- 1Facultad de Ciencias Agrarias, Departamento de Producción Animal, Universidad Nacional de Colombia, Medellín, Colombia
- 2International Center for Tropical Agriculture (CIAT), Cali, Colombia
- 3Mohammed VI Polytechnic University (UM6P), AgroBioSciences (AgBS), Agricultural Innovations and Technology Transfer Center (AITTC), Benguerir, Morocco
Pasture conditions influence the nutrients use efficiency and nitrogen (N) losses from deposited excreta. Part of the N is lost as nitrous oxide (N2O), a potent greenhouse gas. The objective of this study was to characterize apparent N recovery in milk of dual-purpose cattle and to quantify N2O emissions from the urine they deposit following grazing on Megathyrsus maximus cv. Mombasa. The N content in the grass and the milk produced by the cattle and the milk urea N (MUN) content were quantified in two contrasting regions of Colombia (Casanare and Atlántico). Dry matter intake (DMI) by the cattle was estimated using the Cornell Net Carbohydrate and Protein System. We used a closed static chamber technique to measure N2O emissions from soils in areas with and without urine patches (21 days in Atlántico and 35 Days in Casanare). Estimated DMI values were 11.5 and 11.6 kg DM day−1, milk production was 6.5 and 5.9 L day−1, apparent N recovery in milk was 24 and 23%, and the MUN content was 4.4 and 17.2 mg N dl−1 in Casanare and Atlántico, respectively. N applied to soil in the form of urine corresponded at rates of 20 and 64 g N m−2 and net cumulative N2O emissions were 350 and 20 mg N2O-N m−2 in Casanare and Atlántico, respectively. Despite low digestibility of offered diet, N recovery in milk was above the values reported at dairy cattle in tropical conditions. High urine-N inputs at Atlántico site did not result in high N2O emissions suggesting that the default Tier 1 emission factor (EF) which is based on N inputs would have overestimated urine-based N2O emissions in Atlántico. Comparing previous studies conducted in Colombia, we observed inter-regional differences by urine-based N2O emissions. This observation suggests that to increase certainty in estimating urine-based N2O emissions, Colombia needs to move toward more region-specific Tier 2 EF and reduce its dependence on the default IPCC Tier 1 EF. In addition, the adoption of Tier 2 EF in the cattle sector will facilitate accounting for the effect of animal diets on N2O inventories.
Introduction
Cattle production occupies 37 million ha and represents a major land-use option, which is a source of income and livelihood for small- to large-scale farmers in Colombia (FEDEGAN, 2018). In the case of Colombia's national greenhouse gas (GHG) inventories, total emissions are estimated to be 214 M tons CO2-eq of which the AFOLU sector is the main emitter that is responsible for 55% of these GHG emissions (IDEAM, 2018). Despite the significant contribution of the cattle sector to Colombia's national GHG emissions, the country still relies on the IPCC's global Tier 1 emission factors (EF) to estimate nitrous oxide (N2O) emissions from excreta deposited on pastures during cattle grazing.
Colombia's national cattle herd is estimated at 27 million animals (ICA, 2019), 41.4% dedicated to breeding, 37.3% to the dual-purpose (beef and milk) production, 21.2% to solely beef production, and 0.1% to milk production only (FEDEGAN, 2018). The cattle production systems are mainly extensively grazed systems based on native and naturalized pastures that are characterized by drastically low biomass production during the dry season (Mahecha et al., 2002). Extensive grazing systems are associated with low rates of live-weight gain and low milk outputs, which translate to lower profitability for livestock farmers (FAO, 2013). Since pasture management is generally absent in extensively grazed pastures, overgrazing, and signs of pasture degradation are common occurrences (Murgueitio and Ibrahim, 2001; Bacab et al., 2013). Animal excreta (i.e., urine) are randomly deposited in extensively grazed pastures, resulting in the formation of patches where N turnover and potential losses are high.
The loss of N from urine patches as NH3 or N2O depends on the pasture type, which influences N uptake, the urine composition, especially the amounts of excreted N (Voglmeier et al., 2018), which depends on efficiencies in the use of dietary N (Lessa et al., 2014; Rivera et al., 2019a). Part of the dietary N is retained in milk and other part is excreted in urine and dung. The amounts on N retained in milk depend on N intake; in systems characterized by high N intake the percentage of retained N in milk relative to the total consumed is low (Correa et al., 2012). Therefore, inefficiencies in apparent N recovery in milk will result in higher amounts of N being lost in urine and dung (Dijkstra et al., 2011). According to the IPCC (2019), N losses from excreta are between 40 and 70 kg of N head−1 year−1 for cattle, and the N2O-N EF for excreted N is correspondingly 0.2 and 0.6% under dry (<1000 mm rainfall) and wet (>1000 mm rainfall) climates. Cattle urine is a source highly soluble N, that stimulates nitrification processes and their subsequent transformation into N2O (Sordi et al., 2014). At the same time, it is also a volatile source of N depending on the ambient temperature and soil texture (Oenema et al., 2005). Several previous studies conducted in Latin America show considerable variations in N2O emissions from urine patches ranging between 0.7 and 1.16% (Kelliher et al., 2014; Lessa et al., 2014; Byrnes et al., 2017; Chirinda et al., 2019). However, in most of these studies, the peak emissions were reported to occur within the first 10–20 days after urine application and the emissions usually extend up to 30 days post-urine application. The pattern of urine-based N2O emissions is mainly regulated by microbial activity, mineral N, and oxygen dynamics (Rubol et al., 2013; García et al., 2014). Soil moisture is also a determining factor in the activity of soil nitrifying microorganisms (Oenema et al., 2005; Du et al., 2008; Laudone et al., 2011; Laville et al., 2011; Alves et al., 2012; Li et al., 2015; Marsden et al., 2016). In a study conducted under temperate conditions, Bell et al. (2015) reported that high soil moisture resulted in increased denitrification rates, and elevated N2O emissions from deposited animal excreta.
Globally, the general aims of the current forage genetic improvement programmes are to increase the quantity and quality of forage production and, consequently, increase cattle productivity in different agro-ecological regions. An example is Megathyrsus maximus (M. maximus), which produces between 20 and 30 t of DM ha−1 per year, has a protein level that fluctuates between 10 and 14% and a digestibility level between 60 and 70% (Arango et al., 2016). M. maximus has the potential to adapt to climate change, as it is a versatile pasture that adapts to intensive grazing and can be subjected to forage conservation processes in times of intense rainfall or prolonged periods of drought. However, information on greenhouse gas emissions in urine patches and bovine manure associated with this pasture is scant. Several studies have demonstrated the potential of M. maximus to increase dry matter consumption and increase animal productivity (Mahecha et al., 2007; Suárez et al., 2011; Gaviria et al., 2012; Rivera et al., 2019b). However, M. maximus is produced optimally under rotational compared to continuous grazing as, under the latter, the levels of insoluble fiber (lignin) can dramatically increase. An increase in the fiber content of grass reduces its digestibility and increases the amounts of excreted N in urine and manure (Barahona and Sanchez, 2005). A recent study (Villegas et al., 2020) showed that M. maximus has the potential to reduce nitrification rates by 50% compared to bare soil under controlled conditions. This finding suggests that M. maximus has the potential to increase cattle productivity and reduce the environmental impact of waste from animal production.
Due to the lack of data to generate local EF, Colombia relies on the IPCC's Tier 1 N2O-N EF to quantify emissions in this key emissions category. Regional differences in offered diets, plant N uptake rates, edaphic factors, and climatic conditions (Bell et al., 2015) generate variations in the primary drivers of soil N2O emissions. The determination of national N2O-N EF is required to advance toward the Tier 2 method of N2O emission estimation. We hypothesized that urine deposited on grazed pastures in the wetter Casanare region will have higher cattle urine-based N2O emissions compared to urine deposited on pastures in the drier Atlántico region. We also hypothesized that the low quality of the diet offered in dual-purpose production systems results in low concentrations of N in the urine of grazing cattle.
Materials and Methods
Study Sites
This study was conducted on two farms located in the Casanare (5°12'43“N−72°20'19.6”W 350 m altitude) and Atlántico (10°45'04.95“N−74°55'06.96”W 123 m altitude) regions of Colombia. According to the Köppen and Geiger classification (Kottek et al., 2006), the Atlántico and Casanare localities, correspondingly have Aw–Equatorial climate with dry winter and Am–tropical monsoon climatic conditions (Climate-data.org., 2008a). These regions are two of the most important cattle-producing regions of Colombia. The Atlántico has an average relative humidity of 78%, a mean air temperature of 27.4°C and a mean annual precipitation of 1,074 mm (Climate-data.org., 2008a). While, the Casanare region has an average relative humidity of 66%, a mean air temperature of 26.3°C, and a mean annual precipitation of 3,009 mm (Climate-data.org., 2008b). In 2015, when the current study was conducted, the Atlántico region experienced a severe drought attributed to the “El Niño” climate phenomenon (IDEAM, 2016), (IDEAM. Code: 29040020-Montebello Baranoa-Atlántico). During the measurement period, rainfall data was obtained from direct measurements conducted at the sites or from weather stations of the IDEAM Institute of Hydrology (Taluma site: code 35010010-Puerto López; Patía site: 21050220-San Luis).
Soil Properties
According to Gardi et al. (2014), the soils corresponding to the study sites are classified as Cambisols. Specifically, soils at the Atlántico site are classified as Vertic-Cambisols (CMVr) and those at Casanare are classified as Ferralic-Cambisols (CMFI).
At both sites, soil samples were collected at 0–20 cm depths and characterized for soil pH in 1:1 soil: water solutions, bulk density using a ring of known volume (5cm high × 5cm diameter), total carbon (C) and N using dry a combustion technique, and soil texture using the hydrometer method (Bouyoucos, 1962) at the analytical laboratory in CIAT. The water-filled pore space (WFPS) was calculated using the gravimetric water content, the soil bulk density, and a particle density of 2.65 Mg m−3.
Characteristics of Animals
In each region, 10 dual-purpose cows with commercial Zebu crosses were selected to be sources of the urine and milk used in the current study. These cows which were not permanently with their calves were in the second phase of lactation (100–200 days post-calving), with an average body weight of 475 ± 7.2 and 445 ± 4.6 kg in Casanare and Atlántico sites, respectively.
Dry Matter Intake
Dry matter intake of the cows in each region was estimated using the Cornell Net Protein and Carbohydrate System-CNCPS® version 6.0 model (Tylutki et al., 2008), which estimates beef and dairy cattle requirements and nutrient supply for different animal types under specific environmental conditions (i.e., climatic factors), management, and feeding regimes, through the computational engine. Cattle in Casanare were fed with tropical grass M. maximus cv. Mombasa (CIAT 6962), and those at the Atlántico site also fed on M. maximus cv. Mombasa (CIAT 6962) and a supplement that was based on barley, wheat, and corn grains and palm kernel cake. The supplement that was used at the Atlántico site was offered at a rate of 1 kg animal−1 day−1.
Chemical Composition and Digestibility of Pastures
For the collection of forage samples, the methodology described by Haydock and Shaw (1975) was used. In this method three reference points were established within the grassland. The first point corresponded to an area (0.5 m2 quadrats) where there was the lowest level of forage (quantity and height), point two was an area with intermediate forage production and point three was an area with the highest amount of forage. The grass samples were collected at the beginning of the experiment, before the animals accessed the grassland. Samples from the three reference points were mixed and the homogeneous sample was taken to the laboratory for the characterization of its chemical composition. The M. maximus pasture was harvested at 55 and 45 days of regrowth at the Casanare and Atlántico site, respectively. This regrowth age corresponds to the rest period of the pasture determined by the farmers in each study region, based on local experience with variables such as soil fertility, cattle stocking rate, and environmental parameters such as precipitation and temperature. At the Casanare and Atlántico sites, the pastures had an average height of 107 and 93 cm, respectively. To simulate grazing events pastures were cut at height of 30 cm from the ground.
For collected grasses were characterized for different nutritional quality parameters. Specifically, N content was quantified using the Kjeldahl method [Kjeldahl AN 3001 FOSS Association of Official Analytical Chemists (AOAC, 1990: method 984.14)] and the amount of N obtained from this analysis was converted to crude protein (CP) content, using the following equation: CP = N × 6.25; neutral detergent fiber (NDF) and acid detergent fiber (ADF) were determined as proposed by Van Soest et al. (1991) adapted to an Ankom Fiber Analyzer AN 3805 (Ankom® Technology Corp USA). Forage digestibility was determined as described by Goering and Van Soest (1970). The insoluble protein in neutral and acid detergent was determined through the N content in the residue of the NDF and ADF as described by Goering et al. (1972). Dry matter was determined through the thermogravimetric method by drying at 60°C for 72 h in a forced ventilation oven. These analyses were conducted at the Forage Quality and Animal Nutrition Laboratory of the International Center for Tropical Agriculture (CIAT).
Chemical Composition of Milk
At both study sites, we collected 50 ml milk samples from each of the 10 cows once every 8 days, for a period of 35 days. The milk was characterized for N content using the Kjeldahl method, CP was calculated using the following equation: CP = N × 6.38, and milk urea nitrogen (MUN) content was determined using Infrared Spectrometry (MilkoScan, ISO-9622 standard method) (Milk I. S. O., 2013). Apparent nitrogen recovery in milk was estimated from the efficiency of incorporation of consumed N in milk using the relationship described by Van Horn et al. (1994) which we expressed follow (Equation 1).
Experimental Set-Up for Soil N2O Measurements
At both sites, a representative area of 160 m2 was selected to conduct GHG measurements. In line with common farmer practice, no fertilizer was applied to the selected areas in recent years. To simulate grazing, grass in each plot was cut to ~30 cm sward height, seven days before the initial collection of gas and soil samples. In both regions, urine samples were collected through stimulation of the vulva zone from 10 dual-purpose cows. The samples obtained from each cow were immediately mixed to obtain a homogeneous sample. Urine sub-samples (500 mL) were immediately applied uniformly into the respective chambers (isolated area 0.5 m2). Cylindrical PVC static chamber bases with a 26-cm internal diameter and 10 cm height demarcated the area and the insertion depth was 5 cm. The urine-N application rates were 20 and 64 g N m−2 at the Casanare and Atlántico, respectively. These rates correspond to the N concentrations in urine resulting from different diets consumed at the two sites. At both study sites eight bases were used. Four bases with urine and four bases without urine as control treatments, therefore eight bases were installed in the soil at each site. The chamber bases were distributed in a completely randomized design with four replicates that were established on land with a slope ~2%.
On the different gas sampling dates, chamber tops with a 25-cm height were connected to the chamber bases and sealed with a thick custom-made rubber band to prevent gas leakage during the sampling period (Alves et al., 2012). Gas samples were collected from 9:00 to 10:00 am as described by Byrnes et al. (2017). The first gas sample was taken immediately after connecting the chamber top and the chamber base, subsequent samples were taken every 15 min resulting in sampling times of 0, 15, 30, and 45 min after chamber closure. Based on dynamic of urine-based N2O emissions reported by several studies carried out in Latin America (Kelliher et al., 2014; Lessa et al., 2014; Byrnes et al., 2017; Chirinda et al., 2019), in our study, the gas samples were collected on five consecutive days following urine application followed by 3–4 additional sampling campaigns within the 35-day monitoring period. Due to logistical challenges we were unable to conduct the gas monitoring during the planned 35 day period at the Atlántico site. We were only able to conduct measurements over 21 days.
The N2O concentrations were analyzed by gas chromatography (GC–Shimadzu 2014). The daily gas fluxes were calculated by regressing N2O concentrations with the corresponding sampling times and correcting for temperature and pressure. All flux data were checked for linearity by visual inspection during data analysis. Cumulative fluxes were calculated from mean N2O emissions by interpolation between measurement days (Dobbie et al., 1999). Specifically, we determined the mean N2O emissions between two consecutive sampling days and them the sum of the calculated averages for the monitoring period. The cumulative N2O-N EF for the Atlántico and Casanare sites were correspondingly calculated for periods of 21 and 35 days, using the following Equation:
At each site, a sub-sample of urine (50 mL) was acidified with 0.5 mL of 1 M sulphuric acid and frozen at −20°C to avoid N loss before analysis. Total N in the urine was quantified using the Kjeldahl method [Kjeldahl AN 3001 FOSS; Association of Official Analytical Chemists (AOAC, 1990: method 984.14)]. Urine N contents were used in the calculation of N2O EF (Equation 2).
Statistical Analyses
A completely randomized design was used, with four repetitions, where the experimental unit corresponded to the static chamber, where there were two treatments equivalent to soil with urine and without urine. Statistical analyses were conducted using generalized linear model (GLM) procedure of the statistical Software Statistical Analysis Systems (SAS ®, version 9,4) (SAS Institute, 2012). In the GLM model the response of cumulative N2O emissions to urine application was tested. Relationships between N2O emission and percentage of water-filled pore space (WFPS) as well as N2O emissions and rainfall were explored. The PROC REG procedure was used for regression analyses. The figures were constructed with python 2.7.14, Numpy 1.18.1, Scipy 1.4.1, and Motplotlib 3.2.1.
Results
Expected Dry Matter Intake and Feed Quality
Similar levels of dry matter intake were estimated for animals at the Casanare (11.5 kg DM d−1) and Atlántico site (11.6 kg DM d−1). The estimated energy balance from the CNCPS model was 2.44 and 3.34 Mcal in Casanare and Atlántico sites, respectively. The CP content of the M. maximus at Casanare and Atlántico site was similar (8%). However, due to the drought experienced at the Atlántico site, the animals received a supplement that had a high CP content (21%) (Table 1), which increased the amount of N intake around 20% by the animals at this site (Table 2). At both study sites, pasture digestibility was low (<50%) and NDF was high (>70%).
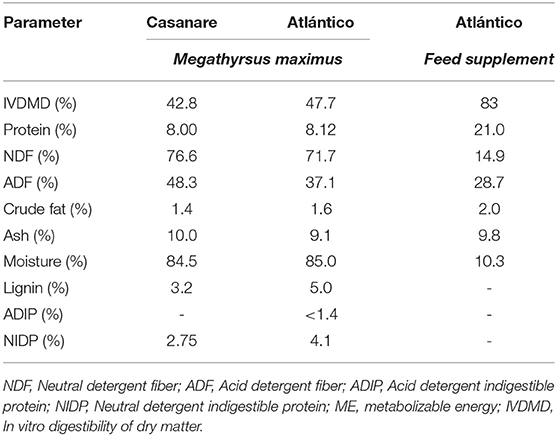
Table 1. Chemical composition of pastures and feed supplements collected in Casanare and Atlántico localities.
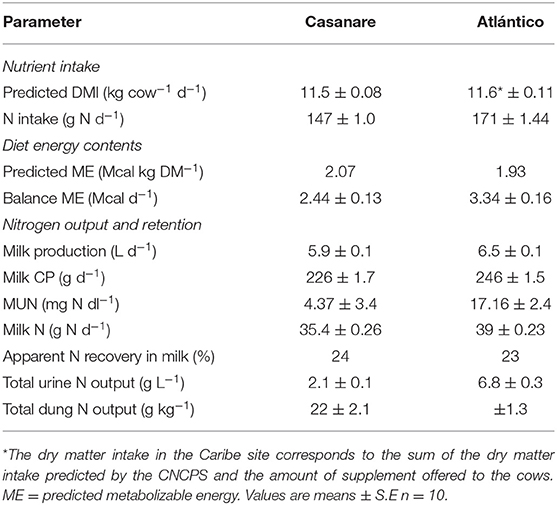
Table 2. Nitrogen intake, apparent nitrogen recovery in milk, and nitrogen content in urine and dung in dual-purpose cows in Casanare and Atlántico regions of Colombia.
Apparent Nitrogen Recovery in Milk and N Content in Urine
The 8-day average of N content measured in milk was similar at the Casanare and Atlántico sites. The relationship between milk N/ intake N showed that both regions had optimal apparent N recovery in milk values. The MUN concentrations of animals at the Atlántico site was above the range suggested by Peña (2002) and Cerón et al. (2014) (>16–18 mg dl−1). While at the Casanare site, the mean MUN concentration of animals was below the threshold values (<12 mg dl−1). The N content in urine at the Atlántico site was over three-fold higher than values reported at the Casanare site (Table 2).
Soil Properties
Soil pH was 5.2 and 6.7, bulk density was 1.3 and 1.4 g cm−3, and soil texture was clayey and loamy in the Casanare and Atlántico sites, respectively. Total N and total C content were similar at both sites (0.11 and 1.1%, respectively).
Nitrous Oxide Emissions
At both study sites, the N2O emission peaks were observed 3 days after the urine application, which also coincided with precipitation events (Figure 1). The application of urine in both regions generated an increase in the cumulative N2O fluxes compared to soil without urine (389 mg N2O m−2, p < 0.0001 and 27.6 mg N2O m−2, p < 0.0002 for Casanare and Atlántico sites, respectively) (Figure 1). A positive exponential relationship between N2O fluxes and %WFPS was found in the Casanare site (p = 0.027 R2 = 0.45). While in the Atlántico site this relationship was not significant (p = 0.176 R2 = 0.19).
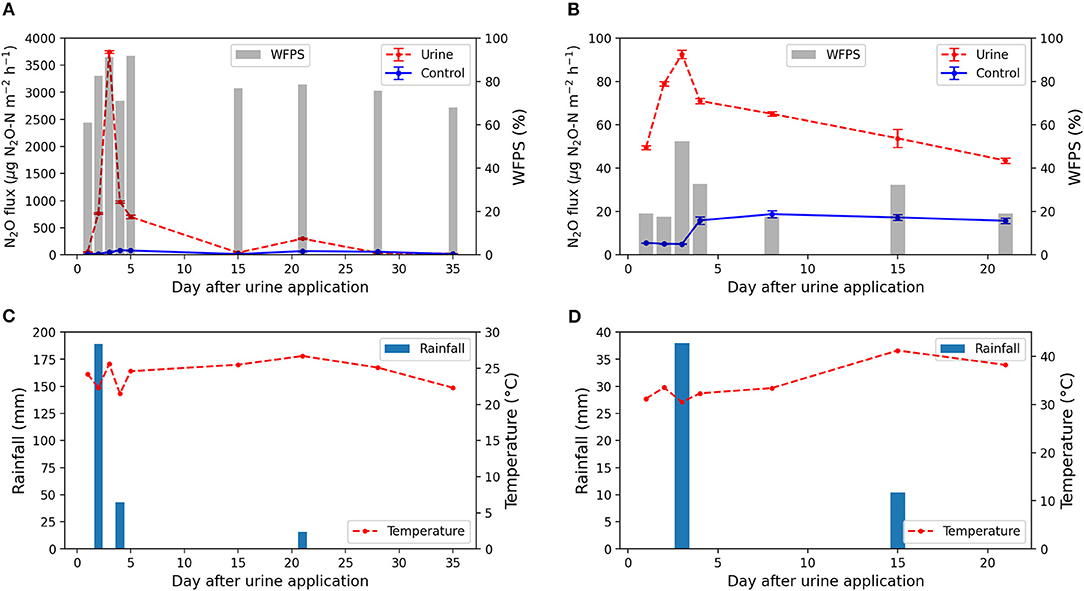
Figure 1. Soil nitrous oxide emission following urine application and percent water-filled pore space saturation (WFPS) (A-Casanare and B- Atlántico), and Rainfall and temperature (C-Casanare and D-Atlántico). Solid arrows indicate time of urine application. Vertical bars in (A) and (B) show the standard error (n = 4).
N2O-N Emitted From Urine Treatment
Overall, the different EF calculated in the present study and those from other studies conducted in Colombia showed variations in EF under different rainfall conditions (Table 3). Taking into account the results of this study and additional data available for Colombia (Table 3), we find evidence for a positive, non-linear relationship between rainfall and EF (p < 0.0001; R2 = 0.95) (Figure 2).
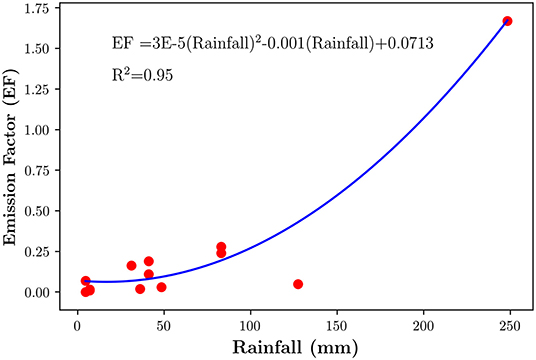
Figure 2. Relationship between EF and rainfall obtained using Byrnes et al. (2017), Chirinda et al. (2019), Durango (unpublished) data and measurements conducted in the current study. At each site, rainfall data includes recordings of precipitation events from 8 days before greenhouse gas measurement commenced to the end at the gas measurement period. The model was chosen based on statistical fit (R2).
Discussion
It is important to note that this study was conducted in an El niño year which was characterized by low rainfall. The unique climatic conditions associated with the El niño phenomenon influenced feed availability resulting in a need to supplement the grazing systems in the drier Atlántico region. Despite the fact that the El niño phenomenon was experienced across the country, differences in feed composition and rainfall intensities observed at the two study sites appear to have influenced the observed N2O emission patterns. The N concentration in applied urine would certainly influence N2O emissions, but calculating emission factor enabled us to normalize for N content. Another factor that may have affected our cumulative N2O emissions is the fact that due to logistical challenges we were unable to conduct N2O monitoring for the same period at the two study sites. However, since most of the peak emissions in the current and previous studies were reported to occur within the first 10–20 days (Kelliher et al., 2014; Lessa et al., 2014; Byrnes et al., 2017; Chirinda et al., 2019), our monitoring period allowed us to gain important insights on N2O emission patterns during this short-term monitoring period.
Expected Dry Matter Intake and N Intake
At both Casanare and Atlántico, the DM intake was similar. The similarity in DM intake may have been due to the similar NDF contents in the diet, a major determinant of intake in grazing cattle (Barahona and Sanchez, 2005). The digestibility values suggest that DM intake reported in this study is high, which may be due to a selectivity response of the animals, given the height of the forage that in both regions was >90 cm and the cutting height of the forage for the chemical composition analysis was 30 cm. The estimated N intake by cattle at both study sites (147 and 171 g N day−1 at the Casanare and Atlántico, respectively) were within the range frequently observed for open-grazing tropical cattle (40–170 g N day−1) (Cole and Todd, 2008; Nha et al., 2008). Higher N intakes (>300 g d−1) have been reported by other authors in cattle fed on fertilized pastures and feed supplements (Correa et al., 2012; Dickhoefer et al., 2018; Castro et al., 2019). Pastures in the tropics are typically characterized by low digestible protein and high fiber content (Mahecha and Rosales, 2005), partly due to poor agronomic management (Pelster et al., 2016), and this may be corrected through the use of protein supplementation. However, the low dry matter digestibility of pastures can exacerbate nutritional imbalance resulting in greater excretion of nutrients (Broderick, 2003; Barahona and Sanchez, 2005). Under the pasture conditions in our study, moderate N intake can be considered an advantage over excess protein in relation to the available energy that commonly occurs in tropical diets and can have a positive impact on N inputs to the soil, since according to Dijkstra et al. (2013), lowering intakes of CP is a strategy effective in reducing total and especially urinary N excretion.
Apparent Nitrogen Recovery in Milk
The MUN results reported for the Casanare site (4.37 mg dl−1) suggest that there was an inadequate amount of degradable protein ingested and that soluble carbohydrates may not have been balanced in the rumen. As MUN is the result of the diffusion of urea from the blood serum in the secretory cells of the mammary gland, MUN values are indicative of excess or deficiency of rumen available soluble carbohydrates, relative to dietary N (Peña, 2002). Conversely, at the Atlántico site, where cows received supplementation with a protein source, MUN values were above the range frequently observed in lactating cows under tropical conditions (Acosta and Delucchi, 2002; Peña, 2002). However, the MUN value reported for Atlántico does not suggest an excess of protein in the diet in comparison to the 10 and 19 mg dl−1 range reported by Hess et al. (1999), for dual-purpose cows in Colombia. Further research is required to understand the metabolic processes associated with the increased MUN observed at the Atlántico site. At both sites, the pastures contributed low amounts of N to the animal diets, which probably led to the inadequate synthesis of microbial protein, which in turn is the main metabolizable protein source for the animal (Agricultural Food Research Council, 1992; Ma et al., 2010).
Based on the average milk protein content observed in the current study, The amount of N retained from the total N ingested in the diet is on average 24 and 23% for at the Casanare and Atlántico, respectively, while 76 and 77% is excreted by feces and urine. This is consistent with the values reported by Colmenero and Broderick (2006) using multiparous cows 120 days post-calving, receiving low N diets. Correa et al. (2012) in a study carried out in Colombia with dairy cows, reported an apparent recovery of N in milk of 20% under following N intake of 389 g d−1. According to Steinshamn et al. (2006), the higher the N intake in the diet, the greater the excretion of N through the urine and the lower the percentage retained. León et al. (2008), reported N retention percentages in milk of 15.6% with respect to N intake of 667 g d−1. The N intake at both study sites was much lower than was reported by other authors. Consequently, we have found a higher percentage of N retained. Based on this information, the results found in this study suggest that the moderate protein intake of typical tropical diets such as M. maximus, can favor N retention in milk for cows in dual-purpose systems. However, other variables could have influenced the apparent N recovery in milk observed in the current study, such as the number of days since calving and the low level of milk production. In both regions, the cows had passed their peak lactation period, which has a higher energy requirement for milk synthesis. According to Lapierre and Lobley (2001), the recycling of urea N synthesized in the liver can substantially contribute to N availability in the intestines which mainly occurs when sufficient metabolizable energy is available in the diet. Kennedy and Milligan (1980) also indicated that urea transfer to the rumen is inversely proportional to the ammonium concentration in the rumen. It is likely that under these circumstances, the activation of urea N recycling mechanisms toward the rumen was stimulated, which may explain the high apparent nitrogen recovery in milk observed for both regions. In the same sense, the excretion of N in urine in both groups of animals did not exceed the range reported by Bolan et al. (2004) (1–20 g L−1).
Our results suggest that during this physiological stage (~100–200 days in lactation), the use of protein supplementation, which farmers consider as an important practice, may not have been necessary. This is in agreement with previous reports in the tropics, where energy availability can be a more limiting dietary factor for adequate animal productivity than CP availability (Barahona and Sanchez, 2005). Perhaps nutritional strategies such as reducing the levels of structural carbohydrates (e.g., ADF) in the diet (Table 1) through improved grazing management, (e.g., reducing grazing periods in rotational grazing systems) could increase feed digestibility as reported by previous studies evaluating pasture quality at different regrowth stages (Chacón and Vargas, 2009; Valles de la Mora et al., 2016). This would also contribute to an increase of milk yield as well as its compositional quality (Rabelo et al., 2003). These results suggest that with the diets offered in these milk production systems, and with cows in the second stage of lactation, urinary excretions of N were low. This implies a reduction in the substrate for soil nitrifying and denitrifying microorganisms.
N2O Fluxes Emissions
The cumulative net N2O emissions and the transformation rate of urinary N into N2O-N was very different at the Casanare (350 mg m−2; 1.76% EF) and Atlántico (20 mg m−2, 0.03% EF) sites. This supports the proposal that region-specific EF could greatly improve the accuracy of national GHG inventories. In addition, data generated during the different sampling campaigns aimed at developing region-specific EF could also be used to validate and improve Tier 3 models that are capable of integrating different factors regulating N2O emissions from urine patches such as the pasture type, climatic and soil conditions (Saggar et al., 2004; Mazzetto et al., 2014). At the Atlántico site, the cumulative N2O fluxes were lower compared to those reported in other previous studies conducted in the Latin America region [Sordi et al., 2014, (3,198 μg N2O-N m−2 h−1, 1,934 g N m−2); Simon et al., 2018 (3,700 μg N2O-N m−2 h−1, 256 g N m−2); Chirinda et al., 2019 (1,125 μg N2O-N m−2 h−1, 78.9 g N m−2)]. Several factors could have led to the lower N2O peaks including the low N application rate in the urine patched (Table 3), low precipitation regime and the low amounts of total carbon and N in the soil.
The peak N2O emissions observed at the Casanare site (3,745 μg N2O-N m−2 h−1) (Figure 1A) were lower than those reported by Byrnes et al. (2017) (25,000 μg N2O-N m−2 h−1) under similar tropical conditions. Rivera et al. (2019a) reported a higher peak N2O emissions value (9,450 μg N2O-N m−2 h−1) for intensively managed pastures that received external N inputs through chemical fertilization in the Andean region of Colombia. According to Anger et al. (2003), the activity of nitrifying and denitrifying microorganisms is much greater in soils with high N inputs compared to those where N availability is low; the latter was the case in the regions evaluated in the present study where the pastures did not receive N fertilizers prior the study. On the other hand, the high values of bulk density (≥1.3 g cm-3, Jaramillo et al., 2002), suggest lower aeration conditions, which according to Klefoth et al. (2014) reduce the diffusivity of N2O and increase the probability of its transformation to molecular N or dinitrogen (N2) possibly explaining the observed low N2O emission values. Skiba and Ball (2002), reported high N2O emissions in clay soils that were characterized by poor aeration (high %WFPS) and high bulk densities, conditions similar to those at the Casanare site.
Villegas et al. (2020) reported that the accession M. maximus cv. Mombasa can reduce nitrification rates by 50% when compared to a bare-soil control. They suggest that this reduction in nitrification rates is facilitated through the release of enzymatic complexes that inhibit the activity of bacterial nitrifiers. Brachiaria humidicola is another forage pasture species that has been reported to reduce soil nitrification by 60% (Subbarao et al., 2009). The ability of forage species to inhibit nitrification represents a potential N2O reduction mechanism. Megathyrsus' ability to inhibit nitrification, could have influenced the low cumulative fluxes observed in the current study.
Although in both regions the evaluation period corresponded to the end of the rainy season, in the Casanare site, a higher rainfall regime was experienced compared to the Atlántico region (Figures 1C,D). Consequently, soils at the Atlántico site had low vegetation cover. According to Oenema et al. (2005), between 3 and 15% of N in urine is lost as NH3 by volatilization, under conditions of high soil compaction, high temperatures, and low moisture; as prevalent at the Atlántico site. Additionally, Voglmeier et al. (2018), found a greater loss of N from urine as NH3 when the urine-N concentration was higher. These alternative N loss pathways probably resulted in lower amounts of N being available for nitrification and denitrification processes. On the other hand, according to Porre et al. (2016), the connectivity between pores and the size of the pore influence the N2O diffusivity and ultimate emission to the atmosphere. In our study, the soil texture was different between the sites. Specifically, whereas the Casanare soil had a clay texture, the soil at the Atlántico site was a loam. A clay texture supposes a combination of medium pores and micropores, which favors the water retention in the soil and inhibits the diffusion of O2 from the atmosphere, creating anaerobic microsites in the soil profile (Porre et al., 2016). While a loam soil would have higher macroporosity, with less moisture retention capacity due to higher sand and silt content and more aerobic conditions. Thus, the more clayey soil and frequent rainfall events at the Casanare site probably resulted in long periods with optimum moisture conditions for microbe-mediated N transformation processes in the deposited urine (Bateman and Baggs, 2005; Laudone et al., 2011; Dijkstra et al., 2013) and induced the higher N2O emissions observed at this site. This is consistent with the study by Adhikari et al. (2020), who reported less N2O emissions in soils with coarse (sandy) textures and in soils with free drainage compared to clay soils that were characterized by high bulk densities/compaction and subsequent elevated water-filled pore space. The positive relationship between the N2O emissions and % WFPS at the Casanare site (P = 0.027 R2 = 0.45) further provides evidence for the influence of water in driving the high N2O emissions observed at the Casanare site (Figure 1A). previous studies (Oenema et al., 2005; Laudone et al., 2011; Marsden et al., 2016) also reported the influence of %WFPS on denitrification processes. Specifically, N2O emissions associated with denitrifier activity are typically highest at values between 60 and 80% WFPS (Sey et al., 2008; Klefoth et al., 2014) with higher moisture contents leading to complete denitrification that produced dinitrogen as the end product.
There was no relationship between % WFPS and N2O emissions at the Atlántico site (P = 0.176 R2 = 0.19). The coarse-textured soil at the Atlántico site was more compacted which probably reduced water infiltration and moisture retention. This low infiltration and water retention rates probably explain the poor relationship between N2O emissions and % WFPS at the Atlántico site. These findings corroborate with Sordi et al. (2014) who suggested that the % WFPS, does not closely reflect the rain pattern, because the moisture in the soil depends on the evapotranspiration and the drainage between the rain event and the moment of taking the soil sample.
Based on the information from previous studies [Byrnes et al., 2017; Chirinda et al., 2019; Durango (unpublished)], as well as findings in the current study under tropical conditions, the rate of transformation of N in urine patches to N2O was found to be mainly influenced by soil moisture, associated with precipitation events (Figure 2). The loss N as N2O is affected by several factors, however, as has been previously discussed, these results suggest that under Colombian tropical condition, higher losses of N, in the form of N2O, appear to occur during the wet season. This result is consistent with studies where higher emissions have been found following high precipitation events (Bolan et al., 2004). In contrast, Sordi et al. (2014) obtained lower EF during the wet season, which they attributed to the lower temperatures recorded during the study period (13.9°C). However, in the evaluated regions, the average temperatures recorded are above (≥23°) those reported by Sordi et al. (2014).
The N2O-N EF obtained in the current study, corroborate with those reported in other studies developed under tropical conditions in unfertilized grasslands in Colombia [0.0002–0.471% EF Chirinda et al., 2019) and Brazil (0.2% EF. Barneze et al., 2014); 0.1–1.93% EF Lessa et al., 2014)]. The N2O-N EF obtained in the Atlántico region (0.03%) was lower than Tier1 EF given by the IPCC (2019) for these climatic regions (0.6% of N in bovine excreta).
Conclusions
The apparent nitrogen recovery in milk was similar at both sites and despite the low digestibility and high fiber content of the offered diets, the recovery of N in milk was above the values reported at dairy cattle under tropical conditions. The N concentrations in the applied urine were low compared to those reported in previous studies. This suggests that local cattle production systems may be characterized by low urine-N inputs which may imply potential low environmental impacts. On the other hand, the transformation rate of urinary N into N2O-N differed at the two locations although the same M. maximus grass cover was used. Our study provides further evidence on the need to determine site-specific EF as N2O emissions are affected by multiple biotic and abiotic factors.
Data Availability Statement
The original contributions presented in the study are included in the article/supplementary materials, further inquiries can be directed to the corresponding author/s.
Ethics Statement
Ethical review and approval was not required for the animal study because the study did not require approval by the ethics committee because the animals were not directly intervened, diets were not changed and only external samples such as milk, urine, and dung were taken. None of the activities compromised their animal health and welfare. The measurements carried out can be considered routine and qualified personnel were always present to take samples.
Author Contributions
SD conceived and designed the experiments, conducted laboratory analyses, and led the writing of the manuscript. SD, NC, RB, DB, JA, and LV, analyzed the data. All authors provided editorial advice and revised manuscript. All authors contributed to the article and approved the submitted version.
Funding
This work was implemented as part of the CGIAR Research Program (CRP) on Climate Change, Agriculture and Food Security (CCAFS), and the Livestock CRP which are carried out with support from CGIAR Fund Donors and through bilateral funding agreements. For details, please visit https://ccafs.cgiar.org/donors. We also acknowledge the financial assistance of BBSRC grant Advancing sustainable forage-based livestock production systems in Colombia (CoForLife) (BB/S01893X/1) and GROW Colombia from the UK Research and Innovation (UKRI) Global Challenges Research Fund (GCRF) (BB/P028098/1).
Conflict of Interest
The authors declare that the research was conducted in the absence of any commercial or financial relationships that could be construed as a potential conflict of interest.
Acknowledgments
We thank all donors that globally support the work of the program through their contributions to the CGIAR system. We would like to thank Arvey Alvarez and Aracelly Vidal for their technical assistance as well as Sandra Loaiza and Jonathan Nuñez for scientific support in performing this research.
References
Acosta, Y., and Delucchi, I. (2002). Determinación de urea en leche. Montevideo: Instituto Nacional de Investigación Agropecuaria.
Adhikari, K. P., Saggar, S., Luo, J., Giltrap, D., Berben, P., Palmada, T., et al. (2020). “Nitrous oxide emissions and emission factors from urine-deposited ‘hot-spots' in dairy pastures—winter trials,” in Nutrient Management in Farmed Landscapes, eds C. L. Christensen, D. J. Horne, and R. Singh (Palmerston North: Farmed Landscapes Research Centre, Massey University), 9 pages. Occasional Report No. 33. https://www.massey.ac.nz/~flrc/workshops/20/Manuscripts/Paper_Adhikari_2020.pdf
Agricultural Food Research Council (1992). Nutritive requirements of ruminant animal: protein. Nutr. Abstracts Rev. 62, 787–835.
Alves, B. J. R., Smith, K. A., Flores, R. A., Cardoso, A. S., Oliveira, W. R. D., Jantalia, C. P., et al. (2012). Selection of the most suitable sampling time for static chambers for the estimation of daily mean N2O flux from soils. Soil Biol. Biochem. 46, 129–135. doi: 10.1016/j.soilbio.2011.11.022
Anger, M., Hoffman, C., and Kühbauch, W. (2003). Nitrous oxide emissions from artificial urine patches applied to different N-fertilized swards and estimated annual N2O emissions for differently fertilized pastures in an upland location in Germany. Soil Use Manag. 19, 104–111. doi: 10.1079/SUM2003175
Arango, J., Gutiérrez, J. F., Mazabel, J., Pardo, P., Enciso, K., Burkart, S., et al (2016). Estrategias tecnológicas para mejorar la productividad y competitividad de la actividad ganadera: herramientas para enfrentar el cambio climático. Cali, CO: Centro Internacional de Agricultura Tropical (CIAT), 58. Retrieved from: www.ciat.cgiar.org (accessed March 11, 2021).
Association of Official Analytical Chemists (AOAC) (1990). Protein (crude) Determination in Animal Feed: Copper Catalyst Kjeldahl Method. (984.13), 15th Edn. Gaithersburg, MD: Official Methods of Analysis.
Bacab, H. M., Madera, N. B., Solorio, F. J., Vera, F., and Marrufo, D. F. (2013). Los sistemas silvopastoriles intensivos con Leucaena leucocephala: una opción para la ganadería tropical. Avances Invest. Agro. 17, 67–81. Retrieved from: https://www.redalyc.org/articulo.oa?id=83728497006
Barahona, R., and Sanchez, S. (2005). Limitaciones fìsicas y quìmicas de la digestibilidad de pastos tropicales. Rev. Corpoica 6, 69–82. doi: 10.21930/rcta.vol6_num1_art:39
Barneze, A. S., Mazzetto, A. M., Zani, C. F., Misselbrook, T., and Cerri, C. C. (2014). Nitrous oxide emissions from soil due to urine deposition by grazing cattle in Brazil. Atmos. Environ. 92, 394–397. doi: 10.1016/j.atmosenv.2014.04.046
Bateman, E. J., and Baggs, E. M. (2005). Contributions of nitrification and denitrification to N2O emissions from soils at different water-filled pore space. Biol. Fertil. Soils 4, 379–388. doi: 10.1007/s00374-005-0858-3
Bell, M. J., Rees, R. M., Cloy, J. M., Topp, C. F. E., Bagnall, A., Chadwick, D. R., et al. (2015). Nitrous oxide emissions from cattle excreta applied to a Scottish Grassland: Effects of soil and climatic conditions and a nitrification inhibitor. Sci. Total Environ. 508, 343–353. doi: 10.1016/j.scitotenv.2014.12.008
Bolan, N. S., Saggar, S., Luo, J. F., Bhandral, R., and Singh, J. (2004). Gaseous emissions of nitrogen from grazed pastures: processes, measurements and modelling, environmental implications, and mitigation. Adv. Agron. 84, 37–120. doi: 10.1016/S0065-2113(04)84002-1
Bouyoucos, G. J. (1962). Hydrometer method improved for making particle size analyses of soils 1. Agron. J. 54, 464–465. doi: 10.2134/agronj1962.00021962005400050028x
Broderick, G.A. (2003). Effects of varying dietary protein and energy levels on the production of lactating dairy cows. J. Dairy Sci. 86, 1370–1381. doi: 10.3168/jds.S0022-0302(03)73721-7
Byrnes, R. C., Nùñez, J., Arenas, L., Rao, I., Trujillo, C., Alvarez, C., et al. (2017). Biological nitrification inhibition by brachiaria grasses mitigates soil nitrous oxide emissions from bovine urine patches. Soil Biol. Biochem. 107, 156–163. doi: 10.1016/j.soilbio.2016.12.029
Castro, M., Gownipuram, J. R., Mendoza, M., Solano, N., López, F., Dickhöfer, U., and Corea, E. E. (2019). Effects of feeding tropical forage legumes on nutrients digestibility, nitrogen partitioning, and performance of crossbred milking cows. Anim. Feed Sci. Technol. 247, 32–40. doi: 10.1016/j.anifeedsci.2018.10.017
Cerón, F. M., Henao, A. V., Múnera, O., Herrera, A. R., Giraldo, A. D., Parra, A. M., et al. (2014). Concentración de Nitrógeno Ureico En Leche Interpretación y Aplicación Práctica. Medellín: Fondo Editorial Biogénesis.
Chacón, H. P. A., and Vargas, R. C. (2009). Digestibilidad y calidad del pennisetum purpureum cv. king grass a tres edades de rebrote. Agron. Mesoam. 20, 399–408. doi: 10.15517/am.v20i2.4956
Chirinda, N., Loaiza, S., Arenas, L., Ruiz, V., Faverín, C., Alvarez, C., et al. (2019). Adequate Vegetative cover decreases nitrous oxide emissions from cattle urine deposited in grazed pastures under rainy season conditions. Sci. Rep. 9:908. doi: 10.1038/s41598-018-37453-2
Climate-data.org. (2008a). Clima Baranoa: Temperatura, Climograma y Tabla climática para Baranoa - Climate-Data.org. Retrieved from: https://es.climate-data.org/america-del-sur/colombia/atlantico/baranoa-50360/#climate-graph
Climate-data.org. (2008b). Clima Casanare: Climograma, Temperatura y Tabla climática para Casanare - Climate-Data.org. Retrieved from: https://es.climate-data.org/america-del-sur/colombia/casanare-97/
Cole, N. A., and Todd, R. W. (2008). Opportunities to enhance performance and efficiency through nutrient synchrony in concentrate-fed ruminants1,2,3. J. Anim. Sci. 86(Suppl. 14), E318–E333. doi: 10.2527/jas.2007-0444
Colmenero, J. J. O., and Broderick, G. (2006). Effect of dietary crude protein concentration on milk production and nitrogen utilization in lactating dairy cows. J. Dairy Sci. 89, 1704–1712. doi: 10.3168/jds.S0022-0302(06)72238-X
Correa, H. J., Rodríguez, Y. G., Pabón, Y. G., and Carulla, J. E. (2012). Efecto de la oferta de pasto kikuyo (Pennisetum clandestinum) sobre la producción, la calidad de la leche y el balance de nitrógeno en vacas Holstein. Livest. Res. Rural Dev. 24:204. Retrieved from: http://www.lrrd.org/lrrd24/11/cont2411.htm
Dickhoefer, U., Glowacki, S., Gómez, C. A., and Castro, J. M. (2018). Forage and protein use efficiency in dairy cows grazing a mixed grass-legume pasture and supplemented with different levels of protein and starch. Livest. Sci. 216, 109–118. doi: 10.1016/j.livsci.2018.08.004
Dijkstra, J., Oenema, O., and Bannink, A. (2011). Dietary strategies to reducing n excretion from cattle: implications for methane emissions. Curr. Opin. Environ. Sustainab. 3, 414–422. doi: 10.1016/j.cosust.2011.07.008
Dijkstra, J., Oenema, O., Groenigen, J. W., Spek, J. W., van Vuuren, A. M., and Bannink, A. (2013). Diet effects on urine composition of cattle and N2O emissions. Animal 7, 292–302. doi: 10.1017/S1751731113000578
Dobbie, K. E., Mctaggart, I. P., and Smith, K. A. (1999). Nitrous oxide emissions from intensive agricultural systems: variations between crops and seasons, key driving variables, and mean emission factors. J. Geophy. Res. 104, 26891–26899. doi: 10.1029/1999JD900378
Du, Y., Cui, Y., Xu, X., Liang, D., Long, R., and Cao, G. (2008). Nitrous oxide emissions from two alpine meadows in the Qinghai–Tibetan Plateau. Plant Soil 311, 245–254. doi: 10.1007/s,11104-008-9727-9
FAO, Programa de las Naciones Unidas para la Alimentación y la Agricultura. (2013). El Estado Mundial De La Agricultura Y La Alimentación. Available online at: http://www.fao.org/3/a-i3300s.pdf (Retrieved May 11, 2021).
Federación Colombiana de Ganaderos (FEDEGAN) (2018). Cifras de Referencia del Sector Ganadero Colombiano. Available online at: https://www.fedegan.org.co/estadisticas/ (accessed Agosto 27, 2019).
García, M. S., Ravella, S. R., Chadwick, D., Vallejo, A., Gregory, A. S., and Cárdenas, L. M. (2014). Ranking factors affecting emissions of GHG from incubated agricultural soils. Eur. J. Soil Sci. 65, 573–583. doi: 10.1111/ejss.12143
Gardi, C., Angelini, M., Barceló, S., Comerma, J., Cruz Gaistardo, C., Encina Rojas, A., et al. (2014). Atlas de Suelos de América Latina y El Caribe. Comisión Europea - Oficina de Publicaciones de La Unión Europea. Vol. 17630. Luxemburgo: Comisión Europea - Oficina de Publicaciones de la Unión Europea. doi: 10.2788/37334
Gaviria, U. X., Sossa, C. P., Montoya, C., Chará, J., Lopera, J. J., Córdoba, C. P., and Barahona, R. R. (2012). Producción de Carne Bovina En Sistemas Silvopastoriles Intensivos En El Trópico Bajo Colombiano. Belén: VII Congreso Latinoamericano de Sistemas Agroforestales Para La Producción Animal Sostenible. 1–5.
Goering, H. K., Gordon, C. H., Hemken, R. W., Waldo, D. R., Van Soest, P. J., and Smith, L. W. (1972). Analytical estimates of nitrogen digestibility in heat damaged forages. J. Dairy Sci. 55, 1275–1280. doi: 10.3168/jds.S0022-0302(72)85661-3
Goering, H. K., and Van Soest, P. J. (1970). Forage Fiber Analyses: Apparatus, Reagents, Procedures, and Some Applications. No. 379. Washington, DC: Agricultural Research Service, US Department of Agriculture.
Haydock, K. P., and Shaw, N. H. (1975). The comparative yield method for estimating dry matter yield of pasture. Aust. J. Exp. Agric. Anim. Husb. 15, 663–670. doi: 10.1071/EA9750663c
Hess, H. D., Florez, H., Lascano, C. E., Becerra, A., and Ramos, J. (1999). Fuentes de variación en la composición de la leche y niveles de urea en sangre y leche de vacas en sistemas de doble propósito en el trópico bajo de Colombia. Pasturas Tropicales 21. Retrieved from: http://ciat-library.ciat.cgiar.org/Articulos_CIAT/Vol21_hess.pdf (accessed October 21, 2021).
IDEAM (2016). IMPACTO DEL FENÓMENO “EL NIÑO” 2015-2016 EN LOS NEVADOS Y ALTA MONTAÑA EN COLOMBIA. Bogotá: Subdirección de Ecosistemas e Información Ambiental Grupo de suelos y tierras. Retreived from: http://www.ideam.gov.co/documents/11769/132669/Impacto+de+El+Ni%C3%B1o+en+la+alta+monta%C3%B1a+colombiana.pdf/
IDEAM, UNAL. (2018). La Variabilidad Climática y El Cambio Climático En Colombia. Bogotá: IDEAM. Available online at: http://www.andi.com.co/Uploads/variabilidad.pdf
Instituto Colombiano Agropecuario (ICA) (2019). Censo Pecuario Nacional. Retrieved from: https://www.ica.gov.co/areas/pecuaria/servicios/epidemiologia-veterinaria/censos-2016/censo-2018 (accessed March 22, 2020).
IPCC, Intergovernmental Panel on Climate Change. (2019). Chapter 11: N2O Emissions From Managed Soils, and Co2 Emissions From Lime and Urea Application. 2019 Refinement to the 2006 IPCC Guidelines for National Greenhouse Gas Inventories.
Jaramillo, D., Rodriguez, E., and Diaz, K. (2002). Introducción a La Ciencia Del Suelo. Bogotá: Universidad Nacional de Colombia. Facultade de Ciencias, 1–30.
Kelliher, F. M., Cox, N., Van Der Weerden, T. J., De Klein, C. A. M., Luo, J., Cameron, K. C., et al. (2014). Statistical analysis of nitrous oxide emission factors from pastoral agriculture field trials conducted in New Zealand. Environ. Pollut. 186, 63–66. doi: 10.1016/j.envpol.2013.11.025
Kennedy, P. M., and Milligan, L. P. (1980). The degradation and utilization of endogenous urea in the gastrointestinal tract of ruminants: a review. Can. J. Anim. Sci. 60, 205–221. doi: 10.4141/cjas80-030
Klefoth, R. R., Clough, T. J., Oenema, O., and Van Groenigen, J. W. (2014). Soil bulk density and moisture content influence relative gas diffusivity and the reduction of nitrogen-15 nitrous oxide. Vadose Zone J. 13:vzj2014.07.0089. doi: 10.2136/vzj2014.07.0089
Kottek, M., Grieser, J., Beck, C., Rudolf, B., and Rubel, F. (2006). World map of the Köppen-Geiger climate classification updated. Meteorol. Zeitschr. 15, 259-263. doi: 10.1127/0941-2948/2006/0130
Lapierre, H., and Lobley, G. E. (2001). Nitrogen recycling in the ruminant: a review. J. Dairy Sci. 84, E223–E236. doi: 10.3168/jds.S0022-0302(01)70222-6
Laudone, G. M., Matthews, G. P., Bird, N. R. A., Whalley, W. R., Cardenas, L. M., and Gregory, A. S. (2011). A model to predict the effects of soil structure on denitrification and N2O emission. J. Hydrol. 409, 283–290. doi: 10.1016/j.jhydrol.2011.08.026
Laville, P., Lehuger, S., Loubet, B., Chaumartin, F., and Cellier, P. (2011). Effect of management, climate, and soil conditions on N2O and NO emissions from an arable crop rotation using high temporal resolution measurements. Agric. Meteorol. 15, 1228–12240. doi: 10.1016/j.agrformet.2010.10.008
León, J. M., Mojica, J. E., Castro, E., Cárdenas, E. A., Pabón, M. L., and Carulla, J. E. (2008). Balance de Nitrógeno y Fosforo de vacas lecheras en pastoreo con diferentes ofertas de kikuyo (Pennisetum clandestinum) suplementadas con ensilaje de avena (Avena sativa). Rev. Colomb. Cienc. Pecu. 21, 559–570. Retrieved from: https://www.redalyc.org/articulo.oa?id=295023543004
Lessa, A. R., Madari, B. E., Paredes, D. P., Boddey, R. M., Urquiaga, S., Jantalia, C. P., et al. (2014). Bovine urine and dung deposited on Brazilian savannah pastures contribute differently to direct and indirect soil nitrous oxide emissions. Agric. Ecosyst. Environ. 190, 104–111. doi: 10.1016/j.agee.2014.01.010
Li, Y. Y., Dong, S. K., Liu, S., Zhou, H., Gao, Q., Cao, G., et al. (2015). Seasonal changes of CO2, CH4, and N2O fluxes in different types of alpine grassland in the Qinghai-Tibetan Plateau of China. Soil Biol. Biochem. 80, 306–314. doi: 10.1016/j.soilbio.2014.10.026
Ma, H., Zhang, W., Zhu, X., Song, W., Liu, J., and Zhihai, J. (2010). Effects of rumen-protected tryptophan on growth performance, fibre characteristics, Nutrient utilization and plasma essential amino acids in cashmere goats during the cashmere slow growth period. Livest. Sci. 131, 227–233. doi: 10.1016/j.livsci.2010.04.005
Mahecha, L., Gallego, L. A., and Peláez, F. J. (2002). Situación Actual de La Ganadería de Carne En Colombia y Alternativas Para Impulsar Su Competitividad y Sostenibilidad. Rev. Colomb. Cienc. Pecu. 2, 213–225. Retrieved from: https://dialnet.unirioja.es/servlet/articulo?codigo=3242901
Mahecha, L., Monsalve, M. A., and Arroyave, J. F. (2007). Evaluación del silvopastoreo de novillos Cebú en un sistema de Eucalyptus tereticornis y Panicum maximum, en la reforestadora San Sebastián. Livest. Res. Rural Dev. 19:94. Retrieved from: http://www.lrrd.org/lrrd19/7/mahe19094.htm
Mahecha, L., and Rosales, M. (2005). Valor nutricional del follaje de botón de oro tithonia diversifolia (Hemsl.) gray, en la producción animal en el trópico. Livest. Res. Rural Dev. 17:100. Retrieved from: http://www.lrrd.org/lrrd17/9/mahe17100.htm
Marsden, K. A., Jones, D. L., and Chadwick, D. R. (2016). The urine patch diffusional area: an important N2O source? Soil Biol. Biochem. 92, 161–170. doi: 10.1016/j.soilbio.2015.10.011
Mazzetto, A. M., Barneze, A. S., Feigl, B. J., Van Groenigen, J. W., Oenema, O., and Cerri, C. C. (2014). Temperature and moisture affect methane and nitrous oxide emission from bovine manure patches in tropical conditions. Soil Biol. Biochem. 76, 242–248. doi: 10.1016/j.soilbio.2014.05.026
Milk, I. S. O. (2013). Liquid Milk Products—Guidelines for the Application of Mid-Infrared Spectrometry (ISO: 9622-2013). Geneva: International Organization for Standardization.
Murgueitio, E., and Ibrahim, M. (2001). Agroforestería pecuaria para la reconversión de la ganadería en Latinoamérica. Livestock Res. Rural Dev. 13:26.
Nha, P. T., Thu, N. V., and Preston, T. R. (2008). Effects of different levels and sources of crude protein supplementation on feed intake, digestibility and nitrogen retention in swamp buffaloes compared to local cattle. Livest. Res. Rural Dev. 20(Suppl.). Retrieved from: http://www.lrrd.org/lrrd20/supplement/nha2.htm
Oenema, O., Wrage, N., Velthof, G. L., Van Groenigen, J. W., Dolfing, J., and Kuikman, P. J. (2005). Trends in global nitrous oxide emissions from animal production systems. Nutr. Cycl. Agroecosyst. 72, 51–65. doi: 10.1007/s10705-004-7354-2
Pelster, D. E., Gisore, B., Goopy, J., Korir, D., Koske, J. K., Rufino, M. C., et al. (2016). Methane and nitrous oxide emissions from cattle excreta on an East African Grassland. J. Environ. Qual. 45, 1531–1539. doi: 10.2134/jeq2016.02.0050
Peña, C. F. (2002). Importancia del nitrógeno uréico de la leche como índice para evaluar la eficiencia productiva y reproductiva de las vacas lecheras. Revista Acovez. 27, 3–9. Retrieved from: http://www.acovez.org/index.php/component/search/?searchword=volumen%2027&searchphrase=all&start=20
Porre, R. J., van Groenigen, J. W., De Deyn, G. D., de Goede, R. G. M., and Lubbers, I. M. (2016). Exploring the relationship between soil mesofauna, soil structure, and N2O emissions. Soil Biol. Biochem. 96, 55–64. doi: 10.1016/j.soilbio.2016.01.018
Rabelo, E., Rezende, R. L., Bertics, S. J., and Grummer, R. R. (2003). Effects of Transition diets varying in dietary energy density on lactation performance and ruminal parameters of dairy cows. J. Dairy Sci. 86, 916–925. doi: 10.3168/jds.S0022-0302(03)73674-1
Rivera, J. E., Chará, J., and Barahona, R. R. (2019a). CH4, CO2, and N2O Emissions from grasslands and bovine Excreta in two intensive tropical dairy production systems. Agrofor. Syst. 93, 915–928. doi: 10.1007/s10457-018-0187-9
Rivera, J. E., Chará, J., Murgueitio, E., Molina, J. J., and Barahona, R. R. (2019b). Feeding Leucaena to dairy cows in intensive silvopastoral systems in Colombia and Mexico. Trop. Grassl. Forrajes Trop. 7, 370–374. doi: 10.17138/tgft(7)370-374
Rubol, S., Manzoni, S., Bellin, A., and Porporato, A. (2013). Modeling soil moisture and oxygen effects on soil biogeochemical cycles including Dissimilatory Nitrate Reduction to Ammonium (DNRA). Adv. Water Resour. 62, 106–124. doi: 10.1016/j.advwatres,.2013.09.016
Saggar, S., Bolan, N. S., Bhandral, R., Hedley, C. B., and Luo, J. (2004). A review of emissions of methane, ammonia, and nitrous oxide from animal excreta deposition and farm effluent application in grazed pastures. New Zeal. J. Agric. Res. 47, 513–544. doi: 10.1080/00288233.2004.9513618
Sey, B. K., Manceur, A. M., Whalen, J. K., Gregorich, E. G., and Rochette, P. (2008). Small-scale heterogeneity in carbon dioxide, nitrous oxide, and methane production from aggregates of a cultivated sandy-loam soil. Soil Biol. Biochem. 40, 2468–2473. doi: 10.1016/j.soilbio.2008.05.012
Simon, P. L., Dieckow, J., De Klein, C. A. M., Zanatta, J. A., Van Der Weerden, T. J., Ramalho, B., et al. (2018). Nitrous oxide emission factors from cattle urine and dung, and dicyandiamide (DCD) as a Mitigation Strategy in Subtropical Pastures. Agric. Ecosyst. Environ. 267, 74–82. doi: 10.1016/j.agee.2018.08.013
Skiba, U., and Ball, B. (2002). Integrated control of strawberry. Soil Use Manag. 3, 56–60. doi: 10.1079/SUM2002101
Sordi, A., Dieckow, J., Bayer, C., Alburquerque, M. A., Piva, J. T., Zanatta, J. A., et al. (2014). Nitrous oxide emission factors for urine and dung patches in a subtropical Brazilian pastureland. Agric. Ecosyst. Environ. 190, 94–103. doi: 10.1016/j.agee.2013.09.004
Steinshamn, H., Hoglind, M., Garmo, T. H., Thuen, E., and Brenøe, U.T. (2006). Feed nitrogen conversion in lactating dairy cows on pasture as affected by concentrate supplementation. Anim. Feed Sci. Technol. 131, 25–41. doi: 10.1016/j.anifeedsci.2006.02.004
Suárez, E., Reza, S., García, F, Pastrana, I., and Díaz, E. (2011). Comportamiento ingestivo diurno de bovinos de ceba en praderas del pasto Guinea (Panicum maximum cv. Mombasa). Corp. Cienc. Tecnol. Agropecu. 12, 167–174. doi: 10.21930/rcta.vol12_num2_art:228
Subbarao, G. V., Nakahara, K., Hurtado, M. P., Ono, H., Moreta, D. E., Salcedo, A. F., et al. (2009). Evidence for biological nitrification inhibition in brachiaria pastures. Proc. Natl. Acad. Sci.U.S.A. 106, 17302–17307. doi: 10.1073/pnas.0903694106
Tylutki, T. P., Fox, D. G., Durbal, V. M., Tedeschi, L. O., Russel, J. B., Van Amburgh, M. E., et al. (2008). Cornell net carbohydrate and protein system: a model for precision feeding of dairy cattle. Anim. Feed Sci. Technol. 143, 74–202. doi: 10.1016/j.anifeedsci.2007.05.010
Valles de la Mora, B., Castillo, G. E., and Bernal, B. H. (2016). Rendimiento y degradabilidad ruminal de materia seca y energía de diez pastos tropicales cosechados a cuatro edades. Rev. Mex. Cienc. Pecu. 7, 141–158. doi: 10.22319/rmcp.v7i2.4170
Van Horn, H. H., Wilkie, A. C., Powers, W. J., and Nordstedt, R. A. (1994). Components of dairy manure management systems. J. Dairy Sci. 77, 2008–2030. doi: 10.3168/jds.S0022-0302(94)77147-2
Van Soest, P. J., Robertson, J. B., and Lewis, B.A. (1991). Methods for Dietary fiber, neutral detergent fiber, and nonstarch polysaccharides in relation to animal nutrition. J. Dairy Sci. 74, 3583–3597. doi: 10.3168/jds.S0022-0302(91)78551-2
Villegas, D., Arevalo, A., Nuñez, J., Mazabel, J., Subbarao, G., Rao, I., et al. (2020). Biological Nitrification Inhibition (BNI): phenotyping of a core germplasm collection of the tropical forage grass megathyrsus maximus under greenhouse conditions. Front. Plant Sci. 11:820. doi: 10.3389/fpls.2020.00820
Keywords: cattle systems, milk urea nitrogen, cattle urine-based nitrogen, megathyrsus maximus, greenhous gases
Citation: Durango Morales SG, Barahona R, Bolívar DM, Arango J, Verchot L and Chirinda N (2021) Apparent Nitrogen Recovery in Milk and Early Dry Season Nitrous Oxide Emission Factors for Urine Deposited by Dual-Purpose Cattle on Different Soil Types. Front. Sustain. Food Syst. 4:602657. doi: 10.3389/fsufs.2020.602657
Received: 03 September 2020; Accepted: 09 December 2020;
Published: 18 January 2021.
Edited by:
Dietmar Schwarz, Leibniz Institute of Vegetable and Ornamental Crops, GermanyReviewed by:
Kamal Prasad Adhikari, Manaaki Whenua Landcare Research, New ZealandDavid Kanter, New York University, United States
João Gabriel Rossini Almeida, University of West of Santa Catarina, Brazil
Copyright © 2021 Durango Morales, Barahona, Bolívar, Arango, Verchot and Chirinda. This is an open-access article distributed under the terms of the Creative Commons Attribution License (CC BY). The use, distribution or reproduction in other forums is permitted, provided the original author(s) and the copyright owner(s) are credited and that the original publication in this journal is cited, in accordance with accepted academic practice. No use, distribution or reproduction is permitted which does not comply with these terms.
*Correspondence: Sandra Guisela Durango Morales, cy5kdXJhbmdvJiN4MDAwNDA7Y2dpYXIub3Jn