- 1Department of Food Science and Technology, Bowen University, Iwo, Nigeria
- 2Food and Nutrition Sciences Laboratory, International Institute of Tropical Agriculture (IITA), Southern Africa Hub, Lusaka, Zambia
- 3Food and Nutrition Sciences Laboratory, International Institute of Tropical Agriculture (IITA), Ibadan, Nigeria
- 4Maize Breeding Unit, International Institute of Tropical Agriculture (IITA), Ibadan, Nigeria
Kokoro from provitamin A (PVA) maize genotypes, produced through conventional breeding, was studied to improve the indigenous white maize-based snack deficient in provitamin A carotenoid commonly consumed in South-western Nigeria. The chemical composition, carotenoid retention, and acceptability of Kokoro from three PVA maize genotypes and one landrace yellow maize variety (control) were estimated. Chemical composition showed significant differences (p < 0.05) in parameters with high crude fat content (23.21–32.11%). The sensory evaluation result revealed that Kokoro from DT STR SYN2-Y (control) was the most acceptable, while among the PVA Kokoro, PVA SYN HGBC1 was acceptable. The pre-processing for the estimated carotenoids (μg g−1); lutein, zeaxanthin, total β-carotene, and PVA in maize genotypes ranged from 10.38 to 12.87, 6.03 to 10.97, 3.83 to 6.18, and 5.96 to 8.43, while after processing to Kokoro, total β-carotene ranged from 1.47 to 3.10 μg g−1 and total PVA content 2.43–4.00 μg g−1. The carotenoid retention in Kokoro from PVA maize genotypes ranged from zeaxanthin 5.89–8.39%; lutein 2.74–4.45%; total β-carotene 38.24–66.14%, and total PVA 37.98–67.05%. Degradation of carotenoid was observed due to the unit operations in the processing method that led to the exposure of the food matrix to direct sunlight, heat, light, metals, and oxygen resulting in the formation of cis-isomers and loss of provitamin A quantity. The maize genotype PVASYNHGBC0 had the highest PVA value and carotenoid retention after processing. The study observed that PVA retention of Kokoro was genotype-dependent, and genotype PVASYNHGBC0 (Provitamin A maize HGA cycle zero) retained the highest carotenoid content. Also, PVASYNHGBC0 (for all the servings' size; 100 and 150 g) in all age groups had the highest percentage contribution of vitamin A to the recommended daily allowance. However, further improvement in the carotenoid content of maize genotypes is needed to enable the production of nutritious Kokoro with higher vitamin A percentage contribution and retinol equivalent.
Introduction
Snacks are food substances consumed between major meals. Indigenous snacks consumed in Nigeria are numerous and include puff puff, kuli-kuli, robo, akara bite, and Kokoro. Kokoro is one of the famous traditional snacks consumed in South-western Nigeria comprising of six states; Lagos, Oyo, Ogun, Osun, Ondo, and Ekiti (Otunola et al., 2012; Fasasi and Alokun, 2013). The consumption is mostly by Nigerians from these geographical locations. It is a maize-based product from 100% whole white maize that undergoes a 3-day intensive traditional process with several unit operations such as drying, cleaning, boiling, fermentation, milling, mixing, dough formation, kneading, rolling and cutting, deep frying, draining, second deep frying for development of color and aroma, cooling, and packaging (Fasasi and Alokun, 2013; Adegunwa et al., 2015; Oranusi and Dahunsi, 2015). Kokoro is consumed most, especially by school-aged children in South-western states in Nigeria, because it is inexpensive in comparison with other snacks (Adelakun et al., 2005; Fasasi and Alokun, 2013; Idowu, 2015). The Kokoro snack is made into ring form with a maximum of four rings in a package, and the typical consumption window is 1–2 weeks because it is a fast-selling item and the local producers make the product every day. The shelf life of Kokoro from different producers varies; this is mostly dependent on hygiene, production procedures, quality of vegetable oil, packaging material, and storage. The production of Kokoro in Nigeria takes place majorly in three villages: Imashayi, Joga, and Iboro, all in Yewa North Local Government Area, Ogun State, Nigeria (Oranusi and Dahunsi, 2015).
In sub-Saharan Africa (SSA) and South Asia, about one-third of children were reported to be vitamin A deficient, which is attributed to more than 95% of deaths with high child mortality (Stevens et al., 2015). There is a dearth of recent information regarding the prevalence of Vitamin A deficiency (VAD) in Nigeria. However, Abolurin et al. (2018) reported the prevalence of VAD of 5.6% among under-five children in Ilesa, Osun State, Nigeria. They determined the Serum retinol levels and VAD was defined as serum retinol level < 20.0 μg/dL (0.70 μmol/l) while severe VAD was defined as a value < 10.0 μg/dL (0.35 μmol/L). Akinyinka et al. (2001) reported the prevalence of VAD in Ibadan, South-West Nigeria to be 31.6%, they reported that the proportional morbidity rates of VAD defined by serum retinol concentrations (7.3%) and CIC-T (6.2%) was similar (p > 0.05) and children aged < 3 years accounted for 70% of VAD cases. Oso et al. (2003) reported VAD for preschool children in the Ijaye Orile community in Lagos State, Nigeria. 26.8% children were deficient in serum retinol levels (< 10 μg/dL) while 47.9% had low levels (10–19 μg/dL). Ajaiyeoba (2001) reported the national prevalence of xerophthalmia was 1.1% whilst the national prevalence of VAD using serum retinol < 20 μg/dL (or 0.7 μmol/L) as cut-off was 28.1%. Maziya-Dixon et al. (2006) reported that 29.5% of children < 5 years old were vitamin A deficient (serum retinol < 0.70 μmol/L). Due to the prevalence of vitamin A deficiency in Africa, especially in Nigeria, biofortification of staple crops with increased micronutrient was introduced by the HarvestPlus Challenge Program to reduce adverse consequences affecting mostly women and children with micronutrient malnutrition (Srivastava, 2016). The program ensures that the quantity of micronutrients (iron, zinc, and provitamin A carotenoids) in staple food crops has no adverse effect on agronomic qualities, such as high yield, resistance to diseases, and environmental stress (De Moura et al., 2015).
Maize is an indispensable food crop consumed by Africans in various processed forms, such as Kokoro (Abdulrahaman and Kolawole, 2006; Nuss and Tanumihardjo, 2010; Idowu, 2015). However, the production of Kokoro is solely from white maize, which is reported to be deficient in some nutrients such as carotenoids. Kokoro from white maize has been a concern for authors to improve its nutritional quality through fortification and supplementation with legumes such as groundnut, soybean, lima bean, African yam bean to improve its nutritional quality. Ayinde et al. (2011) used beniseed cake, Abegunde et al. (2014) used cowpea, Oluwafemi et al. (2018) and Dauda et al. (2020) used groundnut. However, information on the use of orange maize for Kokoro production is scanty. Orange maize is a biofortified crop which has been developed recently by the Maize breeders and is a source of provitamin A carotenoids that include beta-cryptoxanthin, alpha, and beta-carotene, also known as precursors of vitamin A essential to prevent diet-related chronic disease in the human body (Pixley et al., 2013). Quantification of the carotenoids (PVA and non-PVA) after processing of biofortified crops is vital to determine the effectiveness of biofortification. Many authors (Alamu et al., 2014; Awoyale et al., 2016; Eyinla et al., 2019) have reported the retention of the provitamin A after processing in foods. The acceptability of foods from provitamin A biofortified crop (such as cassava and orange-fleshed sweet potatoes) has been reported (Udensi et al., 2011; Ukpabi et al., 2012; Amajor et al., 2014; Etuk and Umoh, 2014; Ayinde and Adewunmi, 2016; IIona et al., 2017; Ukom et al., 2019). However, information on the acceptability of Maize Kokoro from provitamin A maize needs to be generated. More nutritious, affordable and acceptable snacks such as maize Kokoro from biofortified maize will be of great advantage to health.
Recent efforts on breeding for provitamin A maize genotypes by maize breeders to curb vitamin A deficiency has called for an urgent research study to evaluate these maize lines for product development such as the traditional Kokoro snack. Also, to establish the retention of carotenoid, chemical composition, and consumer acceptability of the products. The production of Kokoro snacks rich in carotenoids could be a better alternative and replacement of Kokoro from white maize. Hence, this study aimed at investigating the chemical composition, carotenoid retention, and acceptability of Kokoro made from the three PVA maize genotypes using the traditional method of production. The information generated from this study will help to determine the possibility of integrating and promoting the PVA maize as a suitable raw material for the production of maize Kokoro in Nigeria.
Materials and Methods
Materials
The Six maize genotypes (planted in 3 replications and two seasons) were obtained from the research farms of the Maize Improvement Program at the International Institute of Tropical Agriculture (IITA), Ibadan, Nigeria. The genotypes were obtained through conventional breeding from different planting cycles and parent lineages and named as follows: PVA SYN HGBC0 (provitamin A maize HGB cycle zero); PVA SYN HGBC1 (provitamin A maize HGB cycle one); PVA SYN HGAC0 (provitamin A maize HGA cycle zero), and DT STR SYN2-Y (yellow maize variety, used as control 1). The three PVA maize genotypes used for Kokoro production were selected from the group of six genotypes based on different levels of provitamin A content (high and low) in the maize flours. The three PVA maize genotypes (PVA SYN HGBC0, PVA SYN HGBC1, PVA SYN HGAC0) were labeled Maize 1, Maize 2, Maize 3, respectively, and Maize 4 was DT STR SYN2-Y, a yellow landrace maize variety as control. Maize 1 to Maize 3 were used to produced Kokoro 1, Kokoro 2, and Kokoro 3, respectively, while Kokoro 4 is from the landrace yellow maize variety used as control sample 1. Commercial Kokoro (“Kokoro Egba”) produced from white maize was used as a control sample 2. The commercial Kokoro was purchased at Imashayi, Yewa North Local Government Area in Abeokuta, Ogun State, Nigeria, with coordinates 7°09′N 2°55′E bordering the Republic of Benin. It is a community known for its age-long tradition of processing Kokoro commercially.
Methods
Product Preparation
Maize Flour
One kilogram of maize grains were sorted, cleaned, and 100 g of each kernel were milled individually to 0.5 mm particle size using a laboratory mill (3100 Perten Instrument). The milled samples were left to cool in a dark room, packaged in an airtight high-density polyethylene whirl pack after which it was put in a white envelope to prevent exposure to light and kept in a −80°C freezer before laboratory analysis. All analysis was done within 48 h of sample preparation to prevent degradation of carotenoids by light and oxygen.
Kokoro Production
Figure 1 shows the traditional processing method for Kokoro production. The researcher went on a 3-day research trip to the processing village to understudy and learn the traditional processing method. About 2 kg of cleaned maize samples were boiled for 1 h, with immediate dewatering of grains followed by steeping in clean tap water overnight to ferment. The fermented grains were sieved out from the steeped water with the aid of a plastic sieve and left for about 5 min to ensure proper drainage of the water. The grains were wet milled using a locally fabricated attrition mill. A little quantity of water was added during this process to ensure the formation of thick dough-like consistency. Two tablespoons of pure iodized salt with 2 cups of wet-milled onions (Allium cepa) were added and mixed with the thick dough-like consistency to enhance the taste. The thick dough-like consistency was then cut into small portions, kneaded, and molded by hand on a flat, wooden tray to produce thin rings of Kokoro. The thin rings of Kokoro were pre-fried in high temperatures of vegetable oil for 3 min to form a semi-finished yellow-colored product, which is unsuitable for consumption because it lacks the crisp nature. The semi-finished product was sieved to drain the oil and kept in a covered wooden weaved basket overnight before a final and second round of frying was done at a very high temperature of 170°C the following day for 1–2 min to enhance crispness. Figure 2 shows the color of the final product, which was a light to deep yellow. Sub-sampling of about 1 kg each of the Kokoro samples was done from the whole lot. Some were taken to be blended for analysis using a USHA Stainless steel mixer grinder (Model-MG 2053N, USHA International) and put in an airtight, high-density polyethylene whirl pack, and kept in the dark envelope. Then, it was put in a −80°C freezer before the laboratory analysis. All analysis was done within 48 h of sample preparation to prevent degradation of carotenoids by light and oxygen, and each sample was analyzed in triplicate. Figure 2 shows Kokoro from three provitamin A maize genotype, yellow maize (DT STR SYN2-Y), and commercially purchased Kokoro.
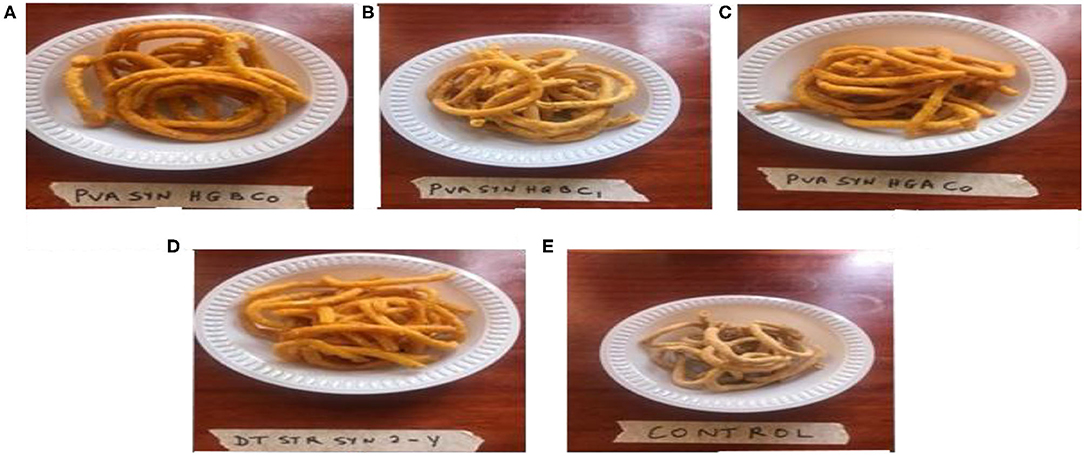
Figure 2. Kokoro from three provitamin A maize genotype; (A) PVA SYN HGBC0 (B) PVA SYN HGBC1 (C) PVA SYN HGAC0 (D) DT STR SYN2-Y (Control 1) (E) Commercially purchased Kokoro (Control 2).
Proximate Analyses
Proximate composition analyses for moisture, ash, crude protein, crude fat, and crude fiber were carried out on the samples by the methods described by the Association of Official Analytical Chemists (AACC, 2005; AOAC, 2012). Carbohydrate content was determined by difference. All analyses were done in triplicate.
Determination of Carotenoid Using HPLC
The method of Howe and Tanumihardjo (2006) was adapted for carotenoid analysis, and the quantity of β-cryptoxanthin, α-carotene and trans with cis-isomers of β-carotene were extracted as described by Alamu et al. (2014). Carotenoid extraction was done by weighing 0.6 g of the maize flour/Kokoro in a screw cap tube, 10 mL of ethanol, which contains 0.1% butylated hydroxyl toluene (BHT) was added and vortex thoroughly for 1 min. The tubes were placed in a water bath for 5 min at 85°C to achieve ethanol precipitation. After this, the tubes were taken out of the water bath, and 0.5 mL of 80% Potassium hydroxide (KOH) was added to the mixture for the saponification of any interfering oil. The samples were mixed using a vortex mixer for 30 s, after which the samples were placed in a water bath (85°C) for 5 min. The mixture was vortex again before returning to the water bath for another 5 min. The tubes were removed from the water bath and immersed immediately in an ice bath followed by the addition of 3 mL of cold deionized water. Separation of carotenoids was done three times with the addition of 3 ml of hexane using a vortex mixer for 10 s and then centrifuged for 10 s at 1,000 rpm using SORVALL GLC1 centrifuge (model 06470, USA). The mixture containing combined hexane fractions were washed with deionized water thrice, vortex, and centrifuged for 10 s at 1,000 rpm. The upper phase was pipette into a 15 mL concentrator tube and with the aid of the TurboVap LIV concentrator under nitrogen gas, the mixture containing hexane fractions was dried down. Reconstitution of the extract was done using methanol/dichloromethane (1 mL, 50:50 v/v), after which 100 μL aliquot was injected into the HPLC system for analyses of a-carotene, b-carotene (cis and trans isomers), and b-cryptoxanthin. Quantification of carotenoids was carried out by the use of Waters HPLC system (Water Corporation, Milford, MA), which consist of a guard column, C30 YMC Carotenoid column (4.6 9 250 mm, 3 lm), Waters 626 binary HPLC pump, 717 auto-sampler and a 2,996 photodiode array detector (PDA) with operating system Empower 1 software (Waters Corporation).
The constituent of solvent A includes; methanol: water (92:8 v/v) with 10 mmol/L ammonium acetate while solvent B includes; 100% methyl tertiary-butyl ether. The performance of gradient elution took place at 1 mL/min with other conditions such as; 29 min linear gradient from 83 to 59% A, 6 min linear gradient from 59 to 30% A, 1 min hold at 30% A, 4 min linear gradient from 30 to 83% A and a 4 min hold 83%. β-carotene eluted at 25 min. Generation of chromatograms was achieved at 450 nm, and identification of a-carotene, β-carotene (cis and trans isomers), and β-cryptoxanthin were determined using standard and external method based on the calibration curve from pure standards and verification of absorption spectrum and co-elution with available authentic standards. α-carotene, β-carotene, and β-cryptoxanthin standards were purchased from CaroteNature, GmbH (Lupsingen, Switzerland). The solvents used were HPLC grade.
Provitamin A (PVA) was obtained by the summation of the amount of β-carotene (13-cis-β-carotene, 9-cis-β-carotene, and trans isomers) to half of the amounts of a-carotene and β-cryptoxanthin (Alamu et al., 2014). α-carotene and β-cryptoxanthin have 50% of the provitamin A activity of β-carotene because when these compounds are centrally cleaved, there is a production of one molecule of retinal while β-carotene can produce two.
Apparent Retention of Carotenoids
The percent apparent retention (AR) of carotenoids was determined by dividing the carotenoid content (μg g−1) of the processed product (Kokoro) by the carotenoid content (μg/g) of unprocessed food (maize flour) (Bechoff et al., 2017) according to the formula:
Vitamin A Percentage Contribution of Kokoro
The calculation for percentage contribution of vitamin A in Kokoro was done by using the provitamin A carotenoids values for each maize genotype through extrapolation. The Kokoro snack is usually packaged with an average weight of 100 or 150 g. In this study, we assumed these packaging sizes to be serving sizes. The serving size of Kokoro 100 g for boys and girls age 9–18 years and adult (female and male; >19) was used. Also, 150 g for adult (female and male; >19) per serving of Kokoro was estimated. The retinol equivalent was calculated using ratio 12:1, which is 12 μg of Provitamin A (PVA) is equal to 1 μg of Retinol Equivalent [Institute of Medicine (IOM), 2001].
Sensory Evaluation
The sensory evaluation was carried out on the Kokoro samples, and the commercially purchased Kokoro (control 2) for color, taste, crispness, and aroma sensory attributes. The overall acceptability of all samples was also rated in comparison with the control samples. The preference test based on the degree of likeness was used. In the test, 20 untrained panelists comprising male and female staff and students from Bowen University, Iwo, Osun State were engaged. These panelists were familiar with the quality attributes of Kokoro. The sensory evaluation questionnaires were based on a nine-point hedonic scale where 1 represents “dislike extremely,” and 9 represents “like extremely” for all the quality attributes employed (Iwe, 2002). The samples for evaluation were all placed at the same time in each of the well-illuminated booths, and the participants were seated into separate booth. The sitting of each panelist in a separate booth was to prevent bias decisions and produce an individual judgment. One ring each of three Kokoro samples (provitamin A maize), landrace yellow maize and commercially purchased Kokoro was presented on the white plastic plates and identified with a three-digit code. The samples were in triplicate in each panelist booth all at the same time making it a total of 15 samples per panelist with a serviette for wiping hands and a glass of clean water for rinsing the mouth in between the evaluations to prevent a carry-over effect.
Statistical Analysis
All the data were statistically analyzed using SPSS 20. The analysis of variance (ANOVA) was used to establish a significant difference at p < 0.05. The means were separated using Duncan's multiple range test (DMRT). Tables 1, 3, 5 estimated four maize genotypes in two seasons with two replications in field and in three replications in the laboratory. Tables 2, 4 estimated four maize genotypes in two seasons with two replications in field, one commercial maize snack and three replications in the laboratory. Table 5 sensory evaluation had four maize genotypes, three replications in the sensory test with 20 panelist.
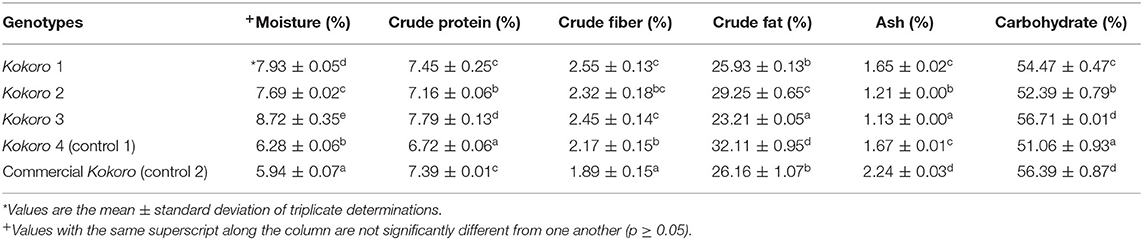
Table 2. Chemical composition of Kokoro from provitamin A maize genotypes, yellow maize and commercially purchased Kokoro.

Table 3. Carotenoid (μg g−1) profile and retinol activity equivalent of maize flour from provitamin A maize genotypes and yellow maize (dry weight basis).
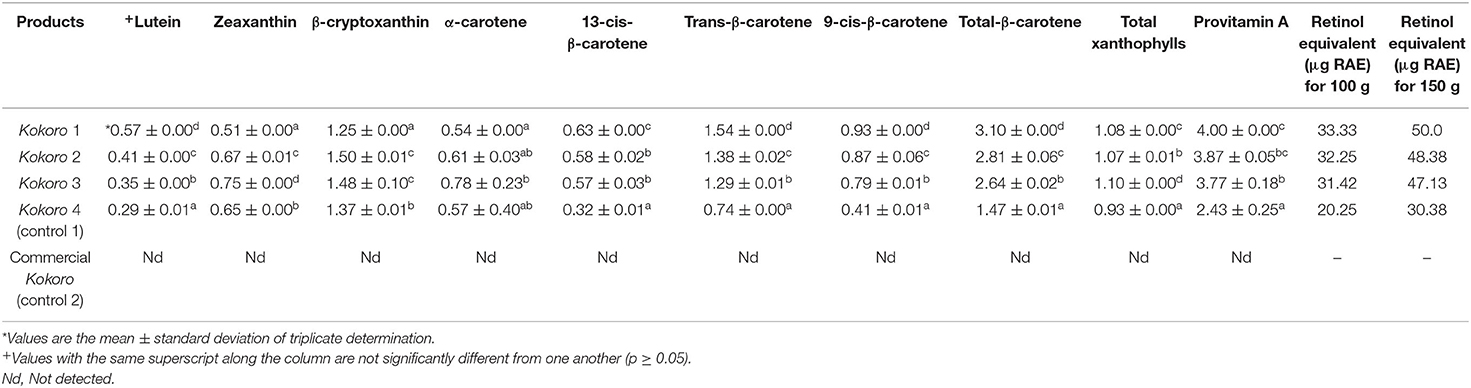
Table 4. Carotenoid (μg g−1) profile and retinol activity equivalent of serving sizes of kokoro from provitamin A maize genotypes and commercially purchased kokoro.

Table 5. Carotenoid apparent retention profile of Kokoro from provitamin A maize genotypes and yellow maize.
Results and Discussion
The chemical composition before (maize flour) and after processing (Kokoro) is presented in Tables 1, 2. The maize flour result ranged from 10.62 to 11.57% for moisture content, crude protein 6.35–7.54%, crude fiber 1.94–2.49%, crude fat 3.57–6.13%, ash 1.11–1.45%, and carbohydrate 71.17–75.07%, while Kokoro result; moisture content 5.94–8.72%, crude protein 6.72–7.79%, crude fiber 1.89–2.55%, crude fat 23.21–32.11%, ash 1.13–2.24%, and carbohydrate 51.06–56.71%. There were significant differences in all the parameters at a p-value of 0.05 for Tables 1, 2.
Moisture content in Maize 4 had the highest value (11.57%), and Maize 3 was the lowest (10.62%), while Maize 1 contained the highest value (11.49%) amongst the PVA genotypes. Previous authors reported comparable results with ranges of 9.95–11.35 and 6.09–11.57%, respectively (Ndukwe et al., 2015; Sagbo et al., 2017). The result observed did not exceed 14% because flour above 14% will breed organisms due to the high moisture content causing rapid deterioration (Iwe et al., 2016).
The crude protein content for Maize 1, Maize 2, and Maize 3; 7.27, 7.20, 7.45%, respectively, are not significantly different from each other while Maize 4 (control 1) had the lowest content (6.35%). The significant difference could be attributed to varietal properties and agronomic practices such as the type and quantity of fertilizer applied. The crude fiber in Maize 1 (2.49%) was observed to be the highest content, while the lowest content was in Maize 4 (1.16%). However, Maize 2, 3, and 4 are not significantly different from each other (Table 1). Maize 1 has the highest and could be considered high fiber maize. Ndukwe et al. (2015) and Shaista et al. (2017) reported comparable results. Crude fiber is reported to slow down the release of glucose into the blood and decrease intracolonic pressure, thereby reducing the risk of colon cancer (Gibney, 1989).
Maize 1 gave the significantly highest crude fat content (6.13%), and Maize 2 and Maize 3 contains the lowest amount of crude fat (3.70; 3.57%), respectively, presented in Table 1. Maize 2 and Maize 3 flour samples are from different parent line and cycle while Maize 1 and Maize 2 are from the same parent line, but different planting cycle resulting in different crude fat content. This could be due to genes controlling this trait, gene selection, soil type, and farming practices
The ash content in the maize flour samples ranged from 1.12 to 1.45%, with Maize 1 having high content (1.45%) and Maize 3 the lowest content (1.12%). The values observed could be attributed to environmental conditions and soil composition. Ash content of a food is reported to give an idea of the mineral elements present, and it indicates the composition of inorganic constituents after organic materials such as fats, proteins, and carbohydrates, as well as moisture, have been removed by incineration (Iwe et al., 2016).
Maize 3 (75.07%) had the highest carbohydrate content, while the lowest content was observed in Maize 1 (71.17%). The result obtained could be attributed to the composition of other nutrients and genetic properties. It is comparable with those from Sagbo et al. (2017) and Shaista et al. (2017). Carbohydrate content of flour samples indicated the quantity of energy that might be gotten from food products made from them because carbohydrate serves as a good source of energy (Iwe et al., 2016).
The chemical properties of Kokoro are presented in Table 2. Moisture is an essential factor that can affect the quality of Kokoro. The lower the moisture content, the lower the water activity for organisms to survive. The moisture content of different maize cultivars varied from 5.94 to 8.72%, presented in Table 2. The significantly highest amount was found in Kokoro 3 (8.72%) compared to Kokoro 4 (control 1) (6.28%). Significantly, the lowest amount of moisture was found in the commercially purchased Kokoro (5.94%). The result presented here can be compared similarly with earlier Kokoro studies (Ayinde et al., 2011; Oranusi and Dahunsi, 2015; Akoja et al., 2016; Adebowale and Komolafe, 2018). Although the PVA Kokoro results are not as low as the commercial Kokoro (control 2), this will pose a minimal adverse effect on the quality attribute during storage.
The crude protein in the results was significantly different (p < 0.05) in each sample. This could be attributed to the effect of various processing unit operations on genetic composition resulting in loss of proteins by denaturation. Previous authors reported a similar result (Oranusi and Dahunsi, 2015), but a lower result was reported by Ayinde et al. (2011). However, Fasasi and Alokun (2013) and Akoja et al. (2016) reported higher values (8.32 and 9.64%, respectively).
The crude fat content of Kokoro ranged from 25.93 to 32.11%. This result agrees with Oranusi and Dahunsi (2015) and contrary to the report by some authors (Ayinde et al., 2011; Fasasi and Alokun, 2013; Idowu, 2015) who reported a crude fat content of 14.70% for the sample made from 100% white maize. The rate of oil absorption during the displacement of water when frying (pre-frying and post-frying) during processing might have resulted in the values obtained. Also, the result observed is relatively high, which implies a high susceptibility to rancidity resulting in an off-flavor and a shorter shelf life.
The crude fiber content for Kokoro 1, Kokoro 2, and Kokoro 3 are not significantly different from each other in Table 2. Ayinde et al. (2011) reported a lower value of 1.45%, while Oranusi and Dahunsi (2015) had a similar result obtained in this study. Crude fiber aids in digestion and bowel movement and all Kokoro study samples have the potential of conferring crude fiber properties. Commercially purchased Kokoro (control 2) was observed to have a lower value.
Commercially purchased Kokoro contained more ash content (2.24%), and the lowest content was observed in Kokoro 3 (1.13%). The result observed is comparable to these authors (Fasasi and Alokun, 2013; Idowu, 2015; Oranusi and Dahunsi, 2015; Akoja et al., 2016), who obtained 1.88%; 2.21, 1.97–2.31, and 1.83%, respectively. The commercial sample had higher ash content than the PVA Kokoro samples. However, the higher ash content of the commercial sample could be due to contamination during the wet milling process from the wearing out of metals of the milling machine and minerals in the water. Also, the mineral composition in the soil could contribute to the high ash content of all the samples.
The total carbohydrate value of the Kokoro samples ranged from 51.06 to 56.71%, and they are significantly (p < 0.05) different from one another. Kokoro 3 recorded the highest carbohydrate value (56.71%,) while Kokoro 4 (control 1) had the lowest (51.06%). Fasasi and Alokun (2013) and Oranusi and Dahunsi (2015) reported comparable results in the range of 57.13 and 49.21–61.96%, respectively. Total carbohydrate is an indication of organic matter present in the samples and is directly proportional to the dry matter content. The difference in total carbohydrate content among the Kokoro samples can be attributed to the composition of other nutrients and the effect of processing on the maize samples.
Carotenoid Profile of Maize Flour and Kokoro Samples
The carotenoid profile result before (maize flour) and after the production of Kokoro with the retinol equivalent (μg RE) from provitamin A maize genotypes, landrace yellow maize, and commercially purchased Kokoro are presented in Tables 3, 4. The carotenoids in PVA maize studied consist of the non-provitamin A (nPVA) and provitamin A (PVA) carotenoids. The nPVA carotenoids are lutein, zeaxanthin, and total xanthophylls, while the PVA carotenoids are made up of β-cryptoxanthin, α-carotene, and β-carotene (Alamu et al., 2014, 2015; Pillay et al., 2014; Awoyale et al., 2016). The result of variance (ANOVA) in Table 3 shows a significant difference in the carotenoid profile of maize flour.
Table 4 shows significant differences at p < 0.05 and can be attributed to carotenoid isomerization or degradation during Kokoro production caused by the various unit operations (boiling, steeping, wet milling, pre-frying, cooling, post-frying, and final cooling). The unit operations in the processing method led to the exposure of the food matrix to direct sunlight, heat, light, metals, and oxygen that resulted in the formation of cis-isomers, loss of provitamin A quantity (Aman et al., 2005; Ilg et al., 2009; Rodriguez-Amaya et al., 2011; De Moura et al., 2015). Oritz et al. (2018) stated that changes during dry milling of kernels might be related to thermal sensitive triggering isomerization/degradation reaction in carotenoid. Pillay et al. (2014) reported an increased concentration of available carotenoid retention in mealie meal, which is contrary to the result observed in this study. Awoyale et al. (2016) reported the production of ogi and its provitamin A retention. The retention results presented in the study are compared similarly before storage. In comparison with data on boiled maize presented by Alamu et al. (2015), reported much lower retention of β-carotene. The carotenoid retention in this study will be much lower because Kokoro undergoes series of processing stages.
Also, in Table 3, carotenoids were not detected in the commercially purchased Kokoro because it was produced from white maize, which lacks pigment (Ford, 2000). The retinol activity equivalent (RAE) in Table 3 shows that Maize 2 had the highest value while in Table 4, it was observed that Kokoro 1 maize snack's retinol equivalent RAE) at 100 g and 150 g/serving size was the highest while Kokoro 4 (yellow maize) at both serving sizes was the lowest.
The lutein content before processing ranged from 10.38 to 12.87 μg g−1, while after processing, it ranged from 0.29 to 0.57 μg g−1. It was observed that the Maize 1 genotype had the highest lutein content before and after processing, followed by Maize 2 with (cycle 1), which is from a different planting cycle. It implies that cycle 0 (Maize 1) can retain lutein compared to other maize samples. Maize 2 and Maize 4 flour samples showed high values of zeaxanthin of 10.97 and 11.05 μg g−1, respectively, while Maize 1 had the lowest value of (6.03 μg g−1). However, Kokoro from Maize 3 contained the highest zeaxanthin value (0.75 μg/g), but the original content of flour was (9.91 μg g−1), while Maize 1 had the lowest zeaxanthin before and after processing, respectively (0.51; 6.03 μg g−1). The result implies that Maize 3 genotype retained zeaxanthin content, although it had a low value initially before processing into Kokoro. Maize 1 was observed to be the lowest before and after processing. The retaining ability of Maize 3 might be attributed to the genetic variation and cycle of the seed. Zeaxanthin is reported to be isomeric with lutein, and it provides the primary yellow color in maize (Sajilata et al., 2008). Lutein and zeaxanthin are carotenoids with no vitamin A activity, but they possess anti-oxidative properties. Reports have been made as to its presence in the macular pigment of the human eye, reducing the risk of macular degeneration, cataracts, age-related macular degeneration, and other degenerative diseases (Pixley et al., 2013; Vishwanathan and Johnson, 2013; Bernstein et al., 2016).
The summation of lutein and zeaxanthin is xanthophylls, and after processing, severe degradation was observed in all the maize samples from the initial values. This could be due to the exposure to light, high temperature, and oxygen during Kokoro processing steps and degradation and isomerization have been reported to be caused by the factors mentioned above (Rodriguez-Amaya, 2001; Ambrosio et al., 2006; Boon et al., 2010). However, Kokoro 1 was observed to have had the highest value, which implies the highest degradation amongst the Kokoro from all other maize samples.
β-cryptoxanthin is a vitamin A precursor providing only one-half of the provitamin A activity of β-carotene. Maize flour β-cryptoxanthin ranged from 1.94 to 4.06 μg g−1, and in Kokoro, it ranges from 1.25 to 1.50 μg g−1. Maize 4 was observed to contain the highest β-cryptoxanthin amount (4.06 μg/g), and Maize 2 had the highest value (3.54 μg g−1) among the PVA maize genotypes. Kokoro 2 and Kokoro 3 were not significantly different, while Kokoro 1 recorded the lowest value (1.25 μg g−1). From the result, it was observed that β-cryptoxanthin was the most abundant provitamin A carotenoid among the Kokoro samples. Furthermore, it was observed that β-cryptoxanthin was generally higher in the Kokoro from PVA maize genotypes than the landrace yellow maize.
The α-carotene content before and after processing is presented in Tables 3, 4. It was observed that Maize 1 had the lowest content before and after processing, and Kokoro 3 had the highest content in Table 4. This implies that Kokoro 3 will contribute more to the α-carotene precursor during provitamin A activity. α-carotene is a precursor of vitamin A, providing provitamin A activity, although it is not as potent as β-carotene because it provides only one molecule of vitamin A [Institute of Medicine (IOM), 2001; Grune et al., 2010].
Trans-β-carotene composition in the maize flour samples ranged from 2.10 to 3.25 μg g−1, while in the Kokoro samples, it was 0.74 to 1.54 μg g−1. Maize 2 flour had the highest trans-β-carotene content (3.25 μg g−1), and Maize 4 contains the lowest amount (2.10 μg g−1). Kokoro 1 recorded the highest value of 1.54 μg g−1 although, it had the lowest value (2.42 μg g−1) among the PVA maize before processing, while Kokoro 4 (control 1) had the lowest value (0.74 μg g−1). β-carotene, which is a precursor to vitamin A, and it is the most potent vitamin A active carotenoid because it can provide two molecules of retinol (Institute of Medicine (IOM), 2001; Grune et al., 2010). The result shows that Kokoro 1 has a high converting potential to vitamin A, and it would confer the highest provitamin A activity in comparison with other Kokoro samples from the maize genotypes.
For 13-cis-β-carotene, the maize flour samples ranged from 0.85 to 1.31 μg g−1 while 9-cis-β-carotene ranges from 0.88 to 1.67 μg g−1. The occurrence of cis-isomers (13-cis-β-carotene and 9-cis-β-carotene) can be attributed to the exposure and disintegration of the maize kernel matrix during pulverization to obtain the maize flour for the estimation of the carotenoid profile before processing. Maize 3 had the highest content (1.31 μg g−1 of 13-cis-β-carotene, and Maize 1 had the lowest content (0.93 μg g−1) among the PVA maize genotype while Maize 4 had 0.85 μg g−1. Maize flour from Maize 2 recorded the highest 9-cis-β-carotene value (1.67 μg/g), and Maize 1 had the lowest content (1.34 μg g−1) among the PVA maize genotypes. It can be implied from the result that Maize 3 and Maize 2 experienced isomerization faster to form a high content of 13-cis- and 9-cis, respectively, during the pulverization of maize grains to maize flour. In contrast, the isomerization formation rate during milling of Maize 1 grains was low. The 13-cis-β-carotene values for Kokoro ranged from 0.32 to 0.63 μg g−1 while 9-cis-β-carotene was from 0.41 to 0.93 μg g−1. Kokoro 1 was observed to have undergone the fastest isomer formation among the maize genotypes with the highest 13-cis value of (0.63 μg g−1) and 9-cis (0.93 μg g−1), respectively. In contrast, Kokoro 3 had the lowest isomer formation content for both 13-cis (0.57 μg g−1) and 9-cis (0.79 μg g−1). The exposure of the Maize 1 genotype to many unit operations during Kokoro processing brought about the high amount of 13-cis and 9-cis formation. In contrast, when exposed to short processing (pulverization to maize flour), isomer formation quantity was low. Aman et al. (2005) reported that the occurrence of carotenoid isomers like 13-cis and 9-cis and 15-cis β-carotene is attributed to varietal difference, sample storage, handling, and food processing. Also, the presence of elevated amounts of isomer formation in Kokoro 1 can cause loss of color, low/loss of vitamin A activity, and less absorption ability in humans compared with the trans component of the carotenoid.
Total β-carotene was found to be the highest in Maize 1 (3.10 μg g−1) after processing to Kokoro 1 from the initial value of 4.69 μg g−1 while Control 1 had the lowest total β-carotene in Kokoro of 1.47 μg g−1 from 3.83 μg g−1. Total β-carotene is the summation of trans, 13-cis, and 9-cis isomer. It can be implied from the result that Kokoro 1 carotenoids will be converted more to vitamin A.
Provitamin A (PVA) activity is derived from the summation of the amount of β-carotene (13-cis-β-carotene, 9-cis-β-carotene, and trans isomers) to half of the amounts of a-carotene and β-cryptoxanthin (Alamu et al., 2014). The provitamin A content of Kokoro samples ranged from 2.43 to 4.00 μg g−1, and they are significantly different from one another. Kokoro 1 had the highest value (4.00 μg g−1), while Kokoro 4 (control) had the lowest value (2.43 μg g−1). Kokoro 1, with the highest PVA quantity among the Kokoro samples, will have more conversion ability to vitamin A in the body. However, the amount in μg g−1 is lower in comparison with the HarvestPlus target value of 17 μg g−1 (dry weight) (Mulualem, 2015). In this study, it was observed that Maize 1 flour had a low quantity (5.96 μg g−1 before processing but retained more in Kokoro (4.00 μg g−1) while the highest PVA content in maize flour was Maize 2 (8.43 μg g−1) with a product of 3.87 μg g−1. However, Kokoro 3 had the lowest PVA content (3.77 μg g−1) amongst the PVA maize genotype with an initial value of 8.13 μg g−1. Thus, it could be inferred that the retention of PVA is genotype dependent. Alamu et al. (2015) who reported that PVA retention for boiled maize was genotype dependent. This finding has corroborated the overall objective of this study that aimed to test the improved and promising pipelines genotypes for suitability for product development.
Carotenoid Apparent Retention Profile
The carotenoid apparent retention result for Kokoro is presented in Table 5. The knowledge about the quantity of carotenoids present after processing is essential to determine the retention of the carotenoids and for increasing breeding targets during the creation of new breeds. It was observed in the profile that lutein and zeaxanthin had low percentage values compared to other carotenoids present. The degradation could be due to the polienic chain in the xanthophylls (lutein and zeaxanthin) causing an increased light absorption. However, this could result in high susceptibility to oxidative degradation and geometrical isomerization due to the exposure to light, high temperature, and oxygen in the many unit operations during processing (Britton et al., 1995). Kokoro 1 had an apparent PVA retention of 67.05%, Kokoro 2 had 45.88%, Kokoro 3 had 47.34%, and Kokoro 4 (control 1) 37.98%. The processing of these maize genotypes into Kokoro has many operations (boiling, steeping to ferment, wet milling, cutting, rolling, pre-frying, cooling, and post-frying) with a lengthy process duration (3 days). The increment in surface area of the maize resulted in the exposure of the carotenoid to the mechanism of isomerization/degradation through light, heat, atmospheric oxygen, etc. (Rodriguez-Amaya et al., 2011), which brought about a reduction in micronutrient level (carotenoid). Based on the result, Kokoro 1 had the highest retention value, which implies the presence of more micronutrient (carotenoid) compared to the others. The retention value observed could be a result of genes.
Percentage Contribution of PVA-Kokoro to Vitamin A Per Day
The percentage contribution of PVA-Kokoro per day for boys, girls, men, and women at 100 g and 150/serving sizes is presented in Table 6. Kokoro 1 (PVA SYN HGBC0) for all the servings shows the highest percentage contribution of vitamin A while Kokoro 4 (control 1) was the lowest. The result in Table 6 illustrates that PVA-Kokoro has almost twice the percentage contribution ability than yellow maize. This is important because the PVA maize genotypes contain increased carotenoid quantity targeted at reducing vitamin A deficiency.
Sensory Attributes
The sensory attributes of Kokoro are presented in Table 7. All attributes showed significant (p < 0.05) differences among all the samples except aroma and color characteristics. The color values ranged from 6.55 to 6.83 and were not significant from each other. The Kokoro samples from the PVA maize genotypes with Kokoro 4 (control 1) and commercial Kokoro (control 2) were liked slightly. This implies the panelists cared less about the color and had a slight likeness, whether it is white or yellow. The preference of the taste ranged from neither like nor dislike to liking moderately. It was observed that Kokoro 1 and 2 taste alike and were preferred among PVA maize genotypes, while Kokoro 4 (control 1) is similar in taste to commercial Kokoro (control 2). Based on the result, Kokoro 1, 2, and 4 (control 1) are familiar in taste to commercial Kokoro (control 2). The aroma parameter showed no significant differences when compared to the commercially purchased Kokoro due to the same range of mean scores obtained in Table 7. This can imply that the aroma was the same or in a close range as the commercially purchased Kokoro. The crispness of Kokoro 4 (control 1) and commercial Kokoro (control 2) were the most preferred. The least preferred was Kokoro 3, and it was neither liked nor disliked. Color and aroma did not influence the sensory perception of the panelists. However, taste and crispness might have had a substantial effect on the overall acceptability. Kokoro 4 (control 1) and commercial Kokoro (control 2) had a high mean sensory score in overall acceptability, and among the PVA maize genotypes, Kokoro 1 was the highest. Kokoro 3 sample was observed to be the least preferred in terms of taste, color, crispness, aroma, and overall acceptability. However, all the samples had general acceptability for all evaluated sensory attributes as none scored below the minimum acceptable rating of 5 on the 9-point hedonic scale reported by Adebowale and Komolafe (2018), Idowu (2015), and Ayinde et al. (2011).
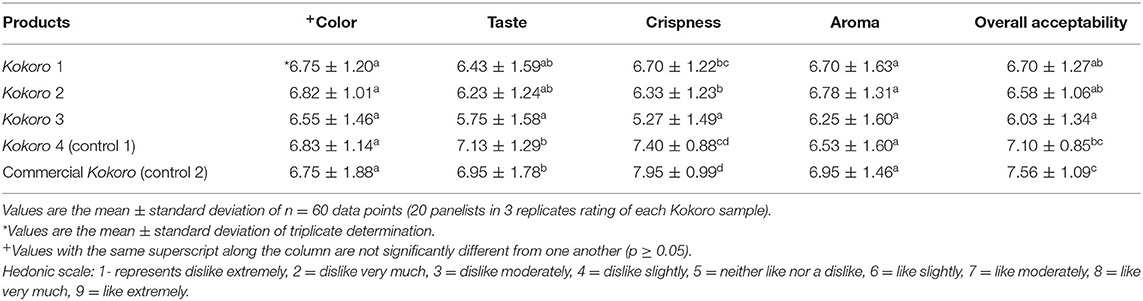
Table 7. Sensory attributes of Kokoro from provitamin A maize genotypes and commercially purchased Kokoro.
Kokoro 4 (control 1) and commercially purchased Kokoro had values that were very close to each other for all parameters estimated. This can mean that Kokoro 4 was not significantly different from the purchased commercial Kokoro (control 2), Kokoro 4 is the most acceptable sample. However, among the PVA maize genotypes, Kokoro 2 had the highest overall acceptability mean score, implying it is acceptable. Various authors have reported the increase of nutrient quality acceptability of Kokoro with the aid of supplementation with legumes; Ayinde et al. (2011) reported that the Kokoro composition of 90:10 maize-beniseed blend was the most preferred while 60:40 was the least accepted and this could be due to the nutty beniseed. Abegunde et al. (2014) stated that the supplementation of Kokoro with cowpea caused a decreased acceptability with increase in cowpea flour.
Furthermore, Dauda et al. (2020) reported that maize snack (Kokoro) fortified with groundnut (10 and 20%) was the most preferred. Various authors have also reported the acceptability of other food product from biofortified crops. Ukpabi et al. (2012) stated the potential use of orange-fleshed sweet potato (OFSP) chips and reported low acceptability between varieties. Ukom et al. (2019) reported increased nutrient in maize ogi with supplementation of OFSP and African yam bean and Alamu et al. (2014) reported high acceptability of roasted orange maize hybrid without husk.
Conclusion
The study has shown that nutritious Kokoro with carotenoid content can be produced from PVA maize genotypes compared to the commercially purchased Kokoro that lacks carotenoid content. The chemical composition of maize flour and Kokoro were comparable with previous authors in terms of moisture, crude fiber, ash, and carbohydrate. The carotenoid profile before processing (maize flour) had high levels of carotenoid content. The Kokoro processing steps (boiling, steeping, wet-milling, cutting, rolling, pre-frying, cooling and post-frying) triggered isomerization and degradation of carotenoids. In terms of PVA content, Maize 1 had the highest PVA content and carotenoid retention after processing to Kokoro 1. Also, the sensory perception showed that Kokoro 1 is generally acceptable among PVA maize genotypes. Amongst the maize genotypes, Kokoro 1 (PVA SYN HGBC0) for all the servings size (100 and 150 g) had the highest percentage contribution of vitamin A. The Kokoro made from both the landrace yellow maize (control 1) and the provitamin A maize genotypes could serve as a better alternative snack for the general populace, most especially school children as compared to the white commercial Kokoro that is devoid of carotenoids. However, it is essential to mention that there is a need for a substantial level of carotenoids after processing in food products to prevent the effects of the deficiency. Therefore, there is need for maize breeders to improve further the level the provitamin A carotenoids in biofortified maize to have a high retention of PVA after the rigorous processing. Thus, PVA maize consumption will contribute significantly to the daily intake of vitamin A and retinol activity equivalent (RAE) of the target consumers. Further studies on this product are storage (shelf-life) and packaging of PVA maize Kokoro to establish the shelf life and appropriate packaging material.
Data Availability Statement
The raw data supporting the conclusions of this article will be made available by the authors, without undue reservation.
Ethics Statement
The studies involving human participants were reviewed and approved by International Institute of Tropical Agriculture Internal Research Ethics Board. The patients/participants provided their written informed consent to participate in this study.
Author Contributions
EA and BO: conceptualization and supervision. EA and BM-D: methodology and funding acquisition. BO and OA: software. EA, BO, BM-D, and AM: validation. OA, EA, and BO: formal analysis, investigation, and data curation. BM-D and AM: resources. OA: writing—original draft preparation. EA, BO, BM-D, and AM: writing—review and editing. All authors have read and agreed to the published version of the manuscript.
Conflict of Interest
The authors declare that the research was conducted in the absence of any commercial or financial relationships that could be construed as a potential conflict of interest.
Acknowledgments
This work was supported by the Bill & Melinda Gates Foundation (BMGF) through a grant OPP1178942, CGIAR-CRP Maize and IITA's Graduate Research Fellowship Program. The support of Bowen University (Department of Food Science and Technology), Staff of Food and Nutrition Science Laboratory (FNSL, IITA), especially Mr Adesokan Michael and the IITA Maize Improvement Program, especially Mr. Tayo Ojo is also acknowledged.
References
Abdulrahaman, A. A., and Kolawole, O. M. (2006). Traditional preparations and uses of maize in Nigeria. Ethnobotanical Leaflets 10, 219–227.
Abegunde, T. A., Bolaji, O. T., and Adeyemo, T. B. (2014). Quality evaluation of maize chips (Kokoro) fortified with cowpea flour. Nigerian Food J. 32, 97–104. doi: 10.1016/S0189-7241(15)30101-6
Abolurin, O. O., Adegbola, A. J., Oyelami, O. A., Adegoke, S. A., and Bolaji, O. O. (2018). Prevalence of vitamin A deficiency among under-five children in South-Western Nigeria. Nigerian Postgraduate Med. J. 25, 13–15. doi: 10.4103/npmj.npmj_20_18
Adebowale, O. J., and Komolafe, O. M. (2018). Effect of supplementation with defatted coconut paste on proximate composition, physical and sensory qualities of a maize-based snack. J. Culinary Sci. Technol. 16, 40–51. doi: 10.1080/15428052.2017.1315322
Adegunwa, M. O., Adeniyi, O. D., Adebowale, A. A., and Bakare, H. A. (2015). Quality evaluation of Kokoro produced from maize-pigeon pea flour blends. J. Culinary Sci. Technol. 13, 200–213. doi: 10.1080/15428052.2015.1015665
Adelakun, O. E., Adejuyitan, J. A., Olajide, J. O., and Alabi, B. K. (2005). Effect of Soybean substitution on some physical, compositional and sensory properties of Kokoro (a local maize snack). Eur. Food Res. Technol. 220, 79–82. doi: 10.1007/s00217-004-1034-y
Ajaiyeoba, A. I. (2001). Vitamin A deficiency in Nigerian children. Afr. J. Biomed. Res. 4, 107–110. doi: 10.4314/ajbr.v4i3.53882
Akinyinka, O. O., Usen, S. O., Akanni, A., Falade, A. G., Osinusi, K., Ajaiyeoba, I. A., et al. (2001). Vitamin A status of preschool children in Ibadan (South West Nigeria), risk factors and comparison of methods of diagnosis. West Afr. J. Med. 20, 243–248.
Akoja, S. S., Adebowale, O. J., Makanjuola, O. M., and Salaam, H. (2016). Functional properties, nutritional and sensory qualities of maize-based snack (Kokoro) supplemented with protein hydrolysate prepared from pigeon pea (CajanusCajan) seed. J. Culinary Sci. Technol. 15, 306–319. doi: 10.1080/15428052.2016.1259134
Alamu, E. O., Maziya-Dixon, B., Menkir, A., and Olaofe, O. (2014). Effects of husk and harvest time on carotenoid content and acceptability of roasted fresh cobs of orange maize hybrids. Food Sci. Nutr. 2, 811–820. doi: 10.1002/fsn3.179
Alamu, E. O., Maziya-Dixon, B., Menkir, A., and Olaofe, O. (2015). Effects of husk and harvesting on provitamin A activity and sensory properties of boiled fresh orange maize hybrids. J. Food Qual. 38, 387–395. doi: 10.1111/jfq.12158
Amajor, J. U., Oti, E., Ekeledo, N., Omodamiro, R., Amajor, E. E., and Aniedu, C. (2014). Studies on the characteristic properties of fermented, sun-dried orange-fleshed sweet potato flour. Nigerian Food J. 32, 45–53. doi: 10.1016/S0189-7241(15)30095-3
Aman, R., Schieber, A., and Carle, R. (2005). Effects of heating and illumination on trans-cis isomerization and degradation of β-carotene and lutein in isolated spinach chloroplasts. J. Agric. Food Chem. 53, 9512–9518. doi: 10.1021/jf050926w
Ambrosio, C. L. B., Campos, F. A. C. S., and Faro, Z. P. (2006). Carotenoids como alternative contra a hipovitaminose A. Rev. Nutr. 19, 233–243. doi: 10.1590/S1415-52732006000200010
AOAC (2012). Official Methods of Analysis of the Association of Analytical Chemists. 19th ed. Washington, DC.
Awoyale, W., Maziya-Dixon, B., and Abebe, M. (2016). Retention of provitamin A carotenoids in ogi powder as affected by packaging materials & storage conditions. J. Food Nutr. Res. 4, 88–93.
Ayinde, F. A., Bolaji, O. T., Abdus-Salaam, R. B., and Osidipe, O. (2011). Functional properties and quality evaluation of “Kokoro” blended with beniseed cake Sesame indicium. Afr. J. Food Sci. 6, 117–123. doi: 10.5897/AJFS11.086
Ayinde, O. E., and Adewunmi, M. O. (2016). Risk and adoption analysis of innovation in cassava production in Oyo State, Nigeria: a case study for vitamin A variety. World Congress Root and Tuber Crops Nanning (Guangxi).
Bechoff, A., Taleon, V., Carvalho, L. M. J., Carvalho, J. L. V., and Boy, E. (2017). Micronutrient (Provitamin A and Iron/Zinc) retention in biofortified crops. Afr. J. Food Agric. Nutr. Dev. 17, 11893–11904. doi: 10.18697/ajfand.78.HarvestPlus04
Bernstein, P. S., Li, B., Vachali, P. P., Gorusupudi, A., Shyam, R., Henriksen, B. S., et al. (2016). Lutein, zeaxanthin, and meso-zeaxanthin: the basic and clinical science underlying carotenoid-base nutritional interventions against ocular disease. Progress Retinal Eye Res. 50, 34–66. doi: 10.1016/j.preteyeres.2015.10.003
Boon, C. S., McClement, D. J., Weiss, J., and Decker, E. A. (2010). Factors influencing the chemical stability of carotenoids in foods. Critical Rev. Food Sci. Nutr. 50, 512–532. doi: 10.1080/10408390802565889
Britton, G., Liaaen-Jensen, S., and Pfander, H. (1995). “Carotenoids today and challenges for the future,” in Carotenoids, eds G. Briiton, S. Liaaen-Jenssen, and H. Pfander (Berlin: Birkhauser Verlag), 13–26.
Dauda, A. O., Kayode, R. M. O., and Salami, K. O. (2020). Quality attributes of snack made from substituted with groundnut. Ceylon J. Sci. 49, 21–27. doi: 10.4038/cjs.v49i1.7702
De Moura, F. F., Miloff, A., and Boy, E. (2015). Retention of provitamin A carotenoids in staple crops targeted for biofortification in Africa: cassava, maize, and sweet potato. Crit. Rev. Food Sci. Nutr. 55, 1246–1269. doi: 10.1080/10408398.2012.724477
Etuk, U., and Umoh, I. (2014). Adoption pattern of pro-vitamin A technology among farmers in Akwa Ibom State. Nigerian J. Agric. Food Environ. 10, 135–138.
Eyinla, T. E., Maziya-Dixon, B., Alamu, E. O., and Sanusi, R. A. (2019). Retention of pro-vitamin A content in products from new biofortified cassava varieties. Foods 8:177. doi: 10.3390/foods8050177
Fasasi, O. S., and Alokun, A. O. (2013). Physicochemical properties, vitamins, antioxidant activities and amino acid composition of ginger-spiced maize snack ‘Kokoro' enriched with soy flour (a Nigeria based snack). Agric. Sci. 4, 73–77. doi: 10.4236/as.2013.45B014
Ford, R. (2000). Inheritance of kernel colour in corn: explanation and Investigation. Am. Biol. Teach. 62:3. doi: 10.2307/4450870
Grune, T., Lietz, G., Palou, A., Ross, A. C., Stahl, W., Tang, G., et al. (2010). Beta-carotene is an important vitamin A source for humans. J. Nutr. 140, 2268S−2285S. doi: 10.3945/jn.109.119024
Howe, J. A., and Tanumihardjo, S. A. (2006). Evaluation of analytical methods for carotenoid extractions from biofortified maize (Zea mays sp.). J. Agric. Food Chem. 54, 7992–7997. doi: 10.1021/jf062256f
Idowu, A. O. (2015). Nutrient composition and sensory properties of Kokoro (a Nigerian snack) made from maize and African yam bean flour blends. Int. Food Res. J. 22, 739–744.
IIona, P., Bouis, H. E., Palenberg, M., Moursi, M., and Oparinde, A. (2017). Vitamin A cassava in Nigeria: crop development and delivery. Afr. J. Food Agric. Nutr. Dev. 17, 12000–12025. doi: 10.18697/ajfand.78.HarvestPlus09
Ilg, A., Beyer, P., and Al-Babili, S. (2009). Characterization of the rice carotenoid cleavage dioxygenase 1 reveals a novel route for geranial biosynthesis. FEBS J. 276, 736–747. doi: 10.1111/j.1742-4658.2008.06820.x
Institute of Medicine (IOM) (2001). Dietary Reference Intakes for Vitamin A, Vitamin K, Arsenic, Boron, Chromium, Copper, Iodine, Iron, Manganese, Molybdenum, Nickel, Silicon, Vanadium and Zinc. Washington, DC: National Academy Press. p. 800.
Iwe, M. O., Onyeukuw, U., Agiriga, A. N., and Fatih, Y. (2016). Proximate, functional and pasting properties of FARO 44 rice, African yam bean and Brown cowpea seeds composite flour. Cogent Food Agric. 2:1. doi: 10.1080/23311932.2016.1142409
Maziya-Dixon, B. B., Akinyele, I. O., Sanusi, R. A., Oguntona, T. E., Nokoe, S. K., and Harris, E. W. (2006). Vitamin A deficiency is prevalent in children less than 5 y of age in Nigeria. J. Nutr. 136, 2255–2261. doi: 10.1093/jn/136.8.2255
Mulualem, T. (2015). Application of bio-fortification through plant breeding to improve the value of staple crops. Biomed. Biotechnol. 3, 11–19.
Ndukwe, O. K., Edeoga, H. O., and Omosun, G. (2015). Varietal differences in some nutritional composition of ten maize (Zea Mays L.) varieties grown in Nigeria. Int. J. Acad. Res. Reflect. 3, 1–10.
Nuss, E. T., and Tanumihardjo, S. A. (2010). Maize a paramount staple crop in the context of global nutrition. Comprehens. Rev. Food Sci. Food Safety 9, 417–436. doi: 10.1111/j.1541-4337.2010.00117.x
Oluwafemi, G. I., Seidu, K. T., and Akinruli, B. O. (2018). Production and quality evaluation of maize chips (Kokoro) produced from maize and whole limabean seed flour blends. J. Adv. Food Technol. 1:102. doi: 10.15744/2639-3328.1.102
Oranusi, S., and Dahunsi, S. O. (2015). Preliminary study on hazards and critical control points of Kokoro, a Nigerian indigenous fermented maize snack. SpringerPlus 4:253. doi: 10.1186/s40064-015-1026-3
Oritz, D., Ponrajan, A., Bonnet, J. P., Rocheford, T., and Ferruzzi, M. G. (2018). Carotenoid stability during dry milling, storage, and extrusion processing of biofortified maize genotypes. J. Agric. Food Chem. 66, 4683–4691. doi: 10.1021/acs.jafc.7b05706
Oso, O. O., Abiodun, P. O., Omotade, O. O., and Oyewole, D. (2003). Vitamin A status and nutritional intake of carotenoids of preschool children in Ijaye Orile community in Nigeria. J. Trop. Paediatr. 49, 42–27. doi: 10.1093/tropej/49.1.42
Otunola, E. T., Sunny-Roberts, E. O., Adejuyitan, J. A., and Famakinwa, A. O. (2012). Effects of addition of partially defatted groundnut paste on some properties of ‘Kokoro' (a popular snack made from maize paste). Agric. Biol. J. North Am. 3, 280–286. doi: 10.5251/abjna.2012.3.7.280.286
Pillay, K., Siwela, M., Derera, J., and Veldman, F. K. (2014). Provitamin A carotenoids in biofortified maize and their retention during processing and preparation of South African maize foods. J. Food Sci. Technol. 51, 634–644. doi: 10.1007/s13197-011-0559-x
Pixley, K., Palacios-Rojas, N., Babu, R., Mutale, R., Surles, R., and Simpungwe, E. (2013). “Biofortification of maize with provitamin A carotenoids,” in Carotenoids and Human Health, ed S. A. Tanumihardjo (New York, NY: Springer Science), 271–292. https://doi.org/10.1007/978-1-62703-203-2_17
Rodriguez-Amaya, D., Nutti, M., and de Carvalho, J. L. (2011). “Carotenoids of sweet potato, cassava and maize and their use in bread and flour fortification,” in Flour and Breads and Their Fortification in Health and Disease Prevention, eds V. B. Patel, V. R. Preedy, R. R. Watson (London: Academic Press; Elsevier), 301–311. https://doi.org/10.1016/B978-0-12-380886-8.10028-5
Rodriguez-Amaya, D. B. (2001). A Guide to Carotenoid Analysis in Foods. Washington, DC: ILSI and Human Nutrition Institute. p. 64.
Sagbo, F. Y. S., Aïssi, M. V., Hounkpatin, W. A., Houedo, C., Dansi, A., and Soumanou, M. M. (2017). Physicochemical and pasting properties of some local and improved maize varieties cultivated in Benin. Int. J. Biol. Chem. Sci. 11, 1753–1765. doi: 10.4314/ijbcs.v11i4.27
Sajilata, M. G., Singhal, R. S., and Kamat, M. Y. (2008). The carotenoid pigment zeaxanthin- a review. Comprehens. Rev. Food Sci. Food Safety 7, 29–49. doi: 10.1111/j.1541-4337.2007.00028.x
Shaista, Q., Muhammad, A., Fahrulzaman, H., and Muhammad, A. J. (2017). Comparative study for the determination of nutritional composition in commercial and non-commercial maize flours. Pakistan J. Bot. 49, 519–523.
Srivastava, R. P. (2016). “Anti-nutrients restraining biofortification,” in Biofortification of Food Crops (Springer India), 333–348. doi: 10.1007/978-81-322-2716-8_24
Stevens, G. A., Bennett, J. E., Hennocq, Q., Lu, Y., De-Regil, L. M., Rogers, L., et al. (2015). Trends and mortality effects of vitamin A deficiency in children in 138 low-income and middle-income countries between 1991 and 2013: a pooled analysis of population-based surveys. Lancet Global Health 3, e528–536. doi: 10.1016/S2214-109X(15)00039-X
Udensi, U. E., Tarawali, G., and Favour, E. U. (2011). Adoption of selected improved cassava varieties among small holder farmers in South Eastern Nigeria. J. Food Agric. Environ. 99, 329–335.
Ukom, A. N., Adiegwu, E. C., Ojmelukwe, P. C., and Okwunodulu, I. N. (2019). Quality sensory acceptability of yellow maize ogi porridge enhriched with orange-fleshed sweet potato and African yam bean seed flours for infants. Sci. Afr. 6, 1–24. doi: 10.1016/j.sciaf.2019.e00194
Ukpabi, U. J., Ekeldo, E. N., and Ezigbo, V. U. (2012). Potential use of roots of orange fleshed sweet potato genotypes in the production of β-carotene rich chips in Nigeria. Afr. J. Food Sci. 6, 29–33. doi: 10.5897/AJFS11.186
Keywords: Kokoro, chemical composition, provitamin A maize, carotenoid retention, acceptability
Citation: Akinsola OT, Alamu EO, Otegbayo BO, Menkir A and Maziya-Dixon B (2020) Evaluation of Quality and Acceptability of Snack (Kokoro) Produced From Synthetic Provitamin A Maize (Zea mays) Genotypes. Front. Sustain. Food Syst. 4:576217. doi: 10.3389/fsufs.2020.576217
Received: 25 June 2020; Accepted: 04 November 2020;
Published: 26 November 2020.
Edited by:
Abdul Wakeel, University of Agriculture, Faisalabad, PakistanReviewed by:
Agnieszka Barbara Najda, University of Life Sciences of Lublin, PolandBryan M. Gannon, Cornell University, United States
Copyright © 2020 Akinsola, Alamu, Otegbayo, Menkir and Maziya-Dixon. This is an open-access article distributed under the terms of the Creative Commons Attribution License (CC BY). The use, distribution or reproduction in other forums is permitted, provided the original author(s) and the copyright owner(s) are credited and that the original publication in this journal is cited, in accordance with accepted academic practice. No use, distribution or reproduction is permitted which does not comply with these terms.
*Correspondence: Emmanuel Oladeji Alamu, oalamu@cgiar.org