- 1School for Environment and Sustainability, University of Michigan, Ann Arbor, MI, United States
- 2Smithsonian Migratory Bird Center, National Zoological Garden, Washington, DC, United States
- 3Departamento de Agricultura, Sociedad y Ambiente, El Colegio de la Frontera Sur, Tapachula, Mexico
- 4Department of Environmental Studies, University of California, Santa Cruz, Santa Cruz, CA, United States
- 5Department of Biology, Lake Forest College, Lake Forest, IL, United States
Volant vertebrate insectivores, including birds and bats, can be important regulators of herbivores in forests and agro-ecosystems. Their effects can be realized directly through predation and indirectly via intraguild predation. This paper examines data from bird and bat exclosures in coffee farms in Chiapas, Mexico in order to determine their effect on herbivores. Arthropods were sampled in 32 exclosures (with 10 coffee plants in each) and their paired controls three times during 6 months. After 3 months, herbivore and spider abundance increased, underscoring the importance of both intertrophic predation between volant vertebrate insectivores and herbivores and intraguild predation between volant vertebrate insectivores and spiders. After 6 months, herbivore abundance increased in the exclosures, which is indicative of a direct negative effect of birds and bats on herbivores. We suggest that intraguild predation is important in this food web and that seasonality may change the relative importance of intraguild vs. intertrophic predation. Results suggest a dissipating trophic cascade and echo the growing body of evidence that finds birds and bats are regulators of herbivores in agro-ecosystems.
Introduction
Defining trophic levels and outlining the connections between them has long been a fundamental goal in ecology. Much theoretical and empirical work has gone into understanding the factors controlling dominant pathways and the immense complexity in terrestrial food webs (Schoener, 1989; Spiller and Schoener, 1990; Hairston et al., 1997; McCann et al., 1998; Oksanen and Oksanen, 2000; Yodzis, 2000; Lambers and Dickerman, 2003; Stouffer et al., 2007). Inter-trophic consumption (i.e., feeding between trophic levels, as when a predator eats an herbivore) is undoubtedly important in structuring food webs, but is complicated by intraguild predation. Intraguild predation (i.e., organisms in the same trophic level consuming one another) is also very common (Gagnon et al., 2011). The feeding bias of predators—that is, whether they are primarily predating within or outside of their guild or trophic level—can have important consequences on food web structure.
The relative importance of inter-trophic vs. intra-guild predation has particular practical significance in managed ecosystems (Montoya et al., 2003). Natural enemies in the food web represent one of many tools for holistic pest management. But, for natural enemies to contribute to biocontrol, it is essential to understand the relative strength of intraguild predation and inter-trophic predation in the food web. For example, land managers might be interested in increasing the density of a predator in an effort to control pests. This intervention implicitly assumes that the primary effect of the predator on the pest is through direct inter-trophic predation. Alternatively, if the predator is also practicing intraguild predation and predating upon other predators of the pest, the managed predator could indirectly facilitate the pest by reducing the overall number of predators. The widespread benefits of holistic pest management are well-known (Lewis et al., 1997), but effective implementation requires an in-depth understanding of not just the pest and potential predator, but the food web within which they are embedded.
Volant vertebrate insectivores (VVIs), which includes birds and bats, have received a great deal of attention within the food web literature, in an effort to both conserve VVIs and ascertain their impact on managed ecosystems (Greenberg et al., 2000; Van Beal et al., 2003; Perfecto et al., 2004; Philpott et al., 2004, 2009; Borkhataria et al., 2006; Whelan et al., 2008; Johnson et al., 2010; Mooney et al., 2010; Böhm et al., 2011; Karp and Daily, 2014; Maas et al., 2016; Nyffeler et al., 2018). Early studies on the effects of birds on arthropods in temperate ecosystems concluded that birds exert little control over most arthropod groups (Holmes et al., 1979). If true and birds have little impact, there should be little net effect of VVIs on plants, since the positive effects of predation on herbivores should be counterbalanced by negative effects of intraguild predation depressing arthropod insectivore populations (Pejchar et al., 2018).
More recent work has shown that VVIs can be important in controlling arthropod populations (Greenberg et al., 2000; Van Beal et al., 2003; Perfecto et al., 2004; Philpott et al., 2004, 2009; Borkhataria et al., 2006; Whelan et al., 2008; Johnson et al., 2010; Mooney et al., 2010; Böhm et al., 2011; Karp and Daily, 2014; Maas et al., 2016; Nyffeler et al., 2018). Furthermore, studies in tropical forests and coffee agroforestry systems have found the effects of birds and bats (Kalka et al., 2008; Williams-Guillén et al., 2008; Morrison and Lindell, 2012), and of birds and lizards (Borkhataria et al., 2006), to be additive. These and other studies argue that VVIs serve an important function as regulators of arthropods, including herbivores, in forested and agricultural systems (Sekercioglu, 2006; Mooney et al., 2010; Maas et al., 2016). The food web structure can determine the net effect of VVIs on herbivory if VVIs are consuming predators or mesopredators, as demonstrated in cereal systems (Grass et al., 2017). Maintaining VVI populations could be important for pest control in coffee agro-ecosystems, but VVIs are also associated with a host of other ecosystem goods and services including pollination, climate regulation, and nutrient cycling and carbon sequestration (Jha et al., 2014).
Intraguild predation could be important in determining the effects of VVIs on arthropod herbivores not only if VVIs consume other predators, but also if arthropod predators consume one another. For example, while VVIs tend to prefer larger arthropods (Holmes et al., 1979; Marquis and Whelan, 1994; Greenberg et al., 2000; Van Beal et al., 2003; Gruner, 2004; Philpott et al., 2004), parasitoids are consumed mainly by spiders, especially web weavers (Polis and Hurd, 1995; Ibarra-Núñez et al., 2001). Thus, the feeding behavior of VVIs and arthropod predators could shape food web dynamics and pest control dynamics in the coffee system. However, the effects of intraguild predation among arthropods are less frequently considered in VVI exclosure experiments [for exceptions, see Martin et al. (2013), Maas et al. (2013), Karp and Daily (2014)].
In a further complication, in systems with marked seasonal shifts, the dominance of a trophic scheme and the importance of intraguild predation could change with the season (Pejchar et al., 2018). In the tropics, where this study was carried out, distinct dry and rainy seasons could have major impacts on life cycle dynamics, migration, and foraging strategies (Greenberg, 1995). In the dry season, ants are less abundant (Philpott et al., 2006), spiders are more abundant (Rendón et al., 2006) and migratory birds join the year-long residents, nearly doubling overall bird abundances (Greenberg et al., 1997). Shifting abundances of predators and prey can alter feeding habits and thus the importance of intraguild predation.
Arthropods can represent a significant threat to yields in agroforestry systems, including coffee agro-forestry systems. The coffee leaf miner (Leucoptera coffeella) and the coffee berry borer (Hypothenemus hampei) are the predominant threats to coffee yields with the former reducing photosynthetic potential of plants (Borkhataria et al., 2006) and leading to less fruit production and the latter boring into fruits, eating the seed and rendering the coffee unsaleable (Damon, 2000). In intensified coffee systems, pests are often managed with chemicals, to varying levels of success and with a number of negative externalities (Staver et al., 2001). In less intensively managed coffee systems, namely shaded agro-forestry systems, a diversified food web is relied upon to keep pest densities below a problematic threshold (Staver et al., 2001; Jha et al., 2014; Vandermeer et al., 2019).
We conducted this study in coffee agroecosystems of southern Mexico to assess the effect of VVIs on arthropod herbivores—an outcome dependent, in part, on the relative importance of inter-trophic consumption and intraguild predation—by experimentally excluding VVIs from target coffee plants. We explore three hypotheses regarding the general structure of the system: (1) direct inter-trophic interaction between the VVIs and herbivores, (2) intraguild predation between VVIs and arthropod predators, and (3) intraguild predation between VVIs and arthropod predators plus intraguild predation among arthropod predators (Figure 1). The exclusion of VVIs will result in an increase in herbivores for #1 and #3, and a decrease in herbivores for #2. In our system, the arthropod predators include beetles, lacewings, wasps, robber flies, ants and spiders, with spiders being the most common (Perfecto, unpublished data). The vertebrate predators include frogs, toads, lizards, bats and birds, with bats and birds being the most common, and the ones addressed in this study.
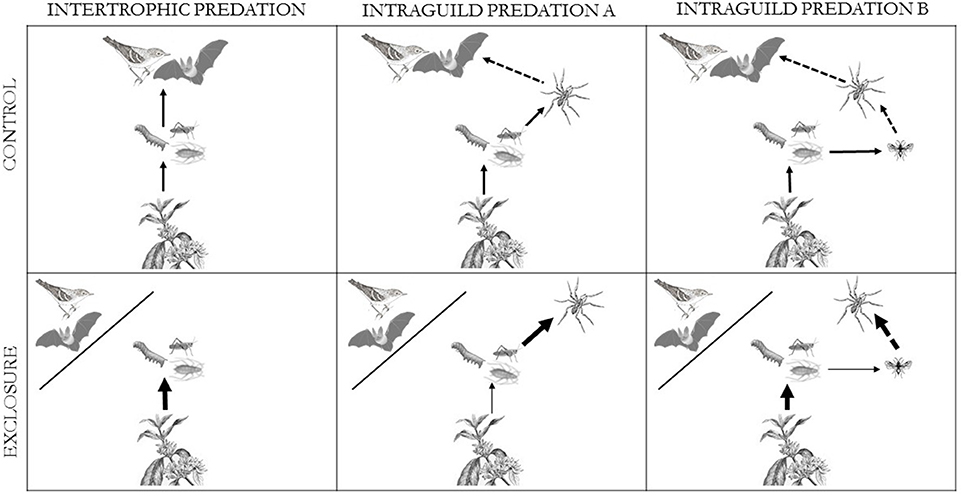
Figure 1. Conceptual figure illustrating potential trophic dynamics with (1) only direct inter-trophic consumption, (2) intraguild predation between VVIs and arthropod predators, and (3) intraguild predation between both VVIs and arthropod predators and among arthropod predators. Intraguild predation is indicated by a dashed arrows and inter-trophic interactions are denoted with solid arrows. Modified from Perfecto and Vandermeer (2015) with additional vector elements from Tracey Saxby (Integration and Application Network, ian.umces.edu/imagelibrary/).
In experimentally removing the effect of VVIs, we can infer the general food web structure based on the responses of herbivores and arthropod predators (particularly spiders). If inter-trophic consumption is controlling the net effect of VVIs on herbivores, we expect the exclusion of VVIs will lead to an increase in herbivores. If intraguild predation is more important, we expect an increase in arthropod predators with the removal of VVIs, followed by a concomitant decrease in herbivores due to increased predation by arthropod predators. Further, we expect that effects of intraguild predation will be greater in the dry season vs. the wet season, because spiders and birds are both more abundant during this time.
Methods
Working on four coffee farms in the Soconusco region of Chiapas, Mexico, we established 32 large bird/bat exclosures (ten in each of two farms and six in the other two farms). The farms included Finca Irlanda, Finca Hamburgo, Finca Bélgica, and Finca Belen. The last two farms were united under the single name [Finca Belén] right before we started working there, but continued to be managed in different ways. All farms are located on the Pacific side of the Sierra Madre mountain range, in a region dominated by coffee farms [Figure 2, see Perfecto et al. (2003), Philpott et al. (2006), Gordon et al. (2009)]. They are managed under a gradient of management styles from rustic to commercial polyculture management (Moguel and Toledo, 1999). These differences were not taken into account in the analysis, which is a conservative approach, and are discussed elsewhere (Perfecto et al., 2003; Philpott et al., 2006).
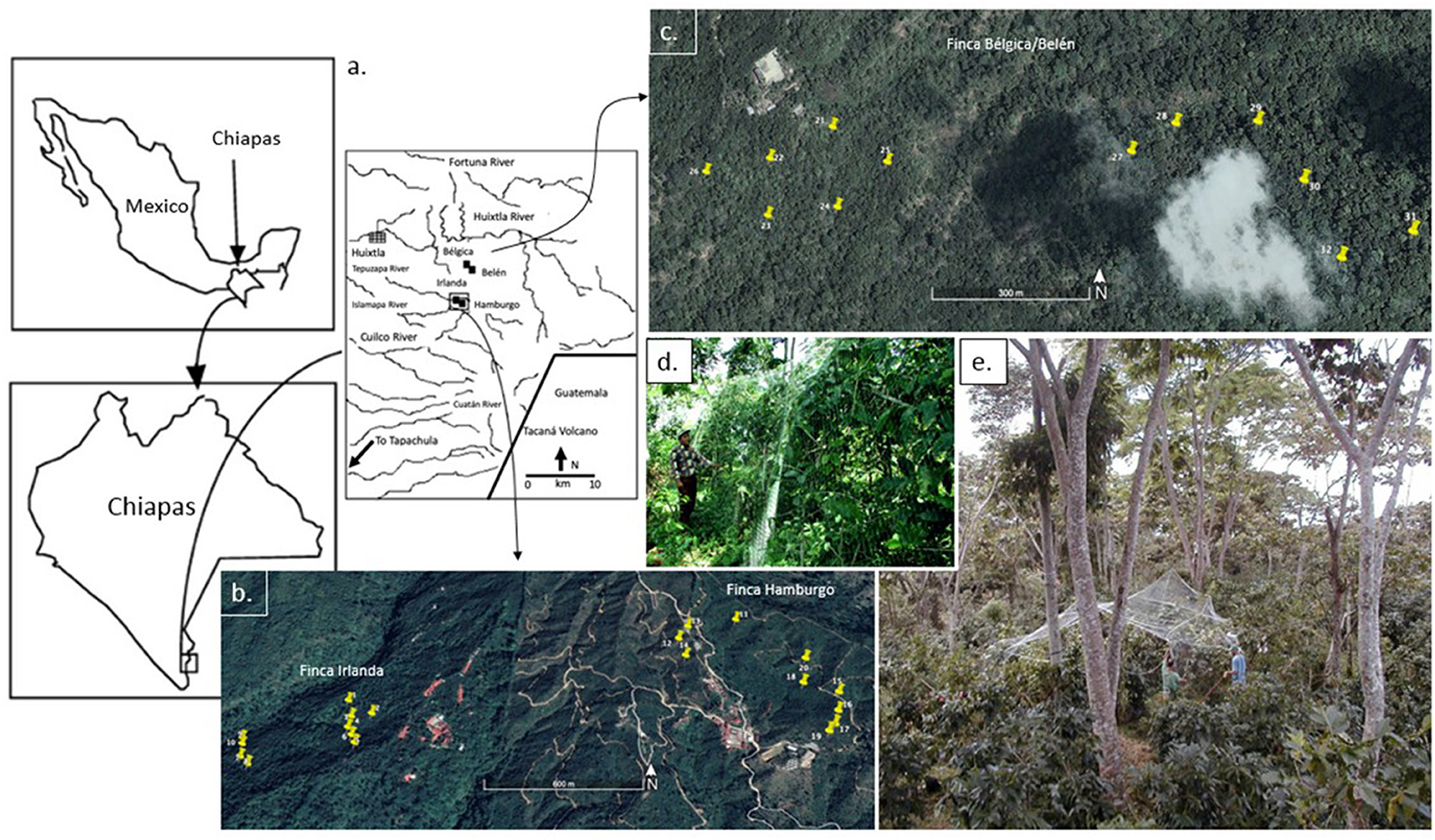
Figure 2. Location of study sites and exclosures; (a) regional map indicating study sites; (b) location of exclosures in the Finca Irlanda and Finca Hamburgo sites; (c) location of exclosures in the Finca Bélgica/Belén sites; (d) photo from outside one of the exclosures; (e) setting up an exclosure.
Exclosures were constructed of transparent monofilamentous nylon (5 cm2 mesh) fishing net. This mesh size is comparable to the mesh size used in other exclosure studies (e.g., Morrison and Lindell, 2012) and was chosen to exclude birds and bats, but permit most arthropods to pass through the cages (Figures 2d,e). They were established in November of 2000, and left for the duration of the experiment. Each exclosure enclosed 10 coffee plants [with the exception of five exclosures that enclosed seven (3), eight (1), and nine (1) plants] and each was ~10 m long, 5 m wide, and 3 m high (Figure 2e). The same number of control plants were selected from a parallel row of coffee ~2–3 m from the paired exclosure. Each pair was separated by at least 50 m, with the majority separated by more than 100 m. In total, 616 coffee plants were sampled, half of which were inside the exclosures and half outside. The coffee farms represented a variety of management systems, from shaded organic farms to unshaded conventional farms. Farm management can directly and indirectly influence VVIs and arthropods—some of the effects of management at these sites are discussed in relation to arboreal ants (Philpott et al., 2006) and ground foraging ants (Perfecto et al., 2003).
Arthropods were sampled using a D-vac (a reversed leaf blower modified with a fine mesh that allowed the collection of micro-arthropods), passed over a branch for a standardized amount of time. The contents of each sample were carefully transferred from the mesh and stored in 70% ethanol until identifications were made. Two coffee branches were randomly selected for arthropod sampling from each of 10 coffee bushes inside and outside exclosures (except in five exclosures and their controls where a lower number of plants were available for sampling). Samples were taken 2–3 days after the establishment of the exclosures (for baseline data) and at 3 and 6 months after establishment. This sampling method is destructive, with all arthropods on the two branches of the focal plants effectively removed; however, three months between sampling periods was assumed to be more than sufficient for arthropods to re-establish on the focal plants. The study site experiences distinct rainy and dry seasons. The 3 months sampling, in February, was in the middle of the dry season. The 6 months sampling in May occurred at the start of the rainy season.
Total foliar biomass on each sampled branch was estimated by measuring the length and width of each leaf and assuming the area results from an ellipsoid relationship between length and width (a justified assumption given the shape of coffee leaves). One hundred leaves from each farm (10 from 10 coffee plants) were collected at random. The area was estimated and the leaves were dried in an oven to constant weight. With that data, an empirically derived regression equation relating area with weight (weight = (0.025*area) − 0.08) was used to estimate the biomass of each leaf.
Arthropods were sorted to order and some to family. The information at the family level was used to assign individuals to the herbivore trophic level (within Coleoptera, Hemiptera, etc.). Insects in the order Hymenoptera were separated into formicids (ants) and non-formicids (bees and wasps). Numbers of arthropods are presented as number per unit of foliar biomass. Our use of abundance, rather than biomass, as a measure of arthropod populations likely underestimates the effects of VVIs, given that they preferentially feed on larger arthropods. We did not estimate biomass because of the high variability and lack of precision associated with biomass estimation methods (Gruner, 2003).
A random sample of 15 leaves was taken from each sampled plant at the beginning of the experiment and after 6 months. Herbivore damage, approximated by leaf area loss, was estimated through image analysis using NIH-Image. Yield data were obtained by repeatedly harvesting all mature (red) berries for all the experimental plants from August 2001 to January 2002. Berries were counted and weighed for each individual plant.
We built generalized linear models using the “glmmTMB” function in the “glmmTMB” package in R (Brooks et al., 2017). Models for herbivores, spiders and parasitoids included the date (i.e., seasonality), treatment (control or exclosure), and interaction between date and exclosure, and farm. For the purposes of this analysis and paper, we were not interested in assessing the inter-farm differences in management, but with four farms, there were too few levels to include it as a random effect. We used exclosure replicate nested by farm as a random intercept to account for increased correlation between repeated measure at the exclosure (and control) sites. The data for herbivores, spiders and wasps was non-normal semi-continuous data, because it was adjusted by foliar biomass. We used a Gamma distribution and check the model fit using the “DHARMa” package in R (Hartig, 2019).
We used post-hoc tests to generate contrasts that allowed us to make pairwise comparisons between all three sampling points. We calculated the estimated marginal means, also known as least square means, using the “emmeans” function from the “emmeans” package in R (Lenth and Lenth, 2018). This allowed us to compare across sampling dates and the factorial combinations of treatments. The model could be re-paramaterized using difference reference categories, but using contrasts provides comparisons between all levels of the factor with re-calculating the intercepts.
Similarly, we used generalized linear mixed models with the same random effects structure to assess the impact of treatment (control or exclosure) on herbivory and yield. This data was taken just once, so no effect of seasonality and no interaction with seasonality and treatment could be assessed.
To test the robustness of our results, arthropod, herbivory and yield data were also analyzed using non-parametric Wilcoxon paired tests. The non-parametric Wilcoxon test was used because the data failed to meet the assumption of normality required of parametric tests. Paired tests were executed between treatments (control and exclosures) at each time point.
Results
The vast majority of the herbivores collected were in two suborders of Hemiptera: Sternorrhyncha and Auchenorrhyncha (e.g., in the first sampling period 93% of all herbivores were in these two suborders). An overwhelming majority of the non-formicid hymenopterans (>95%) were parasitic wasps (i.e., parasitoids). There was high variability in ant abundances and our collection method was not well-suited to capture differences in ants, given their eusociality. The majority of the spiders captured in our study were web builders in the families Theridiidae (24%), Tetragnatidae (11%), and Araneidae (10.3%).
The three major groups of interest—herbivores, spiders and parasitoids—responded differently to the treatments over time (Figure 3, Supplementary Figure 1). There were no differences in the abundance of herbivores or spiders between treatments at the start of the experiment (emmeans, herbivore p = 0.9949, spiders p = 0.9985). GLMM results showed no difference between parasitoid abundances at the start of the experiment (emmeans, p = 0.6686). However, the non-parametric Wilcoxin test was marginally significant (Supplementary Table 1, p = 0.0545).
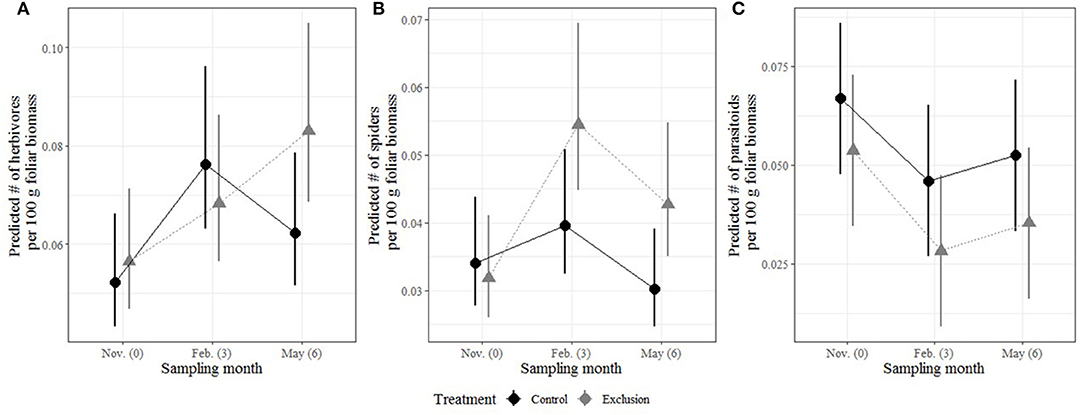
Figure 3. Means and confidence intervals predicted from the GLMM output for herbivores (A), spiders (B), and parasitoids (C) per 100 g foliar biomass. Black circles represent the control treatment and gray triangles represent the exclosure treatment. Note that the y-axes are different in each panel.
Three months after VVIs were excluded we found an increase in herbivore abundance across both treatments (emmeans, p = 0.0178). Spiders were also more abundant after 3 months (GLMM, p = 0.0114), and there was a significant interaction between treatments between November and February (emmeans, p = 0.0467). After 6 months, herbivore abundance increased (emmeans, p = 0.0259) in both treatments and there was a significant interaction between treatments in November and May (emmeans, p = 0.0263). Spider abundance did not differ between November and May (emmeans, p = 0.79) and there was a marginally significant decrease in abundance between February and May (emmeans, p = 0.0621). Parasitoid abundance was lower in exclosures after 3 (emmeans, p < 0.001) and 6 months (emmeans, p = 0.0037).
There were more spiders, regardless of time, in the exclosures (GLMM, p = 0.0388) and fewer parasitoids (GLMM, p = 0.001). Treatment alone was not a significant predictor of herbivore abundance (GLMM, p = 0.428). Model results (Table 1) are expanded upon with full pairwise interactions in Supplementary Table 1.
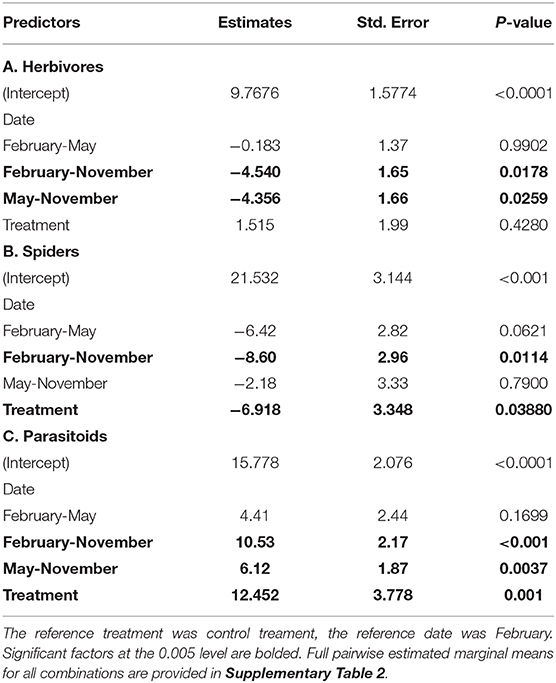
Table 1. Generalized linear mixed model results and pairwise estimated marginal means contrasts of dates for herbivores (A), spiders (B), and parasitoids (C).
When summing all arthropods captures, there were season differences, but no differences between treatments (Supplementary Table 3, Supplementary Figure 1A). All arthropods captured are detailed in Supplementary Table 4.
Six months after the exclosures were established, foliar herbivory was an average of 0.17% lower in the exclosures (GLMM, std error = 0.04603, p = 0.00013). However, herbivory levels across both treatments were quite low (~1–2%, Supplementary Figure 2A). Higher herbivory in the absence of VVIs did not translate into lower coffee yields (GLMM, std error < 0.001, p = 0.4987, Supplementary Figure 2B) and there was no correlation between herbivory and yield (Supplementary Figure 2C).
Discussion
Our results highlight the role of intraguild predation in food webs with VVIs, as well as the direct role of VVIs in consuming herbivores. Our findings also support recent studies that indicate that VVIs can contribute to biocontrol, limiting arthropod populations in forests and agroecosystems (Greenberg et al., 2000; Kalka et al., 2008; Van Bael et al., 2008; Williams-Guillén et al., 2008; Johnson et al., 2010; Böhm et al., 2011; Morrison and Lindell, 2012; Karp and Daily, 2014; Gras et al., 2016; Maas et al., 2016). The implicit assumption of these and other exclosure studies is that vertebrate predators provide an important ecosystem function via inter-trophic predation. We do find evidence of direct consumption, but also suggest intraguild predation within the predator guild could be a key determinant in the net impact of VVIs on herbivores.
With significantly higher herbivore abundance inside the exclosures after 3 and 6 months, we find evidence of top-down control of herbivores by VVIs and of inter-trophic predation. A common concern in exclosure studies is that birds may use closures as a perch, artificially increasing the density of birds in the area. However, even the most intensified site in our study had a canopy of shade trees, so perch sites are unlikely to be limiting. While we find evidence of top down control by VVIs, higher spider abundance in the exclosures at both sampling points also underscores the role of intraguild predation. This is congruent with past work that finds intraguild effects of birds preying on spiders (Sherry, 1984; Burger et al., 1999; Greenberg et al., 2000; Strong, 2000; Van Beal et al., 2003; Gruner, 2004; Philpott et al., 2004; Gunnarsson, 2007; Karp and Daily, 2014) and spiders preying on parasitoids (Gunnarsson, 2007) can alter the net effect of VVIs on herbivores.
Our design does not allow us to disentangle the role of birds from bats, but each group is likely acting differently as predators. Other studies that have used diurnal and nocturnal exclosures have found a negative, additive effect of birds and bats on arthropod abundance (Williams-Guillén et al., 2008) and that bats can have a stronger effect than birds on arthropod abundances and resultant herbivory (Kalka et al., 2008). Exclosure studies suggest bats affect all arthropod groups, except spiders, though analysis of bird and bat feces in another coffee growing region found little evidence that bats are controlling the coffee berry borer (Karp et al., 2013). Unfortunately, the method we used for sampling arthropods is not good for sampling the coffee berry borer, which is found inside the berries, nor coffee leaf miners, which is inside the leaf tissue. Therefore, we cannot make inferences about the effect of VVIs on these two important coffee pests. Gut or fecal analysis would be useful in further defining these trophic relationships and determining the role of birds and bats broadly and determining which species are involved in these trophic relationships.
Parasitoid abundance was lower when VVIs were excluded, and parasitoid abundance was negatively correlated with spider abundance both in and outside of exclosures. This might suggest an indirect positive effect of VVIs on parasitoids, mediated by their consumption of spiders. Although the number of parasitoids was marginally lower within the exclosures at the initiation of the experiment (according to the Wilcoxin test, but not the GLMM), the difference between the controls and exclosures got larger and more highly significant both 3 and 6 months after establishment, which indicates that the exclosures had an important effect on the parasitoids. We know of no methodological bias that would lead to lower parasitoid numbers at baseline, but the baseline data was collected 2–3 days after the establishment of the exclosures, so it's possible that parasitoids were highly sensitive to any disturbance created during the establishment of the exclosures and therefore their numbers were marginally lower a few days after the disturbance. Physical structures are often limiting for web-building spiders (Uetz, 1991); the exclosure apparatus may have inflated the number of spider webs, and augmented the effect of spiders on parasitoids, but this is unlikely to have happened within 2–3 days of establishment. Regardless, this finding highlights the need to consider both arthropod predators and parasitoids together in food web studies and focus further research on the role of parasitoids.
Ants and beetles from this study design have been analyzed elsewhere (Philpott et al., 2006; Gordon et al., 2009) and showed no significant differences between exclosures and control. In another study conducted in some of the same sites as this study, Philpott et al. (2004) excluded VVIs from tree branches and found a marginally significant effect of VVIs on ants with VVIs reducing ants by 68% (p = 0.07). The main difference between these two studies is that in our study we excluded VVIs from coffee plants, while in the Philpott et al. study VVIs were excluded from branches on the shade trees. These are the sites where birds spend more time foraging and perching and therefore are more likely to affect the arthropod community, including ants. It has been shown that some resident birds shift their foraging from the shade layer to the coffee layer during the dry season, when the migratory birds arrive in high abundances (Jedlicka et al., 2006). However, that did not seem to be sufficient to cause a reduction of ants in the control as compared to the exclosures. Gordon et al. (2009) reported no effects of the exclosures on the beetle as a whole. However, they reported 293 morpho species of beetles belonging to 42 families, with representation of many predator families as well as herbivore families. Further research is needed to get a more complete picture of the role of these predator and parasitoid communities in the coffee food web and, as mentioned above, gut or fecal analysis would be useful in determining species-level trophic interactions.
Interpretation of results across all groups is complicated by seasonality. Sampling date, which could represent time from the treatment set up or seasonality, was significant for all of the major groups tested. Our exclosures were set up at the start of the dry season and the first sampling event after the baseline collection also took place during the dry season and our second sample, 6 months after set-up, occurred at the start of the rainy season. During the dry season (3 months survey, February), herbivore and spider abundance was higher in both exclosures and control. During the rainy season (6 months survey, May), herbivore abundance in the exclosures continued to rise, but abundances on the control plants decreased. The effect of the exclosures remained for spiders in the rainy season, though abundances decreased relative to the 3 months sample. Spiders in this system are key players in intraguild predation, as both prey of VVIs and predators of parasitoids. Thus, the overall importance of intraguild predation in structuring food web dynamics may be greater in the dry season when spiders are more abundant.
Seasonality can also alter foraging behavior (Philpott et al., 2004). For example, birds have been found to take more Lepidopteran prey, which tend to be larger in size, in the rainy season, though this seasonal difference is more pronounced in the shade layer of the agro-ecosystem than on the coffee plants themselves (Dietsch et al., 2007). Life cycle traits and migration could both contribute to seasonal effects. Williams-Guillén et al. (2008) found a greater impact of birds on arthropods in the dry season, when insectivorous overwintering migrants are present (Greenberg, 1995), and a greater impact of bats during the wet season, possibly due to more bat reproduction and higher abundances. If VVIs are predating upon more large herbivores in the rainy season, we might expect an increase in herbivore abundance (though not biomass) and less of a difference between the exclosure and control plants. We do find a higher abundance of herbivores in the exclosures during the rainy season relative to both the control plants during the rainy season and the exclosures during the dry season. Without finer scale time series data, it is difficult to parse apart the effects of seasonality and the exclosures, but foraging behavior provides another mechanism by which the relative importance of intraguild predation could be seasonally dependent.
Herbivory, as measured by leaf area loss, was significantly higher in the exclosures than controls after 6 months, which suggests a trophic cascade initiated by removal of the top predators. VVIs may preferentially be eating the relatively large leaf chewing herbivores, including Orthopteran and Lepidopteran, which more commonly cause leaf area loss. Given that large leaf chewing herbivores are known to be common in coffee agro-ecosystems (Dietsch et al., 2007), the lack of large herbivores in our samples is a bit surprising, even if VVIs are feeding preferentially. However, the lack of large leaf chewers and prevalence of hemipteran in our samples is consistent with the low documented levels of herbivory across treatments. Herbivory measures also may not have captured any damage caused by the coffee leaf miner, as coffee plants often respond to coffee leaf miner damage by dropping the damaged leaves (Guerreiro Filho, 2006). Thus, leaves sampled at the end of the experiment would have underestimated this damage. In previous work biocontrol services provided by VVIs were mediated by local and landscape level forest cover, but landscape level forest cover decreased leaf loss (Librán-Embid et al., 2017). Our study operates at the landscape level, with sites across farms in one coffee-growing region. Though pesticides were not used across all sites, usage may have decreased herbivory at some farms adding to some of the variability between farms.
We find the effect of top predators diminishes through the food web; we couldn't detect a significant effect of the exclosures on coffee yield. Other work in coffee systems has found a decrease in fruit set with the exclusion of vertebrates (Classen et al., 2014) and yield has been shown to decrease as much as 31% with the exclusion of VVIs in cacao systems (Maas et al., 2013). Still, our overall result is congruent with meta-analyses showing weak responses of terrestrial plants to the elimination of predators, even with a significant reduction of herbivores [Schmitz et al., 2000; Shurin et al., 2002; but see Croll et al. (2005) and Borer et al. (2005)]. However, herbivory levels found in this study were also very low (~1–2%), so a lack of an effect on yield was not altogether surprising. In future studies, data should be taken on damage by the coffee berry borer, which affect coffee seed quality and weight rather than number of berries.
Shaded coffee farms have received much attention in the last 30 years because of their conservation potential (Perfecto and Vandermeer, 2015). High diversity and density of migrant and resident birds has become the basis for green certification labels, both to encourage conservation of migratory and resident species and in acknowledgment of the array of ecosystem services—including biocontrol—provided by birds and bats. This study provides empirical support of the role of VVIs in structuring the arthropod community in these managed agroecosystems. The response of herbivores, if not herbivory itself, does provide evidence that predators, including birds and bats, can contribute to preventative pest control in coffee agro-ecosystems. Most importantly, our results underscore the importance of considering intraguild predation between VVIs and spiders and spiders and parasitoids when investigating or managing for VVI control of arthropod herbivores.
Data Availability Statement
The datasets generated for this study are available on request to the corresponding author.
Author Contributions
RG, GI-N, and IP conceived of the ideas and designed the methodology. RG, GI-N, IP, PB, and CG contributed to data collection and analysis. LS led the writing of the manuscript. All authors contributed to the article and approved the submitted version.
Funding
This study was funded by NSF Grant no. DEB 9981526 to IP, GI-N, and RG.
Conflict of Interest
The authors declare that the research was conducted in the absence of any commercial or financial relationships that could be construed as a potential conflict of interest.
Acknowledgments
We thank J. Vandermeer, T. Schoener, E. Werner, T. Dietsch, S. Philpott, B. Lin, D. Gruner, and S. Uno, and several reviewers for providing useful comments on versions of this manuscript. J. C. López de León, H. Yard, A. Mendizabal, B. Esteban Chilel, Gustavo López Batista, and Alvaro García Ballinas helped with field data collection and sorting and identifying arthropods. We thank the Peters and Edelman Families and the ISMAN Cooperative for permission to work on their farms.
Supplementary Material
The Supplementary Material for this article can be found online at: https://www.frontiersin.org/articles/10.3389/fsufs.2021.512998/full#supplementary-material
References
Böhm, S. M., Wells, K., and Kalko, E. K. (2011). Top-down control of herbivory by birds and bats in the canopy of temperate broad-leaved oaks (Quercus robur). PLoS ONE 6:e17857. doi: 10.1371/journal.pone.0017857
Borer, E. T., Seabloom, E. W., Shurin, J. B., Anderson, K. E., Blanchette, C. A., Broitman, B., et al. (2005). What determines the strength of a trophic cascade? Ecology 86, 528–537. doi: 10.1890/03-0816
Borkhataria, R. R., Collazo, J. A., and Groom, M. J. (2006). Additive effects of vertebrate predators on insects in a Puerto Rican coffee plantation. Ecol. Appl. 16, 696–703. doi: 10.1890/1051-0761(2006)016[0696:AEOVPO]2.0.CO;2
Brooks, M. E., Kristensen, K., Van Benthem, K. J., Magnusson, A., Berg, C. W., Nielsen, A., et al. (2017). glmmTMB balances speed and flexibility among packages for zero-inflated generalized linear mixed modeling. R J. 9, 378–400. doi: 10.32614/RJ-2017-066
Burger, J. C., Patten, M. A., Rotenberry, J. T., and Redak, R. A. (1999). Foraging ecology of the California gnatcatcher deduced from fecal samples. Oecologia 120, 304–310. doi: 10.1007/s004420050862
Classen, A., Peters, M. K., Ferger, S. W., Helbig-Bonitz, M., Schmack, J. M., Maassen, G., et al. (2014). Complementary ecosystem services provided by pest predators and pollinators increase quantity and quality of coffee yields. Proc. Royal Soc. B Biol. Sci. 281:20133148. doi: 10.1098/rspb.2013.3148
Croll, D. A., Maron, J. L., Estes, J. A., Danner, E. M., and Byrd, J. V. (2005). Introduced predators transform subartic islands from grasslands to tundra. Science 307, 1959–1961. doi: 10.1126/science.1108485
Damon, A. (2000). A review of the biology and control of the coffee berry borer, Hypothenemus hampei (Coleoptera: Scolytidae). Bullet. Entomol. Res. 90, 453–465. doi: 10.1017/S0007485300000584
Dietsch, T. V., Perfecto, I., and Greenberg, R. (2007). Avian foraging behavior in two different types of coffee agroecosystem in Chiapas, Mexico. Biotropica 39, 232–240. doi: 10.1111/j.1744-7429.2006.00248.x
Gagnon, A. È., Heimpel, G. E., and Brodeur, J. (2011). The ubiquity of intraguild predation among predatory arthropods. PLoS ONE 6:e28061. doi: 10.1371/journal.pone.0028061
Gordon, C. E., McGill, B., Ibarra-Núñez, G., Greenberg, R., and Perfecto, I. (2009). Simplification of a coffee foliage-dwelling beetle community under low-shade management. Basic Appl. Ecol. 10, 246–254. doi: 10.1016/j.baae.2008.04.004
Gras, P., Tscharntke, T., Maas, B., Tjoa, A., Hafsah, A., and Clough, Y. (2016). How ants, birds and bats affect crop yield along shade gradients in tropical cacao agroforestry. J. Appl. Ecol. 53, 953–963. doi: 10.1111/1365-2664.12625
Grass, I., Lehmann, K., Thies, C., and Tscharntke, T. (2017). Insectivorous birds disrupt biological control of cereal aphids. Ecology 98, 1583–1590. doi: 10.1002/ecy.1814
Greenberg, R. (1995). Insectivorous migratory birds in tropical ecosystems: the breeding currency hypothesis. J. Avian Biol. 260–264. doi: 10.2307/3677328
Greenberg, R., Bichier, P., Angon, A. C., MacVean, C., Perez, R., and Cano, E. (2000). The impact of avian insectivory on arthropods and leaf damage in some Guatemalan coffee plantations. Ecology 81, 1750–1755. doi: 10.1890/0012-9658(2000)081[1750:TIOAIO]2.0.CO;2
Greenberg, R., Bichier, P., and Sterling, J. (1997). Bird populations in rustic and planted shade coffee plantations of eastern Chiapas, Mexico. Biotropica 29, 501–514. doi: 10.1111/j.1744-7429.1997.tb00044.x
Gruner, D. S. (2003). Regressions of length and width to predict arthropod biomass in the Hawaiian Islands. Pacific Sci. 57, 325–336. doi: 10.1353/psc.2003.0021
Gruner, D. S. (2004). Attenuation of top-down and bottom-up forces in a complex terrestrial community. Ecology 85, 3010–3022. doi: 10.1890/04-0020
Guerreiro Filho, O. (2006). Coffee leaf miner resistance. Brazil. J. Plant Physiol. 18, 109–117. doi: 10.1590/S1677-04202006000100009
Gunnarsson, B. (2007). Bird predation on spiders: ecological mechanisms and evolutionary consequences. J. Arachnol. 35, 509–529. doi: 10.1636/RT07-64.1
Hairston, N. G., and Hairston, Jr., N. G. (1997). Does food web complexity eliminate trophic-level dynamics? Am. Natural. 149, 1001–1007. doi: 10.1086/286035
Hartig, F. (2019). Dharma: Residual Diagnostics for Hierarchical (Multi-Level/Mixed) Regression Models CRAN. CRAN (2019). Available online at: http://florianhartig.github.io/DHARMa/ (accessed March 11, 2021).
Holmes, R. T., Schultz, J. C., and Nothnagle, P. (1979). Bird predation on forest insects: an exclosure experiment. Science 206, 462–463. doi: 10.1126/science.206.4417.462
Ibarra-Núñez, G., Garcia, J. A., Lopez, J. A., and Lachaud, J. P. (2001). Prey analysis in the diet of some ponerine ants (Hymenoptera: Formicidae) and web-building spiders (Aranae) in coffee plantations in Chiapas, Mexico. Sociobiology 37, 723–755.
Jedlicka, J. A., Greenberg, R., Perfecto, I., Philpott, S. M., and Dietsch, T. V. (2006). Seasonal shift in the foraging niche of a tropical avian resident: resource competition at work?. J. Trop. Ecol. 2006, 385–395. doi: 10.1017/S0266467406003191
Jha, S., Bacon, C. M., Philpott, S. M., Ernesto Méndez, V., Läderach, P., and Rice, R. A. (2014). Shade coffee: update on a disappearing refuge for biodiversity. BioScience 64, 416–428. doi: 10.1093/biosci/biu038
Johnson, M. D., Kellermann, J. L., and Stercho, A. M. (2010). Pest reduction services by birds in shade and sun coffee in Jamaica. Anim. Conserv. 13, 140–147. doi: 10.1111/j.1469-1795.2009.00310.x
Kalka, M. B., Smith, A. R., and Kalko, E. K. (2008). Bats limit arthropods and herbivory in a tropical forest. Science 320:71. doi: 10.1126/science.1153352
Karp, D. S., Mendenhall, C. D., Sandí, R. F., Chaumont, N., Ehrlich, P. R., Hadly, E. A., et al. (2013). Forest bolsters bird abundance, pest control and coffee yield. Ecol. Lett. 16, 1339–1347. doi: 10.1111/ele.12173
Karp, D. S., and Daily, G. C. (2014). Cascading effects of insectivorous birds and bats in tropical coffee plantations. Ecology 95, 1065–1074. doi: 10.1890/13-1012.1
Lambers, R. H. R., and Dickerman, U. (2003). Competition and predation in simple food webs: intermediately strong trade-offs maximize coexistence. Proc. Royal Soc. Lond. Ser. B Biol. Sci. 270, 2591–2598. doi: 10.1098/rspb.2003.2532
Lenth, R., and Lenth, M. R. (2018). Package ‘lsmeans’. Am. Statist. 34, 216–221. doi: 10.1080/00031305.1980.10483031
Lewis, W. J., Van Lenteren, J. C., Phatak, S. C., and Tumlinson, J. H. (1997). A total system approach to sustainable pest management. Proc. Natl. Acad. Sci. U. S. A. 94, 12243–12248. doi: 10.1073/pnas.94.23.12243
Librán-Embid, F., De Coster, G., and Metzger, J. P. (2017). Effects of bird and bat exclusion on coffee pest control at multiple spatial scales. Landscape Ecol. 32, 1907–1920. doi: 10.1007/s10980-017-0555-2
Maas, B., Clough, Y., and Tscharntke, T. (2013). Bats and birds increase crop yield in tropical agroforestry landscapes. Ecol. Lett. 16, 1480–1487. doi: 10.1111/ele.12194
Maas, B., Karp, D. S., Bumrungsri, S., Darras, K., Gonthier, D., Huang, J. C. C., et al. (2016). Bird and bat predation services in tropical forests and agroforestry landscapes. Biol. Rev. 91, 1081–1101. doi: 10.1111/brv.12211
Marquis, R. J., and Whelan, C. J. (1994). Insectivorous birds increase growth of white oak through consumption of leaf-chewing insects. Ecology 75, 2007–2014. doi: 10.2307/1941605
Martin, E. A., Reineking, B., Seo, B., and Steffan-Dewenter, I. (2013). Natural enemy interactions constrain pest control in complex agricultural landscapes. Proc. Natl. Acad. Sci. U. S. A. 110, 5534–5539. doi: 10.1073/pnas.1215725110
McCann, K., Hastings, A., and Huxel, G. R. (1998). Weak trophic interactions and the balance of nature. Nature 395, 794–798. doi: 10.1038/27427
Moguel, P., and Toledo, V. M. (1999). Biodiversity conservation in traditional coffee systems of Mexico. Conserv. Biol. 13, 11–21.
Montoya, J. M., Rodríguez, M. A., and Hawkins, B. A. (2003). Food web complexity and higher-level ecosystem services. Ecol. Lett. 6, 587–593. doi: 10.1046/j.1461-0248.2003.00469.x
Mooney, K. A., Gruner, D. S., Barber, N. A., Van Bael, S. A., Philpott, S. M., and Greenberg, R. (2010). Interactions among predators and the cascading effects of vertebrate insectivores on arthropod communities and plants. Proc. Natl. Acad. Sci. U. S. A. 107, 7335–7340. doi: 10.1073/pnas.1001934107
Morrison, E. B., and Lindell, C. A. (2012). Birds and bats reduce insect biomass and leaf damage in tropical forest restoration sites. Ecol. Appl. 22, 1526–1534. doi: 10.1890/11-1118.1
Nyffeler, M., Sekercioglu, Ç. H., and Whelan, C.J. (2018). Insectivorous birds consume an estimated 400–500 million tons of prey annually. Sci. Nat. 105:47. doi: 10.1007/s00114-018-1571-z
Oksanen, L., and Oksanen, T. (2000). The logic and realism of the hypothesis of exploitation ecosystems. Am. Natural. 155, 703–723. doi: 10.1086/303354
Pejchar, L., Clough, Y., Ekroos, J., Nicholas, K. A., Olsson, O. L. A., Ram, D., et al. (2018). Net effects of birds in agroecosystems. BioScience 68, 896–904. doi: 10.1093/biosci/biy104
Perfecto, I., Mas, A., Dietsch, T., and Vandermeer, J. (2003). Conservation of biodiversity in coffee agroecosystems: a tri-taxa comparison in southern Mexico. Biodiversity Conserv. 12, 1239–1252. doi: 10.1023/A:1023039921916
Perfecto, I., and Vandermeer, J. (2015). Coffee Agroecology: A New Approach to Understanding Agricultural Biodiversity, Ecosystem Services and Sustainable Development. London: Routledge. doi: 10.4324/9780203526712
Perfecto, I., Vandermeer, J., Lopez, G., Ibarra Nuñez, G., Greenberg, R., Bichier, P., et al. (2004). Greater predation of insect pests in a diverse agroecosystem: the role of resident Neotropical birds in shade coffee farms. Ecology 85, 2677–2681. doi: 10.1890/03-3145
Philpott, S. M., Greenberg, R., Bichier, P., and Perfecto, I. (2004). Impacts of major predators on tropical agroforest arthropods: comparisons within and across taxa. Oecologia 140, 140–149. doi: 10.1007/s00442-004-1561-z
Philpott, S. M., Perfecto, I., and Vandermeer, J. (2006). Effects of management intensity and season on arboreal ant diversity and abundance in coffee agroecosystems. Biodiversity Conserv. 15, 139–155. doi: 10.1007/s10531-004-4247-2
Philpott, S. M., Soong, O., Lowenstein, J. H., Pulido, A. L., Lopez, D. T., Flynn, D. F., et al. (2009). Functional richness and ecosystem services: bird predation on arthropods in tropical agroecosystems. Ecol. Appl. 19, 1858–1867. doi: 10.1890/08-1928.1
Polis, G. A., and Hurd, S. D. (1995). Extraordinarily high spider densities on islands: flow of energy from the marine to terrestrial food webs and the absence of predation. Proc. Natl. Acad. Sci. U. S. A. 92, 4382–4386. doi: 10.1073/pnas.92.10.4382
Rendón, M. A. P., Ibarra-Nú, G., Parra-Tabla, V., García-Ballinas, J. A., and Hénaut, Y. (2006). Spider diversity in coffee plantations with different management in Southeast Mexico. J. Arachnol. 34, 104–113. doi: 10.1636/M03-044.1
Schmitz, O. J., Hamback, P. A., and Beckerman, A. P. (2000). Trophic cascades in terrestrial systems: a review of the effects of carnivore removals on plants. Am. Natural. 155, 141–153. doi: 10.1086/303311
Schoener, T. (1989). Food webs from the small to the large: The Robert H. MacArthur Award Lecture. Ecology 70, 1559–1589. doi: 10.2307/1938088
Sekercioglu, C. H. (2006). Increasing awareness of avian ecological function. Trends Ecol. Evol. 21, 464–471. doi: 10.1016/j.tree.2006.05.007
Sherry, T. W. (1984). Comparative dietary ecology of sympatric, insectivorous neotropical flycatchers (Tyranidae). Ecol. Monogr. 54, 313–338. doi: 10.2307/1942500
Shurin, J. B., Borer, E. T., Seabloom, E. W., Anderson, K., Blanchette, C. B., Broitman, B., et al. (2002). A cross-ecosystem comparison of the strength of trophic cascades. Ecol. Lett. 5, 785–779. doi: 10.1046/j.1461-0248.2002.00381.x
Spiller, D. A., and Schoener, T. W. (1990). A terrestrial field experiment showing impact of eliminating top predators on foliage damage. Nature 347, 469–472. doi: 10.1038/347469a0
Staver, C., Guharay, F., Monterroso, D., and Muschler, R. G. (2001). Designing pest-suppressive multistrata perennial crop systems: shade-grown coffee in Central America. Agroforestr. Syst. 53, 151–170. doi: 10.1023/A:1013372403359
Stouffer, D. B., Camacho, J., Jiang, W., and Amaral, L. A. N. (2007). Evidence for the existence of a robust pattern of prey selection in food webs. Proc. Royal Soc. Lond. B Biol. Sci. 274, 1931–1940. doi: 10.1098/rspb.2007.0571
Strong, A. M. (2000). Divergent foraging strategies of two Neotropical migrant warblers: implications for winter habitat use. Auk 117, 381–392. doi: 10.1093/auk/117.2.381
Uetz, G. W. (1991). “Habitat structure and spider foraging,” in Habitat Structure (Dordrecht: Springer), 325–348. doi: 10.1007/978-94-011-3076-9_16
Van Bael, S. A., Philpott, S. M., Greenberg, R., Bichier, P., Barber, N. A., Mooney, K. A., et al. (2008). Birds as predators in tropical agroforestry systems. Ecology 89, 928–934. doi: 10.1890/06-1976.1
Van Beal, S. A., Brawn, J. D., and Robinson, S. K. (2003). Birds defend trees from herbivores in a Neotropical forest canopy. Proc. Natl. Acad. Sci. U. S. A. 100, 8304–8307. doi: 10.1073/pnas.1431621100
Vandermeer, J., Armbrecht, I., De la Mora, A., Ennis, K. K., Fitch, G., Gonthier, D. J., et al. (2019). The community ecology of herbivore regulation in an agroecosystem: lessons from complex systems. BioScience 69, 974–996. doi: 10.1093/biosci/biz127
Whelan, C. J., Wenny, D. G., and Marquis, R. J. (2008). Ecosystem services provided by birds. Ann. N. Y. Acad. Sci. 1134, 25–60. doi: 10.1196/annals.1439.003
Williams-Guillén, K., Perfecto, I., and Vandermeer, J. (2008). Bats limit insects in a neotropical agroforestry system. Science 320:70. doi: 10.1126/science.1152944
Keywords: coffee agroecosystem, food web, intraguild predation, trophic cascade, spiders, parasitoids, biological control, ecosystem function
Citation: Schmitt L, Greenberg R, Ibarra-Núñez G, Bichier P, Gordon CE and Perfecto I (2021) Cascading Effects of Birds and Bats in a Shaded Coffee Agroforestry System. Front. Sustain. Food Syst. 5:512998. doi: 10.3389/fsufs.2021.512998
Received: 18 November 2019; Accepted: 22 March 2021;
Published: 27 April 2021.
Edited by:
Kris A. G. Wyckhuys, Chinese Academy of Agricultural Sciences (CAAS), ChinaReviewed by:
Daniel Sol Karp, University of California, Davis, United StatesFelipe Librán Embid, University of Göttingen, Germany
Copyright © 2021 Schmitt, Greenberg, Ibarra-Núñez, Bichier, Gordon and Perfecto. This is an open-access article distributed under the terms of the Creative Commons Attribution License (CC BY). The use, distribution or reproduction in other forums is permitted, provided the original author(s) and the copyright owner(s) are credited and that the original publication in this journal is cited, in accordance with accepted academic practice. No use, distribution or reproduction is permitted which does not comply with these terms.
*Correspondence: Lauren Schmitt, lschmit@umich.edu
†Deceased