- 1Unidad de Salud y Bienestar, Universidad Católica de Cuenca, Av. Las Américas y Calle Humboldt, Cuenca, Ecuador
- 2Centro de Investigación, Innovación y Transferencia de Tecnología (CIITT), Universidad Católica de Cuenca, Ricaurte, Ecuador
- 3Facultad de Ciencias Agropecuarias, Universidad de Cuenca, Cuenca, Ecuador
As in other Andean countries, smallholder agriculture is the base that supports food and nutrient security in Ecuador. Ironically, in spite of their importance, the development of context-appropriate technologies for these farming systems remains still at its infancy. Today, most smallholders in the Ecuadorian Andes practice a type of hybrid agriculture that merges traditional local practices and modern technologies. This mixture of traditional and “modern” conventional technologies does not always result in resource-efficient sustainable practices. Although they represent only part of a global solution, microbial-based technologies offer a great potential to improve the functioning of smallholder farms in the Ecuadorian Andes. From nutrient cycling to biocontrol and plant growth promotion, microbial technology applications match existing needs for technology development in these systems; however, as in many cases, knowledge gaps and context-adapted implementation are some of the challenges that slow down the spreading and efficient use of these technologies. Here we offer a review of the efforts made as of today to characterize, develop and test microbial-based technologies that could boost smallholder Andean agriculture with a particular focus in the Ecuadorian context. We also propose potential lines of action to increase or accelerate the impact of these technologies.
Introduction
Smallholder farming plays a pivotal in the economy and, more importantly, the food security of Ecuador. However, in spite of its relevance, this sector has not received enough attention in terms of developing technological innovations that could improve their sustainability, of which, microbial technologies are among the most promising due to the high diversity of microbial resources in Ecuador. Furthermore, several microbe-based technologies have already been adopted by some smallholders, offering a platform from where to launch new innovations. Nonetheless, Ecuadorian smallholders are a very heterogeneous group, which precludes the proposal of one-size-fits-all innovations demanding instead solutions tailored to each biophysical and social context that will require a better characterization of existing microbial diversity. In this minireview, we have focused our attention in the group that Berdegué and Escobar (2002) define as “subsistence and transitional smallholder farms” since innovations in these farms show the greatest potential to generate life-changing impacts (Berdegué and Fuentealba, 2011). Furthermore, we have focused this review in subsistence and smallholder farms in the mountainous region of the country, since they represent the majority of smallholders in Ecuador and grow a greater diversity of products intended for internal consumption, thus having a significant contribution to the country's food and nutrient security.
Smallholder Farming in the Ecuadorian Andes: Setting the Context
Due to the extreme diversity of smallholdings (Figure 1), a simple definition of these system is unrealistic; however, a common feature of these systems in Latin America, as proposed by Berdegué and Fuentealba (2011) is the strong reliance of these systems on family administration and labor, alongside a relative small size for their local context, in other words, the definition of “smallholder farming” overlaps considerably that of “family farming” in the Latin American context. Based on the endowment of assets of smallholder farms, Berdegué and Escobar (2002) classified these farms three different groups, namely, subsistence-, transitional- and consolidated smallholder farms. This classification is practical and useful to describe the actual diversity of smallholdings in the Ecuadorian context. Using this classification, and data from the last agricultural census in Ecuador, ~88% (739,952) of all farms in Ecuador could be considered as smallholder farms (Soto Baquero et al., 2007). Most of these farms (58%) were located in the Andean region, with almost 99% of them falling within the category of transitional (~33%) or subsistence (~66%) farms. Succinctly, the main characteristic of these two types of farm is that both show some level of limitation in terms of their resource base (e.g., soils, topography, and weather) that affects their productivity, being this limitation more marked in subsistence farms. It is noteworthy to mention that, even though subsistence farms have been the focus of many agricultural development programs; it is actually transitional farms, the ones that show greater potential for agricultural development programs to boost sustainable livelihoods and inclusive economic growth (Berdegué and Fuentealba, 2011).
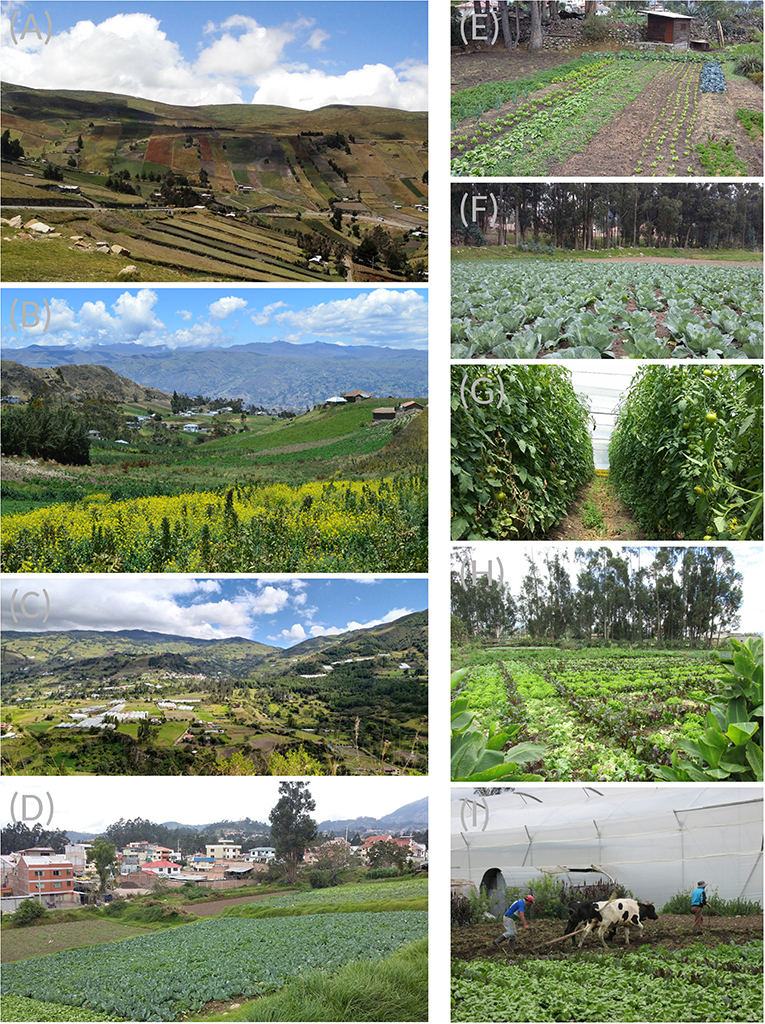
Figure 1. Diversity in smallholder farms in the Ecuadorian Andes. Typical landscapes of regions were smallhoder farming is practiced: (A,B) Farms at high elevations (potato, grains, pulses, pastures, other tuber/root crops), panel (B) shows a typical rotation consisting in pasture (background), potato, fallow and fava beans (foreground), (C) Farms in lower mountain slopes and valleys (vegetables, fruits, greenhouse horticulture), (D) Urban and periurban farms (vegetables). Panels (E–I) show representative subsistence (E) and transitional (F) systems, greenhouse (G) and diversified agroecological (H) systems and the combination of traditional (oxen plow) and modern technologies (greenhouses) in some of these farms (I).
The production of smallholdings in the Ecuadorian Andes consist primarily on vegetables, fruits, roots/tuber crops, grains, pulses, and pastures (Soto Baquero et al., 2007). Typically, these farms are diversified systems in which horticultural, agronomic, forest, and medicinal species are co-cultivated. The diversity in some of these farms is so rich, that they contribute significantly to the conservation of agrobiodiversity (Wong and Ludeña, 2006; Oyarzun et al., 2013). Nonetheless, it must be noted, that often this agrobiodiversity is largely composed of many introduced species, with native crops occupying only a small share of the cultivated area in these farms (Oyarzun et al., 2013). Furthermore, space is preferentially allocated to a few dominant, commercial crops such as potatoes, pulses, or corn, with much less space devoted to traditional or native crops (Oyarzun et al., 2013; Córdova et al., 2018). Smallholder vegetable farms are commonly managed intensively using polycultures (3–4 simultaneous crops) with almost no fallow periods (Zea et al., 2020). Some smallholder farms consist almost exclusively of passively ventilated greenhouses, with even more intense management and greater dependence on external inputs.
Geographically, smallholder agriculture is distributed along production belts of different altitudes, with fruit and vegetable farms located near the bottom of valleys, and grain, pulses and tuber/root crops farms located at the highest altitudes up to 3,800 as described by Harden (1988) for the Ambato river basin, an agriculturally important basin in Ecuador. In terms of technology, smallholder farms are highly heterogeneous, although a syncretism of traditional and “modern” technology is a common feature in most cases. The adoption of “modern” production technologies is represented primarily by the use of synthetic inputs (fertilizers, pesticides) and mechanization. Interestingly, a revival of the use of traditional, and introduction of alternative technologies has been apparent in recent years.
Bioprospecting Potentially Useful Microbes for Mountain Agriculture
In the Andean context, several native crops and soils have been prospected for useful plant-growth promoting microorganisms (PGPM) (Table 1). Among those, potato is -by far- the most studied. This tuber has been grown for millennia by Andean people and is the most important staple crop in the region. Unfortunately, although in recent decades chemical fertilizers and pesticides have been applied to counteract the effect of unfavorable soil conditions and fungal pathogens, potato production yields remain low in the region (Aubron et al., 2009). In view of this situation, several quests have been conducted to identify potato-beneficial microbes, of which, perhaps the most important have been those conducted by the International Potato Center and the VALORAM Consortium (Oswald et al., 2007, 2010; Vélez et al., 2008; Calvo et al., 2009, 2010; Oswald and Calvo, 2009; Calvo and Zúñiga, 2010; Ghyselinck et al., 2013; Velivelli et al., 2015).
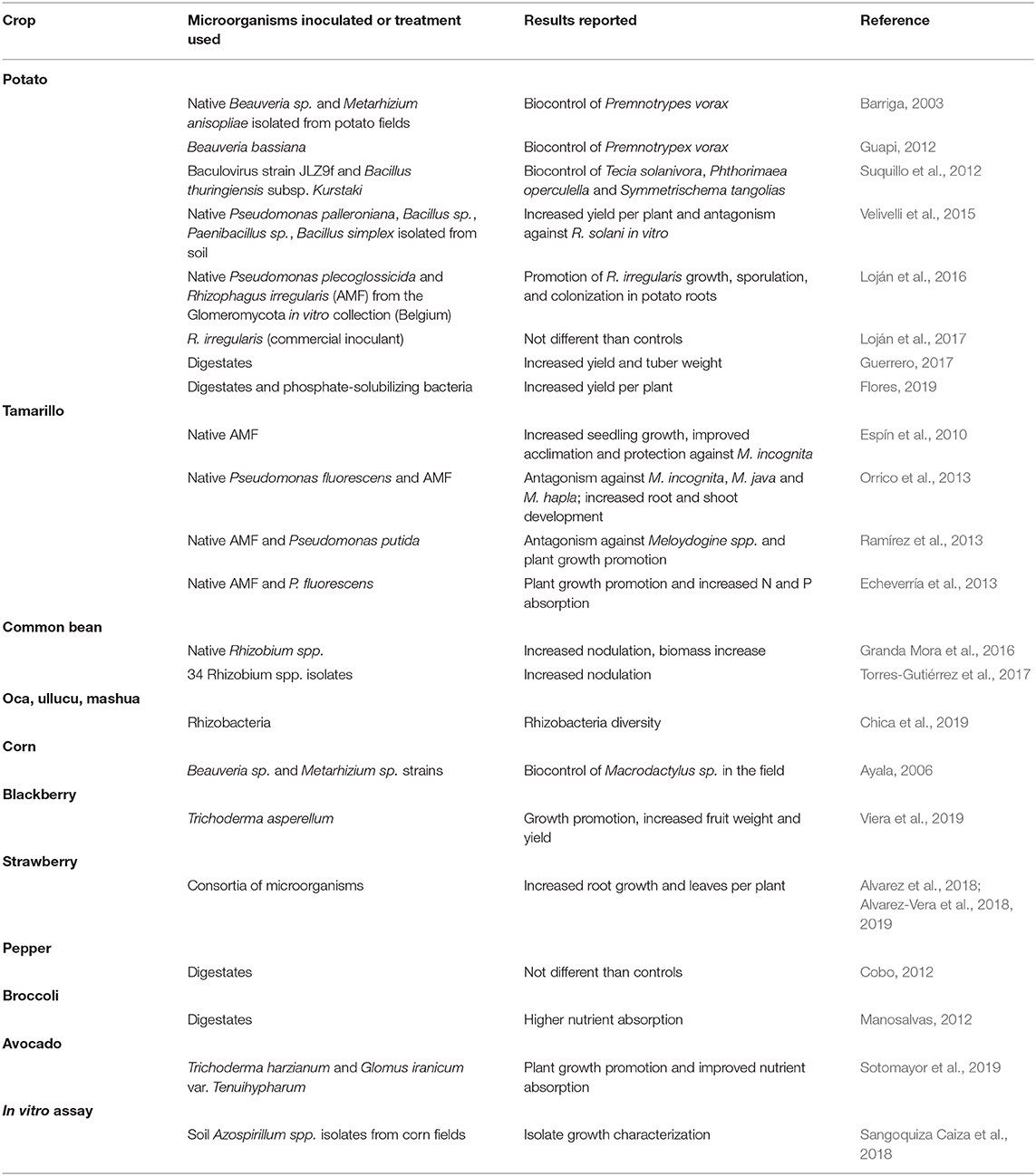
Table 1. Studies reporting results of the prospection and evaluation of microbial technologies in the Ecuadorian Andes.
For instance, a study conducted by Velivelli et al. (2015) in Ecuador, showed that inoculation of potato fields with native soil bacteria significantly raised yields of potato by increasing the number of tubers per plant. Some strains identified as Pseudomonas palleroniana, Bacillus sp., Paenibacillus sp., and Bacillus simplex also showed antagonism against Rhizoctonia solani in vitro, even though this phenotype could not be clearly related to the incidence or severity of disease symptoms on tubers.
Another two studies conducted in the Ecuadorian Andes by members of the VALORAM consortium evaluated the inoculation with Rhizophagus irregularis, an arbuscular micorrhizal fungus widely used as a biofertilizer (Berruti et al., 2014), on potato. In the first of these studies, Loján et al. (2016) found that several rhizobacteria isolated from the potato rizosphere behaved as antagonists to the establishment of R. irregularis mycorrhizal associations with potato, although one isolate (namely Pseudomonas plecoglossicida R-67094) promoted R. irregularis growth, during the pre-symbiotic phase of the fungus. The same strain, entrapped within alginate beads, behaved as a mycorrhiza-helper bacteria, inducing R. irregularis sporulation while improving potato root colonization. In the second study (Loján et al., 2017), four commercial products containing R. irregularis were inoculated in potato under field; however, none of them had any effect on potato yields, reportedlydue to poor establishment of the AMF in the rhizosphere of potato plants.
Other native Andean tuber crops (ATC) have also been prospected for rhizosphere-associated microbes. Recently, Chica et al. (2019) used high throughput sequence analysis of 16S rRNA genes to describe the bacterial diversity of rhizosphere soils associated to oca (Oxalis tuberosa), ullucu (Ullucus tuberosus), and mashua (Tropaeolum tuberosum). Unfortunately, this study was not followed by plant-growth promotion assays.
Another important crop, native to the Andean region is Phaseolus vulgaris L., the common bean (Tohme et al., 1995). This legume contributes minerals and vitamins to the human diet, and also constitutes a major source of dietary protein (Sathe, 2002; Broughton et al., 2003). Since P. vulgaris is commonly associated to root-nodule forming bacteria (i.e., rhizobia), it fixes atmospheric nitrogen biologically (López-Guerrero et al., 2012). In the Andean region, some taxonomic studies have been conducted by Ribeiro et al. (2013) allowed the discovery of three novel Rhizobium lineages in Ecuador, with one of them dominant in beans from this country. Further, the genetic diversity of Agrobacterium strains colonizing the nodules of this legume has been recently shown to be high, and it was proposed that members of this bacterial genus might contribute to plant growth (Delamuta et al., 2020).
Even though the symbiotic association between Rhizobium spp. and Phaseolus has been thoroughly studied in the past, there is still much to learn concerning the possibility of using these bacteria to promote growth and development of the legume. Toward this aim, Granda Mora et al. (2016) isolated six native strains of Rhizobium from P. vulgaris (cultivar Mantequilla) in Southern Ecuador, and showed their promoting effect on legume nodulation, biomass, nitrogen fixation, and symbiotic efficiency in a greenhouse experiment. These results encouraged the authors to propose the possibility of using these strains to develop bioinoculants for P. vulgaris bioinoculants. One year later, the same group isolated, characterized and identified 34 Rhizobium isolates from plants grown in Southern Ecuador (Torres-Gutiérrez et al., 2017). The strains belonged to nine species and were both phenotypically and genetically diverse; most of them promoted nodulation and nitrogen fixation, but the results were highly variable. Several strains did also produce high amounts of indolacetic acid, a well-known auxin involved in plant cell division/differentiation and vascular bundle formation (Theunis et al., 2004). Once again, the authors claimed on the utility of such native Rhizobium strains to develop biofertilizers, but insisted on conducting more trials.
Corn is a very important cereal grown in the Andean mountains. In 2018, Sangoquiza Caiza and et al. reported on some phenotypic and physiological characteristics of three Azospirillum strains isolated from the rhizosphere of corn plants grown at more than 2,000 m.a.s.l. in the Ecuadorian mountains. The authors claimed on the biotechnological utility of such strains, without presenting any further details.
In order to prospect for potentially useful PGPM for agricultural purposes, Alvarez et al. (2018); Alvarez-Vera et al. (2018, 2019) tried a different experimental approach. Instead of monitoring the promoting effect of one microbial isolate at a time, they assembled together several consortia of beneficial microorganisms, originally isolated from different organs (stems, leaves, and roots) of native plant species in Southern Ecuador. The list of species included coffee (Coffea arabica L.), plantain (Musa paradisiaca L.), chamomile (Matricaria chamomilla L.), mugwort (Artemisia vulgaris), and rue (Ruta graveolens L.), among several others. The plants—and their corresponding microbes—were grouped following the altitude at which they were grown. The microbial strains included yeasts (Saccharomyces sp., Kloeckera sp., and Rhodotorula sp.), bacilli and lactobacilli (Bacillus subtilis/amyloliquefaciens, Lactobacillus delbrueckii, and L. plantarum), and streptomycetes (Streptomyces sanglieri, S. lushanensis, S. griseorubens, S. thermocarboxydus, and S. bungoensis). Different consortia were prepared by combining isolates from each altitude, and inoculated to strawberries (Fragaria sp.) grown in the field. The results were highly heterogeneous: whereas some consortia increased in a significant way the number of leaves per plant, as well their root growth, others did not produce any detectable effect. However, it became evident that the approach followed by the researchers was effective in isolating potentially useful PGPM.
Studies aimed at improving fruit tree growth are really scarce in the Andean context with only a few reports on Tamarillo (Solanum betaceum), commonly known as tree tomato. This species, native to the Andes, is grown nowadays worldwide in “exotic” countries like Australia, New Zealand, and India (Bohs, 1989; Carrillo-Perdomo et al., 2015). Tamarillo is a rich source of vitamins and organic acids, and is also consumed as a potent antioxidant (Vasco et al., 2009; Acosta-Quezada et al., 2015). The tree is usually grown in small orchards following traditional management systems; unfortunately, its productivity is frequently challenged by diseases like anthracnose and powdery mildew (Tamayo, 2001), but also by herbivore nematodes (Prohens and Nuez, 2000).
To fight against the deleterious effect of such pathogens, some Ecuadorian researchers focused on antagonistic bacterial and fungal species, native to these lands. For instance, native Ecuadorian isolates of arbuscular mycorrhizal fungi (AMF) were shown to promote Tamarillo plantlet development and acclimation, in addition to protecting them from Meloidogyne incognita infection (Espín et al., 2010). Further, Orrico et al. (2013) reported on the protection of Tamarillo tree by native Pseudomonas fluorescens strains and AMF. The strains, isolated from organically grown trees were mixed in a biopesticide formulation, and shown to antagonize Meloidogyne incognita, M. java and M. hapla, in addition of reducing the formation of root-knots in the trees. Similar results were published the same year by Ramírez et al. (2013) and by Echeverría et al. (2013), but this time by either combining native strains of both AMF and Pseudomonas putida, or native AMF and P. fluorescens, respectively.
Experiences With Microbial Technologies in Smallholder Andean Agriculture
Adoption of microbial technologies among Ecuadorian smallholders is not widespread. Some of the probable causes for this are (i) the greater perceived convenience, habituation, and immediate effectiveness of synthetic inputs, (ii) mixed experiences with microbial technologies tested, (iii) more complex management skills required for their implementation, and (iv) the limited availability of microbial based products along with their price. In spite of their current limited adoption, interest in these technologies has been growing steadily aided by the work of government agencies, farmer's associations and NGOs. While adoption of microbial technologies by smallholders, as of today, has been focused mostly on a few well-known groups of microorganisms and microbe-derived products, a great potential for developing new microbe-based technologies from local biodiversity remains high as this diversity has barely been explored (Castillo Carrillo, 2020).
As mentioned earlier, some smallholder farmers have already included several microbe-related technologies into their production systems. Unfortunately, evaluation of these experiences remain largely confined to “gray” literature or await independent validation. Smallholder access to microbe-based technologies comes through both commercial products and homemade preparations. A large catalog of commercial microbe-based products is available from local vendors. These products fall mainly in three categories: (i) biocontrol agents (e.g., Trichoderma spp., Beauveria bassiana, Bacillus thuringiensis, Bacillus amyloliquefaciens, Bacillus subtilis, other Bacillus spp., Paecilomyces spp., Arthrobotrys spp., Lecanicillium spp., Pesudomonas fluoresecens, Acremonium butyri, Metarhizium spp.); (ii) plant growth promotion agents and digestates (e.g., products based on Acalulospora spp., Glomus spp., Sclerocystis spp., Pseudomonas fluorescens, Bacillus amyloliquefaciens or their extracts); and, (iii) biofertilizers (e.g., Rhizobium spp., Azotobacter spp., mycorhizae, composts and different types of digestates). While many of these products are imported, several local companies have been producing their own microbial formulations for some time already (Castillo Carrillo, 2020). In spite of their easy availability, the price of these products has hampered their wider adoption by smallholder farmers.
In contrast, homemade microbial preparations have been more rapidly adopted due to their relative ease of implementation and almost ubiquitous training from different actors (i.e., NGOs, farmer's associations, and government agencies). Of these preparations, manure digestates (known locally as “biols”), compost (including “bokashi compost”), and some inoculants (e.g., “effective microorganisms” and native biocontrol agents such as Trichoderma spp., Beauveria spp., and Bacillus thuringiensis) are the most commonly used. Some of the effects reported for these microbe-derived products are: growth promotion in blackberry and avocado by Trichoderma spp. (Sotomayor et al., 2019; Viera et al., 2019), biocontrol of Tecia solanivora, Phthorimaea operculella, Symmetrischema tangolias in potato based on Bacillus thuringiensis (Ayala, 2006; Suquillo et al., 2012), biocontrol of Premnotrypes vorax in potato and Macrodactylus sp. in corn based on Beauveria brogniartii and Metarhizium anisopliae (Barriga, 2003; Guapi, 2012), and growth promotion by digestates in several crops (Cobo, 2012; Manosalvas, 2012; Guerrero, 2017; Flores, 2019). However, replication and independent evaluation are common limitations in most of these reports.
Current Challenges and Perspectives in the Ecuadorian Context
Microbial technologies offer great potential to increase the sustainability of smallholder agriculture in the tropical Andes (Yarzábal and Chica, 2017); however, key challenges must be overcome. Perhaps, the main challenge could be finding a way to balance the reliability and proven effectiveness seen in commercial formulations, with the ease and self-sufficiency characteristics of homemade formulations and interventions. Merely focusing future work on new formulations of bioproducts does not seem to be compatible with the dynamic of smallholdings in the Ecuadorian Andes; plus, it risks falling in an input substitution trap that could threat long term sustainability goals (Rosset and Altieri, 1997). On the other hand, continuation of the existing transfer model for homemade formulation based on a few, barely-characterized products, precludes building sound data-based foundations to support the development of effective and proven microbial technologies. Thus, future microbial technologies amenable for adoption in smallholder farms in Ecuador would need to be effective and as self-sustaining as possible. To do this, it will be necessary to shift the focus from producing specific biocontrol/biostimulant/biofertilizer agents to produce engineered microbial communities and to integrate these microbial formulations with production practices such a tillage, crop rotations, soil amendments, and the selection of less disruptive synthetic inputs. Ideally, these emerging microbial technologies would be based on the local biodiversity in order to avoid introduction of exotic microorganisms and to improve the chances of stabilizing these communities. For the Ecuadorian context, it would be key to increase country-wide collaborations from multiple disciplines to tackle these challenges. These collaborations would necessarily include not only researchers, but also farmers, development organizations and regulators. The engagement of the latter is of paramount importance, due to their role in designing processes that foster biodiscovery and innovations in microbial technologies while avoiding unsustainable, depleting, or unfair use of native microbial resources. Also, to facilitate visibility and attract new collaborations, more effort should also be put on finding better avenues for dissemination of the acquired knowledge, to minimize the amount of experiences that remain shaded in the gray literature. Combined, these efforts would allow to put forward the idea of ecological agroecosystems engineering as a way to increase the sustainability of smallholder farms and consequently protect the livelihoods of these families.
Author Contributions
Both authors have made a substantial, direct and intellectual contribution to the work, and approved it for publication.
Funding
This review was financially supported by Universidad Católica de Cuenca.
Conflict of Interest
The authors declare that the research was conducted in the absence of any commercial or financial relationships that could be construed as a potential conflict of interest.
References
Acosta-Quezada, P. G., Raigón, M. D., Riofrío-Cuenca, T., García-Martínez, M. D., Plazas, M., Burneo, J. I., et al. (2015). Diversity for chemical composition in a collection of different varietal types of tree tomato (Solanum betaceum Cav.), an Andean exotic fruit. Food Chem. 169, 327–335. doi: 10.1016/j.foodchem.2014.07.152
Alvarez, M., Tucta, F., Quispe, E., and Meza, V. (2018). Incidencia de la inoculación de microorganismos benéficos en el cultivo de fresa (Fragaria sp.). Sci. Agropecu. 9, 33–42. doi: 10.17268/sci.agropecu.2018.01.04
Alvarez-Vera, M., Vázquez, J., Castillo, J., Tucta, F., Quispe, E., and Meza, V. (2018). Potencial de la flora de la provincia del Azuay (Ecuador) como fuente de microorganismos benéficos. Sci. Agropecu. 9, 561–568. doi: 10.17268/sci.agropecu.2018.04.12
Alvarez-Vera, M. S., Soto-Valenzuela, J. O., Quevedo -Vázquez, J. O., and Giler-Escandón, L. V. (2019). Obtención de consorcios microbianos benéficos y su incidencia en la población microbiana nativa de la rizósfera de plantas de fresa (Fragaria sp.). Pol. Con. 4, 149-179–179. doi: 10.23857/pc.v4i11.1179
Aubron, C., Cochet, H., Brunschwig, G., and Moulin, C.-H. (2009). Labor and its productivity in Andean dairy farming systems: a comparative approach. Hum. Ecol. 37, 407–419. doi: 10.1007/s10745-009-9267-9
Ayala, O. (2006). Determinación de agresividad de hongos entomopatógenos para Macrodactylus sp. (catzo del maíz) Chillanes—Bolívar. Available online at: http://repositorio.iniap.gob.ec/handle/41000/1398 (accessed October 1, 2020).
Barriga, E. (2003). Evaluación en laboratorio de la patogenicidad de aislamientos nativos de Beauveria sp. y Metarhizium anisopliae para el control de Premnotrypes vorax. Rev. Latinoam. Papa 13:104–111. doi: 10.37066/ralap.v13i1.126
Berdegué, J., and Escobar, G. (2002). Rural diversity, agricultural innovation policies and poverty reduction. Available online at: https://www.odi.org/sites/odi.org.uk/files/odi-assets/publications-opinion-files/5208.pdf (accessed September 23, 2020).
Berdegué, J. A., and Fuentealba, R. (2011). “Latin America: the state of smallholders in agriculture,” in IFAD Conference on New Directions For Smallholder Agriculture (Rome), 25.
Berruti, A., Borriello, R., Orgiazzi, A., Barbera, A. C., Lumini, E., and Bianciotto, V. (2014). “Arbuscular mycorrhizal fungi and their value for ecosystem management,” in Biodiversity: The Dynamic Balance of the Planet (Rijeta: InTech), 159–191. doi: 10.5772/58231
Bohs, L. (1989). Ethnobotany of the genus Cyphomandra (Solanaceae). Econ. Bot. 43, 143–163. doi: 10.1007/BF02859855
Broughton, W. J., Hernández, G., Blair, M., Beebe, S., Gepts, P., and Vanderleyden, J. (2003). Beans (Phaseolus spp.) – model food legumes. Plant Soil 252, 55–128. doi: 10.1023/A:1024146710611
Calvo, P., Martínez, C., Rico, M., Rojas, M., and Oswald, A. (2009). “Microbiotic biodiversity and their functionality in roots and rhizosphere of potato plants,” in 15th Triennial ISTRC Proceedings (Lima, Peru), 110–116.
Calvo, P., Ormeño-Orrillo, E., Martínez-Romero, E., and Zúñiga, D. (2010). Characterization of Bacillus isolates of potato rhizosphere from andean soils of Peru and their potential PGPR characteristics. Braz. J. Microb. 41, 899–906. doi: 10.1590/S1517-83822010000400008
Calvo, P., and Zúñiga, D. (2010). Caracterización fisiológica de cepas de Bacillus spp. aisladas de la rizósfera de papa (Solanum tuberosum). Ecol. Apli. 9, 31–39. doi: 10.21704/rea.v9i1-2.393
Carrillo-Perdomo, E., Aller, A., Cruz-Quintana, S. M., Giampieri, F., and Alvarez-Suarez, J. M. (2015). Andean berries from Ecuador: a review on botany, agronomy, chemistry and health potential. J. Berry Res. 5, 49–69. doi: 10.3233/JBR-140093
Castillo Carrillo, C. I. (2020). “Biodiversity in ecuador and its immense potential for agricultural pest control,” in Agricultural, Forestry and Bioindustry Biotechnology and Biodiscovery, eds P. A. Chong, D. J. Newman, and D. A. Steinmacher (Cham: Springer International Publishing), 143–161. doi: 10.1007/978-3-030-51358-0_9
Chica, E., Buela, L., Valdez, A., Villena, P., Peña, D., and Yarzábal, L. A. (2019). Metagenomic survey of the bacterial communities in the rhizosphere of three Andean tuber crops. Symbiosis 79, 141–150. doi: 10.1007/s13199-019-00631-5
Cobo, M. (2012). Efecto de la fertilización a base de biol en la producción de pimiento (Capsicum annum L) híbrido Quetzal bajo condiciones de invernadero (Bachelor's thesis). Universidad San Francisco de Quito. Quito, Ecuador. Available online at: http://repositorio.usfq.edu.ec/handle/23000/2021 (accessed October 1, 2020).
Córdova, R., Hogarth, N. J., and Kanninen, M. (2018). Sustainability of smallholder livelihoods in the Ecuadorian highlands: a comparison of agroforestry and conventional agriculture systems in the indigenous territory of Kayambi people. Land 7:45. doi: 10.3390/land7020045
Delamuta, J. R. M., Scherer, A. J., Ribeiro, R. A., and Hungria, M. (2020). Genetic diversity of Agrobacterium species isolated from nodules of common bean and soybean in Brazil, Mexico, Ecuador and Mozambique, and description of the new species Agrobacterium fabacearum sp. nov. Int. J. Syst. Evol. Microbiol. 70, 4233–4244. doi: 10.1099/ijsem.0.004278
Echeverría, E. J., Ponce, L. K., and Medina, M. E. (2013). Efecto de la interacción de hongos micorrícicos arbusculares y Pseudomonas fluorescens sobre el desarrollo y la nutrición de plántulas de tomate de árbol (Solanum betaceum). Rev. Cienc. 15:45–52.
Espín, E., Medina, M. E., Jadán, M., and Proaño, K. (2010). Utilización de hongos micorrícico-arbusculares en plántulas de tomate de árbol (Solanum betaceum) cultivadas in vitro: efectos durante la fase de aclimatación. Rev. Cienc. 13:86–91.
Flores, S. (2019). Alternativas de fertilización para el cultivo de papa (Solanum tuberosum L.) con el empleo de biol de producción local, microorganismos solubilizadores de fósforo y extracto de algas en la Comunidad de Canchaguano, Montúfar, Carchi (Bachelor's thesis). Universidad Politécnica Estatal del Carchi, Tulcán, Ecuador. Available online at: http://www.repositorio.upec.edu.ec/handle/123456789/762 (accessed October 1, 2020).
Ghyselinck, J., Velivelli, S. L. S., Heylen, K., O'Herlihy, E., Franco, J., Rojas, M., et al. (2013). Bioprospecting in potato fields in the Central Andean Highlands: screening of rhizobacteria for plant growth-promoting properties. Syst. Appl. Microbiol. 36, 116–127. doi: 10.1016/j.syapm.2012.11.007
Granda Mora, K. I., Nápoles García, M. C., Robles Carrión, A. R., Alvarado Cap,ó, Y., and Torres Gutiérrez, R. (2016). Response of Phaseolus vulgaris cv. Mantequilla to inoculation of Rhizobium native strains of the Ecuador in greenhouse. Centro Agrí. 43, 49–56. Available online at: http://cagricola.uclv.edu.cu/descargas/pdf/V43-Numero_4/cag07416.pdf (accessed on February 19, 2021).
Guapi, A. (2012). Evaluación de la eficacia del bioformulado de Beauveria bassiana, y tipos de aplicación para el control del gusano blanco de la Papa (Premnotrypes vorax), en dos localidades de la provincia de Chimborazo (Bachelor's thesis). Escuela Superior Politécnica de Chimborazo, Riobamba, Ecuador. Available online at: http://dspace.espoch.edu.ec/handle/123456789/2201 (accessed October 1, 2020).
Guerrero, M. (2017). Evaluación del uso de tres formulaciones de biol en la producción de papa (Solanum tuberosum L.) variedad Cecilia (Bachelor's thesis). Universidad Técnica de Ambato, Ambato, Ecuador. Available online at: https://repositorio.uta.edu.ec:8443/jspui/handle/123456789/26395 (accessed October 1, 2020).
Harden, C. (1988). Mesoscale estimation of soil erosion in the rio Ambato drainage, Ecuadorian sierra. Mt. Res. Dev. 8, 331–341. doi: 10.2307/3673556
Loján, P., Demortier, M., Velivelli, S. L. S., Pfeiffer, S., Suárez, J. P., de Vos, P., et al. (2016). Impact of plant growth-promoting rhizobacteria on root colonization potential and life cycle of Rhizophagus irregularis following co-entrapment into alginate beads. J. Appl. Microbiol. 122, 429–440. doi: 10.1111/jam.13355
Loján, P., Senés-Guerrero, C., Suárez, J. P., Kromann, P., Schüßler, A., and Declerck, S. (2017). Potato field-inoculation in Ecuador with Rhizophagus irregularis: no impact on growth performance and associated arbuscular mycorrhizal fungal communities. Symbiosis 73, 45–56. doi: 10.1007/s13199-016-0471-2
López-Guerrero, M. G., Ormeño-Orrillo, E., Velázquez, E., Rogel, M. A., Acosta, J. L., Gónzalez, V., et al. (2012). Rhizobium etli taxonomy revised with novel genomic data and analyses. Syst. Appl. Microbiol. 35, 353–358. doi: 10.1016/j.syapm.2012.06.009
Manosalvas, R. (2012). Determinación de la efectividad de “biol Biogest Potencializado”, como fuente nutricional complementaria en el cultivo de brócoli (Brassica oleracea) en la Provincia de Cotopaxi (Bachelor's thesis). Escuela Politécnica Nacional, Quito, Ecuador. Available online at: http://bibdigital.epn.edu.ec/handle/15000/4599 (accessed October 1, 2020).
Orrico, G. D., Ulloa, S. M., and Medina, M. E. (2013). Efecto de los hongos micorrícicos arbusculares y Pseudomonas fluorescens en el control de Meloidogyne spp. en plantas de tomate de árbol (Solanum betaceum). Rev. Cienc. 15:1–10.
Oswald, A., and Calvo, P. (2009). “Using rhizobacteria to improve productivity of potato,” in 15th Triennial ISTRC Proceedings (Lima, Peru), 29–33.
Oswald, A., Calvo, P., Sanchez, J., and Zúñiga, D. (2007). “Using plant growth promoting rhizobacteria to improve potato production and plant health,” in Proceedings of the 16th International Symposium of the International Scientific Centre for Fertilizers (CIEC)—Mineral Versus Organic Fertilization Conflict or Synergism? eds S. De Neve, J. Salomez, A. van den Bossche, S. Haneklaus, O. van Cleemput, G. Hofman, et al. (Gent, Belgium), 401–409.
Oswald, A., Velez, P. C., Dávila, D. Z., and Pineda, J. A. (2010). Evaluating soil rhizobacteria for their ability to enhance plant growth and tuber yield in potato. Ann. Appl. Biol. 157, 259–271. doi: 10.1111/j.1744-7348.2010.00421.x
Oyarzun, P. J., Borja, R. M., Sherwood, S., and Parra, V. (2013). Making sense of agrobiodiversity, diet, and intensification of smallholder family farming in the highland Andes of Ecuador. Ecol. Food Nutr. 52, 515–541. doi: 10.1080/03670244.2013.769099
Prohens, J., and Nuez, F. (2000). The Tamarillo (Cyphomandra betacea). Small Fruits Rev. 1, 43–68. doi: 10.1300/J301v01n02_06
Ramírez, I. F., Ulloa, S. M., and Medina, M. E. (2013). Efecto de la inoculación combinada de hongos micorrícicos arbusculares y Pseudomonas putida en plantas de tomate de árbol (Solanum betaceum) infectadas con Meloidogyne spp. Rev. Cienc. 15, 75–86.
Ribeiro, R. A., Ormeño-Orrillo, E., Dall'Agnol, R. F., Graham, P. H., Martinez-Romero, E., and Hungria, M. (2013). Novel Rhizobium lineages isolated from root nodules of the common bean (Phaseolus vulgaris L.) in Andean and Mesoamerican areas. Res. Microbiol. 164, 740–748. doi: 10.1016/j.resmic.2013.05.002
Rosset, P. M., and Altieri, M. A. (1997). Agroecology versus input substitution: a fundamental contradiction of sustainable agriculture. Soc. Nat. Resour. 10, 283–295. doi: 10.1080/08941929709381027
Sangoquiza Caiza, C. A., Viera Tamayo, Y., and Yánez Guzmán, C. F. (2018). Biological response of Azospirillum spp. to different types of stress. Revista Centro Agrícola Universidad Central. “Marta Abreu” de las Villas 45, 40–46.
Sathe, S. K. (2002). Dry bean protein functionality. Crit. Rev. Biotechnol. 22, 175–223. doi: 10.1080/07388550290789487
Soto Baquero, F., Rodríguez Fazzone, M., and Falconi, C. (2007). Políticas para la agricultura familiar en América latina y el Caribe. Organización de la Naciones Unidas para la Alimentación y la Agricultura (FAO)/ Banco Interamericano para el Desarrollo (BID).
Sotomayor, A., Gonzáles, A., Cho, K. J., Villavicencio, A., Jackson, T. A., and Viera, W. (2019). Effect of the application of microorganisms on the nutrient absorption in avocado (Persea americana Mill.) seedlings. J. Korean Soc. Int. Agric. 31, 17–24. doi: 10.12719/KSIA.2019.31.1.17
Suquillo, J., Rodríguez, P., Gallegos, P., and Orbe, K. (2012). Manual para la elaboración del bioinsecticida Bacu-Turin a través de premezclas concentradas para el control de las polillas de la papa: Tecia solanivora, Phthorimaea operculella y Symmetrischema tangolias. Available online at: http://repositorio.iniap.gob.ec/handle/41000/849 (accessed October 1, 2020).
Tamayo, P. J. (2001). Tomate de árbol. Principales enfermedades del tomate árbol, la mora y el lulo en Colombia. Corporación Colombiana de Investigación Agropecuaria – CORPOICA Boletín Tecnico No 12. Available online at: http://hdl.handle.net/20.500.12324/1175 (accessed on February 19, 2021).
Theunis, M., Kobayashi, H., Broughton, W. J., and Prinsen, E. (2004). Flavonoids, NodD1, NodD2, and Nod-Box NB15 modulate expression of the y4wEFG locus that is required for Indole-3-Acetic acid synthesis in Rhizobium sp. strain NGR234. MPMI 17, 1153–1161. doi: 10.1094/MPMI.2004.17.10.1153
Tohme, J., Toro, O. Ch., Vargas, J., and Debouck, D. G. (1995). Variability in Andean nuña common beans (Phaseolus vulgaris, Fabaceae). Econ. Bot. 49, 78–95. doi: 10.1007/BF02862280
Torres-Gutiérrez, R., Granda-Mora, K. I., Alvarado-Cap,ó, Y., Rodriguez, A. S., Mogollón, N. G. S., Almeida, J. R., et al. (2017). Genetic and phenotypic diversity of Rhizobium isolates from Southern Ecuador. Ciênc. Agrotec. 41, 634–647. doi: 10.1590/1413-70542017416008517
Vasco, C., Avila, J., Ruales, J., Svanberg, U., and Kamal-Eldin, A. (2009). Physical and chemical characteristics of golden-yellow and purple-red varieties of tamarillo fruit (Solanum betaceum Cav.). Int. J. Food Sci. Nutr. 60, 278–288. doi: 10.1080/09637480903099618
Vélez, P. C., Meneses, L. R., and Dávila, D. Z. (2008). Estudio de las poblaciones microbianas de la rizósfera del cultivo de papa (Solanum tuberosum) en zonas altoandinas. Ecol. Apli. 7, 141–148. doi: 10.21704/rea.v7i1-2.369
Velivelli, S. L. S., Kromann, P., Lojan, P., Rojas, M., Franco, J., Suarez, J. P., et al. (2015). Identification of mVOCs from Andean Rhizobacteria and field evaluation of bacterial and mycorrhizal inoculants on growth of potato in its center of origin. Microb. Ecol. 69, 652–667. doi: 10.1007/s00248-014-0514-2
Viera, W., Noboa, M., Martínez, A., Báez, F., Jácome, R., Medina, L., et al. (2019). Trichoderma asperellum increases crop yield and fruit weight of blackberry (Rubus glaucus) under subtropical Andean conditions. Vegetos 32, 209–215. doi: 10.1007/s42535-019-00024-5
Wong, S., and Ludeña, C. (2006). Informe Preliminar 1: Caracterización de la agricultura familiar en Ecuador. Guayaquil, Ecuador: Organización de la Naciones Unidas para la Alimentación y la Agricultura (FAO)/Banco Interamericano para el Desarrollo (BID).
Yarzábal, L. A., and Chica, E. J. (2017). “Potential for developing low-input sustainable agriculture in the tropical andes by making use of native microbial resources,” in Plant-Microbe Interactions in Agro-Ecological Perspectives: Volume 2: Microbial Interactions and Agro-Ecological Impacts, eds D. P. Singh, H. B. Singh, and R. Prabha (Singapore: Springer Singapore), 29–54. doi: 10.1007/978-981-10-6593-4_2
Zea, P., Chilpe, J., Sánchez, D., and Chica, E. J. (2020). Energy efficiency of smallholder commercial vegetable farms in Cuenca (Ecuador). Trop. Subtrop. Agroecosyst. 23. Available online at: http://www.revista.ccba.uady.mx/ojs/index.php/TSA/article/view/2995 (accessed September 30, 2020).
Keywords: family farming, development, sustainability, microbes, mountain, biodiversity
Citation: Yarzábal LA and Chica EJ (2021) Microbial-Based Technologies for Improving Smallholder Agriculture in the Ecuadorian Andes: Current Situation, Challenges, and Prospects. Front. Sustain. Food Syst. 5:617444. doi: 10.3389/fsufs.2021.617444
Received: 14 October 2020; Accepted: 09 February 2021;
Published: 05 March 2021.
Edited by:
Everlon Cid Rigobelo, Universidade Estadual Paulista, BrazilReviewed by:
Stefan Bäckman, University of Helsinki, FinlandNoemi Carla Baron Cozentino, Universidade Estadual Paulista, Brazil
Erin Anders, Michigan State University, United States
Copyright © 2021 Yarzábal and Chica. This is an open-access article distributed under the terms of the Creative Commons Attribution License (CC BY). The use, distribution or reproduction in other forums is permitted, provided the original author(s) and the copyright owner(s) are credited and that the original publication in this journal is cited, in accordance with accepted academic practice. No use, distribution or reproduction is permitted which does not comply with these terms.
*Correspondence: Eduardo J. Chica, eduardo.chica@ucuenca.edu.ec