- 1School of GeoSciences, University of Edinburgh, Edinburgh, United Kingdom
- 2School of Biotechnology, Guru Gobind Singh Indraprastha University, New Delhi, India
- 3School of Biotechnology, Kalinga Institute of Industrial Technology, Bhubaneswar, India
- 4Department of Botany, Aligarh Muslim University, Aligarh, India
- 5ICAR-Indian Agricultural Research Institute, New Delhi, India
- 6National Institute of Agricultural Botany, Cambridge, United Kingdom
- 7Indian Institute of Tropical Meteorology, IITM, Pune, India
- 8UK Centre for Ecology & Hydrology, Penicuik, United Kingdom
- 9Division of Plant and Crop Sciences, School of Biosciences, University of Nottingham, Loughborough, United Kingdom
- 10International Crops Research Institute for the Semi-Arid Tropics, Patancheru, India
- 11Indian Institute for Rice Research, Hyderabad, India
- 12Department of Plant Sciences, University of California, Davis, Davis, CA, United States
- 13National Institute for Plant Biotechnology, New Delhi, India
- 14National Rice Research Institute, Cuttack, India
- 15Rothamsted Research, Harpenden, United Kingdom
- 16Department of Plant Sciences, University of Oxford, Oxford, United Kingdom
- 17School of Biological Science, University of Aberdeen, Aberdeen, United Kingdom
- 18Indian Institute of Soil Science, Bhopal, India
- 19Indian Institute of Wheat and Barley Research, Karnal, India
- 20Department of Soil Science, Punjab Agricultural University, Ludhiana, India
- 21Food and Agriculture Organization of the United Nations, Animal Production and Health Division, Rome, Italy
- 22Animal Production Systems Group, Wageningen University and Research, Wageningen, Netherlands
In the last six decades, the consumption of reactive nitrogen (Nr) in the form of fertilizer in India has been growing rapidly, whilst the nitrogen use efficiency (NUE) of cropping systems has been decreasing. These trends have led to increasing environmental losses of Nr, threatening the quality of air, soils, and fresh waters, and thereby endangering climate-stability, ecosystems, and human-health. Since it has been suggested that the fertilizer consumption of India may double by 2050, there is an urgent need for scientific research to support better nitrogen management in Indian agriculture. In order to share knowledge and to develop a joint vision, experts from the UK and India came together for a conference and workshop on “Challenges and Opportunities for Agricultural Nitrogen Science in India.” The meeting concluded with three core messages: (1) Soil stewardship is essential and legumes need to be planted in rotation with cereals to increase nitrogen fixation in areas of limited Nr availability. Synthetic symbioses and plastidic nitrogen fixation are possibly disruptive technologies, but their potential and implications must be considered. (2) Genetic diversity of crops and new technologies need to be shared and exploited to reduce N losses and support productive, sustainable agriculture livelihoods. (3) The use of leaf color sensing shows great potential to reduce nitrogen fertilizer use (by 10–15%). This, together with the usage of urease inhibitors in neem-coated urea, and better management of manure, urine, and crop residues, could result in a 20–25% improvement in NUE of India by 2030.
1. Introduction
The global nitrogen (N) cycle has been strongly altered by human intervention, leading to rapidly growing environmental concerns that increase in parallel with the rise in food demand fuelled by the expanding global human population. To feed humanity, N must be in a reactive form (Nr), such as ammonium and nitrate, which are essential to support crop production. However, such Nr compounds are sparse in the environment. Therefore, to satisfy the global need for Nr, large quantities of unreactive atmospheric nitrogen gas (N2) is converted to Nr as a base of fertilizers. A major step toward this goal was the invention of the Haber–Bosch process, producing ammonia (NH3) from its elements (N2 and hydrogen gas), doubling the world's Nr flows since its invention in the early 20th century (Galloway et al., 2003).
Although the production and usage of fertilizers provides Nr to help feed the population, it poses a series of interconnected environmental issues. The root of the problem is that only a proportion of Nr content in applied fertilizers is used effectively by crops and grasslands. Therefore, the “nitrogen use efficiency” (NUE) of the global food system integrating crop and livestock production systems is very low, being around 15% (Sutton et al., 2017 based on Sutton et al., 2013). This means that 85% of Nr applied is lost to air, groundwater or surface runoff, threatening the quality of air, soils, and fresh waters, driving climate change, and thereby endangering ecosystems and human-health (Galloway J. N. et al., 2008; Aneja et al., 2009; Sutton et al., 2011; Fowler et al., 2013).
In India, fertilizer consumption shows a continuously growing trend, with a yearly increase of 6% since 1970 (Sutton et al., 2017 based on FAO, 2016). This can be observed on Figure 1, which shows the annual fertilizer consumption in India in comparison with population (showing a much weaker annual growth rate), and the annual GDP (showing a closer, but much clearer exponential growth over the years). This increased fertilizer consumption has resulted in growing losses of Nr, of which one of the clearest indicators is the annual emission of ammonia (NH3)—the predominant fertilizer-related atmospheric Nr loss—with a growing trend in the last six decades (EDGAR, 2016). The environmental implications, as well as the health impacts of the increasing environmental losses of Nr in India, have been subjects of growing scientific interest (Aneja et al., 2006; Abrol et al., 2007; Bijay-Singh and Yadvinder-Singh, 2008; Galloway J. et al., 2008).
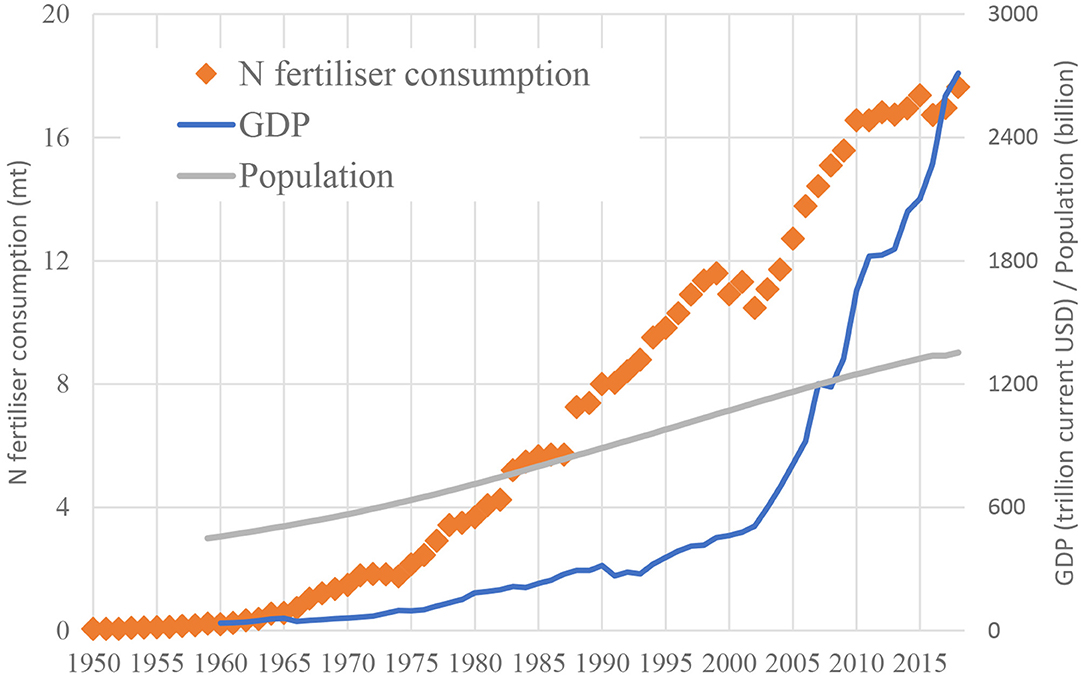
Figure 1. The annual nitrogen fertilizer consumption in India (Mt; FAI, 2020) together with the annual GDP (109 current USD; World Bank, 2020a) and population (106 people, World Bank, 2020b) of India.
While the consumption of fertilizers has been increasing in India, the NUE of cropping systems (expressed here as N in harvested arable products divided by fertilizer inputs) has been gradually decreasing, dropping from about 55% to as low as 35% between 1960 and 2010 (Bijay-Singh, 2017 based on Lassaletta et al., 2014). Although Figure 1 suggests that fertilizer consumption may have stabilized since 2010, the latest projections of the Food and Agriculture Organizations of United Nations (FAO, 2018) suggests that India may double its fertilizer input by 2050. Based on this, it is evident that for further sustainable development, a better scientific understanding of Nr flows and losses as well as improved technologies to increase NUE are needed.
A step toward this goal was taken when the BBSRC (Biotechnological and Biological Sciences Research Council, UK), DBT (Department of Biotechnology, India), and NERC (Natural Environment Research Council, UK) agreed to fund virtual joint centers (VJCs) to investigate the ways of managing agricultural N, improving crop production while reducing energy inputs and Nr losses to the environment. To achieve these goals, they identified three key options:
1) the enhancement of nitrogen-fixation in legumes and introducing its biological nitrogen fixation (BNF) potential to non-leguminous cropping systems,
2) the improvement of NUE at a plant level,
3) the improvement of NUE through agronomical practices.
To support and further implement these alternatives, four VJCs were established in 2016, linking the leading N research communities of India and the UK. These four virtual centers are:
• CINTRIN (Cambridge-India Network for Translational Research in Nitrogen), which aims to reduce the use of agricultural N by exploiting native variation and plant genetics and informing on-farm decision support;
• INEW (Indo-UK Center for The Improvement of Nitrogen Use Efficiency in Wheat), which investigates the genetic control and biochemical basis of NUE in wheat as well as precision application of N fertilizers;
• IUNFC (India-UK Nitrogen Fixation Center), which focuses on pigeon pea rhizobial N fixation and contribution of endophytes in N nutrition of rice; and
• NEWS India-UK (Newton-Bhabha Virtual Center on Nitrogen Efficiency of Whole-cropping Systems for improved performance and resilience in agriculture), which explores the options for increasing NUE and better planning of the whole crop rotation, and subsequently aims to apply the new biological and agronomic advances to the farm scale as well as to national scale.
In October 2017, a joint conference and workshop, entitled “Challenges and Opportunities for Agricultural Nitrogen Science in India” was organized at the National Agricultural Science Complex (NASC), in New Delhi. At the event, 85 experts came together (see the list of participants in Supplementary Table 1) from the four virtual joint centers to share findings in order to stimulate cooperation and to develop a long-term vision for N research and its policy applications in India. The primary aim of this paper is to summarize the outcomes of this conference and workshop.
The event was organized around three topics: BNF, biological NUE, and agronomic NUE including the environmental impacts. In the first part, at the conference, some of the latest results were presented. This was followed by a workshop where participants were working in three groups, corresponding to the three topics of the event. Each group had to discuss and report their answers to the same set of questions:
- What are the key findings?
- What are the common challenges?
- What are the future and science cooperation priorities?
- Are there key messages for the South Asian Nitrogen Assessment?
- What are the 2 or 3 best options emerging for better nitrogen management for your topic?
- How would you formulate a global goal for each option for 2030?
- What could each option realistically achieve (quantitatively) in terms of efficiency improvement or nitrogen saving by 2030?
- What are the main messages for current and future policies?
- What would be your group's vision statement?
The findings of each group were then reported in plenary to the other groups, allowing revision, and agreement by the conference as a whole. In this paper we firstly provide an overview of the main findings drawing on the findings of the groups according to the questions given. Secondly, we summarize the recommendations of the working groups for options for better N management in the Indian agriculture, and present a long-term vision for action. Each of these sections is organized around the three main topics of the conference. Finally, we conclude with the key vision statements agreed by the conference, encapsulating the core messages of the meeting.
NUE can be defined and measured in many different ways depending on the most accepted norms in each discipline (Raghuram and Sharma, 2019) and each of these measurements are precise in their own way. As such, different projects and their partners approached NUE at different levels using different tools based on their expertise and approved within the work packages of their VJCs. While harmonizing these different approaches of NUE toward a universally accepted definition or measurement is highly desirable, it is beyond the scope of this manuscript. Therefore, where no detailed explanation is provided for the calculation of NUE, we use the term NUE here as a general concept, in which we consider a change as “increase” if the output N grows (total plant N, grain N; biomass yield or grain yield) or N loss decreases (via atmospheric emission or leaching of nitrogen compounds) at a given N input level (total N, soil N, or N-fertilizer applied). We consider a change in NUE as “decrease” if the opposite is true. Biological NUE and agronomic NUE should be understood generally, as NUE on plant level and on the level of an agronomic system, respectively.
2. Key Findings of the Virtual Joint Centers
2.1. Biological Nitrogen Fixation (BNF)
The focus of this study was pigeon pea because it is the most important pulse crop in summer in the majority of India's drylands. It constitutes 15% of pulse area grown in 3.9 million ha, with an average grain yield of only 850 kg ha−1 (dry matter), fixing about 0.22 Tg N yr−1 out of a total legume BNF of 0.94 Tg yr−1 (Rao and Balachandar, 2017). Since BNF is limited by drought and poor nodulation, improvement strategies were based on identifying tolerant pigeon pea genotypes and Rhizobium strains that have greater tolerance to environmental stresses in the field (Trotman and Weaver, 1995). This strategy allowed us to identify the best combinations. Using standard agronomic practices, the principal objective was to improve nodulation and select for sustained nodulation since nodulation and N fixation in pigeon pea declines at early stages with little activity at flowering stage (Kumar Rao and Dart, 1987). The improvement of soil biology by inoculation with plant growth-promoting bacteria and rhizobia, as well as quantitative assessment of BNF rates, are also a priority. Further research is also needed on the benefits of microbial inoculation of rice to increase BNF and other nutrient acquisition. Rice harbors several endophytes, including non-N fixing bacteria as well as rhizobia, and inoculation of these has been shown to improve rice growth (Ladha et al., 2016; Chalk et al., 2017). Thus, there is a need to improve endophytic BNF and downstream assimilation of resulting Nr by rice plants.
Within IUNFC, a number of rhizobial strains of nodulating pigeon pea in farmers' fields have been isolated from three different soil types in India where pigeon pea is widely cultivated: Inceptisols of North India, Vertisols of Central India, and Alfisols of Peninsular India. A broad range of mainly slow-growing and some fast-growing rhizobia were isolated, characterized for their nodulation efficiency (Figure 2) and sequenced for the 16S rRNA gene. Nearly 25 species of rhizobia have been detected in three Indian soils belonging to six genera, Bradyrhizobium, Ensifer, Rhizobium, Neorhizobium, Burkholderia, and Cupriavidus.
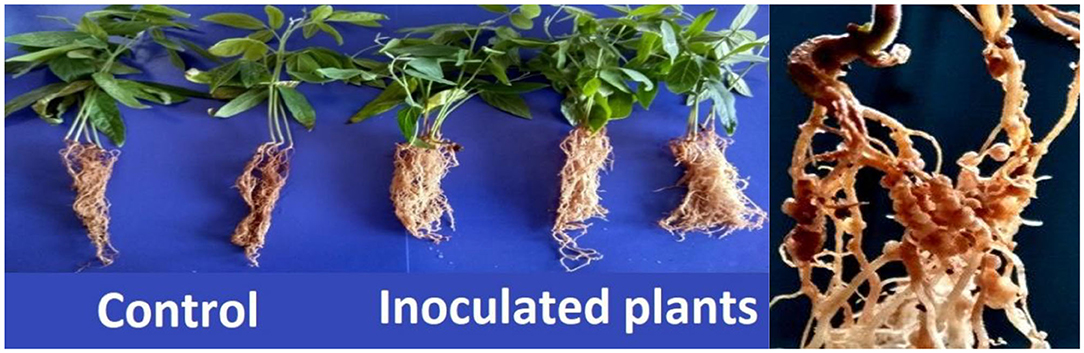
Figure 2. Screening of rhizobial strains for nodulation of pigeon pea in sterile sand microcosms (left); close up of a well-nodulated plant (right) (IUNFC).
The number of pigeon pea rhizobia in all the soils were counted using plant infection methods and the most probable number (MPN) counts were found to range from very low (102 cells/g) to low (103 cells/g) with very few exceptions where it was optimum (104 cells/g). Rhizobial numbers decrease drastically in dry hot summer lasting 2 months, reducing the numbers of rhizobia in the soil to <100 cells/g and causing a decline in diversity. Therefore, there is a need to select rhizobia that are symbiotically superior under stress (Rao, 2014). Some very high performing rhizobia have already been identified and have been put back into the field for inoculation testing. These rhizobia are being further tested within the All-India Network of the Indian Council of Agricultural Research (ICAR) at multiple locations before their release for mass-scale biofertilizer production and subsequent supply to farmers. In addition, rhizobia with greater ability to solubilize phosphorous in the rhizosphere through enhanced secretion of 2-keto gluconic acid have been engineered and these will be tested in pigeon pea rhizobia.
Microbiome selection by plants in soils is highly conserved (i.e., plants select a largely common root microbiome) (Sanchez-Canizares et al., 2017). A large number of rice genotypes, including some landraces, were selected and characterized for high secretion of dicarboxylic acids into the rhizosphere (e.g., malate and citrate secretion), which will support higher levels of fixation by diazotrophs (N fixing bacteria). Five promising genotypes are now being studied for changes in bacterial community in the rice rhizosphere. Four rice varieties that differ in efficiency in N assimilation have been characterized for the endophytic bacterial community that were represented by about six genera and nine species. These strains exhibit plant growth promoting (PGP) traits, such as N fixation and secretion of auxins, and are now being characterized for their ability to promote assimilation of nitrogen fixed as ammonia. Genome sequencing has been done for different bradyrhizobial strains isolated from peanut/rice intercropped field. Six bradyrhizobial strains were isolated from rice roots (WBOS01, WBOS02, WBOS04, WBOS07, WBOS08, and WBOS16), and another six bradyrhizobial strains were isolated from peanut (WBAH10, WBAH23, WBAH30, WBAH33, WBAH41, and WBAH42). Both WBOS or WBAH type strains are able to nodulate peanut, however only WBAH strains do it in an effective way. Furthermore, both groups of strains promote growth on peanut and rice plants under control conditions (Guha et al., 2016).
Studies of genomes and phylogenetic structure of a large group of 96 elite rhizobial strains that infect pigeon pea are currently being conducted to understand the genetic structure of the bacteria and to mine for genetic determinants of competitiveness and effectiveness in nodulation. Alongside this, considerable progress has been made in understanding the mechanism of action of rice endophytes that are also able to nodulate peanut. These are novel strains whose genomes have been sequenced and their mechanisms of action are now being investigated. Considerable progress has also been made in screening lines for enhanced secretion of organic acids by rice which is capable of supporting elevated N fixation by endophytic diazotrophs.
2.2. Biological Nitrogen Use Efficiency
While agronomic measures help realize the innate genetic potential of the crop's NUE in the short term (see Section 2.3), biological crop improvement is necessary to break the agronomic barrier for further enhancement in the medium to long term (Mandal et al., 2018; Raghuram and Sharma, 2019). The lack of detailed biological understanding of the crop phenotypes and genotypes required for good N response and NUE has been a major limitation in the development of genetic approaches to improve the efficiency of utilization of fertilizer N. A number of phenotypic characteristics have been reported to be associated with N response and NUE. These include plant height and the timing of the onset of post-anthesis senescence in wheat (Subedi et al., 2007; Gaju et al., 2011), root length, root density and panicle density and form in rice (Morita et al., 1988; Yang et al., 2012; Sun et al., 2014; Peng et al., 2015; Rogers and Benfey, 2015; Steffens and Rasmussen, 2016). N-responsive changes in root system architecture have been the main focus of studies on N response and NUE in rice (Li et al., 2015, 2016). More extensive characterization of the phenotypic traits and components conferring efficient NUE is required for different crops.
It is important to address biological NUE at different levels—morphological, physiological, genetic, genomic, proteomic, or bioinformatics—and using different approaches. Insights from Arabidopsis and rice indicate the value of identifying contrasting lines based on their N-sensitivity or responsiveness to the measured growth parameters. Some contrasting lines of Indica rice have already been identified and compared for their N-responsive germination, growth, and yield parameters at normal and low N doses using urea and/or nitrate (Sharma et al., 2018). The lifelong evaluation of a few contrasting lines identified from the above study within NEWS enabled the shortlisting of additional traits for NUE in rice. While some of these parameters are commonly measured in relation to many agronomic traits, some are specific to NUE either individually or in combination. Further shortlisting of these parameters is necessary to screen germplasm on a large scale for crop improvement by selection or breeding. Work in CINTRIN is focussed on the branching response to N application which is well-defined in model species and could offer new avenues for manipulating crop N response.
Plants often modulate their root system architecture to compensate for the fluctuations of external N concentration in soil and the actual N demand to increase its N-uptake efficiency (Vidal and Gutiérrez, 2008; Krouk et al., 2010; Alvarez et al., 2012; Sinha et al., 2018). Therefore, for precise phenotyping of root system architecture traits (under optimum and starvation conditions), selected wheat genotypes were evaluated for modulation in root traits in hydroponics and N-free solid media (pot) at seedling stage under controlled conditions under the INEW VJC. Furthermore, in order to understand the genetic variability in nitrate-uptake capacity (considering both high and low affinity nitrate transporters in selected high and low yielding Indian and UK wheat genotypes), nitrate-flux were evaluated using 15N stable isotope as N source through isotope ratio mass spectrometry. The program also included characterization of the contrasting genotypes (selected based on field evaluation) for N-utilization efficiency traits using carbon and N metabolizing enzymes and their corresponding genes (Sinha et al., 2015; Gayatri et al., 2018). Results showed considerable genotypic variation for both the component traits, both at transcript as well as enzyme activity level. The practical relevance of these studies has been evaluated by growing selected genotypes in “precision nutrition” facilities, where fertilizer was applied in direct proximity to the roots by fertigation. Training of personnel was also carried out at the precision nutrition facility of the Borlaug Institute for South Asia, Ludhiana, India, to promote wider agronomic adoption, field validation, and use.
The establishment of diversity panels of rice, wheat, sorghum and millets, and the availability of associated genotyping or genomic information has provided a major resource for identifying significant marker-trait associations in available germplasm. Research conducted by NEWS found genotypic variation in NUE in 472 rice genotypes of Indian origin from the 3,000 sequenced accessions from the International Rice Research Institute (Alexandrov et al., 2015) and the 260 strong Bengal and Assam Aus Panel (Norton et al., 2018). These are being assessed at graded N levels at the Indian Institute for Rice Research (IIRR) as part of the NEWS VJC, and the data are being used for genome wide association mapping. Field studies with 10 rice genotypes indicated 50% variation in NUE between genotypes. In pearl millet, a total of 25 million whole genome single nucleotide polymorphisms (SNPs) are being tested by CINTRIN for association with NUE-related traits on the association mapping panel. Similar approaches are being pursued in sorghum and wheat in CINTRIN for exploiting natural/synthetic variation for NUE and mapping of the related traits.
Using the N-responsive genes identified in rice by microarray analysis and literature sources, activation-tagged transgenic rice lines have been identified by NEWS so far for over 50 N-responsive genes, including some G-protein regulated genes. Additional lines are expected from the ongoing screening of the remaining activation tagged lines. These lines need to be confirmed for the overexpression of the N-responsive gene(s) before assessing their impact on N-response and/or NUE. The identification of specific N-responsive genes/alleles that contribute to NUE will open opportunities for marker development toward marker-assisted selection and/or breeding, or gene-editing of the target genes in popular high yielding lines for in situ genetic manipulation of NUE. The CINTRIN VJC is modifying a number of candidate NUE ideotype genes by gene-editing and over-expression to confirm their role in modulating N-response in both Brachypodium and wheat.
Although all plant organs contain the same complement of genome, both the expression of genes and the accumulation of proteins varies widely and therefore, proteomics (a study of proteins) can provide us with a biological snapshot of a tissue, organ, or organelle at a particular point in time. At the Aligarh Muslim University, under NEWS, a stage-specific, cultivar-specific and N treatment-specific proteomic analysis of rice cultivars was conducted. The leaf proteome analysis reported 34 polypeptides that were affected by N treatments. Four polypeptides showed differential expression as a genotypic effect irrespective of N treatments. Expression of some proteins changed consistently at different developmental stages of rice. These proteins are yet to be identified. The identities of proteins that account for variation between treatments, genotypes, and stages of development are of crucial value for shortlisting the target genes/proteins for crop improvement, in view of earlier results from this group (Hakeem et al., 2012, 2013).
In INEW, work has focussed on relating the phenological crop growth development of wheat to NUE using nested associated mapping (NAM) of lines grown in replicate field trials on three sites in India and two sites in the UK. Most of the lines are from populations derived from crosses between accessions from the A. E. Watkins collection, a global collection of landraces originating from the 1920s and 1930s (to maximize genetic diversity), and a European spring wheat, developed at the John Innes Center, UK. The selection of related lines which are adapted for growth in India and the UK allows the datasets from the two countries to be combined, increasing the power of the analysis. In addition, spring types of a composite cross of European elite wheats are being studied in both countries, and a diversity panel of Indian genotypes in India only. All these accessions have been SNP genotyped and quantitative trait loci (QTLs) are being mapped. These studies are being underpinned by laboratory analyses of root phenotypes and gene expression analyses. The relationship with the timing of N application and NUE is also being studied using the precision agriculture facilities at the Borlaug Institute for South Asia (BISA).
2.3. Agronomic Nitrogen Use Efficiency and Environmental Impacts
An important step toward improving agronomic NUE is the development of crop genotypes with increased NUE under field conditions. The preliminary results from a major field experiment—conducted during the kharif season of 2016 at the research farm of the Indian Agricultural Research Institute (IARI), New Delhi, India, as part of the research program of NEWS—tested 10 rice genotypes for the most common NUE-related indices. Preliminary results from this analysis showed that the lines Nagina-22, Himdhan, and Taipe-309, could be considered as the most efficient N users. Compared to other genotypes, the values of grain yield were higher in these varieties with higher NUE (Figure 3), while MTU-1010 had the highest harvest index. Furthermore, these efficient N user genotypes showed higher agronomic efficiency (AE), physiological efficiency (PE), and partial factor productivity (PFP) of applied N in rice (Figure 4, see the caption for the definition of AE, PE, and PFP). Further investigations are underway to quantify how much N can potentially be saved nationally by using such genotypes.
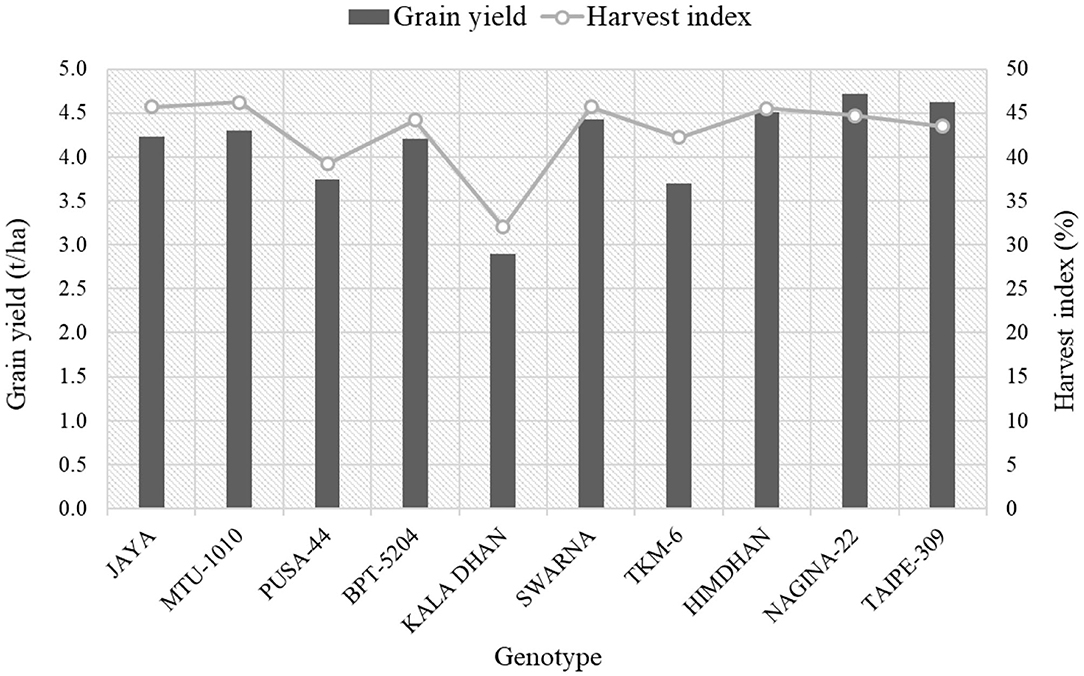
Figure 3. Grain yield and harvest index of different rice genotypes during the 2016 kharif season (by IARI under NEWS).
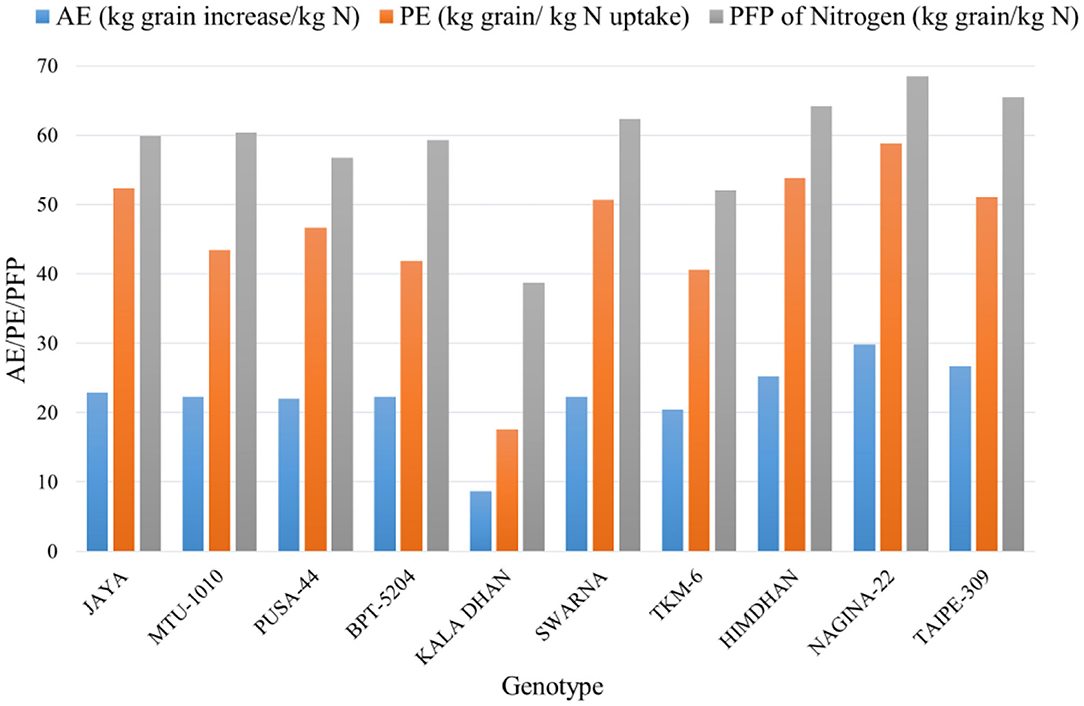
Figure 4. Agronomic efficiency (AE = [Grain yield (kg/ha) in N treated plot – Grain yield (kg/ha) in control plot]/N rate (kg/ha)), physiological efficiency (PE = [Grain yield (kg/ha) in N treated plot – Grain yield (kg/ha) in control plot]/[N uptake (kg/ha) in N treated plot – N uptake (kg/ha) in control plot]) and partial factor productivity [PFP = Grain yield (kg/ha)/N rate (kg/ha)] of applied N in different rice genotypes during the 2016 kharif season (by IARI under NEWS).
Another significant approach to improve agronomic NUE is to match fertilizer application rates to the actual needs of the plants. This can be identified by monitoring the color of the leaves (i.e., a proxy for chlorophyll content). For this purpose, one of the simplest and probably the cheapest option (costing about 100 Indian rupees or £1) is to use a leaf color chart (LCC). An LCC is a plastic color palette that can help farmers to determine the N needs of the plant based on the appearance of the crop. The concept of deciding fertilizer N application timings based on the greenness of the leaves was initially introduced for rice by the International Rice Research Institute (IRRI), Philippines (IRRI, 1996). Figure 5 shows an LCC refined by the Punjab Agricultural University (PAU) within CINTRIN, referred to as the “PAU-LCC.” In case of this LCC, farmers can access the decision support system based on the “PAU-LCC” recommendations at their smart phones through “PAU Urea Guide” application freely available both for Android and IOS users. Other versions of the LCC are available from the National Rice Research Institute (NRRI, India) and IIRR as well, where the colors are optimized for different crop contexts. The main advantage of the LCC approach apart from its low price, is that farmers can be easily trained to use the tool so that they can work out themselves the need-based N fertilizer dose, independently from the crop type, the season or soil type, without any analytical laboratory procedures or use of technology requiring a reliable power supply or calibration.
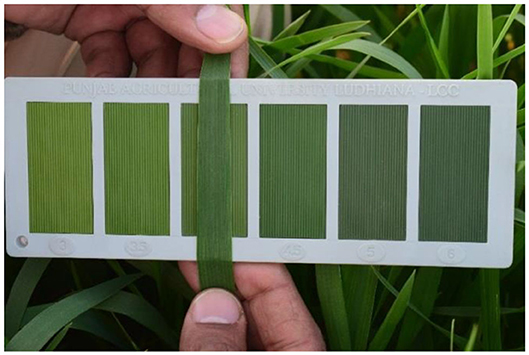
Figure 5. Leaf color chart as implemented by the Punjab Agricultural University within CINTRIN (“PAU-LCC”).
The LCC technology has been demonstrated to produce potential yields with low N optimum dose in transplanted rice (Varinderpal-Singh et al., 2007, 2014), maize (Varinderpal-Singh et al., 2011, 2014), wheat (Varinderpal-Singh et al., 2012, 2017), direct seeded rice, basmati rice, and cotton (PAU, 2018). Under CINTRIN, a village (Bassian, Ludhiana) has been developed as a role model village in the Indian state of Punjab with the active support of an NGO, the Atam Pargas Social Welfare Council. The farmers of the village harvested equivalent yields with the saving of an average of 75 kg N/ha for rice (2017) and 50 kg N/ha for wheat (2017–18) compared to conventional farmers' practice. The positive effects of the LCC approach were also highlighted by the findings of Bhatia et al. (2012). In this study, when 120 kg/ha urea was applied to rice using the LCC approach, the yield was 12–24% (depending on the LCC scores used) larger than in the case when the same amount of fertilizer was applied in the conventional way, in three equal splits at fixed times.
Although the LCC approach is easily accessible for farmers, computer-based tools (so-called “microcontroller-based” tools) may provide a more accurate solution. These tools are currently relatively expensive (about £25); however, work is ongoing within NEWS to make these approaches more affordable to farmers, such as in the “Green-Check” crop health meter of IARI. In the case of all the mentioned tools, further research should investigate the social barriers of adaptation of such methods by farmers.
It is important to note that although the crop N supply mainly depends on the N requirement of the crop, climatic, and environmental factors can play an important role in influencing NUE (Sharma and Bali, 2018). The amount of applied N that will be taken up by crops or lost from the soil-plant system depends not only on the soil (texture, pH, and moisture content) and the available water (amount, salinity) but also the atmospheric factors, such as temperature, rainfall, and wind speed (Cameron et al., 2013; Fagodiya et al., 2020). Timing of rainfall and irrigation are key factors in determining NUE (Abebe and Feyisa, 2017). If the N application coincides with a rainfall event, most of the applied N is lost as runoff, lowering NUE. In case of a sandy soil, the applied N fertilizer may be leached below the root zone with the rainfall or heavy irrigation, again reducing the NUE. If urea N is applied to dry soil with high pH, then much of the N applied may be lost as ammonia. Thus, for improving NUE, not only the crop (growth stage, variety), but also environmental factors need to be considered.
A method to improve agronomic NUE across the whole farm is by reducing losses of N through organic wastes (manures, urine, and crop residues), by promoting their uptake as key sources of plant nutrients. Within the NEWS VJC, work in the Mumtajpur village cluster (Pataudi Block in Haryana state) is experimenting to increase the quantity of N in organic wastes that is recycled to the soil, and to improve the quality of the organic fertilizers produced. This is being done by using a closed system to compost organic wastes instead of the traditional unlined pit or open manure heap. A polytex bag (3.7 × 1.2 × 0.6 m, Figure 6) is staked in the field and covered with a UV-protected transparent plastic sheet. The bag is layered with fresh cow dung, urine soaked bedding and soil, followed by single super phosphate, which helps reduce the loss of ammonia during decomposition. This sequence of layers is repeated until the bag is full, which usually takes about 30–35 days from three animals. Monitoring of greenhouse gas and ammonia emissions indicates lower emissions than in conventional practices (Figure 7—ammonia emission not shown). The organic fertilizer is ready to use after just 3 months of storage/preparation, and contains 0.82–0.89% N on a dry weight basis, compared to 0.35–0.5% N by traditional methods. Dry matter recovery is ~70% of the organic wastes, compared to 50–55% by conventional open manure storage. Organic fertilizers made by the improved and traditional methods are currently being trialed with vegetables, rice and wheat crops in farmers' fields.
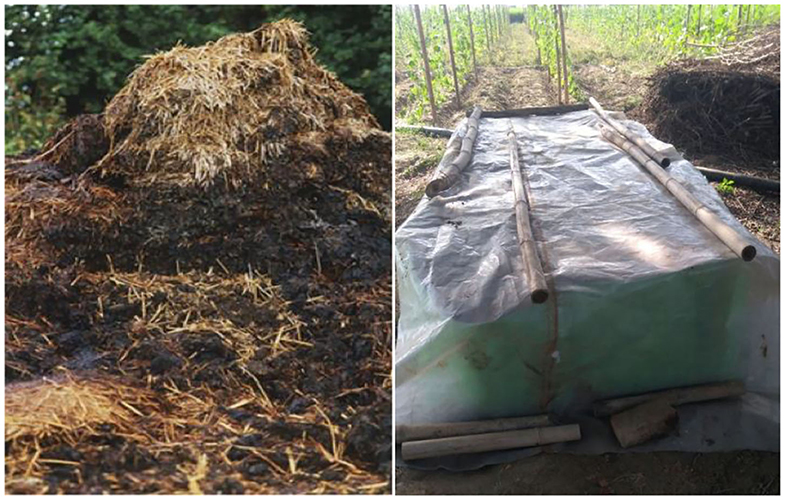
Figure 6. The conventional way of manure storage in a heap (on the left), and the improved way of farm yard manure making, using polytex bag at farmers' field in Mumtajpur, Pataudi Block, Haryana (on the right) (by IARI under NEWS).
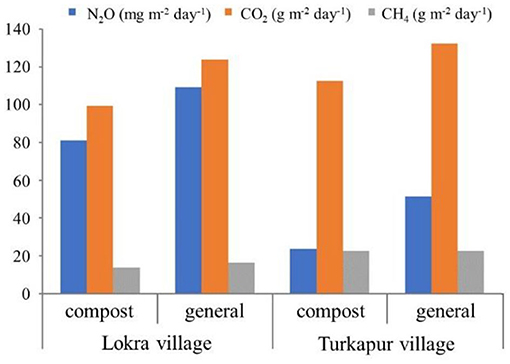
Figure 7. Measurement of N2O, CO2, and CH4 fluxes at two villages of Mumtajpur village cluster, Lokra and Turkapur, in the case of two different farm yard manure storage methods: composting using the improved composting method, in polytex bags (“compost”) and storing in a traditional open manure heap (“general”) (by IARI under NEWS).
Related to the environmental impacts of agronomic practices, at the conference, regional-scale studies using several chemistry transport models were also presented. These demonstrate that the examination of NH3 related atmospheric processes (emission, dispersion, chemical reactions, and deposition) over India is crucial. Figure 8 shows the average surface NH3 concentration in the summer derived by three models for 3 different years as follows:
- Figure 8A: EMEP model (Simpson et al., 2012) for 2015. The simulation was carried out by the global version of the EMEP model (version 4.10) with a resolution of 1°. The input meteorological data were derived by the WRF (Weather Research Forecast) weather forecast model (Vieno et al., 2016), and the emissions originate from the EDGAR (Emission Database for Global Atmospheric Research) database (for 2005).
- Figure 8B: UKCA model (Bellouin et al., 2011; O'Connor et al., 2014) for 2008. To obtain these results, UKCA was run with the aerosol scheme CLASSIC. The meteorology was simulated by the UM climate model and driven by sea surface temperatures and sea ice climatologies. Anthropogenic emissions were from HTAP v2.2 year 2008. Fire emissions were from VUA 1.2 Global Biomass Burning Emissions year 2008.
- Figure 8C: MOZART model (Emmons et al., 2010) for 2010. The input meteorological data set for driving the model was obtained from the MERRA (Modern Era Retrospective-analysis for Research and Applications) database of GEOS DAS (Goddard Earth Observing System Data Assimilation System) for the year 2010. For emissions, the HTAP_v2 (Hemispheric Transport of Air Pollution, Version 2) dataset was used.
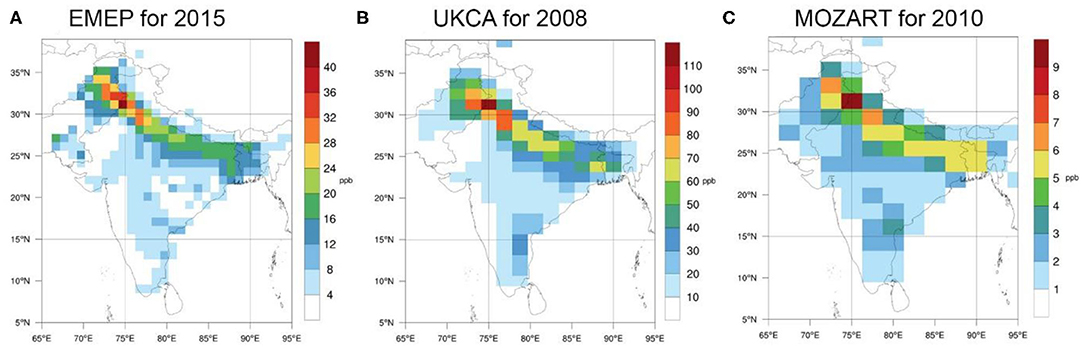
Figure 8. Average surface NH3 concentration (ppb) for the summer (June-July-August) of 2015 (A), 2008 (B) and 2010 (C), using the EMEP model, the UKCA model, and the MOZART model, respectively. For the specifications of these atmospheric chemistry transport models, see the main text.
Although in Figure 8, the different scales suggest very different surface concentration values, it is clear that all three models indicate greatly increased NH3 concentrations over the Indo-Gangetic Plain during summer, which is in agreement with earlier satellite observations (Clarisse et al., 2009). The difference between the models in the magnitude of concentrations could be caused by various model features, for example, the different heights of the surface layer, different dynamics, different chemistry schemes. The possible sources of differences in the resulting surface ammonia concentrations should be the scope of future model comparison studies, where the same emission datasets and the same input meteorological dataset should be used in order to avoid differences originating from the differences between these datasets. This comparison is important as satellite observations (Clarisse et al., 2009) indicate that the Indo-Gangetic Plain has the highest regional NH3 concentrations on Earth.
3. Options for Better N Management
3.1. Biological Nitrogen Fixation
The majority of Indian soils are low in soil organic matter (SOM) and soil N available for plants. A key way to increase SOM, and soil N, is to increase legume acreage and to encourage crop rotations with legumes, which also potentially improves soil biodiversity and ultimately, soil health. Planting a deep-rooted legume like pigeon-pea has potential to scavenge nitrate and phosphate and other nutrients from lower soil layers since its roots go as deep as 150 cm (Sheldrake and Narayanan, 1979). When planted legumes are fixing nitrogen optimally, they reduce the requirements of N fertilizers of the subsequent cereals planted due to the residual effect of the fixed N (Rao, 2014). The amount of nutrients in pigeon pea litter available as residual benefits to succeeding wheat crop have been estimated at about 40 kg N, 2 kg P, 7 kg K, and 2 kg S ha−1, respectively, in North India (Rao and Gill, 1995) which is known to farmers and the very reason for growing it to improve soil fertility.
In legumes, improving grain yields in tropical agriculture is best achieved by breeding genotypes under low-N conditions so as to select for high nodulators, employ best-bet agronomic practices and intercropping (Vanlauwe et al., 2019). A positive N balance can occur when there are no abiotic constraints or nutritional deficiencies that limit legume growth. Due to various constraints N fixation can be very low and there is hence a big range in pigeon pea BNF from 7 to 235 kg/ha/crop (Peoples et al., 1995) with the proportion of N fixation ranging from 10 to 81%. In a study in India, BNF by pigeon pea was low, 69 kg/ha and amounted to only 50% of plant N uptake (Kumar Rao and Dart, 1987). Improvement of N fixation under drought stress depends as much on host genotype selection as on rhizobial selection and improving soil organic matter and repeated inoculation. Crop rotation with grain legumes, forage, and other green manures builds SOM content and protects microbial biomass and diversity, populations, and their activity (Aparna et al., 2016). Such soil stewardship is essential to improve soil health and sustainability under intensive agriculture.
Currently, there is emerging enthusiasm among some farmers to avoid fertilizer entirely. A major regional example is the Zero Budget Natural Farming (ZBNF) system, which represents a system based on agroecological principles. One of the key features of the ZBNF movement of Andhra Pradesh is its rapid uptake by farmers, where the state policy aims that all six million farmers of Andhra Pradesh would practice ZBNF by 2024 (RySS, 2018). This movement takes the name (“zero budget”) from its focus to avoid all purchased inputs, such as pesticides and fertilizers. It aims to regenerate soil health by increasing SOM with a full dependence on BNF (and atmospheric inputs) for all Nr supply and thus make farming more profitable.
At present, the nitrogen performance of many different BNF-based farming systems, including ZBNF, remains to be quantified. Long-term nutrient budget studies are required to quantify nutrient inputs achieved through a mix of nitrogen fixing crops and intercrops in different contexts. For example, recent modeling assessment suggests that available nutrient sources in ZBNF examples maybe sufficient for extensive agricultural production but not sufficient to allow high output agricultural production, risking the potential for soil N depletion (Smith et al., 2020). Farmers may also supplement N inputs on their own or adopt hybrid models (Raghuram, 2020). Conversely, as BNF provides a gradual input of fixed N to the soil, it may be expected to lead to lower levels of surplus mineral N in the soil, suggesting that the fraction of N inputs that are lost to air and water pollution will be smaller than in fertilizer-based systems. However, this still needs to be tested, while plowing-in and decomposition of legume based green manures may nevertheless be associated with significant N leaching losses. Such knowledge would be useful in better understanding the system limitations and could guide further refinement of improved practices.
Under IUNFC, a strong focus of research has been given to understanding the controls in performance of pigeon pea. This crop is selected especially for its importance in dry land systems. There is a huge diversity of pigeon pea germplasm to exploit in various regions. Two hundred genomes of pigeon pea have been sequenced at the International Crops Research Institute for the Semi-arid Tropic (ICRISAT) in Hyderabad, which can be further studied. The translation of the findings described in Section 2.1, in terms of transferring the improved elite strains of pigeon pea rhizobia to farmers fields and similarly, the endophytes to rice farmers, are under testing in pan-India network field trials coordinated by the Indian Council of Agricultural Research (ICAR). Subsequently, these strains will be transferred to the inoculant industry for large scale multiplication and deployment.
India has a robust microbial inoculant industry (FAI, 2018) and the improved strains can easily be transferred to farmers for coating legume seeds both as carrier inoculant and liquid inoculants. Post-planting inoculation with liquid formulations of rhizobia is also possible and compensates partially for yield losses due to not inoculating the seeds at sowing. Similarly, the elite endophyte strains isolated in rice will eventually be deployed by the inoculant industry to inoculate and benefit not only the rice, but even the subsequent crops grown in rotation.
Work on other projects funded by the Bill and Melinda Gates Foundation (BMGF) and BBSRC/NSF may result in disruptive technologies for N utilization. BMGF has funded work on the expression of nitrogenase complexes in mitochondria and good progress has been made in the successful expression of the Fe protein in yeast mitochondria (López-Torrejón et al., 2016). This is still a medium-long term project, but it deserves careful consideration as it could be a game changer in the N economy of cereals. Likewise, BBSRC/NSF funded work on developing synthetic symbioses has made good progress in establishing signaling between plants and microbes, which is a prerequisite for controlled interaction between cereals and N fixing endophytes. If nodulation can be established in cereals then work on synthetic symbioses and nodulating cereals would come together.
3.2. Biological Nitrogen Use Efficiency
There is evidence that excessive application of N fertilizers harms yield, and attracts pests and diseases (Huber et al., 2012). This requires increased attention of future research programs. There are also major differences between crop types. For example it has been suggested that millets need relatively low levels of N input to fix carbon due to their physiological characteristics (Wang et al., 2018) compared with other cereals, and are also healthier on other accounts (Anuradha et al., 2017). Recognizing that it would require a re-design of supply chains, further investigation of the case to promote consumption of millets instead of rice and wheat is warranted to examine how this could contribute to increased NUE in the food chain. This is a major objective of the UK-India TIGR2ESS GCRF GROW project (TIGR2RESS, 2019).
In order to exploit better the biological potential for improving NUE in crops, genetic diversity from outside the standard breeders' germplasm should be further explored. This is particularly true for those crops (like rice and wheat in India) that are generally bred/selected under high N input conditions (Mandal et al., 2018; Raghuram and Sharma, 2019). Although significant steps have been made toward finding alleles that contribute to NUE (Section 2.2), some cultivars containing such alleles may either have been lost or screened out unintentionally in yield-centric screening programs that emphasized N-response over NUE (Mandal et al., 2018; Raghuram and Sharma, 2019). Landraces, such as the A.E. Watkins collection utilized in the INEW VJC, and wild varieties may bring in the missing alleles and give important clues toward finding new genetic avenues for improving NUE, thereby providing agronomists with better genotypes on which they can further optimize N management.
Efficient procedures for timely access to promising crop germplasm with reduced N demand could be a critical driver in this regard, as sometimes it may take a year or more to obtain seeds, especially from overseas. Establishment of low N-input breeding programs for all major N-fertilizer-consuming cereal crops and a system for efficiently sharing information and plant material are important policy aspects for better N management and increased NUE. Further investments are also needed in research toward phenotype and genotype characterization, and identification of contrasting genotypes for NUE. Field phenomics, and remote sensing, gene-editing and other emerging technologies can improve research efficiencies in phenotyping and early breeding.
3.3. Agronomic Nitrogen Use Efficiency and Environmental Impacts
Based on the key findings mentioned in Section 2.3, one of the most promising options to improve agronomic NUE is to monitor crop color, and to decide on the amount and timing of N application according to the appearance of the crop, using for example, LCCs, or electronic sensors. There is an opportunity and a need to compare the performance and limitations of a variety of LCCs such as those used by several research institutes (like PAU, IIRR, NRRI, etc.).
The preliminary national-scale model results clearly highlight the role of NH3 emission in atmospheric N pollution in India, especially in the context of worsening air quality across the Indo-Gangetic Plain. A possible option to reduce NH3 emission from urea application, could be to combine neem-coated (i.e., urea coated with neem oil, a natural nitrification inhibitor) with a urease inhibitor (“Neem Plus”), which could potentially increase NUE and decrease N losses. Currently, only artificial urease inhibitors are available in India, such as N-(n-Butyl) thiophosphoric triamide (NBPT), phenylphosphorodiamidate (PPD/PPDA), and hydroquinone. However, a plant-based urease inhibitor, similar to the neem oil used as nitrification inhibitor, would be expected to increase social acceptance. Thus, the great challenge is to find molecules that are of natural origin, have low toxicity, are chemically stable, efficient at low concentrations, and are competitively priced. Some natural plant phenolic products, such as protocatechuic aldehyde and vanillin derivatives, have been reported as having soil urease inhibition properties (Horta et al., 2016); however, this requires further investigation. Furthermore, future research should also examine the effectiveness of the usage of a urease inhibitor along with the neem coating of urea.
There are potential opportunities in integrating agronomic strategies (i.e., practice changes) with opportunities for increased biological NUE (i.e., plant genotypes with increased N efficiency). The extent of possible synergies between improved genetics and improved agronomy, and the extent to which agronomic changes need to be made to exploit the genetic potential are not yet known. In principle, there is a major opportunity to reduce the costs of agricultural production and levels of environmental pollution at the same time (Fageria and Santos, 2014). Future research should focus on integrating outcomes from breeding for higher NUE into field studies in relation to agronomic practices that demonstrate how environmental sustainability can go hand in hand with reduced and more efficient use of fertilizers (Ladha et al., 1998; Cheng et al., 2011).
Further work is also vital to improve recycling of organic wastes to the soil. This needs to consider crop residue, animal urine, and human excreta. The movement of produce to markets results in a year-on-year loss of N from the agricultural system, and leaves an excess of organic wastes in urban areas. Drying and pelletizing of composts, slurries from anaerobic digestion and biochar from pyrolysis of market wastes have a great potential to facilitate the return of N to agricultural areas. Development of technologies is also needed to avoid loss of NH3 during drying of these products. There is ongoing work in upscaling nutrient recovery, such as by ammonia stripping from the liquid remaining following anaerobic digestion of organic material (Buckwell and Nadeu, 2016), which may provide a major opportunity to include recycled N in new fertilizer products.
Finally, substantial improvements could be achieved in NUE by combining agronomic research with research on the environmental and health impacts of N pollution from agriculture in order to develop a more holistic perspective. Scaling up this approach from farm level to national and food system level, in tandem with modeling the economic impacts could demonstrate the opportunities for India, with potential policy benefit. For example, the interventions to improve NUE at crop level and the recycling of organic wastes can result in the overall increase of NUE for the overall food chain. These interventions must be coupled with other solutions to limit N losses from other stages of the food production systems, such as avoidance of post-harvest losses or reduction of food wastes and losses (HLPE, 2014; Uwizeye et al., 2016).
4. Actionable Recommendations: Global Goals for 2030
Based on the latest research results and the possible options for better N management in Indian agriculture, the participants of the workshop identified a set of global goals related to all three topics for 2030.
For BNF, one of the most important goals is to extend knowledge on how legumes can be used to improve NUE. Firstly, the research has identified the need to establish a pan-Indian, virtual or physical center for legume research. As indicated in Section 2.1 pigeon pea has a large potential to enhance NUE. Currently, this crop has an average yield of 850 kg/ha. Secondly, the workshop agreed a goal for 2030 is to double or even triple this yield by 2030. Along with these aims, it is reasonable to set a third, more general goal, namely, by 2030, to provide a substantial amount of cereal N via synthetic N symbioses and/or plastidic N fixation (Section 3.1).
As mentioned in Section 2.2, numerous traits have been identified for NUE in rice, wheat, sorghum and millets. These promising results represent clear steps for the future. Based on this, one of the goals set in terms of improving biological NUE is to better define the biological signatures of crop N status, including some that are farmer-friendly. The findings presented at the conference clearly support the biological potential for NUE improvement and encourage information and germplasm exchange. As such, the goal set for 2030 is to increase biological NUE by at least 20–30%.
The latest research clearly shows the success of leaf color-based fertilizer application methods, especially that of the LCC approach (Section 2.3), in improving NUE on arable farms. Therefore, one of the main goals for 2030 is to ensure that all arable farmers use LCC or other sensor technologies to decide on N applications. In the first instance, the focus is on conventional farmers optimizing their use of fertilizer to avoid excessive and wasteful inputs. However, there may also be interest in considering the use of leaf color sensing technology in relation to organic farming. Such an approach would require a plant available source of organic fertilizer N that could provide rapid plant response following application, such as is provided by bioslurry. Conversely, an LCC could be used to test for N deficiency in BNF based cropping systems.
To meet the goal for leaf color sensing by 2030 such tools should be provided to every farmer in India, along with documented success stories. With these technologies, based on expert judgement, 20–25% N could be saved (Lassaletta et al., 2014; Zhang et al., 2015). The positive outcomes of the presented research (Section 2.3) also allow a goal to be set for farms with livestock production. An important goal would be to re-use all manure and urine to maximize their fertilizer value, allowing reduced fertilizer inputs and subsidy savings. Future research should explore the social barriers of the application of these measures, building on the ongoing work of the UKRI GCRF South Asian Nitrogen Hub (SANH) and the International Nitrogen Management System (INMS). The expected increase in NUE using these approaches could be 5–10%, which could also lead to a proportional reduction in N fertilizer requirement. Large-scale social movements that focus on BNF rather than mineral fertilizers, may have a very important part to play in achieving the necessary transition to an India characterized by high NUE, low N pollution, and improved livelihoods.
Finally, the results (Section 2.3) highlight the role of NH3 in the N pollution of India. In order to manage the high levels of NH3 emissions it is recommended that, by 2030, all urea products should be both neem-coated and include a urease inhibitor (“Neem Plus”). This option is expected to offer a 5% N saving over neem-coated urea (which already achieves a 5–10% savings above uncoated urea). Since natural additives are culturally better accepted in India, the ultimate goal should be the development of viable plant-based urease inhibitors.
While we identified specific measures of key importance, we recognize for a wider nitrogen cycle perspective that there is a need to reduce overall nitrogen losses from agriculture, in addition to NH3 loss, including N2O, NO, and N2 emissions, as well as nitrate and other forms of nitrogen leaching. Each of these losses is driven by different processes. For example, ammonia loss is driven by immediate excess ammonium levels after fertilization with urea or organic sources (urine, manure, etc.). Conversely, losses of N2O, NO, and N2 tend to be more driven by excess nitrogen availability in the soil (beyond plant needs), while nitrate leaching is substantially exacerbated during periods of bare soil. This means that there is a need for further research to develop coherent “packages of measures” that seek to reduce multiple forms of nitrogen loss (and their impacts) simultaneously, thereby also providing opportunities to improve NUE (TFRN, 2020).
Altogether, this suite of changes would be expected to make a major contribution to the recently agreed commitment of the International Nitrogen Initiative “to support a global goal to halve nitrogen waste by 2030” (NEWS, 2018). How the different elements fit together toward a coherent holistic N approach should be the subject of future research in interaction with farmers, policy makers and wider society. This should include identification of the barriers to the widespread adoption of the above solutions/measures at the social, economic and policy levels, as well as options for their mitigation. While the Indian Nitrogen Assessment has laid the ground for this work (Abrol et al., 2017; Bhattacharya et al., 2017), further work can build on the ongoing work of SANH, INMS.
5. Conclusion
This paper brings together the main outcomes of the conference and workshop, “Challenges and Opportunities for Agricultural Nitrogen Science in India” organized in October 2017, in New Delhi, India. After sharing knowledge at the conference and working toward developing a joint vision on how agricultural nitrogen management could be made better in India at the workshop, the event concluded with three vision statements:
1. Stewardship is essential and legumes need to be planted in rotation with cereals. Synthetic symbioses and plastidic nitrogen fixation are possibly disruptive technologies; we must consider both their potential and implications.
2. Genetic diversity and new technologies need to be shared and exploited to reduce nitrogen fertilizer use for productive, sustainable agriculture livelihoods, and reducing the associated losses.
3. The use of leaf color sensing shows great potential to reduce nitrogen fertilizer use on-farm by 10–15%. This tool, together with the usage of urease inhibitors when using urea-based fertilizers, and better management of manure, urine and crop residues, could result in a 20–25% improvement in NUE of India by 2030.
Author Contributions
AM led the formulation, coordination, and writing of the manuscript. All authors contributed to manuscript content and revision.
Funding
Funding was provided by NEWS India-UK (BB/N013492/1), with contributions from CINTRIN (BB/N013441/1), INEW (BB/N013360/1) and IUNFC (BB/N013387/1) – all supported through the Newton-Bhabha Fund, by the UKRI and the Indian Department of Biotechnology –, as well as UK Natural Environment Research Council (NERC) as part of SANH (NE/S009019/1, supported through UKRI Global Challenges Research Fund), and SUNRISE (NE/R000131/1). The conference was supported by INMS (‘‘Targeted research for improving understanding of the global nitrogen cycle towards the establishment of an International Nitrogen Management System (INMS)'', 5400, supported by the Global Environment Facility and the United Nations Environment Programme).
Conflict of Interest
The authors declare that the research was conducted in the absence of any commercial or financial relationships that could be construed as a potential conflict of interest.
Acknowledgments
This paper is an outcome of the joint conference and workshop, Challenges and Opportunities for Agricultural Nitrogen Science in India supported by the UK Biotechnology and Biological Sciences Research Council (BBSRC), the UK Natural Environment Research Council (NERC), the Indian Department of Biotechnology (DBT), and INMS (Toward the Establishment of an International Nitrogen Management System). The authors are grateful for all the support they received for this overview paper from the four VJCs, under the lead of NEWS India-UK (BBSRC BB/N013492/1), with contributions from CINTRIN, INEW, and IUNFC, as well as the UKRI GCRF South Asian Nitrogen Hub, RySS and SUNRISE (Sustainable Use of Natural Resources to Improve Human Health and Support Economic Development). The paper is a contribution to the work of the International Nitrogen Initiative (INI). The development of the village Bassian as a role model village for adoption of PAU-LCC was voluntarily supported by the Atam Pargas Social Welfare Council.
Supplementary Material
The Supplementary Material for this article can be found online at: https://www.frontiersin.org/articles/10.3389/fsufs.2021.505347/full#supplementary-material
References
Abebe, Z., and Feyisa, H. (2017). Effects of nitrogen rates and time of application on yield of maize: rainfall variability influenced time of N application. Int. J. Agron. 2017:1545280. doi: 10.1155/2017/1545280
Abrol, Y. P., Adhya, T. K., Aneja, V. P., Raghuram, N., Pathak, H., Kulshrestha, U., et al. (2017). The Indian Nitrogen Assessment: Sources of Reactive Nitrogen, Environmental and Climate Effects, Management Options, and Policies. Amsterdam: Elsevier.
Abrol, Y. P., Raghuram, N., and Sachdev, M. S. (2007). Agricultural Nitrogen Use and Its Environmental Implications. New Delhi: IK International.
Alexandrov, N., Tai, S., Wang, W., Mansueto, L., Palis, K., Fuentes, R. R., et al. (2015). SNP-Seek database of SNPs derived from 3000 rice genomes. Nucleic Acids Res. 43, D1023–D1027. doi: 10.1093/nar/gku1039
Alvarez, J. M., Vidal, E. A., and Gutiérrez, R. A. (2012). Integration of local and systemic signaling pathways for plant N responses. Curr. Opin. Plant Biol. 15, 185–191. doi: 10.1016/j.pbi.2012.03.009
Aneja, V. P., Schlesinger, W. H., and Erisman, J. W. (2009). Effects of agriculture upon the air quality and climate: research, policy, and regulations. Environ. Sci. Tech. 43, 4234–4240. doi: 10.1021/es8024403
Aneja, V. P., Schlesinger, W. H., Nyogi, D., Jennings, G., Gilliam, W., Knighton, R. E., et al. (2006). Emerging national research needs for agricultural air quality. Eos Trans. Am. Geophys. Union 87, 25–29. doi: 10.1029/2006EO030001
Anuradha, N., Satyavathi, C. T., Bharadwaj, C., Nepolean, T., Sankar, S. M., Singh, S. P., et al. (2017). Deciphering genomic regions for high grain iron and zinc content using association mapping in pearl millet. Front. Plant Sci. 8:412. doi: 10.3389/fpls.2017.00412
Aparna, K., Rao, D. L. N., and Balachandar, D. (2016). Microbial populations, activity and gene abundance in tropical vertisols under intensive chemical farming. Pedosphere 26, 725–732. doi: 10.1016/S1002-0160(15)60079-0
Bellouin, N., Rae, J., Jones, A., Johnson, C., Haywood, J., and Boucher, O. (2011). Aerosol forcing in the Climate Model Intercomparison Project (CMIP5) simulations by HadGEM2-ES and the role of ammonium nitrate. J. Geophys. Res. Atmos. 116:D20206. doi: 10.1029/2011JD016074
Bhatia, A., Pathak, H., Jain, N., Singh, P. K., and Tomer, R. (2012). Greenhouse gas mitigation in rice-wheat system with leaf color chart-based urea application. Environ. Monit. Assess. 184, 3095–3107. doi: 10.1007/s10661-011-2174-8
Bhattacharya, S., Adhya, T. K., Pathak, H., Raghuram, N., and Sharma, C. (2017). “Issues and policies for reactive nitrogen management,” in The Indian Nitrogen Assessment: Sources of Reactive Nitrogen, Environmental and Climate Effects, Management Options, and Policies, eds Y. P. Abrol, T. K. Adhya, V. P. Aneja, N. Raghuram, H. Pathak, U. Kulshrestha, et al. (Cambridge: Elsevier),491–512.
Bijay-Singh (2017). “Management and use efficiency of fertilizer nitrogen in production of cereals in Indiad issues and strategies,” in The Indian Nitrogen Assessment: Sources of Reactive Nitrogen, Environmental and Climate Effects, Management Options, and Policies, eds Y. P. Abrol, T. K. Adhya, V. P. Aneja, N. Raghuram, H. Pathak, U. Kulshrestha, et al. (Cambridge: Elsevier), 9–28.
Bijay-Singh and Yadvinder-Singh (2008). Reactive nitrogen in Indian agriculture: inputs, use efficiency and leakages. Curr. Sci. 94, 1382–1393. Available online at: http://www.jstor.org/stable/24100495
Buckwell, A., and Nadeu, E. (2016). Nutrient Recovery and Reuse (NRR) in European agriculture. A Review of the Issues, Opportunities, and Actions. Brussels: RISE Foundation.
Cameron, K., Di, H., and Moir, J. (2013). Nitrogen losses from the soil/plant system: a review. Ann. Appl. Biol. 162, 145–173. doi: 10.1111/aab.12014
Chalk, P. M., He, J.-Z., Peoples, M. B., and Chen, D. (2017). 15N2 as a tracer of biological N2 fixation: a 75-year retrospective. Soil Biol. Biochem. 106, 36–50. doi: 10.1016/j.soilbio.2016.12.010
Cheng, J.-f., Jiang, H.-y., Liu, Y.-b., Dai, T.-b., and Cao, W.-x. (2011). Methods on identification and screening of rice genotypes with high nitrogen efficiency. Rice Sci. 18, 127–135. doi: 10.1016/S1672-6308(11)60018-8
Clarisse, L., Clerbaux, C., Dentener, F., Hurtmans, D., and Coheur, P.-F. (2009). Global ammonia distribution derived from infrared satellite observations. Nat. Geosci. 2, 479–483. doi: 10.1038/ngeo551
EDGAR (2016). Emissions Database for Global Atmospheric Research v4.3.1. Available online at: http://edgar.jrc.ec.europa.eu/overview.php?v=431 (accessed February 15, 2018).
Emmons, L. K., Walters, S., Hess, P. G., Lamarque, J. F., Pfister, G. G., Fillmore, D., et al. (2010). Description and evaluation of the Model for Ozone and Related chemical Tracers, version 4 (MOZART-4). Geosci. Model Dev. 3, 43–67. doi: 10.5194/gmd-3-43-2010
Fageria, N. K., and Santos, A. B. (2014). Lowland rice genotypes evaluation for nitrogen use efficiency. J. Plant Nutr. 37, 1410–1423. doi: 10.1080/01904167.2013.868482
Fagodiya, R. K., Kumar, A., Kumari, S., Medhi, K., and Shabnam, A. A. (2020). “Role of nitrogen and its agricultural management in changing environment,” in Contaminants in Agriculture, eds M. Naeem, A. Ansari, and S. Gill (Cham: Springer) 247–270.
FAI (2020). Statistical Database: All-India Consumption of Fertiliser Nutrients - 1950-51 to 2018-19. Available online at: https://www.faidelhi.org/statistics/statistical-database (accessed September 10, 2020).
FAO (2016). United Nations Food and Agriculture Organization, Statistics. Available online at: http://www.fao.org/faostat/ (accessed October 15, 2016).
FAO (2018). The Future of Food and Agriculture - Alternative Pathways to 2050. Rome: United Nations Food and Agriculture Organization.
Fowler, D., Coyle, M., Skiba, U., Sutton, M. A., Cape, J. N., Reis, S., et al. (2013). The global nitrogen cycle in the twenty-first century. Philos. Trans. R. Soc. Lond. B Biol. Sci. 368:20130164. doi: 10.1098/rstb.2013.0164
Gaju, O., Allard, V., Martre, P., Snape, J. W., Heumez, E., Legouis, J., et al. (2011). Identification of traits to improve the nitrogen-use efficiency of wheat genotypes. Field Crop Res. 123, 139–152. doi: 10.1016/j.fcr.2011.05.010
Galloway, J., Raghuram, N., and Abrol, Y. P. (2008). A perspective on reactive nitrogen in a global, Asian and Indian context. Curr. Sci. 94, 1375–1381. Available online at: https://www.jstor.org/stable/2410049
Galloway, J. N., Aber, J. D., Erisman, J. W., Seitzinger, S. P., Howarth, R. W., Cowling, E. B., et al. (2003). The nitrogen cascade. BioScience 53, 341–356. doi: 10.1641/0006-3568(2003)053[0341:TNC]2.0.CO;2
Galloway, J. N., Townsend, A. R., Erisman, J. W., Bekunda, M., Cai, Z., Freney, J. R., et al. (2008). Transformation of the nitrogen cycle: recent trends, questions, and potential solutions. Science 320, 889–892. doi: 10.1126/science.1136674
Gayatri, Rani, M., Mahato, A. K., Sinha, S. K., Dalal, M., Singh, N. K., et al. (2018). Homeologue specific gene expression analysis of two vital carbon metabolizing enzymes-citrate synthase and NADP-isocitrate dehydrogenase-from wheat (Triticum aestivum L.) under nitrogen stress. Appl. Biochem. Biotechnol. Enzyme Eng. Biotechnol. 188, 569–584. doi: 10.1007/s12010-018-2912-2
Guha, S., Sarkar, M., Ganguly, P., Uddin, M. R., Mandal, S., and DasGupta, M. (2016). Segregation of nod-containing and nod-deficient bradyrhizobia as endosymbionts of Arachis hypogaea and as endophytes of Oryza sativa in intercropped fields of Bengal Basin, India. Environ. Microbiol. 18, 2575–2590. doi: 10.1111/1462-2920.13348
Hakeem, K. R., Chandna, R., Ahmad, A., Qureshi, M. I., and Iqbal, M. (2012). Proteomic analysis for low and high nitrogen-responsive proteins in the leaves of rice genotypes grown at three nitrogen levels. Appl. Biochem. Biotechnol. 168, 834–850. doi: 10.1007/s12010-012-9823-4
Hakeem, K. R., Mir, B. A., Qureshi, M. I., Ahmad, A., and Iqbal, M. (2013). Physiological studies and proteomic analysis for differentially expressed proteins and their possible role in the root of N-efficient rice (Oryza sativa L.). Mol. Breed. 32, 785–798. doi: 10.1007/s11032-013-9906-0
HLPE (2014). Food Losses and Waste in the Context of Sustainable Food Systems. A report by the High Level Panel of Experts on Food Security and Nutrition of the Committee on World Food Security, Rome.
Horta, L. P., Mota, Y. C. C., Barbosa, G. M., Braga, T. C., Marriel, I. E., de Fatima, A., et al. (2016). Urease inhibitors of agricultural interest inspired by structures of plant phenolic aldehydes. J. Braz. Chem. Soc. 27, 1512–1519. doi: 10.21577/0103-5053.20160208
Huber, D., Römheld, V., and Weinmann, M. (2012). “Relationship between nutrition, plant diseases and pests,” in Mineral Nutrition of Higher Plants, 3rd edn, ed. H. Marschner (Beijing: Science Press), 283–298.
IRRI (1996). Use of leaf colour chart (LCC) for N management in rice. Crop Resource. Manage Network Technol Brief 2, Manila, PH.
Krouk, G., Crawford, N. M., Coruzzi, G. M., and Tsay, Y.-F. (2010). Nitrate signaling: adaptation to fluctuating environments. Curr. Opin. Plant Biol. 13, 265–272. doi: 10.1016/j.pbi.2009.12.003
Kumar Rao, J. V. D. K., and Dart, P. J. (1987). Nodulation, nitrogen fixation and nitrogen uptake in pigeon pea (Cajanus cajan (L.) Millsp) of different maturity groups. Plant Soil 99, 255–266. doi: 10.1007/BF02370872
Ladha, J. K., Kirk, G. J. D., Bennett, J., Peng, S., Reddy, C. K., Reddy, P. M., et al. (1998). Opportunities for increased nitrogen-use efficiency from improved lowland rice germplasm. Field Crop Res. 56, 41–71. doi: 10.1016/S0378-4290(97)00123-8
Ladha, J. K., Tirol-Padre, A., Reddy, C. K., Cassman, K. G., Verma, S., Powlson, D. S., et al. (2016). Global nitrogen budgets in cereals: a 50-year assessment for maize, rice, and wheat production systems. Sci. Rep. 6:19355. doi: 10.1038/srep19355
Lassaletta, L., Billen, G., Grizzetti, B., Anglade, J., and Garnier, J. (2014). 50 year trends in nitrogen use efficiency of world cropping systems: the relationship between yield and nitrogen input to cropland. Environ. Res. Lett. 9:105011. doi: 10.1088/1748-9326/9/10/105011
Li, P., Chen, F., Cai, H., Liu, J., Pan, Q., Liu, Z., et al. (2015). A genetic relationship between nitrogen use efficiency and seedling root traits in maize as revealed by QTL analysis. J. Exp. Bot. 66, 3175–3188. doi: 10.1093/jxb/erv127
Li, X., Zeng, R., and Liao, H. (2016). Improving crop nutrient efficiency through root architecture modifications. J. Integr. Plant Biol. 58, 193–202. doi: 10.1111/jipb.12434
López-Torrejón, G., Jiménez-Vicente, E., Buesa, J. M., Hernandez, J. A., Verma, H. K., and Rubio, L. M. (2016). Expression of a functional oxygen-labile nitrogenase component in the mitochondrial matrix of aerobically grown yeast. Nat. Commun. 7, 11426. doi: 10.1038/ncomms11426
Mandal, V., Sharma, N., and Raghuram, N. (2018). “Molecular targets for improvement of crop nitrogen-use efficiency: current and emerging options,” in Engineering Nitrogen Utilization in Crop Plants, eds A. Shrawat, A. Zayed, and D. A. Lightfoot (Cham: Springer), 77–93.
Morita, S., Suge, T., and Yamazaki, K. (1988). The relationship between root length density and yield in rice plants. Jpn. J. Crop. Sci. 57, 438–443. doi: 10.1626/jcs.57.438
NEWS (2018). INI Commits to Support a Global Goal to Halve Nitrogen Waste by 2030. Available online at: http://news-india-uk.international/latest_news/our_ocean_conference_2018 (accessed February 11, 2019).
Norton, G. J., Travis, A. J., Douglas, A., Fairley, S., Alves, E. D. P., Ruang-areerate, P., et al. (2018). Genome wide association mapping of grain and straw biomass traits in the rice bengal and Assam aus panel (BAAP) grown under alternate wetting and drying and permanently flooded irrigation. Front. Plant Sci. 9:1223. doi: 10.3389/fpls.2018.01223
O'Connor, F. M., Johnson, C. E., Morgenstern, O., Abraham, N. L., Braesicke, P., Dalvi, M., et al. (2014). Evaluation of the new UKCA climate-composition model - Part 2: the troposphere. Geosci. Model Dev. 7, 41–91. doi: 10.5194/gmd-7-41-2014
Peng, X., Yang, Y., Yu, C., Chen, L., Zhang, M., Liu, Z., et al. (2015). Crop management for increasing rice yield and nitrogen use efficiency in northeast China. Agron. J. 107, 1682–1690. doi: 10.2134/agronj15.0013
Peoples, M. B., Herridge, D. F., and Ladha, J. K. (1995). Biological nitrogen fixation: an efficient source of nitrogen for sustainable agricultural production? Plant Soil 174, 3–28. doi: 10.1007/978-94-011-0053-3_1
Raghuram, N. (2020). Zeroing in on Farm Budgets or Zero Budget Natural Farming? A Perspective from India. Perspectives 37, UN Environment Programme. Available online at: https://www.unenvironment.org/resources/perspective-series/zeroing-farm-budgets-or-zero-budget-natural-farming-unep-perspective (accessed September 10, 2020).
Raghuram, N., and Sharma, N. (2019). “Improving crop nitrogen use efficiency,” in Comprehensive Biotechnology, Vol. 4, ed. M. Moo-Young (Pergamon: Elsevier), 211–220.
Rao, D. L. N. (2014). Recent advances in biological nitrogen fixation in agricultural systems. Proc. Indian Natl. Sci. Acad. 80, 359–378. doi: 10.16943/ptinsa/2014/v80i2/55114
Rao, D. L. N., and Balachandar, D. (2017). “Nitrogen inputs from biological nitrogen fixation in Indian agriculture,” in The Indian Nitrogen Assessment: Sources of Reactive Nitrogen, Environmental and Climate Effects, Management Options, and Policies, eds Y. P. Abrol, T. K. Adhya, V. P. Aneja, N. Raghuram, H. Pathak, U. Kulshrestha, et al. (Cambridge: Elsevier),117–132.
Rao, D. L. N., and Gill, H. S. (1995). Biomass production and nutrient recycling through litter from pigeonpea (Cajanus cajan L. Millsp.). Bioresour. Technol. 54, 123–128. doi: 10.1016/0960-8524(95)00102-6
Rogers, E. D., and Benfey, P. N. (2015). Regulation of plant root system architecture: implications for crop advancement. Curr. Opin. Biotechnol. 32, 93–98. doi: 10.1016/j.copbio.2014.11.015
RySS (2018). Andhra Pradesh: India's 1st Natural Farming State. Zero-Budget Natural Farming, Guntur.
Sanchez-Canizares, C., Jorrin, B., Poole, P., and Tkacz, A. (2017). Understanding the holobiont: the interdependence of plants and their microbiome. Curr. Opin. Microbiol. 38, 188–196. doi: 10.1016/j.mib.2017.07.001
Sharma, L. K., and Bali, S. K. (2018). A review of methods to improve nitrogen use efficiency in agriculture. Sustainabiliity 10, 51. doi: 10.3390/su10010051
Sharma, N., Sinha, V. B., Gupta, N., Rajpal, S., Kuchi, S., Sitaramam, V., et al. (2018). Phenotyping for nitrogen use efficiency: rice genotypes differ in N-responsive germination, oxygen consumption, seed urease activities, root growth, crop duration, and yield at low N. Front. Plant Sci. 9:1452. doi: 10.3389/fpls.2018.01452
Sheldrake, A. R., and Narayanan, A. (1979). Growth, development and nutrient uptake in pigeonpeas (Cajanus cajan). J. Agric. Sci. 92, 513–526. doi: 10.1017/S0021859600053752
Simpson, D., Benedictow, A., Berge, H., Bergström, R., Emberson, L. D., Fagerli, H., et al. (2012). The EMEP MSC-W chemical transport model; technical description. Atmos. Chem. Phys. 12, 7825–7865. doi: 10.5194/acp-12-7825-2012
Sinha, S. K., Rani, M., Bansal, N., Gayatri, Venkatesh, K., and Mandal, P.K. (2015). Nitrate starvation induced changes in root system architecture, carbon:nitrogen metabolism, and miRNA expression in nitrogen-responsive wheat genotypes. Appl. Biochem. Biotechnol. Enzyme Eng. Biotechnol. 177, 1299–1312. doi: 10.1007/s12010-015-1815-8
Sinha, S. K., Rani, M., Kumar, A., Kumar, S., Venkatesh, K., and Mandal, P. K. (2018). Natural variation in root system architecture in diverse wheat genotypes grown under different nitrate conditions and root growth media. Theor. Exp. Plant Physiol. 30, 223–234. doi: 10.1007/s40626-018-0117-2
Smith, J., Yeluripati, J., Smith, P., and Nayay, D. R. (2020). Potential yield challenges to scale-up of zero budget natural farming. Nat. Sustain. 3, 247–252. doi: 10.1038/s41893-019-0469-x
Steffens, B., and Rasmussen, A. (2016). The physiology of adventitious roots. Plant Physiol. 170, 603. doi: 10.1104/pp.15.01360
Subedi, K. D., Ma, B. L., and Xue, A. G. (2007). Planting date and nitrogen effects on grain yield and protein content of spring wheat. Crop Sci. 47, 36–44. doi: 10.2135/cropsci2006.02.0099
Sun, H., Qian, Q., Wu, K., Luo, J., Wang, S., Zhang, C., et al. (2014). Heterotrimeric G proteins regulate nitrogen-use efficiency in rice. Nat. Genet. 46, 652. doi: 10.1038/ng.2958
Sutton, M. A., Bleeker, A., Howard, C. M., Bekunda, M., Grizzetti, B., deVries, W., et al. (2013). Our Nutrient World: The Challenge to Produce More Food and Energy With Less Pollution. Global Overview of Nutrient Management. Centre for Ecology and Hydrology on behalf of the Global Partnership on Nutrient Management and the International Nitrogen Initiative, Edinburgh.
Sutton, M. A., Drewer, J., Moring, A., Adhya, T. K., Ahmed, A., Bhatia, A., et al. (2017). “The Indian nitrogen challenge in a global perspective,” in The Indian Nitrogen Assessment: Sources of Reactive Nitrogen, Environmental and Climate Effects, Management Options, and Policies, eds Y. P. Abrol, T. K. Adhya, V. P. Aneja, N. Raghuram, H. Pathak, and U. Kulshrestha (Cambridge: Elsevier), 9–28.
Sutton, M. A., Howard, C. M., Erisman, J. W., Bealey, W. J., Billen, G., Bleeker, A., et al. (2011). “The challenge to integrate nitrogen science and policies: the European Nitrogen Assessment approach,” in The European Nitrogen Assessment: Sources, Effects and Policy Perspectives, eds M. A. Sutton, C. M. Howard, J. W. Erisman, G. Billen, A. Bleeker, and P. Grennfelt (Cambridge: Cambridge University Press), 82–96.
TFRN (2020). Guidance Document on Integrated Sustainable Nitrogen Management. UNECE Convention on Long-range Transboundary Air Pollution, Geneva. Available online at: http://www.unece.org/fileadmin/DAM/env/documents/2020/AIR/WGSR/Final_For_submission_Draft_guidance_23_June.pdf (accessed September 10, 2020).
TIGR2RESS (2019). TIGR2ESS: Transforming India's Green Revolution by Research and Empowerment for Sustainable food Supplies. Available online at: https://tigr2ess.globalfood.cam.ac.uk/ (accessed April 25, 2019).
Trotman, A. P., and Weaver, R. W. (1995). Tolerance of clover rhizobia to heat and desiccation stresses in soil. Soil Sci. Soc. Am. J. 59, 466–470. doi: 10.2136/sssaj1995.03615995005900020028x
Uwizeye, A., Gerber, P. J., Schulte, R. P. O., and de Boer, I. J. M. (2016). A comprehensive framework to assess the sustainability of nutrient use in global livestock supply chains. J. Clean. Prod. 129, 647–658. doi: 10.1016/j.jclepro.2016.03.108
Vanlauwe, B., Hungria, M., Kanampiu, F., and Giller, K. E. (2019). The role of legumes in the sustainable intensification of African smallholder agriculture: lessons learnt and challenges for the future. Agric. Ecosyst. Environ. 284:106583. doi: 10.1016/j.agee.2019.106583
Varinderpal-Singh, Bijay-Singh, Thind, H. S., Yadvinder-Singh, Gupta, R. K., Satinderpal-Singh, et al. (2014). Evaluation of leaf colour chart for need-based nitrogen management in rice, maize and wheat in north-western India. J. Res. Punjab Agr. Univ. 51, 239–245.
Varinderpal-Singh, Bijay-Singh, Yadvinder-Singh, Thind, H. S., Buttar, G. S., Kaur, S., Meharban, S., Kaur, S., and Bhowmik, A. (2017). Site-specific fertilizer nitrogen management for timely sown irrigated wheat (Triticum aestivum L. and Triticum turgidum L. ssp durum) genotypes. Nutr. Cycl. Agroecosys. 109, 1–16. doi: 10.1007/s10705-017-9860-z
Varinderpal-Singh, Bijay-Singh, Yadvinder-Singh, Thind, H. S., Gobinder, S., Satwinderjit, K., et al. (2012). Establishment of threshold leaf colour greenness for need-based fertilizer nitrogen management in irrigated wheat (Triticum aestivum L.) using leaf colour chart. Field Crop Res. 130, 109–119. doi: 10.1016/j.fcr.2012.02.005
Varinderpal-Singh, Yadvinder-Singh, Bijay-Singh, Baldev-Singh, Gupta, R. K.Jagmohan, S., et al. (2007). Performance of site-specific nitrogen management for irrigated transplanted rice in northwestern India. Arch. Agron. Soil Sci. 53, 567–579. doi: 10.1080/03650340701568971
Varinderpal-Singh, Yadvinder-Singh, Bijay-Singh, Thind, H. S., Kumar, A., and Vashistha, M. (2011). Calibrating the leaf colour chart for need based fertilizer nitrogen management in different maize (Zea mays L.) genotypes. Field Crop Res. 120, 276–282. doi: 10.1016/j.fcr.2010.10.014
Vidal, E. A., and Gutiérrez, R. A. (2008). A systems view of nitrogen nutrient and metabolite responses in Arabidopsis. Curr. Opin. Plant Biol. 11, 521–529. doi: 10.1016/j.pbi.2008.07.003
Vieno, M., Heal, M. R., Williams, M. L., Carnell, E. J., Nemitz, E., Stedman, J. R., et al. (2016). The sensitivities of emissions reductions for the mitigation of UK PM2.5. Atmos. Chem. Phys. 16, 265–276. doi: 10.5194/acp-16-265-2016
Wang, J., Vanga, K. S., Saxena, R., Orsat, V., and Raghavan, V. (2018). Effect of climate change on the yield of cereal crops: a review. Climate 6:41. doi: 10.3390/cli6020041
World Bank (2020a). Data: GDP (current US$). Available online at: https://data.worldbank.org/indicator/NY.GDP.MKTP.CD?locations=IN (accessed September 3, 2020).
World Bank (2020b). Data: Population, Total. Available online at: https://data.worldbank.org/indicator/SP.POP.TOTL?locations=IN (accessed September 3, 2020).
Yang, J.-c., Zhang, H., and Zhang, J.-h. (2012). Root morphology and physiology in relation to the yield formation of rice. J. Integr. Agric. 11, 920–926. doi: 10.1016/S2095-3119(12)60082-3
Keywords: nitrogen, nitrogen use efficiency, Indian agriculture, nitrogen management, fertilizer
Citation: Móring A, Hooda S, Raghuram N, Adhya TK, Ahmad A, Bandyopadhyay SK, Barsby T, Beig G, Bentley AR, Bhatia A, Dragosits U, Drewer J, Foulkes J, Ghude SD, Gupta R, Jain N, Kumar D, Kumar RM, Ladha JK, Mandal PK, Neeraja CN, Pandey R, Pathak H, Pawar P, Pellny TK, Poole P, Price A, Rao DLN, Reay DS, Singh NK, Sinha SK, Srivastava RK, Shewry P, Smith J, Steadman CE, Subrahmanyam D, Surekha K, Venkatesh K, Varinderpal-Singh, Uwizeye A, Vieno M and Sutton MA (2021) Nitrogen Challenges and Opportunities for Agricultural and Environmental Science in India. Front. Sustain. Food Syst. 5:505347. doi: 10.3389/fsufs.2021.505347
Received: 16 October 2019; Accepted: 08 January 2021;
Published: 18 February 2021.
Edited by:
Engracia Madejon, Institute of Natural Resources and Agrobiology of Seville (CSIC), SpainReviewed by:
Peter Sørensen, Aarhus University, DenmarkMaria Pilar Bernal, Spanish National Research Council, Spain
Copyright © 2021 Móring, Hooda, Raghuram, Adhya, Ahmad, Bandyopadhyay, Barsby, Beig, Bentley, Bhatia, Dragosits, Drewer, Foulkes, Ghude, Gupta, Jain, Kumar, Kumar, Ladha, Mandal, Neeraja, Pandey, Pathak, Pawar, Pellny, Poole, Price, Rao, Reay, Singh, Sinha, Srivastava, Shewry, Smith, Steadman, Subrahmanyam, Surekha, Venkatesh, Varinderpal-Singh, Uwizeye, Vieno and Sutton. This is an open-access article distributed under the terms of the Creative Commons Attribution License (CC BY). The use, distribution or reproduction in other forums is permitted, provided the original author(s) and the copyright owner(s) are credited and that the original publication in this journal is cited, in accordance with accepted academic practice. No use, distribution or reproduction is permitted which does not comply with these terms.
*Correspondence: Andrea Móring, QW5kcmVhLk1vcmluZ0BlZC5hYy51aw==; Claudia E. Steadman, Q2xhdWRpYS5TdGVhZG1hbkBlZC5hYy51aw==