- 1Institute for Resources, Environment, and Sustainability, University of British Columbia, Vancouver, BC, Canada
- 2Perennial Agriculture Institute, Holyoke, MA, United States
- 3Global Evergreening Alliance, Burwood East, VIC, Australia
- 4Faculty of Land and Food Systems, University of British Columbia, Vancouver, BC, Canada
- 5School of Public Policy and Global Affairs, University of British Columbia, Vancouver, BC, Canada
Staple crops, which have large amounts of carbohydrates, proteins, and/or fats, provide the bulk of calories in people's diets. Perennial plants, which can be productive for many years without the need for replanting, can produce staple foods and environmental benefits, but their agronomic and nutritional properties haven't been considered synthetically in comparison to annual staples. Here we offer a framework to classify perennial staple crops according to their nutritional categories and cultivation status. We assemble literature to report on the yield potential of 51 perennial staple crops, only 15 of which are well-characterized in existing global datasets. We show the extent and distribution of perennial staple crop production in relation to annual crop types, calculate the carbon stocks they hold, and analyze their nutritional content for three macronutrients and nine micronutrients. We found that most perennial staple crops are regional crops (not globally traded) that grow in the subtropics to tropics. At least one perennial staple crop in each of the five nutritional categories has yields over 2.5 t/ha, in some cases considerably higher, competitive with and in many cases exceeding those of nutritionally comparable annual staples. Perennial staple crops only comprise ~4.5% of total cropland. They hold a modest ~11.4 GtC above and below ground, less than one third of the anthropogenic carbon-equivalent emissions for the year 2018, but more than the ~9 GtC held by the same amount of annual cropland. If linear growth in land under perennial staple production continues to 2040, and replaces only annual cropland, an additional ~0.95 GtC could be sequestered. Many perennial crops also had competitive macronutrient density and yield (per unit area) compared to annual staples; moreover, specific perennial staples are abundant in specific micronutrients, indicating that they can be a nutrient-dense part of diets, unlike the most ubiquitous annual staple crops (corn, wheat, rice) that do not appear in the top 85th percentile for any of the nine micronutrients analyzed. Transition of land and diets to perennial staple crops, if judiciously managed, can provide win-win solutions for both food production and ecosystems.
Introduction
The challenge of growing and supplying food to a growing world population involves balancing the production of multiple outputs (food, fodder, raw materials, livelihoods) with multiple ecosystem services and impacts (climate regulation, soil conservation, wildlife habitat, energy use, pollution, etc.; Robertson and Swinton, 2005; Foley et al., 2011; Campbell et al., 2017; IPBES, 2019a). Recent literature has recognized that both protected areas and a favorable working landscape matrix are required for conservation of natural services and wildlife (Kremen, 2015; IPBES, 2019a). The current agricultural system worldwide is dominated by annual monocrops that do not constitute such favorable matrix environments, namely wheat (Triticum spp.), maize (Zea maysL.), soybean (Glycine max [L.] Merr.), and rice (Oryza sativa L.), which cover over 1.29 billion hectares of land globally (FAO, 2020). These systems, which experienced steep yield gains over the post-war decades in most of the world (Ray et al., 2012) are heavily reliant on external inputs of energy, fertilizers, and pesticides, leading to severe ecological consequences. These include 10–12% of greenhouse gas emissions leading to global warming (IPCC, 2015), nutrient pollution leading to eutrophication in aquatic ecosystems (Withers et al., 2014), erosion of soils leading to loss of arable land and carbon cycle disruption (Lal, 2003), and the pervasive decline of life on Earth due to landscape simplification that degrades habitat for wildlife (Foley et al., 2005; Díaz et al., 2019; IPBES, 2019a). Perennial agriculture, which includes both woody and herbaceous crops that are grown for multiple years without replacement, is a transformative alternative to the annual systems driving these impacts (Wolz et al., 2017).
Perennials are a key component in the habitat quality of the agricultural landscape matrix, and are thus often accounted for in landscape ecology and landscape genetics studies, as they allow for better movement of organisms between habitat patches (Benjamin et al., 2008; Brady et al., 2011; Eycott et al., 2012) or serve as primary habitat patches themselves (Bailey et al., 2010; Horak et al., 2013). In addition to their benefits to wildlife habitat and biodiversity, ecological studies link perenniality in agricultural landscapes to additional ecosystem functions and services that directly or indirectly contribute to the well-being of people: soil carbon sequestration (Young et al., 2009; Cates et al., 2016), pollination (Morandin and Kremen, 2013; Bennett and Isaacs, 2014), pest regulation (Landis et al., 2000; Karp et al., 2018), water quality (Gao et al., 2004; Blesh and Drinkwater, 2013), and soil erosion (Brady and Weil, 1986; Zuazo and Pleguezuelo, 2007). Meta-analyses of empirical findings from agroforestry systems (i.e., agricultural systems that have a woody perennial component) have confirmed their ability to enhance multiple ecosystem services, though the specific services they bolster are highly context dependent (Pumariño et al., 2015; Torralba et al., 2016).
Applied ecological literatures (agroecology and agroforestry) as well as the grassroots permaculture movement have long advocated for the integration of long-lived perennial species in diversified agricultural landscapes (Mollison and Slay, 1995; Jose, 2009; Ferguson and Lovell, 2013; Asbjornsen et al., 2014). Agroforestry is defined as a system that produces crops and/or livestock products and includes woody plants. While most agroforestry practices involve integrating non-food producing perennials into the edges of annual agricultural landscapes (as buffers, hedges etc.), in some cases, the woody plants are themselves the primary crop, and tree crops have recently been added to the definition of agroforestry by some authors (van Noordwijk et al., 2016). This integration is a welcome development, as it helps break down the somewhat artificial boundary that has separated agroforestry practices that are established and studied chiefly for ecosystem services (especially in the temperate North) and tree crop systems that are established and studied chiefly for food production (Lovell et al., 2018). Food production from perennial crops is an important and underappreciated dimension in the expanded definition of agroforestry that needs to be centered and understood alongside the environmental benefits of these multifunctional systems.
The potential of perennial systems has been reflected in recent, major UN and gray literature reports on climate change that list agroforestry and tree crops among the top solutions for both climate mitigation and food production (Hawken, 2017; IPCC, 2019a). However, as discussed, any potential environmental benefits of growing food perennially must be assessed in the context of the yield intensity and nutritional value (as well as the economics) of perennial crops compared to those of annual crops.
Efforts to this end are actively taking place through breeding projects to either perennialize herbaceous annual staple crops, or domesticate wild perennial grasses for food production (e.g., Kernza—Thinopyrum intermedium [Host] Barkworth & D.R.Dewey, a domesticated version of a wild perennial wheatgrass; Glover et al., 2012). For these breeding projects, and for a few shorter-lived perennial staple crops that have congeneric annual crops (such as beans), agronomic and environmental outcomes can be directly compared to the equivalent annual species (Vico and Brunsell, 2018; Schier et al., 2019). But scientific analyses on a global food system scale are lacking for already existing and nutritionally important perennial staple crops. This may be due to the higher complexity of comparing many dissimilar species, the perception that perennials are not an important part of diets, the relatively lower prevalence of perennial staples in the temperate North, and the long generation times needed for breeding these crops (Mehlenbacher, 2003; Molnar et al., 2013).
Perennial staple crops have been a part of people's diets throughout history, often signaling the transition from nomadic lifestyles to long-term settlement (Smith, 2010). In the Mediterranean basin, olive (Olea europaea L.), date (Phoenix spp.), carob (Ceratonia siliqua L.), and chestnut (Castanea spp.) endure as important crops from before recorded history (Zohary, 2002; Zohary et al., 2012). The acorn (Qercus spp.), now seldom eaten, was the carbohydrate base of numerous human societies, called “balanocultures” (Bainbridge, 1985). Other perennial staples have been more persistent: the “Castagnetu,” or domestic/wild chestnut forest, still constitutes the basis of agrarian life in inland Corsica (Michon, 2011). Olives still dominate parts of the Mediterranean landscape and form the basis of cultural identity in several regions (Fleskens et al., 2009). In the tropics, many species of palms used for their starchy trunks, and sugary or oily fruit, are staple foods and have been for millennia (Toensmeier, 2016). The walnut (Juglans regia L.) and pistachio (Pistacia vera) forests of Kyrgyzstan have an anthropogenic origin one to two thousand years ago and remain an important source of resources and livelihoods today (Beer et al., 2008). In North and South America, flour made from mesquite pods (Prosopis spp.) was a staple for many Indigenous cultures (Felker, 2005). Despite their persistence in some regions, colonization and the adoption of cereal-based annual agriculture in more parts of the world has generally spelled the decline of ancient perennial crop-based societies (Smith, 2010). However, perennial staple foods remain important sources of nutrition, and several are sought-after for their health and nutritional properties.
Most academic studies of perennials focus on economically, but not nutritionally important commodities in the tropics (coffee—Coffea spp. and cocoa—Theobroma cacao Tussac), or on sugar and micronutrient-rich fruits in temperate regions (apples—Malus domestica (Suckow) Borkh., pears—Pyrus spp., etc.). However, diverse species of perennials also provide staple nutrition in the form of carbohydrates, fats, and proteins that, in some cases, compare favorably to the yields of annual staple crops. The contribution of perennial staple crops to food production at present, and their potential for growth in the future is not yet clear because the yields, distribution, and nutritional value of these crops have not been systematically categorized, synthesized, and compared to annual crops in the scientific literature.
In this paper, we address this gap and ask the questions: (1) What are the yield ranges of under-studied perennial staple crops? (2) What is the current extent and spatial distribution of perennial staple crop production? (3) What are the nutritional properties of perennial staple crops? (4) How much carbon are existing perennial staples holding in both biomass and soil? To this end, we define and categorize perennial staple crops, compare their yields to those of annual staple crops in nutritionally analogous groups, report their total production, map their geographical distribution, synthesize nutritional information, and estimate their standing carbon stock. We hope that this baseline information on the production, nutrition, and climate regulation of perennial staple crops will enable future work that incorporates these important, but under-studied, foods into global and regional planning for land transitions, market interventions, and agricultural policy that are required for achieving sustainable food-security.
Perennial Staple Crops
The term staple crop is a food that is eaten regularly and comprises a major part of people's diets. Woody perennials often bring to mind sugary fruits or stimulants like coffee or tea [Camellia sinensis (L.) Kuntze] rather than staple crops. But perennial plants are staple crops in some parts of the world (for example, avocado—Persea americana Mill., banana—Musa spp., coconut—Cocos nucifera L., and many others), providing core nutrition in the form of carbohydrates, proteins and fats. We define perennial staple crops as those that have significant amounts of these three fundamental nutrients (carbohydrates, proteins and fats). This definition distinguishes perennial staple crops from other categories of perennial crops, like sugar-producing crops, industrial crops, perennial pastures, and spices/stimulant/medicinal crops (Box 1).
Box 1. Perennial crop types.
Staple crops: Perennials whose edible products contain high proportions of carbohydrates, proteins, and fats. E.g., nuts, olive (Olea europaea), oil palm (Elaeis guineensis Jacq.), avocado (Persea americana), perennial beans (Phaseolus spp.), mesquite (Prosopis spp.).
Sugar crops: Perennial crops grown mainly for their sugar content. E.g., cane sugar (Saccharum spp.), date (Phoenix dactylifera L.).
Perennial vegetables: Perennials that are used for their leafy greens, shoots, or flowers. These are often rich in micronutrients but not calorie-dense. E.g., bamboo shoot (Bambusa vulgaris Nees), asparagus (Asparagus officinalis L.), moringa leaf (Moringa oleifera Lam.), grape leaf (Vitis spp.).
Non-staple fruit crops: Typical orchard fruit that do not contain large amounts of carbohydrates, proteins, and fats but are often culturally important and rich in micronutrients. E.g., apples (Malus domestica), peaches (Prunus persica [L.] Batsch), oranges (Citrus sinensis Pers.), etc.
Beverage/spice/medicinal: These are non-staple specialty foods that can be economically and culturally important. E.g., Coffee (Coffea spp.), cocoa (Theobroma cacao), spices, medicinal plants.
Perennial grains (in development): Breeders are currently working on cross-breeding annual staples (grains) with wild relatives to produce perennial grains, but with limited success; most lines are still weakly perennial. The more successful breeding attempt is in the opposite direction: domesticating a wild perennial grass. The Land Institute in Kansas, USA is currently producing small amounts of Kernza (Thinopyrum intermedium), a perennial wheatgrass bred for improved grain production.
Fodder crops: Perennials grown as animal feed. These overlap with both staple crops (mesquite—Prosopis spp., chestnuts—Castanea spp., honey locust—Gleditsia triacanthos L.) and non-staple fruit crops [persimmon—(Diospyros kaki Thunb.), mulberry—(Morus spp.)].
Pasture: Many pasture and natural grassland species are long-lived herbaceous (non-woody) perennials, which are either grazed directly or mowed for hay year after year. E.g., perennial grasses, alfalfa (Medicago sativa L.).
Industrial crops: Fiber, wood, chemical extracts. E.g., rubber (Ficus elastica Roxb.), inedible oil, wax, gums.
We further categorize perennial staple crops into five nutritional categories based on the proportions of carbohydrates, proteins, and fats in the dry, edible portion of the crops. Our synthesis of yields uses this categorization in order to select appropriate annual comparator species. While calorically important perennial staple crops are the focus of this paper, Box 2 situates them alongside other categories of perennial crops.
Box 2. Nutritional categories of perennial staple crops.
Basic starch crops: (0–8% protein, 0–5% oil). These crops include crops such as bananas (Musa spp.), chestnuts (Castanea spp.), plantains (Musa spp.), breadfruit (Artocarpus altilis [Parkinson] Fosberg), air potato (Dioscorea bulbifera), and starchy trunks (Metroxylon sagu Rottb., Ensete ventricosum (Welw.) Cheesman). Comparable annual crops include cassava, sweet potato, taro, and yams.
Balanced carbohydrate crops: (8.1–15% protein, 5.1–15% oil). Carbohydrates can be from either starch or sugar. These crops include crops such as Mayan breadnut (Brosimum alicastrum Sw.), mesquite pods (Prosopis spp.), Tahitian chestnut (Inocarpus fagifer [Parkinson] Fosberg), and Yeheb nut (Cordeauxia edulis Hemsl.). Comparable annual crops include maize, wheat, rice, and potato.
Protein crops: (15.1+% protein, 0–15% oil). These crops include perennial beans (Phaseolus spp.) and honey locust pods (Gleditsia triacanthos), as well as processed leaf protein concentrates of herbaceous perennials like alfalfa (Medicago sativa) and stinging nettle (Urtica dioica L). Comparable annual crops include annual beans, chickpeas, lentils, pigeon peas, and cowpeas.
Protein-oil crops: (over 15 % both protein and oil). These crops include seeds, beans, and nuts such as almonds (Prunus dulcis [Mill.] Rchb.), Brazil nut (Bertholletia excelsa O.Berg), pistachio (Pistacia vera), walnut (Juglans regia), and hazelnut (Corylus avellana). Comparable annual crops include soybeans, peanuts, and sunflower seeds.
Oil crops: (0–15% protein 16+% oil). These crops are sometimes consumed whole, while some are pressed for oil. They include olive (Olea europea), coconut (Cocos nucifera), avocado (Persea americana), oil palm (Elaeis guineensis), pecan (Carya illinoiensis), and macadamia (Macadamia spp.). Comparable annual crops include rapeseed (canola).
Some perennial staple crops (almond, banana, coconut, palm oil) are already familiar global commodities (>$ 1 billion USD/year, see Box 3). Others are minor global commodities. Many though, are regional crops with fairly limited total production, distribution and trade value. Box 3 shows the different categories of crop's cultivation status, market-readiness and commodification.
Box 3. Cultivation status of perennial staple crops. Global crops are defined by FAO statistical service. The remaining categories were adapted following Toensmeier (2016).
Global crop: Crops are grown and traded around the world and have an annual value greater than $1 billion USD. E.g., coconuts (Cocos nucifera), avocado (Persea americana), almonds (Prunus dulcis), bananas (Musa spp.).
Minor global crop: Crops are grown and traded around the world, but on a smaller scale than global commodity staple crops, having an annual value lower than $1 billion USD. E.g., breadfruit (Artocarpus altilis), sago palm (Metroxylon sagu), carob (Ceratonia siliqua), akee (Blighia sapida).
Regional crop: Crops have been domesticated and cultivated regionally but adopted elsewhere. Sometimes traded globally, sometimes only traded regionally. E.g., peach palm (Bactris gasipaes), yeheb nut (Cordeauxia edulis), honey locust (Gleditsia triacanthos).
Wild crop: Crops that have strong historic or contemporary use as staples, but are not domesticated and are little cultivated if at all. E.g., mongongo nut (Schinziophyton rautenenii), Brazil nut (Bertholletia excelsa), North American pinion pines (Pinus spp.).
New, in development, and experimental crops: Crops that are under development by breeders to domesticate or perennialize them. E.g., tacay nut (Caryodendron orinococense), perennial wheatgrass (Thinopyrum intermedium).
Methods
Categorization of Perennial Staple Crop Into Nutritional Groups
Perennial staple crops were defined as perennial plants producing carbohydrates, proteins, and fats at levels above certain thresholds (Box 2). These were assembled based on the perennial staple crops listed in Toensmeier (2016), only a subset of which are included here due to the availability of adequate data. We grouped crops into these five nutritional categories following Toensmeier (2017) to generate intuitive “common sense” groupings for staple crops. For example, most root crops, as well as bananas and plantains, are “basic starch” crops. Pulse crops tend to be “protein” crops (e.g., cowpea, chickpea), and nuts and seeds tend to be protein-oil or oil crops. The categories have been updated from Toensmeier (2017) in that they are now based on edible dry weight macronutrient values. Though it would be preferable to categorize based on nutrition percentages from the foods as they are eaten (i.e., cooked grains, fresh avocado, etc.), the nutritional values for most crops that require cooking were not available for the cooked form. We therefore chose to group and compare all crops on a zero moisture (dry weight) basis, so as not to compare some fresh products to other dry products. The disadvantages of this are that some perennial staples are not eaten dry (avocado), while some are mostly eaten as re-hydrated dry products (mesquite flour, enset flour, dry beans), and some are eaten both fresh and dry (coconut). By using dry weights, crops that are typically not consumed as staples, or not widely known as staples, can be evaluated for their potential as staple crops. Inedible portions like banana peels, avocado pits, and nut shells were also removed from the calculations, resulting in a dry, edible weight that was used for both yield and nutritional comparisons. The disadvantage of using dry edible weights is that farmers are more often paid on the basis of unprocessed (or semi-processed) fresh weight, making our yield estimates less easily converted to financial return.
Yields of Perennial Staple Crops
We used national average data from the Food and Agriculture Organization (FAO) where available, and supplemented with other sources of yield information (often gray literature) to cover additional perennial staple crops. For each crop, we searched for papers, and reports that reported on yields of the perennial staple crops in the five nutritional categories. Searches were conducted using the crop name (both scientific and common) and a variety of keywords (yield, harvest, farm, production, productivity, fruit, seeds, nuts) using a Google scholar and google proper internet search, and the search results were screened up to page 7. Searches were not exact, since for some crops, results were more readily found than for others, which required more iterations to find relevant results. Studies were included if they contained information on crop production per area, from at least one field. Yield data extrapolated from individual trees or a small number of trees (common for poorly-studied crops) were not included. However, in some cases gray literature reports that lacked robust primary data, but were based on seemingly reliable farmer or expert assessments, were included. Units were converted to tons per hectare. Each reported yield was tagged with a data type based on the scale of the observations or experiments they originated from. The following data types were used: (1) research station, (2) on-farm study, (3) multiple sites, (4) farmer/expert assessment (5) regional assessment, (6) national assessment (mostly FAO data), (7) Global average yields (FAO). The category “multiple sites” refers to studies where several on-farm sites were studied, in contrast to regional assessments, which assessed average yields for an entire sub-national region or administrative unit. Global average yields for crops with country-level FAO data were not included in the yield plots with the other data types except for Brazil nut, where country-level information was not available. Due to the dearth of yield information for some crops, we did not require strict peer-review standards for this information, but we did require that the information be traceable to a study location or an author. The data category “farmer/expert assessment” was something of a catch-all category for reports that were from a trustworthy source (such as a government ministry, academic, or farmer), but did not contain explicit methods for the area or number of plants assessed.
To minimize large variation in fresh weight yields, we calculated dry weight yields for only the edible portion of each product (i.e., no shells, husks etc.). Sources and full calculations for yield information and dry edible weights are available in the Supplementary Materials available on Figshare figshare.com/projects/Perennial_Staple_Crops/78756.
Global Distribution of Perennial Cropland
We re-analyzed a dataset of global geospatial crop distributions (Monfreda et al., 2008) to show the contrasting distributions of herbaceous perennial crops and woody perennial crops, as well as the distinction between staple and non-staple woody perennial crops. The raster dataset on 175 crops for the year 2000 was assembled as described in Monfreda et al. (2008), and includes 15 perennial staple crops, the same ones with FAO data. Wee recategorized the crop types for our purposes into three nested layers of categories. First, all cropland was classified into (1) annual crops, (2) annual/perennial grasses, (3) perennial crops. The perennials were further classified into (1) food, (2) fodder/forage, and (3) fiber/raw materials. The perennial food crops were further classified into (1) staples (2) fruit, (3) beverage/spice/medicinal plants. Herbaceous perennials used chiefly to feed animals (alfalfa and clover) were in the fodder/forage category. Most perennial food crops in the Monfreda et al. (2008) are woody, but two (banana and plantain) are large herbaceous monocots. For each cell, we produced decimal ratios by dividing the amount of cover within each cell in a sub-category of interest by the area total of its larger category: namely, perennial crop area/total crop area; perennial food crop area/perennial crop area; and perennial staple crop area/perennial food crop area. We produced the maps using these ratios combined with a color scheme highlighting high and low ratios.
Data on the harvested area of the 15 perennial staple crops that the FAO reports on were collected and averaged for the years 2013–2017. To supplement this data we also found harvested area data for a few additional crops (pecan, macadamia, sago) in other literature, including the US Department of Agriculture (USDA, 2020), and the Mexican Secretariat of Agriculture and Rural Development (SIAP, 2020).
Nutrition of Perennial Staple Crops
Nutritional values of each crop were collected from multiple sources in the literature and averaged. Nutritional data for the three macronutrients (carbohydrates, protein and fat) and nine micronutrients (fiber, calcium, iron, magnesium, zinc, vitamin A, folate, vitamin C, and vitamin E) were collected. Traditional malnutrition is a set of nutrient deficiencies with iron, zinc, vitamin A, iodine, and folate most prominent among them (Muthayya et al., 2013). In contrast, deficiencies in industrial diets increase risks of obesity, diabetes, heart disease, high blood pressure, and osteoporosis. Key nutrient deficiencies in these industrial diets are fiber, calcium, magnesium, and antioxidants like vitamins A, C, and E (Suter, 2005; USDA USHHS, 2011; de Baaij et al., 2015; Siti et al., 2015). Accordingly, we selected micronutrients that are deficient from either traditional or industrial diets: fiber, calcium, iron, magnesium, zinc, folate, and vitamins A, C, and E following Toensmeier et al. (2020). Iodine is not present in most terrestrial foods, so it was not included.
Similarly to the yield calculations, we calculated the nutrient content on the basis of the dry, edible portion of each crop. Error bars (95% confidence intervals) were calculated. When only one nutrient value was found in the literature, no error bars were calculated. Pigeon peas (Cajanus cajan) can be grown both annually and perennially, so nutrient information reported for this species (which did not specify annual or perennial) was used for both. Nutrition information for Leaf Protein Concentrate (LPC) of stinging nettle wasn't available, so nutrition information for blanched nettle leaves was used instead as a rough approximation. Rapeseed, which was the only annual comparator for perennial crops exclusively pressed for oil (and not also consumed as a fruit, seed, or nut), did not have nutrition information available on the basis of the seed (only the pressed oil); there is no annual comparator for nutrition in the oil category for this reason. Data tables with full references for the sources of nutritional data are available on the figshare data repository under the project “Perennial Staple Crops” figshare.com/projects/Perennial_Staple_Crops/78756.
To calculate macronutrient yield per hectare, nutrient content was combined with yield information. For crops with FAO yield data, the FAO global yields averaged from the years 2013–2017 were used. For crops with no FAO data, average yields from our literature review were calculated (regardless of data type). These average yields cannot be considered true estimates because they do not take into account data type or attempt to weight the different yield reports, and are therefore not robust, but merely rough calculations of yield potential. Since uncertainty is not provided from the FAO for their global estimates, and since our own yield calculations cannot provide a true mean and standard deviation, uncertainty was propagated from nutrition data only (not from yield data) by the standard formula for error propagation by multiplication/division (Taylor, 1997).
Nutritional values on total carbohydrates were entered as reported in the literature. The challenge is that this value includes not only starch but also sugar and dietary fiber, which are also “carbohydrates” in that they are composed solely of carbon, hydrogen, and oxygen. Thus, a value for starch is unavailable for most crops.
Systems for reporting Vitamin A content have varied over the past decades. Here, all values are converted to mg Retinol Activity Equivalent (RAE), the current international standard.
Carbon Stocks
The total carbon stock for a perennial system is the sum of carbon from different pools: above-ground biomass (AGB), below ground biomass (BGB), dead biomass, and soil organic carbon. For above- and below-ground biomass, we looked for mean standing carbon stocks over the lifetime of a crop rather than carbon sequestration rates. This is because biomass (and thus carbon) accumulates over the life of a perennial system in a non-linear fashion. However, mean stocks can only be reported when biomass measurements for different sizes of trees (or allometric equations that correlate with age) are combined with information about the typical length of a perennial crop's productive life. Such means were only sometimes reported in the literature. Thus, for crops where we found multiple citations or reports on biomass for multiple ages of trees, these were averaged. For crops for which we could only find a single report from a sample of a certain age, it was used, or if the age was considered mature, the biomass was halved for a conservative estimate of mean standing stock. For crops for which we found no information on biomass, we substituted values from similar crops (for example plantain and banana). Regarding the inconsistency of carbon pools reported for biomass across publications (AGB and BGB and undergrowth/dead biomass), where only AGB was reported we obtained BGB by multiplying AGB with the conversion factor 0.21, which is a conservative BGB:AGB ratio from the range for forest cover from volume 4, chapter 4, table 4.4 of the Refinement to the 2006 IPCC Guidelines for National Greenhouse Gas Inventories: Agriculture, Forestry and Other Land Use (IPCC, 2019b). We did not include litter and dead biomass unless these were reported together and couldn't be separated. To obtain values of tons carbon/ha we additionally carried out the conversion of C = 0.5*(mass of dry matter) if the publication had not already done so. In addition, we sometimes obtained separate information about typical planting density for a particular crop so that we could use publications that reported on biomass or generated allometric equations from a small number of trees (i.e., publications that did not report carbon stocks on a per hectare basis or report planting density).
We used the soil organic carbon stock estimates for 3 climates (temperate, tropical, and Medditerannean) from Ledo, Smith, Zerihun, Whitaker, Vicente-Vicente and Qin (2020) meta-analysis of soil carbon under perennial agriculture (Table 1). These soil organic carbon stocks were matched to each crop depending on its growing conditions, and summed with the biomass carbon estimates discussed above. To obtain an estimate of standing stock from perennial staples globally, these per-crop mean carbon stocks were multiplied with the harvested areas of the 15 perennial staple crops reported from the UN FAO, as well as those for 4 more crops (pecan, peach palm, sago, and macadamia) which were obtained from other references. Standing carbon stock was calculated as follows:
Where i is the perennial staple crop, and j is the climate it matches (Table 1). For all the biomass values found in literature, and how these were applied to the 19 crops, refer to Supplementary Tables on figshare figshare.com/projects/Perennial_Staple_Crops/78756.
To estimate changes in biomass carbon for several scenarios of land conversion to 2040, data on the adoption of 15 perennial staple crops from 1968 to 2018 from the FAO Statistical service was used to project harvested area 20 years forward (to 2040) using a linear projection. The changes in biomass of carbon stocks were calculated by subtracting the standing stock of carbon of a comparator comparator land cover type (forest, grassland, or annual cropland) from mean standing stock of carbon for each of the perennial species (calculated above). The carbon stocks of the comparator cover types in different climates were obtained and matched to the climates of each of the perennial crops as follows: the mean AGB carbon stock of various forest types were retrieved from Volume 4: Agriculture, Forestry and Other Land Use, Chapter 4, table 4.7 of the Refinement to the 2006 IPCC Guidelines for National Greenhouse Gas Inventories (IPCC, 2019b), and multiplied by the ABG:BGB ratios from table 4.4 and matched to the environments where perennial staple crops are grown. These were multiplied with AGB:BGB carbon ratios obtained from table 4.4 to obtain the below-ground carbon estimates for each forest type. These were then summed to obtain biomass carbon estimates that include above and below ground carbon. Biomass carbon stocks (above and below ground combined) for grassland were obtained from Volume 4: Agriculture, Forestry and Other Land Use, Chapter 6, table 6.4 of 2006 IPCC Guidelines for National Greenhouse Gas Inventories (IPCC, 2006). Biomass carbon stocks for annual cropland were obtained through a re-analysis of the dataset from Monfreda et al. (2008) for annual crops only in 14 world ecoregions. For harvested area values from the linear projection and for the values of biomass carbon for the comparison land cover types that were matched with each perennial crop by climate, refer to Supplementary Tables on figshare figshare.com/projects/Perennial_Staple_Crops/78756.
For changes in soil carbon stocks after conversion from other cover types to perennial crops, we used the change in carbon stock/ha over 20 years after conversion from Ledo et al. (2020) (reproduced in Table 2). This was summed to the change in biomass carbon stock and multiplied by the change in harvested area for that species (equation 2).
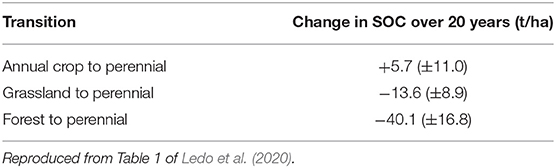
Table 2. Soil Organic carbon transitions to 100 cm below the surface over 20 years after transition.
Where i is the specific perennial staple crop, and j the comparison land cover (annual cropland, grassland, or forest). biomass carbonj,i is thus the biomass carbon land cover type j, matched to climate and humidity of perennial i as discussed above; this value is set to 0 for j = annual cropland. Δsoil carbon over 20 yrsj is the change in carbon over 20 years from the land transition between the comparison land cover, j, and perennial cropland (Table 2). Δarea harvestedi is the change in area harvested for a particular crop based on a subtracting the current area harvested (2013–2017 average) from the linear projection to 2040.
Results
Characteristics and Yields of Perennial Staple Crops
With the literature review, we expanded the set of available yield estimates for perennial staple crops from 15 (records kept by the United Nations Food and Agriculture Organization) to 51 (Supplementary Table 1).
In the basic starch category, two perennial crop species stand out for having high yield potential: sago and enset (Figure 1A). Sago (Metroxylon sagu) is a palm grown mostly in Indonesia and Malaysia (Jong, 2018) which is used for its starchy trunk. Enset (Ensete ventricosum), like banana, is a giant non-woody perennial monocot which is grown in Ethiopia and used for its large, starchy corm and trunk. Both plants are consumed as regional staples, and are similar in that their edible starches are derived from destructive harvest and processing of vegetative parts of the plant after several years of growth as opposed to the harvest of an edible fruit year after year (in the case of sago, only one trunk of the multi-stemmed palm is removed at a time leaving the rest to continue growing; in the case of enset, the corm may be left behind to regrow, or it may be harvested with the trunk, thus killing the plant). While the yield reports we found for these crops had a wide spread, yields exceeding 8 t/ha have been reported, well above typical yields of all the annual staple comparators in this category. Breadfruit (Artocarpus altilis), a tropical tree with a starchy fruit that is related to the familiar jackfruit, and carob (Ceratonia siliqua), a mediterranean legume tree with edible pods, are additional carbohydrate-rich crops that have similar yield levels to the familiar perennial staples, banana and plantain, and to the annual starchy tuber comparators in this category.
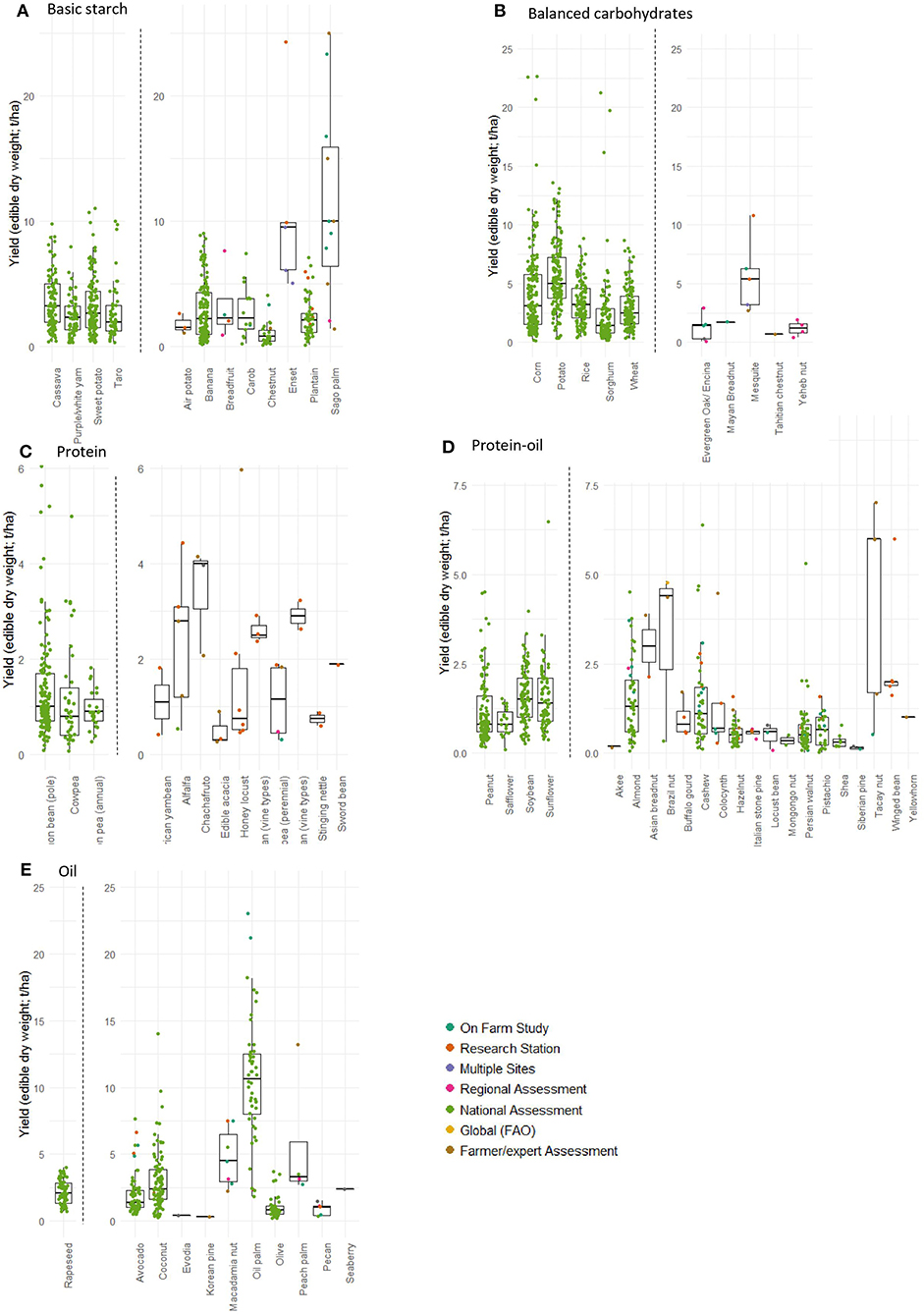
Figure 1. Yields of perennial staple crops. Left panels contain annual crops, right panels contain perennial crops. Each point represents one data point (t/ha of dry, edible portion) from the literature, color coded by data type (see methods for full reference list). Box plots show the mean (line through box), 25th and 75th percentile (top and bottom of box), largest and smallest value within 1.5 times of interquartile range (top and bottom of whiskers). Green points (National Assessments) are mainly FAO data. (A) Basic starch crops; (B) balanced carbohydrate crops; (C) protein crops; (D) protein-oil crops; (E) oil crops.
In the balanced carbohydrate category, which contains many of our most familiar annual staples including corn, wheat, potato, and rice, the perennial mesquite (Prosopis spp.) stands out as a crop with high yield potential, while the other perennials in the category have lower yields than the annuals (Figure 1B). Mesquite is the common name for several species of leguminous tree in the genus Prosopis, native to the Americas, Africa and Southern Asia and ranging widely in habitat from tropical and hot arid, to temperate climates. The trees have wholly edible pods that can be used for animal fodder or processed as flour for human consumption, and some species can grow in arid and high salinity conditions (Felker et al., 1981). Mesquite was used extensively as a staple food by Indigenous peoples in the Southeast of North America, Argentina, and Peru, and also as a fodder crop and fertility enhancer for intercropped annual grains in the Indian subcontinent for centuries (Felker, 2005). While Mesquite has been studied as a raw ingredient for the food industry (Meyer et al., 1986; Del Valle et al., 1989), and found some success commercially and through community programs that offer milling services (Desert Harvesters, 2008), it is still overexploited for timber and charcoal, not yet extensively grown on purpose for commercial food production and could still be improved through trait selection across large parts of its range (Felker, 2005).
The protein category (Figure 1C), is unsurprisingly composed of leguminous species. Some of these are bean trees (Chachafruto—Erythrina edulis Triana, Honey Locust—Gleditsia triacanthos, Edible Acacia—Acacia colei Maslin & L.A.J.Thomson), while others are species of vining beans that become woody and persist for many years in warmer climates (vine types of runner bean—Phaseolus coccineus L., lima bean—Phaseolus lunatus L., sword bean—Canavalia gladiata [Jacq.] Kuntze). Pigeon pea (Cajanus cajan [L.] Millsp.) is a perennial legume bush which is a widespread staple in India. Interestingly, it is chiefly grown as an annual, but has received some attention as a perennial component in annual intercropping systems in place of the non-food producing leguminous trees (like Leucaena) typically used in arid alley cropping (Daniel and Ong, 1990; Waldman et al., 2017). The African yam bean (Sphenostylis stenocarpa Harms) is an interesting crop in that it is grown for both its beans (which can be harvested perennially), and its large edible tuber (thus ending its life); this underutilized crop is being studied for traits and genetic markers to assist in breeding (Adewale et al., 2012, 2015). Finally, the protein category also includes alfalfa (Medicago sativa) and stinging nettle (Urtica dioica) for their leaf protein concentrate (LPC), a tofu-like product created by deriving protein-rich curds from leaf juice or pulp. LPC can be made from a wide variety of palatable leaves, but these are included because of the availability of data. Of the bean trees, vines/bushes, and LPC crops, chachafruto, vining runner & lima bean, and alfalfa LPC have the highest reported yields, respectively. Honey locust has large variation in reported yields.
The protein-oil category was the largest of the five nutritional categories, with 18 perennial staple crops including many familiar nut species (Figure 1D). Of these, the species with the highest reported yields are the Asian breadnut (Artocarpus camansi Blanco), Inche/tacay nut (Caryodendron orinocense H.Karst.), and Brazil nut (Bertholletia excelsa O.Berg). Asian breadnut is the wild ancestor of the breadfruit (Artocarpus altilis) and is still an important staple in New Guinea. Both its flesh (when immature) and seeds (when mature) are consumed. Inche/Tacay nut and Brazil nut are both South American nuts that are chiefly still wild-harvested and have not undergone domestication. Tacay nut can be cultivated and grown in plantations, though it isn't clear to what extent it has been planted (Wickens, 1995; PFAF, 2020). In contrast, Brazil nut requires pollination from wild bees, and attempts at plantations have had low yields (Cavalcante et al., 2012). In summary, all three of these species are essentially undomesticated and partially to largely still wild-harvested, yet they have reported yields significantly higher than the widely domesticated and cultivated species of perennials (such as walnuts, hazelnuts, pistachio and cashew) as well the annual comparators in the same category.
The oil category (Figure 1E) is dominated by the intensively produced African oil palm (Elaeis guineensis) which boasts high national average yields and is a familiar global commodity ubiquitous in the processed food industry. The crop with the second highest yield, macadamia nut, includes three species from the genus Macadamia, all of which are native to Australia, where they are commercially produced along with Australia, South Africa, Hawaii, and elsewhere. Though they are familiar high-value nuts, this crop still lacks official UN FAO data. Peach palm (Bactris gasipaes Kunth) is the next highest yielding crop in the oil category. Like the African oil palm, it is also a palm tree, but native to the New World. It has many edible uses: its palm heart and flower shoot are eaten as vegetables, while its starchy and oily fruit is cooked and eaten as a staple in many forms (our reported yields relate only to the fruit). The peach palm was domesticated before European colonization, and encompasses many landraces with variable genetics and characteristics (Mora-Urpi et al., 1997). While the heart of palm has been commercialized successfully, the fruit is still cultivated mainly as a subsistence crop for local consumption and sale; its use by Indigenous peoples as a staple with many processed products has failed to gain commercial traction and only small amounts are processed and sold internationally (Clement et al., 2010; Graefe et al., 2013). Coconut, avocado, and olive, three important and globally commodified staple perennial crops in the oil category, have generally lower yields than the less ubiquitous perennial staples peach palm and macadamia.
Global Distribution of Perennial Staple Crops
While yield information is crucial for understanding the intensity of production, the geographic distribution and harvested areas of these crops are necessary to understand their current extent. Based on Monfreda et al. (2008), perennial crops comprise 12 percent of global cropland (Figure 2). Of this, 80 percent produces food, while the remaining 20 percent produces forage and fiber/raw materials. Previous spatial analyses have investigated tree cover on agricultural land, but haven't distinguished between different classes of food-producing perennials (Zomer et al., 2009). We therefore distinguished between perennial staples and other food-producing perennials. Out of all food-producing perennial croplands, 34 percent is in perennial staple crops. The remainder is devoted to non-staple perennial crops: fruit (46.7 percent) and beverages, spices, and various medicinal plants like coffee, cocoa, kola nut, cardamom, etc. (18.5%). The spatial analysis of area harvested only includes the 15 perennial staple crops included in the Monfreda dataset, which are the same as those with UN data (Supplementary Table 1).
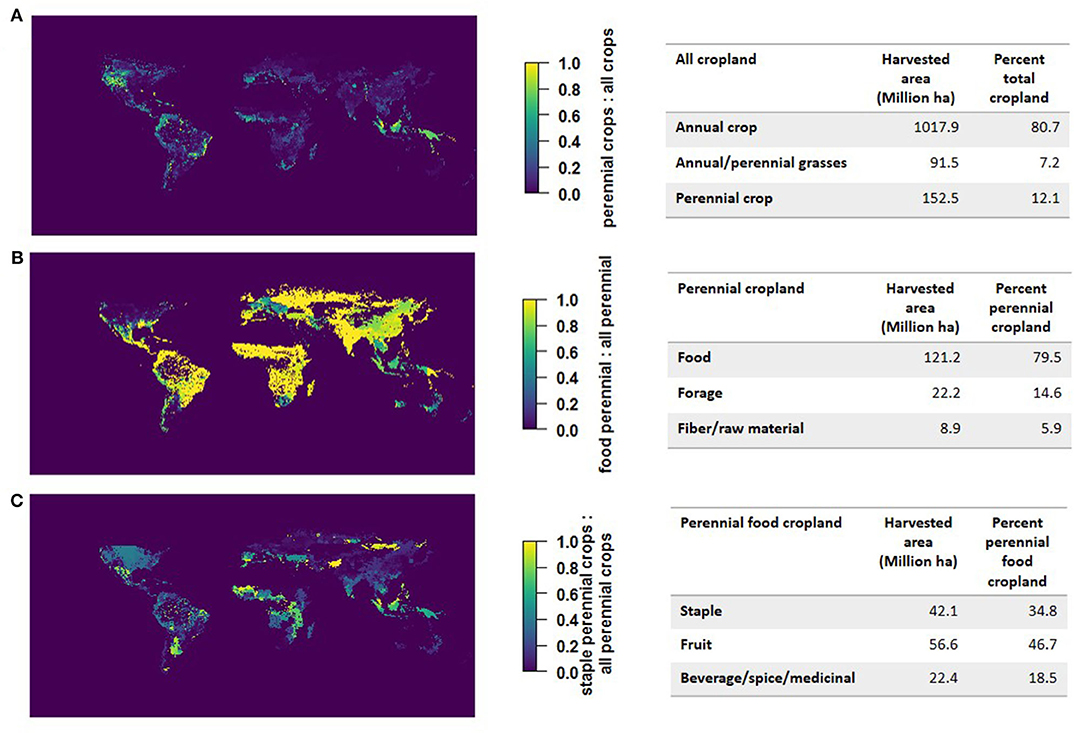
Figure 2. Land in perennial production. (A) Perennial cropland as a ratio of total cropland. (B) Food-producing perennial cropland as a ratio of total perennial cropland. (C) Staple crop producing perennial cropland as a ratio of total food-producing perennial cropland. Data categorized and re-analyzed from EarthStat database (Monfreda et al., 2008). Figures and table results were produced in R using the raster, rasterVis, and maps packages.
The estimate of 42.1 million hectares, or 4.2% of total cropland in perennial staple crop production (Figure 2) is an underestimate for two reasons: first, the Monfreda et al. (2008) dataset is more than a decade old; more recent UN FAO data gives a total of 65.4 million ha, or 4.7% of total cropland in perennial staple crop production (FAO, average of 2013-2017). Second, as mentioned, both the UN FAO and Monfreda et al. (2008) datasets are based only on 15 perennial staple crops. For example, pecan, macadamia, and sago palm are three perennial staple crops with at least some accessible harvested area data that are not included in these global datasets. The area of pecans harvested from the US and Mexico (which dominate world production) in 2019 totalled ~320 000 ha (SIAP, 2020; USDA, 2020). The area of macadamia harvested in 2018 is around ~65 000 ha (calculated by dividing global production in pounds by Hawaii yields; AgriOrbit, 2018; USDA, 2020). The area of sago palm harvested is as high as 6.5 million ha according to Bintoro et al. (2018), though some of this area overlaps with diverse smallholder polycultures. These estimates, together with the minor global and regional crops that have limited or no available area data indicate that even the more recent UN FAO estimates are low. Nonetheless, the geographic distributions and overall ratios between the different crop types summarized in Figure 2 are likely to be similar given that underreporting in the UN FAO data may be occurring for both minor perennial and annual crops.
Of the 51 perennial staple crops in our dataset, 62% are subtropical or tropical, 19% are temperate or Mediterranean, and 19% span across temperate to tropical ranges (Table 4; Supplementary Table 1).
Whether in the tropics or in temperate climates, the current area harvested of perennial crops does not necessarily correspond to yields (i.e., production per unit area; Figure 3A). Oil palm is an outlier in that it far outcompetes the other crops in both the yield and area of production, but if this crop is left out of the data, there is no correlation between the yield and area in production of perennial staple crops for which production data is available (P = 0.94). This is not unexpected in a system that optimizes for profitability rather than food production, but it highlights the potential gains in food production that are possible under differently regulated market conditions.
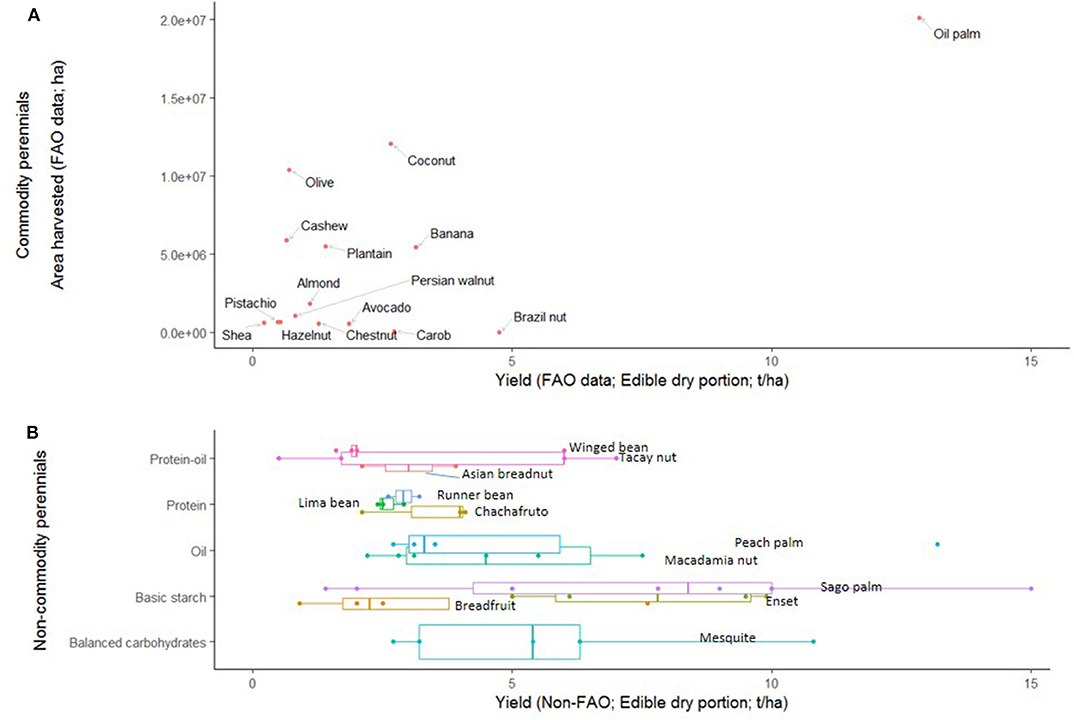
Figure 3. Yields and areas harvested. (A) Perennial staple commodity crops with both yield and area harvested data from the UN FAO. Yields and area harvested are not significantly correlated if oil palm is removed from the data (P = 0.94). (B) Top-yielding perennial staple crops (>2.5 t/ha on average) that do not have FAO production data, plotted on the same scale.
Indeed, many perennial crops with potentially high yields are not global commodity crops (Table 4), and therefore their total production and harvested areas are not known. These high-yield potential non-commodity perennial staples (those with a mean yield of >2.5 t/ha; Figure 3B) have comparable or potentially higher yields to many global commodity crops. Indeed, only 29% of the perennials in our wider dataset of 51 crops were defined as global crops, while the remaining 71% were minor global, regional, or new/historical/wild/experimental crops (Table 3; Supplementary Table 1).
Nutrition of Perennial Staple Crops
The three macronutrients on which we based our categorization (carbohydrates, proteins, and fats) fulfill people's basic caloric needs, while micronutrients (vitamins and minerals) provide essential additional components of nutrition. Figure 4 shows the crops with the highest proportion of the three staple nutrients on the basis of dry edible weight (Figures 4A–C). For carbohydrates, sago palm, cassava, and enset have the highest proportion of starch. This is unsurprising since both sago palm and enset's nutritional data pertain to a processed product which is extracted from the tree trunk. Breadfruit, chestnut, mesquite and plantain also rank in the top 85 percentile for carbohydrate content.
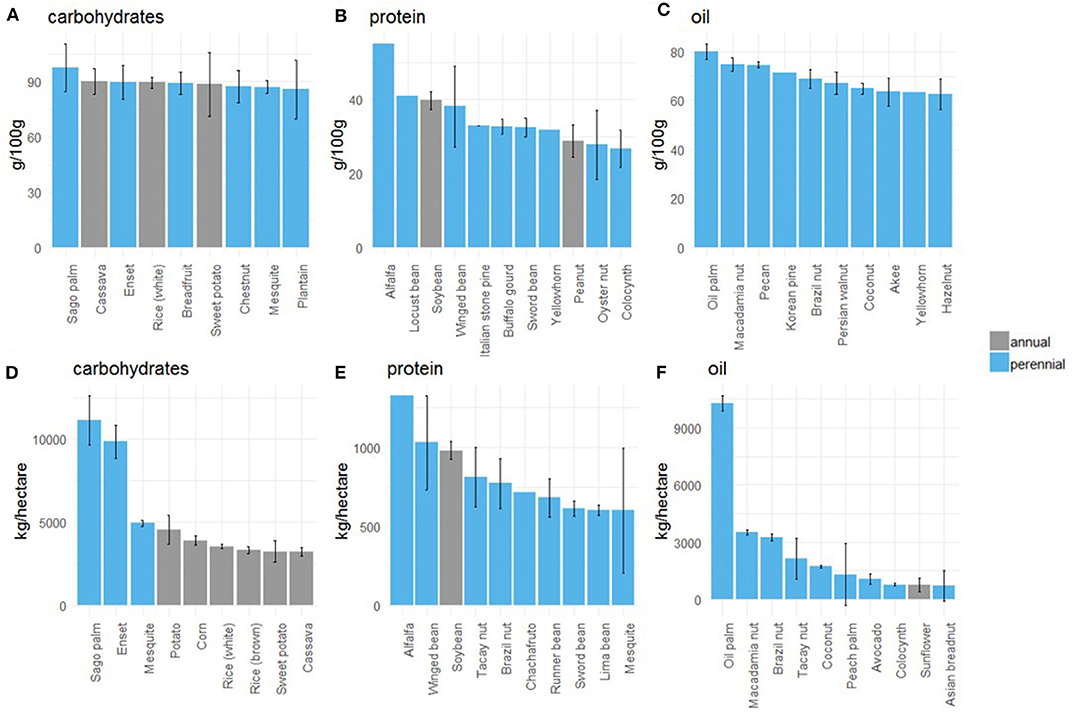
Figure 4. Macronutrient content and yields of perennials relative to select annuals. (A–C) Show staple nutrient content (g/100 g) for leading crops (those in the 85th percentile and higher for that nutrient). Nutrient content is based on the dry, edible portion of each crop. (D–F) Show estimated yields of each staple nutrient (kg/hectare) for leading crops (those in the 85th percentile and higher for each respective nutrient yield). If available, yields from the UN FAO were used; for crops without UN yield data, we used a mean yield estimate from our literature review. Error bars are 95% CIs based on distribution of nutrient data only; if absent, only one source of nutritional data was available for that nutrient of that crop.
For protein content, alfalfa LPC ranks first; this nutritional information pertains to a processed tofu-like extract, not the raw leaf. A variety of legumes as well as less familiar perennial crops (yellowhorn—Xanthoceras sorbifolium Bunge, oyster nut—Telfairia pedata Hook., colocynth—Citrullus colocynthis [L.] Schrad.) make the top 85th percentile along with the annuals soybean and peanut.
The crops with the highest concentration of oil were all perennials. Rapeseed (Brassica napus L.), an annual which is chiefly grown for oil, was not included because its nutrient content was not available in raw form (only as a pressed oil), but other annuals which are regularly pressed for oil (sunflower, safflower, soybean, corn etc.) were included.
Based on the combination of nutritional density with yield estimates, perennial crops can produce large amounts of macronutrients per unit area (nutrient yield; Figures 4D–F). These macronutrient yields provide several insights: for example, mesquite, a perennial we classified in the “balanced carbohydrate” category could produce more carbs per unit area than other perennial crops in the “basic starch” category like plantain and banana (these two do not appear in the top 85th percentile of carbohydrate production per unit area). Thus, crops with a lower proportion of a particular nutrient might still produce more of that nutrient per unit area if their yields are high. This analysis shows that for all three categories, the top crop for nutrient content (sago palm, alfalfa LPC, and oil palm Figures 4A–C) is also the top crop for nutrient production per unit area (Figures 4D,E). The presence and rankings of subsequent crops differ variously in each category. In all three categories, nutrient production per unit area (Figures 4D–F) dropped off faster than nutrient content (Figures 4A–C), indicating that yields are more variable than the staple nutrient content of top staple crops, particularly for carbohydrates and oil.
Perennial staple crops also provide other micronutrients, which are important for addressing both traditional and industrial malnutrition (see methods), including fiber, calcium, iron, magnesium, zinc, folate, and vitamins A, C, and E. We found that specific perennial staples are abundant in specific micronutrients, indicating that they can be a nutrient-dense part of diets, unlike the most ubiquitous annual staple crops in people's diets (corn, wheat, rice) that do not appear in the top 85th percentile for any of the 9 micronutrients (Figure 5).
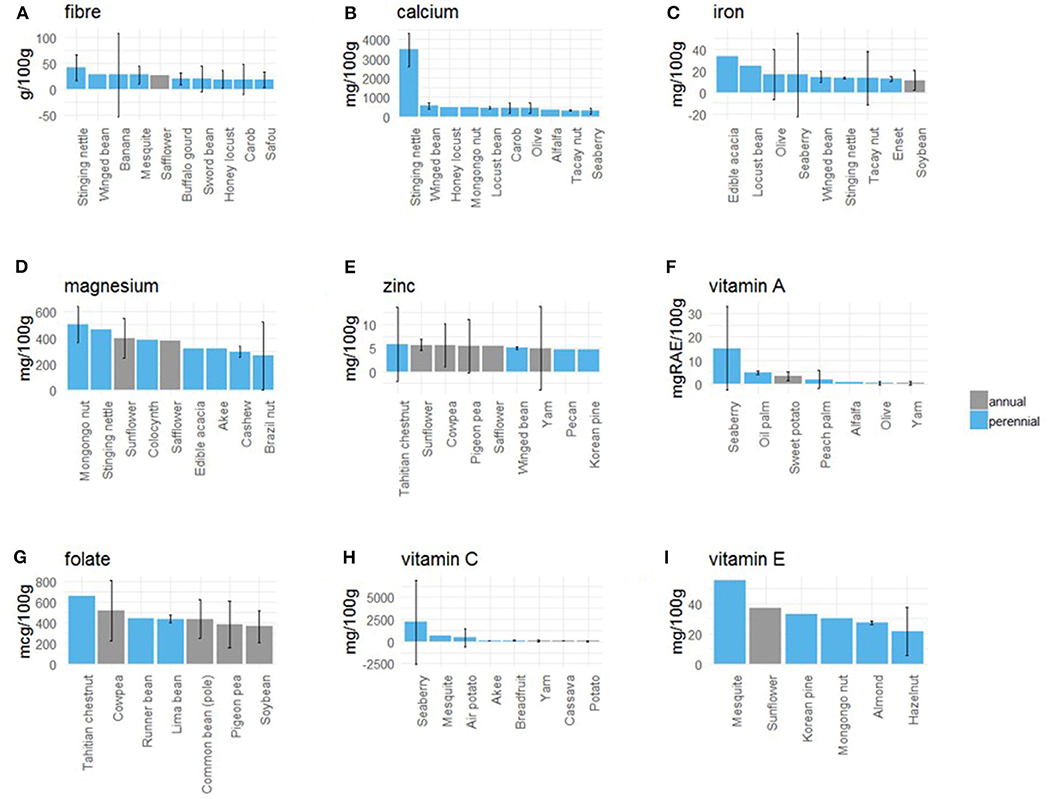
Figure 5. Micronutrient content of perennials. Plots (A–I) show the crops in the 85th percentile and higher for micronutrient content (g/100 g). Error bars are 95% CIs; if absent, only one source of nutritional data was available for that nutrient of that crop. Nutrient content is based on the dry, edible portion of each crop. Note that pigeon pea can be grown as an annual or perennial, but there isn't separate nutritional information for each. Error bars reflect wide nutrient composition variation reported in the literature, and may reflect different crop varieties, soils, and growing systems. For example, there are hundreds of varieties of bananas, seaberry (Elaeagnus rhamnoides) is a new crop with a wide genetic base, and Tahitian chestnut (Inocarpus fagifer) is grown on hundreds of Pacific islands, with populations that have been isolated by thousands of miles for millennia.
Carbon Sequestration of Perennial Staple Crops
Perennial staple crops, like other perennial land cover types, accumulate and hold carbon in their biomass and soils over their lifetimes. From biomass carbon and soil carbon estimates with harvested areas for 19 of the most commercialized perennial staple crops (see methods), we found that perennial staple crops are currently holding ~11,386 MTC globally (Table 5), less than one third of the annual anthropogenic emissions from all sources for 2018 of 37 Gt CO2 equivalents (Le Quéré et al., 2018). These stocks do not take into account the fate of stored carbon at the end of a typical growing cycle, which could differ significantly depending on how biomass and soil are managed during clearing and replanting, but rather represent mean standing stock. If this same agricultural land was under annual cover, it would be sequestering ~8,958 MTC globally, due to lower soil carbon stocks and lower mean biomass of annual crops.
Based on a linear projection of the increase in land under perennial staple production for 15 crops, this standing stock would grow to 14,389 MTC in the year 2040. The land cover that is replaced by perennial staple crops in this projection determines whether this standing stock is likely to represent a net increase or decrease in stored carbon: conversion from annual cropland and grassland would likely increase sequestered carbon (Table 4). The opposite is true for land transition from forested land to perennial staple crops, as the latter generally hold substantially lower carbon stocks than do intact forests (Lasco, 2002). For carbon stocks and projected changes in carbon per crop, see Supplementary Table 2.
While these simplified calculations of 100% conversion from one cover type (annual cropland, grassland or forest) to perennial staple crops do take into account the climate in which the conversion takes place (see Methods) they do not represent more realistic scenarios where increases in harvested area for each perennial staple crop replaces a geographically-informed amount of cropland, grassland, and forest. Our estimates are conservative in that they only include crops for which we had harvested area data at present (19 crops) and historically (15 crops) (Supplementary Table 1).
Discussion
Agronomy of and Nutrition From Perennial Staples
Our collation of yield data indicates that numerous perennial staple crops have yield ranges that are competitive with familiar annual staples. The extent of perennial staple crop production is currently modest covering 4.2–4.7% of total cropland. In addition, specific perennial staple crops have high macronutrient and micronutrient content necessary for fulfilling people's dietary needs.
The yield ranges we found in the literature were often wide. This reflects the diverse climates, soils, and management practices under which crops are cultivated, as well as different levels of maturity of crops in various studies. The yields reported here for non-FAO crops should be interpreted as the range of potential yields, not as actual estimates of global yields. Indeed, even some global crops (macadamia and pecan, two highly commercialized crops in the oil category), do not have FAO yield and production data. Nonetheless, the ranges of yields collected here highlight crops with high yield potential. Eleven of the 12 perennial staple crops with the highest yield potential (estimated yields above 2.5 t/ha) are subtropical to tropical crops (Figure 3B). Perennial staple crops in the temperate North and Mediterranean are certainly not to be disregarded, but the variety of perennial staples with high yields in the tropics and subtropics justifies particular research and policy attention.
As this study only includes perennial staple crops for which we could find yield estimates with adequate evidence, many interesting crops were not included here at all. Crops with no available yield information, or those without citable yield information, should not be neglected; on the contrary, they require more scientific attention. For example, oyster nut (Telfairia pedata Hook.), safou/butterfruit (Dacryodes edulis [G.Don] H.J.Lam), African breadnut (Treculia africana Okafor), and souari nut (Caryocar villosum Pers.) are all crops that are used as regional staples, but for which we could not find citable yield information. Toensmeier (2016) has assembled comprehensive lists of perennial staple crops, most of which lack yield information, which could be the basis of further study. Other clearinghouses for information on plants such as Plant Resources of Africa should ensure that the information they provide is cited to its source.
Nutritional information was generally more available than information on crop yields. This may be due to the fact that many of the diverse and barely-documented perennial staple crops cited in Toensmeier (2016) are grown in diverse agroforestry settings rather than plantation settings (discussed further below) where per-hectare yields are hard to calculate and not well-documented. These little-documented local and traditional crops are likely to be contributing substantially to both the nutritional and cultural health of communities, which rely on diversity of foods, not only quantity of calories (Smith and Haddad, 2015). Of course, many annual staple crops are also nutritionally important and constitute an essential part of gastronomic cultures. Moreover, specific annual crops may be more nutritionally dense than their perennial counterparts in some cases, as documented in one study of micronutrients of annual and perennial Phaseolus species (Schier et al., 2019). We do not advocate for the outright replacement of annual crops, but rather, for a re-emphasis on perennial staple crops in those geographic and cultural contexts where exotic annual staple crops have been privileged by development agencies and colonial sensibilities over culturally established, Indigenous, perennial staple crops (Brandt et al., 1997; Chifamba, 2011; Rogé et al., 2016).
Perenniality and Growth Form
While most of the plants discussed here are woody trees and shrubs, some are perennial vines, some are perennial herbs (alfalfa, stinging nettle), and some like banana, plantain and enset, are giant tree-like herbs. The range of lifespans among the crops is large: from just 2 to 3 years (pigeon pea) to many hundreds or up to a thousand years (Brazil nut, olive). Several short-lived perennial staple crops including sorghum (Sorghum bicolor [L.] Moench) and cassava (Manihot esculenta Crantz) have not been included here, because while they can be grown perennially for 2–3 seasons, they are usually cultivated as annuals, and data on their yields as perennials is lacking. The practice of ratooning (cutting crops near their crown after a first harvest) is an Indigenous management practice in Western Africa that predates the cultivation of sorghum and pigeon pea as annuals, and can result in consistent second and third harvests with minimal effort under some conditions (Rogé et al., 2016). Thus, while we were able to include pigeon pea in this study, more data is needed on the yields and other outcomes of growing other herbaceous or shorter-lived perennial crops for multiple seasons, and the cultural practices that enable this.
Most of the crops in this study produce edible fruit (including soft fruits, nuts, beans, and capsules) that are harvested year after year without damaging the plant. In contrast, the edible content of sago palm and enset, two of the highest yielding crops in this study, is stored in their trunks or underground corm, and are harvested at the end of a multi-year growth cycle. For sago, the individual trunks are destroyed when they are harvested, but the plant is a multi-stemmed palm that continues to produce new trunks for many years, and will have multiple trunks of different ages present in each clump at any given time. Enset can be harvested for its trunk only, in which case stems will regrow, but also for its corm, in which case the harvest is terminal. African yam bean and air potato are interesting in that they produce staple foods on an ongoing basis perennially (beans and aerial bulbils, respectively), but also have edible tubers that can be harvested at the end of the plant's productive life or when they are needed. The destructive, terminal harvest of vegetative parts of perennial plants can serve as a “famine buffer” in times of scarcity.
For example, enset ensured greater food security for millions of people in Ethiopia's Southern highlands during the famines of the 1970s and 1980s (Brandt et al., 1997). But, enset stands are sometimes being harvested earlier than the full 4–11 year cycle, thereby depleting stands before they mature (Borrell et al., 2020). Thus, while the harvest of stored calories in enset trunks and corms can mitigate food shortages for a time (Brandt et al., 1997), the depletion of enset stands or their loss due to other reasons reduces their subsequent ability to mitigate further disruptions in the food supply and they take longer to recover (Quinlan et al., 2015). This example demonstrates how perennials with edible vegetative parts that are destructively harvested can serve as food “insurance” but cannot do so indefinitely. If they are used as a line of defense when annual food supplies diminish, they must be accumulated ahead of time and replenished during times of plenty.
Perennial Staple Crops in Agroforestry Systems
Perennial staple crops can be managed in many ways, from carbon-intensive fertilized and sprayed monocultures, to intensively managed but lower input diversified perennial polycultures that integrate several species of woody and herbaceous plants as well as animals. When perennial crops are managed in diverse systems, they can embody the ecological principles of spatial and temporal heterogeneity, redundancy, and functional diversity which are key for supporting biodiversity, ecosystem services, and resilience in agroecosystems (Jose, 2009; Cabell and Oelofse, 2012).
As discussed in greater depth in Toensmeier (2017), perennial staple crops are already featured in many agroforestry systems across many climates (Table 5). These include using perennial staples as edge components like field boundary plantings and riparian buffers; integrating perennial staples with annual crops in linear alley-cropping systems or in scattered irregular patterns; grazing livestock in the understory of perennial staples including livestock directly consuming dropped fruit and nuts; and using perennial staples as the main productive components of fully integrated mutistrata systems.
Tropical homegardens, which embody the more diverse end of this management spectrum, feature staples like bananas, avocados, and coconuts. These systems, which have been extensively studied, provide many social, economic, and environmental functions, with particular benefits for food and nutrition (Méndez et al., 2001; Scales and Marsden, 2008). Intensive agroforestry systems (diversified, larger-scale, multistrata systems) that are common on Pacific islands, typically focus on the staple crops like coconut and breadfruit (Toensmeier, 2017). In contrast, other multistrata agroforestry systems have lost their traditional functions and structural properties to various extents, and function mainly as providers of export commodities (producing coffee, cocoa, tea, fruit, and vanilla) rather than calorically and nutritionally important foods. However, some of these are shade crop plantations which include overstory trees that produce perennial staples as well, such as peach palm, coconut, macadamias, and bananas over coffee or cocoa (Toensmeier, 2017). In the US, Multifunctional Woody Polyculture (MWP) is a staple crop system under development, featuring woody staple crops that are typically machine harvested combined with annual crops, hay, or grazing in the understory and alleys (Lovell et al., 2018).
Despite these numerous examples of perennial staple crops in agroforestry systems, the five agroforestry practices as defined by the United States Department of Agriculture (USDA; riparian buffers, forest farming, alley cropping, shelterbelts, and silvopasture) do not include growing tree crops in and of themselves, whether as monocultural plantations or as mutistrata systems (USDA, 2019). We agree with some authors who have suggested including all tree crops under the agroforestry umbrella (van Noordwijk et al., 2016). The inclusion of tree crops (and perennial staple crops among them) in agroforestry literature is essential to forward the integration of agronomic and environmental information about these systems for the full range of conditions under which they are grown—from simplified monoculture, to diverse polyculture; from wild harvested to fully domesticated.
Climate Regulation and Ecology of Perennial Adoption
Perennial staple crops hold a relatively modest amount of carbon within the global carbon budget, comprising less than one third of the annual emissions from the year 2018 (Le Quéré et al., 2018). Our estimates indicate that land transition from annual crops to perennials will increase these carbon stocks by approximately one additional GtC over 20 years, while transition from mature forests to perennials will reduce carbon stocks by over three times as much (Supplementary Table 1). When perennial staple crops replace grassland, there is carbon sequestration globally, but whether carbon is sequestered or emitted is highly context dependent and is determined by the crop and the climate of the grassland that is being replaced (Supplementary Table 1). The relatively modest amount of carbon held in perennial staple crops, and the small but positive sequestration from transitioning land from annual to perennial food crops indicates that such land transitions can only be one small part of the transformational change necessary to avert climate and ecological disaster (IPBES, 2019b; IPCC, 2019a).
This analysis was exploratory and did not include several important factors, including (1) a comparison to grasslands degraded by excessive grazing, which are likely to benefit more from a transition to perennials in terms of carbon and soil health (including dust-reduction) than the grasslands represented in this analysis; (2) non-CO2 emissions like nitrous oxide and methane resulting from fertilization regimes and livestock occupation, and (3) management of perennial staple crops together with a grassy understory, in which case the carbon sequestration of woody perennial crops and grassland may be additive, rather than the former replacing the latter.
Future work using more spatially explicit scenarios of land transitions to perennials for each crop would improve the rough global estimates presented here. This work is particularly important because many of the high-yielding crops assessed here are tropical, where forest conservation is of critical importance to biodiversity and climate regulation (Wilcove and Koh, 2010). However, such modeling work will need to confront a lack of available data: the climate regulation services provided by long-lived perennials is well-documented for perennial grasslands, forest cover, and timber plantations (IPCC, 2019b), but the same is not the case for tree crops: current IPCC carbon estimates for tree crops only include two perennial staple crop species (olive and oil palm) out of a total of only six tree crop species (volume 4, chapter 5 table 5.3; IPCC, 2019b). This dearth of information about tree crops in the most updated and world-class dataset available is troublesome, and will hamper planning and policy making efforts. We have improved on available data by collecting biomass carbon data and typical life spans from the literature for an additional 17 crops (freely available on Figshare figshare.com/projects/Perennial_Staple_Crops/78756). These estimates are still limited samples that hinder robust calculations of the mean and maximum carbon stocks (above and below-ground) for various species over the course of their productive lifetimes from available literature. The management-dependent nature of these properties of perennials means that there will always be considerable variability within one crop, yet even with this caveat, the literature on the carbon sequestration capacities of tree crops is still underdeveloped compared to forestry and agroforestry systems.
From a biodiversity and ecosystem services perspective, research has shown that tree crops that are managed with permanent or diversified understory vegetation can boost ecosystem services including pest control (Simon et al., 2010), increased root infiltration of beneficial mycorrhizal fungi (Ishii et al., 2008; Cruz and Ishii, 2012), soil carbon content (Merwin et al., 1994; Xi-rong, 2005), and water retention (Merwin et al., 1994; Huai and Lin, 2005), as well as decrease nutrient loss from soil (Gómez et al., 2009). In addition, diversified perennial systems in the tropics harbor higher biodiversity compared to simplified perennial plantations and annual crops (Perfecto et al., 2003; Frishkoff et al., 2014). Following studies in the tropics and meta-analyses of non-food producing agroforestry systems in temperate climates (Torralba et al., 2016) it is likely that temperate tree crops with greater complexity also support more biodiversity. But, the magnitude and context dependence of other benefits from diversified management, particularly in the temperate North, are largely unknown due to a lack of synthetic meta-analysis on understory management practices of tree crops for various services (apart from pest control, covered in Simon et al., 2010). In addition, some woody perennial crops (such as nut trees in California; Wilson et al., 2016) require more water than annual crops and can increase water scarcity in arid environments. Thus, planting tree crops, like other reforestation efforts, must take into account water availability so that they do not worsen water availability for other uses (Zastrow, 2019).
Taken together, the expansion of perennial staple cropland (as well as non-staple perennial cropland and agroforestry practices like silvopasture) into existing annual cropland or existing pasture land represents a potential win-win scenario between environmental benefits and sustained food production. But realizing such benefits will likely require environmentally friendly management practices like appropriate species choice in water-restricted areas, woody polyculture, continuous diversified groundcover/cover-cropping, or mulching (see references in paragraphs above).
Societal Aspects of Perennial Adoption
Crop adoption and land transition is a multifaceted process that involves market demand, land tenure, environmental regulations (or lack thereof), farm subsidies and incentive programs, need for and availability of capital, insurance and labor, and other local and regional political dynamics. But if there are so many perennial staple crops with good yields, abundant nutrition, and environmental benefits, why are they not already a larger part of the global food system?
Before discussing barriers, it is important to recognize that many of the crops discussed here (and ones that are not included in this study), while not heavily commercialized and unfamiliar in the West, are already important products locally and regionally. While some of these are being developed through research and business enterprises (peach palm, tacay nut, sago) some of these crops are declining in production, and losing valuable genetic variation in the process (enset, yeheb nut; Tsegaye and Struik, 2002; Yusuf et al., 2013).
Perennial crops face several barriers. In the developed world, the economics of investing in perennials aren't well-supported. Perennial enterprises entail a delayed return on investment due to the length of time between planting and bearing; moreover, the relative permanence (i.e., lower flexibility to convert) of perennial crops gives them a lower option value, thereby lowering farmer's propensity to adopt these practices even if they are profitable on paper (Frey et al., 2013). This reality means that perennial farmers and businesses need access to capital years in advance. Given price volatility and/or undeveloped markets for many perennial staples such enterprises may be risky and harder to capitalize than shorter term enterprises (Wolz et al., 2017). Crop insurance programs and futures markets help mitigate agricultural risk, but these are not available for many perennial staple crops, even ones that are already global commodities. In addition, access to land for long-term farming enterprises is unusual (outside of land ownership). Farmers that do not own their land need to have access to long-term leases with terms that ensure their investments if land is sold, and other long-term provisions. This issue has begun to receive some attention in the US Midwest (for example, the non-profit organization Savanna Institute offers a long-term lease workbook for farmers and landowners), but such long-term arrangements are still highly unusual. Third, research and breeding development devoted to perennial staple crops is lacking compared to annual crops, and more difficult due to longer generation times (Mehlenbacher, 2003; Molnar et al., 2013). A research and development agenda laid out nearly a century ago by the scholar J Russel Smith in his book Tree Crops, A Perennial Agriculture (Smith, 1929) to accelerate breeding of tree crops for food and fodder in order to replace tilled agriculture on hilly landscapes is just as relevant today and largely unfulfilled.
In areas of the developing world that are vulnerable to food insecurity, the agendas of aid agencies have concentrated heavily on improvement in cereal production at the expense of other crops including perennial staples that are grown traditionally (Brandt et al., 1997; Chifamba, 2011; Rogé et al., 2016). Such an approach may be counterproductive for both local food security as well as cultural values.
Finally, perennial crops are vulnerable to war and other social upheavals. Some cultures have established protections for perennial crops, such as the laws of war in the Hebrew Bible that dictated that food-producing trees were not to be cut down during siege and warfare (Deuteronomy 20:19). Nevertheless, perennial crops have fallen victim to conflicts throughout history, including in this century (Orians and Pfeiffer, 1970), and unlike annual crops which can be replanted the next season, take years or decades to recover.
Despite these barriers, in-demand global perennial staples like oil palm, avocado, and almond have become highly lucrative commodities. For example, oil palm production has replaced large tracts of rainforest (Guillaume et al., 2018), the Mexican avocado industry has been taken over by gangs (Flannery, 2017), and almonds in California exert unsustainable water demand on the state (Wilson et al., 2016). Thus, expansion of perennial staple cropland is not a solution divorced from specific environmental and social contexts, which can be exploitative and unsustainable. Nevertheless, unlike these rapidly-expanding crops, some perennial staples (for example olives in the Mediterranean basin, chestnuts and evergreen oak in Corsica, Portugal and Spain, mesquite in the American Southeast, and a large variety of perennial staples in tropical homegardens) have been sustained in ecologies and ways of life for millennia (e.g., Michon, 2011; Uylaşer and Yildiz, 2014).
The preservation and active development of diverse germplasm from perennial staple crop species—many of which are culturally important but largely neglected in agronomic research—is a key benefit for both nutrition and the evolutionary ecosystem service of genetic resources (Faith et al., 2010). Undoubtedly, these crops require crop-specific research programs, and bridging organization champions, such as Dr. Daniel Adewale's research group working on African yambean, Dr. Diane Ragone and the Breadfruit Institute's work on breadfruit, and Dr. Peter Felker and Desert Harvesters work on mesquite (among many others). According to an assessment of breeding potential in nuts, the main impediment to quick progress in genetic improvement of these crops is the limited size and resources of breeding programs, not the available genetic resources, which are vast (Mehlenbacher, 2003). Thus, the research resources, cultural resonance, and social capital devoted to perennial staple crops are paramount to their uptake, not just their current nutritional and botanical properties.
Policy solutions that aim to promote tree-planting are often fraught with difficulties and can even be counterproductive for carbon sequestration and wildlife if they result in the replacement of native vegetation with plantations, as has occurred in Chile and other locations (Heilmayr et al., 2020). However, while incentive programs like Payments for Ecosystem Services (PES) can backfire, they can also have substantial positive effects if designed well (Rode et al., 2015; Chan et al., 2017). Reviews show that effective PES incentive programs also provide technical expertise and co-payments (rather than covering the entire cost), recognizing that farmers often have non-monetary incentives to manage their lands in environmentally benign ways (Rosa et al., 2004; Wilcove and Lee, 2004; Chan et al., 2017; Clot et al., 2017). Designing and implementing tree crop programs to reflect local relationships and cultural values regarding species and practices is a key for uptake and program success (Chapman et al., 2019, 2020). Despite this opportunity, there may be little use in creating additional boutique incentive programs if underlying policies still lend conventional practices disproportionate favor (for example, large subsidies or the lack of crop insurance for annual crops; Chan et al., 2019).
Considering the various social and ecological dynamics we have discussed, the most prevalent global perennial staples may or may not be the best candidates for further production increases. Other high-yielding crops (sago, enset, breadfruit, mesquite, peach palm, Asain breadnut, perennial vining beans, tacay nut) that are currently only regional or experimental crops, could be candidates for production increases. However, if such increases occur under a business-as-usual model, they would likely fall prey to similar problems that other “superfoods” that have experienced a rise in popularity have driven, namely carbon and environmental impacts from land clearing, use of chemicals, and transportation, coupled with a boom-and-bust dynamic that causes social problems for communities (Magrach and Sanz, 2020). As with these “superfoods,” the implications of increasing production of perennial staple crops depends deeply on their social, cultural and economic contexts. For example, in the case of intensifying production of one perennial staple crop in a system that is already predominantly polycultural, transitions could lead to a loss of plant cover of other species and a loss of culturally important landscapes. In contrast, transitions to perennial staples from some annual croplands, or transition of perennial monocultures to more complex perennial polycultures could drive increased biodiversity and provide cultural benefits, provided that these systems are developed by and for local people (Kreitzman, 2020).
Whether production of perennial staple crops and land transitions are geared toward export, or local consumption, whether they are fueled by smallholders or highly capitalized enterprises, and whether they are governed using ecological as well as economic priorities will ultimately dictate their outcomes.
Conclusion
This study demonstrates a substantial potential for perennial staple crops to contribute to the global food supply. Its framework of perennial staple crops enables researchers and practitioners to identify or classify crops according to nutritional categories and cultivation status. We found that despite structural barriers to perennial adoption in a system that emphasizes short term returns and continuous increases in cereal yields, perennial staple crops constitute a small but significant (4.5%), and growing portion of global cropland. At least one perennial staple crop in each of the five nutritional categories had yields over 2.5 t/ha, in some cases considerably higher, competitive with and in many cases exceeding those of nutritionally comparable annual staples, suggesting that transition of agricultural land to perennial crops may not entail yield losses in some areas. Most perennial staple crops, including many of those with high potential yields, are regional crops (not globally traded) that grow in the subtropics to tropics, suggesting that there is space for market expansion and increased investment in some crops.
Many perennial crops also had macronutrient densities and yields (per unit area) that were competitive with annual staples; moreover, specific perennial staples are abundant in specific micronutrients, indicating that they can be a nutrient-dense part of diets, unlike the most ubiquitous annual staple crops (corn, wheat, rice), which do not appear in the top 85th percentile for any of the nine micronutrients analyzed. Perennial staple crops currently hold 11,386 MtC above and below ground contributing to climate regulation. If linear growth in land under perennial staple production continues to 2040, and replaces annual cropland, 965 MtC could be sequestered. The yield, nutrition, and carbon sequestration data assembled in this study indicate that transition of land and diets to perennial staple crops, if judiciously managed, can provide win-win solutions for both food production and ecosystems. However, examples of unsustainable expansion of perennial staple crops mean that such ideal scenarios are by no means to be taken for granted. If implemented to replace existing agricultural land rather than natural land cover, and while prioritizing local values and equitable access to nutrition rather than absolute yields, transition of land and diets to perennial crops can play a positive role in global food systems.
Data Availability Statement
The datasets presented in this study can be found in online repositories. The names of the repository/repositories and accession number(s) can be found at: https://figshare.com/projects/Perennial_Staple_Crops/78756.
Author Contributions
MK did the data collection for yields and carbon sequestration and did the analysis. ET did the data collection for nutrition. All co-authors wrote the manuscript.
Funding
This research was funded by the Ekhaga Foundation grant # 2017-52. MK was supported by a Canadian Natural Science and Engineering Research Council (NSERC) PGS-D scholarship.
Conflict of Interest
The authors declare that the research was conducted in the absence of any commercial or financial relationships that could be construed as a potential conflict of interest.
Acknowledgments
We would like to thank Dr. Alicia Ledo for her generous guidance on the carbon stock analysis and for providing soil carbon stock estimates, Santiago Tomassi for his work in the data collection for this project, and Julie Fortin for her assistance on GIS mapping.
Supplementary Material
The Supplementary Material for this article can be found online at: https://www.frontiersin.org/articles/10.3389/fsufs.2020.588988/full#supplementary-material
References
Adewale, B. D., Dumet, D. J., Vroh-Bi, I., Kehinde, O. B., Ojo, D. K., Adegbite, A. E., et al. (2012). Morphological diversity analysis of African yam bean (Sphenostylis stenocarpa Hochst. ex A. Rich.) harms and prospects for utilization in germplasm conservation and breeding. Genet. Resour. Crop Evol. 59, 927–936. doi: 10.1007/s10722-011-9734-1
Adewale, B. D., Vroh-Bi, I., Dumet, D. J, Nnadi, S., Kehinde, O. B., Ojo, D. K., et al. (2015). Genetic diversity in African yam bean accessions based on AFLP markers: towards a platform for germplasm improvement and utilization. Plant Genet. Resour. 13, 111–118. doi: 10.1017/S1479262114000707
Asbjornsen, H., Hernandez-Santana, V., Liebman, M., Bayala, J., Chen, J., Helmers, M., et al. (2014). Targeting perennial vegetation in agricultural landscapes for enhancing ecosystem services. Renew. Agric. Food Syst. 29, 101–125. doi: 10.1017/S1742170512000385
Bailey, D., Schmidt-Entling, M. H., Eberhart, P., Herrmann, J. D., Hofer, G., Kormann, U., et al. (2010). Effects of habitat amount and isolation on biodiversity in fragmented traditional orchards. J. Appl. Ecol. 47, 1003–1013. doi: 10.1111/j.1365-2664.2010.01858.x
Bainbridge, D. A. (1985). The rise of agriculture: a new perspective. Ambio 14, 148–151. doi: 10.1080/00690805.1985.10438308
Beer, R., Kaiser, F., Schmidt, K., Ammann, B., Carraro, G., Grisa, E., et al. (2008). Vegetation history of the walnut forests in Kyrgyzstan (Central Asia): natural or anthropogenic origin? Quat. Sci. Rev. 27, 621–632. doi: 10.1016/j.quascirev.2007.11.012
Benjamin, R., Cédric, G., and Pablo, I. (2008). Modeling spatially explicit population dynamics of Pterostichus melanarius I11 (Coleoptera: Carabidae) in response to changes in the composition and configuration of agricultural landscapes. Landsc. Urban Plan. 84, 191–199. doi: 10.1016/j.landurbplan.2007.07.008
Bennett, A. B., and Isaacs, R. (2014). Landscape composition influences pollinators and pollination services in perennial biofuel plantings. Agric. Ecosyst. Environ. 193, 1–8. doi: 10.1016/j.agee.2014.04.016
Bintoro, M. H., Nurulhaq, M. I., Pratama, A. J., Ahmad, F., and Ayulia, L. (2018). “Chapter 2: growing area of sago palm and its environment,” in Sago Palm: Multiple Contributions to Food Security and Sustainable Livelihoods (Springer), 20–29. Available online at: https://www.springer.com/gp/book/9789811052682
Blesh, J., and Drinkwater, L. E. (2013). The impact of nitrogen source and crop rotation on nitrogen mass balances in the Mississippi River basin. Ecol. Appl. 23, 1017–1035. doi: 10.1890/12-0132.1
Borrell, J. S., Goodwin, M., Blomme, G., Jacobsen, K., Wendawek, A. M., Gashu, D., et al. (2020). Enset-based agricultural systems in Ethiopia: a systematic review of production trends, agronomy, processing and the wider food security applications of a neglected banana relative. Plants People Planet 2, 212–228. doi: 10.1002/ppp3.10084
Brady, M. J., McAlpine, C. A., Possingham, H. P., Miller, C. J., and Baxter, G. S. (2011). Matrix is important for mammals in landscapes with small amounts of native forest habitat. Landsc. Ecol. 26, 617–628. doi: 10.1007/s10980-011-9602-6
Brady, N. C., and Weil, R. R. (1986). “Chapter 17: soil erosion and its control,” in The Nature and Properties of Soils, 11th Edn (Prentice-Hall, NJ, Upper Saddle River).
Brandt, S. A., Spring, A., Hiebsch, C., McCabe, J. T., Tabogie, E., Diro, M., et al. (1997). Tree Against Hunger: Enset-Based Agricultural Systems in Ethiopia. American Association for the Advancement of Science.
Cabell, J. F., and Oelofse, M. (2012). An indicator framework for assessing agroecosystem resilience. Ecol. Soc. 17:18. doi: 10.5751/ES-04666-170118
Campbell, B., Beare, D., Bennett, E., Hall-Spencer, J. M., Ingram, J. S. I., Jaramillo, F., et al. (2017). Agriculture production as a major driver of the earth system exceeding planetary boundaries. Ecol. Soc. 22:8. doi: 10.5751/ES-09595-220408
Cates, A. M., Ruark, M. D., Hedtcke, J. L., and Posner, J. L. (2016). Long-term tillage, rotation and perennialization effects on particulate and aggregate soil organic matter. Soil Tillage Res. 155, 371–380. doi: 10.1016/j.still.2015.09.008
Cavalcante, M. C., Oliveira, F. F., Maués, M. M., and Freitas, B. M. (2012). “Pollination requirements and the foraging behavior of potential pollinators of cultivated Brazil nut (Bertholletia excelsa Bonpl.) Trees in Central Amazon Rainforest,” in Psyche (Stuttgart). Available online at: https://www.hindawi.com/journals/psyche/2012/978019/ (accessed April 10, 2020).
Chan, K. M. A., Agard, J., Liu, J., Ana Paula, D. D. A., Armenteras, D., Boedhihartono, A. K., et al. (2019). “Pathways towards a Sustainable Future,” in Global Assessment Report of the Intergovernmental Science-Policy Platform on Biodiversity and Ecosystem Services, eds E. S. Brondízio, J. Settele, S. Díaz, and H. Ngo. doi: 10.5281/zenodo.3832100
Chan, K. M. A., Anderson, E., Chapman, M., Jespersen, K., and Olmsted, P. (2017). Payments for ecosystem services: rife with problems and potential—for transformation towards sustainability. Ecol. Econ. 140, 110–122. doi: 10.1016/j.ecolecon.2017.04.029
Chapman, M., Satterfield, T., and Chan, K. M. A. (2019). When value conflicts are barriers: can relational values help explain farmer participation in conservation incentive programs? Land Use Policy 82, 464–475. doi: 10.1016/j.landusepol.2018.11.017
Chapman, M., Satterfield, T., Wittman, H., and Chan, K. M. A. (2020). A payment by any other name: is Costa Rica's PES a payment for services or a support for stewards? World Dev. 129:104900. doi: 10.1016/j.worlddev.2020.104900
Chifamba, E. (2011). Cultivation and commercialization of indigenous fruit trees to improve household food security in dry regions of Buhera Zambabwe. J. Sustain Dev. Afr. 13, 95–98.
Clement, C. R., Weber, J. C., van Leeuwen, J., Domian, C. A., Cole, D. M., Lopez, L. A. A., et al. (2010). Why extensive research and development did not promote use of peach palm fruit in Latin America. Agrofor. Syst. 61, 195–206. doi: 10.1023/B:AGFO.0000028999.84655.17
Clot, S., Grolleau, G., and Méral, P. (2017). Payment vs. compensation for ecosystem services: do words have a voice in the design of environmental conservation programs? Ecol. Econ. 135, 299–303. doi: 10.1016/j.ecolecon.2016.12.028
Cruz, A. F., and Ishii, T. (2012). Sod culture management contributes to sustainable fruit growing through propagation of arbuscular mycorrhizal fungi and their helper-microorganisms and decreasing of agricultural inputs. Acta Hortic. 933, 477–484. doi: 10.17660/ActaHortic.2012.933.62
Daniel, J. N., and Ong, C. K. (1990). Perennial pigeonpea: a multi-purpose species for agroforestry systems. Agrofor. Syst. 10, 113–129. doi: 10.1007/BF00115360
de Baaij, J. H. F., Hoenderop, J. G. J., and Bindels, R. J. M. (2015). Magnesium in man: implications for health and disease. Physiol. Rev. 95, 1–46. doi: 10.1152/physrev.00012.2014
Del Valle, F. R., Marco, E., Becker, R., and Saunders, R. M. (1989). Development of products containing mesquite (Prosopis spp.) pod flour and their nutritional and organoleptic evaluation. J. Food Process Preserv. 13, 447–456. doi: 10.1111/j.1745-4549.1989.tb00118.x
Desert Harvesters (2008). “About Mesquite millings,” in Desert Harvest. Appreciating Native Foods Southwest. Available online at: https://www.desertharvesters.org/how-we-run-mesquite-millings/ (accessed April 9, 2020).
Díaz, S., Settele, J., Brondízio, E. S., Ngo, H. T., Agard, J., Arneth, A., et al. (2019). Pervasive human-driven decline of life on earth points to the need for transformative change. Science 366:eaax3100. doi: 10.1126/science.aax3100
Eycott, A. E., Stewart, G. B., Buyung-Ali, L. M., Bowler, D. E., Watts, K., Pullin, A. S., et al. (2012). A meta-analysis on the impact of different matrix structures on species movement rates. Landsc. Ecol. 27, 1263–1278. doi: 10.1007/s10980-012-9781-9
Faith, D. P., Magallón, S., Hendry, A. P., Conti, E., Yahara, T., Donoghue, M. J., et al. (2010). Evosystem services: an evolutionary perspective on the links between biodiversity and human well-being. Curr. Opin. Environ. Sustain. 2, 66–74. doi: 10.1016/j.cosust.2010.04.002
FAO (2020). FAOSTAT - Statistics Division Food and Agriculture Organization of the United Nations. Available online at: http://www.fao.org/faostat/en/#data/QC (accessed June 9, 2020).
Felker, P. (2005). Mesquite flour: new life for an ancient staple. Gastronomica 5, 85–89. doi: 10.1525/gfc.2005.5.2.85
Felker, P., Clark, P. R., Laag, A. E., and Pratt, P. F. (1981). Salinity tolerance of the tree legumes: mesquite (Prosopis glandulosa var.torreyana, velutina P, and Particulata) Algarrobo (chilensis) P, Kiawe (pallida) P, Tamarugo (P. tamarugo) grown in sand culture on nitrogen-free media. Plant Soil. 61, 311–317. doi: 10.1007/BF02182012
Ferguson, R. S., and Lovell, S. T. (2013). Permaculture for agroecology: design, movement, practice, and worldview. A review. Agron. Sustain. Dev. 34, 251–74. doi: 10.1007/s13593-013-0181-6
Flannery, N. P. (2017). Mexico's avocado army: how one city stood up to the drug cartels. The Guardian.
Fleskens, L., Duarte, F., and Eicher, I. (2009). A conceptual framework for the assessment of multiple functions of agro-ecosystems: a case study of trás-os-montes olive groves. J. Rural Stud. 25, 141–155. doi: 10.1016/j.jrurstud.2008.08.003
Foley, J. A., DeFries, R., Asner, G. P., Barford, C., Bonan, G., Carpenter, S. R., et al. (2005). Global consequences of land use. Science 309, 570–574. doi: 10.1126/science.1111772
Foley, J. A., Ramankutty, N., Brauman, K. A., Cassidy, E. S., Gerber, J. S., Johnston, M., et al. (2011). Solutions for a cultivated planet. Nature 478, 337–342. doi: 10.1038/nature10452
Frey, G. E., Mercer, D. E., Cubbage, F. W., and Abt, R. C. (2013). A real options model to assess the role of flexibility in forestry and agroforestry adoption and disadoption in the Lower Mississippi Alluvial Valley. Agric. Econ. 44, 73–91. doi: 10.1111/j.1574-0862.2012.00633.x
Frishkoff, L. O., Karp, D. S., M'Gonigle, L. K., Mendenhall, C. D., Zook, J., Kremen, C., et al. (2014). Loss of avian phylogenetic diversity in neotropical agricultural systems. Science 345, 1343–1346. doi: 10.1126/science.1254610
Gao, C., Zhu, J. G., Zhu, J. Y., Gao, X., Dou, Y. J., and Hosen, Y. (2004). Nitrogen export from an agriculture watershed in the taihu lake area, China. Environ. Geochem. Health 26, 199–207. doi: 10.1023/B:EGAH.0000039582.68882.7f
Glover, J. D., Reganold, J. P., and Cox, C. M. (2012). Agriculture: plant perennials to save Africa's soils. Nature 489, 359–61. doi: 10.1038/489359a
Gómez, J. A., Guzmán, M. G., Giráldez, J. V., and Fereres, E. (2009). The influence of cover crops and tillage on water and sediment yield, on nutrient and organic matter losses in an olive orchard on a sandy loam soil. Soil Tillage Res. 106, 137–144. doi: 10.1016/j.still.2009.04.008
Graefe, S., Dufour, D., van Zonneveld, M., Rodriguez, F., and Gonzalez, A. (2013). Peach palm (Bactris gasipaes) in tropical Latin America: implications for biodiversity conservation, natural resource management and human nutrition. Biodivers. Conserv. 22, 269–300. doi: 10.1007/s10531-012-0402-3
Guillaume, T., Kotowska, M. M., Hertel, D., Knohl, A., Krashevska, V., Murtilaksono, K., et al. (2018). Carbon costs and benefits of Indonesian rainforest conversion to plantations. Nat. Commun. 9:2388. doi: 10.1038/s41467-018-04755-y
Hawken, P. (2017). Drawdown: The Most Comprehensive Plan Ever Proposed to Reverse Global Warming. Penguin.
Heilmayr, R., Echeverría, C., and Lambin, E. F. (2020). Impacts of Chilean forest subsidies on forest cover, carbon and biodiversity. Nat. Sustain. 3, 701–709. doi: 10.1038/s41893-020-0547-0
Horak, J., Peltanova, A., Podavkova, A., Safarova, L., Bogusch, P., Romportl, D., et al. (2013). Biodiversity responses to land use in traditional fruit orchards of a rural agricultural landscape. Agric. Ecosyst. Environ. 178, 71–77. doi: 10.1016/j.agee.2013.06.020
Huai, L. G., and Lin, Y. H. (2005). Influences of sod culture on the soil water content,effect of soil nutrients,fruit yield and quality in citrus orchard. Chin. J. Eco Agric. 2. Available online at: http://en.cnki.com.cn/Article_en/CJFDTOTAL-ZGTN200502053.htm
IPBES (2019a). Global Assessment Report of the Intergovernmental Science-Policy Platform on Biodiversity and Ecosystem Services. IPBESSecretariat.
IPBES (2019b). Summary for Policymakers of the Global Assessment Report on Biodiversity and Ecosystem Services of the Intergovernmental Science-Policy Platform on Biodiversity and Ecosystem Services. The Intergovernmental Science-Policy Platform on Biodiversity and Ecosystem Services, Bonn.
IPCC (2006). 2006 IPCC Guidelines for National Greenhouse Gas Inventories. Task Force on National Greenhouse Gas Inventories.
IPCC (Ed.). (2015). “Agriculture, forestry and other land Use (AFOLU),”. in Climate Change 2014: Mitigation of Climate Change: Working Group III Contribution to the IPCC Fifth Assessment Report. Cambridge: Cambridge University Press, 811–922.
IPCC (2019a). Summary for Policy Makers: Climate Change and Land: An IPCC special Report on Climate Change, Desertification, Land Degradation, Sustainable land Management, Food Security, and Greenhouse Gas Fluxes in Terrestrial Ecosystems. Internation Panel on Climate Change
IPCC (2019b). 2019 Refinement to the 2006 IPCC Guidelines for National Greenhouse Gas Inventories. Task Force on National Greenhouse Gas Inventories.
Ishii, T., Aketa, T., Motosugi, H., and Cruz, A. F. (2008). Mycorrhizal development in a chestnut orchard introduced by a sod culture system with Vulpia myuros L. C. C. Gmel. Acta Hortic. 767, 429–434. doi: 10.17660/ActaHortic.2008.767.49
Jong, F. S. (2018). “An overview of sago industry development, 1980–2015,” in Sago Palm: Multiple Contributions to Food Security and Sustainable Livelihoods, eds H. Ehara, Y. Toyoda, and D. V. Johnson (Singapore: Springer), 75–89. doi: 10.1007/978-981-10-5269-9_6
Jose, S. (2009). Agroforestry for ecosystem services and environmental benefits: an overview. Agrofor. Syst. 76, 1–10. doi: 10.1007/s10457-009-9229-7
Karp, D. S., Chaplin-Kramer, R., Meehan, T. D., Martin, E. A., DeClerck, F., Grab, H., et al. (2018). Crop pests and predators exhibit inconsistent responses to surrounding landscape composition. Proc. Natl. Acad. Sci. U.S.A. 115:E7863–E7870. doi: 10.1073/pnas.1800042115
Kreitzman, M. (2020). Perennial Agriculture: Agronomy and Environment in Long-Lived Food Systems. University of British Columbia.
Kremen, C. (2015). Reframing the land-sparing/land-sharing debate for biodiversity conservation. Ann. N Y Acad. Sci. 1355, 52–76. doi: 10.1111/nyas.12845
Lal, R. (2003). Soil erosion and the global carbon budget. Environ. Int. 29, 437–450. doi: 10.1016/S0160-4120(02)00192-7
Landis, D. A., Wratten, S. D., and Gurr, G. M. (2000). Habitat management to conserve natural enemies of arthropod pests in agriculture. Annu. Rev. Entomol. 45, 175–201. doi: 10.1146/annurev.ento.45.1.175
Lasco, R. D. (2002). Forest carbon budgets in Southeast Asia following harvesting and land cover change. Sci. China 45:10.
Le Quéré, C., Andrew, R. M., Friedlingstein, P., Sitch, S., Hauck, J., Pongratz, J., et al. (2018). Global carbon budget 2018. Earth Syst. Sci. Data 10, 2141–2194. doi: 10.5194/essd-10-2141-2018
Ledo, A., Smith, P., Zerihun, A., Whitaker, J., Vicente-Vicente, J. L., Qin, Z., et al. (2020). Changes in soil organic carbon under perennial crops. Glob. Change Biol. 26, 4158–4168. doi: 10.1111/gcb.15120
Lovell, S. T., Dupraz, C., Gold, M., Jose, S., Revord, R., Stanek, E., et al. (2018). Temperate agroforestry research: considering multifunctional woody polycultures and the design of long-term field trials. Agrofor. Syst. 92, 1397–1415. doi: 10.1007/s10457-017-0087-4
Magrach, A., and Sanz, M. J. (2020). Environmental and social consequences of the increase in the demand for ‘superfoods' world-wide. People Nat. 2, 267–278. doi: 10.1002/pan3.10085
Mehlenbacher, S. A. (2003). Progress and prospects in nut breeding. Acta Hortic. 622, 57–79. doi: 10.17660/ActaHortic.2003.622.4
Méndez, V. E., Lok, R., and Somarriba, E. (2001). Interdisciplinary analysis of homegardens in nicaragua: micro- zonation, plant use and socioeconomic importance. Agrofor. Syst. 51, 85–96. doi: 10.1023/A:1010622430223
Merwin, I. A., Stiles, W. C., and van Es, H. M. (1994). Orchard groundcover management impacts on soil physical properties. J. Am. Soc. Hortic. Sci. 119, 216–222. doi: 10.21273/JASHS.119.2.216
Meyer, D., Becker, R., Gumbmann, M. R., Vohra, P., Neukom, H., and Saunders, R. M. (1986). Processing, composition, nutritional evaluation, and utilization of mesquite (Prosopis spp.) pods as a raw material for the food industry. J. Agric. Food Chem. 34, 914–919. doi: 10.1021/jf00071a037
Michon, G. (2011). Revisiting the resilience of chestnut forests in corsica: from social-ecological systems theory to political ecology. Ecol. Soc. 16:205. doi: 10.5751/ES-04087-160205
Molnar, T. J., Kahn, P. C., Ford, T. M., and Funk, C. J. (2013). Tree crops, a permanent agriculture: concepts from the past for a sustainable future. Resources 2, 457–488. doi: 10.3390/resources2040457
Monfreda, C., Ramankutty, N., and Foley, J. A. (2008). Farming the planet: 2. Geographic distribution of crop areas, yields, physiological types, and net primary production in the year 2000. Glob. Biogeochem. Cycles 22:GB1022. doi: 10.1029/2007GB002947
Morandin, L. A., and Kremen, C. (2013). Hedgerow restoration promotes pollinator populations and exports native bees to adjacent fields. Ecol. Appl. 23, 829–839. doi: 10.1890/12-1051.1
Mora-Urpi, J., Weber, J. C., and Clement, C. R. (1997). Peach Palm: Bactris gasipaes Kunth. Rome: IPK, IPGRI.
Muthayya, S., Rah, J. H., Sugimoto, J. D., Roos, F. F., Kraemer, K., and Black, R. E. (2013). The global hidden hunger indices and maps: an advocacy tool for action. PLoS ONE 8:e67860. doi: 10.1371/journal.pone.0067860
Orians, G. H., and Pfeiffer, E. W. (1970). Ecological effects of the war in Vietnam. Science 168:544. doi: 10.1126/science.168.3931.544
Perfecto, I., Mas, A., Dietsch, T., and Vandermeer, J. (2003). Conservation of biodiversity in coffee agroecosystems: a tri-taxa comparison in southern Mexico. Biodivers. Conserv. 12, 1239–1252. doi: 10.1023/A:1023039921916
PFAF (2020). Plant Database Caryodendron Orinocense Taccy Nut, Nuez de Barinas. Available online at: https://pfaf.org/user/Plant.aspx?LatinName=Caryodendron+orinocense (accessed April 10, 2020).
Pumariño, L., Sileshi, G. W., Gripenberg, S., Kaartinen, R., Barrios, E., Muchan, M. N., et al. (2015). Effects of agroforestry on pest, disease and weed control: a meta-analysis. Basic Appl. Ecol. 16, 573–582. doi: 10.1016/j.baae.2015.08.006
Quinlan, R. J., Quinlan, M. B., Dira, S., Caudell, M., Sooge, A., Assoma, A. A., et al. (2015). Vulnerability and resilience of sidama enset and maize farms in southwestern Ethiopia. J. Ethnobiol. 35, 314–36. doi: 10.2993/etbi-35-02-314-336.1
Ray, D. K., Ramankutty, N., Mueller, N. D., West, P. C., and Foley, J. A. (2012). Recent patterns of crop yield growth and stagnation. Nat. Commun. 3:1293. doi: 10.1038/ncomms2296
Robertson, G. P., and Swinton, S. M. (2005). Reconciling agricultural productivity and environmental integrity: a grand challenge for agriculture. Front. Ecol. Environ. 3, 38–46. doi: 10.1890/1540-9295(2005).0030038:RAPAEI2.0.CO
Rode, J., Gómez-Baggethun, E., and Krause, T. (2015). Motivation crowding by economic incentives in conservation policy: a review of the empirical evidence. Ecol. Econ. 117, 270–282. doi: 10.1016/j.ecolecon.2014.11.019
Rogé, P., Snapp, S., Kakwera, M. N., Mungai, L., Jambo, I., Peter, B., et al. (2016). Ratooning and perennial staple crops in Malawi. A review. Agron. Sustain. Dev. 36:50. doi: 10.1007/s13593-016-0384-8
Rosa, H., Kandel, S., and Dimas, L. (2004). Compensation for environmental services and rural communities: lessons from the Americas. Int. For. Rev. 6, 187–94. doi: 10.1505/ifor.6.2.187.38401
Scales, B. R., and Marsden, S. J. (2008). Biodiversity in small-scale tropical agroforests: a review of species richness and abundance shifts and the factors influencing them. Environ. Conserv. 35, 160–72. doi: 10.1017/S0376892908004840
Schier, H. E., Eliot, K. A., Herron, S. A., Landfried, L. K., Migicovsky, Z., Rubin, M. J., et al. (2019). Comparative analysis of perennial and annual phaseolus seed nutrient concentrations. Sustainability 11:2787. doi: 10.3390/su11102787
SIAP (2020). Agri-Food and Fisheries Information Service. Available online at: https://www.gob.mx/siap/ (accessed April 11, 2020).
Simon, S., Bouvier, J.-C., Debras, J.-F., and Sauphanor, B. (2010). Biodiversity and pest management in orchard systems. A review. Agron. Sustain. Dev. 30, 139–152. doi: 10.1051/agro/2009013
Siti, H. N., Kamisah, Y., and Kamsiah, J. (2015). The role of oxidative stress, antioxidants and vascular inflammation in cardiovascular disease. A review. Vascul. Pharmacol. 71, 40–56. doi: 10.1016/j.vph.2015.03.005
Smith, L. C., and Haddad, L. (2015). Reducing child undernutrition: past drivers and priorities for the post-MDG era. World Dev. 68, 180–204. doi: 10.1016/j.worlddev.2014.11.014
Suter, P. M. (2005). “Carbohydrates dietary fiber,” in Atherosclerosis: Diet and Drugs, eds A. von Eckardstein (Berlin; Heidelberg: Springer), 231–261.
Taylor, J. R. (1997). An Introduction to Error Analysis: The Study of Uncertainties in Physical Measurements. Sausalito, CA: University Science Books.
Toensmeier, E. (2016). The Carbon Farming Solution: A Global Toolkit of Perennial Crops and Regenerative Agriculture Practices for Climate Change Mitigation and Food Security. White River Junction, VT: Chelsea Green Publishing Company.
Toensmeier, E. (2017). “Perennial staple crops agroforestry for climate change mitigation,” in Integrating Landscapes: Agroforestry for Biodiversity Conservation and Food Sovereignty, eds F. Montagnini (Cham: Springer International Publishing), 439–451.
Toensmeier, E., Ferguson, R., and Mehra, M. (2020). Perennial vegetables: a neglected resource for biodiversity, carbon sequestration, and nutrition. PLoS ONE 15:e0234611. doi: 10.1371/journal.pone.0234611
Torralba, M., Fagerholm, N., Burgess, P. J., Moreno, G., and Plieninger, T. (2016). Do European agroforestry systems enhance biodiversity and ecosystem services? A meta-analysis. Agric. Ecosyst. Environ. 230, 150–161. doi: 10.1016/j.agee.2016.06.002
Tsegaye, A., and Struik, P. C. (2002). Analysis of enset (Ensete ventricosum) indigenous production methods and farm-based biodiversity in major enset-growing regions of southern Ethiopia. Exp. Agric. 38, 291–315. doi: 10.1017/S0014479702003046
USDA and USHHS (2011). U.S. Department of Agriculture and U.S. Department of Health and Human Services, Dietary Guidelines for Americans, 2010, 7th Edn. USDA, US Government Printing Office.
Uylaşer, V., and Yildiz, G. (2014). The historical development and nutritional importance of olive and olive oil constituted an important part of the mediterranean diet. Crit. Rev. Food Sci. Nutr. 54, 1092–1101. doi: 10.1080/10408398.2011.626874
van Noordwijk, M., Coe, R., and Sinclair, F. (2016). Central Hypotheses for the Third Agroforestry Paradigm Within a Common Definition. World Agroforestry Centre (ICRAF).
Vico, G., and Brunsell, N. A. (2018). Tradeoffs between water requirements and yield stability in annual vs. perennial crops. Adv. Water Resour. 112, 189–202. doi: 10.1016/j.advwatres.2017.12.014
Waldman, K. B., Ortega, D. L., Richardson, R. B., and Snapp, S. S. (2017). Estimating demand for perennial pigeon pea in Malawi using choice experiments. Ecol. Econ. 131, 222–30. doi: 10.1016/j.ecolecon.2016.09.006
Wilcove, D. S., and Koh, L. P. (2010). Addressing the threats to biodiversity from oil-palm agriculture. Biodivers. Conserv. 19, 999–1007. doi: 10.1007/s10531-009-9760-x
Wilcove, D. S., and Lee, J. (2004). Using economic and regulatory incentives to restore endangered species: lessons learned from three new programs. Conserv. Biol. 18, 639–45. doi: 10.1111/j.1523-1739.2004.00250.x
Wilson, T. S., Sleeter, B. M., and Cameron, D. R. (2016). Future land-use related water demand in California. Environ. Res. Lett. 11:054018. doi: 10.1088/1748-9326/11/5/054018
Withers, P. J. A., Neal, C., Jarvie, H. P., and Doody, D. G. (2014). Agriculture and eutrophication: where do we go from here? Sustainability 6, 5853–5875. doi: 10.3390/su6095853
Wolz, K. J., Lovell, S. T., Branham, B. E., Eddy, W. C., Keeley, K., Revord, R. S., et al. (2017). Frontiers in alley cropping: transformative solutions for temperate agriculture. Glob. Change Biol. 24, 883–894. doi: 10.1111/gcb.13986
Xi-rong, G. A. O. (2005). Effects of sod culture on nutrient content of soil and apple tree in orchard. Acta Horticult. 1058, 175–180. doi: 10.17660/ActaHortic.2014.1058.19
Young, R. R., Wilson, B., Harden, S., and Bernardi, A. (2009). Accumulation of soil carbon under zero tillage cropping and perennial vegetation on the liverpool plains, eastern Australia. Soil Res. 47, 273–285. doi: 10.1071/SR08104
Yusuf, M., Teklehaimanot, Z., and Gurmu, D. (2013). The decline of the vulnerable Yeheb Cordeauxia edulis, an economically important dryland shrub of Ethiopia. Oryx 47, 54–58. doi: 10.1017/S0030605311000664
Zastrow, M. (2019). China's tree-planting drive could falter in a warming world. Nature 573, 474–475. doi: 10.1038/d41586-019-02789-w
Zohary, D. (2002). Domestication of the carob (Ceratonia siliqua L.). Isr. J. Plant Sci. 50, 141–145. doi: 10.1560/BW6B-4M9P-U2UA-C6NN
Zohary, D., Weiss, E., and Hopf, M. (2012). “Chapter 6: Fruit trees and nuts,” in Domestication of Plants in the Old World: The Origin and Spread of Domesticated Plants in Southwest Asia, Europe, and the Mediterranean Basin (Oxford, UK: Oxford University Press). Available online at: https://www.oxfordscholarship.com/view/10.1093/acprof:osobl/9780199549061.001.0001/acprof-9780199549061-chapter-6.
Zomer, R. J., Trabucco, A., Coe, R., and Place, F. (2009). Trees on farm: analysis of global extent and geographical patterns of agroforestry. ICRAF Work Paper (World Agroforestry Centre).
Keywords: perennial staple crops, perennial crops, agroforestry, yield, nutrition, carbon sequestration, tree crops, food production
Citation: Kreitzman M, Toensmeier E, Chan KMA, Smukler S and Ramankutty N (2020) Perennial Staple Crops: Yields, Distribution, and Nutrition in the Global Food System. Front. Sustain. Food Syst. 4:588988. doi: 10.3389/fsufs.2020.588988
Received: 30 July 2020; Accepted: 13 October 2020;
Published: 04 December 2020.
Edited by:
Ana Isabel Moreno-Calles, Universidad Nacional Autónoma de México, MexicoReviewed by:
Liming Ye, Ghent University, BelgiumSuprasanna Penna, Bhabha Atomic Research Centre (BARC), India
Copyright © 2020 Kreitzman, Toensmeier, Chan, Smukler and Ramankutty. This is an open-access article distributed under the terms of the Creative Commons Attribution License (CC BY). The use, distribution or reproduction in other forums is permitted, provided the original author(s) and the copyright owner(s) are credited and that the original publication in this journal is cited, in accordance with accepted academic practice. No use, distribution or reproduction is permitted which does not comply with these terms.
*Correspondence: Maayan Kreitzman, bWtyZWl0em1hbkBpcmVzLnViYy5jYQ==