured meat can (i) reduce the demand for livestock products, (ii) create a novel nutrition variant for people with dietary restrictions, (iii) favor the control and design of the composition, quality, and flavor of the product, and (iv) reduce the need for land, transportation costs (it can be produced locally), waste production, and greenhouse gas emissions (Bhat and Fayaz, 2011). Moreover, the controlled production of cultured meat can eliminate the presence of unwanted elements, such as saturated fat, microorganisms, hormones, and antibiotics (Bhat and Fayaz, 2011). One of the most important events for cultured meat took place in a 2013 press conference in London, when cultured beef burger meat was tasted by the public for the first time (O'Riordan et al., 2017). After this, cultured meat has inspired several start-ups around the world and some examples are presented in Table 3 (Clean Meat News Australia, 2019).
However, cellular agriculture has the potential to produce more than only animal-derivative products. A recent study conducted by the VTT Technical Research Centre of Finland explored the growing of plant cell cultures from cloudberry, lingonberry, and stoneberry in a plant growth medium. The cells were described to be richer in protein, essential polyunsaturated fatty acids, sugars, and dietary fibers than berry fruits, and additionally to have a fresh odor and flavor (Nordlund et al., 2018). Regarding their use, berry cells can be used to replace berry fruits in smoothies, yogurt, jam, etc. or be dried and incorporated as ingredients in several preparations (e.g., cakes, desserts, and toppings).
Insects are potentially an important source of essential nutrients such as proteins, fat (including unsaturated fatty acids), polysaccharides (including chitin), fiber, vitamins, and minerals. Edible insects are traditionally consumed in different forms (raw, steamed, roasted, smoked, fried, etc.) by populations in Africa, Central and South America, and Asia (Duda et al., 2019; Melgar-Lalanne et al., 2019). The production of edible insects is highly efficient, yielding various generations during the year with low mortality rates and requiring only little space, such as vertical systems (Ramos-Elorduy, 2009). Additionally, the cultivation of edible insects utilizes very cheap materials, usually easily found in the surrounding area. Indeed, insects can be fed by food waste and agricultural by-products not consumed by humans, which fits well in the circular bioeconomy models (section The circular economy). The introduction of insect proteins could diversify and create more sustainable dietary alternatives. However, the resistance of consumers to the ingestion of insects needs to be overcome (La Barbera et al., 2018). The introduction of insects in the form of powder or flour can help solve consumer resistance (Duda et al., 2019; Melgar-Lalanne et al., 2019). Several technologies are used to transform insect biomass into food ingredients, including drying processes (freeze-drying, oven-drying, fluidized bed drying, microwave-drying, etc.) and extraction methods (ultrasound-assisted extraction, cold atmospheric pressure plasma, and dry fractionation) (Melgar-Lalanne et al., 2019). Recently, cricket powder was used for enriching pasta, resulting in a significant increase in protein, fat, and mineral content, and additionally improving its texture and appearance (Duda et al., 2019). Chitin, extracted from the outer skeleton of insects, is a precursor for bioactive derivatives, such as chitosan, which presents potential to prevent and treat diseases (Azuma et al., 2015; Kerch, 2015). Regenerated chitin has been recognized as a promising emulsifier (Xiao et al., 2018), with potential applications including stabilizing yogurt, creams, ice cream, etc. Whole insects, insect powder, and food products from insects such as flavored snacks, energy bars and shakes, and candies are already commercialized around the world. However, food processing and technology is currently needed to help address consumer neophobia and meet sensory requirements (Melgar-Lalanne et al., 2019).
Algae and microalgae are a source of nutrients in various Asian countries (Priyadarshani and Rath, 2012; Wells et al., 2017; Sathasivam et al., 2019), that can be consumed as such (bulk material) or as an extract. The extracts consists of biomolecules that are synthesize more efficiently than plants (Torres-Tiji et al., 2020). Some techniques used for improving algae and microalgae productivity and their nutritional quality are genotype selection, alteration, and improvement, and controlling growing conditions (Torres-Tiji et al., 2020). Although their direct intake is more traditional (e.g., nori used in sushi preparation), in recent years the extraction of bioactive compounds from algae and microalgae for the preparation of functional food has attracted great interest. Spirulina and Chlorella are the most used microalgae species for this purpose, being recognized by the European Union for uses in food (Zarbà et al., 2020). These microalgae are rich in proteins (i.e., phycocyanin), essential fatty acids (i.e., omega-3, docosahexaenoic acid, and eicosapentaenoic acid), β-glucan, vitamins from various groups (e.g., A, B, C, D2, E, and H), minerals like iodine, potassium, iron, magnesium, and calcium, antioxidants (i.e., ß-carotene), and pigments (i.e., astaxanthin) (Priyadarshani and Rath, 2012; Vigani et al., 2015; Wells et al., 2017; Sathasivam et al., 2019). The latter molecules can be recovered using, for example, pulsed electric field, ultrasound, microwaves, and supercritical CO2 (Kadam et al., 2013; Buchmann et al., 2018).
Finally, in addition to proteins, lipids, and digestible carbohydrates, it is necessary to introduce fiber in to the diet. Dietary fibers include soluble (pectin and hydrocolloids) and insoluble (polysaccharides and lignin) fractions, which are usually obtained through the direct ingestion of fruits, vegetables, cereals, and grains (McKee and Latner, 2000). Although appropriate dietary fiber intake leads to various health benefits, the proliferation of low fiber foods, especially in Western countries resulted in low dietary intake (McKee and Latner, 2000; Anderson et al., 2009). This lack of consumed dietary fibers created the demand for fiber supplementation in functional foods (McKee and Latner, 2000; Doyon and Labrecque, 2008). As additives, besides all benefits in health and well-being, dietary fibers contribute to food structure and texture formation (Sakagami et al., 2010; Tolba et al., 2011; Jones, 2014; Aura and Lille, 2016).
Sources of dietary fibers include food crops (e.g., wheat, corn, oats, sorghum, oat, etc.), vegetables/fruits (e.g., apple and pear biomasses recovered after juicing process, orange peel and pulp, pineapple shells, etc.) (McKee and Latner, 2000) and wood (Pitkänen et al., 2018). The use of plant-based derivatives and waste aligns with the circular bioeconomy framework and contributes to the sustainability of the food chain.
It is worth mentioning that new and alternative sources of food and food ingredients require approval in the corresponding regulatory systems before commercialization. In Europe, safety assessment is carried out according to the novel food regulation of the European Union [Regulation (EU) 2015/2283]. Important aspects such as composition, stability, allergenicity, and toxicology should be evaluated for each new food or food ingredient (Pitkänen et al., 2018). Such regulatory assessments are responsible for guaranteeing that new food and food ingredients are safe for human consumption.
Food Design
Humans are at the center of the food supply ecosystem, with diverse and dynamic expectations. To impart sustainability in food supply by utilizing novel materials and technologies discussed in the preceding chapters, the framework of food production and consumption should go beyond creating edible objects and integrate creativity to subvert neophobic characteristics of consumers and enhance acceptability of sustainable product innovations. These innovations should also consider changing consumer demographics, lifestyle and nutritional requirements. Food design is a newly practiced discipline to foster human-centric innovation in the food value chain by applying a design thinking process in every step of production to the disposal of food (Olsen, 2015). The design concept utilizes the core ideas of consumer empathy, rapid prototyping, and mandate the collaboration of a multitude of sectors involved in designing food and the distribution of food to the space where we consume it (Figure 4) (Zampollo, 2020).
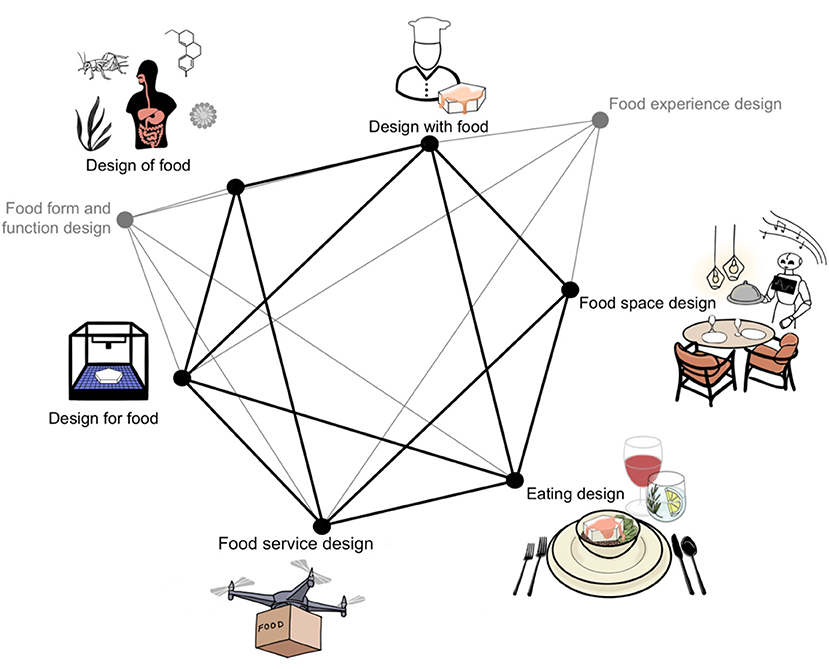
Figure 4. Neural network graphical representation of the major disciplines (black dots) in the food design concept and their interconnections. Sub-disciplines arising through communion of ideas of some major disciplines indicated by gray dots.
The sub-discipline of food product design relates to the curation of food products from a technological perspective utilizing innovative process and structured engineering methodologies to translate consumer wishes into product properties. In the future, food producers need to shift their focus from the current conventional approach of mass production, to engineering of food products that emphasizes food structure-property-taste. Through food product design, it is possible to influence the health of consumers by regulating nutrient bioavailability, satiety, gut health, and developing feelings of well-being, as well as encompass consumer choice by modulating consumers sensorial experience. These aspects become important with the introduction of new materials and healthy alternatives where the neophobic characteristic of humans can lead to poor food choices and eating habits due to consumer prejudices or inferior sensorial experience. For example, environmental concerns related to meat substitutes were less relevant for consumers, and sensorial properties were the decisive factor (Hoek et al., 2011; Weinrich, 2019). In this regard, food designers and chefs will have an important role in influencing sustainable and healthy eating choices by increasing the acceptability of food products, using molecular gastronomy principles. Innogusto (www.innogusto.com), a start-up founded in 2018, aims to develop gastronomic dishes based on meat substitutes to increase their acceptability.
To stimulate taste sensations, electric and thermal energy have been studied, referred to as “digital taste” (Green and Nachtigal, 2015; Ranasinghe et al., 2019). For example, reducing the temperature of sweet food products can increase sweet taste adaptation and reduce sweetness intensity (Green and Nachtigal, 2015). On the other hand, electric taste augmentation can modulate the perception of saltiness and sourness in unsalted and diluted food products leading to a possible reduction of salt (Ranasinghe et al., 2019). Another external stimulus that can modify the sensorial experience during food consumption, is social context. In this case, interaction with other people leads to a resonance “mirror” mechanism, that allow people to tune in to the emotions of others. Indeed, positive emotions such as happiness increase the desirability and acceptability of food, contrarily to neutral and negative emotions (angriness) (Rizzato et al., 2016). Also, auditory responses such as that to background music, referred to as “sonic seasoning” (Reinoso Carvalho et al., 2016) have been studied in the context of desirability and overall perception of food. Noise is able to reduce the perception of sweetness and enhance the perception of an umami taste (Yan and Dando, 2015). Bridging the interior design concepts with the sensory perception in a holistic food space design is an interesting opportunity to influence healthy habits and accommodate unconventional food in our daily lives.
Food packaging which falls under the Design for food sub-discipline is expected to play an integral role to tackle issues of food waste/loss. Potential solutions to food waste/loss at the consumers level can be realized by the design of resealable packages, consideration of portion size, clear labeling of “best by” and expiration dates, for example. Although a clear understanding on the interdependency of food waste and packaging design in the circular economy has not yet been established, the design of smart packaging to prolong shelf life and quality of highly perishable food like fresh vegetables, fruits, dairy, and meat products has been considered the most efficient option (Halloran et al., 2014). Packaging is a strong non-verbal medium of communication between product designers and consumers which can potentially be used to favor the consumption of healthier and sustainable options (Plasek et al., 2020). Packaging linguistics has shown differential effect on taste and quality perceptions (Khan and Lee, 2020), whereas designs have shown to create emotional attachment to the product surpassing the effect of taste (Gunaratne et al., 2019). Visual stimuli such as weight, color, size, and shape of the food containers have been linked to the overall liking of the food (Piqueras-Fiszman and Spence, 2011; Harrar and Spence, 2013). Food was perceived to be dense with higher satiety when presented in heavy containers compared with light-weighted containers (Piqueras-Fiszman and Spence, 2011).
In light of emerging techniques in food production, it is envisioned that technologies like 3D printing, at both the industrial and household level, will be widely used to design food and recycle food waste (Gholamipour-Shirazi et al., 2020). Upprinting Food (https://upprintingfood.com/), a start-up company, has initiated the production of snacks from waste bread using 3D printing. These initiatives will also encourage the inclusion of industrial side streams (discussed in section the circular economy) in the mainstream using novel technologies. In addition to the increasing need for healthy food, it is envisioned that the food industry will see innovation regarding personalized solutions (Poutanen et al., 2017). In the latter, consumers will be at the center of the food production system, where they can choose food that supports their personal physical and mental well-being, and ethical values. Techniques such as 3D printers can be applied in smart groceries and in the home, where one can print personalized food (Sun et al., 2015) inclusive of molecular gastronomy methods (D'Angelo et al., 2016). A challenge will be to incorporate the food structure-property-taste factor in such systems. In a highly futuristic vision, concepts of personalized medicine are borrowed to address the diverse demands of food through personalized or “smart” food, possibly solving food-related diseases, while reducing human ecological footprint.
Digitalization
Many major challenges faced by global food production, as discussed previously and presented in Table 1 (eating habits and dietary choices, food waste and loss, biodiversity, diseases, and resource availability), can be addressed by food system digitalization. The most recent research advances aim to overcome these challenges using digitalization (summarized in Table 4 and Figure 5). The rapidly advancing information and communication technology (ICT) sector has enabled innovative technologies to be applied along the agri-food chain to meet the demands for safe and sustainable food production (i.e., traceability) (Demartini et al., 2018; Raheem et al., 2019).
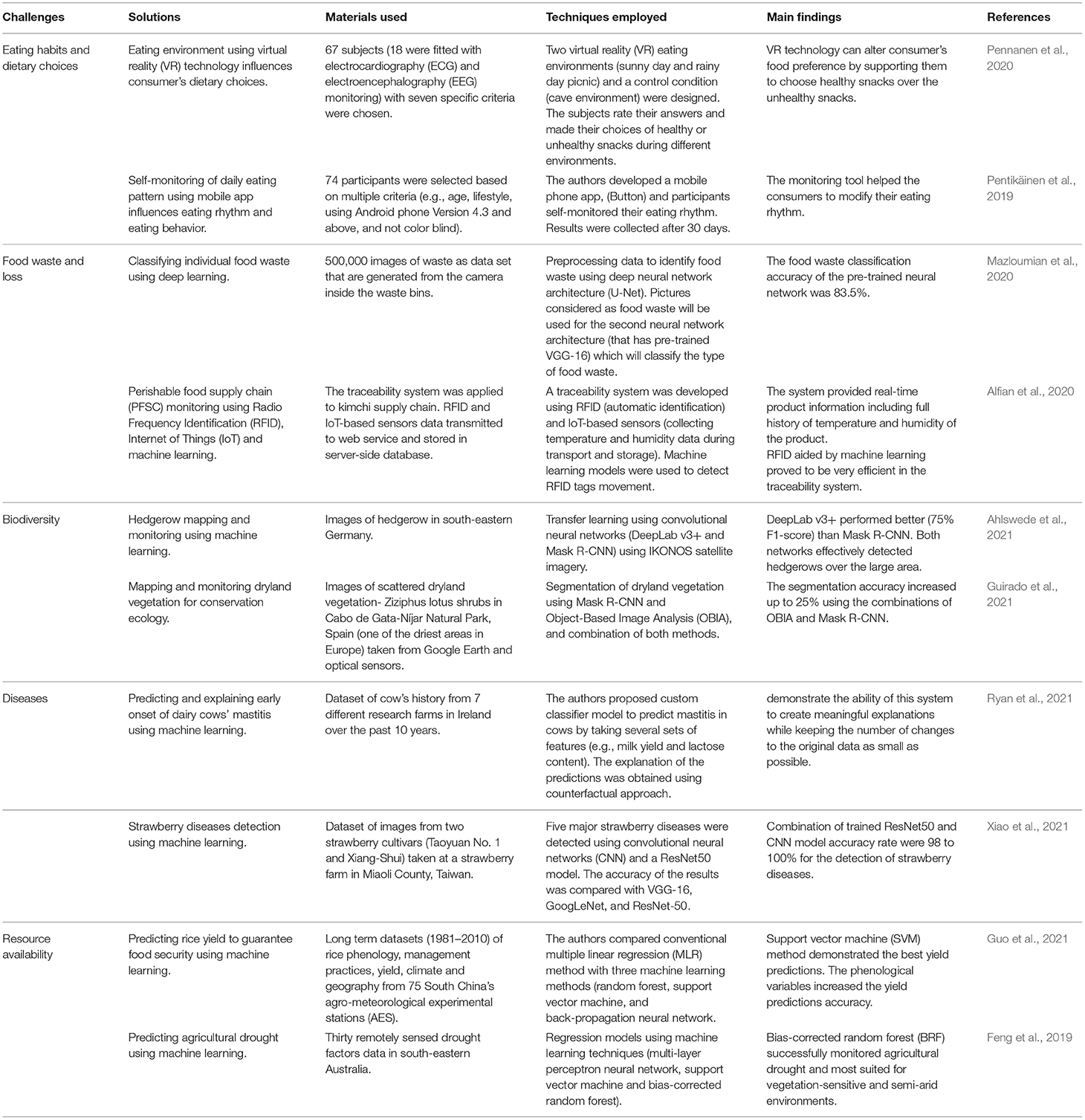
Table 4. Recent research advances in digitalization solutions to overcome challenges in global food production.
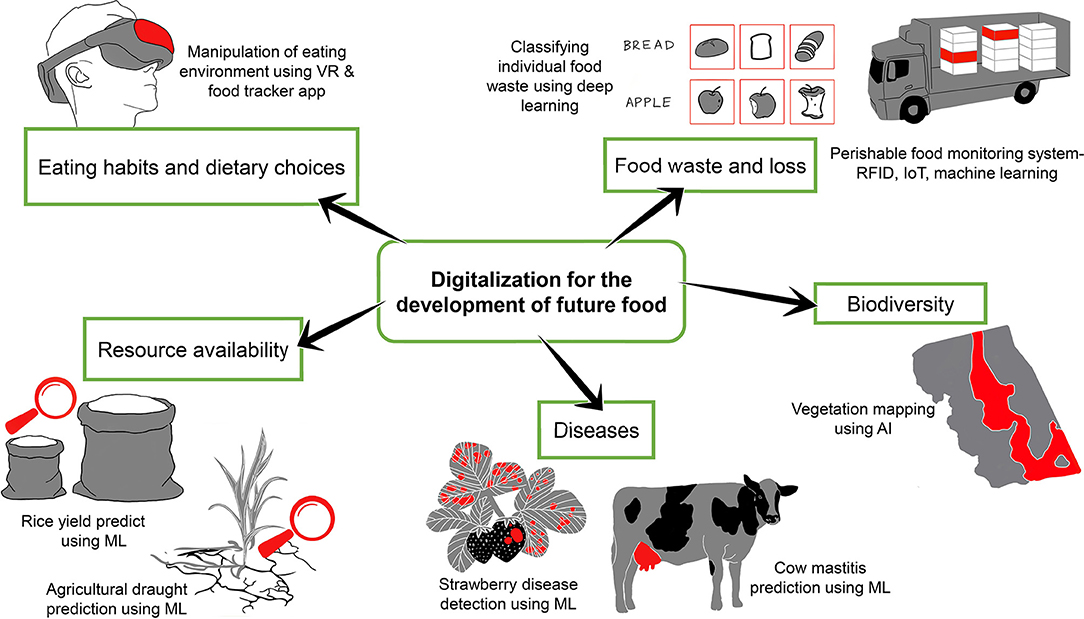
Figure 5. Digitalization solutions for the development of future food. Red area represents digitalization-enabled targets. IoT, Internet of Things; ML, Machine Learning; RFID, Radio Frequency Identification; AI, Artificial Intelligence.
An interesting part of ICT is artificial intelligence (AI). The latter is a field of computer science that allows machines, especially computer systems, to have cognitive functions like humans. These machines can learn, infer, adapt, and make decisions based on collected data (Salah et al., 2019). Over the past decade, AI has changed the food industry in extensive ways by aiding crop sustainability, marketing strategies, food sales, eating habits and preferences, food design and new product development, maintaining health and safety systems, managing food waste, and predicting health problems associated with food.
Digitalization can be used to modify our perception of food and help solve unsustainable eating behaviors. It is hoped that a better insight into how the neural network in the human brain works upon seeing food can be discovered using AI in the future and can thus direct consumer preference toward healthier diets. Additionally, it can be used to assist the development of new food structures and molecules such as modeling food gelling agents (e.g., using fuzzy modeling to predict the influence of different gum-protein emulsifier concentration on mayonnaise), and the design of liquid-crystalline food (by predicting the most stable liquid crystalline phases using predictive computer simulation tool based on field theory) (Mezzenga et al., 2006; Ghoush et al., 2008; Dalkas and Euston, 2020). In addition, the development of aroma profiles can be explored using AI. Electronic eyes, noses, and tongues can analyze food similarly to sensory panelists and help in the optimization of quality control in food production (Loutfi et al., 2015; Nicolotti et al., 2019; Xu et al., 2019). Companies like GastrographAI (https://gastrograph.com/) and Whisk (https://whisk.com/) are using AI and natural language processing to model consumer sensory perception, predict their preferences toward food and beverage products, map the world's food ingredients, and provide specific advertisements based on consumer personalization and preferences.
With the advancement of augmented reality (AR) and virtual reality (VR), in the future, digitalization can offer obesity-related solutions, where consumers can eat healthy food while simultaneously seeing unhealthy desirable food. This possibility has been studied by Okajima et al. (2013) using an AR system to change visual food appearance in real time. In their study, the visual appearance of food can highly influence food perception in terms of taste and perceived texture.
AI also provides a major solution to food waste problems by estimating food demand quantity, predicting waste volumes, and supporting effective cleaning methods by smart waste management (Adeogba et al., 2019; Calp, 2019; Gupta et al., 2019).
AI-enabled agents, Internet of Things (IoT) sensors, and blockchain technology can be combined to maximize the supply network and increase the revenue of all parties involved along the agri-food value chain (Salah et al., 2019). Blockchain is a technology that can record multiple transactions from multiple parties across a complex network. Changing the records inside the blockchain requires the consensus of all parties involved, thus giving a high level of confidence in the data (Olsen et al., 2019). Blockchain technology can support the traceability and transparency of the food supply chain, possibly increasing the trust of consumers, and in combination with AI, intelligent precision farming can be achieved, as illustrated in Figure 6.
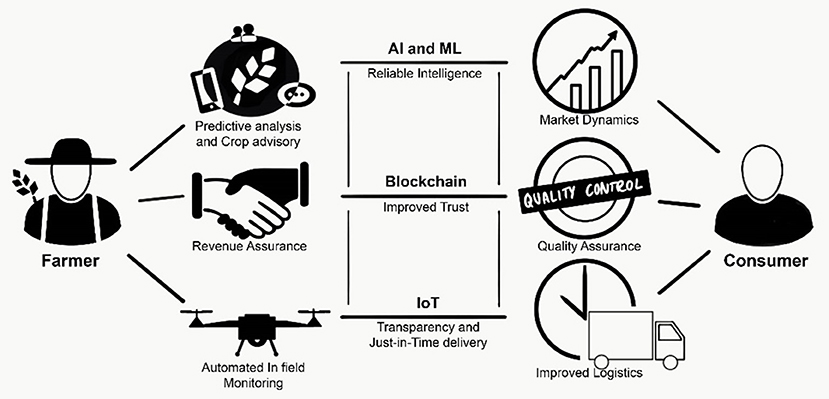
Figure 6. Digitalization in the food supply chain: intelligent precision farming with artificial intelligence (AI) and blockchain. IoT, Internet of Things; ML, Machine Learning. Modified from Salah et al. (2019) and reproduced with permission from IEEE.
The physical flow of the food supply chain is supported by the digital flow, consisting of different interconnected digital tools. As each block is approved, it can be added to the chain of transactions, and it becomes a permanent record of the entire process. Each blockchain contains specific information about the process where it describes the crops used, equipment, process methods, batch number, conditions, shelf-time, expiration date, etc. (Kamath, 2018; Kamilaris et al., 2019).
Traceability and transparency of the complex food supply network are continuously increasing their importance in food manufacturing management. Not only are they an effective way to control the quality and safety of food production, but they can also be effective tools to monitor the flow of resources from raw materials to the end consumer. In the future, it will be essential to recognize the bottlenecks of the entire food supply chain and redirect the food resource allocation accordingly to minimize food waste.
The digital tools reviewed here can be combined with all the solutions proposed before, enabling fast achievement of the necessary conditions for feeding the increasing world population while maintaining our natural resources.
The Effect of Novel Coronavirus Disease (COVID-19) Pandemic on the Food System
Although the strategies examined in this review can possibly help reaching food security in 2050, the entire food system has been facing a new challenge because of COVID-19 pandemic. Since December 2019, a new severe acute respiratory syndrome (SARS) caused by a novel Coronavirus started spreading worldwide from China. To contain the diffusion of the novel Coronavirus and avoid the collapse of national sanitary systems, several governments locked down entire nations. These actions had severe consequences on global economy, including the food system.
As first consequence, the lockdown changed consumer purchasing behavior. At the initial stage of the lockdown, panic-buying behavior was dominant, in which consumers were buying canned foods and stockpiling them, leading to shortage of food in several supermarkets (Nicola et al., 2020). However, as the lockdown proceeded, this behavior become more moderate (Bakalis et al., 2020). The problems faced by the food supply chain in assuring food availability for the entire population have risen concerns about its architecture. Indeed, as discussed by Bakalis et al. (2020), the western world food supply chain has an architecture with a bottleneck at the supermarkets/suppliers interface where most of the food is controlled by a small number of organizations. Additionally, as noted by these authors, problems with timely packaging of basic foods (such as flour) led to their shortage. Bakalis et al. (2020) suggest that the architecture of the food system should be more local, decentralized, sustainable, and efficient. The COVID-19 pandemic highlighted the vulnerability of the food system, indicating that the aid of future automation (robotics) and AI would help to maintain an operational supply chain. Therefore, the entire food system should be rethought with a resilient and sustainable perspective, which can assure adequate, safe, and health-promoting food to all despite of unpredictable events such as COVID-19, by balancing the roles of local and global producers and involving policymakers (Bakalis et al., 2020; Galanakis, 2020).
Another problem caused by the lockdown was food waste. Indeed, restaurants, catering services, and food producers increased their food waste due to forced closure and rupture of the food chain (Bakalis et al., 2020). On the other hand, consumers become more aware of food waste and strived to reduce household food waste. Unfortunately, the positive behavior of consumers toward reducing food waste has been more driven by the COVID-19 lockdown situation rather than an awareness (Jribi et al., 2020).
COVID-19 has also showed the importance of designing food products that can help boosting our immune system and avoid the diffusion of virions through the entire food chain (Galanakis, 2020; Roos, 2020). Virions can enter the food chain during food production, handling, packing, storage, and transportation and be transmitted to consumers. This possibility is increased with minimally processed foods and animal products. Therefore, packaging and handling of minimally processed foods should be considered to reduce viral transfer while avoiding increasing waste. The survival of virions in food products can be reduced by better designing and engineering foods taking into consideration for example not only thermal inactivation of virions but also the interaction between temperature of inactivation, water activity of food, and food matrix effects (Roos, 2020).
Therefore, to reach food security by 2050, besides the solutions highlighted in section (Food science and technology solutions for global food security), it is of foremost important to implement actions in the entire food system that can counteract exceptional circumstances such as the global pandemic caused by the novel Coronavirus.
Conclusions and Outlook
To achieve food security in the next 30 years while maintaining our natural bioresources, a transition from the current food system to a more efficient, healthier, equal, and consumer- and environment-centered food system is necessary. This transition, however, is complex and not straightforward. First, we need to fully transition from a linear to a circular economy where side streams and waste are valorized as new sources of food materials/ingredients, leading to more efficient use of the available bioresources. Secondly, food production has to increase. For this, vertical farming, genetic engineering, cellular agriculture, and unconventional sources of ingredients such as microalgae, insects, and wood-derived fibers can make a valid contribution by leading to a more efficient use of land, an increase in food and ingredient productivity, a shift from global to local production which reduces transportation, and the transformation of non-reusable and inedible waste into ingredients with novel functionalities. However, to obtain acceptable sustainable food using novel ingredients and technologies, the aid of food design is necessary in which conceptualization, development, and engineering in terms of food structure, appearance, functionality, and service result in food with higher appeal for consumers. To complement these solutions, digital technology offers an additional potential boost. Indeed, AI, blockchain, and VR and AR are tools which can better manage the whole food chain to guarantee quality and sustainability, assist in the development of new ingredients and structures, and change the perception of food improving acceptability, which can lead to a reduction of food-related diseases.
By cooperating on a global scale, we can envision that in the future it may be common to, for example, 3D print a steak at home using cells or plant-based proteins. The understanding of the interaction between our gastrointestinal tract and the food ingredients/structures aided by AI and biosensors might allow the 3D printed steak to be tailored in terms of nutritional value and individual preferences. The food developed in the future can possibly also self-regulate its digestibility and bioavailability of nutrients. In this context, the same foodstuff consumed by two different people would be absorbed according to the individuals' needs. In this futuristic example, the food of the future would be able to solve food-related diseases such as obesity and type 2 diabetes, while maintaining the ability of the Earth to renew its bioresources.
However, the strategies and solutions proposed here can possibly only help to achieve sustainable food supply by 2050 if they are supported and encouraged globally by common policies. Innovations in food science and technology can ensure the availability of acceptable, adequate, and nutritious food, and can help shape the behavior of consumers toward a more sustainable diet. Finally, the recent COVID-19 global pandemic has highlighted the importance of developing a resilient food system, which can cope with exceptional and unexpected situations. All these actions can possibly help in achieving food security by 2050.
Author Contributions
FV wrote abstract, sections introduction, the effect of novel Coronavirus disease (COVID-19) pandemic on the food system, and conclusions and outlook, and coordinated the writing process. MA and FA wrote section the circular economy. DM and JS wrote section alternative technologies and sources for food production. MB and JV wrote section food design. AA and EP wrote section digitalization. FV and KM revised and edited the whole manuscript. All authors have approved the final version before submission and contributed to planning the contents of the manuscript.
Funding
FV, MA, FA, and KM acknowledge the Academy of Finland for funding (FV: Project No. 316244, MA: Project No. 330617, FA: Project No. 322514, KM: Project No. 311244). DM acknowledges Tandem Forest Values for funding (TFV 2018-0016).
Conflict of Interest
The authors declare that the research was conducted in the absence of any commercial or financial relationships that could be construed as a potential conflict of interest.
Publisher's Note
All claims expressed in this article are solely those of the authors and do not necessarily represent those of their affiliated organizations, or those of the publisher, the editors and the reviewers. Any product that may be evaluated in this article, or claim that may be made by its manufacturer, is not guaranteed or endorsed by the publisher.
Acknowledgments
We thank JV for drawing Figures 2–6, and Mr. Troy Faithfull for revising and editing the manuscript.
References
Adeogba, E., Barty, P., O'Dwyer, E., and Guo, M. (2019). Waste-to-resource transformation: gradient boosting modeling for organic fraction municipal solid waste projection. ACS Sustain. Chem. Eng. 7, 10460–10466. doi: 10.1021/acssuschemeng.9b00821
Ahlswede, S., Asam, S., and Röder, A. (2021). Hedgerow object detection in very high-resolution satellite images using convolutional neural networks. J. Appl. Remote Sens. 15:018501. doi: 10.1117/1.JRS.15.018501
Alfian, G., Syafrudin, M., Farooq, U., Ma'arif, M. R., Syaekhoni, M. A., Fitriyani, N. L., et al. (2020). Improving efficiency of rfid-based traceability system for perishable food by utilizing iot sensors and machine learning model. Food Control 110:107016. doi: 10.1016/j.foodcont.2019.107016
Alvarez, C., Gonzalez, A., Alonso, J. L., Saez, F., Negro, M. J., and Gullon, B. (2020). Xylooligosaccharides from steam-exploded barley straw: structural features and assessment of bifidogenic properties. Food Bioproducts Process. 124, 131–142. doi: 10.1016/j.fbp.2020.08.014
Anderson, J. W., Baird, P., Davis, R. H. Jr., Ferreri, S., Knudtson, M., Koraym, A., et al. (2009). Health benefits of dietary fiber. Nutr. Rev. 67, 188–205. doi: 10.1111/j.1753-4887.2009.00189.x
Aura, A. M., and Lille, M. (2016). Wood Components to Boost the Quality of Food Products. VTT – Technical Research Center of Finland Ltd. Available online at: https://news.cision.com/vtt-info/r/wood-components-to-boost-the-quality-of-food-products.c2020810
Azais-Braesco, V., Brighenti, F., Paoletti, R., Peracino, A., Scarborough, P., Visioli, F., et al. (2009). Healthy food and healthy choices: a new european profile approach. Atheroscler. Suppl. 10, 1–11. doi: 10.1016/j.atherosclerosissup.2009.09.001
Azuma, K., Nagae, T., Nagai, T., Izawa, H., Morimoto, M., Murahata, Y., et al. (2015). Effects of surface-deacetylated chitin nanofibers in an experimental model of hypercholesterolemia. Int. J. Mol. Sci. 16, 17445–17455. doi: 10.3390/ijms160817445
Bain, L. E., Awah, P. K., Geraldine, N., Kindong, N. P., Sigal, Y., Bernard, N., et al. (2013). Malnutrition in sub-saharan africa: burden, causes and prospects. Pan Afr. Med. J. 15:120. doi: 10.11604/pamj.2013.15.120.2535
Bakalis, S., Valdramidis, V. P., Argyropoulos, D., Ahrne, L., Chen, J., Cullen, P. J., et al. (2020). Perspectives from co+re: how covid-19 changed our food systems and food security paradigms. Curr. Res. Food Sci. 3, 166–172. doi: 10.1016/j.crfs.2020.05.003
Berners-Lee, M., Kennelly, C., Watson, R., and Hewitt, C. N. (2018). Current global food production is sufficient to meet human nutritional needs in 2050 provided there is radical societal adaptation. Elementa Sci. Anthropocene 6, 52–66. doi: 10.1525/elementa.310
Bhat, Z. F., and Fayaz, H. (2011). Prospectus of cultured meat—advancing meat alternatives. J. Food Sci. Technol. 48, 125–140. doi: 10.1007/s13197-010-0198-7
Bhatt, S., Lee, J., Deutsch, J., Ayaz, H., Fulton, B., and Suri, R. (2018). From food waste to value-added surplus products (vasp): consumer acceptance of a novel food product category. J. Consum. Behav. 17, 57–63. doi: 10.1002/cb.1689
Buchmann, L., Bocker, L., Frey, W., Haberkorn, I., Nyffeler, M., and Mathys, A. (2018). Energy input assessment for nanosecond pulsed electric field processing and its application in a case study with chlorella vulgaris. Innovative Food Sci. Emerg. Technol. 47, 445–453. doi: 10.1016/j.ifset.2018.04.013
Calp, M. (2019). An estimation of personnel food demand quantity for businesses by using artificial neural networks. J. Polytech. 22, 675–686. doi: 10.2339/politeknik.444380
Caporgno, M. P., and Mathys, A. (2018). Trends in microalgae incorporation into innovative food products with potential health benefits. Front. Nutr. 5:58. doi: 10.3389/fnut.2018.00058
Castro-Muñoz, R., and Yáñez-Fernández, J. (2015). Valorization of nixtamalization wastewaters (nejayote) by integrated membrane process. Food Bioprod. Process. 95, 7–18. doi: 10.1016/j.fbp.2015.03.006
Cattaneo, C., Lavelli, V., Proserpio, C., Laureati, M., and Pagliarini, E. (2018). Consumers' attitude towards food by-products: the influence of food technology neophobia, education and information. Int. J. Food Sci. Technol. 54, 679–687. doi: 10.1111/ijfs.13978
Cencic, A., and Chingwaru, W. (2010). The role of functional foods, nutraceuticals, and food supplements in intestinal health. Nutrients 2, 611–625. doi: 10.3390/nu2060611
Clean Meat News Australia (2019). Clean Meat Startups: 10 Lab-Grown Meat Producers to Watch. Available online at: https://www.cleanmeats.com.au/2019/07/24/clean-meat-startups-10-lab-grown-meat-producers-to-watch/ (accessed: October 30, 2019).
Dalkas, G., and Euston, S. R. (2020). “Modelling and computer simulation approaches to understand and predict food structure development: Structuring by gelation and self-association of biomolecules,” in Handbook of Food Structure Development, eds F. Spyropoulos, A. Lazidis and I. Norton, editors. (The Royal Society of Chemistry), 383–401. doi: 10.1039/9781788016155-00383
D'Angelo, G., Hansen, H. N., and Hart, A. J. (2016). Molecular gastronomy meets 3d printing: layered construction via reverse spherification. 3D Printing Addit. Manuf. 3, 153–159. doi: 10.1089/3dp.2016.0024
de Souza, R. R., Bergamasco, R., da Costa, S. C., Feng, X., Faria, S. H. B., and Gimenes, M. L. (2010). Recovery and purification of lactose from whey. Chem. Eng. Process. 49, 1137–1143. doi: 10.1016/j.cep.2010.08.015
Demartini, M., Pinna, C., Tonelli, F., Terzi, S., Sansone, C., and Testa, C. (2018). Food industry digitalization: from challenges and trends to opportunities and solutions. IFAC-PapersOnLine 51, 1371–1378. doi: 10.1016/j.ifacol.2018.08.337
Doyon, M., and Labrecque, J. (2008). Functional foods: a conceptual definition. Br. Food J. 110, 1133–1149. doi: 10.1108/00070700810918036
Duda, A., Adamczak, J., Chelminska, P., Juszkiewicz, J., and Kowalczewski, P. (2019). Quality and nutritional/textural properties of durum wheat pasta enriched with cricket powder. Foods 8:46. doi: 10.3390/foods8020046
Dudley, N., and Alexander, S. (2017). Agriculture and biodiversity: a review. Biodiversity 18, 45–49. doi: 10.1080/14888386.2017.1351892
Ehrlich, P. R., and Holdren, J. P. (1971). Impact of population growth. Science 171, 1212–1217. doi: 10.1126/science.171.3977.1212
Ellen MacArthur Foundation (2019). Cities and Circular Economy for Food. Available online at: https://www.ellenmacarthurfoundation.org/assets/downloads/Cities-and-Circular-Economy-for-Food_280119.pdf (accessed: october 30, 2019).
FAO (2019). The State of Food and Agriculture 2019. Moving Forward on Food Loss and Waste Reduction. Available online at: http://www.fao.org/3/ca6030en/ca6030en.pdf
Feng, P. Y., Wang, B., Liu, D. L., and Yu, Q. (2019). Machine learning-based integration of remotely-sensed drought factors can improve the estimation of agricultural drought in south-eastern australia. Agric. Syst. 173, 303–316. doi: 10.1016/j.agsy.2019.03.015
Floros, J. D., Newsome, R., Fisher, W., Barbosa-Canovas, G. V., Chen, H. D., Dunne, C. P., et al. (2010). Feeding the world today and tomorrow: the importance of food science and technology an ift scientific review. Compr. Rev. Food Sci. Food Saf. 9, 572–599. doi: 10.1111/j.1541-4337.2010.00127.x
Fritsch, C., Staebler, A., Happel, A., Marquez, M. A. C., Aguilo-Aguayo, I., Abadias, M., et al. (2017). Processing, valorization and application of bio-waste derived compounds from potato, tomato, olive and cereals: a review. Sustainability 9:1492. doi: 10.3390/su9081492
Galanakis, C. M. (2020). The food systems in the era of the coronavirus (covid-19) pandemic crisis. Foods 9:523. doi: 10.3390/foods9040523
Gholamipour-Shirazi, A., Kamlow, M. -A. T., Norton, I., and Mills, T. (2020). How to formulate for structure and texture via medium of additive manufacturing-a review. Foods 9:497. doi: 10.3390/foods9040497
Ghoush, M. A., Samhouri, M., Al-Holy, M., and Herald, T. (2008). Formulation and fuzzy modeling of emulsion stability and viscosity of a gum–protein emulsifier in a model mayonnaise system. J. Food Eng. 84, 348–357. doi: 10.1016/j.jfoodeng.2007.05.025
Global Footprint Network (2020a). Earth Overshoot Day. Available online at: https://www.overshootday.org (accessed: June 20, 2020).
Global Footprint Network (2020b). Earth Overshoot Day, Food. Available online at: https://www.overshootday.org/solutions/food/ (accessed: June 6, 2020)
Govindaraj, M. (2015). Is fortification or bio fortification of staple food crops will offer a simple solution to complex nutritional disorder in developing countries? J. Nutr. Food Sci. 5:351. doi: 10.4172/2155-9600.1000351
Green, B. G., and Nachtigal, D. (2015). Temperature affects human sweet taste via at least two mechanisms. Chem. Senses 40, 391–399. doi: 10.1093/chemse/bjv021
Guirado, E., Blanco-Sacristan, J., Rodriguez-Caballero, E., Tabik, S., Alcaraz-Segura, D., Martinez-Valderrama, J., et al. (2021). Mask r-cnn and obia fusion improves the segmentation of scattered vegetation in very high-resolution optical sensors. Sensors 21:320. doi: 10.3390/s21010320
Gunaratne, N. M., Fuentes, S., Gunaratne, T. M., Torrico, D. D., Francis, C., Ashman, H., et al. (2019). Effects of packaging design on sensory liking and willingness to purchase: a study using novel chocolate packaging. Heliyon 5:e01696. doi: 10.1016/j.heliyon.2019.e01696
Guo, Y. H., Fu, Y. S., Hao, F. H., Zhang, X., Wu, W. X., Jin, X. L., et al. (2021). Integrated phenology and climate in rice yields prediction using machine learning methods. Ecol. Indic. 120:106935. doi: 10.1016/j.ecolind.2020.106935
Gupta, P. K., Shree, V., Hiremath, L., and Rajendran, S. (2019). “The use of modern technology in smart waste management and recycling: artificial intelligence and machine learning,” in Recent Advances in Computational Intelligence, eds R. Kumar and U. K. Wiil (Cham: Springer International Publishing), 173–188. doi: 10.1007/978-3-030-12500-4_11
Gutiérrez-Uribe, J. A., Rojas-Garcia, C., Garcia-Lara, S., and Serna-Saldivar, S. O. (2010). Phytochemical analysis of wastewater (nejayote) obtained after lime-cooking of different types of maize kernels processed into masa for tortillas. J. Cereal Sci. 52, 410–416. doi: 10.1016/j.jcs.2010.07.003
Halloran, A., Clement, J., Kornum, N., Bucatariu, C., and Magid, J. (2014). Addressing food waste reduction in denmark. Food Policy 49, 294–301. doi: 10.1016/j.foodpol.2014.09.005
Harrar, V., and Spence, C. (2013). The taste of cutlery: how the taste of food is affected by the weight, size, shape, and colour of the cutlery used to eat it. Flavour 2:21. doi: 10.1186/2044-7248-2-21
Henderson, K., and Loreau, M. (2018). How ecological feedbacks between human population and land cover influence sustainability. PLoS Comput. Biol. 14:e1006389. doi: 10.1371/journal.pcbi.1006389
Hirvonen, K., Bai, Y., Haedey, D., and Masters, W. A. (2020). Affordability of the eat– lancet reference diet: a global analysis. Lancet Glob Health 8:e59–e66. doi: 10.1016/S2214-109X(19)30447-4
Hoek, A. C., Luning, P. A., Weijzen, P., Engels, W., Kok, F. J., and de Graaf, C. (2011). Replacement of meat by meat substitutes. a survey on person- and product-related factors in consumer acceptance. Appetite 56, 662–673. doi: 10.1016/j.appet.2011.02.001
Huang, C., Lai, C., Wu, X., Huang, Y., He, J., Huang, C., et al. (2017). An integrated process to produce bio-ethanol and xylooligosaccharides rich in xylobiose and xylotriose from high ash content waste wheat straw. Bioresour. Technol. 241, 228–235. doi: 10.1016/j.biortech.2017.05.109
Jones, J. M. (2014). Codex-aligned dietary fiber definitions help to bridge the 'fiber gap'. Nutr. J. 13:34. doi: 10.1186/1475-2891-13-34
Jørgensen, S., and Pedersen, L. J. T. (2018). “The circular rather than the linear economy,” in Restart Sustainable Business Model Innovation, eds S. Jørgensen and L. J. T. Pedersen (London: Palgrave Macmillan), 103–120. doi: 10.1007/978-3-319-91971-3_8
Jribi, S., Ben Ismail, H., Doggui, D., and Debbabi, H. (2020). Covid-19 virus outbreak lockdown: what impacts on household food wastage? Environ. Dev. Sustain. 22, 3939–3955. doi: 10.1007/s10668-020-00740-y
Jurgilevich, A., Birge, T., Kentala-Lehtonen, J., Korhonen-Kurki, K., Pietikainen, J., Saikku, L., et al. (2016). Transition towards circular economy in the food system. Sustainability 8:69. doi: 10.3390/su8010069
Kadam, S. U., Tiwari, B. K., and O'Donnell, C. P. (2013). Application of novel extraction technologies for bioactives from marine algae. J. Agric. Food Chem. 61, 4667–4675. doi: 10.1021/jf400819p
Kamath, R. (2018). Food traceability on blockchain: walmart's pork and mango pilots with ibm. J. Br. Blockchain Assoc. 1, 47–53. doi: 10.31585/jbba-1-1-(10)2018
Kamilaris, A., Fonts, A., and Prenafeta-Bold?, F. X. (2019). The rise of blockchain technology in agriculture and food supply chains. Trends Food Sci. Technol. 91 640–652. doi: 10.1016/j.tifs.2019.07.034
Kandra, P., Challa, M. M., and Jyothi, H. K. (2012). Efficient use of shrimp waste: present and future trends. Appl. Microbiol. Biotechnol. 93, 17–29. doi: 10.1007/s00253-011-3651-2
Karayannakidis, P. D., and Zotos, A. (2016). Fish processing by-products as a potential source of gelatin: a review. J. Aquat. Food Product Technol. 25, 65–92. doi: 10.1080/10498850.2013.827767
Kerch, G. (2015). The potential of chitosan and its derivatives in prevention and treatment of age-related diseases. Mar. Drugs 13, 2158–2182. doi: 10.3390/md13042158
Khan, H., and Lee, R. (2020). Does packaging influence taste and quality perceptions across varying consumer demographics? Food Qual. Prefer. 84:103932. doi: 10.1016/j.foodqual.2020.103932
La Barbera, F., Verneau, F., Amato, M., and Grunert, K. (2018). Understanding westerners' disgust for the eating of insects: the role of food neophobia and implicit associations. Food Qual. Prefer. 64 120–125. doi: 10.1016/j.foodqual.2017.10.002
Lagrange, V., Whitsett, D., and Burris, C. (2015). Global market for dairy proteins. J. Food Sci. 1, A16–22. doi: 10.1111/1750-3841.12801
Loutfi, A., Coradeschi, S., Mani, G. K., Shankar, P., and Rayappan, J. B. B. (2015). Electronic noses for food quality: a review. J. Food Eng. 144, 103–111. doi: 10.1016/j.jfoodeng.2014.07.019
Lowe, M. R., and Butryn, M. L. (2007). Hedonic hunger: a new dimension of appetite? Physiol. Behav. 91, 432–439. doi: 10.1016/j.physbeh.2007.04.006
Lynch, H., Johnston, C., and Wharton, C. (2018). Plant-based diets: considerations for environmental impact, protein quality, and exercise performance. Nutrients 10:1841. doi: 10.3390/nu10121841
Mazloumian, A., Rosenthal, M., and Gelke, H. (2020). Deep Learning for Classifying Food Waste. arXiv preprint (Ithaca, NY).
McKee, L. H., and Latner, T. A. (2000). Underutilized sources of dietary fiber: a review. Plant Foods Hum. Nutr. 55, 285–304. doi: 10.1023/A:1008144310986
Mela, D. J. (2006). Eating for pleasure or just wanting to eat? Reconsidering sensory hedonic responses as a driver of obesity. Appetite 47, 10–17. doi: 10.1016/j.appet.2006.02.006
Melgar-Lalanne, G., Hernández-Álvarez, A. J., and Salinas-Castro, A. (2019). Edible insects processing: traditional and innovative technologies. Compr. Rev. Food Sci. Food Saf. 18, 1166–1191. doi: 10.1111/1541-4337.12463
Merli, R., Preziosi, M., and Acampora, A. (2018). How do scholars approach the circular economy? A systematic literature review. J. Cleaner Prod. 178, 703–722. doi: 10.1016/j.jclepro.2017.12.112
Mezzenga, R., Bo Lee, W., and Fredrickson, G. H. (2006). Design of liquid-crystalline foods via field theoretic computer simulations. Trends Food Sci. Technol. 17, 220–226. doi: 10.1016/j.tifs.2005.11.009
Monteiro, A., Paquincha, D., Martins, F., Queiros, R. P., Saraiva, J. A., Svarc-Gajic, J., et al. (2018). Liquid by-products from fish canning industry as sustainable sources of omega3 lipids. J. Environ. Manage. 219, 9–17. doi: 10.1016/j.jenvman.2018.04.102
Moore, D., Cranston, G., Reed, A., and Galli, A. (2012). Projecting future human demand on the earth's regenerative capacity. Ecol. Indic. 16, 3–10. doi: 10.1016/j.ecolind.2011.03.013
Ni, Q., Xu, G., Wang, Z., Gao, Q., Wang, S., and Zhang, Y. (2012). Seasonal variations of the antioxidant composition in ground bamboo sasa argenteastriatus leaves. Int. J. Mol. Sci. 13, 2249–2262. doi: 10.3390/ijms13022249
Nicola, M., Alsafi, Z., Sohrabi, C., Kerwan, A., Al-Jabir, A., Iosifidis, C., et al. (2020). The socio-economic implications of the coronavirus pandemic (covid-19): a review. Int. J. Surg. 78, 185–193. doi: 10.1016/j.ijsu.2020.04.018
Nicolotti, L., Mall, V., and Schieberle, P. (2019). Characterization of key aroma compounds in a commercial rum and an australian red wine by means of a new sensomics-based expert system (sebes)-an approach to use artificial intelligence in determining food odor codes. J. Agric. Food Chem. 67, 4011–4022. doi: 10.1021/acs.jafc.9b00708
Nirmala, C., Bisht, M. S., Bajwa, H. K., and Santosh, O. (2018). Bamboo: A rich source of natural antioxidants and its applications in the food and pharmaceutical industry. Trends Food Sci. Technol. 77, 91–99. doi: 10.1016/j.tifs.2018.05.003
Nordlund, E., Lille, M., Silventoinen, P., Nygren, H., Seppanen-Laakso, T., Mikkelson, A., et al. (2018). Plant cells as food - a concept taking shape. Food Res. Int. 107, 297–305. doi: 10.1016/j.foodres.2018.02.045
Okajima, K., Ueda, J., and Spence, C. (2013). Effects of visual texture on food perception. J. Vis. 13, 1078–1078. doi: 10.1167/13.9.1078
Olsen, N. V. (2015). Design thinking and food innovation. Trends Food Sci. Technol. 41, 182–187. doi: 10.1016/j.tifs.2014.10.001
Olsen, P., Borit, M., and Syed, S. (2019). Applications, Limitations, Costs, and Benefits Related to the Use of Blockchain Technology in the Food Industry. Nofima rapportserie. Available online at: http://hdl.handle.net/11250/2586121
O'Riordan, K., Fotopoulou, A., and Stephens, N. (2017). The first bite: imaginaries, promotional publics and the laboratory grown burger. Public Underst. Sci. 26, 148–163. doi: 10.1177/0963662516639001
Panesar, R., Kaur, S., and Panesar, P. S. (2015). Production of microbial pigments utilizing agro-industrial waste: a review. Curr. Opin. Food Sci. 1, 70–76. doi: 10.1016/j.cofs.2014.12.002
Papargyropoulou, E., Lozano, R., Steinberger, J. K., Wright, N., and bin Ujang, Z. (2014). The food waste hierarchy as a framework for the management of food surplus and food waste. J. Clean. Prod. 76, 106–115. doi: 10.1016/j.jclepro.2014.04.020
Pennanen, K., Närväinen, J., Vanhatalo, S., Raisamo, R., and Sozer, N. (2020). Effect of virtual eating environment on consumers' evaluations of healthy and unhealthy snacks. Food Qual. Prefer. 82:103871. doi: 10.1016/j.foodqual.2020.103871
Pentikäinen, S., Tanner, H., Karhunen, L., Kolehmainen, M., Poutanen, K., and Pennanen, K. (2019). Mobile phone app for self-monitoring of eating rhythm: field experiment. JMIR mHealth uHealth 7:e11490. doi: 10.2196/11490
Perry, R. A., Mallan, K. M., Koo, J., Mauch, C. E., Daniels, L. A., and Magarey, A. M. (2015). Food neophobia and its association with diet quality and weight in children aged 24 months: a cross sectional study. Int. J. Behav. Nutr. Phys. Act. 12:13. doi: 10.1186/s12966-015-0184-6
Piqueras-Fiszman, B., and Spence, C. (2011). Do the material properties of cutlery affect the perception of the food you eat? An exploratory study. J. Sens. Stud. 26, 358–362. doi: 10.1111/j.1745-459X.2011.00351.x
Pitkänen, L., Heinonen, M., and Mikkonen, K. S. (2018). Safety considerations of phenolic-rich plant polysaccharides for food use: case study on softwood galactoglucomannan. Food Funct. 9, 1931–1943. doi: 10.1039/C7FO01425B
Plasek, B., Lakner, Z., and Temesi, A. (2020). Factors that influence the perceived healthiness of food-review. Nutrients 12:1881. doi: 10.3390/nu12061881
Plazzotta, S., Manzocco, L., and Nicoli, M. C. (2017). Fruit and vegetable waste management and the challenge of fresh-cut salad. Trends Food Sci. Technol. 63, 51–59. doi: 10.1016/j.tifs.2017.02.013
Post, M. J. (2014). Cultured beef: medical technology to produce food. J. Sci. Food Agric. 94, 1039–1041. doi: 10.1002/jsfa.6474
Poutanen, K., Nordlund, E., Paasi, J., Vehmas, K., and Åkerman, M. (2017). Food Economy 4.0. VTT - Technical Research Center of Finland Ltd. Available online at: https://www.vtt.fi/inf/pdf/visions/2017/V10.pdf
Priyadarshani, I., and Rath, B. (2012). Commercial and industrial applications of micro algae—a review. J. Algal Biomass Util. 3, 89–100.
Quested, T., and Johnson, H. (2009). Household Food and Drink Waste in the Uk. WRAP. Available online at: https://wrap.org.uk/resources/report/household-food-and-drink-waste-uk-2009
Raheem, D., Shishaev, M., and Dikovitsky, V. (2019). Food system digitalization as a means to promote food and nutrition security in the barents region. Agriculture 9:168. doi: 10.3390/agriculture9080168
Rahmanian, N., Jafari, S. M., and Galanakis, C. M. (2014). Recovery and removal of phenolic compounds from olive mill wastewater. J. Am. Oil Chem. Soc. 91, 1–18. doi: 10.1007/s11746-013-2350-9
Ramos-Elorduy, J. (2009). Anthropo-entomophagy: cultures, evolution and sustainability. Entomol. Res. 39, 271–288. doi: 10.1111/j.1748-5967.2009.00238.x
Ranasinghe, N., Tolley, D., Nguyen, T. N. T., Yan, L., Chew, B., and Do, E. Y. (2019). Augmented flavours: modulation of flavour experiences through electric taste augmentation. Food Res. Int. 117, 60–68. doi: 10.1016/j.foodres.2018.05.030
Reinoso Carvalho, F., Velasco, C., van Ee, R., Leboeuf, Y., and Spence, C. (2016). Music influences hedonic and taste ratings in beer. Front. Psychol. 7:636. doi: 10.3389/fpsyg.2016.00636
Rizzato, M., Di Dio, C., Fasano, F., Gilli, G., Marchetti, A., and Sensidoni, A. (2016). Is food desirability affected by social interaction? Food Qual. Prefer. 50, 109–116. doi: 10.1016/j.foodqual.2016.02.005
Rockenfeller, P., and Madeo, F. (2010). Ageing and eating. Biochim. Biophys. Acta 1803, 499–506. doi: 10.1016/j.bbamcr.2010.01.001
Roos, Y. H. (2020). Water and pathogenic viruses inactivation—food engineering perspectives. Food Eng. Rev. 12, 251–267. doi: 10.1007/s12393-020-09234-z
Ryan, C., Gúeret, C., Berry, D., Corcoran, M., Keane, M. T., and Mac Namee, B. (2021). Predicting Illness for a Sustainable Dairy Agriculture: Predicting and Explaining the Onset of Mastitis in Dairy Cows. arXiv preprint.
Sabaté, J., and Soret, S. (2014). Sustainability of plant-based diets: back to the future. Am. J. Clin. Nutr. 100, 476S–482S. doi: 10.3945/ajcn.113.071522
Saini, A., Panesar, P. S., and Bera, M. B. (2019). Valorization of fruits and vegetables waste through green extraction of bioactive compounds and their nanoemulsions-based delivery system. Bioresour. Bioprocess. 6:26. doi: 10.1186/s40643-019-0261-9
Sakagami, H., Kushida, T., Oizumi, T., Nakashima, H., and Makino, T. (2010). Distribution of lignin-carbohydrate complex in plant kingdom and its functionality as alternative medicine. Pharmacol. Ther. 128, 91–105. doi: 10.1016/j.pharmthera.2010.05.004
Salah, K., Rehman, M. H. U., Nizamuddin, N., and Al-Fuqaha, A. (2019). Blockchain for ai: review and open research challenges. IEEE Access 7, 10127–10149. doi: 10.1109/ACCESS.2018.2890507
Sasson, A. (2012). Food security for africa: an urgent global challenge. Agric. Food Secur. 1:2. doi: 10.1186/2048-7010-1-2
Sathasivam, R., Radhakrishnan, R., Hashem, A., and Abd Allah, E. F. (2019). Microalgae metabolites: a rich source for food and medicine. Saudi J. Biol. Sci. 26, 709–722. doi: 10.1016/j.sjbs.2017.11.003
Siegrist, M., and Hartmann, C. (2020). Consumer acceptance of novel food technologies. Nat. Food 1, 343–350. doi: 10.1038/s43016-020-0094-x
Siró, I., Kapolna, E., Kapolna, B., and Lugasi, A. (2008). Functional food. Product development, marketing and consumer acceptance-a review. Appetite 51, 456–467. doi: 10.1016/j.appet.2008.05.060
Smithers, G. W. (2008). Whey and whey proteins—from ‘gutter-to-gold'. Int. Dairy J. 18, 695–704. doi: 10.1016/j.idairyj.2008.03.008
Springmann, M., Mason-D'Croz, D., Robinson, S., Garnett, T., Godfray, H. C., Gollin, D., et al. (2016). Global and regional health effects of future food production under climate change: a modelling study. Lancet 387, 1937–1946. doi: 10.1016/S0140-6736(15)01156-3
Stephens, N., Di Silvio, L., Dunsford, I., Ellis, M., Glencross, A., and Sexton, A. (2018). Bringing cultured meat to market: technical, socio-political, and regulatory challenges in cellular agriculture. Trends Food Sci. Technol. 78, 155–166. doi: 10.1016/j.tifs.2018.04.010
Sun, J., Zhou, W. B., Huang, D. J., Fuh, J. Y. H., and Hong, G. S. (2015). An overview of 3d printing technologies for food fabrication. Food Bioprocess Technol. 8, 1605–1615. doi: 10.1007/s11947-015-1528-6
Tolba, R., Wu, G., and Chen, A. (2011). Adsorption of dietary oils onto lignin for promising pharameutical and nutritional applications. Bioresources 6, 1322–1335.
Toldrá, F., Aristoy, M. C., Mora, L., and Reig, M. (2012). Innovations in value-addition of edible meat by-products. Meat Sci. 92, 290–296. doi: 10.1016/j.meatsci.2012.04.004
Torres-Tiji, Y., Fields, F. J., and Mayfield, S. P. (2020). Microalgae as a future food source. Biotechnol. Adv. 41:107536. doi: 10.1016/j.biotechadv.2020.107536
van Huis, A., and Oonincx, D. G. A. B. (2017). The environmental sustainability of insects as food and feed. A review. Agron. Sustain. Dev. 37, 43. doi: 10.1007/s13593-017-0452-8
Vigani, M., Parisi, C., Rodriguez-Cerezo, E., Barbosa, M. J., Sijtsma, L., Ploeg, M., et al. (2015). Food and feed products from micro-algae: market opportunities and challenges for the eu. Trends Food Sci. Technol. 42, 81–92. doi: 10.1016/j.tifs.2014.12.004
Wang, Q. J., Mielby, L. A., Junge, J. Y., Bertelsen, A. S., Kidmose, U., Spence, C., et al. (2019). The role of intrinsic and extrinsic sensory factors in sweetness perception of food and beverages: a review. Foods 8:211. doi: 10.3390/foods8060211
Weinrich, R. (2019). Opportunities for the adoption of health-based sustainable dietary patterns: a review on consumer research of meat substitutes. Sustainability 11:4028. doi: 10.3390/su11154028
Wells, M. L., Potin, P., Craigie, J. S., Raven, J. A., Merchant, S. S., Helliwell, K. E., et al. (2017). Algae as nutritional and functional food sources: revisiting our understanding. J. Appl. Phycol. 29, 949–982. doi: 10.1007/s10811-016-0974-5
Wieben, E. (2017). Food loss and Waste and the Linkage to Global Ecosystems. Food and Agriculture Organization of the United Nations. Available online at: http://www.fao.org/publications/card/en/c/7fed720c-18e6-4be4-83d2-385b05b79ace/
Xiao, J.-R., Chung, P.-C., Wu, H.-Y., Phan, Q.-H., Yeh, J.-L. A., and Hou, M. T.-K. (2021). Detection of strawberry diseases using a convolutional neural network. Plants 10:31. doi: 10.3390/plants10010031
Xiao, Y., Chen, C., Wang, B., Mao, Z., Xu, H., Zhong, Y., et al. (2018). In vitro digestion of oil-in-water emulsions stabilized by regenerated chitin. J. Agric. Food Chem. 66, 12344–12352. doi: 10.1021/acs.jafc.8b03873
Xu, M., Wang, J., and Zhu, L. (2019). The qualitative and quantitative assessment of tea quality based on e-nose, e-tongue and e-eye combined with chemometrics. Food Chem. 289, 482–489. doi: 10.1016/j.foodchem.2019.03.080
Yan, K. S., and Dando, R. (2015). A crossmodal role for audition in taste perception. J. Exp. Psychol. 41, 590–596. doi: 10.1037/xhp0000044
Zampollo, F. (2020). Food Design and Food Design Thinking. Available online at: http://francesca-zampollo.com/category/uncategorized/
Keywords: food loss and food waste, circular economy, food production and food security, food structure design, new ingredients, digitalization, food design
Citation: Valoppi F, Agustin M, Abik F, Morais de Carvalho D, Sithole J, Bhattarai M, Varis JJ, Arzami ANAB, Pulkkinen E and Mikkonen KS (2021) Insight on Current Advances in Food Science and Technology for Feeding the World Population. Front. Sustain. Food Syst. 5:626227. doi: 10.3389/fsufs.2021.626227
Received: 30 November 2020; Accepted: 23 September 2021;
Published: 21 October 2021.
Edited by:
Kathleen L. Hefferon, Cornell University, United StatesReviewed by:
Shalini Gaur Rudra, Indian Agricultural Research Institute, IndiaGabriele Carullo, University of Siena, Italy
Copyright © 2021 Valoppi, Agustin, Abik, Morais de Carvalho, Sithole, Bhattarai, Varis, Arzami, Pulkkinen and Mikkonen. This is an open-access article distributed under the terms of the Creative Commons Attribution License (CC BY). The use, distribution or reproduction in other forums is permitted, provided the original author(s) and the copyright owner(s) are credited and that the original publication in this journal is cited, in accordance with accepted academic practice. No use, distribution or reproduction is permitted which does not comply with these terms.
*Correspondence: Fabio Valoppi, fabio.valoppi@helsinki.fi