- 1Department of Grain Science Technology, Central Food Technological Research Institute, Mysore, India
- 2Department of Biochemistry and Nutrition, Central Food Technological Research Institute, Mysore, India
- 3Department of Microbiology and Fermentation Technology, Central Food Technological Research Institute, Mysore, India
Monascus purpureus is known to produce pigment molecules. The pigments were extracted from M. purpureus fermented rice. In-vitro antioxidant effects of pigments were observed and presumed to alleviate oxidative stress related atherosclerosis effect in rats fed with high fat diet (HFD) for 14 weeks. The formation of lipid peroxide due to the oxidation of serum lipid was higher in rats fed with HFD. While, the feeding of fermented rice (groups III-V) significantly lowered the formation of lipid peroxide (27.1–51.7%) in serum of rats, indicated antioxidative effect of pigments. In addition, feeding of fermented rice lowered serum cholesterol and triacylglycerol by 44.82 and 45.30%, respectively. Whereas, LDL-cholesterol levels were decreased by 70.12% and HDL-cholesterol increased by 34.58%. The atherogenic indices (LDL/HDL and TC/HDL) were reduced by 77.80 and 61.05%, respectively, in rats fed with fermented rice. These data confirmed the anti-atherosclerotic effect of pigments. Further liver enzyme, 3-hydroxy-3-methylglutaryl-coenzyme A (HMG-CoA) reductase activity was significantly inhibited up to 54%. The identification of statins, sterols and fatty acids in fermented rice revealed the HMG-CoA reductase inhibitory activity. This was confirmed by synthesis of lower levels of cholesterol and triacylglycerol in liver of rats fed with fermented rice. Accordingly antioxidant, inhibition of HMG-CoA reductase, anti-atherogenic functions of M. purpureus fermented rice is attributed to the collective effect of bioactive metabolites.
Introduction
Red mold rice (RMR) is a traditional food additive of the Chinese for thousands of years. In many Asian countries, Monascus fermented rice has been consumed as food and folk medicine. Monascus spp. are well-known for various bioactive metabolites, viz., pigments (red pigments: monascorubramine and rubropunctamine, orange pigments: monascorubrin and rubropunctatin, yellow pigments: ankaflavin and monascin), isoflavones, polyketides, lipids, dimerumic acid an antioxidant (Aniya et al., 2000), γ-Aminobutyric Acid (GABA), an antihypertensive metabolite (Feng et al., 2012), metabolites with antibacterial, antitumor, and immunosuppressive properties (Martinkova et al., 1999) and, structural analogs of lovastatin (pravastatin, compactin, monacolin J). Monacolins, in particular monacolin K (MK), inhibitor of 3-hydroxy-3-methylglutaryl coenzyme A (HMG-CoA) reductase is marketed as lovastatin drug (Hachem et al., 2020). Apart from food color, Monascus pigments extended its use in biological activities, like anti-inflammatory, anticancer and antihyperlipidemic activities (Lin et al., 2011).
Monacolin K, a new hypocholesterolemic agent was first isolated from M. ruber by Endo (1979a), that competes with HMG-CoA reductase due to its structure similarity with HMG-CoA (3-Hydroxy 3-Methylglutaryl Coenzyme A). Thus, inhibits the cholesterol synthesis by prohibiting the formation of mevalonic acid an essential intermediate product for the cholesterol biosynthesis.
Dihydromonacolin-MV and dehydromonacolin MV2 isolated from M. purpureus and its hyper pigment mutant have shown biological activities (Dhale et al., 2007a,b). Dihydromonacolin-L, a potent inhibitor of cholesterol biosynthesis was isolated from the M. ruber (Endo et al., 1985). Our earlier toxicological studies of RMR did not show any adverse effect in rats (Mohan-Kumari et al., 2009).
In recent years, a high fat and cholesterol diet has been implicated as one of the risk factors for cardiovascular diseases (CVD) related problems in developed and developing countries. Atherosclerosisis is the accumulation of cholesterol-rich plaques in arteries, a key contributor to CVD, prevents sufficient blood flow to the heart. Atherosclerosis greatly progress due to the elevation of blood lipids from circulating lipoproteins and inflammation (Hansson, 2005). The atherogenic index (AI) is expressed for cholesterol level in the blood can be a risk factor for heart disease and atherosclerosis. Atherogenic risks also include oxidative stress, inflammation, obesity, diabetes, endothelial dysfunction, shear stress, homocysteine, infection, and genetic factors. Therefore, lowering the cholesterol levels in patients with cardiovascular diseases has become an important concern. Even though the diet and exercise is successful in certain patients for the management of hyperlipidemia, pharmacological intervention is required to effectively lower cholesterol. Cholesterol lowering agents that inhibit HMG-CoA reductase (EC 1.1.1.34) are prominent among the drugs of choice for treating hyperlipidemia. The available hypolipidemic drugs lowers circulating cholesterol levels either by prevention of cholesterol absorption, or increasing hepatic uptake of low density lipoproteins (LDL) or inhibit cholesterol synthesis. The HMG-CoA reductase inhibitors significantly reduce cholesterol biosynthesis in animal and human subjects owing to the structural similarity of HMG-CoA (substrate of the enzyme) and statins. Lovastatin produced by Aspergillus terreus, M. purpureus, and other fungi (Endo, 1979b) is a well-known inhibitor of HMG-CoA reductase and widely used as a hypocholesterolemic drug in humans (Alberts et al., 1980).
During the M. purpureus fermentation process multitude of secondary metabolites, pigments, monacolins, phytosterols, isoflavonoids, fatty acids, and others are produced. This study is aimed to investigate these metabolites effect which attributes antioxidant effect of RMR on total cholesterol (TC), triacylglycerol (TG), lipoprotein cholesterol (low density lipoprotein-cholesterol (LDL-C), high density lipoprotein-cholesterol (HDL-C), serum lipid, hepatic lipid, and HMG-CoA reductase enzyme in hypercholesterolemic albino rats.
Materials and Methods
Chemicals
Cholesterol and bile acid mixtures were purchased from Sigma chemical Co. (St. Louis, MO, USA). Mineral mix, vitamin mix and cellulose were obtained from Sisco Research Laboratories, Mumbai, India. Casein was purchased from Nimesh Corporation, Mumbai. The rice used for cultivating M. purpureus was purchased locally. Kits used were as follows: Total cholesterol (TC) assay kit (Product No. 11403002, Kerala, India), triacylglycerol (TG) assay kit (Product No. 11410102, Kerala, India), were purchased from Agappe Diagnostics Ltd. Kerala, India. All the solvents used were of analytical grade.
Microorganism and Red Mold Rice Preparation
M. purpureus MTCC 410 obtained from Microbial Type Culture Collection (MTCC), Institute of Microbial Technology (IMTECH) Chandigarh, India was maintained on potato dextrose agar (PDA, Hi Media, India) slants at 4–8°C. It was sub cultured every 30 days.
Seed culture was prepared by inoculating a loopful of M. purpureus MTCC 410 to 100 ml basal medium (pH 6.0) containing (dextrose (100 g), peptone (10 g), KNO3(2 g), NH4H2PO4 (2 g), MgSO4.7 H2O (0.5 g), CaCl2 (0.1 g) in 1L distilled water (Su et al., 2003). After incubation at 30°C for 48 h at 110 rpm, 5% seed medium (volume/weight) was used for solid state fermentation.
The traditional RMR was prepared by inoculating 500 g of rice (sterilized/cooked) with 5% (v/w) seed culture of M. purpureus (Su et al., 2003) under solid state fermentation and inoculated rice was incubated at 30°C [Adolf Khuner Therm-Lab, Birfelden (Basel), Switzerland] for 14 days in a slanting position with intermittent shaking. After fermentation, RMR was dried at 45–50° C for 24 h and then powdered. The powdered RMR was used for the experiments.
M. purpureus Bioactive Molecules
Pigments
The pigments were extracted with 95% ethanol at 30°C for 60 min on rotary shaker (150 rpm). After filtration, the pigments were quantified by measuring the optical density (OD) with appropriate dilution. The OD was measured at 375, 475, and 500 nm for yellow, orange, and red pigments, respectively. Pigment yield was calculated as OD Units using following formula.
Statins
RMR powder added to 75% ethanol (1:10, w/v) was kept at 30°C for 1 h on rotary shaker [Adolf KhunerTherm-Lab, Birfelden (Basel), Switzerland] at 180 rpm. After centrifuging for 10 min at 10,000 rpm, samples were filtered through a 0.45 μm millex-LH (Millipore Corp., Bedford, MA 01730) filter before injection. The identification of statins were carried out by HPLC equipped with linear isocratic system, using Shimadzu Liquid Chromatograph LC-10A (Shimadzu, Japan) fitted with water's ODS (5 μm, 25 cm × 4.6 mm i.d.) column with a UV detector (237 nm) eluted with acetonitrile:water (72:28, v/v) at a elution of 1.0 ml/min maintained column temperature at 30°C with the injection volume of 20 μl. Statins were identified by comparing with the retention time of standards.
Fatty Acids
Total lipid extraction from dry biomass and analysis of fatty acids was carried out according to the method (Somashekar et al., 2003). The fatty acids were identified with the retention time of authentic fatty acid standards from Sigma. Fatty acids were identified by converting the fatty acids to fatty acid methyl esters (FAMEs) as described earlier (Kate, 1964) with slight modification. FAMEs were separated by Shimadzu GC-15A equipped with a DEGS packed column with isothermal temperature with flame ionization detector. The injector temperature was set at 220°C, detector temperature was maintained at 230°C and column temperature at 180°C. N2 was used as carrier gas at a flow rate of 40 ml min−1.
Phytosterol
About 10–20 mg of extracted lipid was subjected to saponification by methanolic KOH (6%, w/v) and refluxed for 2 h at 80°C in boiling water bath, cooled and diluted with 1 vol. of water. Total sterol was repeatedly extracted 3 times with hexane. The total extract was pooled together and evaporated to dryness. The final residue was dissolved in 1 ml chloroform and estimated by Liberman-Burchard method (Syed Mubbasher et al., 2003). Briefly to 200 μl sample, 800 μl chloroform and 2 ml of the Liberman-Burchard reagent (5 ml of H2SO4 dissolved in 10 ml acetic anhydride) was added in a graduated test tube. The final volume was made up to 7 ml with chloroform and incubated in dark for 15 min. The absorbance of resultant solution was determined spectrophotometrically at 640 nm (Shimadzu 160 UV A). Sitosterol was used as standard for comparison.
Antioxidant Activity
Antioxidant activity of M. purpureus extract was determined by estimating DPPH radical scavenging activity, inhibition of ascorbate autoxidation, lipid peroxidation, and reducing activity. All the experiments were carried out in triplicates by maintaining appropriate blanks and controls. Butylated hydroxyl anisole was used standard as for comparison.
The DPPH scavenging activity (Blois, 1958) of was measured accordingly (Moon and Terao, 1998). The inhibition of ascorbate autoxidation was determined according to Mishra and Kovachich (1984). Lipid peroxidation inhibitory activity was measured according to Kulkarni et al. (2004). The reducing power was determined according to Oyaizu (1986). Percentage of antioxidant activity was determined using the standard formula.
In vivo Hypolipidemic Action of RMR
Experimental Animals
Sixty four male albino rats (CFT-Wistar strain) weighing 150–180 g were used as experimental animals. The rats were bred in animal house facility at Central Food Technological Research Institute, Mysore, India. The experimental protocol was approved by the Institute animal ethical committee. Animals were grouped by randomized design on a body weight basis and were kept (12 h light/dark cycles, RH 60% and at 25 ± 2°C) in stainless steel cages for 14 week. Four animals in control group were sacrificed immediately to analyze cholesterol and triacylglycerol levels in serum and liver and established a base line lipid profile data.
Treatment Groups and Dosages
American Institute of Nutrition (AIN)-76 diet formulation was followed for the preparation of experimental diets with slight modification. Animals were divided into six groups of 10 animals each. The control group rats (I group) were fed a normal diet of AIN-76 formulation. Group II were fed with high fat diet (HFD, cholesterol). Group III to V rats were fed with HFD supplemented with 8, 12, and 16% of RMR powder, while Group VI rats were fed with HFD supplemented with lovastatin (0.08 g/kg). Appropriate proportion of cholesterol, bile salts, RMR and standard lovastatin were supplemented to the control diet at the expense of corn starch. Cholesterol (1.0%) and bile acids (0.15%) were added to the diet to induce hyperlipidemia in rats. Diets were prepared every week and stored at 4°C. Throughout the study (14 weeks), the animals had free access to food and water ad libitum. The animals were observed thoroughly for the onset of any signs of toxicity. Daily food intake and gain in weekly body weight gained was monitored. At the end of the experimental period (7th and 14th week), overnight fasted rats were sacrificed. Blood samples were collected by cardiac puncture and serum separated by centrifugation at 6,000 g. Organs like liver, heart, brain, kidney, adipose, lungs, spleen and testis were excised, blotted, weighed and stored at −20°C till they were processed. A portion of the liver was stored in 10% neutral formalin and processed for histological examination.
Serum Cholesterol and Triacylglycerol Analysis
Serum cholesterol and triacylglycerol were measured using commercial enzymatic kits, respectively. HDL cholesterol was estimated after precipitation of LDL with heparin-MnCl2 reagent (Warnick and Albers, 1978).
Liver Cholesterol and Triacylglycerol Analysis
Triacylglycerol in liver was estimated by the method of Fletcher (1968). Total lipids from liver after extraction was estimated by the method of Folch et al. (1957). Total cholesterol content of liver was estimated by the method of Searcy and Bergquist (1960).
3-Hydroxy-3-Methylglutaryl-CoA Reductase Activity
Preparation of microsomes and HMG CoA reductase activity was assayed according to the method of Hulcher and Oleson (1973). The reaction mixture was containing 0.5–1.0 mg of microsomal protein, 150 nmoles of HMG-CoA, 2 μmoles of NADPH and 0.8 ml of 0.1 M triethanolamine in 0.02 M EDTA buffer (pH 7.4) without dithiothreitol. The final volume was made up to1 ml and kept for incubation at 37°C for 30 min (Hulcher and Oleson, 1973).
Statistical Analysis
The statistical analyses of experimental data e were subjected to one-way analysis of variance (ANOVA) using Duncan's test. Statistical significance was considered when P < 0.05. Statistical analysis were performed by software SPSS, release 9.0 (SPSS, Inc.).
Results
Identification of Bioactive Molecules
Major bioactive molecules were identified using various techniques like HPLC, GCMS and UV visible spectroscopy.
Pigments
Production of more red pigment (202.8 OD units) was observed compared to orange (183.4 OD units) and yellow pigments (120.3 OD units). These results indicated the production of three major colored pigment like yellow, orange and red (Table 1).
Statins
Qualitative and Quantitative Analysis by TLC and HPLC
Statins (lovastatin and pravastatin) from M. purpureus RMR, extracted with ethanol, were identified after TLC and HPLC analysis. For TLC, pravastatin and lovastatin standards obtained from sigma were used. After performing TLC, Rf values were determined in relation to the standards. Standards showed an Rf values of 0.52 and 0.39 for pravastatin and lovastatin, respectively. Spots appearing at this Rf, with the ethanol extract of M. purpureus identified (Figure 1A) the presence of two statins. Hence for conformation, HPLC was carried out.
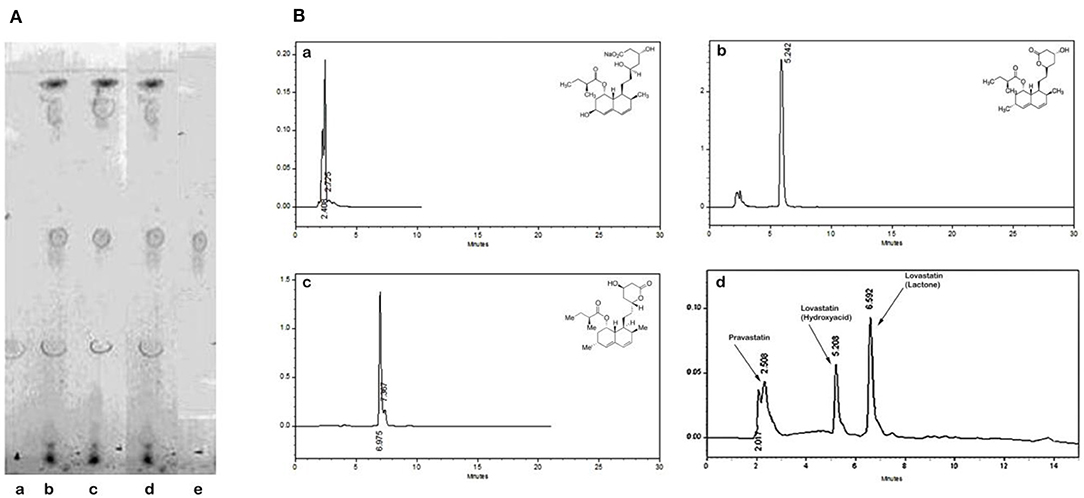
Figure 1. Comparison of chromatograms of pravastatin (a), lovastatin hydroxyacid form (b), lovastatin lactone form (c), and RMR extract (d). (A) TLC. (a) Standard lovastatin, (b-d) RMR, e-Standard pravastatin. (B) HPLC. (a) Elution profile of standard pravastatin; (b) Elution profile of standard hydroxyl acid form of lovastatin; (c) Elution profile of standard lactone form of lovastatin; (d) Elution profile of statins from RMR extract.
Further the HPLC chromatograms confirmed the presence of lovastatin (hydroxyacid and lactone form) and pravastatin in RMR extract. The retention time (Rt) was 5.22 and 6.97 min for hydroxyacid and lactone form of lovastatin, respectively. The pravastatin showed elution time at 2.40 min when compared with standard (Figure 1B). HPLC data shows, the lactone form was the major form of lovastatin.
These results indicated the biosynthesis of lovasatin (hydroxyacid and lactone form) and pravastatin in RMR.
Fatty Acids
Unsaturated fatty acids such as oleic, linoleic, and linolenic acids also help to reduce serum lipids (Ma et al., 2000). In order to quantitate useful fatty acids in M. purpureus RMR, the profile were estimated by GC. Each of the fatty acid was identified using the retention time with reference to that of the standard. Palmitic, stearic, oleic, linoleic and linolenic acids were identified as the major fatty acids in M. purpureus RMR (Figure 2). RMR had higher concentrations of unsaturated fatty acids (linoleic and oleic acids) than saturated fatty acids (palmitic and stearic acid). Production of linolenic acid (0.2 ± 0.66%) concentration was very less compared to other fatty acids like oleic acid (27.90 ± 0.90%) and Linoleic acid (24.60 ± 0.66%).
Antioxidant Activity
A compound can exert its antioxidant activity by scavenging radicals, decomposing peroxides, or chelating metal ions. The antioxidant activity of crude methanol extract was carried out by in vitro assays for DPPH radical scavenging, inhibition of ascorbate autoxidation, reducing activity, and lipid peroxidation (Table 2). All the experiments were carried out in triplicates by maintaining appropriate blanks and controls.
The phenolic content of methanol extract of RMR was 141.32 ± 0.01 μg ml−1 and expressed as mg gallic acid equivalent/100 g dry weight. The IC50 value to scavenge DPPH radical for methanol extract, and BHA were found to be 100.78, 50.01 and 18.96 μg ml−1, respectively (Table 2).
Inhibition of ascorbate autoxidation and reducing activity by crude methanol extract of RMR was found to be more compared to BHA. The BHA showed lipid peroxidation inhibition activity of 38.02 ± 1.53 μg/ml (Table 2).
In vivo Hypolipidemic Action of RMR
Effect of RMR on Food Intake and Body Weight Gain in Rats
The normal, high fat and RMR supplemented diets were prepared. Cholesterol and bile acids were added to diet at 1.0 and 0.15% respectively to induce hypercholesterolemia in rats. Effects of RMR supplementation to HCD on food intake and body weight gain were presented in Tables 3, 4. No significant differences in average food intake were observed between control and RMR fed groups. RMR fed groups results with lower average body weight gain compared to control group at initial periods of feeding (1–7 weeks), however, no significant change in body weight gain was observed during 8–14 weeks of feeding. There were no marked differences in mean relative organ weights of various vital organs except in liver of HCD and RMR fed groups compared to that of control groups. Changes in liver weight are ascribed to high fat diet. The average weight of the liver of HCD fed rats increased by 14.7% over that control diet and addition of RMR reduced liver weights ranges from 10.46 to 8.27 g in rats fed diets containing 8, 12 and 16 % RMR, respectively (Tables 5, 6). The results indicate that the daily food intake and gain in body weight of rats were normal and did not differ among various experimental groups.
Effect of RMR on Serum and Liver TC and TG Levels
Change in serum and liver TC and TG levels of rats fed HCD and HCD supplemented with different concentration of RMR were given in Table 7. High cholesterol diet fed group showed 2.26 and 1.97 fold increase in serum and liver cholesterol levels. Similarly high cholesterol diet induced 1.54 and 1.97 fold increase in serum and liver triacylglycerols, respectively. RMR supplementation in high cholesterol diet resulted in a dose dependent decrease in serum and liver cholesterol and triacylglycerol levels compared to high cholesterol fed group at 7 and 14 weeks. The cholesterol and triacylglycerol levels in serum were significantly decreased by 44.82 and 45.30%, respectively, in rats fed with 16% RMR for 7 weeks. Similarly, 48.45 and 42.08% decrease in liver cholesterol and triacylglycerols were observed in 16% RMR fed group for 7 weeks compared to high cholesterol fed group. However, at the end of 14 week, the decrease observed in serum and liver cholesterol and triacylglycerol levels in RMR fed groups were not relatively higher than 7 weeks feeding of RMR.
Effect of RMR on Serum and Liver HDL and LDL-C Levels
The effects of various concentration of RMR on serum HDL- and LDL-C levels are presented in Table 8. High cholesterol diet induced 3.62 fold increases in LDL-C level and 4.12 fold in LDL/HDL ratio compared to control animals. LDL-C level was increased by 5.14 fold in high cholesterol diet group. Supplementation of 16% RMR in the diet for 14 weeks significantly decreased LDL-C by 3.37 fold compared to high cholesterol control group.
3-Hydroxy-3-methylglutaryl-CoA Reductase Activity
3-Hydroxy-3-methylglutaryl coenzyme A reductase is generally considered to catalyze the rate-limiting reaction in cholesterol biosynthesis. High cholesterol feeding decreased HMG-CoA reductase activity by 56.45 % in control rats (Figure 3). RMR supplementation in high cholesterol diet resulted in a dose dependent inhibition of HMG-CoA reductase activity in liver compared to high cholesterol HCD fed group at 14 weeks. RMR feeding reduced HMG-CoA reductase activity by 22–54% compared HCD fed rats.
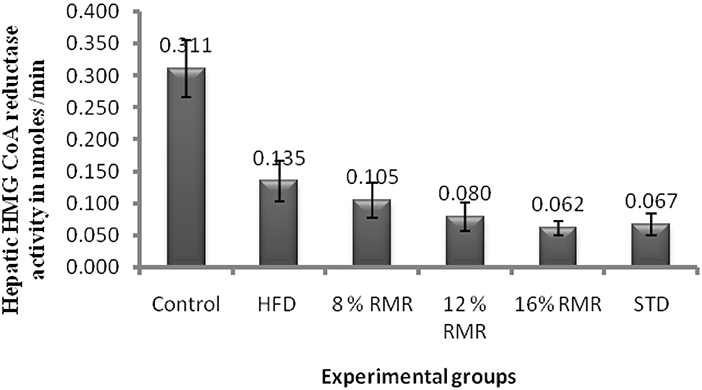
Figure 3. Hepatic 3-hydroxy-3-methylglutaryl (HMG-CoA) reductase activity in rats fed with control and experimental diets for 14 weeks. Eight, twelve, and sixteen percentage fermented rice contains 4, 6, and 8 mg/g of lovastatin. Mean values within each group are significantly different Duncan's test at p < 0.05.
No significant damage in liver tissue of RMR groups was observed in microscopic examination compared to control group and HCD group (Figure 4). Liver sections of group III, IV, V supplemented with RMR showed relatively moderate cholesterol infiltration compared to that of group II fed with HCD. Group VI fed with lovastatin showed fatty infiltration similar to that of RMR fed groups.
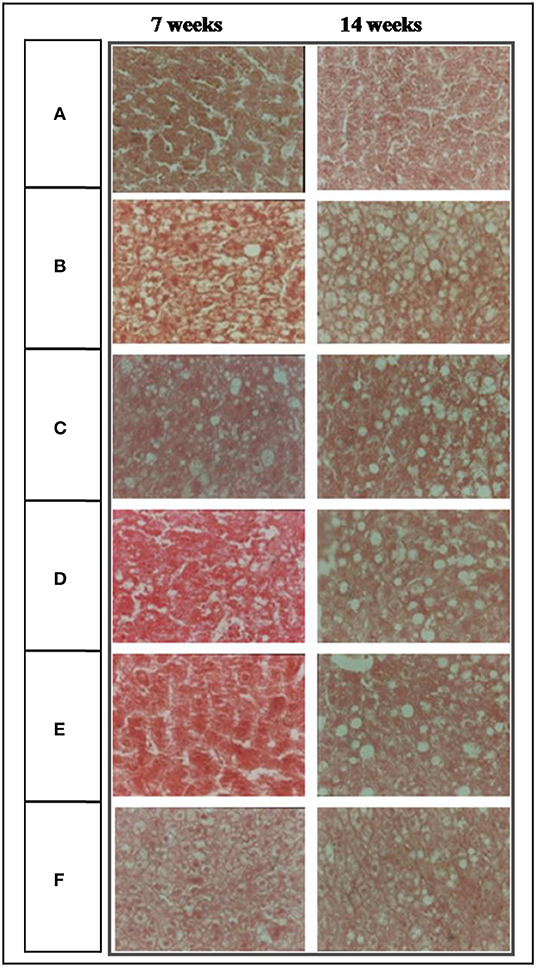
Figure 4. Photomicrographs (400X) of liver of experimental rats. (A) Normal diet, (B) high fat diet (HFD), (C) (HFD +8% RMR), (D) (HFD +12% RMR), (E) HFD +16% RMR, and (F) HFD +0.08 mg lovastatin.
Discussion
M. purpureus MTCC 410 RMR produces polyketides, statins (hydroxyl and lactone form of lovastatin and pravastatin), sterols and significant amount of saturated (palmitic and stearic) and unsaturated fatty acids (oleic, linoleic, and linolenic) by solid-state fermentation. Production of higher concentration of metabolites in solid-state fermentation can be attributed to better hyphal adhesion and invasion into the microstructure of the rice grain (Johns and Stuart, 1991). The hyphal mode of fungal growth is also tolerant to low water activity and high osmotic pressure conditions. Thus, the conditions apparently made the fungus competitive for efficient bioconversion of solid substrates to produce these products of interest in higher concentration.
Moreover, plant phytosterols, saponins, fungal metabolites such as mevinolin, monacolin K are being investigated for their antihyperlipidemia and antiatherosclerotic properties (Kroon et al., 1982; Harwood et al., 1993; Ikeda and Sugano, 1998). Monacolin K, the secondary metabolite produced during fermentation of rice by Monascus spp. has been shown to reduce cholesterol by inhibiting HMG-CoA reductase enzyme in liver. But the safety of RMR as a dietary supplement to reduce high cholesterol was a concern due to the presence of high concentrations of a mycotoxin- citrinin in different commercial Monascus products.
Hypercholesterolemia is considered as the major risk factors for atherosclerosis and cardiovascular diseases. Management of hyperlipidemia with healthy less atherogenic diet, less stressful life style, and physical activity is successful for certain patients, but a majority of patients need therapeutical intervention to effectively lower hyperlipidemia. Statins that inhibit HMG CoA reductase are widely used drugs to control hypercholesterolemia (Alberts, 1990; Blankenhorn et al., 1993).
In this study, supplementation of RMR of M. purpureus MTCC 410 to rats at a dose of 16% for 7 weeks showed, 44.82 and 48.45% reduction in serum and liver cholesterol levels; 45.30 and 42.08% reduction in serum and liver triacylglycerol, respectively, compared to HCD fed group. LDL-C levels decreased by 66.81% while HDL-C increased by 46.63% in serum of 16% RMR fed rats for 7 weeks. RMR feeding to hypercholesterolemia rats resulted in 22–54% inhibition of HMG-CoA reductase activity in liver. Thus, RMR is very effective in reducing cholesterol and triacylglycerol and LDL-C levels in hyperlipidemia rats. The combination of dietary sterols with statin drugs was also suggested for lowering cholesterol more effectively than statin alone (Plat and Mensink, 2001). The bioactive molecules sterols and statins identified in extract were attributed to the lipid lowering activity. The RMR been developed as a health food in human dietary supplements due to its functional properties like lowering cholesterol level and blood pressure. Further, RMR reduced liver weight and fatty infiltration, suggesting inhibition of lipids/fats deposition in liver. These findings are in concurrence with reported decrease in liver weight, lipid deposition in liver and atheroma in aorta of rabbits fed with Monascus purpureus (Li et al., 1998). While the clinical trials with Monascus spp. as dietary supplement in human subjects showed an effective reduction in serum cholesterol and triacylglycerol with mild side effects (Wang et al., 1997).
Functional food product marketed in the United States as CholestinTM by Pharmanex Inc., Simi Valley, CA (Wang et al., 1997). It is reported to contain many nutritional components including phytosterols, saponin, sapogenin, unsaturated fatty acids, isoflavones, trace elements zinc, selenium, and HMG-CoA reductase inhibitor (Wang et al., 1997). The exact mechanism by which RMR reduces serum cholesterol and triacylglycerols is not completely understood, because many Monascus species could synthesize small quantity (<500 mg Kg−1) of monocolin K and only monacolin K cannot be accounted for hypolipidemic effect of Monascus (Chang et al., 2002; Casas-López et al., 2003). Therefore, Monascus species which can produce high monacolin K are being developed to apply as functional food. It is believed that, atherosclerotic index (ratio of non-HDL-cholesterol to HDL-cholesterol) is important risk factor for atherosclerosis. Our data clearly demonstrate that RMR significantly decreased the ratio, even in the lower dose group. The inhibition of HMG-CoA reductase by other forms of monacolins like, monacolin J, L, M, X, and their hydroxy acid form, as well as dehydromonacolin (MV2 and K), dihydromonacolin (MV and L), compactin, 3α-hydroxy-3,5-dihydromonacolin L are also accounted for the reduced cholesterol synthesis and anti-atherogenic effect of RMR (Li et al., 2004; Dhale et al., 2007a,b). Another risk factor for atherosclerosis is lower level of HDL-cholesterol. Whereas, feeding of RMR increased serum HDL-C, even in the lower dose group to increase HDL-C significantly. The HCD diet induced an increase in plasma and liver peroxides (malnoldehyde, lipid hydroperoxide and conjugated dienes) which may indicate elevated oxidative stress in the body. Monacolins, known as statins, isolated from Monascus spp. were found to protect body organs against oxidation of LDL and inhibit HMG-CoA reductase (Istvan and Deisenhofer, 2001). The statins play important role in atherosclerosis as antioxidant by preventing the oxidation of LDL during oxidative stress. Cholesterol diet induces oxidative stress by conceding antioxidant molecules and antioxidant enzymes. The statins and supplementation of RMR containing statin, pigment and other secondary metabolites effectively reduced the oxidative stress by alleviating antioxidant molecules and enzymes in the liver of hypercholesterolemic rats (Mohan-Kumari et al., 2011a,b). The coronary heart disease (CHD) do not always have elevated levels of LDL but, the normal LDL levels with high levels of stress are more prone to CHD (Rosenson, 2004; Mohan-Kumari et al., 2011a). The increased oxidation of serum lipid and LDL-cholesterol in serum and its entry into artery leads to the formation of atherosclerotic lesion. The formation of atherosclerotic plaque in macrophages due to foam cells is prone to damage (Osterud and Bjorklid, 2003; Rosenson, 2004).
The feeding of RMR reduced such oxidative stress by modulating the antioxidant enzymes and molecules (Mohan-Kumari et al., 2011b). The data suggested RMR can lower or slowing down oxidative stress related atherosclerosis pathological process. However, the authentic comparison of the secondary metabolites of RMR is in their effects on severity of cholesterol-induced atherosclerosis. The cholesterol lowering and anti-atherogenic effect of RMR in this study is comparable to therapeutic drug, lovastatin. The monacolins also increase the activity of LDL-receptors and reduce total cholesterol and LDL levels in the body (Pitman et al., 1998). Thus, the significant inhibition of HMG Co-A reductase and anti-atherogenic effect of RMR observed in this study can be attributed to synergistic effect of monacolins (statins) which are suppressing cholesterol synthesis.
Commercial RMR products of Monascus contain high levels of citrinin, a mycotoxin affecting liver and kidney. Hence, the safety of RMR is of big concern to use it as a food supplement. Our earlier work, acute and sub-acute toxicological studies on RMR of M. purpureus MTCC 410 did not show any adverse effect in rats (Mohan-Kumari et al., 2009) and produced low levels of 1.427 mg kg−1 of citrinin. RMR supplementation in HCD did not induce any histopathological alterations in liver but moderately reduced fatty infiltration in liver compared to HCD fed group.
Conclusions
In conclusion, our data suggest that M. purpureus RMR can be considered as a potent anti-atherogenic food supplement with no adverse effects and possess health benefits related to regulation of blood lipids by inhibiting the HMG CoA reductase. The RMR significantly decreased LDL-C level in serum. The HDL value and HDL-C to LDL-C ratio were increased in rats fed on RMR.
Data Availability Statement
The original contributions presented in the study are included in the article/supplementary material, further inquiries can be directed to the corresponding author/s.
Ethics Statement
The animal study was reviewed and approved by Institute Animal Ethics Committee, Central Food Technological Research Institute (CSIR), Mysore.
Author Contributions
HPMK conceptualized, executed the experiments, data analysis, and wrote the paper. KAN and GV designed the experiment and reviewed the paper. KN carried out histopathology. All the authors read and approved the final manuscript.
Funding
HPMK acknowledges Council of Scientific and Industrial Research, New Delhi, India and University of Mysore for supporting research through the award of senior research fellowship. CSIR, New Delhi, University of Mysore. This work was partially supported by Minstry of Food Processing India, New Delhi (No. Q/2/2020-R&D).
Conflict of Interest
The authors declare that the research was conducted in the absence of any commercial or financial relationships that could be construed as a potential conflict of interest.
Acknowledgments
We thank Director, CSIR-CFTRI, Mysore, India for encouragement and providing the facility.
References
Alberts, A. W. (1990). Lovastatin and simvastatin inhibitors of HMG-CoA and cholesterol biosynthesis. Cardiology 77, 12–21. doi: 10.1159/000174688
Alberts, A. W., Chen, J., Kuron, G., Hunt, V., Huff, J., Hoffman, C., et al. (1980). Mevinolin: a highly potent competitive inhibitor of hydroxymethylglutaryl-coenzyme A reductase and a cholesterol lowering agent. Proc. Natl. Acad. Sci. U.S.A. 77, 3957–3961. doi: 10.1073/pnas.77.7.3957
Aniya, Y., Ohtani, I., Higa, T., Miyagi, C., Gibo, H., Shimabukuro, M., et al. (2000). Dimerumic acid as an antioxidant of the mold: Monascus anka. Free Rad. Biol. Med. 28, 999–1004. doi: 10.1016/S0891-5849(00)00188-X
Blankenhorn, D. H., Azen, S. P., Kramsch, D. M., Mack, W. J., Cashin-Hemphill, L., Hodis, H. N., et al. (1993). Coronary angiographic changes with lovastatin therapy: the monitored atherosclerosis regression study (MARS). Ann. Intern. Med. 119, 969–976. doi: 10.7326/0003-4819-119-10-199311150-00002
Blois, M. S. (1958). Antioxidant determinations by the use of a stable free radical. Nature 181, 1199–1200. doi: 10.1038/1811199a0
Casas-López, J. L., Sánchez-Pérez, J. A., Fernández-Sevilla, J. M., Acién-Fernández, F. G., Molina-Grima, E., and Chisti, Y. (2003). Production of lovastatin by Aspergillus terreus: effects of the C: N ratio and the principal nutrients on growth and metabolite production. Enzyme Microb. Technol. 33, 270–277. doi: 10.1016/S0141-0229(03)00130-3
Chang, Y. N., Lin, Y. C., Lee, C. C., Liu, B. L., and Tzeng, Y. M. (2002). Effect of rice-glycerol complex medium on the production of lovastatin by Monascus ruber. Folia Microbiol. 47, 677–684. doi: 10.1007/BF02818671
Dhale, M. A., Divakar, S., Umesh-Kumar, S., and Vijayalakshmi, G. (2007a). Isolation and characterization of dihydromonacolin-MV from Monascus purpureus for antioxidant properties. Appl. Microbiol. Biotechnol. 73, 1197–1202. doi: 10.1007/s00253-006-0578-0
Dhale, M. A., Divakar, S., Umesh-Kumar, S., and Vijayalakshmi, G. (2007b). Characterization of dehydromonacolin-MV2 from Monascus purpureus mutant. J. Appl. Microbial. 130, 2168–2173. doi: 10.1111/j.1365-2672.2007.03457.x
Endo, A. (1979a). Monacolin K, a new hypocholesterolemic agent produced by a Monascus species. J. Antibiot. 32, 852–854. doi: 10.7164/antibiotics.32.852
Endo, A. (1979b). Monacolin K, a new hypocholesterolemic agent produced by Monascus species. J. Antibiot. 32, 852–854.
Endo, A., Hasumi, K., Nakamura, T., Kunishima, M., and Masuda, M. (1985). Dihydromonacolin-L and monacolin-X, new metabolites those inhibit cholesterol-biosynthesis. J. Antibiot. 38, 321–327. doi: 10.7164/antibiotics.38.321
Feng, Y., Shao, Y., and Chen, F. (2012). Monascus pigments. Appl. Microbiol. Biotechnol. 96, 1421–1440. doi: 10.1007/s00253-012-4504-3
Fletcher, M. J. (1968). Colorimetric method for estimating serum triglycerides. Clin. Chem. Acta 22, 303–307. doi: 10.1016/0009-8981(68)90041-7
Folch, J., Lees, M., and Stanley, G. H. S. (1957). A simple method for the isolation and purification of total lipids from animal tissues. J. Biol. Chem. 226, 497–509. doi: 10.1016/S0021-9258(18)64849-5
Hachem, R., Assemat, G., Balayssac, S., Martins-Froment, N., Gilard, V., Martino, R., et al. (2020). Comparative chemical profiling and monacolins quantification in red yeast rice dietary supplements by 1H-NMR and UHPLC-DAD-MS. Molecules 25:317. doi: 10.3390/molecules25020317
Hansson, G. K. (2005). Inflammation, atherosclerosis, and coronary artery disease. N. Engl. J. Med. 352, 1685–1695. doi: 10.1056/NEJMra043430
Harwood, H. J., Chandler, C. E., Pellarin, L. D., Bangerter, F. W., Wilkins, R. W., Long, C. A., et al. (1993). Pharmacologic consequences of cholesterol absorption inhibition: alteration in cholesterol metabolism and reduction in plasma cholesterol concentration induced by the synthetic saponin b-tigogenin cellobioside (CP-88818; tiqueside). J. Lipid Res. 34, 377–395. doi: 10.1016/S0022-2275(20)40730-8
Hulcher, F. H., and Oleson, W. H. (1973). Simplified spectrophotometric assay for microsomal 3-hydroxy-3-methylglutaryl CoA reductase by measurement of coenzyme. J. Lipid Res. 14, 625–631. doi: 10.1016/S0022-2275(20)36843-7
Ikeda, I., and Sugano, M. (1998). Inhibition of cholesterol absorption by plant sterols for mass intervention. Curr. Opin. Lipidol. 9, 527–531. doi: 10.1097/00041433-199812000-00003
Istvan, E. S., and Deisenhofer, J. (2001). Structural mechanism of statin inhibition of HMG CoA reductase. Science 29, 1160–0064. doi: 10.1126/science.1059344
Johns, M. R., and Stuart, D. M. (1991). Production of pigments by Monascus purpureus in soild culture. J. Ind. Microbial. 8, 23–28. doi: 10.1007/BF01575587
Kroon, P. A., Hand, K. M., Huff, J. W., and Alberts, J. W. (1982). Effects of mevinolin on serum cholesterol levels of rabits with endogenous hypercholesterolemia. Atherosclerosis 44, 41–47. doi: 10.1016/0021-9150(82)90051-X
Kulkarni, A. P., Aradhya, M., and Divakar, S. (2004). Isolation and identification of a radical scavenging antioxidant- punicalgin from pith and carpellary membrane of pomegranate fruit. Food Chem. 87, 551–557. doi: 10.1016/j.foodchem.2004.01.006
Li, C. L., Zhu, Y., Wang, Y. Y., Zhu, J. S., Chang, J., and Kritchevsky, D. (1998). Monascus purpureus-fermented rice (red yeast rice): a natural food product that lowers blood cholesterol in animal models of hypercholesterolemia. Nutr. Res. 18, 71–81. doi: 10.1016/S0271-5317(97)00201-7
Li, Y.-G., Zhang, F., Wang, Z.-T., and Hua, Z.-B. (2004). Identification and chemical profiling of monacolins in red yeast rice using high-performance liquid chromatography with photodiode array detector and mass spectrometry. J. Pharm. Biomed. Anal. 35, 1101–1112. doi: 10.1016/j.jpba.2004.04.004
Lin, C. P., Lin, Y. L., Huang, P. H., Tsai, H. S., and Chen, Y. H. (2011). Inhibition of endothelial adhesion molecule expression by Monascus purpureus-fermented rice metabolites, monacolin K, ankaflavin, and monascin. J. Sci. Food Agric. 91, 1751–1758. doi: 10.1002/jsfa.4371
Ma, J., Li, Y., Ye, Q., Li, J., Hua, Y., Ju, D., et al. (2000). Constituents of red yeast rice, a traditional Chinese food and medicine. J. Agric. Food Chem. 48, 5220–5225. doi: 10.1021/jf000338c
Martinkova, L., Juzlova, P., Kren, V., Kucerouva, Z., Havlicek, V., Olsovsky, P., et al. (1999). Biological activities of oligoketide pigments of Monascus purpureus. Food Addit. Contam. 16, 15–24. doi: 10.1080/026520399284280
Mishra, O. P., and Kovachich, G. B. (1984). Inhibition of the auto-oxidation of ascorbic acid and norepinephrine by extracts of Clostridium butyrium, Megasphaera elsdelii and E. coli. Life Sci. 35, 849–854. doi: 10.1016/0024-3205(84)90410-7
Mohan-Kumari, H. P., Dhale, M. A., Gaonkar, V., and Keni, S. (2011a). Statins: 3-Hydroxy-3-methylglutaryl-CoA (HMG-CoA) reductase inhibitors demonstrate anti-atherosclerotic character due to their antioxidant capacity. Appl. Biochem. Biotechnol. 163, 215–222. doi: 10.1007/s12010-010-9031-z
Mohan-Kumari, H. P., Naidu, K. A., Dhale, M. A., and Vijayalakshmi, G. (2011b). Antioxidant effect of red mould rice (RMR) in hypercholesterolemic wistar male rats. Cell Biochem. Funct. 29, 597–602. doi: 10.1002/cbf.1793
Mohan-Kumari, H. P., Naidu, K. A., Vishwanatha, S., Narasimhamurthy, K., and Vijayalakshmi, G. (2009). Safety evaluation of Monascus purpureus red mould rice in albino rats. Food Chem. Toxicol. 47, 1739–1746. doi: 10.1016/j.fct.2009.04.038
Moon, J. H., and Terao, J. (1998). Antioxidant effect of caffeeic acid and dihydrocaffeeic acid in lard and human low-density lipoprotein. J. Agricult. Food Chem. 46, 5062–5065. doi: 10.1021/jf9805799
Osterud, B., and Bjorklid, E. (2003). Role of monocytes in atherogenesis. Physiol. Rev. 83, 1069–1112. doi: 10.1152/physrev.00005.2003
Oyaizu, M. (1986). Studies on products of browning reactions antioxidative activities of products of browning reaction prepared from glucosamine. Jpn. J. Nutr. 307–315. doi: 10.5264/eiyogakuzashi.44.307
Pitman, W. A., Osgood, D. P., Smith, D., Schaefer, E. J., and Ordovas, J. M. (1998). The effects of diet and lovastatin on regression of fatty streak lesions and on hepatic and intestinal mRNA levels for the LDL-C receptor and HMG-CoA reductase in F1B rats. Atherosclerosis 138, 43–52. doi: 10.1016/S0021-9150(97)00302-X
Plat, J., and Mensink, R. P. (2001). Effects of plant sterols and stanols on lipid metabolism and cardiovascular risk. Nutr. Metab. Cardiovasc. Dis. 11, 31–34.
Rosenson, R. S. (2004). Statins in atherosclerosis: lipid-lowering agents with antioxidant capabilities. Atherosclerosis 173, 1–12. doi: 10.1016/S0021-9150(03)00239-9
Searcy, R. L., and Bergquist, L. M. (1960). A new color reaction for quantification of serum cholesterol. Clin. Chem. Acta 5, 192–196. doi: 10.1016/0009-8981(60)90035-8
Somashekar, D., Venkateswaran, G., Sambaiah, K., and Lokesh, B. R. (2003). Effect of culture conditions on lipid and gamma linolenic acid production in mucoraceous fungi. Proc. Biochem. 38, 1719–1724. doi: 10.1016/S0032-9592(02)00258-3
Su, Y., Wang, J. J., Lin, T. T., and Pan, T. M. (2003). Production of the secondary metabolites c-aminobutyric acid and monacolin K by Monascus. J. Indust. Microbiol. Biotechnol. 30, 40–46. doi: 10.1007/s10295-002-0001-5
Syed Mubbasher, S., Imran, H., and Syed Dinawaz, A. G. (2003). Estimation of sterols in edible fats and oils. Pak. J. Nutr. 2, 178–181. doi: 10.3923/pjn.2003.178.181
Wang, J., Lu, Z., and Chi, J. (1997). Multicenter clinical trial of the serum lipid-lowering effects of a Monascus purpureus (red yeast) rice preparation from traditional Chinese medicine. Curr. Ther. Res. 58, 964–978. doi: 10.1016/S0011-393X(97)80063-X
Keywords: antioxidant, HMG-CoA reductase, Monascus, pigment, anti-atherogenic
Citation: Mohankumari HP, Naidu KA, Narasimhamurthy K and Vijayalakshmi G (2021) Bioactive Pigments of Monascus purpureus Attributed to Antioxidant, HMG-CoA Reductase Inhibition and Anti-atherogenic Functions. Front. Sustain. Food Syst. 5:590427. doi: 10.3389/fsufs.2021.590427
Received: 01 August 2020; Accepted: 13 January 2021;
Published: 15 February 2021.
Edited by:
Lourdes Morales-Oyervides, Universidad Autónoma de Coahuila, MexicoReviewed by:
Guadalupe Virginia Nevárez-Moorillón, Autonomous University of Chihuahua, MexicoRangaswamy Lakshminarayana, Bangalore University, India
Copyright © 2021 Mohankumari, Naidu, Narasimhamurthy and Vijayalakshmi. This is an open-access article distributed under the terms of the Creative Commons Attribution License (CC BY). The use, distribution or reproduction in other forums is permitted, provided the original author(s) and the copyright owner(s) are credited and that the original publication in this journal is cited, in accordance with accepted academic practice. No use, distribution or reproduction is permitted which does not comply with these terms.
*Correspondence: G. Vijayalakshmi, vij19_99@yahoo.com; H. P. Mohankumari, mohankumari@cftri.res.in