- Graduate School of Horticulture, Chiba University, Chiba, Japan
Two types of commercial salted Japanese apricot (ume) pickle products with different textures were studied and their physicochemical and biochemical properties were compared. Considering the effects of fruit raw material ripeness and the pickle processing method, a pickled unripe-hard texture fruit (9% salinity) called “Karikari-ume” and a pickled ripe-soft texture ume fruit (10% salinity) called “Umeboshi” were used as sample materials. The results showed that the pH and moisture content of Karikari-ume (3.18 and 81.99%, respectively) were higher than that of umeboshi (2.84 and 74.08%, respectively). Meanwhile, the TSS and TA of citric acid and the TA of lactic acid value of the Karikari-ume (4.45, 0.92, and 1.30%, respectively) were lower than the Umeboshi (7.17, 1.79, and 2.52%, respectively). Karikari-ume also showed higher bioactive compounds and antioxidant activities assessed by DPPH•, ABTS•+, FRAP, and MIC assays (17.48–130.58 unit per gram of sample dry weight). These results suggested that the ripeness of the fruit material used in pickle processing could influence the physicochemical and biochemical properties of salted Japanese apricot pickles.
Introduction
The Japanese apricot (Prunus mume) known as “ume” in Japan is rich in organic acids, edible fiber, minerals, and phenolic compounds. Owing to the high citric acid content of the mature fruit (up to 6–7% of fresh weight) in edible parts, the fresh fruit is extremely sour (Mitani et al., 2018). Ume fruit also contains very high contents of organic acids and phenolic compounds, mainly hydroxycinnamic acid derivatives (Mitani et al., 2013). Meanwhile, the immature green fruit contains cyanogenetic glycosides such as amygdalin (Go et al., 2018). Thus, ume fruit is not commonly consumed as fresh produce due to its sourness and toxicity; most of the fruit requires processing.
Traditionally, ume fruit is simply pickled with salt and processed by drying, because it can be used for medicinal purposes (Chung et al., 2013), e.g., as a hangover remedy, for liver rejuvenation, for detoxification, to treat nausea, and as an appetite stimulant. Besides consumption as traditional ume pickles, processed ume fruits are widely consumed in Asian and Southeast Asian countries as a sauce, juice, food garnish or decoration, and liquor (Chen et al., 2017). Owing to their high content of catalytic enzymes, probiotics, and bioactive compounds such as flavanols, ume could also provide antioxidative and antibacterial effects, as well as anti-inflammatory, hepatoprotective, and anticancer properties. Regarding the health aspect, the consumption of foods rich in flavanols was proven to decrease the risk of certain chronic diseases (Bailly, 2020; Gossard and Lipski, 2020). Salted ume pickles can also prevent the growth of some microbes (Mouritsen, 2018; Tylewicz et al., 2020).
There are two types of ume pickles: (1) pickled unripe ume fruit, commercially called “Karikari-ume,” and (2) pickled ripe ume fruit, normally called “Umeboshi.” These products show a different texture related to ripeness of the initial raw fruit material; Karikari-ume has a hard and crispy mouthfeel from pickling at the unripe green stage, while Umeboshi has a soft and slight mushy texture. Both salted pickles are often marinated with aromatic red perilla leaves (Shiso) as a natural coloring (red-purple color). As a pickle processing method for longer preservation, a salt-based osmotic agent is used to reduce the free water in raw materials. This type of osmotic agent normally adsorbs free water and becomes a hypertonic solution, which generates a water transfer process by which free water in water-containing tissue is transferred to a more concentrated hypertonic solution. Meanwhile, the osmotic agent also diffuses into the material. Thus, a salt osmotic treatment influences the salt and moisture content of the fruit and can also affect its water activity (Osman and Faruk, 2016). For more acidic fruits like ume, osmotic salt treatment can affect the pH of processed material that includes an exuded salty solution, which could change the physicochemical and biochemical properties of processed pickle products. The rate of osmosis could also influence the acidity of the processed material (Ramya and Jain, 2017).
This study aimed to investigate and assess the physicochemical and biochemical properties of two different types of commercialized salted Japanese apricot pickles with different textures. Firmness, acidity, bioactive compound contents, and antioxidant activities were also investigated and compared between fruit types.
Materials and Methods
Chemicals and Reagents
All chemicals and reagents used were of analytical grade or above. Chemicals used in the determination of antioxidant activity, such as 1,1-diphenyl-2-picrylhydrazyl (DPPH•), 2,4,6-tri(2-pyridyl)-s-triazine (TPTZ®), 3-(2-pyridyl)-5,6-diphenyl-1,2,4-triazine-p, p′-disulfonic acid monosodium salt hydrate (FerroZine®),
FeCl3, gallic acid monohydrate, and (+)-catechin hydrate, were obtained from Sigma-Aldrich Ltd. (St. Louis, MO, USA). Folin-Ciocalteu phenol reagent, Trolox® (6-hydroxy-2,5,7,8-tetramethylchroman-2-carboxylic acid), 2,2′-Azino-bis (3-ethylbenzothiazoline-6-sulfonic acid) diammonium salt (ABTS•+), FeCl2, and FeSO4 were purchased from Wako Pure Chemical Co. (Tokyo, Japan), and ethylenediaminetetraacetic acid disodium salt (EDTA) was acquired from Dojin Chemical Laboratory Co., Ltd. (Tokyo, Japan).
Pickle Samples and Physicochemical Property Determination
A commercial Karikari-ume (hard texture) and Umeboshi (soft texture) were purchased from a supermarket in Matsudo, Chiba, Japan. Figure 1 displays the appearance of two types of ume pickles. The moisture content (MC) of the pickle samples was measured using a hot air oven (WFD-400; Eyela, Tokyo, Japan) at 100 ± 5°C for 16 h according to AOAC method number 930.15 (Horwitz and Latimer, 2005). pH, total soluble solids (TSS), and titratable acidity (TA) were determined using a pH meter (AS800; As One, Osaka, Japan), a digital hand-held pocket refractometer (PAL-1; Atago, Tokyo, Japan), and the titration method according to Islam et al. (2013). The salinity (%) was measured using a salt concentration refractometer (RAS-28, As One, Tokyo, Japan). The TA was also determined in the same juice by titration: 15 g of juice was added to a beaker, and the pH values were measured from the start until the endpoint (pH 9.0) of titration. The volume of NaOH solution required for titration was recorded and the percent titratable acidity (expressed as % acid equivalent) was calculated from the molecular weight of citric acid and lactic acid of 192.1 and 90.08 g/mol, respectively, with the following formula:
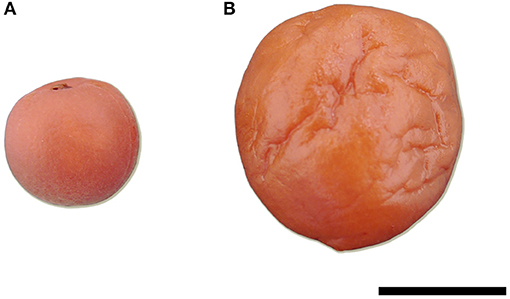
Figure 1. Photograph of Karikari-ume, unripe ume fruit salted pickle which has a hard texture (A) and Umeboshi, ripe ume fruit salted pickle which has a soft texture (B). Scale bar = 1 cm.
All above determinations were performed in triplicate. Pickle firmness was determined using a Creep Meter (RE2-3305S, Yamaden, Tokyo, Japan), and the conical probe was equipped with a 20 N load cell and a 2.00-mm-diameter probe for the karikari-ume (hard texture) and a 3.00-mm-diameter probe for the umeboshi (soft texture). Pickle seeds were removed before testing, and 10 replications were performed for each sample. The forces and cross-sectional area of loaded probes were recorded for the computation and converted to stress (N m−2) (Moore and Booth, 2015).
Biochemical Property Determinations
The prepared pickles were extracted and quantified by the procedure described by Ketnawa et al. (2020). The determinations of all spectrometric methods were measured by a Thermo Scientific Multiskan® FC microplate photometer (Thermo Fisher Scientific, MA, USA), and the assays were completed in 96-well microplates with slight modifications as described by Reginio et al. (2020). The extracted samples were stored at −20°C until further determinations.
Total Phenolic Content (TPC)
Total phenolic content (TPC) was determined using the Folin-Ciocalteu reagent test method based on the assay of Singleton et al. (1999) with slight mofidifications according to Reginio et al. (2020). The extracted sample (25 μL) or gallic acid standard (0–120 mg/L) was mixed with 125 μL of 10% (v/v) Folin-Ciocalteu solution and 100 μL of 7.5% (w/v) sodium carbonate solution. The mixture was incubated for 1 h at room temperature in the dark, and the absorbance was then measured at 740 nm; distilled water was used as a blank. TPC was reported as milligrams (mg) of gallic acid equivalents (GAE) per gram of sample dry weight (DW).
Total Flavonoid Content (TFC)
The total flavonoid content (TFC) was determined using an aluminum chloride colorimetric assay according to Zhishen et al. (1999) with slight modifications for 96-well microplate assays. A 33 μL of extracted sample or catechin standard solution (0–100 mg/L) was added to 113 μL distilled water, and 10 μL of 5% NaNO2 was mixed in. After 5 min, 10 μL of 10% AlCl3 was added. At 6 min, 67 μL of 1 M NaOH was added to the mixture. Immediately, 80 μL of distilled water was added to the reaction mixture and mixed well. The absorbance of the mixture was determined at 520 nm. The TFC was expressed as milligrams (mg) of catechin equivalents (CE) per gram of sample DW.
DPPH• Scavenging Activity (DPPH)
The DPPH• radical scavenging activity of the sample was investigated by a later modification of the assay of Brand-Williams et al. (1995) with slight modifications by Ketnawa et al. (2020). Briefly, 5 μL of extracted sample was added to 195 μL of 60 μM DPPH•. The mixture was vigorously shaken and allowed to stand in the dark for 30 min at 23°C. The absorbance of the resulting solution was measured at 517 nm. The DPPH radical scavenging activity was calculated by comparison with a Trolox standard curve (0–1,000 μmol/L) and expressed as micromoles (μmol) of Trolox equivalents (TE) per gram of sample DW.
ABTS•+ Scavenging Activity (ABTS)
ABTS•+ scavenging activity was evaluated according to Ketnawa and Ogawa (2019), with slight modifications. ABTS solution was prepared by dissolving 7 mM ABTS•+ in 2.45 mM potassium persulfate, allowing the mixture to react in the dark for 12 h at room temperature before analysis. The ABTS solution was diluted with distilled water to obtain an absorbance of 0.7 ± 0.03 at 740 nm. At the beginning of the reaction, 10 μL of the extracted sample was mixed with 320 μL of diluted ABTS solution. The absorbance was measured at 740 nm after incubation for 10 min at 30°C in the dark. An ascorbic acid standard (0–100 μmol/L) was prepared in the same manner as a reference standard, and ABTS•+ radical scavenging activity was determined in millimoles (mmol) of ascorbic acid equivalents (AsA) per gram of sample DW.
Ferric-Reducing Antioxidant Power (FRAP)
The ferric-reducing antioxidant power (FRAP) was measured by the FRAP assay of Benzie and Szeto (1999) with slight modifications by Reginio et al. (2020). FRAP reagent (300 mM acetate buffer [pH 3.6], 10 mM TPTZ® solution in 40 mM-HCl, and 20 mM FeCl3·6H2O solution in a ratio of 10 :1 :1) was prepared fresh and 130 μL mixed with 20 μL of the extracted sample. The mixture was incubated at the same temperature in the dark for 30 min. The absorbance at 595 nm was determined after incubation with distilled water instead of the sample as a blank. FRAP was calculated from the standard curve of FeSO4 (0–500 μmol/L) and expressed as millimoles (mmol) of FeSO4 equivalents per gram of sample DW.
Metal Ion Chelating (MIC) Activity
The chelating activity on Fe2+ was identified regarding Ketnawa and Ogawa (2019) with some modifications. The diluted sample (300 μL) was mixed with 2 mM FeSO4 (5 μL) and 5 mM FerroZine® (5 μL). The mixture was vigorously mixed and allowed to stand for 10 min at room temperature. The absorbance was then measured at 560 nm with distilled water as a blank. EDTA standard (0–10 mmol/L) was prepared and used as a standard curve. Ferrous chelating activity was expressed as millimoles (mmol) of EDTA equivalents per gram of sample DW.
Statistical Analysis
Triplicate determinations were analyzed throughout the experimental period using IBM SPSS Statistics for Windows, Version 26.0 (Armonk, NY: IBM Corp). Data and mean comparisons were performed using a t-test. Differences were considered statistically significant at p < 0.05.
Results and Discussion
Physicochemical Properties
The physicochemical properties of the two pickles used are shown in Table 1. The pH of Umeboshi (10.0% salinity) was lower than that of Karikari-ume (9.0% salinity), which could be related to the lower MC of Umeboshi. According to a previous study by Odake et al. (1999), MC of fresh fruit (~90%) decreased to ~77% due to the salted processing. Thus, it was considered that Karikari-ume would be a less salted condition than the umeboshi. The TSS and TA of citric acid and the TA of lactic acid for Karikari-ume were 4.45, 0.92 ± 0.01, and 1.30 ± 0.02%, respectively, whilst Umeboshi showed higher percentages of 7.17, 1.79 ± 0.03, and 2.52 ± 0.04%, respectively. These results relate to the lower in pH value of Umeboshi relative to Karikari-ume. A previous report by Wu et al. (2005) showed that malic acid, citric acid, quinic acid, and shikimic acid are major acids in Prunus fruits. Besides, Yu et al. (2015) also reported that the dominant organic acid in ume fruits was citric acid, which reaches 39.3 g/kg. Odake et al. (1999) reported that 9.87% NaCl of Umeboshi pickle had TA and moisture contents of ~3.8 and 77%, respectively. Karikari-ume produced significantly higher pickle firmness than Umeboshi. According to the processing method, Umeboshi has longer pickling time using the ripe ume fruit, meanwhile, Karikari-ume made from unripe small green ume fruit was pickled by a different processing method depending on the fruit conditions. The differences in physicochemical parameters between them could depend not only on the original fresh product properties but also the processing conditions.
Bioactive Compounds
Bioactive compounds for both types of salted pickles were determined and reported as total phenolic content (TPC) and total flavonoid content (TFC) (Figure 2). TPC values for Karikari-ume and Umeboshi were 1.99 and 2.10 mg GAE/g DW, respectively, with no significant differences (p > 0.05), whereas TFC was considerably higher in Umeboshi than in Karikari-ume. This may be due to the difference in the initial level of TFC in the fresh raw material and could be attributed to the different biochemical, physiological, and structural reactions and modifications that occur during the fruit maturation process. Previous research found that a fresh Japanese apricot fruit contained high values of phenolic acids such as a 3-O-caffeoylquinic acid, 5-O-caffeoylqunic acid, chlorogenic acid, and tetra-O-acylated sucrose-related compounds (Mitani et al., 2013). The range of contents was from 150 to 320 μmol per gram trolox equivalents, which depended on the stage of fruit ripeness (Mitani et al., 2013). The observation of the decrement of phenolic contents and antioxidant activities as ripening progressed was reported in previous studies (Macheix et al., 1990; Reginio et al., 2020), thus affecting the contents of polyphenols and their antioxidant activities of ume pickles in the study.
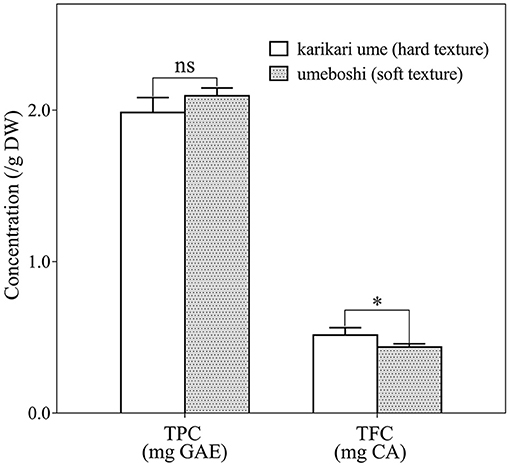
Figure 2. Total bioactive compounds on total phenolic content (TPC) and total flavonoid content (TFC) in Karikari-ume (hard texture) and Umeboshi (soft texture) by microtiter plate assay, no statistical significance (ns), *p < 0.05 (n = 3).
It was reported that a Japanese traditional pseudo-medicinal product named Bainiku Ekisu, a paste extracted and concentrated from Japanese apricot fruit, could inhibit bacterial growth (E. faecalis, S. aureus, and E. coli) in concentrations between 1.0 and 10.0 mg/mL (Yang et al., 2014). Additionally, Mitani et al. (2018) reported that Umezu, a salty extracted juice by-product of umeboshi processing, contained high amounts phenolic compounds, further clarified by microbial growth inhibitory activity research. Umezu was reported to have antimicrobial activity against enterobacteria due to the action of phenolic compounds (Mitani et al., 2018). Phenolic compounds modify the permeability of bacterial cell membranes and cause changes in various intracellular functions by hydrogen bonding to enzymes or modification of cell wall rigidity with integrity losses due to different interactions with the cell membrane. This may induce irreversible damage of the cytoplasmic membrane and coagulation of the cell contents that can even lead to the inhibition of intracellular enzymes of microorganisms (Cushnie and Lamb, 2011). Although it could not be simply compared with the current results, Karikari-ume might have more functional potential than Umeboshi, which is indicated by the significant differences in flavonoid content. The antimicrobial properties of pickles prepared under standard laboratory conditions in comparison with commercial ones, therefore, need to be investigated in the future.
Antioxidant Activities
Antioxidant activities were evaluated by means of DPPH, ABTS, FRAP, and MIC assays, and the results are depicted in Figure 3. Even though the difference in TPC between Umeboshi and Karikari-ume was non-significant (Figure 2), the antioxidant activity did not follow the same trend. All the antioxidant activity measurements on Karikari-ume were significantly greater than those of Umeboshi (p < 0.05, Figure 3). Karikari-ume had the highest ABTS and free-radical-scavenging ability: DPPH and ABTS ranged from 17.48 to 130.58 units per gram of sample DW. In addition, the FRAP and MIC values of Karikari-ume were 37.29 mmol FeSO4 and 23.24 mmol EDTA equivalents per gram of sample DW, respectively, while the FRAP and MIC of Umeboshi were 6- to 8-fold lower. Debnath et al. (2012) reported that mume fructus extract inhibited scavenging activity against free radicals including DPPH•, ABTS•+, hydroxyl, and superoxide with half maximal inhibitory concentrations (IC50) of 0.40, 0.36, 1.75, and 1.60 mg/mL, respectively. We assumed that the types of phenolics, which depended on the raw materials, maturity, and pickle processing method, could influence the antioxidant activity. For instance, the number of hydroxyl groups in phenolic molecules have an effect on antioxidant activities (Shahidi and Ambigaipalan, 2015). The lactic acid fermentation process for plant-based foods improved antioxidative activity due to microbial hydrolytic breakdown of plant cell walls and developed phenolic compounds (Hur et al., 2014). However, the hard cell wall of unripe ume fruit material may help to preserve bioactive compounds in their active form, whereas soft-type fruits may be prone to loss of activity to extracted juice during pickle manufacturing. Thus, further investigations of optimization of the ume pickle processing, characterization of phenolic acids, and flavonoid profiles are needed in the future.
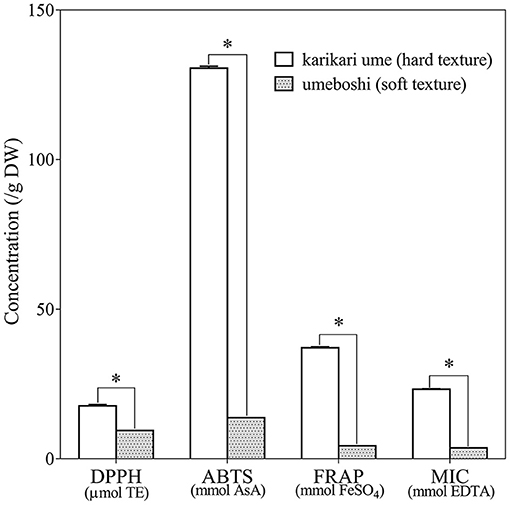
Figure 3. Antioxidant activities assessed by DPPH, ABTS, FRAP, and MIC in Karikari-ume (hard texture) and Umeboshi (soft texture) by microtiter plate assay, *p < 0.05 (n = 3).
Conclusion
In this study, differences were observed in the physicochemical properties between two types of ume pickles. The bioactive compounds present in ume pickles depended on the ripeness of the raw material and acidification processing, thus affecting the antioxidant properties. Karikari-ume- presented higher concentrations of total flavonoids and antioxidant activities. Considering that the raw material conditions and manufacturing process contributed to differential physiochemical properties, especially the lower moisture content and pH, these factors may affect water activity and antimicrobial agents in ume fruit. This was also related to acidification processes, and further research is needed on pickle manufacturing process optimization, the relationship between phenolic profiles and antioxidant activities, as well as other bioactivities. These data could benefit further research and the industrial sector by optimizing the pickling process, providing the highest amount of bioactive compounds with potential bioactivities. Moreover, they could help researchers to discover and develop novel healthy products and further study the release of bioactive compounds though digestion and antimicrobial properties.
Data Availability Statement
The raw data supporting the conclusions of this article will be made available by the authors, without undue reservation.
Author Contributions
JS designed the experiment, performed the experiments, analyzed the data and prepared the figures, and wrote the manuscript. SK performed, verified the analytical assay, and reviewed the manuscript. YO supervised and critically reviewed the manuscript. All authors contributed to the article and approved the submitted version.
Conflict of Interest
The authors declare that the research was conducted in the absence of any commercial or financial relationships that could be construed as a potential conflict of interest.
Acknowledgments
The authors are grateful to the Mitsubishi Corporation for providing the scholarship, International Scholarship (JEES Sponsor-Crowned Scholarship) to the first author.
References
Bailly, C. (2020). Anticancer properties of Prunus mume extracts (Chinese plum, Japanese apricot). J. Ethnopharmacol. 246:112215. doi: 10.1016/j.jep.2019.112215
Benzie, I. F. F., and Szeto, Y. T. (1999). Total antioxidant capacity of teas by the ferric reducing/antioxidant power assay. J. Agric. Food Chem. 47, 633–636. doi: 10.1021/jf9807768
Brand-Williams, W., Cuvelier, M. E., and Berset, C. (1995). Use of a free radical method to evaluate antioxidant activity. LWT Food Sci. Technol. 28, 25–30. doi: 10.1016/S0023-6438(95)80008-5
Chen, Z.-Y., Lin, Y.-S., Liu, X.-M., Cheng, J.-R., and Yang, C.-Y. (2017). Chemical composition and antioxidant activities of five samples of Prunus mume umezu from different factories in south and east China. J. Food Qual. 2017:4878926. doi: 10.1155/2017/4878926
Chung, H.-S., Kim, D.-S., Kim, H.-S., Lee, Y.-G., and Seong, J.-H. (2013). Effect of freezing pretreatment on the quality of juice extracted from Prunus mume fruit by osmosis with sucrose. LWT Food Sci. Technol. 54, 30–34. doi: 10.1016/j.lwt.2013.05.016
Cushnie, T. T., and Lamb, A. J. (2011). Recent advances in understanding the antibacterial properties of flavonoids. Int. J. Antimicrob. Agents 38, 99–107. doi: 10.1016/j.ijantimicag.2011.02.014
Debnath, T., Bak, J. P., Samad, N. B., Jin, H. L., Lee, B. R., and Lim, B. O. (2012). Antioxidant activity of mume fructus extract. J. Food Biochem. 36, 224–232. doi: 10.1111/j.1745-4514.2010.00529.x
Go, M. R., Kim, H. J., Yu, J., and Choi, S. J. (2018). Toxicity and toxicokinetics of amygdalin in Maesil (Prunus mume) syrup: protective effect of Maesil against amygdalin toxicity. J. Agric. Food Chem. 66, 11432–11440. doi: 10.1021/acs.jafc.8b03686
Gossard, C. M., and Lipski, E. (2020). “Superfood for digestive health,” in Integrative Gastroenterology, eds G. E. Mullin, M. Singh, and A. Parian (Oxford: Oxford University Press), 141–173.
Horwitz, W., and Latimer, G. W. (2005). Official Methods of Analysis of AOAC International. Gaithersburg, MD: AOAC International.
Hur, S. J., Lee, S. Y., Kim, Y.-C., Choi, I., and Kim, G.-B. (2014). Effect of fermentation on the antioxidant activity in plant-based foods. Food Chem. 160, 346–356. doi: 10.1016/j.foodchem.2014.03.112
Islam, M. K., Khan, M. Z. H., Sarkar, M. A. R., Absar, N., and Sarkar, S. K. (2013). Changes in acidity, TSS, and sugar content at different storage periods of the postharvest mango (Mangifera indica L.) influenced by Bavistin DF. Int. J. Food Sci. 2013:939385. doi: 10.1155/2013/939385
Ketnawa, S., and Ogawa, Y. (2019). Evaluation of protein digestibility of fermented soybeans and changes in biochemical characteristics of digested fractions. J. Funct. Foods 52, 640–647. doi: 10.1016/j.jff.2018.11.046
Ketnawa, S., Suwannachot, J., and Ogawa, Y. (2020). In vitro gastrointestinal digestion of crisphead lettuce: changes in bioactive compounds and antioxidant potential. Food Chem. 311:125885. doi: 10.1016/j.foodchem.2019.125885
Macheix, J.-J., Fleuriet, A., and Billot, J. (1990). Fruit Phenolics. Boca Raton, FL: CRC Press Inc, 1–39.
Mitani, T., Horinishi, A., Kishida, K., Kawabata, T., Yano, F., Mimura, H., et al. (2013). Phenolics profile of mume, Japanese apricot (Prunus mume Sieb. et Zucc.) fruit. Biosci. Biotechnol. Biochem. 77, 1623–1627. doi: 10.1271/bbb.130077
Mitani, T., Ota, K., Inaba, N., Kishida, K., and Koyama, H. A. (2018). Antimicrobial activity of the phenolic compounds of Prunus mume against enterobacteria. Biol. Pharm. Bull. 41, 208–212. doi: 10.1248/bpb.b17-00711
Moore, P., and Booth, G. (2015). “2 - structures under load,” in The Welding Engineers Guide to Fracture and Fatigue, eds P. Moore and G. Booth (Oxford: Woodhead Publishing), 11–21. doi: 10.1533/9781782423911.1.11
Mouritsen, O. G. (2018). Tsukemono-crunchy pickled foods from Japan: a case study of food design by gastrophysics and nature. Int. J. Food Design 3:103. doi: 10.1386/ijfd.3.2.103_1
Odake, S., Otoguro, C., and Kaneko, K. (1999). The effects of fruit maturation and sodium chloride concentration on the compositions of pickled sun-dried ume fruit, ‘umeboshi'. Food Sci. Technol. Res. 5, 113–118. doi: 10.3136/fstr.5.113
Osman, E. T., and Faruk, B. (2016). “Food preservation by reducing water activity,” in Food Microbiology: Principles into Practice (New York, NY: Wiley), 44–58. doi: 10.1002/9781119237860.ch30
Ramya, V., and Jain, N. K. (2017). A review on osmotic dehydration of fruits and vegetables: an integrated approach. J. Food Process. 40:e12440. doi: 10.1111/jfpe.12440
Reginio, F. C., Qin, W., Ketnawa, S., and Ogawa, Y. (2020). Bio-properties of Saba banana (Musa ‘saba', ABB Group): influence of maturity and changes during simulated in vitro gastrointestinal digestion. Sci. Rep. 10:6701. doi: 10.1038/s41598-020-63501-x
Shahidi, F., and Ambigaipalan, P. (2015). Phenolics and polyphenolics in foods, beverages and spices: antioxidant activity and health effects – a review. J. Funct. Foods 18, 820–897. doi: 10.1016/j.jff.2015.06.018
Singleton, V. L., Orthofer, R., and Lamuela-Raventós, R. M. (1999). Analysis of total phenols and other oxidation substrates and antioxidants by means of folin-ciocalteu reagent. Meth. Enzymol. 299, 152–178. doi: 10.1016/S0076-6879(99)99017-1
Tylewicz, U., Oliveira, G., Alminger, M., Nohynek, L., Dalla Rosa, M., and Romani, S. (2020). Antioxidant and antimicrobial properties of organic fruits subjected to PEF-assisted osmotic dehydration. Innovative Food Sci. Emerging Technol. 62:102341. doi: 10.1016/j.ifset.2020.102341
Wu, B. H., Quilot, B., Génard, M., Kervella, J., and Li, S. H. (2005). Changes in sugar and organic acid concentrations during fruit maturation in peaches, P. davidiana and hybrids as analyzed by principal component analysis. Sci. Hortic. 103, 429–439. doi: 10.1016/j.scienta.2004.08.003
Yang, D.-J., Chen, H.-Y., and Liu, S.-C. (2014). Study of the antibacterial efficacy of bainiku-ekisu against pathogens. Int. J. Bacteriol. 2014:460395. doi: 10.1155/2014/460395
Yu, Y., Xiao, G., Xu, Y., Wu, J., Zhang, Y., and Chen, W. (2015). Changes of quality in the fruits of Prunus mume during deacidification by fermentation with Lactobacillus fermentium. J. Food Sci. 80:M405-410. doi: 10.1111/1750-3841.12769
Keywords: Japanese apricot, salted pickle, firmness, acidity, bioactive compounds, antioxidant activity
Citation: Suwannachot J, Ketnawa S and Ogawa Y (2021) Comparative Study of the Physico- and Biochemical Properties of Two Types of Salted Japanese Apricot (Prunus mume) Pickles. Front. Sustain. Food Syst. 5:606688. doi: 10.3389/fsufs.2021.606688
Received: 15 September 2020; Accepted: 15 January 2021;
Published: 09 February 2021.
Edited by:
Md Shafiur Rahman, Sultan Qaboos University, OmanReviewed by:
Touseef Wani, University of Kashmir, IndiaSuelen Avila, Federal University of Paraná, Brazil
Copyright © 2021 Suwannachot, Ketnawa and Ogawa. This is an open-access article distributed under the terms of the Creative Commons Attribution License (CC BY). The use, distribution or reproduction in other forums is permitted, provided the original author(s) and the copyright owner(s) are credited and that the original publication in this journal is cited, in accordance with accepted academic practice. No use, distribution or reproduction is permitted which does not comply with these terms.
*Correspondence: Yukiharu Ogawa, ogwy@faculty.chiba-u.jp