- 1Institut Sénégalais de Recherches Agricoles/Centre National de Recherches Agronomiques (ISRA/CNRA), Dakar, Senegal
- 2Laboratoire Mixte International Adaptation des Plantes et Microorganismes Associés aux Stress Environnementaux (LAPSE), Centre de Recherche de Bel Air, Dakar, Senegal
- 3Département d'Agroforesterie, Université Assane Seck de Ziguinchor, Ziguinchor, Senegal
- 4Laboratoire Commun de Microbiologie IRD/ISRA/UCAD, Centre de Recherche de Bel Air, Dakar, Senegal
- 5Département de Biologie Végétale, Université Cheikh Anta Diop de Dakar, Dakar, Senegal
- 6LSTM, University of Montpellier, CIRAD, INRAE, IRD, Institut Agro, TA A-82/J, Campus International De Baillarguet, Montpellier, France
- 7Laboratoire de Microbiologie Appliquée, Département de Microbiologie, Faculté des Sciences de la Nature et de la Vie, Université Ferhat Abbas Sétif, Sétif, Algérie
Salinity is one of the main abiotic stresses limiting plant growth and development. However, the use of salt-tolerant plants combined with beneficial soil microorganisms could improve the effectiveness of biological methods for saline soil recovery. The aim of this study is to identify the Casuarina obesa/ Arbuscular Mycorrhizal fungi (AMF)/Plant Growth Promoting Rhizobacteria (PGPR) association that could be used in salt-land rehabilitation programs. Thus, the plants were grown under greenhouse on sandy soil, inoculated either with PGPR (Pantoea agglomerans and Bacillus sp.), or with AMF (Rhizophagus fasciculatus and Rhizophagus aggregatum) or co inoculated with PGPR and AMF and watered with a saline solution (0, 150, and 300 mM). After 4 months of cultivation, the plants were harvested and the results obtained showed that inoculation improves the survival rate, height and biomass of the plants compared to the control plants. The results also showed that inoculation increases the total amount of chlorophyll and the accumulation of plant proline at all levels of salt concentration. However, P. agglomerans and Bacillus sp. strains alone or in combination with R. fasciculatus increased plant growth. This study showed that these strains of PGPR, whether or not associated with AMF, could be biological tools to improve C. obesa performance under saline stress conditions.
Introduction
Soil salinization is one of the major environmental stress for plant growth (Barnawal et al., 2014). About 10% of the total arable land as being affected by salinity and sodicity (Shahid et al., 2018). This phenomenon is constantly increasing and constitutes an obstacle to agricultural production (Munns and Tester, 2008). In Senegal, about 55% of arable land is affected by salinization (LADA, 2009), which considerably affects the potential for national agricultural production. Land salinization is most often the result of natural factors such as rainfall deficiency, seawater intrusion, capillary rise due to evaporation and rock solubilization, but also of anthropogenic factors such as poor agricultural practices (Legros, 2009). This salinization leads to a degradation of the biological, chemical and physical properties of soils, resulting in decreased of soil fertility and crop yields (Ndour, 2006). With increasing population growth, access to land is becoming more difficult. Therefore, there is an urgent to develop effective strategies to rehabilitate salt land despite the many economic and climatic constraints around the world. Thus, several strategies have been adopted by rural populations and development actors. These include: (i) mechanical control through the installation of anti-salt structures (dams, or bunds), (ii) chemical control through the application of chemical amendments such as gypsum, sulfur or sulphuric acid to neutralize alkalinity, and (iii) biological control through the introduction of salt-tolerant plant species or organic matter to rehabilitate saline soils (Fall et al., 2018). The use of salt-tolerant plants is an appropriate approach to salt land management (Singh, 2000). Indeed, the presence of trees favors the development of micro flora and micro fauna essential for biodegradation processes, which represent the basis for the soil fertility. However, the success of a revegetation strategy in these salt areas will require the use of local or introduced plant species that are best adapted to environmental conditions. To this end, trees of the Casuarinaceae family are widely used in these programs because of their ability to grow on very poor soils (Patil et al., 2005; Diagne et al., 2014). The growth of Casuarina species on these degraded soils may be related to their ability to associate with nitrogen-fixing bacteria (Frankia) or PGPR and AMF that improve the plant's nitrogen and phosphate nutrition. Indeed, these symbiotic associations constitute means of adapting plants to unfavorable environmental conditions such as saline stress (Beltrano et al., 2013). They also play an important role in plant growth under various conditions by modifying their root systems and increasing the mobilization and absorption of essential elements such as N and P (Hashem et al., 2016). The aim of this study were to select the most salt-tolerant C. obesa/PGPR/AMF combinations for the rehabilitation of land degraded by salinization.
Materials and Methods
Plant Material
The plant species used in this experiment were derived from seeds of C. obesa (lot number: 17994) provided by CSIRO/ATSC (Australian Tree Seed Centre; https://www.csiro.au/en/Resarch/Collections/ATSC Purchasing-seed). They were collected in the localities of Mullewa (latitude 28°15' North, longitude 115)38' East, altitude 250) in Australia. About 2,800 viable seeds were counted in a 10 g lot. These seeds were not pre-treated and were stored at 4°C in the laboratory.
Bacterial and Fungal Material
The strains Pantoea agglomerans lma2 (P. agglomerans) and Bacillus sp. were provided by the Laboratory of Applied Microbiology, Department of Microbiology, Faculty of Natural and Life Sciences, Ferhat Abbas Setif-Algeria University. These strains have been isolated in the Bou-saâda region of Algeria (Hasfa, 2014). They were isolated from the rhizosphere of wheat under arid soil affected by salinity. P. agglomerans (accession number GQ478022) is a Gram-negative bacterium and has been identified by sequencing RNA16S. These strains were initially cultured in tubes containing 5 ml of liquid Luria-Bertani (LB) Broth medium and placed under agitation in a bacterial culture chamber at 30°C for 48 h. After a second culture was made in tubes containing 500 ml of Broth LB medium and placed in a culture chamber for 24 h under the same conditions. The liquid bacterial cultures thus produced were used for inoculation of the plants.
The arbuscular mycorrhizal fungi used in this study were: Rhizophagus fasciculatus (Rf) (Thaxt.) C. Walker and A. Schüßler DAOM227130 isolated in Quebec (Schüßler and Walker, 2010) and Rhizophagus aggregatum (Ra) (N.C. Schenck and G.S. Sm.) C. Walker DAOM2277128 isolated in Burkina Faso. These AMF provide from the collection of “Laboratoire Commun de Microbiologie” (LCM). AMF inoculum was produced during 6 months under greenhouse using a mycotrophic plant (Zea mays L.) grown in the presence of AMF in pots containing 1.5 kg of sandy soil previously sterilized at 120°C for 2 h. The soil used for the study was collected at Sangalkam in Senegal (14° 46'52” N, 17° 13'40” O) with the following physico-chemical characteristics: pH (H2O) 6.5; clay 3.6%; fine silt 7.4%; fine sand 36.6%; coarse sand 21.55%; total carbon 0.54%; total nitrogen 0.06%; C/N 8.5; total phosphorus 39 mg kg-1 and soluble phosphorus 4.8 mg kg-1 (Diouf et al., 2005). The inoculum consisted of a mixture of spores and root fragments. The spore count was estimated using the Gerdemann and Nicolson (1963) method. The spore density of R. fasciculatus and R. aggregatum per 100 g of soil was 1,210 and 1,635 spores, respectively.
Plant Growth, Experimental Design, and Application of Salt Stress
C. obesa plants were cultivated in nursery (30°C) on sterile soil and watering with tap water at a frequency of 2 times/day. After 3 months of cultivation the seedlings were carefully dug out and transferred into pots of dimensions (25 × 12 × 50 cm) filled with a sandy soil sieved to 2 mm and autoclaved at 121°C for 120 min. Each pot was received a seedling and kept in a nethouse at 30°C at ISRA/CNRA (Institute Senegalese of Agriculture Research/National Center for Agronomical Research, 14°71 North - 16°48 West Bambey, Senegal). The soil used in our experiment was taken at CNRA (14°71 North - 16°48 West Bambey, Senegal). The physicochemical characteristics of this soil were determined at Laboratory of soil, plant and water, ISRA/CNRA, Bambey, Senegal and are as follows: pH (H2O) 7.78; pH (KCl) 7.46; EC 218.4 μS/cm; assimilable phosphorus 8.915 ppm; total phosphorus 3.896 ppm; total nitrogen 0.057%; organic carbon 0.631%; organic matter 1.09% and C/N 11.15. Fungal inoculum was applied at the time of plant transplantation. Treatments with AMF received 10 g of inoculum R. aggregatum strain or 10.12 g of the R. fasciculatus strain placed at about 3–5 cm depth. Bacterial inoculum was applied 1 week after transplanting close to the seedling root system. Treatments with bacteria received 5 ml of bacterial inoculum from P. agglomerans or the Bacillus sp. strain with a final absorbance of 0.2 measured at λ = 595 nm for each strain.
Salt stress was applied 2 months after inoculation to allow the establishment of mycorrhizal symbiosis. For each treatment, salt stress was gradually applied twice a week. Then, a weekly increase in NaCl concentration was performed to avoid osmotic shock. After 3 weeks of acclimatization, the control plants were watered with 0 mM and the stressed plants with 150 and 300 mM of NaCl. The choice of these concentrations was made on the basis of previous studies on Casuarina by Djighaly et al. (2018).
After 4 months the plants were harvested. Parameters such as height growth, total biomass, chlorophyll and proline contents and mycorrhizal frequency and intensity rate were evaluated.
The following treatments were applied: C: control plants not inoculated; P. agg: inoculated with Pantoea agglomerans; B25: inoculated with Bacillus sp.; Rf: inoculated with Rhizophagus fasciculatus; Ra: inoculated with Rhizophagus aggregatum; B25 + Rf: co-inoculated with Bacillus sp. and Rhizophagus fasciculatus; B25 + Ra : co-inoculated with Bacillus sp. and Rhizophagus aggregatum; P. agg + Ra: co-inoculated with Pantoea agglomerans and Rhizophagus aggregatum; P. agg + Rf: co-inoculated with Pantoea agglomerans and Rhizophagus fasciculatus. For each treatment seven repetitions were applied randomized device separated into blocks for each treatment to avoid contamination.
Measurement of Height Growth, Above-Ground, Root, and Total Biomass
The height (cm) growth of the plants was measured each month using a graduated ruler. The survival rate was determined using the formula:
After 4 months of greenhouse cultivation, the plants were harvested and the fresh weight of the aerial (BA) and root (BR) biomass produced for each treatment was weighed. The aerial and root parts were dried in the oven at 65°C for 1 week. Once the samples were completely dry, their dry weight was determined using an electronic precision balance TE1245 (Sartorius AG, Germany).
Determination of Chlorophyll and Proline Content
The chlorophyll content (a) and (b) of the aerial parts of the plants was determined using Arnon (1949) method, modified and described by Tahri et al. (1998). The total chlorophyll content (a+b) was calculated according to Arnon (1949) formula:
CHt (mg/l) = [8, 02 x DO (663 nm) + 20, 2 × DO (645 nm)] × V / M where V = volume of acetone (10 ml); M = foliar mass (100 mg); DO = optical density in nm.
Proline levels in the leaves were determined using the method described by Monneveux and Nemmar (1986). A quantity of 100 mg of fresh leaves were used and the samples were measured with a spectrophotometer at a wavelength of 520 nm. Proline contents were calculated using the equation derived from the calibration curve constructed from a range of known and increasing proline concentrations from 0 to 800 μmoles.
Mycorrhization
To determine the frequency and intensity of mycorrhization, the roots of the plants were cleaned and stained using the Phillips and Hayman (1970) method. For each plant, 100 fragments of 1 cm were used for the observations under the microscope. The frequency of mycorrhization was determined by the formula: F% = (number of mycorrhized fragments/total number of observed fragments) ×100. Mycorrhization intensity was analyzed using the method of Trouvelot et al. (1986) using a range of colonization intensity noted from zero (0) to five (5). It was estimated by the formula: I% = (95n5 + 70n4 + 30n3 + 5n2 + n1)/total number of observed fragments where n5 = number of noted fragments 5; n4 = number of noted fragments 4; n3 = number of noted fragments 3; n2 = number of noted fragments 2; n1 = number of noted fragments 1.
Statistical Analysis
The data were subjected to a two-factor analysis of variance (ANOVA) (inoculation and salinity level). The averages of the variables measured at the 5% probability threshold (p ≤ 0.05) were compared using the Student Newman-Keuls test. The tests and statistical analysis of the results were performed with GenStat Edition 17 software.
Results
Analysis of the variance reveals a significant effect of factor Inoculation and salt concentration (NaCl) on growth parameters such as plant height, shoot and root biomass (Supplementary Table 1). The interaction “Inoculation × [NaCl]” had significant effects on chlorophyll and proline content.
Effect of Inoculation With PGPR and/or AMF on the Survival Rate of C. obesa Plants Under Salt Stress Conditions
The results showed that all plants survived 1 month after salt stress application (Table 1). At harvest (2 months after salt application), plant mortality was noted only at 300 mM NaCl. The highest survival rate was observed in plants inoculated with P. agglomerans (100%) at 300 mM. However, this rate decreased in plants inoculated with B25 + Ra and Ra (80.95%), followed by control plants (C) and those inoculated with Rf + B25 and Rf with (85.71%) and in plants inoculated with P.agg + Rf, P.agg + Ra and B25 (90.47%) at 300 mM (Table 1).
Effect of Inoculation With PGPR and/or AMF on Height, Total Dry Biomass of C. obesa Plants Under Saline Stress Conditions
Results obtained after 4 months of growing in greenhouse showed that the increasing of salt concentration lead to a decrease of plant height from 35.39% to 150 mM and 64.67% to 300 mM compared to controls at 0 mM (Table 2). In the presence of 150 mM, inoculation with P. agg + Rf improved the height of C. obesa plants by 13.27% compared to control plants (Table 3). At 300 mM, inoculation with PGPR and/or AMF have no significative effect on C. obesa height.
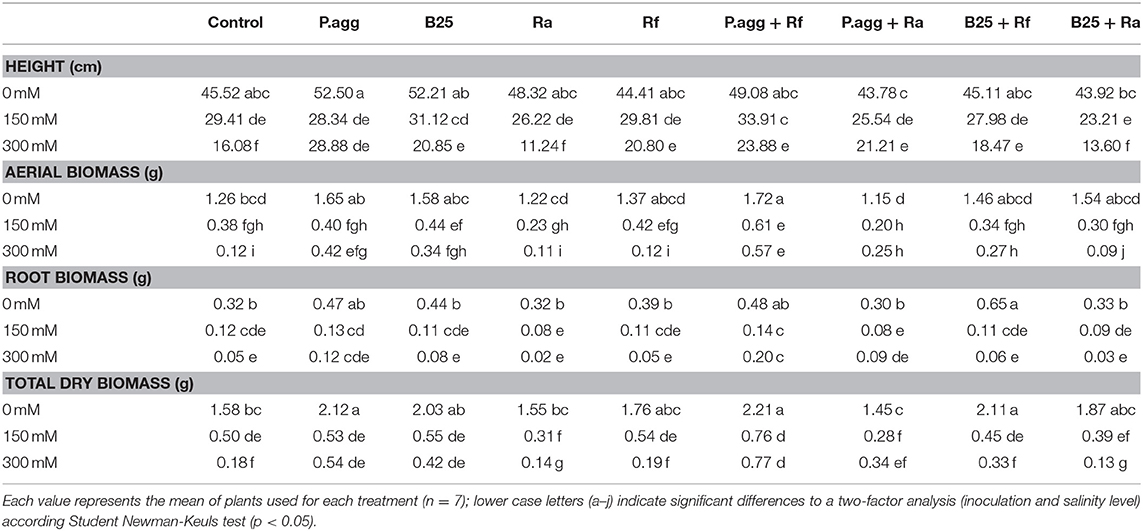
Table 2. Effect of inoculation with PGPR and/or AMF on height, shoot biomass, root biomass and total dry biomass of C. obesa plants under salt stress conditions.
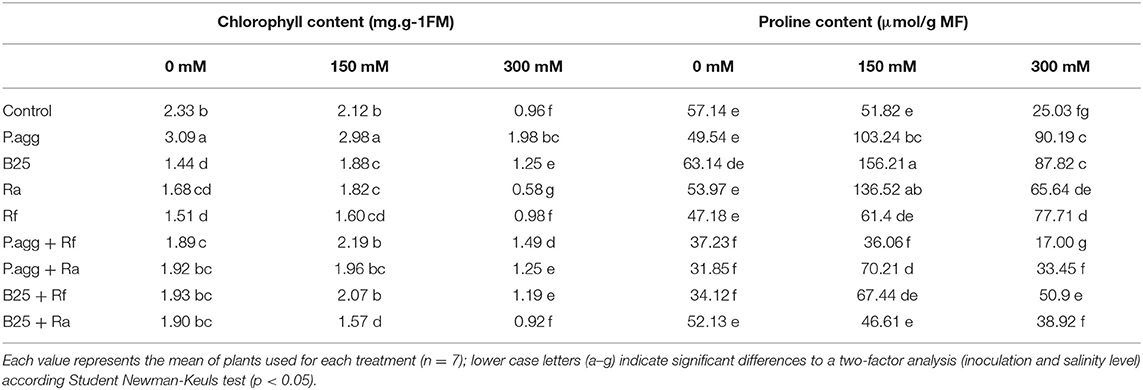
Table 3. Total chlorophyll and proline content of C. obesa plants inoculated with PGPR and/or AMF subject to 0, 150, and 300 mM NaCl.
The Aerial biomass of C. obesa was improved by the inoculation with P. agg + Rf compared to control plants at all concentration of NaCl. At 300 mM, only inoculation with P.agg + Rf improved the root biomass of C. obesa plants.
The total biomass of C. obesa was improved by the inoculation with P. agg, P. agg + Rf and B25 + Rf compared to control plants in the absence of salt. At 150 and 300 mM, a significant increase was observed in plants co-inoculated with P. agg + Rf of 34.24 and 76.62%, respectively, compared to control plants (Table 2).
Effect of Inoculation With PGPR and/or AMF on the Total Chlorophyll and Proline Contents of C. obesa Under Salt Stress Conditions
The total chlorophyll contents of C. obesa plants was evaluated after 4 months of salt stress and the results obtained show a significant increase in chlorophyll contents in plants inoculated with P. agglomerans compared to control plants at all concentration. The inoculation with P. agg increase chlorophyll content by 25.56, 28.85, and 51.55% at 0, 150, and 300 mM, respectively, compared to controls plants (Table 3). At 150 mM, inoculation with P. agg + Rf had increased the total chlorophyll content of C. obesa compared to the control plants.
The results obtained show an increase in proline synthesis as a function of salt concentration. In the absence of salt, the proline content is not significantly different between inoculated and non-inoculated plants. In the presence of 150 mM, there is an increase in proline levels in plants inoculated with P. agg and B25 compared to control plants. At 300 mM, a significant difference was noted in plants inoculated with P. agg and B25 compared to plants (Table 3).
Effect of Salt Stress on the Mycorrhization of C. obesa Plants Under Salt Stress Conditions
The highest frequency of mycorrhization was observed in co-inoculated plants inoculated with P.agg + Rf at all concentration. Concerning the intensity of mycorrhization, the higher intensity was observed with plants co-inoculated P. agg + Rf compared to controls plants at 0 and 150 mM. At 300 mM, no difference was noted between treatments compared to controls plants (Table 4).
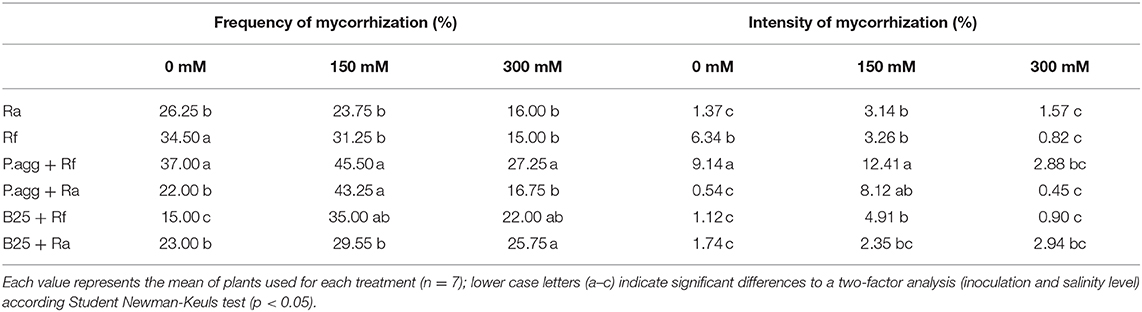
Table 4. Effects of the co-inoculation of PGPR and AMF on the frequency and intensity of mycorrhization of C. obesa plants under salt stress.
Discussion
The effect of inoculation with AMF and PGPR strains was studied on C. obesa plants subjected to different concentrations of NaCl (0, 150, and 300 mM) for 4 months under greenhouse. The results obtained show that inoculation with P. agglomerans strain improves the survival rate of plants compared to control plants. After 2 months of salt stress application, the survival rate of plants inoculated with P. agglomerans strain was 100%. This result could be explained by the intrinsic ability of this strain to tolerate salt. The works of Hasfa (2014) was showed that P. agglomerans lma 2 tolerated high salt concentrations and that its growth increased to NaCl concentrations between 100 and 400 mM. Inoculation with Bacillus sp. and P. agglomerans strains combined with R. fasciculatus or R. aggregatum also increased the survival rate of C. obesa (90.47%) compared to control plantsR. aggregatum strain compared to controls did not improve plant survival.
The efficacy of co-inoculation with P.agg + Rf and P.agg + Ra could be related to the ability of PGPR to solubilize phosphates and the effective absorption of solubilized P from soil through AMF hyphae (Richardson et al., 2009; Saia et al., 2020). This association allows better colonization of the plant by AMF through these PGPR, which are well known as Mycorrhiza Helper Bacteria (Garbaye, 1994). This improved colonization results in better soil exploration by the mycorrhized roots of the plant and better absorption of mineral elements by the hyphae of fungi (Smith and Read, 2008). This statement is in agreement with our results which showed that the frequency of mycorrhization was significantly improved by co-inoculation P.agg + Rf compared to plants inoculated with AMF strains alone in the presence of salt. Several studies have shown that symbiotic associations between beneficial soil bacteria (PGPR or Rhizobia) and the PGPR can improve root colonization of plants by AMF in many species such as: Acacia auriculiformis and Acacia mangium (Diouf et al., 2005), Acacia senegal (Ndoye et al., 2012) and Litchi chinensis (Visen et al., 2017). These microorganisms are regulators of stress by adjusting nutritional and hormonal balance and inducing systemic stress tolerance (Ruiz-Lozano et al., 2012). This regulation can induce an increase in K+ content accompanied by an effective decrease in Na+ in plant tissues. Additional experiments aimed to evaluate the variations of K+ and Na+ and Cl- concentrations in response to the inoculation with P.agg + Rf and P.agg + Ra will help to determine whether a similar mechanism is involved. However, the result obtained with other combinations could be explained by a less effective symbiosis between the two strains. Artursson et al. (2006) showed that the success of co-inoculation depends not only on the symbiotic efficacy of microorganisms but also on the compatibility between different symbiosis partners. Moreira et al. (2019) showed a synergistic effect between AMF and PGPR in increasing maize growth in saline conditions. This effect was related to an increase in K+ content accompanied by an effective decrease in Na+ in plant tissues.
The positive effect co-inoculation P. agg + Rf on growth parameters could be explained by the fact that P. agglomerans, is a halophilic, nitrogen-fixing and phosphate-solubilizing strain, capable of producing of indole acetic acid (AIA) up to 600 mM NaCl (Hasfa, 2014) and AMF (R. fasciculatus) in improving salt tolerance stress among Casuarina species (Djighaly et al., 2018). In the presence of salt, these microorganisms participate in the selective absorption of ions such as phosphorus, nitrogen and magnesium and in the reduction of Na+ ion absorption (Giri and Mukerji, 2004; Chen et al., 2014; Paul and Sinha, 2017). According to Ruiz-Lozano et al. (2012), improving plant tolerance to salinity by adding endomycorrhizae leads to increased photosynthetic activity and better water absorption efficiency.
The higher frequencies and intensities in co-inoculated P.agg + Rf plants would explain the salt tolerance of this strain. The work of Djighaly et al. (2018) showed significant metabolic activity of R. fasciculatus at 300 mM NaCl. But also to the additional effect of PGPR which improves the phosphate nutrition of the plant (Egamberdieva et al., 2019).
The high total chlorophyll content observed in plants inoculated with P. agglomerans in the presence of salt could explain the better growth rates observed in these plants. However, we noted that P. agglomerans strain associated with R. fasciculatus was more effective than R. aggregatum strain associated with the same strains. This result could be explained by a less effective symbiosis between the AMF (R. aggregatum) strain co-inoculated with the PGPR (P. agglomerans). A significant accumulation of proline was obtained with inoculated plants P. agg and B25 in the presence of salt (150 and 300 mM) compared to control plants. Proline accumulation is a mechanism of resistance to saline stress by adjusting intracellular osmotic pressure. These results are in agreement with works of Hasfa (2014) who showed that inoculation with Bacillus sp. and Pantoea agglomerans strain improve salt stress tolerance of wheat. However, our results showed that P. agglomerans + R. fasciculatum treatment had no significantly improve proline concentration in presence of NaCl. This result does not explain the good behavior of C. obesa plants co-inoculated with P. agglomerans + R. fasciculatus. However, it could also be supposed that this combination (P. agglomerans + R. fasciculatus) could establish other salt tolerance mechanisms such as the production of antioxidant enzymes, stress hormones and the overexpression of genes involved in salt stress tolerance. The works of Gond et al. (2015) showed that P. agglomerans improved the growth capacity of tropical maize compared to uninoculated controls. These results were explained by the up-regulation of the aquaporin gene family, especially plasma membrane integral protein (ZmPIP) genes in P. agglomerans-treated plants.
Conclusion
The results of the experiment show that inoculation with PGPR and/or AMF can improve resistance to salinity of C. obesa plants by increasing their growth parameters. In general, chlorophyll and proline content were also improved in plants inoculated under salt stress. This positive effect of inoculation was more pronounced with P. agglomerans and with P. agglomerans + R. fasciculatus. Thus, inoculation with salt tolerant PGPR and/or AMF could be a solution for the rehabilitation of land affected by the salt in Senegal. It would be interesting to carry out further research in field conditions to confirm the performance of these strains and then isolate indigenous strains of PGPR and Frankia from saline soils and determine their impact on salt tolerance in Casuarina inoculated with AMF.
As Casuarina species are associated with Frankia strains that play a critical role on their performance. It would be interesting the study the interaction of Casuarina species, salt tolerant AMF and PGPR strains.
Data Availability Statement
The raw data supporting the conclusions of this article will be made available by the authors, without undue reservation.
Author Contributions
MNd, PID, MNg, GNd and ND did the experimental work and analysis thereof and wrote the manuscript. ND, DNg, SS, and HC-S contributed in designing, supervision, and interpretation of the results. All authors contributed to the article and approved the submitted version.
Funding
This work was supported by the International Foundation for Sciences (IFS, no. AD/22680), the Academy of Sciences for the Developing World (TWAS, no. 11-214 RG/BIO/AF/AC I), The Fonds d'Impulsion de la Recherche Scientifique et Technique (FIRST) of the Ministry of Higher Education and Research of Senegal, The Laboratoire Mixte International Adaptation des Plantes et microorganismes associés aux Stress Environnementaux (LAPSE).
Conflict of Interest
The authors declare that the research was conducted in the absence of any commercial or financial relationships that could be construed as a potential conflict of interest.
Supplementary Material
The Supplementary Material for this article can be found online at: https://www.frontiersin.org/articles/10.3389/fsufs.2020.601004/full#supplementary-material
References
Arnon, D. I. (1949). Copper enzymes in isolated chloroplasts phenoloxidase in Beta vulgaris. Plant Physiol. 24, 1–15. doi: 10.1104/pp.24.1.1
Artursson, V., Finlay, R. D., and Jansson, J. K. (2006). Interactions between arbuscular mycorrhizal fungi and bacteria and their potential for stimulating plant growth. Environ. Microbiol. 8, 1–10. doi: 10.1111/j.1462-2920.2005.00942.x
Barnawal, D., Bharti, N., Maji, D., Chanotiya, C. S., and Kalra, A. (2014). ACC-deaminase containing Arthrobacter protophormiae induces NaCl stress tolerance through reduced ACC oxidase activity and ethylene production resulting in improved nodulation and mycorrhization in Pisum sativum. J. Plant Physiol. 171, 884–894. doi: 10.1016/j.jplph.2014.03.007
Beltrano, J., Ruscitti, M., Arango, M. C., and Ronco, M. (2013). Effects of arbuscular mycorrhiza inoculation on plant growth, biological and physiological parameters and mineral nutrition in pepper grown under different salinity and p levels. J. Soil Sci. Plant. Nutri. 13, 123–141. doi: 10.4067/S0718-95162013005000012
Chen, Y., Fan, J. B., Du, L., Xu, H., Zhang, Q. H., and He, Y. Q. (2014). The application of phosphate solubilizing endophyte Pantoea dispersa triggers the microbial community in red acidic soil. Appl. Soil Ecol. 84, 235–244. doi: 10.1016/j.apsoil.2014.05.014
Diagne, N., Djighaly, P. I., Ngom, M., Prodjinoto, H., Ngom, D., Hocher, V., et al. (2014). “Rehabilitation of saline lands using selected salt-tolerant Casuarina microorganisms combinations,” in Casuarina Improvement for Securing Rural Livelihoods. Proceedings of Fifth International Casuarina Workshop Chennai India, eds A. Nicodemus, K. Pinyopusarerk, C. L. Zhong, and C. Franche (Institute of Forest Genetic and Tree Breeding).
Diouf, D., Duponnois, R., Ba, A. T., Neyra, M., and Lesueur, D. (2005). Symbiosis of Acacia auriculiformis and Acacia mangium with mycorrhizal fungi and Bradyrhizobium spp. improves salt tolerance in greenhouse conditions. Funct. Plant. Biol. 32, 1143–1152. doi: 10.1071/FP04069
Djighaly, P. I., Diagne, N., Ngom, M., Ngom, D., Hocher, V., Fall, D., et al. (2018). Selection of arbuscular mycorrhizal fungi strains to improve Casuarina equisetifolia (L.) and Casuarina glauca (Sieb.) tolerance to salinity. Ann. For. Sci. 75:72. doi: 10.1007/s13595-018-0747-1
Egamberdieva, D., Wirth, S., Bellingrath-Kimura, S. D., Mishra, J., and Arora, N. K. (2019). Salt-tolerant plant growth promoting rhizobacteria for enhancing crop productivity of saline soils. Front. Microbiol. 10:2791. doi: 10.3389/fmicb.2019.02791
Fall, D., Bakhoum, N., Fall, F., Diouf, F., Ndiaye, C., Faye, M. N., et al. (2018). Effect of peanut shells amendment on soil properties and growth of seedlings of Senegalia senegal (L.) Britton, Vachellia seyal (Delile) P. Hurter, and Prosopis juliflora (Swartz) DC in salt-affected soils. Ann. For. Sci. 75:32. doi: 10.1007/s13595-018-0714-x
Garbaye, J. (1994). Interactions between mycorrhizal fungi and other soil organisms. Plant Soil 159, 123–132. doi: 10.1007/BF00000101
Gerdemann, J. W., and Nicolson, T. H. (1963). Spores of mycorrhizal Endogone species extracted from soil by wet sieving and decanting. Trans. Br. Mycol. Soc. 46, 235–244. doi: 10.1016/S0007-1536(63)80079-0
Giri, B., and Mukerji, K. G. (2004). Mycorrhizal inoculant alleviates salt stress in Sesbania aegyptiaca and Sesbania grandiflora under field conditions: evidence for reduced sodium and improved magnesium uptake. Mycorrhiza 14, 307–312. doi: 10.1007/s00572-003-0274-1
Gond, S. K., Torres, M. S., Bergen, M. S., Helsel, Z., and White, J. F. Jr. (2015). Induction of salt tolerance and up-regulation of aquaporin genes in tropical corn by rhizobacterium Pantoea agglomerans. Lett. Appl. Microbiol. 60, 392–399. doi: 10.1111/lam.12385
Hasfa, C. (2014). Amélioration de la croissance du blé dur en milieu salin par inoculation avec Bacillus sp. et Pantoea agglomerans isolées de sols arides (Thése de doctorat). Université Ferhat Abbas Sétif, 177.
Hashem, A., Abd-Allah, E., Alqarawi, A. A., Al-Huqail, A. A., Wirth, S., and Egamberdieva, D. (2016). The interaction between arbuscular mycorrhizal fungi and endophytic bacteria enhances plant growth of Acacia gerrardii under salt stress. Front. Microbiol. 7:1089. doi: 10.3389/fmicb.2016.01089
LADA (2009). Outils de gestion durable des terres au Sénégal: Contribution de LADA (Land Degradation Assessment in Senegal). Rapport.
Legros, J. P. (2009). “La salinisation des terres dans le monde,” in: Conférence n 4069, Bull. 257–269. Available online at: www.ac-sciences-lettres-montpellier.fr › LEGROS2009
Monneveux, P., and Nemmar, M. (1986). Contribution à l'étude de la résistance à la sécheresse chez le blé tendre (Triticum aestivum L.) et chez le blé dur (Triticum durum Desf.): étude de l'accumulation de la proline au cours du cycle de développement. Agronomie 6, 583–590. doi: 10.1051/agro:19860611
Moreira, H., Pereira, S. I. A., Vega, A., Castro, P. M. L., and Marques, A. P. G. C. (2019). Synergistic effects of arbuscular mycorrhizal fungi and plant growth-promoting bacteria benefit maize growth under increasing soil salinity. J. Environ. Manage. 257:109982. doi: 10.1016/j.jenvman.2019.109982
Munns, R., and Tester, M. (2008). Mechanisms of salinity tolerance. Annu. Rev. Plant. Biol. 59, 651–681. doi: 10.1146/annurev.arplant.59.032607.092911
Ndour, B. (2006). Relations sol-plantes dans les écosystèmes terres salées du bassin arachidier du Sénégal (Thèse de doctorat). Université Cheikh Anta Diop de Dakar, 93.
Ndoye, F., Kane, A., Bakhoum, N., Sanon, A., Fall, D., Diouf, D., et al. (2012). Response of Acacia senegal (L.) Willd. seedlings and soil bio-functioning to inoculation with arbuscular mycorrhizal fungi, rhizobia and Pseudomonas fluorescens. Afr. J. Microbiol. Res. 6, 7176–7184. doi: 10.5897/AJMR12.815
Patil, B. N., Patil, S. G., Hebbara, M., Manjunatha, M. V., Gupta, R. K., and Minhas, P. S. (2005). Bioameliorative role of tree species in salt-affected vertisols of india. J. Trop. Forest Sci. 17, 346–354.
Paul, D., and Sinha, S. N. (2017). Isolation and characterization of phosphate solubilizing bacterium Pseudomonas aeruginosa KUPSB12 with antibacterial potential from river Ganga, India. Ann. Agri. Sci. 15, 130–136. doi: 10.1016/j.aasci.2016.10.001
Phillips, J. M., and Hayman, D. S. (1970). Improved procedures for clearing roots and staining parasitic and vesicular-arbuscular mycorrhizal fungi for rapid assessment of infection. Trans. Br. Mycol. Soc. 55, 158–161. doi: 10.1016/S0007-1536(70)80110-3
Richardson, A., Barea, J. M., McNeill, A., and Prigent-Combaret, C. (2009). Acquisition of phosphorus and nitrogen in the rhizosphere and plant growth promotion by microorganisms. Plant Soil 321, 305–339. doi: 10.1007/s11104-009-9895-2
Ruiz-Lozano, J. M., Porcel, R., and Aroca, R. (2012). Regulation by arbuscular mycorrhizae of the integrated physiological reponse to salinity in plants: new challenges in physiological and molecular studies. J. Exp. Bot. 63, 4033–4044. doi: 10.1093/jxb/ers126
Saia, S., Aissa, E., Luziatelli, F., Ruzzi, M., Colla, G., Ficca, A. G., et al. (2020). Growth-promoting bacteria and arbuscular mycorrhizal fungi differentially benefit tomato and corn depending upon the supplied form of phosphorus. Mycorrhiza 30, 133–147. doi: 10.1007/s00572-019-00927-w
Schüßler, A., and Walker, C. (2010). The Glomeromycota: A Species List With New Families and New Genera. Gloucester: Arthur Schüßler & Christopher Walker, The Royal Botanic Garden Edinburgh, The Royal Botanic Garden Kew, Botanische Staatssammlung Munich, and Oregon State University. Available online at: http://www.amf-phylogeny.com
Shahid, S. A., Zaman, M., and Heng, L. (2018). “Soil salinity: historical perspectives and a world overview of the problem,” in Guideline for Salinity Assessment, Mitigation and Adaptation Using Nuclear and Related Techniques, eds M. Zaman, S. A. Shahid, and L. Heng (Cham: Springer), 43–53.
Singh, R. B. (2000). Environmental consequences of agricultural development: a case study from the Green Revolution state of Haryana, India. Agric. Ecosyst. Environ. 82, 97–103. doi: 10.1016/S0167-8809(00)00219-X
Tahri, E. H., Belabed, A., and Sadki, K. (1998). Effet d'un stress osmotique sur l'accumulation de proline, de chlorophylle et des ARNm codant pour la glutamine synthétase chez trois variétés de blé dur (Triticum durum). Bull. Inst. Sci. 21, 81–87.
Trouvelot, A., Kough, J. L., and Gianinazzi-Pearson, V. (1986). “Mesure du taux de mycorhization VA d'un syst radiculaire. Recherches et méthodes d'estimation ayant une signification fonctionnelle,” in Dans: Aspects Physiologiques et Génétiques des Mycorhizes (Dijon: INRA), 217–221.
Keywords: Casuarina obesa, beneficial microorganisms, land rehabilitation, salt stress, plant tolerance
Citation: Diagne N, Ndour M, Djighaly PI, Ngom D, Ngom MCN, Ndong G, Svistoonoff S and Cherif-Silini H (2020) Effect of Plant Growth Promoting Rhizobacteria (PGPR) and Arbuscular Mycorrhizal Fungi (AMF) on Salt Stress Tolerance of Casuarina obesa (Miq.). Front. Sustain. Food Syst. 4:601004. doi: 10.3389/fsufs.2020.601004
Received: 31 August 2020; Accepted: 23 November 2020;
Published: 15 December 2020.
Edited by:
Everlon Cid Rigobelo, Universidade Estadual Paulista, BrazilReviewed by:
Rui S. Oliveira, University of Coimbra, PortugalVanesa Silvani, University of Buenos Aires, Argentina
Copyright © 2020 Diagne, Ndour, Djighaly, Ngom, Ngom, Ndong, Svistoonoff and Cherif-Silini. This is an open-access article distributed under the terms of the Creative Commons Attribution License (CC BY). The use, distribution or reproduction in other forums is permitted, provided the original author(s) and the copyright owner(s) are credited and that the original publication in this journal is cited, in accordance with accepted academic practice. No use, distribution or reproduction is permitted which does not comply with these terms.
*Correspondence: Nathalie Diagne, bmF0aGFsaWVkaWFnbmVAZ21haWwuY29t