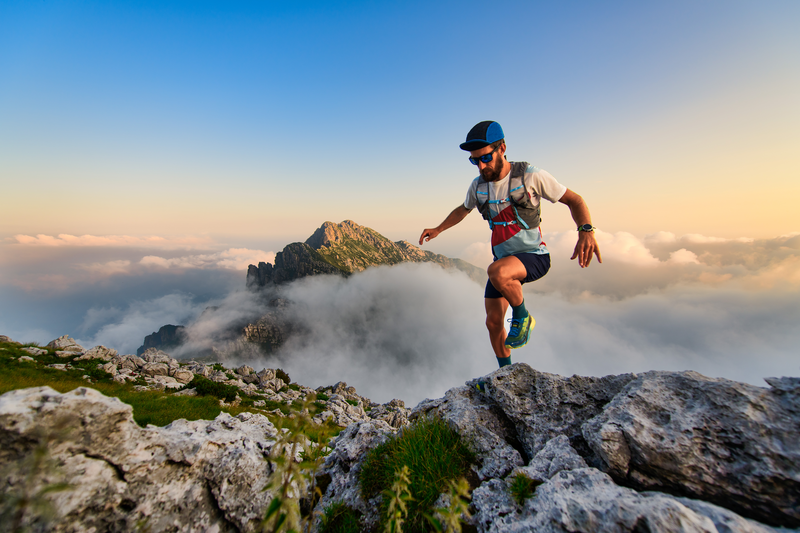
94% of researchers rate our articles as excellent or good
Learn more about the work of our research integrity team to safeguard the quality of each article we publish.
Find out more
SYSTEMATIC REVIEW article
Front. Sustain. Food Syst. , 10 June 2021
Sec. Agroecology and Ecosystem Services
Volume 5 - 2021 | https://doi.org/10.3389/fsufs.2021.606631
This article is part of the Research Topic Can the Trees Save the Crops? Predicting the Services Provided by Traditional and Novel Agroforests in Changing Mediterranean Landscapes View all 6 articles
Agroforestry is the practice of integrating woody vegetation with crops and/or livestock production in order to strengthen ecological services on farmland and achieve a more multi-functional agricultural system. Crop yields determine economic outcomes when trees are young, but information on yields is scattered in the literature and a quantitative overview of crop yields in European agroforestry systems is lacking. We therefore synthesized published information on crop yields in European agroforestry systems, using meta-analysis. A systematic review of the literature was conducted, highlighting quantitative information on yields is available only for traditional Dehesa systems in Spain and Portugal and for modern alley cropping experiments, mostly in northern Europe. Relative cereal crop yields in alley cropping systems (systems with tree rows with interspersed crop strips) were 96% of sole crop yield at tree planting. Crop yields in alley cropping decreased on average with 2.6% per year over the first 21 years of the tree stand, indicating increasing competitive effects of the trees with their age. On the other hand, studies in traditional Dehesa and Montado systems in Southern Europe showed no negative influence of the trees on crop production, indicating that competition between crops and trees plays a less important role in those systems than in alley cropping. Overall, the systematic review showed a need for more experimental data to further substantiate the benefits of agroforestry and elucidate optimal agroforestry practices under European conditions.
Mixing trees and crops in agroforestry systems is common in tropical parts of the world (Nair, 1993) but not so much in Europe (den Herder et al., 2017). Agroforestry in Europe is scarce and mainly dominated by silvopasture grazed woodlands and grasslands with sparse trees or grazed permanent crops such as olive groves in the Mediterranean and fruit orchards in continental and Atlantic regions. Unlike silvopastoral systems which are relatively common, silvoarable agroforestry is present on much smaller areas (den Herder et al., 2017). However beneficial effect of silvoarable systems combining trees with crops should not be neglected as it can play an important role in tackling the issue of climate change (Mosquera-Losada et al., 2018).
Trees mitigate microclimatic extremes, creating more stable environmental conditions for understory species, and avoiding heat stress. Increases in biodiversity also reduce plant diseases (Boudreau, 2013; Arenas-Corraliza et al., 2018). Recently, with the push toward systems that produce ecosystem services in addition to agricultural products (Bommarco et al., 2013; Geertsema et al., 2016), interest in agroforestry (AF) has increased in Europe, especially amongst policy makers. It is expected that mixing trees and/or shrubs with crops can contribute to production of ecosystem services from agricultural land (e.g., carbon storage, soil improvement, erosion control and mitigation of crop pests and diseases) and achieve economically efficient land use with both tree and crop outputs from the same parcel of land (Quinkenstein et al., 2009; García de Jalón et al., 2018).
European agroforestry systems can be split based on geography and cultural history (Riguero-Rodriguez et al., 2009). Traditional AF comprises Dehesa and Montado systems in Spain and Portugal, wood pastures, hedgerows and windbreaks, fruit trees on arable land or grassland (pré-verger in France) and Streuobst in Germany. Traditional systems are declining in area (Nerlich et al., 2013; Krčmarova and Jelecek, 2017), while new systems based on the concept of “alley cropping” are gaining more attention (Wolz and DeLucia, 2018). Alley cropping entails planting trees in rows, with annual crops planted in strips between the tree rows as long as the trees are not too tall and competitive, especially for light. Such systems in Europe mostly consist of walnut, poplar and willow trees while growing wheat, barley and legumes in between the rows (Dupraz et al., 2005; Graves et al., 2009; Cardinael et al., 2015a; Ehret et al., 2015). In such systems it is expected that relative crop yields will start to significantly decrease around year 10 (Graves et al., 2010). In southern Europe, traditional systems still cover large areas, e.g., “Dehesa” in Spain and “Montado” in Portugal (Mosquera-Losada et al., 2012; den Herder et al., 2017). These are low input production systems with low numbers of scattered trees per hectare. Most of the trees are quite old, e.g., a century, and they usually are a species of oak (e.g., Quercus suber, Q. ilex), which may be used for producing cork or acorns for raising pigs (Moreno and Pulido, 2009). The understory consists of grass but also of animal fodder crops such as oats and various legumes (Moreno et al., 2007; Moreno, 2008; Cubera et al., 2009; Gea-Izquierdo et al., 2009). The Dehesa is an ancient system supporting a rural economy based mostly on raising animals. The northern European systems are limited by light while Mediterranean systems are primarily limited by water (Eichhorn et al., 2006).
There are many examples of AF from the tropics, where shading by trees is a benefit for plant species that perform better under shade, e.g., coffee. On poor soils under dry conditions, AF can significantly increase crop yield since tree shading has a positive influence on microclimate, and tree litter can contribute positively to soil quality (Jose, 2009). Trees have been reported to increase pasture yield under the canopy by reducing evapotranspiration, recycling nutrients and promoting hydraulic lift, i.e., the transport of water from moist into drier, in general upper, soil layers through plant roots (Ludwig et al., 2001, 2003, 2004; Mendel et al., 2002; Moreno and Pulido, 2009; Bayala and Prieto, 2019). However, in northern parts of Europe light levels may not be high enough to make shading beneficial for the understorey species. Rather, it is expected that shading will decrease crop yields due to competition (van der Werf et al., 2007). Therefore, the trees can have a beneficial but also a negative effect on understorey specie. According to Rivest et al. (2013), there is little consensus regarding the effect of trees on annual crop yields.
Despite the reported advantages of AF for yields and ecosystem services, traditional AF systems with scattered trees are disappearing world-wide due to intensification of agriculture (Eichhorn et al., 2006; Fisher et al., 2010; Nerlich et al., 2013). On the other hand, interest in alley cropping systems is increasing as a result of an increased demand for food and energy, and the aspiration to make agriculture more sustainable by mixing trees and crops (Dupraz et al., 2005; Tsonkova et al., 2012; Wolz and DeLucia, 2018).
There is a substantial amount of AF research in Europe. Most studies deal with ecological aspects, such as carbon sequestration, nutrient competition, root length and root interaction of trees and crop (Quinkenstein et al., 2009; Plieninger, 2011; Smits et al., 2012; Upson and Burgess, 2013; Cardinael et al., 2015a,b; Medinski et al., 2015). A recent study of Torralba et al. (2016) showed a beneficial effect of AF on biodiversity and ecosystem services. Studies on crop yields in agroforestry have also been conducted, however, the results have not been put together in an overarching analysis. The lack of such analysis makes it difficult to assess the economic benefit of AF in terms of crop yields. Yields are a key aspect that farmers are interested in Graves et al. (2009).
In this study, we reviewed the literature and synthesized the reported crop yields in European AF systems. The aim of our investigation was to assess the following: (1) How does crop production in agroforestry vary across European studies? (2) Are there general trends in yield response to tree age, tree density and distance from the tree, and if so, what are these trends? (3) Are there differences in crop yield response between modern systems with trees in rows (“alley cropping”) and traditional systems with scattered trees such as Dehesa? (4) Are there differences in crop yield response in alley cropping systems between different parts of Europe (south and north)?
A literature search for AF data in Europe was conducted in September 2019 in the Web of Science Core Collection (WoSCC) and SCOPUS. The search consisted of three search combinations in the “topic” field: #1 Agroforestry AND (Europe OR yield) AND alley cropping AND silvoarable; #2 Agroforestry AND Europe AND (yield OR alley cropping) AND silvoarable; #3 Agroforestry AND Europe AND yield AND (alley cropping OR silvoarable). The search identified 339 papers out of which we selected publications containing primary data on crop yields in European AF. We selected only those studies that contained a tree-free control, or, in Dehesa, observation plots that were far enough from the trees to be used as a control. Control treatment information was necessary to be able to conduct a meta-analysis as the effect size estimator was the relative yield, i.e., crop yield mean in AF system relative to the control treatment mean which was a reference yield. Some studies reported yields per unit cropped area (Burgess et al., 2004), some per unit total AF area as well as cropped area (Dufour et al., 2013), some report already relative yields presumably obtained from the total area (Graves et al., 2010) and some (mainly the studies from Dehesa reporting biomass yields) report yields based on the g DM/m2. Such data is also considered to be expressed per unit cropped area, however since in Dehesa is a system with low number of trees (mainly ranging from 7 to 29 trees per ha) we do not expect large differences between yield per unit cropped area and or per unit total area of Dehesa.
Since we have decided to run the analysis using relative yields obtained for the total area and not cropped area we needed to transform the data from Burgess et al. (2004), using information on the cropped area, to yields per total area. As explained earlier for the data from Dehesa systems we assumed it would not be much different due to low number of trees so we used the data as they were. This way we obtained the set of data for relative yields per total area for all 13 papers further used in the analysis (Figure 1). The search identified 13 publications which reported data from 22 locations in Europe. Data from the location “Silsoe” were reported in two publications but from different time periods (Table 1). Thirteen papers might seem as low number but the criterion to synthesize all the available information without prejudice is met in our analysis (Makowski et al., 2019). According to Philibert et al. (2012) number of papers in meta-analysis studies in the field of agronomy is in the range between 5 and 257 studies with a median of 32 studies in the investigated 73 papers on meta-analysis, so the number of studies (papers) can be as low as 5 as long as all of the available papers are included. Torralba et al. (2016) also conduct a meta-analysis of European agroforestry and they also found only 13 papers for silvoarable systems in Europe and that is the number they use as that is the number of papers available.
To avoid high influence of data coming from one study we are using “site” as random factor in the model this way we reduce the weight of particular study/site. In addition, since we have low number of papers, we have run the sensitivity analysis by “Jacknife” approach where we have run the same analysis by excluding one paper (so we run the same model 14 times, once with all 13 papers and thirteen times with 12 papers always excluding one paper). The results of sensitivity analysis are in the Supplementary Material and they confirm that 5 out of 6 of our models have not been influenced by single study. Only model on the effect of distance is not reliable due to the influence of one single study (Moreno et al., 2007), so this model is shown but cannot be trusted. This issue of distance effect is discussed later in the text.
Ten publications reported data from southern Europe only (Moreno et al., 2007; Moreno, 2008; Cubera et al., 2009; Gea-Izquierdo et al., 2009; Hussain et al., 2009; Rivest et al., 2011; Dufour et al., 2013; Lośpez-Carrasco et al., 2015; Arenas-Corraliza et al., 2018; Inurreta-Aguirre et al., 2018) and two reported data from northern Europe only (Burgess et al., 2004; Ehret et al., 2015). Only one publication reported data from both northern and southern Europe (Graves et al., 2010) (Figure 2). Some studies investigated yields of fodder crops, mainly legumes and grasses (Moreno, 2008; Cubera et al., 2009; Gea-Izquierdo et al., 2009; Hussain et al., 2009; Rivest et al., 2011; Ehret et al., 2015; Lośpez-Carrasco et al., 2015), some studies investigated grain yields of cereals and legumes (Dufour et al., 2013; Arenas-Corraliza et al., 2018; Inurreta-Aguirre et al., 2018), while a few investigated both grain yields and fodder yields (Burgess et al., 2004; Graves et al., 2010) (Table 1). From these 13 publications we extracted 267 observation records of the yield data (AF and control site) and information on distance from trees or tree density, tree age, region, crop species and tree species. The data originated from alley cropping studies in France, the UK (Cirencester, Leeds, Silsoe), Spain and Germany, and from traditional systems in Dehesa and Montado. Crop and tree species varied substantially among studies (Table 1).
Figure 2. Map of experimental sites. Alley cropping sites are shown with solid blue circles (•), while Dehesa sites are shown with solid red circles (•). A horizontal line at 45 degrees latitude separates “northern” from “southern” sites. Inset shows the Iberian peninsula enlarged to show locations of sites in the Extremadura (1–8), West-central Spain (1–2), and Evora (1–2). Multiple sites in the same region were identified by number, e.g., 1–8 in Extremadura. See Table 1 for further information on sites.
To standardize crop yields across studies, we focused the analysis on relative yields, defined as the ratio of crop yield in the intercropped system to crop yield in the sole crop treatment (Yu et al., 2015). All of the selected studies had information on crop yield at a control site from which we calculated relative yields in the AF stand; the only exception was Moreno et al. (2007) who investigated the influence of tree distance on crop yield in Dehesa. Here, the authors do not report the yields at a control site but rather the yields at different distances from the tree. Yield at this furthest distance from the tree (30 m) was used as the control yield as the authors state that 30 m from the tree the shading effect will have no or very little influence on crop yield.
Five studies had management treatments (fertilization or grass cutting regimes), with data for AF and sole crop plots with the same treatment, so relative yields could be calculated for different management (Burgess et al., 2004; Moreno et al., 2007; Moreno, 2008; Dufour et al., 2013; Ehret et al., 2015). Some studies collected data over several years (Burgess et al., 2004; Graves et al., 2010; Dufour et al., 2013; Ehret et al., 2015). In these cases, we extracted a data record for each year of observation. In Dehesa systems, experimenters reported crop yields at different distances from trees on the basis of quadrats placed at various locations. Data for different distances from the trees were entered as separate records. In alley cropping, quadrats were also used to measure crop yields, but only the average crop yield in the strips was reported.
We used mixed effects modeling to analyze the effects of AF systems on relative crop yields (Pinheiro and Bates, 2000). The parameters of AF systems include tree distance (defined as half a distance between tree rows in alley cropping and distance from harvested plots to the nearest tree in Dehesa), tree age, tree density and the region (south or north) in which an AF experiment was conducted, as well as cropping system (alley cropping vs. Dehesa). In the case of Dehesa, tree age is in the order of 100 years or more, but information was lacking, therefore the effect of tree age was only analyzed for alley cropping. The experimental site was considered as a random effect. Random effects associated with experiment account for effects of locations, year, and other study effects which are not accounted for by the fixed effects included in the statistical model (Zuur et al., 2009; Makowski et al., 2019). Using experiment as a random factor furthermore accounts for correlation between data originating from the same experiment, thus accounting for imbalance in the number of observations between different experiments, and ensuring that each experiment gets an appropriate weight in the analysis in relation to the number of observation records and the correlation between measurements from the same study (Zuur et al., 2009; Makowski et al., 2019).
Six mixed effects models were fitted to the data (Table 2). Model 1 estimates the mean relative yield for Dehesa and alley cropping. Here β0 is the average relative yield in Dehesa, and β1 is the difference in relative yield between alley cropping and Dehesa. The standard errors of the relative yields in Dehesa and alley cropping were estimated in a model version without intercept (Zuur et al., 2009). The random variation in relative yield between experiments is described by ai. This term is normally distributed with mean zero and a variance which describes the variation in relative yield between experiments. Residual variation is described by εij where i denotes experiment and j denotes an observation in an experiment. Differences in relative yield in alley cropping between northern and southern Europe were analyzed with model 2. In this model, β1 describes the difference in relative yield between northern and southern Europe. The effect of tree density was described with a common model (model 3) for Dehesa and alley cropping after we had explored a model (model 4) allowing for differences between Dehesa and alley cropping in this relationship (but which showed no significant interaction or difference in intercept between Dehesa and alley cropping). Tree density is a continuous variable in models 3 and 4, and is expressed as number of trees/ha. Model 5 was used to estimate the effect of tree age on crop yields. Ages of the trees were only well-known for alley cropping and no studies with young trees have been done in Dehesa; therefore, the effect of tree age was only studied for alley cropping. Six studies (Studies 1–4, 12, and 13 in Table 1) reported the effect of tree age, yielding 69 data records from 10 experimental sites. Studies reported yields of both cereals and fodder crops, hence the similarity of response of these two types of understory crops was investigated in alley cropping. A difference in intercept was included to account for differences in relative yield between grain producing crop species and fodder crop species harvested for their biomass. Furthermore, a model with interaction between crop type and tree age was also fitted, but this model has less support (judged by ΔAIC) than the model without interaction. We fitted a common model (model 6) for the effect of distance to the trees using as the explanatory variable the actual distance to the trees as measured in Dehesa systems, and the distance between the tree lines in alley cropping. In fitting this model, we modeled the variance as a power of tree distance using the varPower function of the package nlme (Pinheiro et al., 2020).
Table 2. Specification of models fitted to the data. The indices i and j represent experiment ID and treatment ID within an experiment, respectively.
All the analyses were conducted in R (R Core Team, 2013), using the R function lme from the R package nlme (Pinheiro et al., 2020). The assumption of equal variance was checked by quantile plots and plots of model residuals against fitted values (Zuur et al., 2009). No violations of model assumptions were found. Figures were made using the R packages plotrix and graphics (Lemon, 2006; R Core Team, 2013).
Grain yields in AF varied from 2 to ~7 t/ha (Figure 3). Fodder crop total biomass yields in Dehesa varied from ~0.25 to 11 tons/ha with large differences between studies (Figure 3).
Figure 3. Boxplot of grain yield (studies 1 and 2) and total biomass (studies 2 and 4–10) in agroforestry systems in Europe. The lower and upper borders of the boxes represent the first and the third quartile of measured yield in the study. The middle bar represents the median. The upper whisker represents the third quartile plus 1.5 times the interquartile range (IQR) or the largest value observed in the study, whichever of the two is closer to the median. The lower whiskers represents the first quartile minus 1.5 times the IQR or the lowest crop yield in a study whichever of the two is closer to the median. Data more extreme than the whiskers are drawn as individual points. Study 3 is not represented in this figure because only relative yield data were available.
When expressed as relative yields (in AF as compared to sole crop), variation in crop yields was moderate for alley cropping, with relative yields mostly ranging between 50 and 80%, whereas relative yields in Dehesa varied greatly (Figure 4). The occurrence of some very high relative yields indicates a large spatial variability of crop yield in Dehesa and a potentially positive effect of trees on crop yield. Relative yields greater than one were not reported from alley cropping systems.
Figure 4. Boxplot of relative crop yield (%) based on grain yield (left part) or based on total biomass (right part) for each study. Studies 2 and 3 reported both relative grain yield and relative biomass, and these are both shown. For further explanation, see legend of Figure 2.
Relative crop yields from Dehesa were on average (102 ± 4.1%, n = 198) significantly higher than those from alley cropping (71 ± 3.7%, n = 67) (Model 1, P < 0.001). When distinction was made between relative crop yields in alley cropping systems in the north and the south, no significant difference was found (Model 2). This north-south comparison was not made for Dehesa because Dehesa only occurs in the Mediterranean region and not in northern Europe.
Relative yield decreased with the density of trees (Model 3; Figure 5) whereby an increase in tree density by 100 trees per ha was associated with a decrease of relative yield by 20% (β1 = −0.2, p- = 0.03). The R2 is low indicating that small percentage of variation is explained by the model suggesting that such model has low precision. However, low p-values still indicate a significant relationship between the relative yields and tree density confirming that the crop yield is dependent on tree density (Figure 5). Cropping system (Alley cropping vs. Dehesa) did not influence the effect of tree density on relative yield (Model 4, β3 = −0.07, P = 0.64). The common relationship between alley cropping and Dehesa indicates that the low density of trees in Dehesas may be an important factor explaining the higher average relative crop yield in Dehesa as compared to alley cropping.
Figure 5. Plot of relative crop yields against tree density. The symbols distinguish experimental sites of data points. The regression line was fitted with Model 3 (Table 2).
A mixed effects model using a common slope for cereals and fodder crops was fitted to the data (Model 5) (Table 2). There was a negative effect of tree age on relative crop yields for both cereals and fodder crops, but the intercepts were significantly different (Figure 6). Fodders had significantly lower relative yields than cereals (β2 = −15.46, P < 0.0001). An increase of tree age with 1 year would result in a decrease of relative yield by 2.6% for both types of understory crop.
Figure 6. Plot of relative crop yields against tree age for cereals (black) and fodder crops (red) in alley cropping trials. A mixed effects model using a common slope was fitted to the data: RYij = β0 + β1*TreeAgeij + β2*Intercropij + ai +εij (Model 5). The symbols distinguish experimental sites of data points.
Studies in Dehesa often report the distance to the nearest tree of the quadrats on which yields are measured. In alley cropping, the half distance between tree lines is a useful proxy for the distance between the crop and the trees. A common analysis was therefore conducted using distance to trees in Deheas and distance between tree rows in alley cropping as a measure for the proximity of crop and trees. There was no interaction between crop system and tree distance, nor was there an identifiable effect of distance for either of the cropping systems when separating the database. Therefore, a model with common slope was fitted to the data (Model 6: RYij = β0 + β1*Distanceij + ai +εij) showing a significant effect of distance on relative crop yields in the combined data of alley cropping and Dehesa (Model 6: β1 = 0.56, P = 0.04), indicating that relative crop yield increased by 0.56% with each additional m distance to the nearest tree. However, sensitivity analysis (Supplementary Material) indicated that study by Moreno et al. (2007) influence our model by changing the distance effect from positive to negative. Therefore, model for distance is not reliable. We also analyzed the interaction of distance and tree age and found that there was no interaction. In the model that includes both tree distance and tree age, tree age was not significant. Variability of relative yields was greatest at a small distance from the tree. All of this variability originated from Dehesa systems.
Key findings of this study are: (1) relative crop yields decrease with tree density, both in alley cropping and Dehesa, (2) relative crop yields decrease with tree age in alley cropping (not studied in Dehesa because of missing data on tree age) (3) relative crop yields tended to be higher in Dehesa than in alley cropping, (4) alley cropping gave similar relative crop yields in northern and southern Europe, and (5) relative yields of fodders in alley cropping systems tended to be lower than those of cereals.
The lack of a north-south difference in relative crop yield in alley cropping suggests that the difference in relative crop yield between alley cropping and Dehesa is due to differences between the two systems, and not due to differences in ecological conditions between north and south, which of course exist, but were also present in the alley cropping data, for which we found no difference in relative crop yields between north and south Europe. However, the comparison between modern AF systems and traditional systems was limited by the data for other traditional systems. The only traditional system that had data of relative crop yields were Dehesa and Montado in southern Europe. So our only conclusion for traditional agroforestry systems came from these systems in southern Europe. There is no data on hedgerows and windbreaks, fruit tree systems on arable land or grassland (prée'-verger in France) and Streuobst in Germany (Nerlich et al., 2013). Therefore, the traditional agroforestry is rather productivity and relative yields of Dehesa systems and not traditional agroforestry in general.
While there are many differences between alley cropping and traditional AF, and between northern and southern Europe, the effect of tree density was the same for both systems as we identified a common relationship between relative crop yield and tree density, regardless of the cropping system. The relative crop yields decreased with increased tree density.
Our results indicate that relative crop yields are higher in Dehesa (traditional AF) than in alley cropping. Tree densities are much higher in alley cropping than in Dehesa, and relative crop yields in response to tree density in alley cropping and Dehesa fit a common regression, while the support of a single regression line was better than for two separate lines. The high relative crop yields in Dehesa as compared to alley cropping are thus associated with a low density of trees and may be due to the lesser shade generated by the few trees per ha and the lower competition for water and nutrients as well as facilitative effects of trees compared to alley cropping systems. Competition for light, water and nutrients are driving forces controlling plant growth and crop yields in AF systems (Burgess et al., 2004; Eichhorn et al., 2006; van der Werf et al., 2007). Previous studies reported light interception by trees as the main parameter influencing crop growth and crop yield (Burgess et al., 2004; Dupraz et al., 2005; Tsonkova et al., 2012) although in hot and dry areas the shading can result with beneficial effect on understorey yields (Moreno, 2008).
When analyzing effect of tree age, we used age as a proxy for the size of the tree, but there are of course substantial differences between tree species in their rate of growth. A 5 year old tree of a fast growing species, e.g., poplar, will cause substantially more shading than a 5 year old specimen of a slow growing species, e.g., walnut. A better proxy for the size and resource capture by trees would be, for instance, their biomass or relative ground cover, but such data were not reported in the synthesized studies. If more data sets are published over time, the age effect could be estimated per species in the future. There is specifically a need for more data on the relationship between tree size and light interception in AF. Furthermore, crop species also differ regarding the tolerance to shade (Garrett et al., 2009). In alley cropping, cereal and fodder crops showed similar, though not identical responses to tree age, in general, with higher age the relative crop yield was lower. Such trend has been observed in temperate alley cropping systems in North America as well where, in some cases, in first few years crop yields were even higher in alley cropping systems than in monoculture fields (Garrett et al., 2009). There was no interaction between tree age and distance. In a model that included both tree distance and tree age, tree age was not significant while tree density was significant, indicating that in the available dataset, tree density is the key factor affecting the crop yield.
The effect of distance on relative crop yields was significant when a model with a common slope was fitted for alley cropping and Dehesa together. However, sensitivity analysis (Supplementary Material) indicated significant effect of one study (Moreno et al., 2007) on the results. Therefore, our model cannot be reliable. When analyzing the data separately for each system no significant effect of distance was observed. The lack of effect of distance in alley cropping is not surprising because of the relatively small variation in width of the alleys (from 6 to 16 m) (Burgess et al., 2004; Graves et al., 2009; Dufour et al., 2013; Ehret et al., 2015; Arenas-Corraliza et al., 2018; Inurreta-Aguirre et al., 2018) and the effect of other factors such as tree density and tree age in the dataset. Greater variation in alley width is needed to evaluate its effect on crop yield. In Cottbus, Germany, alley cropping systems have been installed with widths of 24, 48, and 96 m (Medinski et al., 2015). Data on relative crop yields from such AF systems could improve our knowledge on developing ideal alley cropping designs for Europe. The large scale required for such experiments poses challenges for experimental design, to ensure homogeneity of soil conditions for different treatments (Seserman et al., 2019).
No effect of distance in Dehesa, when analyzed separately, was influenced by opposite findings in particular studies. In Moreno et al. (2007) the relative yields increase with distance while in Gea-Izquierdo et al. (2009) the relative yields decrease with distance. We see that results from Dehesa are site specific and in some studies relative yields are extremely high in vicinity of trees (Moreno, 2008; Gea-Izquierdo et al., 2009) which can be explained by facilitative effects. According to the stress gradient hypothesis, in adverse environmental conditions such as in Dehesa, facilitation may increase in relative importance as compared to competition (Brooker, 2006; Maestre et al., 2009). Facilitation of crops by trees may result from a reduction in water stress due to shading by the tree, improved soil organic matter due to litter input, and associated improvements in the retention of water and nutrients in the soil in the proximity of trees (Moreno and Pulido, 2009). Furthermore, understory species in Dehesa may have been selected to do well in the shade of the trees. In addition, it should be considered that the exact location of trees in Dehesa systems is irregular and not necessarily planned. The location of trees in a field may well represent areas in the field with better soil or water availability due to variation in the terrain. This would result in a higher crop yield near trees, not as a result of facilitation, but as a result of a positive association between the presence of a tree and the local availability of resources in relation to within-field heterogeneity. It is not possible to test this hypothesis in a meta-analysis, but several studies report better soil quality in the vicinity of trees by increased soil organic matter, N mineralization and potassium concentrations (Gallardo et al., 2000; Gallardo, 2003). However, it is still unclear if better soil quality in the vicinity of trees is due to the field heterogeneity or due to the influence of trees, nevertheless their findings show better soil quality in the vicinity of trees. Due to all these adverse effects in our analysis the effect of distance was not possible to model with the obtained dataset.
While traditional AF systems in Europe are disappearing, new AF practices such as alley cropping are gaining interest to secure food production and at the same time increase and preserve biodiversity and provide ecosystem services (Eichhorn et al., 2006; Nerlich et al., 2013). In order to preserve and even increase agroforestry in Europe the disappearance of traditional agroforestry systems needs to cease and at the same time new agroforestry systems need to be encouraged (Nerlich et al., 2013; Pantera et al., 2018; Rois-Díaz et al., 2018). The comparison of crop yields in alley cropping and Dehesa suggests that a longer term production of both crops and trees on the same land can be achieved by lowering tree density, e.g., by widening the alleys. This will maintain the crop production and at the same time maintain the beneficial effect of trees on the system as a whole.
With only thirteen retrieved studies containing primary quantitative information on crop yields in AF under European conditions, we conclude that there is a scarcity of relevant information on yields in AF system under European growing conditions. There is particularly a lack of data on crop yield which is decisive for the economic returns before the trees become productive (Graves et al., 2007). Further data collection on crop yields remains necessary to determine profitability and feasibility of AF in Europe.
The original contributions presented in the study are included in the article/supplementary material, further inquiries can be directed to the corresponding author/s.
VI: developing the idea, search of the database, and writing the manuscript. YY: statistical analysis. WW: statistical analysis, critical review, and writing the manuscript. All authors contributed to the article and approved the submitted version.
This study was supported by the Croatian science foundation project: Intercropping of wood species and agricultural crops as an innovative approach in agroecosystems (UIP-7103) and by the ERASMUS exchange program for staff.
The authors declare that the research was conducted in the absence of any commercial or financial relationships that could be construed as a potential conflict of interest.
The Supplementary Material for this article can be found online at: https://www.frontiersin.org/articles/10.3389/fsufs.2021.606631/full#supplementary-material
Arenas-Corraliza, M. G., López-Díaz, M. L., and Moreno, G. (2018). Winter cereal production in a Mediterranean silvoarable walnut system in the face of climate change. Agric. Ecosyst. Environ. 264, 111–118. doi: 10.1016/j.agee.2018.05.024
Bayala, J., and Prieto, I. (2019). Water acquisition, sharing and redistribution by roots: applications to agroforestry systems. Plant Soil 453, 17–28. doi: 10.1007/s11104-019-04173-z
Bommarco, R., Kleijn, D., and Potts, S. G. (2013). Ecological intensification: harnessing ecosystem services for food security. Trends Ecol. Evol. 28, 230–238. doi: 10.1016/j.tree.2012.10.012
Boudreau, M. A. (2013). Diseases in intercropping systems. Annu. Rev. Phytopathol. 51, 499–519. doi: 10.1146/annurev-phyto-082712-102246
Brooker, R. W. (2006). Plant–plant interactions and environmental change. New Phytol 171, 271–284. doi: 10.1111/j.1469-8137.2006.01752.x
Burgess, P. J., Incoll, L. D., Corry, D. T., Beaton, A., and Hart, B. J. (2004). Poplar (Populus spp) growth and crop yields in a silvoarable experiment at three lowland sites in England. Agrofor. Syst. 63, 157–169. doi: 10.1007/s10457-004-7169-9
Cardinael, R., Chevallier, T., Barthès, B. G., Saby, N. P. A., Parent, T., Dupraz, C., et al. (2015a). Impact of alley cropping agroforestry on stocks, forms and spatial distribution of soil organic carbon — a case study in a Mediterranean context. Geoderma 259–260, 288–299. doi: 10.1016/j.geoderma.2015.06.015
Cardinael, R., Mao, Z., Prieto, I., Stokes, A., Dupraz, C., Kim, J. H., et al. (2015b). Competition with winter crops induces deeper rooting of walnut trees in a Mediterranean alley cropping agroforestry system. Plant Soil 391, 219–235. doi: 10.1007/s11104-015-2422-8
Cubera, E., Nunes, J. M., Madeira, M., and Gazarini, L. (2009). Influence of Quercus ilex trees on herbaceous production and nutrient concentrations in southern Portugal. J. Plant Nutr. Soil Sci. 172, 565–571. doi: 10.1002/jpln.200800191
den Herder, M., Moreno, G., Mosquera-Losada, R. M., Palma, J. H. N., Sidiropoulou, A., Santiago Freijanes, J. J. S., et al. (2017). Current extent and stratification of agroforestry in the European Union. Agric. Ecosyst. Environ. 241, 121–132. doi: 10.1016/j.agee.2017.03.005
Dufour, L., Metay, A., Talbot, G., and Dupraz, C. (2013). Assessing light competition for cereal production in temperate agroforestry systems using experimentation and crop modelling. J. Agro. Crop Sci. 199, 217–227. doi: 10.1111/jac.12008
Dupraz, C., Burgess, P., Gavaland, A., Graves, A., Herzog, F., Incoll, L., et al. (2005). Synthesis of the Silvoarable Agroforestry for Europe Project. Montpellier: INRA, Unitè SYSTEM (Systèmes de Culture Tropicaux et Mèditerranèens).
Ehret, M., Buhle, L., Gra,ß, R., Lamersdorf, N., and Wachendorf, M. (2015). Bioenergy provision by an alley cropping system of grassland and shrub willow hybrids: biomass, fuel characteristics and net energy yields. Agroforest Syst. 89, 365–381. doi: 10.1007/s10457-014-9773-7
Eichhorn, M. P., Paris, P., Herzog, F., Incolli, L. D., Liagre, F., Mantzanas, K., et al. (2006). Silvoarable systems in Europe – past, present and future prospects. Agroforest. Syst. 67, 29–50. doi: 10.1007/s10457-005-1111-7
Fisher, J., Zerger, A., Gibbons, P., Stott, J., and Law, B. S. (2010). Tree decline and the future of Australian farmland biodiversity. Proc. Natl. Acad. Sci. U.S.A. 107, 19597–19602. doi: 10.1073/pnas.1008476107
Gallardo, A. (2003). Effect of tree canopy on the spatial distribution of soil nutrients in a Mediterranean Dehesa. Pedobiologia 47, 117–125. doi: 10.1078/0031-4056-00175
Gallardo, A., Rodríguez-Saucedo, J., Covelo, F., and Fernadez-Ales, R. (2000). Soil nitrogen heterogeneity in a Dehesa ecosystem. Plant Soil 222, 71–82. doi: 10.1023/A:1004725927358
García de Jalón, S., Graves, A., Palma, J. H. N., Williams, A., Upson, M., and Burgess, P. J. (2018). Modelling and valuing the environmental impacts of arable, forestry and agroforestry systems: a case study. Agroforest Syst. 92, 1059–1073. doi: 10.1007/s10457-017-0128-z
Garrett, H. E., McGraw, R. L., and Walter, W. D. (2009). “Alley cropping practices,” in North American Agroforestry: An Integrated Science and Practice, 2nd Edn, Copyright © 2009, ed H. Garrett (Madison, WI: American Society of Agronomy), 133–162.
Gea-Izquierdo, G., Montero, G., and Cañellas, I. (2009). Changes in limiting resources determine spatio-temporal variability in tree–grass interactions. Agroforest. Syst. 76, 375–387. doi: 10.1007/s10457-009-9211-4
Geertsema, W., Rossing, W. A. H., Landis, D. A., Bianchi, F. J. J. A., van Rijn, P. C. J., Schaminée, J. H. J., et al. (2016). Actionable knowledge for ecological intensification of agriculture. Front. Ecol. Environ. 14, 209–216. doi: 10.1002/fee.1258
Graves, A. R., Burgess, P. J., Palma, J. H. N., Herzog, F., Moreno, G., Bertomeu, M., et al. (2007). Development and application of bio-economic modelling to compare silvoarable, arable, and forestry systems in three European countries. Ecol. Eng. 29, 434–449. doi: 10.1016/j.ecoleng.2006.09.018
Graves, A. R., Burgess, P. J., Palma, P., Keesman, K. J., Werf van der, W., Dupraz, C., et al. (2010). Implementation and calibration of the parameter-sparse Yield-SAFE model to predict production and land equivalent ratio in mixed tree and crop systems under two contrasting production situations in Europe. Ecol. Modell. 221, 1744–1756. doi: 10.1016/j.ecolmodel.2010.03.008
Graves, A. R., Burgessa, P. J., Liagre, F., Pisanelli, A., Paris, P., Moreno, G., et al. (2009). Farmer perceptions of silvoarable systems in seven European countries. Adv. Agroforest. 6, 67–89. doi: 10.1007/978-1-4020-8272-6_4
Hussain, M. Z., Otieno, D. O., Mirzae, H., Li, Y. L., Schmidt, M. W. T., Siebke, L., et al. (2009). CO2 exchange and biomass development of the herbaceous vegetation in the Portuguese montado ecosystem during spring. Agric. Ecosyst. Environ. 132, 143–152. doi: 10.1016/j.agee.2009.03.008
Inurreta-Aguirre, H. D., Lauri, P. É., Dupraz, C., and Gosme, M. (2018). Yield components and phenology of durum wheat in a Mediterranean alley-cropping system. Agroforest Syst. 92, 961–974. doi: 10.1007/s10457-018-0201-2
Jose, S. (2009). Agroforestry for ecosystem services and environmental benefits: an overview. Agroforest Syst. 76, 1–10. doi: 10.1007/s10457-009-9229-7
Krčmarova, J., and Jelecek, J. (2017). Czech traditional agroforestry: historic accounts and current status. Agroforest Syst. 91, 1087–1100. doi: 10.1007/s10457-016-9985-0
Lemon, J. (2006). Plotrix: a package in the red light district of R. R-News 6, 8–12. Available online at: https://cran.r-project.org/web/packages/plotrix/citation.html
Lośpez-Carrasco, C., López-Sánchez, A., San Miguel, A., and Roig, S. (2015). The effect of tree cover on the biomass and diversity of the herbaceous layer in a Mediterranean dehesa. Grass Forage Sci. 70, 639–650. doi: 10.1111/gfs.12161
Ludwig, F., Dawson, T. E., De Kroon, H., Berendse, F., and Prins, H. H. T. (2003). Hydraulic Lift in Acacia tortilis trees on an East African savanna. Oecologia 134, 293–300. doi: 10.1007/s00442-002-1119-x
Ludwig, F., De Kroon, H., Berendse, F., and Prins, H. H. T. (2004). The influence of savanna trees on nutrient, water and light availability and the understorey vegetation. Plant Ecol. 170, 93–105. doi: 10.1023/B:VEGE.0000019023.29636.92
Ludwig, F., de Kroon, H., Prins, H. H. T., and Berendse, F. (2001). Effects of nutrients and shade on tree-grass interactions in an East African savanna. J. Veg. Sci. 12, 579–588. doi: 10.2307/3237009
Maestre, F. T., Callaway, R. M., Valladares, F., and Lortie, C. J. (2009). Refining the stress-gradient hypothesis for competition and facilitation in plant communities. J. Ecol. 97, 199–205. doi: 10.1111/j.1365-2745.2008.01476.x
Makowski, D., Piraux, F., and Brun, F. (2019). From Experimental Network to Meta-Analysis. Dordrecht: Springer.
Medinski, T. V., Freese, D., and Bohm, C. (2015). Soil CO2 flux in an alley-cropping system composed of black locust and poplar trees, Germany. Agroforest Syst. 89, 267–277. doi: 10.1007/s10457-014-9764-8
Mendel, M., Hergarten, S., and Neugebauer, H. J. (2002). On a better understanding of hydraulic lift: a numerical study. Water Resour. Res. 38, 1–10. doi: 10.2134/2009.northamericanagroforestry.2ed.c7
Moreno, G. (2008). Response of understorey forage to multiple tree effects in Iberian dehesas. Agric. Ecosyst. Environ. 123, 239–244. doi: 10.1016/j.agee.2007.04.006
Moreno, G., Obrador, J. J., and Garcia, A. (2007). Impact of evergreen oaks on soil fertility and crop production in intercropped dehesas. Agric. Ecosyst. Environ. 119, 270–280. doi: 10.1016/j.agee.2006.07.013
Moreno, G., and Pulido, F. J. (2009). “The functioning, management and persistence of dehesas. Adv. Agroforest. 6, 127–160. doi: 10.1007/978-1-4020-8272-6_7
Mosquera-Losada, M. R., Moreno, G., Pardini, A., McAdam, J. H., Papanastasis, V., Burgess, P. J., et al. (2012). Past, Present and Future of Agroforestry Systems in Europe. Adv. Agrofor. 9, 285–313. doi: 10.1007/978-94-007-4676-3_16
Mosquera-Losada, M. R., Santiago-Freijanesa, J. J., Rois-Díaza, M., Morenod, G., den Herder, M., Aldrey-Vázquezb, J. A., et al. (2018). Agroforestry in Europe: a land management policy tool to combat climate change. Land Use Policy 78, 603–613. doi: 10.1016/j.landusepol.2018.06.052
Nair, P. K. R. (1993). An Introduction to Agroforestry. Dordrecht; Boston, MA; London: Kluwer Academic Publishers, International Centre for Research in Agroforestry.
Nerlich, K., Graeff-Honninger, S., and Claupein, W. (2013). Agroforestry in Europe: a review of the disappearance of traditional systems and development of modern agroforestry practices, with emphasis on experiences in Germany. Agroforest Syst. 87, 475–492. doi: 10.1007/s10457-012-9560-2
Pantera, A., Burgess, P. J., Mosquera Losada, R., Moreno, G., López-Díaz, M. L., Corroyer, N., et al. (2018). Agroforestry for high value tree systems in Europe. Agroforest Syst. 92, 945–959. doi: 10.1007/s10457-017-0181-7
Philibert, A., Loyce, C., and Makowski, D. (2012). Assessment of the quality of meta-analysis in agronomy. Agric. Ecosyst. Environ. 148, 72–82. doi: 10.1016/j.agee.2011.12.003
Pinheiro, J., and Bates, D. (2000). Mixed-Effects Models in S and S-PLUS. New York, NY: Springer. doi: 10.1007/b98882
Pinheiro, J., Bates, D., DebRoy, S., Sarkar, D., and R Core Team (2020). nlme: Linear and Nonlinear Mixed Effects Models. Available online at: https://CRAN.R-project.org/package=nlme (accessed February 20, 2021).
Plieninger, T. (2011). Capitalizing on the carbon sequestration potential of agroforestry in germany's agricultural landscapes: realigning the climate change mitigation and landscape conservation agendas. Landscape Res. 36, 435–454. doi: 10.1080/01426397.2011.582943
Quinkenstein, A., Wollecke, J., Bohm, C., Grunewald, H., Freese, D., Schneider, B., et al. (2009). Ecological benefits of the alley cropping agroforestry system in sensitive regions of Europe. Environ. Sci. Policy 12:1112–1121. doi: 10.1016/j.envsci,.2009.08.008
R Core Team (2013). R: A Language and Environment for Statistical Computing. R Foundation for Statistical Computing, Vienna, Austria. Available online at: http://www.R-project.org/ (accessed February 20, 2021).
Riguero-Rodriguez, A., McAdam, J., and Mosquera-Losada, M. R. (2009). Agroforestry in Europe. Dordrecht: Springer.
Rivest, D., Paquettea, A., Moreno, G., and Messiera, C. (2013). A meta-analysis reveals mostly neutral influence of scattered trees on pasture yield along with some contrasted effects depending on functional groups and rainfall conditions. Agric. Ecosyst. Environ. 165, 74–79. doi: 10.1016/j.agee.2012.12.010
Rivest, D., Roloa, V., López-Díaza, L., and Moreno, G. (2011). Shrub encroachment in Mediterranean silvopastoral systems: Retama sphaerocarpa and Cistus ladanifer induce contrasting effects on pasture and Quercus ilex production. Agr. Ecosyst. Environ. 141, 447–454. doi: 10.1016/j.agee.2011.04.018
Rois-Díaz, M., Lovric, N., Lovric, M., Ferreiro-Domínguez, N., Mosquera-Losada, M. R., den Herder, M., et al. (2018). Farmers' reasoning behind the uptake of agroforestry practices: evidence from multiple case-studies across Europe. Agroforest Syst. 92, 811–828. doi: 10.1007/s10457-017-0139-9
Seserman, D. M., Freese, D., Swieter, A., Langhof, M., and Veste, M. (2019). Trade-Off between Energy Wood and Grain Production in Temperate Alley-Cropping Systems: an Empirical and Simulation-Based Derivation of Land Equivalent Ratio. Agriculture 9:147. doi: 10.3390/agriculture9070147
Smits, N., Dupraz, C., and Dufour, L. (2012). Unexpected lack of influence of tree rows on the dynamics of wheat aphids and their natural enemies in a temperate agroforestry system. Agroforest Syst. 85, 153–164. doi: 10.1007/s10457-011-9473-5
Torralba, M., Fagerholm, N., Burgess, P. J., et al. (2016). Do European agroforestry systems enhance biodiversity and ecosystem services? A meta-analysis. Agric Ecosyst. Environ. 230, 150–161. doi: 10.1016/j.agee.2016.06.002.
Tsonkova, P., Bohm, C., Quinkenstein, A., and Freese, D. (2012). Ecological benefits provided by alley cropping systems for production of woody biomass in the temperate region: a review. Agroforest Syst. 85, 133–152. doi: 10.1007/s10457-012-9494-8
Upson, M. A., and Burgess, P. J. (2013). Soil organic carbon and root distribution in a temperate arable agroforestry system. Plant Soil 373, 43–58. doi: 10.1007/s11104-013-1733-x
van der Werf, W., Keesman, K., Burgess, P. J., Graves, A. R., Pilbeam, D. J., Incoll, L. D., et al. (2007). Yield-SAFE: a parameter-sparse process-based dynamic model for predicting resource capture, growth and production in agroforestry systems. Ecol. Eng. 29, 419–433. doi: 10.1016/j.ecoleng.2006.09.017
Wolz, J. K., and DeLucia, E. H. (2018). Alley cropping: global patterns of species composition and function. Agricult. Ecosyst. Environ. 252, 61–68. doi: 10.1016/j.agee.2017.10.005
Yu, Y., Stomph, T.-J., Makowski, D., and van der Werf, W. (2015). Temporal niche differentiation increases the land equivalent ratio of annual intercrops: a meta-analysis. Field Crops Res. 184, 133–144. doi: 10.1016/j.fcr.2015.09.010
Keywords: alley cropping, dehesa, relative yield, tree age, tree distance, tree density
Citation: Ivezić V, Yu Y and Werf Wvd (2021) Crop Yields in European Agroforestry Systems: A Meta-Analysis. Front. Sustain. Food Syst. 5:606631. doi: 10.3389/fsufs.2021.606631
Received: 15 September 2020; Accepted: 17 May 2021;
Published: 10 June 2021.
Edited by:
Mário Gabriel Santiago Santos, University of Trás-os-Montes and Alto Douro, PortugalReviewed by:
Gerardo Moreno, University of Extremadura, SpainCopyright © 2021 Ivezić, Yu and Werf. This is an open-access article distributed under the terms of the Creative Commons Attribution License (CC BY). The use, distribution or reproduction in other forums is permitted, provided the original author(s) and the copyright owner(s) are credited and that the original publication in this journal is cited, in accordance with accepted academic practice. No use, distribution or reproduction is permitted which does not comply with these terms.
*Correspondence: Vladimir Ivezić, dml2ZXppYy5wZm9zQGdtYWlsLmNvbQ==
Disclaimer: All claims expressed in this article are solely those of the authors and do not necessarily represent those of their affiliated organizations, or those of the publisher, the editors and the reviewers. Any product that may be evaluated in this article or claim that may be made by its manufacturer is not guaranteed or endorsed by the publisher.
Research integrity at Frontiers
Learn more about the work of our research integrity team to safeguard the quality of each article we publish.