- 1Guelph Research and Development Centre, Agriculture and Agri-Food Canada, Guelph, ON, Canada
- 2Department of Animal Biosciences, University of Guelph, Guelph, ON, Canada
With the noticed steady increase of global demand for animal proteins coupled with the current farming practices falling short in fulfilling the requested quantities, more attention is being paid for means and methods intended to maximize every available agricultural-resource in a highly sustainable fashion to address the above growing gap between production and consumers' demand. Within this regard, considerable efforts are being invested either in identifying new animal feed ingredients or maximizing the utilization of already established ones. The public preference and awareness of the importance of using waste products generated by fruit-dependent industries (juice, jams, spirits, etc.) has improved substantially in recent years where a genuine interest of using the above waste(s) in meaningful applications is solidifying and optimization-efforts are being pursued diligently. While many of the earlier reported usages of fruit pomaces as feedstuffs suggested the possibility of using minimally processed raw materials alone, the availability of exogenous digestive and bio-conversion enzymes is promising to take such applications to new un-matched levels. This review will discuss some efforts and practices using exogenous enzymes to enhance fruit pomaces quality as feed components as well as their nutrients' accessibility for poultry and swine production purposes. The review will also highlight efforts deployed to adopt numerous naturally derived and environmentally friendly catalytic agents for sustainable future feed applications and animal farming-practices.
Introduction
Plant-based feed additives are becoming popular in livestock and poultry production chains. Thus, the use of fruit-processing by-products such as pomaces, generated by the industry in millions of tons annually around the globe, could form an integral part of evolving, sustainable, and circular agricultural economies. The use of such pomaces could also be part of the efforts aiming at addressing many of the rising challenges facing modern human societies due to climate-associated changes including the worrying diminish of natural resources. Apart from the historically witnessed trend of promoting the use of such by-products in animal feeding (in order to reduce the environmental burdens resulting from large-scale waste accumulation), a growing interest in creating value-added products/applications is beginning to be materialized. The principle here is to use such fiber/polyphenols-rich raw products in combination with enzymes (in targeted digestions and fermentations) in order to generate value-added end commodities. The two factors that control the outcomes of the above goal(s) are: (1) the chemical composition of the starting materials/substrate(s) and (2) the digestion and fermentation processes themselves with their varying parameters (including the enzymes being used, nature and source of pomaces and their endogenous microbiota, pH, presence or absence of oxygen, etc…).
The use of enzymes is not a completely novel idea as intact microbial products (yeast and bacteria containing active wild-type enzymes) have been conventionally used by many industries in the past (and are still today) to enhance food and feed products. The same trend prevails today but with a slight advantageous skew toward using purified or recombinant enzymatic preparations due to numerous empirical considerations that will be discussed in the following sections.
In recent years, there has been increased research efforts aiming to incorporate many abundantly-available fruit pomaces in animal feed (Das et al., 2020). As it shall be seen in subsequent sections, these pomaces have been shown to possess many important bioactives at varying concentration ranges and functionalities including antimicrobial activities against foodborne pathogens such as Salmonella enterica for example (Das et al., 2019). More novel applications are being explored currently through enzymatic or microbial interventions where the final products of interest are not limited to the active polyphenolic-rich fraction but also other nutritional and industrial factors. A very good example of these later interests, is the most recent study that explored using red grape pomace as a fermentation medium in combination with Lactobacillus plantarum subsp. plantarum (ATCC #14917) and Bacillus subtilis subsp. subtilis (ATCC #6051) cultures to aid in chitin and chitosan extraction, both used as feed supplements (Hirano et al., 1990; Hossain and Blair, 2007; Nuengjamnong and Angkanaporn, 2018; Reddy et al., 2018) from shrimp wastes in a replacement of earlier traditional chemical extraction approaches (Tan et al., 2020). In the above model, red grape pomace replaced glucose (to support the bacterial growth) resulting in recovery levels of chitin and chitosan that were comparable (or surpass) other commercial chitin preparation protocols.
In general, the emerging trend of enhancing pomace usage through enzymatic treatments indicates the possibility of developing many future opportunities that will be discussed in later sections of this review. Furthermore, and while the authors agree that other physical means to enhance pomaces and/or the recovery of bioactive compounds from fruit pomaces {such as the supercritical CO2 extraction of active ingredient/oils (Squillace et al., 2020) as well as freeze-drying approaches coupled with double-emulsions (water-in-oil-in-water) loading of pomace extracts (Eisinaite et al., 2020)} do exist, enzymatic approaches seem to offer a green sustainable strategy. Hence the current review will focus on such bioconversion procedures for value-added feed applications of pomaces in mainly poultry and swine.
Advantages and Disadvantages of Enzyme-Assisted Bioconversions of Pomaces
The possibility to produce high-quality exogenous enzymes is among the top technologies that positively impacted the animal feed industry in the past four decades. The incorporation of commercial enzymatic preparations in animal feed is now widely acceptable by farmers as well as end-users alike. No one would imagine the global animal feed industry operating nowadays without the powerful capabilities of fiber-degrading enzymes or without the phytases and amylases that are used routinely (Kiarie et al., 2016a, 2019; Alagawany et al., 2018).
The biggest advantage of feed enzymes is their specificity. Enzymes are not like any other physical or chemical treatments (i.e., heating and acidification for example) that target feed nutrients and compounds in a universal non-discriminatory fashion. Enzymes are rather very specific in their nature and only target certain chemical groups and bonds without affecting other components within the feed-matrix. This increases their efficiency from one side while decreasing any negative off-target effects (if any) when used with animal feed (Bhatia et al., 2020; Jatuwong et al., 2020).
The second appealing factor of using enzymes in the feed industry is their high acceptance rates by average consumers. These catalytic molecules are perceived as part of refined green-technologies and if the source organism was a GRAS (Generally Recognized As Safe), then the approval process by both regulatory agencies and end-consumers could be generally less complicated, in comparison to enzymes that are obtained by other approaches (such as synthetic biology approaches). The importance of having both consumer-acceptance and the regulatory agencies approval concomitantly cannot be overemphasized for any genuine applications (DeRuiter and Dwyer, 2002).
Among the critical issues related to enzyme usages are the technical difficulties. In fact, the preparation of enzymes with an acceptable purity, stability and sufficient catalytic power are challenging. In the past, suppliers had to start with kilograms (or tons in some cases) of raw materials before delivering milligrams of ready-to-use enzymes. Thus, the utilization of enzymatic-preparations was very expensive and extremely unpredictable with susceptibility to purification-dependent variations in performance from batch to batch. Indeed, the cost of such preparations was among the biggest concerns of farmers toward enzymes-usage as it added up to the total costs of feeding. When the difference between profitability and non-profitability melts down to few cents or pennies per ton of used feed, any extra cost(s) need to be carefully considered and planned ahead of time. With the introduction and refinement of exogenous-enzymes biotechnologies using optimized host microorganisms (bacteria, yeasts or fungi), the process is becoming increasingly manageable and robust. While the cost of discovering novel functional enzymes (the research and development phase more precisely) is still considerably high and requires collaborative efforts of many specialized experts (Howard et al., 2003; Monciardini et al., 2014), the actual costs involved in producing stable enzymatic-preparations (the large-scale production phase) are becoming very reasonable nowadays. Furthermore, the encapsulation and lyophilization of enzymes as well as solid-matrix immobilization techniques reached a pinnacle stage in performance aiding in achieving stable and potent enzymatic preparations continuously (Gifre et al., 2017; Ibrahim et al., 2019).
Most commonly and when purified enzymes are inaccessible or are very expensive to use, fermentation-protocols can be implemented to enhance pomace attributes for animal feed application. The fermentation process is looked at here as a way of harnessing the power of endogenous fungal- or bacterial-enzymes to: (1) naturally degrade the fibrous contents of pomace and release its active polyphenol compounds, (2) convert or transform some compounds to their more active forms, and finally, (3) make use of the involved microbial inoculum (either bacterial, fungal, or yeast) metabolites in combination with the targeted polyphenolics to enhance animal feeding outcomes (performance).
The presence of certain enzymatic co-factors such as reduced nicotinamide adenine dinucleotide (NADH) and nicotinamide adenine dinucleotide phosphate (NADPH) as well as, adenosine triphosphate (ATP) and pyrroloquinoline quinone (PQQ) within the intact microbial hosts (Cutlan et al., 2020) is another advantage for microbial fermentations over purified enzymatic preparations. The host-organism is capable in this case of replenishing such co-factors cheaply and compared to using purified enzymatic preparations where the cofactor(s) need to be constantly supplied, the in-host conversions are more cost-efficient.
The recalcitrance of certain plant contents in pomaces to natural degradation makes them more appealing targets for microbial bioconversions than enzymatic treatments (Troncozo et al., 2019). Raw pomaces (grape pomace for example which results from juice and spirits industries) have significant negative environmental impacts due to their water-retention capacity and their high content of lignins which breakdown to generate hydroxylated and methoxylated monoaromatic compounds associated with strong phytotoxic activities (Troncozo et al., 2019). To reduce the environmental concerns connected to the above compounds, many treatments have been suggested in the past including drying or using them as an extraction source of beneficial compounds such as γ-linolenic acid and carotenoids (Dulf et al., 2019) among many other treatments. Thus subjecting pomaces to microbial fermentations looks the most feasible approach when it comes to processing the large amounts of pomaces generated annually.
The ability of using enzymes with pomaces faces another hurdle that emerges from the unique ability of pomace bioactives [including polyphenols and tannins (Hemingway and Laks, 2012)] at certain concentration to influence (or even inactivate) the functionality of used enzymatic preparations either through specific or non-specific interactions (Martinez-Gonzalez et al., 2017; Yildirim-Elikoglu and Vural, 2019). Many reports showed the ability of certain polyphenolics to negatively influence the functions of natural or industrial enzymes in a similar fashion to their ability to negatively impact microbial growths (Gonçalves and Romano, 2017; Ramos-Pineda et al., 2019). For example, pectinases involved in the enhancement (aroma and color) or clarification of many beverages and juices are reported to be inhibited by apple flesh or pomace (Negoro, 1972). An insoluble complex compound of pectinase and inhibitors is formed to influence the enzyme's activity. The recent further characterizations of such a inhibitory activity highlighted tannic acid and (its derivatives) as a possible cause of the above insoluble complex formation (Negoro, 1972). Similarly, the inhibitory effect of polyphenolic compounds from purple and red potato cultivars on activities of α-amylase, α-glucosidase, and aldose reductase are well-established (Kalita et al., 2018). Anthocyanins were recently categorized as non-competitive inhibitors of such enzymes. In contrast, phenolic acids behaved as non-competitive inhibitors of aldose reductase and as mixed inhibitors of α-amylase and α-glucosidase, respectively (Kalita et al., 2018).
Last but not the least, animal feeds preparation encompasses most often a pelleting step (heating, mechanical mixing and shearing, drying…) which together can adversely affect the involved enzymes and reduce their detectable activity. Such influential factors need to be considered carefully before incorporating any digestive enzymes that are not heat-resistant in the processing of animal feed (Kiarie and Mills, 2019). The same observation concerns the used enzyme's ability to withstand and function under the low pH values of animal digestive systems. In such cases, an encapsulation step might be beneficial.
The above mentioned issues open the door for a variety of optimization studies (Figure 1) that need to carefully consider the composition of used pomaces, their polyphenolic content, enzyme(s) being utilized, and finally the precise usage condition(s) for each unique feeding application to obtain optimum activities while minimizing the chances of unintended inhibition(s). Some of the above issues can also be addressed though protein engineering (Kuddus, 2018; González-Domínguez et al., 2019; Sharma et al., 2019). In fact, and referring to the pectinases example stated above, the industrial enhancement and optimization of such enzymes led to the selection of numerous pectinases with broad activity ranges that can function even at high polyphenol concentrations, diverse pH-values and/or processing temperatures with some being currently commercialized. The same approach is true in regard to engineering feed enzymes to render them more heat resistant or pH stable (Rigoldi et al., 2018). As shown on Figure 2, the Aspergillus fumigatus phytase (Xiang et al., 2004) was engineered through a single mutation of lysine to alanine at position 68 (K68A) leading to a noticeable decrease in its pH optima (with phytic acid as substrate) by 0.5 to 1.0 units with no change or even a slight increase in enzyme's maximum specificity (Tomschy et al., 2002).
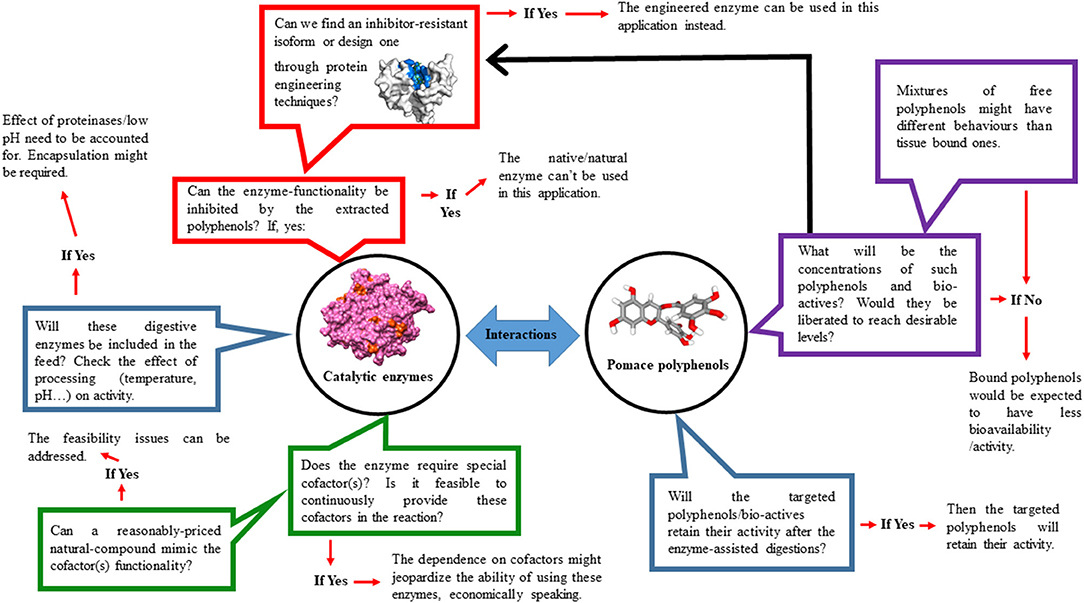
Figure 1. Optimization studies are critically needed when using enzymes and fruit pomaces as animal feed additives. Multiple overlapping factors (pomace composition, its polyphenolic content, enzyme mixes being implemented….) affect the performance of pomaces polyphenols and must be carefully considered for satisfactory and reproducible outcomes.
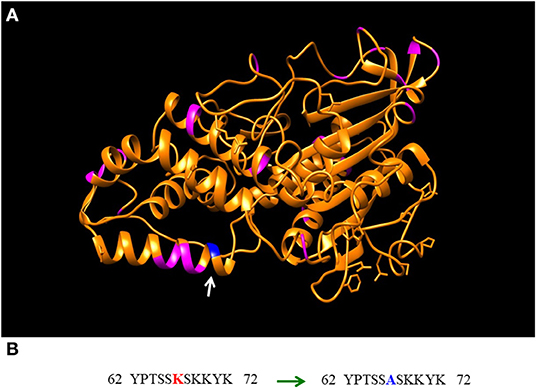
Figure 2. Amino acids sequence (442 residues) and the three-dimensional structure of Aspergillus Fumigatus phytase (accession #1QWO_A) identified an important lysine residue (dark blue) in comparison to all other available lysines (magenta) (A). Mutation of this residue (lysine “K” 68 to alanine “A”) (B), leads to a noticeable decrease of pH optima by at least 0.5 to 1.0 units.
The Fate of Fruit Pomace Bioactives Within the Animals' Digestive Systems
In order to use exogenous enzymes to treat pomaces, it is important to understand what happens to pomaces and their final enzymatic digestion products within the animal's digestive systems.
Pomace has been defined as the by-products of fruits after pressing for juice generated by processing industries. It includes the flesh, peels, stems (or parts of stems), and seeds (Ross et al., 2017; Waldbauer et al., 2017). The chemical characterization demonstrates the presence of over 4,000 metabolites and bioactive compounds in many plants and their fruit pomaces. In the following sections, we will discuss a few selected ones that are commonly encountered in animal feeding applications.
Cranberry and Blueberry Pomace
The characterization of pomaces from American cranberry (Vaccinium macrocarpon) and wild blueberry (Vaccinium angustifolium) by Ross et al. (2017) revealed a wide range of minerals, phenolics, carbohydrate, lipids, and proteins. The detected minerals in these two berries' fruit pomaces included calcium, potassium, iron, copper, zinc, magnesium, manganese, and phosphorus among others, while their specific enriched phenols included tannins, anthocyanins, tartaric esters, and flavanols (Ross et al., 2017), whose amount is mainly abundant in the fruit's peel.
Despite some anti-nutritive properties of tannins in terms of reducing iron absorption, protein digestibility hence growth performance, various studies demonstrated their potential as anti-carcinogen, antibacterial, antioxidant and immune-modulator agents among others (Chung et al., 1998; Kumar Ashok and Upadhyaya, 2012). Ethanolic extracts of above mentioned cranberry and wild blueberry pomaces were enriched in phenolics, tartaric esters, and antioxidant activities compared to original pomaces (Ross et al., 2017). Feeding broiler chicken with cranberry and blueberry pomaces and their extractives, induced metabolic changes in the bird's blood including a significant increase in the levels of plasma quinic-acid suggesting that these products can reduce oxidative stresses and improve metabolic functions against reactive oxygen species' and their associated-damages in chickens (Das et al., 2020).
Apple Pomace
The characterization of apple pomace revealed that it contains about 43.6% dietary fibers (Sato et al., 2010), mono and di-saccharides such as sucrose, arabinose, galactose and fructose (Gabriel et al., 2013), triterpenoids (Almeida-Trasviña et al., 2014), polyphenolic compounds (Vrhovsek et al., 2004), volatile compounds such as glycerol esters and aldehydes (Madrera and Valles, 2011), non-volatile acids such as malic, citric and quinic acids (Chapman and Horvat, 1989), proteins (7.1%), in addition to minerals such as sodium, potassium, calcium, phosphorus, magnesium, iron, manganese, zinc, and copper (Pieszka et al., 2015). Polyphenols in apple are predominantly found in the peel fraction, where they are bound to pectin and other cell-wall structures. This was demonstrated through measuring the quantity of polyphenols including catechins, proanthocyanidins, hydroxycinnamates, flavonols, dihydrochalcones, and anthocyanins in the juice and in the pomace, with the pomace showing higher polyphenolic concentrations (Vrhovsek et al., 2004; Marag et al., 2015). The biological activities and benefits associated with apple pomace consumption (and its derivative compounds) have been documented (Sato et al., 2010; Gabriel et al., 2013; Almeida-Trasviña et al., 2014). Apple pomace fibers were reported to improve the digestion and metabolism in animals through their prebiotic actions and the promotion of the growth of beneficial bacteria in the gut. This in turn enhances the peristaltic movements of the bowel, mediation of homeostasis of cholesterol and triglycerides, which then improves the cardiovascular health together with triterpenoids which enhance the bioactivity of the nitric oxide synthase enzyme (Beermann et al., 2018). Moreover, plasma from lambs fed a fattening diet containing ~10% apple pomace, demonstrated a higher antioxidant activity (Rodríguez-Muela et al., 2015). Wistar rats fed native apple pomace, showed an increased superoxide dismutase (SOD) activity in their red blood cells, demonstrating further the potential of apple's pomace bioactives in mitigating oxidative stresses (Juśkiewicz et al., 2011). In broiler chickens, Colombino et al. (2020) elucidated the importance of apple polyphenols in improving the gut microbiota composition of birds, specifically increasing the α-diversity as compared to blackcurrant and strawberry diets that were also used in these experiments. Moreover, the authors did hypothesize that since the broilers' histomorphometric indices were not negatively affected by the fruit pomaces, then the latter do not hamper gut development and nutrient absorption. Another study in turkey poults by Juskiewicz et al. (2016) utilized apple, blackcurrant and strawberry pomaces at 5% inclusion levels to show a significant decrease in sucrose and maltase mucosal activity in the small intestine of birds fed blackcurrant and strawberry, but not in the apple group. This could be partially due to the varying levels of fibers content in these three pomaces that could influence enzymes activities.
Grape Pomace
Grape pomace has been suggested also as an animal feed additive to take advantage of its proteins, lipids, non-digestible fibers and minerals, in addition to its non-phenolic vitamins such as β-carotenoids and tocopherols that are known to exhibit antioxidant properties (Silva Soares et al., 2018). Despite its rich content in potential nutritionally-important phytochemicals, it is only in the present times that the necessity of fully characterizing of grape-pomace compounds and their nutritional influence has been realized (Makris and Kefalas, 2013). Earlier characterizations demonstrated a wide array of polyphenolic compounds such as catechins, stilbenes, saponins, flavanols, anthocyanins, phenylpropanoids and derivatives of p-coumaric and benzoic acids (Makris and Kefalas, 2013). Further studies showed the anthelmintic, ovicidal and antilarval activities and the stimulatory potential of grape saponins and tannins (up to a certain level, beyond which the tannins would have anti-nutritive effects due to their strong binding to proteins and macromolecules and complex formation) (Silva Soares et al., 2018). The above phytochemicals reflected grapes' pomace potential to inhibit the hatching, growth and movement of free-living Haemonchus contortus worms. In a more recent study (Chedea et al., 2018), extracts from grape were used in pre-weaned piglets that are usually susceptible to physico-chemical damages to their enteric systems associated with the weaning-process stress. Polyphenols derived from grape extracts showed an anti-inflammatory effect within the small intestines of these piglets hence minimizing villus erosion and atrophy. Moreover, the antioxidant potential of grapes significantly increased the levels of glutathione peroxidase and SOD activities in the duodenum and colon sections of the digestive system. The authors suggested possible relationships between the above positive effects with grape pomace polyphenolics including gallic acid, hydroxycinnamic acid, anthocyanins, catechins, and epicatechins (Chedea et al., 2018). In chicken, studies by Goñi et al. (2007) explored the use of grape pomace at 5, 15, and 30 g/kg inclusion levels with vitamin E [α-tocopheryl acetate (200 mg/kg)] in a corn-soybean basal diet. They reported that grape pomace was able to increase the anti-oxidative capacity of the administered diets, birds' excreta, and meat during storage, with the latter having a higher oxidative stability at the highest pomace inclusion level. Additionally, these authors (Goñi et al., 2007) found that liver vitamin E concentrations were significantly high to warrant the consideration of alternatively using grape pomace flavonoids to increase vitamin E in chicken which could reduce costs associated with this vitamin in diet.
Polyphenols Absorption, Transport, and Bioavailability
While absorption and transport of Polyphenols in gut is a controversial topic, recent studies conducted in human subjects have shown that once ingested into the gut, about 5 to 10 per cent of the monomeric and dimeric polyphenols are absorbed by passive diffusion into the enterocytes (Corrêa et al., 2019). Upon this absorption, the aglycones are bio-transformed in the enterocytes and also in the liver hepatocytes with the resultant metabolites distributed to various organs in the body before excretion in the urine. The more complex and larger polyphenols (oligomeric and polymeric such as tannins) reach the large intestine where they are degraded by some microbes resulting in generation of less complex compounds. The gut microbial transformation of these polymeric structures include C-ring cleavage, demethylation, decarboxylation, and dihydroxylation (Corrêa et al., 2019). Whilst still in the gut, the above bioactive or bio-transformed compounds exert their effects based on many factors where bioavailability and absorbability stand on the top of such factors. This bioavailability of polyphenols is influenced by many physicochemical factors such as polarity, molecular mass, type of polyphenols present and their digestibility by the gut enzymes and in turn their absorption (Ozdal et al., 2016). Bio-accessible nutrients are defined as the fraction that is released from the food matrix into the bloodstream. According to Parada and Aguilera (2007) this part of the nutrients reaching the bloodstream is more important than the total amount of nutrients excreted. Furthermore, many beneficial bioactive compounds are present in biological forms that are usually not fully available hence a disruption of the food matrix (and the structures holding such food/feed components) to make them more accessible is a must.
The role of enzymes in enhancing nutrient's bio-accessibility becomes a critical aspect during the in vivo digestion and plays a key role in the bioavailability of numerous pomace bioactives. As the major bioactive compounds found in pomaces (such as those of cranberry, blueberry, apple and grape) are polyphenols described as large heterogeneous molecules with a distinguishing hydroxylated phenyl moiety (Ozdal et al., 2016); this review will focus briefly on their digestion and absorption. Scalbert and Williamson (2000) reported that many polyphenols pass through the gastrointestinal system without being absorbed to end up in the hindgut to be bio-transformed by colonic microbiota into different metabolites. These metabolites engineer a symbiotic relationship within the gut leading to the inhibition of pathogenic microbial populations while supporting beneficial bacterial proliferation and ameliorating the host‘s health. The microflora living in this symbiotic relationship with the polyphenols are mainly bacteria, archaea and eukaryotes (Williamson and Clifford, 2010). Researchers postulated that the three main genera of bacteria that form about 90% of the gut bacterial microflora of chicken are Bacteroides, Clostridium and Eubacterium while genera of Fusobacterium, Peptostreptococcus, and Bifidobacterium being fewer but of significant importance (Iqbal et al., 2020).
Many of the functional and antimicrobial compounds identified in pomaces (such as resveratrol for example) were subjected to stability studies in the past and their fate was tracked during numerous processing steps (Bertelli et al., 1998). As mentioned above, about 5 to 10% of polyphenols are absorbed in the small intestine (Manach et al., 2005; Faria et al., 2014).
In terms of their nature and structures, polyphenols can be categorized into two groups: flavonoids and non-flavonoids phenolics. The structure of flavonoids consists of two benzene rings (A and B), linked together by a heterogeneous pyrone C ring (Figure 3). This group contains compounds such as flavonols, flavones, flavanones, flavone C-glycosides, isoflavones, flavanols, and anthocyanins. These phenolics have very varied structural differences, which influence their metabolism, interaction with gut microbiota, absorption, and assimilation (Silva Soares et al., 2018). In comparison, the non-flavonoid phenolics are more heterogeneous in nature and extend in structure from the simple benzoic acid to more complex compounds such as tannins and lignans (Wu et al., 2009; Ozdal et al., 2016). The latter group encompasses compounds such as phenolic acids, stilbenes, and lignans, which are reported in abundance in cranberries and grapes pomaces. Resveratrol is an example of a stilbenoid that is of great importance in gut health, as it is bio-transformed by gut microbiota to dihydroresveratrol, 3,4′-dihydroxy-trans-stilbene, and 3,4′-dihydroxybibenzyl (lunularin) (Bode et al., 2013).
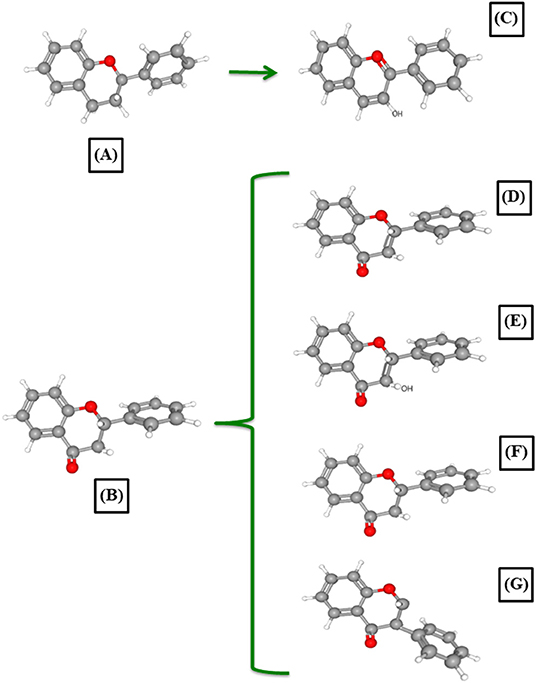
Figure 3. The diverse structure and classification of major plant flavonoids: (A) flavan nucleus; (B) 4-oxo-flavonoid nucleus; (C) anthocyanidins; (D) flavones; (E) flavonols; (F) flavanones; and (G) isoflavones. The presented polyphenols within the mentioned figure are simplified, but show the complexity and diversity of major plant flavonoids. More in-depth depiction of polyphenols was published most recently (Hassan et al., 2019).
In the hindgut the majority of biotransformation products from polyphenolics include: benzoic acids (C6-C1), protocatechuic acid, phenylacetic acids (C6-C2), and 3-(3'-hydroxyphenyl)-propionic acid; which are easily absorbed into blood, taken to tissues, and thereafter excreted via the kidneys (Williamson and Clifford, 2010). Table 1 shows various bio-transformed metabolites of some of the most common flavonoids and non-flavonoid-type phenolics found in pomaces.
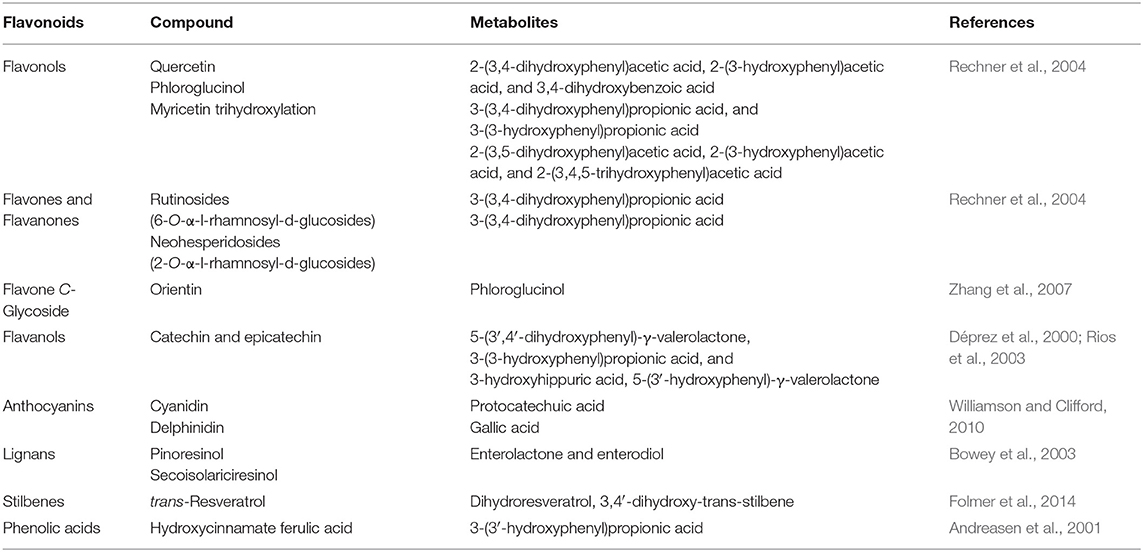
Table 1. A shortlist of the most encountered pomace active compounds and their fate within the digestive system.
The catabolism of pomace bioactives is dependent on the host‘s enzymatic systems and colonic microflora. Bound polyphenols are usually released from the food/feed matrixes. After absorption and uptake in the liver, aglycones and glucosides become conjugated through glucuronidation and sulphation processes and part is returned to the small intestine (Chen et al., 2018). The portion of the polyphenols reaching the colon will be de-conjugated by microbial enzymes such as glucuronidases and sulphatases. This enables the re-uptake or catabolism of the aglycones and glucosides into simple compounds such as hydroxyphenylacetic acids (flavonols), hydroxyphenyl-propionic acids (flavones and flavanones), phenylvalerolactones, and hydroxyphenylpropionic acids (flavanols) (Ozdal et al., 2016). Figure 4 shows the fate of two flavonoids: flavone C-monoglucosides and flavone C-multiglycosides, where the former is de-glycosylated into smaller metabolites and then excreted via the colon, while the latter is absorbed in the small intestine and either re-circulated via the liver or excreted via the kidneys. Other processes in which polyphenols are bio-transformed by gut microflora include deglycosylations, esterifications, methylations, hydrolysis and hydroxylation reactions (Chedea et al., 2018).
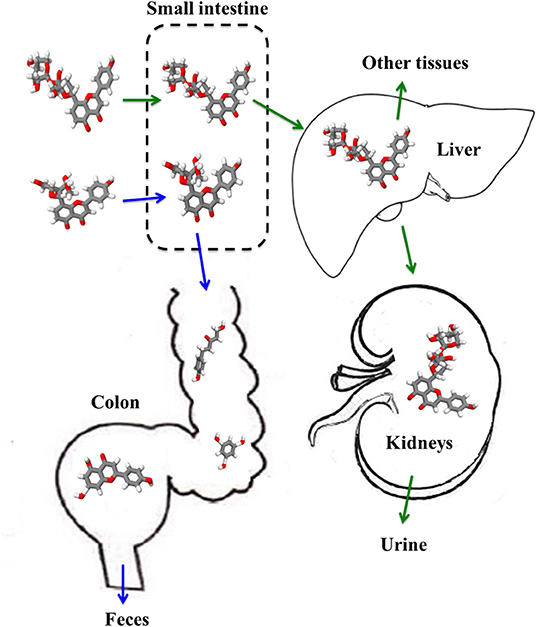
Figure 4. Biotransformation of flavone C-monoglucosides and flavone C-multiglycosides into different flavonoid aglycones, monomers and dimers in various organs and tissues, and their subsequent excretion. The depicted pathway though simplified, does not reflect the complexity of polyphenols absorption, transport, and bioavailability.
Current in vitro Pomace Enhancement Approaches
Enzymatic digestions and/or microbial fermentations introduce fundamental changes in pomace chemical composition, physical structure, or both. Many recent studies have investigated such changes trying to evaluate the final products and tracking specific compounds throughout the entire valorization process. Various factors (including the fruit variety, vintage, and processing techniques) influence the enzyme- and fermentation-induced changes and the overlap between these factors is substantial.
Apple Pomace
The high carbohydrates contents of certain pomaces (such as apple pomace) aids in incorporating them as fermentation substrates in multiple microbial processes for the production of useful bio-compounds such as organic acids, enzymes, ethanol, pigments, and single-cell proteins. Recently, the biomass degradation efficiency of an enzymes-mix targeting apple pomace was demonstrated at 20–30% solid loading with desirable digestion and fermentation end-products of glucose, fructose, and ethanol; respectively (Magyar et al., 2016). Similarly, another study compared the effects of acetic acid and pectinase pre-treatments with cellulase prior to apple pomace at 10% hydrolysis resulted in the highest pectin and fermentable sugar yields (Luo and Xu, 2020). Another study reported that the enzymatic hydrolysis of apple pomace can be optimized at room temperature using un-buffered systems with maximum production of galacturonic acid, glucose, arabinose and galactose achieved through treating apple pomace with Novozyme, Viscozyme and Celluclast (Gama et al., 2015).
In a recent study, pectin extraction from apple pomace with endo-xylanase and endo-cellulase enzymes resulted in 19.8 and 15% extraction efficiency, respectively, when enzymes were used individually whereas the utilization of both enzymes simultaneously led to lower pectin mass and extraction efficiencies. The study also reported that the pectin extracted with different enzymes have varying mass and chemical composition (Wikiera et al., 2016). Finally, apple pomace was used as a substrate for the production of multiple carbohydrases by utilizing the brown rot fungus Rhizopus delemar F2 in solid-state fermentations (SSF). Higher yields of cellulose (18.20 U g−1), xylanase (158.30 U g−1), pectinase (61.50 U g−1), and amylase (21.03 U g−1) were achieved when a microwave pre-treatment was coupled with SSF of apple pomace (Pathania et al., 2018).
Grape Pomace
One recent study reported the fate of polyphenolics in grape skins and seeds after a fermentative maceration step (Guaita and Bosso, 2019). Polyphenolics composition of skins, seeds and pomaces from four fresh grapes varieties namely Albarossa, Barbera, Nebbiolo, and Uvalino were investigated after the fermentative maceration. Despite a varietal dependent polyphenolics composition, the differences between cultivars in the polyphenolic profile disappeared after the fermentative maceration (Guaita and Bosso, 2019). Grape pomace was used recently as a fermentation and growth substrate in the cultivation of two Pleurotus ostreatus and P. pulmonarius to study the influence of different fermentation modes on the consumption of phenolic compounds, the production of mycelial mass and enzymes. Maximum biomass values (~0.5 g/g) were obtained within the submerged cultures while laccase (a polyphenol oxidase belonging to the blue oxidase family and one of the important lignocellulolytic enzyme) production was induced in solid-state fermentations (26.247 U/g). Both P. ostreatus and P. pulmonarius strains were able to degrade up to 79% of the total phenolic content, regardless the fermentation/culture conditions (Papadaki et al., 2019). From the three enzymes used in the above study, Novoferm was reported to induce the strongest effect on phenolics release from grape waste, followed by Pectinex Ultra and Celluclast. Similarly, both cellulase and gluco-amylase were used to extract polyphenols from grape pomace. The addition of cellulase increased the total polyphenolic contents to 41.05 ± 1.07 mg ChAE/g, with the optimal concentration being around 0.25 mg/mL, whereas the addition of gluco-amylase exhibited little effect (Kabir et al., 2015). A water-soluble extract containing high polyphenol contents (12%), peptides, carbohydrates, and lipids was obtained from grape pomace using an endo-protease mixture at 20% solid loading. This extract exhibited anti-inflammatory properties in vitro as it decreased pro-inflammatory factors and inhibited excessive microglial activation (Rodríguez-Morgado et al., 2015). In a recent study, the ultra sonication with 0.5M KOH combined with a mixed enzymes treatment provided the highest lignin degradation activity with a yield of 13% along with the release of many commercially-important polyphenols in substantial amounts (Karpe et al., 2017). Similarly, polyphenolic compounds of wet and dried red grape pomace (Vitis vinifera L.) were extracted utilizing refined enzymatic digestions with a celluclast enzyme providing the highest yield of extracted polyphenols (Ferri et al., 2016).
The solid-state fermentation (SSF) seems to be preferred because of the high concentration and stability of the obtained products, its low operational costs, and the low catabolic repression of involved microbes (Hölker and Lenz, 2005). An extraction of grape pomace, where the commercial enzymes Cellubrix, Neutrase, and Viscozyme were used in combination, resulted in a 20–45% increase in the yields of soluble solids and 25–65% increase in phenols. Maximum phenolic yields were achieved by treating distilled Cabernet Sauvignon pomace with 30 g/kg of the enzyme mixture (Costoya et al., 2010). A different study investigated the production of hydrolytic enzymes through SSF by a mutant strain Aspergillus niger 3T5B8 using grape pomace and wheat bran combinations or solely wheat bran. The addition of grape pomace resulted in more diverse fermentation compounds with a higher proanthocyanidins content and higher antioxidant potential (Teles et al., 2019).
Berry Pomace
An aqueous enzyme-assisted (carbohydrases and proteases) extraction procedure to recover lipophilic compounds (essential fatty acids, tocols, phytosterols, and ellagitannins) and polyphenols from raspberry (Rubus idaeus L.) was developed (Ferri et al., 2016). Under the reported optimized conditions [1.2 units of thermostable alkaline protease, pH 9, 60°C, and 2 h hydrolysis], more than 38% of the total lipophilic content was recovered within the aqueous phase. The recovery of polyphenols and antioxidant activity was 48 and 25%, respectively, and higher than the ones obtained through extractions with a methanol-acetone-water mixture (Saad et al., 2019). The outcomes of the above experiments showed the possibility of using enzymes to overcome the drawbacks of organic solvent use for bioactive polyphenols recovery and the ability of developing eco-friendly and cost-effective alternatives to recover such compounds (Saad et al., 2019). Furthermore, using enzymes presents an economic feasibility compared to more safe extraction procedures such as supercritical carbon dioxide. Studies compared the use of pressurized liquid extractions with supercritical carbon dioxide to enzymatic assisted extractions from solid state fermentation in recovering bio-functional compounds from blackberry pomace (Kitryte et al., 2020). The consecutively applied treatments at optimized parameters yielded lipophilic fractions containing polyunsaturated fatty acids (linoleic 64.1%, α-linolenic 12.9%) while recovering up to 29.1 mg gallic acid and 168.7 mg Trolox equivalents from pomace antioxidants. This study also demonstrated that enzyme-assisted extractions (a commercial multi-enzymes complex, Viscozyme L, with a strong pectolytic activity and a wide range of carbohydrases, including arabanase, cellulase, β-glucanase, hemicellulase, and xylanase) could recover more valuable pomace constituents compared to solvent-based extractions as evidenced by a significantly lower quantities (0.5–0.7 mg/g) of anthocyanins obtained in the solvent-based extraction systems (Kitryte et al., 2020). Moreover, as pomace particle size decreases from 500 to 1,000 μm to <125 μm, the yield of phenolic compounds increased by 1.6 to 5 times (Landbo and Meyer, 2001).
Orange and Tomato Pomace
The production of pectinases by Aspergillus niger was facilitated by SSF with orange pomace substrate. The maximum activity of pectinases produced occurred at 45–55°C and the maximum enzyme productivity occurred at 70% moisture content and a C/N ratio of 10 (Mahmoodi et al., 2017). The production of laccase, xylanase and protease were achieved through SSF on tomato pomace with white-rot fungi P. ostreatus and Trametes versicolor. Significant laccase and protease activities were detected: up to 36 U g−1 and 34,000 U g/1 dry matter, respectively (Iandolo et al., 2011). Using orange pomace as substrate in the process of SSF, Paecilomyces variotii was able to produce tannase and phytase simultaneously. High tannase and phytase activities being 5,000 U/gds after 96 h and 350 U/gds after 72 h, respectively were obtained. In addition, the antioxidant capacity of orange pomace increased around ten-fold as a result of SSF (Madeira et al., 2012).
In essence, laboratory experiments definitely show promising outcomes and strong justification for using enzymes and/or fermentation to enhance the extraction efficiency of polyphenols from pomace. More examples are listed in Table 2; Figure 5 (Sunna and Antranikian, 1997) as reviewed in below sections dealing with the in vivo models of pomace usage in combination with enzyme(s) supplementation for animal feeding applications.
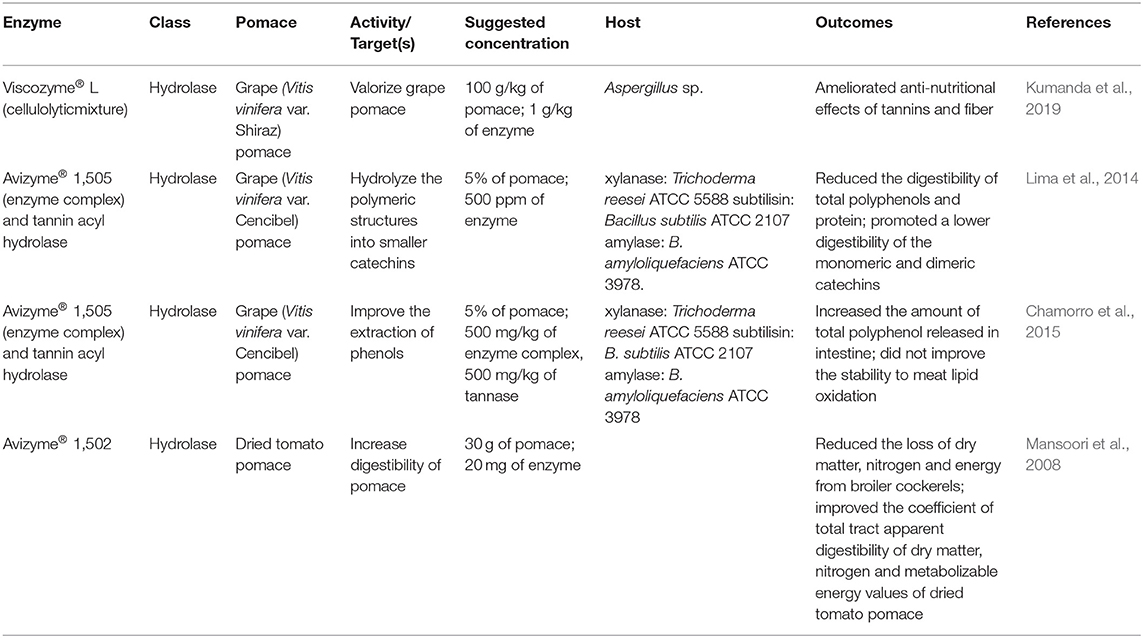
Table 2. Examples of exogenous enzymes used to enhance fruit s pomaces' quality as animal feed additives.
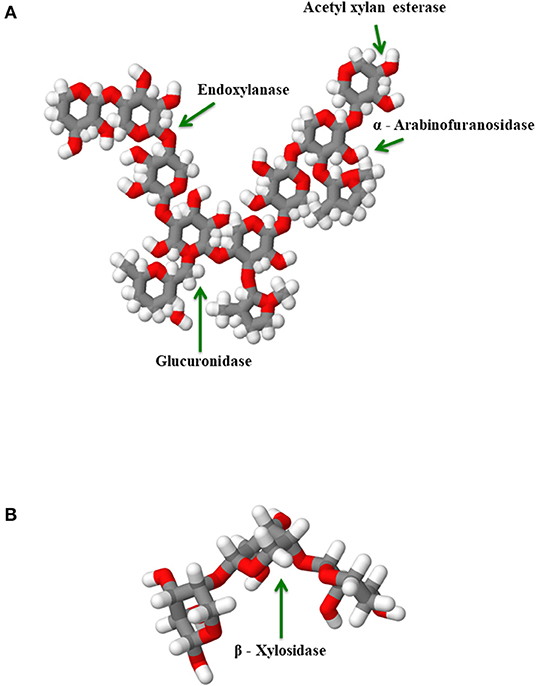
Figure 5. Degradation of xylan polyssacharide and xylooligosaccharide to produce α-arabinofuranose, α-glucuronic acids among other acetyl groups (Wong et al., 1988): (A) Xylan degradation by xylanolitic enzymes to produce α-arabinofuranose and α-glucuronic acid, (B) β-xylosidase enzyme hydrolytically degrading a xylooligosaccharide.
Finally, the use of enzymes should be evaluated individually and case by case based on the desired final product(s) as well as the surrounding factors of the treatment (such as berry composition for example). In a separate study, the effect of enzyme-treatments before juice pressing on the anthocyanin composition of Aronia melanocarpa (black chokeberry) pomace was evaluated. Cold maceration of frozen berries with no enzyme showed the highest concentrations of anthocyanin in pomace (Vagiri and Jensen, 2017). Similarly, no effect was observed with direct enzyme addition to anaerobic digestion of olive pomace in order to enhance methane production; however, methane production increased 71% compared to the control after a pre-treatment of enzymatic maceration (Donoso-Bravo et al., 2016). Later and after optimization, using immobilized laccase on porous nanocomposite of Fe3O4@SiO2@KIT-6 for delignification and phenol extraction generated a degradation rate of lignin and phenol of 77.3 and 76.5%, respectively (Amin et al., 2018).
The in vivo Utilization of Enzymatic-Enhancement Systems
Enzymes are ubiquitous catalysts of biochemical reactions and pathways (Bourlieu et al., 2020). The exogenous enzymes used in the animal industry are biotechnological products derived from microorganisms such as bacteria and fungi; together with the endogenous microbial enzymes they degrade the cell walls of ingested feed to release nutrients/bioactives (Bedford and Schulze, 1998; Slominski, 2011; Kiarie et al., 2013). Apart from their help in enzymatic degradation of feed, the hindgut microbes are also an important source of vitamins as well as acting as a biological deterrent to the growth and colonization of the gut by pathogenic bacteria (Coates, 1987).
Poultry Production
Enzymes in poultry diet have been used to increase nutrients utilization and improve digestibility (Kiarie et al., 2014, 2015a, 2017; Gallardo et al., 2017; Sanchez et al., 2019). They influence the absorption of nutrients and produce nutrients for specific bacterial populations through their actions (Bedford and Cowieson, 2012; Kiarie et al., 2013, 2019). The classes of enzymes commonly used in poultry include phytase, carbohydrases (xylanase, cellulase, α-galactosidase, β-mannanase, α-amylase, and pectinase), and proteases (Kiarie et al., 2013). It has been reported that enzymes such as xylanase which is well-accepted in poultry diets, especially those containing wheat, can reduce digesta viscosity, increase nutrient digestion and passage rate, and modify gut microbiota in broilers (Nian et al., 2011; Kiarie et al., 2014, 2017; Munyaka et al., 2016). As shown in Figure 5, a heteroxylan polysaccharide would not have all its various xylosidic or side-chain substrates' linkages accessible to the xylan degradaing enzymes. Thus, specific xylanase enzyme such as xylosidase, glucoronidase, endoxylanase, acetyl xylan esterase and arabinofuranosidase are required. Moreover, these enzymes work in a synergistic way allowing the hydrolytic action of Acetyl xylan esterase in the same heteroxylan to release of acetic acid, which in turn improves the accessibility of the xylan polysaccharide to endoxylanase (Wong et al., 1988; Sunna and Antranikian, 1997). Proteases release amino acids while improving gut development of broilers, increase their bodyweight and improve feed efficiency (Wang et al., 2016). These authors also reported that broilers fed carbohydrases showed a decreased ileal C. perfringens of 15-day-old broilers, higher levels of plasma zeaxanthin at d 22 and higher levels of plasma lutein at d 15 and 22 (Wang et al., 2016).
Slominski (2011) suggested that the main action of enzymes, specifically carbohydrases, is breaking down of high molecular weight polysaccharides into monosaccharides, oligosaccharides and low molecular weight polysaccharides. Exogenous enzymes such as phytases (Bedford and Cowieson, 2012) and proteases (Cowieson and Roos, 2016) have been shown to have beneficial effects on the integrity of the gastro-intestinal system by improving the integrity of intestinal mucin and enterocyte tight junctions integrity as well as by reducing inflammatory activities in the gut, and undigested substrates that could in turn become substrates for pathogenic bacteria and thus improving the general and gut health of the bird. Microbial enzymes have similar beneficial effects of synthesis of short chain fatty acids for energy utilization, protein breakdown and immune support (Bedford and Cowieson, 2012; Kiarie et al., 2013). However, the challenge with microbes is in maintaining a balance between beneficial and pathogenic microflora populations. Thus, this balance could be achieved by the use of exogenous enzymes for in vivo uniform movement of substrate(s) from the fore gut to the hind gut (Kiarie et al., 2013; Cowieson and Kluenter, 2019). The modalities in which exogenous enzymes have an effect in vivo on digestive health, immunological health, physiological health and microbiological health has been well-described. In terms of digestive health, Cowieson and Kluenter (2019) indicated that it is not important to use exogenous enzymes in very young broiler chicks as a way to improve nutrient utilization because their intestine accounts for a bigger proportion of their body mass and their body mass is not too demanding physiologically-speaking from the intestine; but this changes after day 14 when the birds are growing bigger and the intestine and pancreatic tissue diminish in proportion to the metabolic weight. When it comes to physiological health, Cowieson and Kluenter (2019) highlighted the importance of the physical well-being of the intestine, with regard to the integrity of the enterocytes, villi length and brush border integrity, mucin production and also microbial stability. Cowieson et al. (2004) demonstrated that phytase in the diet reduced the loss of sialic acid, a mucoprotein component, that is exacerbated by ingestion of feed rich in phytic acid, and thus phytase degraded the phytate and prevent this loss. Further research by Fernandez et al. (2000) showed that the addition of xylanase to wheat based diets of broilers also increased the production of sulfates mucins, reduced the numbers of Campylobacter jejuni in the ceca while increasing the digestibility of ingested nutrients along with reducing the loss of proteins by interfering with the endogenous protein flow. Table 3 lists examples of well-established usages of natural and recombinant enzymes in the feed industry.
Exogenous enzymes in broiler diets can induce shift of the site of digestion from the posterior to more proximal sections of the gut (Bedford and Cowieson, 2012), resulting in what is termed as the “starvation of the hind gut microbes” from getting important substrates. With reduced substrates for beneficial microbes to metabolize, their proliferation and population reduces, and this puts the colon and large intestine at the risk of proliferation of pathogenic microflora. Addition of xylanase to wheat and maize based broiler diets leads to production of xylo-oligomers, which are more readily fermentable in the posterior gut (Kiarie et al., 2009a, 2014, 2017; Singh et al., 2012). These xylo-oligomers are thought to influence the release of the hormone peptide YY, which is involved in bowel movement and increase the residence time of ingesta to be acted upon by microflora. Moreover, and in addition to the above activities, enzymes such as lysozymes cleave the bond between N-acetylglucosamine and N-acetylmuramic acid of the peptidoglycan in the bacterial cell wall, leading to bacterial death (Cowieson and Kluenter, 2019). Lysosomes are thought to be important host's antibacterial defense factors.
Lastly, with regard to the immunological considerations, enzymes alter the microbiome which in turn influences the host immunity, intestinal integrity and development (Bedford and Cowieson, 2012). For example, xylanase was shown to have a substantial effect in mucin production, increasing the density of goblet cells, and phytase aided reduction of sialic acid loss. Non-starch polysaccharide (NSP) degrading enzymes (NSPases) have been shown to increase mucin production in poultry (Fernandez et al., 2000). Products that elicit enzymatic activities, such as mannan-oligosaccharide derived from yeast cell walls, have been shown to directly influence the immune systems of production birds by activating mononuclear cells (such as macrophages). Dietary mannan-oligosaccharides was reported to increase the humoral response in poultry, following vaccination against Newcastle disease virus and Gumboro (Oliveira et al., 2009).
Swine Production
Generally, the benefits of using exogenous feed enzymes have not been profound in swine compared to poultry due to various differences between these two species. These differences include gastrointestinal anatomy, characteristics of digesta, the digestive capacity, the chemical environment of the gut, and transit time in the gut (Ravindran and Son, 2011; Owusu-Asiedu et al., 2012; Kiarie et al., 2013, 2016a; Ndou et al., 2015; Walsh et al., 2016; Rho et al., 2018).
With pigs having a longer intestinal length, the ingesta have a longer mean retention time (MRT) of 4-6 h and the nutrients are better extracted. This differs with poultry, which have shorter gut, and hence have shorter MRT of about 30 min to an hour in the gizzard and small intestine, respectively (Ravindran and Son, 2011). Additionally, pigs consume lots of water during feeding improving thus, the viscosity of the digesta, especially from NSP diets like wheat, barley and rye. In contrast, poultry, whether, broilers, layers or turkeys, have a crop where-in the exogenous enzyme will become activated before it reaches the more acidic sections of the gut (Dierick, 1994). The exogenous enzyme's optimal pH value determines whether the enzyme can work in less acidic conditions like the crop or more acidic conditions in the proventriculus and further down the gut. The longer MRT in pigs and their large colon and caecum provide for a higher proliferation of bacteria in these gut regions and translates to better opportunities for microbial fermentation of fibers to produce volatile fatty acids than in poultry. Nonetheless, at the foregut, the proliferation of pathogenic bacteria may cause disturbances in the animal and potentially a state of disease. This large proliferation of gut microbiota is sometimes touted as a reason for lesser exogenous enzyme action in swine, compared to poultry. Nonetheless, the use of exogenous enzymes have shown better performance results in young pigs with various studies (Sudendey and Kamphues, 1995; Kiarie, 2008; Kiarie et al., 2008a, 2009a,b, 2015b; Agyekum et al., 2015) showing the effects of the enzymes in reducing the negative effects of viscosity in wheat and barley based diets. Xylanases are the predominant enzymes used in these diets due to the fact that the main NSPs found in wheat and barley are the arabinoxylans.
Mechanisms behind the efficacy of exogenous enzymes used in swine is complex and need to be established. Several factors related to these complexities include the potential of enzymes to change gut microflora, enzyme combinations and sources of feed. It is also very critical to understand the role of enzymes in gut physiology and its influence on the chemical and hormonal aspects of the gut (Dierick and Decuypere, 1996; Kiarie et al., 2013, 2016a; Agyekum et al., 2016).
The Physiological Capacity of Pomaces-Degrading Enzymes to Support Animal Production
Characterization of fruit pomaces that are destined for animal feeding applications is important due to the varying compositions of such additives. For example, characterized cranberry and blueberry pomaces are usually reported with total carbohydrate contents of 88.78 and 84.91%, respectively (Ross et al., 2017). Similarly, apple pomaces have carbohydrate contents of 84.7%, of which 5.6% is starch on average (Perussello et al., 2017) and encompassing dietary fibers, hemicellulose and pectins.
Complex polysaccharides within the pomace need to be solubilized in the hindgut for proper fermentation, which results in the production of short chain fatty acids that increase energy utilization in the animal. Breaking down carbohydrate-protein bonds in pomaces is also vital to improve amino acid availability for absorption/utilization by the animal. Furthermore, when the NSP are enzymatically hydrolyzed in the hindgut, it results in prebiotic products that ameliorate gut health in chickens and pigs (Kiarie and Nyachoti, 2008; Kiarie et al., 2008b, 2009b, 2010; Slominski, 2011). Some feed components such as pectins, heteropolysaccharides that are composed of galacturonic acid and methoxyl esters, does also interact and bind some of the polyphenols that are found in apple pomace.
Poultry
In formulating pomace-based poultry diets, the pomace ingredients would be formulated together with other ingredients such as corn, soybean meal and wheat that could result in an increase in the NSPs. In the grains, the main NSPs are cellulose and non-cellulosic polysaccharides. In corn, the NSPs are comprised of non-cellulosic polysaccharides such as arabinoxylans and β-glucans; in soybean it consists of arabinans, arabinogalactans, galactans, galactomannans, mannans, and pectic polysaccharides and in wheat the non-cellulosic polysaccharides are more water soluble and viscous β-glucans and arabinoxylans that are notorious for making ingesta doughy and for interfering with digesta movement (Slominski, 2011). This creates problems of sticky fecal droppings in poultry housings and also the impeded digesta does have an effect in reducing the performance and health of the birds (Graham and Åman, 1991).
Therefore, this necessitates the use of NSP degrading-enzymes such as β-glucanases and xylanases, which would aid in better energy and protein utilization of the birds. Due to the complexity of the different NSPs in the diet derived from the varied feed ingredients including pomace, the best approach would be to use a cocktail of multi-carbohydrase enzymes that would induce a better cell-wall breakdown and hydrolysis of the NSPs. The use of multi-carbohydrase enzymes with canola meal has been shown to improve nutrient and energy utilization and fiber (NSP) solubilization for effective hind gut fermentation in broiler chicks (Gallardo et al., 2017). This breakdown is associated with degradation of the anti-nutritive factors in feed (Kiarie et al., 2016b) and according to Slominski (2011) it also leads to the release of starch, fats, proteins and minerals hence improves energy utilization. The use of a cock-tail of enzymes instead of a single pure enzyme(s) is beneficial for pomaces since pomaces are structurally complex and the nutrients and bio-phenols in these pomaces may not exist in simple entities but rather as complexes of proteins, fats, carbohydrates and fibers (Ravindran, 2013). Various studies of using enzymes in poultry feed (Karimi et al., 2013; Gallardo et al., 2017) showed the beneficial effects of their use. Table 4 shows the growth performance of broiler chickens and turkeys that were fed corn-soybean meal-based diets supplemented with different cocktail of enzymes.
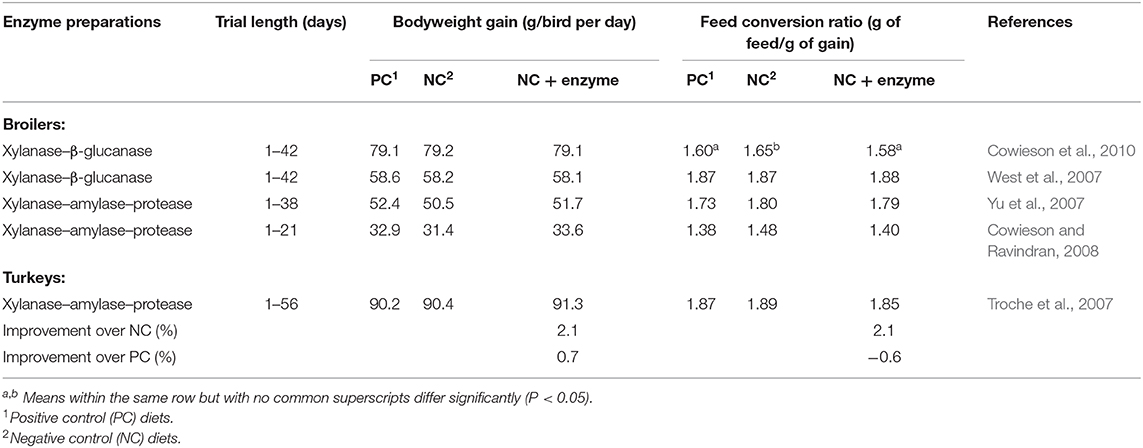
Table 4. Growth performance of broiler chickens and turkeys fed corn-soybean meal based diets with or without different cocktail of enzymes [adapted from Slominski (2011)].
In broilers, studies in the past three decades showed that this disruption of cell-wall matrix and release of encapsulated nutrients greatly leads to the improvement of growth performance of birds and some researchers including Acamovic (2001) have suggested that NSPs from wheat could act as a physical entrapment that prevent or slow down the endogenous enzymes to reach their substrates. Other experiments showed that the intact NSPs cell walls when tracked microscopically encapsulated starch, and that the use of NSP-depolymerizing enzymes released these nutrients for absorption and utilization by birds (Bedford and Autio, 1996).
The efficacy of multiple multi-carbohydrase enzymes to improve nutrient utilization and growth performance in broilers was determined (Meng et al., 2005). Using wheat, soybean meal, canola meal and peas as substrates, the authors showed that the body weight gain of birds reared on enzyme-supplemented diets was higher than in control diets. The combination of enzymes C+P+XG+MC produced the highest improvement in the broilers specific parameters: 4%, 9%, 144kcal/kg, 8%, and 8 units for starch and protein digestibility, apparent metabolizable energy, weight gain, and feed-to-gain ratio, respectively (Meng et al., 2005).
Swine
As is with the poultry diets, pigs are fed mainly grain-based diets around the world making therefore, carbohydrase enzymes pivotal in swine production. The viscosity of digesta again comes up since these cereal-based diets contain high molecular weight and soluble NSPs, which have a negative effect on the digesta movement. It was demonstrated that digesta dry matter flows of piglets and growers, from the fore stomachs to the hind stomach were significantly increased in diets with added exogenous enzymes amylase, β glucanase and xylanase, due to a reduction of viscosity (Sudendey and Kamphues, 1995).
Exogenous enzymes have also been suggested to influence or stimulate feed intake in piglets, which could contribute to higher daily live weight gains than diets without enzymes (Haberer et al., 1997).
It has been reported that exogenous xylanases break down fermentable cecal substrates in the pig's hind gut leading to products that influence enteroglucagon levels, which in turn influence gastrointestinal motility and emptying (Bedford and Schulze, 1998). This loop creates a faster flow rate of digesta than in diets without xylanase, which could be a reason for the increased intake, and nutrient availability in the small intestine, even though further studies are needed to ascertain this physiologic capacity.
Concerns and Perspectives
The antimicrobial benefits of fruit pomaces have been widely researched. However, these products still need to be fully adopted to exploit their promising bioactive molecules. Various products of pomaces such as non-dialyzable extracts of cranberries have been demonstrated, with yet to be determined mechanisms, to have humoral immune response in poultry with phagocytic and bactericidal effects on Staphylococcus aureus, whilst also having antioxidant activity (Islam et al., 2017).
In humans, cranberry juice has been shown to reduce E. coli bacterial adhesion to the urinary tract and Helicobacter to the gastric mucosa, an occurrence that has not been replicated in other pomaces such as blueberry, grape, apple and orange (Johnson-White et al., 2006). On the other hand, grape pomace has been touted for its antioxidant properties inhibiting the lipid oxidation of fish, turkey meat (Pazos et al., 2005), and in rats it has been shown to diminish lipid peroxidation of liver, kidneys and lungs tissues. Despite the present evidence showing that it had no impact on growth performances of chicken, it has been demonstrated that grape pomace extracts interacted with intestinal microflora to reduce the number of undesirable bacteria such as Clostridia, while increasing the population of positive ones such as Lactobacillus and Bifidobacterium (Papadopoulou et al., 2005). The pleiotropic effects of pomace seem to be linked to their numerous chemical contents many of which still need to be identified and characterized in future research.
However, with a limited number of studies showing a depressed growth performance in birds fed with grape seed extracts for instance, attributed probably to high tannin levels (Hughes et al., 2005), a question on the optimization of these products does linger in parallel to the side effects of the known and unknown anti-nutritive factors that might be present in fruit pomaces. Alienable literatures suggest that the beneficial effects of pomaces to both animal- and human-nutrition are forthcoming but are inconclusive awaiting more optimization and standardization. As studies progress toward microbiota-microbiome research, more focus needs to be applied on identifying the relationships between beneficial and pathogenic bacteria under -phytochemicals feeding, their synergy and/or discordance in the gut function and immune status of the host. Investigating the role of other factors such as genetic, epigenetic, environmental, management, diet, etc. that could improve the cost benefits of pomace will provide a broad and in-depth understanding of future research directions as well as what type of questions need to be addressed first.
Conclusions
Overall, this review provides insights on the power of enzyme-assisted fermentation-to enhance fruit pomaces bioactive and nutrient contents for animal (specifically poultry and swine) feeding applications. The growing number of purified fungal and bacterial enzymes and theirs recombinant-products make their usage more practical and economically appealing for both farmers and producers alike. Certainly, there are many technical issues that still exist and which need to be addressed. Many research and development efforts are scrutinizing the conditions surrounding the bioactive-compounds production from pomaces, the chemical and structural targets of the involved enzymes within the utilized pomaces, the molecular targets of the released bioactives within the animal's tissues and organs, and finally animal-responses and the overall impacts of such harnessed bioactives on animal health and performance. However, it is a matter of time before pomaces (still viewed as a burden within some industries) take their proper place within the animal production chain and start to get funneled into more sustainable green solutions within the circular economies. This matter of time, so to say, shall give research and development the space to continue working on fractions and sub-fractions of pomace bioactives that will be more cellular level targeted in their actions. With different pomaces producing varied bioactives, research and development would enable to extract the beneficial pomace components and possibly use them either singly or in combination, as feed additives. Despite that most of previously reported research efforts had focused merely on the industrial usage of pomaces within the ethanol industry rather than animal feed applications and while the ethanol production and processing differs fundamentally from feed operations, lessons learned from that industry (which rely heavily on the usage of commercially available biomass/fiber-degrading enzymes) can give many insights in regard to future usage of pomaces and enzymes in the feed industry.
Author Contributions
MD (the principal investigator), YH, and MK conceptualized the review. MD and EK contributed resources, reviewed and edited the manuscript. MK, YH, and KG wrote the paper. MD provided overall guidance and mentorship, throughout the scope of this review. All authors read and approved the final manuscript.
Funding
This work was supported by Agriculture and Agri-Food Canada (AAFC) through the Organic Science Cluster 3 program (Project PSS #2196, J-002173.001.06) and Natural Sciences and Engineering Research Council (NSERC)-Discovery program #RGPIN-2017-04090.
Conflict of Interest
The authors declare that the research was conducted in the absence of any commercial or financial relationships that could be construed as a potential conflict of interest.
Acknowledgments
The authors thank Xianhua Yin for his assistance and help.
References
Acamovic, T. (2001). Commercial application of enzyme technology for poultry production. World's Poultry Sci. J. 57, 225–242. doi: 10.1079/WPS20010016
Agyekum, A. K., Kiarie, E., Walsh, M. C., and Nyachoti, C. M. (2016). Postprandial portal fluxes of essential amino acids, volatile fatty acids, and urea-nitrogen in growing pigs fed a high-fiber diet supplemented with a multi-enzyme cocktail. J. Anim. Sci. 94, 3771–3785. doi: 10.2527/jas.2015-0077
Agyekum, A. K., Sands, J. S., Regassa, A., Kiarie, E., Weihrauch, D., Kim, W. K., et al. (2015). Effect of supplementing a fibrous diet with a xylanase and beta-glucanase blend on growth performance, intestinal glucose uptake, and transport-associated gene expression in growing pigs. J. Anim. Sci. 93, 3483–3493. doi: 10.2527/jas.2015-9027
Alagawany, M., Elnesr, S. S., and Farag, M. R. (2018). The role of exogenous enzymes in promoting growth and improving nutrient digestibility in poultry. Iran J. Vet. Res. 19, 157–164.
Almeida-Trasviña, F., Medina-González, S., Ortega-Rivas, E., Salmerón-Ochoa, I., and Pérez-Vega, S. (2014). Vacuum drying optimization and simulation as a preservation method of antioxidants in apple pomace. J. Food Process Eng. 37, 575–587. doi: 10.1111/jfpe.12112
Alokika, S. D., and Singh, B. (2018). Utility of acidic xylanase of Bacillus subtilis subsp. subtilis JJBS250 in improving the nutritional value of poultry feed. 3 Biotech 8:503. doi: 10.1007/s13205-018-1526-2
Amin, R., Khorshidi, A., Shojaei, A. F., Rezaei, S., and Faramarzi, M. A. (2018). Immobilization of laccase on modified Fe3O4@ SiO2@ Kit-6 magnetite nanoparticles for enhanced delignification of olive pomace bio-waste. Int. J. Biol. Macromol. 114, 106–113. doi: 10.1016/j.ijbiomac.2018.03.086
Andreasen, M. F., Kroon, P. A., Williamson, G., and Garcia-Conesa, M.-T. (2001). Esterase activity able to hydrolyze dietary antioxidant hydroxycinnamates is distributed along the intestine of mammals. J. Agric. Food Chem. 49, 5679–5684. doi: 10.1021/jf010668c
Bedford, M. R., and Autio, K. (1996). Microscopic examination of feed and digesta from wheat-fed broiler chickens and its relation to bird performance. Poul. Sci. 75.1, 1–14.
Bedford, M. R., and Cowieson, A. J. (2012). Exogenous enzymes and their effects on intestinal microbiology. Anim. Feed Sci. Technol. 173, 76–85. doi: 10.1016/j.anifeedsci.2011.12.018
Bedford, M. R., and Schulze, H. (1998). Exogenous enzymes for pigs and poultry. Nutr. Res. Rev. 11, 91–114. doi: 10.1079/NRR19980007
Beermann, C., Gruschwitz, N., Walkowski, K., and Göpel, A. (2018). Growth modulating properties of polyphenolic apple pomace extract on food associated microorganisms. J. Microbiol. Biotechnol. Food Sci. 3, 176.
Bertelli, A. A., Gozzini, A., Stradi, R., Stella, S., and Bertelli, A. (1998). Stability of resveratrol over time and in the various stages of grape transformation. Drugs Exp. Clin. Res. 24, 207–211.
Bhatia, S., Singh, A., Batra, N., and Singh, J. (2020). Microbial production and biotechnological applications of α-galactosidase. Int. J. Biol. Macromol. 150, 1294–1313. doi: 10.1016/j.ijbiomac.2019.10.140
Bode, L. M., Bunzel, D., Huch, M., G.-,Cho, S., Ruhland, D., Bunzel, M., et al. (2013). In vivo and in vitro metabolism of trans-resveratrol by human gut microbiota. Am. J. Clin. Nutr. 97, 295–309. doi: 10.3945/ajcn.112.049379
Bourlieu, C., Astruc, T., Barbe, S., J.-,Berrin, G., Bonnin, E., Boutrou, R., et al. (2020). Enzymes to unravel bioproducts architecture. Biotechnol. Adv. 41. doi: 10.1016/j.biotechadv.2020.107546
Bowey, E., Adlercreutz, H., and Rowland, I. (2003). Metabolism of isoflavones and lignans by the gut microflora: a study in germ-free and human flora associated rats. Food Chem. Toxicol. 41, 631–636. doi: 10.1016/S0278-6915(02)00324-1
Chamorro, S., Viveros, A., Rebolé, A., Rica, B., Arija, I., and Brenes, A. (2015). Influence of dietary enzyme addition on polyphenol utilization and meat lipid oxidation of chicks fed grape pomace. Food Res. Int. 73, 197–203. doi: 10.1016/j.foodres.2014.11.054
Chapman, G. W., and Horvat, R. J. (1989). Determination of nonvolatile acids and sugars from fruits and sweet potato extracts by capillary GLC and GLC/MS. J. Agric. Food Chem. 37, 947–950. doi: 10.1021/jf00088a026
Chedea, V. S., Palade, L. M., Marin, D. E., Pelmus, R. S., Habeanu, M., Rotar, M. C., et al. (2018). Intestinal absorption and antioxidant activity of grape pomace polyphenols. Nutrients 10:588. doi: 10.3390/nu10050588
Chen, L., Cao, H., and Xiao, J. (2018). “2 - Polyphenols: Absorption, bioavailability, and metabolomics,” in Polyphenols: Properties, Recovery, and Applications, ed C. M. Galanakis, (Fuzhou: Woodhead Publishing), 45–67. doi: 10.1016/B978-0-12-813572-3.00002-6
Chung, K. T., Wong, T. Y., Wei, C. I., Huang, Y. W., and Lin, Y. (1998). Tannins and human health: a review. Crit. Rev. Food Sci. Nutr. 38, 421–464. doi: 10.1080/10408699891274273
Clarke, L., Sweeney, T., Curley, E., Duffy, S., Rajauria, G., and O'Doherty, J. (2018). The variation in chemical composition of barley feed with or without enzyme supplementation influences nutrient digestibility and subsequently affects performance in piglets. J. Anim. Physiol. Anim. Nutr. 102, 799–809. doi: 10.1111/jpn.12870
Coates, M. (1987). Gnotobiotes in nutritional studies. Food/Nahrung 31, 591–598. doi: 10.1002/food.19870310577
Colombino, E., Ferrocino, I., Biasato, I., Cocolin, L. S., Prieto-Botella, D., Zduńczyk, Z., et al. (2020). Dried fruit pomace inclusion in poultry diet: growth performance, intestinal morphology and physiology. J. Anim. Sci. Biotechnol. 11, 63–63. doi: 10.1186/s40104-020-00464-z
Corrêa, T. A. F., Rogero, M. M., N., Hassimotto, M. A., and Lajolo, F. M. (2019). The two-way polyphenols-microbiota interactions and their effects on obesity and related metabolic diseases. Front Nutr. 6:188. doi: 10.3389/fnut.2019.00188
Costoya, N., Sineiro, J., Pinelo, M., Rubilar, M., and Nuñez, M. J. (2010). Enzyme-aided extraction of polyphenols from grape pomace. Elec. J. Env. Agricult. Food Chem. 9, 696–705.
Cowieson, A., Acamovic, T., and Bedford, M. (2004). The effects of phytase and phytic acid on the loss of endogenous amino acids and minerals from broiler chickens. Br. Poult. Sci. 45, 101–108. doi: 10.1080/00071660410001668923
Cowieson, A., Bedford, M., and Ravindran, V. (2010). Interactions between xylanase and glucanase in maize-soy-based diets for broilers. Br. Poult. Sci. 51, 246–257. doi: 10.1080/00071661003789347
Cowieson, A., and Kluenter, A. (2019). Contribution of exogenous enzymes to potentiate the removal of antibiotic growth promoters in poultry production. Anim. Feed Sci. Technol. 250, 81–92. doi: 10.1016/j.anifeedsci.2018.04.026
Cowieson, A., and Ravindran, V. (2008). Effect of exogenous enzymes in maize-based diets varying in nutrient density for young broilers: growth performance and digestibility of energy, minerals and amino acids. Br. Poult. Sci. 49, 37–44. doi: 10.1080/00071660701812989
Cowieson, A. J., and Roos, F. F. (2016). Toward optimal value creation through the application of exogenous mono-component protease in the diets of non-ruminants. Anim. Feed Sci. Technol. 221, 331–340. doi: 10.1016/j.anifeedsci.2016.04.015
Cutlan, R., De Rose, S., Isupov, M. N., Littlechild, J. A., and Harmer, N. J. (2020). Using enzyme cascades in biocatalysis: Highlight on transaminases and carboxylic acid reductases. Biochim. Biophys. Acta Proteins Proteom. 1868:140322. doi: 10.1016/j.bbapap.2019.140322
Das, Q., Islam, M. R., Lepp, D., Tang, J., Yin, X., Mats, L., et al. (2020). Gut microbiota, blood metabolites, and spleen immunity in broiler chickens fed berry pomaces and phenolic-enriched extractives. Front Vet Sci. 7:150. doi: 10.3389/fvets.2020.00541
Das, Q., Lepp, D., Yin, X., Ross, K., McCallum, J. L., Warriner, K., et al. (2019). Transcriptional profiling of Salmonella enterica serovar Enteritidis exposed to ethanolic extract of organic cranberry pomace. PLoS One 14, e0219163. doi: 10.1371/journal.pone.0219163
Déprez, S., p., Brezillon, C., Rabot, S., Philippe, C., Mila, I., et al. (2000). Polymeric proanthocyanidins are catabolized by human colonic microflora into low-molecular-weight phenolic acids. J. Nutr. 130, 2733–2738. doi: 10.1093/jn/130.11.2733
DeRuiter, F. E., and Dwyer, J. (2002). Consumer acceptance of irradiated foods: dawn of a new era? Food Service Technol. 2, 47–58. doi: 10.1046/j.1471-5740.2002.00031.x
Dierick, N. (1994). Decuypere, Enzymes and Growth in Pigs. Nottingham: Nottingham University Press, 169–195.
Dierick, N., and Decuypere, J. (1996). Mode of Action of Exogenous Enzymes in Growing Pig Nutrition. Ghent: University of Ghent.
Donoso-Bravo, A., Ortega-Martinez, E., and Ruiz-Filippi, G. (2016). Impact of milling, enzyme addition, and steam explosion on the solid waste biomethanation of an olive oil production plant. Bioprocess Biosyst. Eng. 39, 331–340. doi: 10.1007/s00449-015-1519-z
Dulf, F. V., Vodnar, D. C., Tosa, M. I., and Dulf, E. H. (2019). Simultaneous enrichment of grape pomace with gamma-linolenic acid and carotenoids by solid-state fermentation with Zygomycetes fungi and antioxidant potential of the bioprocessed substrates. Food Chem. 310:125927. doi: 10.1016/j.foodchem.2019.125927
Eisinaite, V., Leskauskaite, D., Pukalskiene, M., and Venskutonis, P. R. (2020). Freeze-drying of black chokeberry pomace extract-loaded double emulsions to obtain dispersible powders. J Food Sci. 85, 628–638. doi: 10.1111/1750-3841.14995
Faria, A., Fernandes, I., Norberto, S., Mateus, N., and Calhau, C. (2014). Interplay between anthocyanins and gut microbiota. J. Agric. Food Chem. 62, 6898–6902. doi: 10.1021/jf501808a
Fernandez, F., Sharma, R., Hinton, M., and Bedford, M. (2000). Diet influences the colonisation of Campylobacter jejuni and distribution of mucin carbohydrates in the chick intestinal tract. Cell. Mol. Life Sci. 57, 1793–1801. doi: 10.1007/PL00000659
Ferri, M., Bin, S., Vallini, V., Fava, F., Michelini, E., Roda, A., et al. (2016). Recovery of polyphenols from red grape pomace and assessment of their antioxidant and anti-cholesterol activities. New Biotechnol. 33, 338–344. doi: 10.1016/j.nbt.2015.12.004
Folmer, F., Basavaraju, U., Jaspars, M., Hold, G., El-Omar, E., Dicato, M., et al. (2014). Anticancer effects of bioactive berry compounds. Phytochem. Rev. 13, 295–322. doi: 10.1007/s11101-013-9319-z
Gabriel, L. S., Prestes, R. A., Pinheiro, L. A., Barison, A., and Wosiacki, G. (2013). Multivariate analysis of the spectroscopic profile of the sugar fraction of apple pomace. Braz. Arch. Biol. Technol. 56, 439–446. doi: 10.1590/S1516-89132013000300012
Gallardo, C., Dadalt, J. C., Kiarie, E., and Trindade Neto, M. A. d. (2017). Effects of multi-carbohydrase and phytase on standardized ileal digestibility of amino acids and apparent metabolizable energy in canola meal fed to broiler chicks. Poult. Sci. 96, 3305–3313. doi: 10.3382/ps/pex141
Gama, R., Van Dyk, J. S., and Pletschke, B. I. (2015). Optimisation of enzymatic hydrolysis of apple pomace for production of biofuel and biorefinery chemicals using commercial enzymes. 3 Biotech 5, 1075–1087. doi: 10.1007/s13205-015-0312-7
Gifre, L., Arís, A., Bach, À., and Garcia-Fruitós, E. (2017). Trends in recombinant protein use in animal production. Microbial. Cell Factories 16:40. doi: 10.1186/s12934-017-0654-4
Gonçalves, S., and Romano, A. (2017). “Inhibitory properties of phenolic compounds against enzymes linked with human diseases,” in Phenolic Compounds-Biological Activity. Faro: IntechOpen. doi: 10.5772/66844
Goñi, I., Brenes, A., Centeno, C., Viveros, A., Saura-Calixto, F., Rebolé, A., et al. (2007). Effect of dietary grape pomace and vitamin e on growth performance, nutrient digestibility, and susceptibility to meat lipid oxidation in chickens. Poult. Sci. 86, 508–516. doi: 10.1093/ps/86.3.508
González-Domínguez, R., Sayago, A., and Fernández-Recamales, Á. (2019). Engineering Tools in the Beverage Industry. Volume 3: The Science of Beverages. Woodhead. doi: 10.1016/C2017-0-02377-7
Graham, H., and Åman, P. (1991). Nutritional aspects of dietary fibres. Anim. Feed Sci. Technol. 32, 143–158. doi: 10.1016/0377-8401(91)90018-N
Guaita, M., and Bosso, A. (2019). Polyphenolic characterization of grape skins and seeds of four italian red cultivars at harvest and after fermentative maceration. Foods 8:365. doi: 10.3390/foods8090395
Haberer, B., Schulz, E., and Aulrich, K. (1997). Effects of β-glucanase and xylanase supplementation in pigs fed a diet rich in nonstarch polysaccharides: composition of digesta in different prececal segments and postprandial time. J. Anim. Physiol. Anim. Nutr. 78, 84–94. doi: 10.1111/j.1439-0396.1997.tb00859.x
Hassan, Y. I., Kosir, V., Yin, X., Ross, K., and Diarra, M. S. (2019). Grape pomace as a promising antimicrobial alternative in feed: a critical review. J. Agric. Food Chem. 67, 9705–9718. doi: 10.1021/acs.jafc.9b02861
Hemingway, R. W., and Laks, P. E. (2012). Plant Polyphenols: Synthesis, Properties, Significance. Vol. 59. Houghton, MI: Springer Science & Business Media.
Hirano, S., Itakura, C., Seino, H., Akiyama, Y., Nonaka, I., Kanbara, N., et al. (1990). Chitosan as an ingredient for domestic animal feeds. J. Agric. Food Chem. 38, 1214–1217. doi: 10.1021/jf00095a012
Hölker, U., and Lenz, J. (2005). Solid-state fermentation—are there any biotechnological advantages? Curr. Opin. Microbiol. 8, 301–306. doi: 10.1016/j.mib.2005.04.006
Hossain, S. M., and Blair, R. (2007). Chitin utilisation by broilers and its effect on body composition and blood metabolites. Br. Poult. Sci. 48, 33–38. doi: 10.1080/00071660601156529
Howard, R., Abotsi, E., Van Rensburg, E. J., and Howard, S. (2003). Lignocellulose biotechnology: issues of bioconversion and enzyme production. African J. Biotechnol. 2, 602–619. doi: 10.5897/AJB2003.000-1115
Hughes, R. J., Brooker, J. D., and Smyl, C. (2005). Growth rate of broiler chickens given condensed tannins extracted from grape seed. Aust. Poult. Sci. Symp. 17, 65–68.
Iandolo, D., Piscitelli, A., Sannia, G., and Faraco, V. (2011). Enzyme production by solid substrate fermentation of Pleurotus ostreatus and Trametes versicolor on tomato pomace. Appl. Biochem. Biotechnol. 163, 40–51. doi: 10.1007/s12010-010-9014-0
Ibrahim, Y. H. Y., Regdon, G. Jr., Hamedelniel, E. I., and Sovany, T. (2019). Review of Recently Used Techniques and Materials to Improve the Efficiency of Orally Administered Proteins/Peptides. Daru: Springer. doi: 10.1007/s40199-019-00316-w
Iqbal, Y., Cottrell, J. J., H., Suleria, A. R., and Dunshea, F. R. (2020). Gut microbiota-polyphenol interactions in chicken: a review. Animal 10:1391. doi: 10.3390/ani10081391
Islam, M. R., Oomah, D. B., and Diarra, M. S. (2017). Potential immunomodulatory effects of non-dialyzable materials of cranberry extract in poultry production. Poult. Sci. 96, 341–350. doi: 10.3382/ps/pew302
Jatuwong, K., Suwannarach, N., Kumla, J., Penkhrue, W., Kakumyan, P., and Lumyong, S. (2020). Bioprocess for production, characteristics, and biotechnological applications of fungal phytases. Front. Microbiol. 11:188. doi: 10.3389/fmicb.2020.00188
Johnson-White, B., Buquo, L., Zeinali, M., and Ligler, F. S. (2006). Prevention of nonspecific bacterial cell adhesion in immunoassays by use of cranberry juice. Anal. Chem. 78, 853–857. doi: 10.1021/ac051700v
Juskiewicz, J., Jankowski, J., Kosmala, M., Zdunczyk, Z., Slominski, B. A., and Zdunczyk, P. (2016). The effects of dietary dried fruit pomaces on growth performance and gastrointestinal biochemistry of turkey poults. J. Anim. Physiol. Anim. Nutr. 100, 967–976. doi: 10.1111/jpn.12415
Juśkiewicz, J., Zary-Sikorska, E., Zdunczyk, Z., Król, B., Jaroslawska, J., and Jurgoński, A. (2011). Effect of dietary supplementation with unprocessed and ethanol-extracted apple pomaces on caecal fermentation, antioxidant and blood biomarkers in rats. Br. J. Nutr. 107, 1138–1146. doi: 10.1017/S0007114511004144
Kabir, F., Sultana, M. S., and Kurnianta, H. (2015). Polyphenolic contents and antioxidant activities of underutilized grape (Vitis vinifera L.) pomace extracts. Prev. Nutr. Food Sci. 20, 210–214. doi: 10.3746/pnf.2015.20.3.210
Kalita, D., Holm, D. G., LaBarbera, D. V., Petrash, J. M., and Jayanty, S. S. (2018). Inhibition of α-glucosidase, α-amylase, and aldose reductase by potato polyphenolic compounds. PLoS ONE 13:e0191025. doi: 10.1371/journal.pone.0191025
Karimi, A., Min, Y., Lu, C., Coto, C., Bedford, M., and Waldroup, P. (2013). Assessment of potential enhancing effects of a carbohydrase mixture on phytase efficacy in male broiler chicks fed phosphorus-deficient diets from 1 to 18 days of age. Poult. Sci. 92, 192–198. doi: 10.3382/ps.2012-02558
Karpe, A. V., Dhamale, V. V., Morrison, P. D., Beale, D. J., Harding, I. H., and Palombo, E. A. (2017). Winery biomass waste degradation by sequential sonication and mixed fungal enzyme treatments. Fungal Genet. Biol. 102, 22–30. doi: 10.1016/j.fgb.2016.08.008
Kiarie, E. (2008). Dietary Means for Enhanced Gut Health and Function in Piglets: An Evaluation of Carbohydrase Enzymes Targeting Non-Starch Polysaccharides, in Animal Science. Winnipeg: University of Manitoba, 232.
Kiarie, E., Krause, D. O., and Nyachoti, C. M. (2008a). Net fluid and electrolyte losses in enterotoxigenic Escherichia coli-infected piglet small intestine upon perfusion with fumaric acid, zinc oxide, egg yolk antibodies or carbadox. Can. J. Anim. Sci. 88, 485–488. doi: 10.4141/CJAS08011
Kiarie, E., and Nyachoti, P. M. (2008). Dietary Means for Enhanced Gut Health and Function in Piglets: An Evaluation of Carbohydrase Enzymes Targeting Non-starch Polysaccharides. Winnipeg: University of Manitoba, 232–232.
Kiarie, E., Romero, L. F., and Nyachoti, C. M. (2013). The role of added feed enzymes in promoting gut health in swine and poultry. Nutr. Res. Rev. 26, 71–88. doi: 10.1017/S0954422413000048
Kiarie, E., Romero, L. F., and Ravindran, V. (2014). Growth performance, nutrient utilization, and digesta characteristics in broiler chickens fed corn or wheat diets without or with supplemental xylanase. Poult. Sci. 93, 1186–1196. doi: 10.3382/ps.2013-03715
Kiarie, E., Slominski, B. A., Krause, D. O., and Nyachoti, C. M. (2009a). Gastrointestinal ecology response of piglets fed diets containing non-starch polysaccharide hydrolysis products and egg yolk antibodies following an oral challenge with Escherichia coli (K88). Can. J. Anim. Sci. 89, 341–352. doi: 10.4141/CJAS09007
Kiarie, E., Slominski, B. A., Krause, D. O., and Nyachoti, C. M. (2009b). Acute phase response of piglets fed diets containing non-starch polysaccharide hydrolysis products and egg yolk antibodies following an oral challenge with Escherichia coli (K88). Can. J. Anim. Sci. 89, 353–360. doi: 10.4141/CJAS09008
Kiarie, E., Walsh, M., He, L., Velayudhan, D., Yin, Y., and Nyachoti, C. (2016b). Phytase improved digestible protein, phosphorous, and energy contents in camelina expellers fed to growing pigs. J. Anim. Sci. 94, 215–218. doi: 10.2527/jas.2015-9735
Kiarie, E., Walsh, M. C., and Nyachoti, C. M. (2016a). Performance, digestive function, and mucosal responses to selected feed additives for pigs. J. Anim. Sci. 94, 169–180. doi: 10.2527/jas.2015-9835
Kiarie, E., Walsh, M. C., Romero, L. F., Arent, S., and Ravindran, V. (2017). Nutrient and fiber utilization responses of supplemental xylanase in broiler chickens fed wheat based diets are independent of the adaptation period to test diets. Poult. Sci. 96, 3239–3245. doi: 10.3382/ps/pex100
Kiarie, E., Walsh, M. C., Romero, L. F., Yang, X., and Baidoo, S. K. (2015b). Comparative efficacy of a blend of multiple enzymes and an in-feed antibiotic on growth performance and apparent digestibility of energy and protein in nursery pigs. Anim. Prod. Sci. 55:1540. doi: 10.1071/ANv55n12Ab072
Kiarie, E., Woyengo, T., and Nyachoti, C. M. (2015a). Efficacy of new 6-phytase from buttiauxella spp. on growth performance and nutrient retention in broiler chickens fed corn soybean meal-based diets. Asian-Australas J. Anim. Sci. 28, 1479–1487. doi: 10.5713/ajas.15.0059
Kiarie, E. G., Leung, H. R., Akbari Moghaddam Kakhki, Patterson, R., and Barta, J. R. (2019). Utility of feed enzymes and yeast derivatives in ameliorating deleterious effects of coccidiosis on intestinal health and function in broiler chickens. Front. Vet. Sci. 6:473. doi: 10.3389/fvets.2019.00473
Kiarie, E. G., and Mills, A. (2019). Role of feed processing on gut health and function in pigs and poultry: conundrum of optimal particle size and hydrothermal regimens. Front. Vet. Sci. 6:19. doi: 10.3389/fvets.2019.00019
Kiarie, E. G., Slominski, B. A., Krause, D. O., and Nyachoti, C. M. (2008b). Nonstarch polysaccharide hydrolysis products of soybean and canola meal protect against enterotoxigenic Escherichia coli in piglets. J. Nutr. 138, 502–508. doi: 10.1093/jn/138.3.502
Kiarie, E. G., Slominski, B. A., and Nyachoti, C. M. (2010). Effect of products derived from hydrolysis of wheat and flaxseed non starch polysaccharides by carbohydrase enzymes on net absorption in enterotoxigenic Escherichia coli (K88) challenged piglet jejunal segments. Anim. Sci. J. 81, 63–71. doi: 10.1111/j.1740-0929.2009.00716.x
Kitryte, V., Narkeviciute, A., Tamkute, L., Syrpas, M., Pukalskiene, M., and Venskutonis, P. R. (2020). Consecutive high-pressure and enzyme assisted fractionation of blackberry (Rubus fruticosus L.) pomace into functional ingredients: process optimization and product characterization. Food Chem. 312:126072. doi: 10.1016/j.foodchem.2019.126072
Krygier, S., Solbak, A., Shanahan, D., and Ciofalo, V. (2014). Safety evaluation of phytase 50104 enzyme preparation (also known as VR003), expressed in Pseudomonas fluorescens, intended for increasing digestibility of phytate in monogastrics. Regul. Toxicol. Pharmacol. 70, 545–554. doi: 10.1016/j.yrtph.2014.06.010
Kuddus, M. (2018). Enzymes in Food Biotechnology: Production, Applications, and Future Prospects. Hail: Academic Press.
Kumanda, C., Mlambo, V., and Mnisi, C. M. (2019). Valorization of red grape pomace waste using polyethylene glycol and fibrolytic enzymes: physiological and meat quality responses in Broilers. Animals 9:779. doi: 10.3390/ani9100779
Kumar Ashok, P., and Upadhyaya, K. (2012). Tannins are astringent. J. Pharmacogn. Phytochem. 1, 45–50.
Landbo, A. K., and Meyer, A. S. (2001). Enzyme-assisted extraction of antioxidative phenols from black currant juice press residues (Ribes nigrum). J. Agric. Food Chem. 49, 3169–3177. doi: 10.1021/jf001443p
Lima, J. S., d., Cruz, R., Fonseca, J. C., Medeiros, E. V. d., Maciel, M. d. H. C., Moreira, K. A., et al. (2014). Production, characterization of tannase from Penicillium montanense URM 6286 under SSF using agroindustrial wastes, and application in the clarification of grape juice (Vitis vinifera L.). Scientific World. 2014, 1–9. doi: 10.1155/2014/182025
Liu, W.-C., and Kim, I.-H. (2017). Effects of dietary xylanase supplementation on performance and functional digestive parameters in broilers fed wheat-based diets. Poult. Sci. 96, 566–573. doi: 10.3382/ps/pew258
Luo, J., and Xu, Y. (2020). Comparison of biological and chemical pretreatment on coproduction of pectin and fermentable sugars from apple pomace. Appl. Biochem. Biotechnol. 190, 129–137. doi: 10.1007/s12010-019-03088-w
Madeira, J. V., Macedo, J. A., and Macedo, G. A. (2012). A new process for simultaneous production of tannase and phytase by Paecilomyces variotii in solid-state fermentation of orange pomace. Bioprocess Biosyst. Eng. 35, 477–482. doi: 10.1007/s00449-011-0587-y
Madrera, R. R., and Valles, B. S. (2011). Determination of volatile compounds in apple pomace by stir bar sorptive extraction and gas chromatography-mass spectrometry (SBSE-GC-MS). J. Food Sci. 76, C1326-34. doi: 10.1111/j.1750-3841.2011.02406.x
Magyar, M, L., da Costa Sousa, Jin, M., Sarks, C., and Balan, V. (2016). Conversion of apple pomace waste to ethanol at industrial relevant conditions. Appl. Microbiol. Biotechnol. 100, 7349–7358. doi: 10.1007/s00253-016-7665-7
Mahmoodi, M., Najafpour, G., and Mohammadi, M. (2017). Production of pectinases for quality apple juice through fermentation of orange pomace. J. Food Sci. Technol. 54, 4123–4128. doi: 10.1007/s13197-017-2829-8
Makris, D., and Kefalas, P. (2013). Characterization of polyphenolic phytochemicals in red grape pomace. Int. Waste Resour. J. 126. doi: 10.4172/2252-5211.1000126
Manach, C., Williamson, G., Morand, C., Scalbert, A., and Rémésy, C. (2005). Bioavailability and bioefficacy of polyphenols in humans. I. Review of 97 bioavailability studies. Am. J. Clin. Nutr. 81, 230S−242S. doi: 10.1093/ajcn/81.1.230S
Mansoori, B., Modirsanei, M., Radfar, M., Kiaei, M., Farkhoy, M., and Honarzad, J. (2008). Digestibility and metabolisable energy values of dried tomato pomace for laying and meat type cockerels. Anim. Feed Sci. Technol. 141, 384–390. doi: 10.1016/j.anifeedsci.2007.06.029
Marag,ò, E., Iacopini, P., Camangi, F., Scattino, C., Ranieri, A., Stefani, A., et al. (2015). Phenolic profile and antioxidant activity in apple juice and pomace: effects of different storage conditions. Fruits 70, 213–223. doi: 10.1051/fruits/2015015
Martinez-Gonzalez, A. I., Díaz-Sánchez, Á. G., Rosa, L. A., Vargas-Requena, C. L., and Bustos-Jaimes, I. (2017). Polyphenolic compounds and digestive enzymes: in vitro non-covalent interactions. Molecules 22:669. doi: 10.3390/molecules22040669
Meng, X., Slominski, B., Nyachoti, C., Campbell, L., and Guenter, W. (2005). Degradation of cell wall polysaccharides by combinations of carbohydrase enzymes and their effect on nutrient utilization and broiler chicken performance. Poult. Sci. 84, 37–47. doi: 10.1093/ps/84.1.37
Monciardini, P., Iorio, M., Maffioli, S., Sosio, M., and Donadio, S. (2014). Discovering new bioactive molecules from microbial sources. Microbial. Biotechnol. 7, 209–220. doi: 10.1111/1751-7915.12123
Munyaka, P. M., Nandha, N. K., Kiarie, E., Nyachoti, C. M., and Khafipour, E. (2016). Impact of combined beta-glucanase and xylanase enzymes on growth performance, nutrients utilization and gut microbiota in broiler chickens fed corn or wheat-based diets. Poult. Sci. 95, 528–540. doi: 10.3382/ps/pev333
Ndou, S. P., Kiarie, E., Agyekum, A. K., Heo, J. M., Romero, L. F., Arent, S., et al. (2015). Comparative efficacy of xylanases on growth performance and digestibility in growing pigs fed wheat and wheat bran- or corn and corn DDGS-based diets supplemented with phytase. Anim. Feed Sci. Technol. 209, 230–239. doi: 10.1016/j.anifeedsci.2015.08.011
Negoro, H. (1972). Effect of polyphenolic compounds on pectinase action. Eiyo To Shokuryo 25, 1–7. doi: 10.4327/jsnfs1949.25.1
Nian, F., Guo, Y., Ru, Y., Li, F., A.J.A.-A.J.o.Peron, A. S. (2011). Effect of exogenous xylanase supplementation on the performance, net energy and gut microflora of broiler chickens fed wheat-based diets. Asian Australasian J. Animal Sci. 24, 400–406. doi: 10.5713/ajas.2011.10273
Nourmohammadi, R., Khosravinia, H., and Afzali, N. (2018). Effects of feed form and xylanase supplementation on metabolizable energy partitioning in broiler chicken fed wheat-based diets. J. Anim. Physiol. Anim. Nutr. 102, 1593–1600. doi: 10.1111/jpn.12980
Nuengjamnong, C., and Angkanaporn, K. (2018). Efficacy of dietary chitosan on growth performance, haematological parameters and gut function in broilers. Ital. J. Anim. Sci. 17, 428–435. doi: 10.1080/1828051X.2017.1373609
Oliveira, M., Figueiredo-Lima, D., Faria Filho, D., Marques, R., and Moraes, V. M. B.,d. (2009). Effect of mannanoligosaccharides and/or enzymes on antibody titers against infectious bursal and Newcastle disease viruses. Arquivo Brasileiro Med. Vet. Zootecnia 61, 6–11. doi: 10.1590/S0102-09352009000100002
Owusu-Asiedu, A., Kiarie, E., Peron, A., Woyengo, T. A., Simmins, P. H., and Nyachoti, C. M. (2012). Growth performance and nutrient digestibilities in nursery pigs receiving varying doses of xylanase and beta-glucanase blend in pelleted wheat- and barley-based diets. J. Anim. Sci. 90(Suppl. 4), 92–94. doi: 10.2527/jas.51323
Ozdal, T., Sela, D. A., Xiao, J., Boyacioglu, D., Chen, F., and Capanoglu, E. (2016). The reciprocal interactions between polyphenols and gut microbiota and effects on bioaccessibility. Nutrients 8:78. doi: 10.3390/nu8020078
Papadaki, A., Kachrimanidou, V., Papanikolaou, S., Philippoussis, A., and Diamantopoulou, P. (2019). Upgrading grape pomace through Pleurotus spp. cultivation for the production of enzymes and fruiting bodies. Microorganisms 7:207. doi: 10.3390/microorganisms7070207
Papadopoulou, C., Soulti, K., and Roussis, I. G. (2005). Potential antimicrobial activity of red and white wine phenolic extracts against strains of staphylococcus aureus, escherichia coli and Candida albicans. Food Technol. Biotechnol. 43, 41–46.
Parada, J., and Aguilera, J. (2007). Food microstructure affects the bioavailability of several nutrients. J. Food Sci. 72, R21–R32. doi: 10.1111/j.1750-3841.2007.00274.x
Pathania, S., Sharma, N., and Handa, S. (2018). Utilization of horticultural waste (Apple Pomace) for multiple carbohydrase production from Rhizopus delemar F2 under solid state fermentation. J. Genetic Eng. Biotechnol. 16, 181–189. doi: 10.1016/j.jgeb.2017.10.013
Pazos, M., Gallardo, J. M., Torres, J. L., and Medina, I. (2005). Activity of grape polyphenols as inhibitors of the oxidation of fish lipids and frozen fish muscle. Food Chem. 92, 547–557. doi: 10.1016/j.foodchem.2004.07.036
Pekel, A., Horn, N., and Adeola, O. (2016). The efficacy of dietary xylanase and phytase in broiler chickens fed expeller-extracted camelina meal. Poult. Sci. 96, 98–107. doi: 10.3382/ps/pew183
Perussello, C. A., Zhang, Z., Marzocchella, A., and Tiwari, B. K. (2017). Valorization of apple pomace by extraction of valuable compounds. Compr. Rev. Food Sci. Food Saf. 16, 776–796. doi: 10.1111/1541-4337.12290
Pieszka, M., Gogol, P., Pietras, M., and Pieszka, M. (2015). Valuable components of dried pomaces of chokeberry, black currant, strawberry, apple and carrot as a source of natural antioxidants and nutraceuticals in the animal diet. Annals Anim. Sci. 15, 475–491. doi: 10.2478/aoas-2014-0072
Ramos-Pineda, A., Carpenter, G., García-Estévez, I., and Escribano-Bailón, M. (2019). Influence of chemical species on polyphenol–protein interactions related to wine astringency. J. Agric. Food Chem. 68, 2948–2954. doi: 10.1021/acs.jafc.9b00527
Ravindran, V. (2013). Feed enzymes: The science, practice, and metabolic realities. J. Appl. Poultry Res. 22, 628–636. doi: 10.3382/japr.2013-00739
Ravindran, V., and Son, J.-H. (2011). Feed enzyme technology: present status and future developments. Recent Pat. Food Nutr. Agric. 3, 102–109. doi: 10.2174/2212798411103020102
Rechner, A. R., Smith, M. A., Kuhnle, G., Gibson, G. R., Debnam, E. S. S., et al. (2004). Colonic metabolism of dietary polyphenols: influence of structure on microbial fermentation products. Free Radical Biol. Med. 36, 212–225. doi: 10.1016/j.freeradbiomed.2003.09.022
Reddy, P. R. K., Chakravarthi, K., and Ravi, A. (2018). “Chitosan as an alternative to antibiotic feed additive in poultry and pig production,” in 18th Indian Veterinary Congress Compendium (Tirupati), 2–11.
Rho, Y., Kiarie, E., and C. de Lange, F. M. (2018). Nutritive value of corn distiller's dried grains with solubles steeped without or with exogenous feed enzymes for 24 h and fed to growing pigs1. J. Anim. Sci. 96, 2352–2360.
Rigoldi, F., Donini, S., Redaelli, A., Parisini, E., and Gautieri, A. (2018). Engineering of thermostable enzymes for industrial applications. APL Bioeng. 2:011501. doi: 10.1063/1.4997367
Rios, L. Y., M.-,Gonthier, P., Rémésy, C., Mila, I., Lapierre, C., Lazarus, S. A., et al. (2003). Chocolate intake increases urinary excretion of polyphenol-derived phenolic acids in healthy human subjects. Am. J. Clin. Nutr. 77, 912–918. doi: 10.1093/ajcn/77.4.912
Rodríguez-Morgado, B., Candiracci, M., Santa-María, C., Revilla, E., Gordillo, B., Parrado, J., et al. (2015). Obtaining from grape pomace an enzymatic extract with anti-inflammatory properties. Plant Foods Huma. Nutr. 70, 42–49. doi: 10.1007/s11130-014-0459-0
Rodríguez-Muela, C., Rodríguez, H. E., Arzola, C., Díaz-Plascencia, D., Ramírez-Godínez, J. A., Flores-Mariñelarena, A., et al. (2015). Antioxidant activity in plasma and rumen papillae development in lambs fed fermented apple pomace. J. Anim. Sci. 93, 2357–2362. doi: 10.2527/jas.2014-8670
Ross, K. A., Ehret, D., Godfrey, D., Fukumoto, L., and Diarra, M. (2017). Characterization of pilot scale processed canadian organic cranberry (Vaccinium macrocarpon) and blueberry (Vaccinium angustifolium) juice pressing residues and phenolic-enriched extractives. Int. J. Fruit Sci. 17, 202–232. doi: 10.1080/15538362.2017.1285264
Saad, N., Louvet, F., Tarrade, S., Meudec, E., Grenier, K., Landolt, C., et al. (2019). Enzyme-assisted extraction of bioactive compounds from raspberry (Rubus idaeus L.) Pomace. J. Food Sci. 84, 1371–1381. doi: 10.1111/1750-3841.14625
Sanchez, J., Thanabalan, A., Khanal, T., Patterson, R., Slominski, B. A., and Kiarie, E. (2019). Growth performance, gastrointestinal weight, microbial metabolites and apparent retention of components in broiler chickens fed up to 11% rice bran in a corn-soybean meal diet without or with a multi-enzyme supplement. Anim. Nutr. 5, 41–48. doi: 10.1016/j.aninu.2018.12.001
Sato, M. F., Vieira, R. G., Zardo, D. M., Falcão, L. D., Nogueira, A., and Wosiacki, G. (2010). Apple pomace from eleven cultivars: an approach to identify sources of bioactive compounds. Acta Scientiarum. Agronomy 32, 29–35. doi: 10.4025/actasciagron.v32i1.3176
Scalbert, A., and Williamson, G. (2000). Dietary intake and bioavailability of polyphenols. J. Nutr. 130, 2073S−2085S. doi: 10.1093/jn/130.8.2073S
Sharma, A., Gupta, G., Ahmad, T., Mansoor, S., and Kaur, B. (2019). Enzyme engineering: current trends and future perspectives. Food Rev. Int. 37, 121–154. doi: 10.1080/87559129.2019.1695835
Silva Soares, S. C., de Lima, G. C., Carlos Laurentiz, A., Féboli, A., Dos Anjos, L. A., de Paula Carlis, M. S., et al. (2018). In vitro anthelmintic activity of grape pomace extract against gastrointestinal nematodes of naturally infected sheep. Int. J. Vet. Sci. Med. 6, 243–247. doi: 10.1016/j.ijvsm.2018.11.005
Singh, A, H. M., O'neill, Ghosh, T., Bedford, M., and Haldar, S. (2012). Effects of xylanase supplementation on performance, total volatile fatty acids and selected bacterial population in caeca, metabolic indices and peptide YY concentrations in serum of broiler chickens fed energy restricted maize–soybean based diets. Anim. Feed Sci. Technol. 177, 194–203. doi: 10.1016/j.anifeedsci.2012.08.005
Slominski, B. A. (2011). Recent advances in research on enzymes for poultry diets. Poult. Sci. 90, 2013–2023. doi: 10.3382/ps.2011-01372
Squillace, P., Adani, F., and Scaglia, B. (2020). Supercritical CO2 extraction of tomato pomace: Evaluation of the solubility of lycopene in tomato oil as limiting factor of the process performance. Food Chem. 315:126224. doi: 10.1016/j.foodchem.2020.126224
Sudendey, C., and Kamphues, J. (1995). “Effect of enzyme addition (amylase, xylanase and β-glucanase) on digestive processes in the intestinal tract of force fed weaner pigs,” in Proceedings of the Society for Nutrition and Physiology. Frankfurt: DLG-Verl.
Sunna, A., and Antranikian, G. (1997). Xylanolytic enzymes from fungi and bacteria. Crit. Rev. Biotechnol. 17, 39–67. doi: 10.3109/07388559709146606
Tan, Y. N., Lee, P. P., and Chen, W. N. (2020). Microbial extraction of chitin from seafood waste using sugars derived from fruit waste-stream. AMB Express 10:17. doi: 10.1186/s13568-020-0954-7
Teles, A. S., Chávez, D. W., Oliveira, R. A., Bon, E. P., Terzi, S. C., Souza, E. F., et al. (2019). Use of grape pomace for the production of hydrolytic enzymes by solid-state fermentation and recovery of its bioactive compounds. Food Res. Int. 120, 441–448. doi: 10.1016/j.foodres.2018.10.083
Tomschy, A., Brugger, R., Lehmann, M., Svendsen, A., Vogel, K., Kostrewa, D., et al. (2002). Engineering of phytase for improved activity at low pH. Appl. Environ. Microbiol. 68, 1907–1913. doi: 10.1128/AEM.68.4.1907-1913.2002
Troche, C., Sun, X., McElroy, A., Remus, J., and Novak, C. (2007). Supplementation of Avizyme 1502 to corn-soybean meal-wheat diets fed to turkey tom poults: the first fifty-six days of age. Poult. Sci. 86, 496–502 doi: 10.1093/ps/86.3.496
Troncozo, M. I., Ljesevic, M., Beskoski, V. P., Andelkovic, B., Balatti, P. A., and Saparrat, M. C. N. (2019). Fungal transformation and reduction of phytotoxicity of grape pomace waste. Chemosphere 237:124458. doi: 10.1016/j.chemosphere.2019.124458
Vagiri, M., and Jensen, M. (2017). Influence of juice processing factors on quality of black chokeberry pomace as a future resource for colour extraction. Food Chem. 217, 409–417. doi: 10.1016/j.foodchem.2016.08.121
Van Dorn, R., Shanahan, D., and Ciofalo, V. (2018). Safety evaluation of xylanase 50316 enzyme preparation (also known as VR007), expressed in Pseudomonas fluorescens, intended for use in animal feed. Regulatory Toxicol. Pharmacol. 97, 48–56. doi: 10.1016/j.yrtph.2018.05.016
Vrhovsek, U., Rigo, A., Tonon, D., and Mattivi, F. (2004). Quantitation of polyphenols in different apple varieties. J. Agric. Food Chem. 52, 6532–6538. doi: 10.1021/jf049317z
Waldbauer, K., McKinnon, R., and Kopp, B. (2017). Apple pomace as potential source of natural active compounds. Planta Med. 83, 994–1010. doi: 10.1055/s-0043-111898
Walsh, M. C., Kiarie, E., Romero, L., and Arent, S. (2016). Xylanase solubilization of corn and wheat arabinoxylans in mixed growing pig diets subjected to upper gut in-vitro digestion and in ileal digesta. J. Anim. Sci. 94, 111–111. doi: 10.2527/msasas2016-235
Wang, J., Liu, K., Xing, R., and X. J. C., Yan, S. R. (2016). Peptide self-assembly: thermodynamics and kinetics. Chem. Soc. Rev.. 45, 5589–5604. doi: 10.1039/C6CS00176A
West, M., Corzo, A., Dozier Iii, W., Blair, M., and Kidd, M. (2007). Assessment of dietary Rovabio Excel in practical United States broiler diets. J. Applied Poultry Res. 16, 313–321. doi: 10.1093/japr/16.3.313
Wikiera, A., Mika, M., Starzynska-Janiszewska, A., and Stodolak, B. (2016). Endo-xylanase and endo-cellulase-assisted extraction of pectin from apple pomace. Carbohydr. Polym. 142, 199–205. doi: 10.1016/j.carbpol.2016.01.063
Williamson, G., and Clifford, M. N. (2010). Colonic metabolites of berry polyphenols: the missing link to biological activity? Br. J. Nutr. 104, S48–S66. doi: 10.1017/S0007114510003946
Wong, K. K., Tan, L. U., and Saddler, J. N. (1988). Multiplicity of beta-1,4-xylanase in microorganisms: functions and applications. Microbiol. Rev. 52, 305–317. doi: 10.1128/MR.52.3.305-317.1988
Wu, X, H. E., Pittman III, Hager, T., Hager, A., Howard, L., and Prior, R. L. (2009). Phenolic acids in black raspberry and in the gastrointestinal tract of pigs following ingestion of black raspberry. Molecular nutrition & food research 53, S76-S84. doi: 10.1002/mnfr.200800231
Xiang, T., Liu, Q., Deacon, A. M., Koshy, M., Kriksunov, I. A., Lei, X. G., et al. (2004). Crystal structure of a heat-resilient phytase from Aspergillus fumigatus, carrying a phosphorylated histidine. J. Mol. Biol. 339, 437–445. doi: 10.1016/j.jmb.2004.03.057
Yildirim-Elikoglu, S., and Vural, H. (2019). Binding characteristics of polyphenols as milk plasmin inhibitors. J. Sci. Food Agric. 99, 6922–6930. doi: 10.1002/jsfa.9978
Yu, B., Wu, S., Liu, C., Gauthier, R., and Chiou, P. W. (2007). Effects of enzyme inclusion in a maize–soybean diet on broiler performance. Anim. Feed Sci. Technol. 134, 283–294. doi: 10.1016/j.anifeedsci.2006.09.017
Zhang, Y., Tie, X., Bao, B., Wu, X., and Zhang, Y. (2007). Metabolism of flavone C-glucosides and p-coumaric acid from antioxidant of bamboo leaves (AOB) in rats. Br. J. Nutr. 97, 484–494. doi: 10.1017/S0007114507336830
Zhang, S., Wang, C., Sun, Y., Wang, G., Chen, H., Li, D., et al. (2018). Xylanase and fermented polysaccharide of hericium caputmedusae reduce pathogenic infection of broilers by improving antioxidant and anti-inflammatory properties. Oxidative Med. Cell. Longevity. 2018, 1–11. doi: 10.1155/2018/4296985
Keywords: fruit pomaces, exogenous enzymes, fermentation, digestion, microbiota
Citation: Kithama M, Hassan YI, Guo K, Kiarie E and Diarra MS (2021) The Enzymatic Digestion of Pomaces From Some Fruits for Value-Added Feed Applications in Animal Production. Front. Sustain. Food Syst. 5:611259. doi: 10.3389/fsufs.2021.611259
Received: 28 September 2020; Accepted: 08 April 2021;
Published: 05 May 2021.
Edited by:
Tripti Agarwal, National Institute of Food Technology Entrepreneurship and Management, IndiaReviewed by:
Acacio Zielinski, Federal University of Santa Catarina, BrazilMonika Kosmala, Lodz University of Technology, Poland
Copyright © 2021 Kithama, Hassan, Guo, Kiarie and Diarra. This is an open-access article distributed under the terms of the Creative Commons Attribution License (CC BY). The use, distribution or reproduction in other forums is permitted, provided the original author(s) and the copyright owner(s) are credited and that the original publication in this journal is cited, in accordance with accepted academic practice. No use, distribution or reproduction is permitted which does not comply with these terms.
*Correspondence: Moussa S. Diarra, bW91c3NhLmRpYXJyYSYjeDAwMDQwO2NhbmFkYS5jYQ==