- DISTAL – Department of Agricultural and Food Sciences, Alma Mater Studiorum – Bologna University, Bologna, Italy
In recent years, urban agriculture (UA) projects have bloomed throughout the world, finding large applications also in the developed economies of the so-called Global North. As compared to projects in developing countries, where research has mainly targeted the contribution to food security, UA in the Global North has a stronger multifunctional connotation, and results in multiple combinations of farming purposes and business models pursued. The present review paper explores the contribution and role that UA plays in cities from the Global North, defining its functionalities toward ecosystem services (ES) provisioning and analyzing the factors that hinders and promote its regional diffusion and uptake. The manuscript integrates a description of UA growing systems, as well opportunities for crop diversification in the urban environment, and a comprehensive classification of UA business models. The distinctive features in terms of business models, farming purposes and farm size are then applied over an inventory of 470 UA projects in the Global North, allowing for a characterization and comparative analysis of distribution frequency of the different project typologies.
Introduction
First, it was hunting for food and caves to live in. Then, settled agriculture came, in the form of horticulture, primarily practiced by women, to complement the game that men brought home (Hansen et al., 2015). Homes that were built to provide shelter to the family, with horticulture that along the ages would become the first formal organization of nature, following strictly defined structural patterns. While geometry naturally occurs within ecological systems, human mind requires regular forms; therefore, gardens were created following geometrical patterns already in ancient Egypt. Integration of agriculture within the anthropic landscape also emerged in Babylon's hanging gardens (Figure 1) or in the so-called sacred lands devoted to food production in Greek cities in the classical era (Isager and Skydsgaard, 2013). In Roman gardens, exotic species could be found, as emerged in the buried gardens of Pompeii. The practice of plant cultivation in villages and towns further became established in the middle ages in the form of hortus, where applications of relationships, dimensions and figures evolved from the Pythagoreans (Steenbergen and Reh, 2003). The hortus pattern recurred through gardens that complete the village's general geometry and feed the local community. They were often placed between the town defensive walls, enabling food security in times of wars. Horticulture also developed in monasteries where food production and processing were established under the Rule of Saint Benedict (Aben and de Wit, 1999). Arabic gardens combined beauty and sensorial experiences, building on the beneficial effects that plants can bring to humans (Figure 1).
In the modern age, and along the Renaissance, whole farms were conceived following the hortus design, until the Romanticism and toward the time of affirmed urbanism, where a re-unification of the rural-urban continuum occurred. Exotic plant species were grown in tropical glasshouses in private villas of the wealthier or in urban botanical parks. English, Italian and French gardening schools were born, paving the way for modern landscape architecture principles. In the contemporary age, and from the industrial revolution, gardens were found within the fringes of industrial towns, contributing to the food security of the migrant workers (Partalidou and Anthopoulou, 2017). Allotment gardens were established and became formally recognized. During the world conflicts of the twentieth century, war or “victory” gardens were promoted by governments to feed the urban population, while starting from the post-war period, urban horticulture has become a social structure, an economically fundamental element, a source of ecosystem services (ES) for sustainable cities (Keshavarz and Bell, 2016). It assumed the form of political activism, as for the case of community gardens and guerrilla gardening initiatives. In these same years, however, the rapid economic growth of cities was associated with a general decentralization of functions (e.g., with agriculture being moved out from the city, where it was assumed to be healthier thanks to the lower air pollution), and the increased urbanization resulted in severe fragmentation of urban and peri-urban farmed plots (Mok et al., 2014). Nevertheless, from the beginning of the twenty first century, the rapid evolution of agricultural technologies has brought new forms of plant cultivation, allowing for multiple productions and circularity (e.g., in aquaponics systems), but also through the creation of common metabolisms in urban buildings, as for the growing examples of vertical farming and rooftop agriculture projects (Figure 1) (La Rosa et al., 2014).
While fresh horticultural goods represent its main products, urban farming today also explores new crops and novel food products and services. A set of innovative business models takes form, and a plethora of experiences emerge at global level. Meanwhile, a clear differentiation emerges between urban agriculture (UA) specifically aimed at tackling food insecurity and the forms it may take when occurring in wealthier world regions, where the associated ES may even become more relevant than food production per se, at least until the SARS-CoV-2 outbreak (Lal, 2020). Indeed, while the role and functions of UA in developing countries have been addressed in several review papers (Bryld, 2003; de Bon et al., 2010; de Zeeuw et al., 2011; Gallaher et al., 2013; Orsini et al., 2013; Hamilton et al., 2014; Poulsen et al., 2015), a more limited body of literature (e.g., Mok et al., 2014; Lin et al., 2015) considered to date the role and forms it may play within stronger economies of the so-called Global North (e.g., UN classified “developed countries, United Nations, 2020).
In the coming sections, this review paper brings together a comprehensive state of the art of all the above-listed features of agriculture in cities in the Global North, including a classification and inventory of urban farming projects. While the research does not target the comparative analysis of UA in the Global North vs. Global South, it actually builds on data from Global North in order to answer the following research questions:
1) Which are the main ES of UA in the Global North?
2) Which factors affect the development and diffusion of UA projects?
3) Which are the most represented farming systems for UA in the Global North?
4) What is the average farm size in UA projects?
5) How can business models in UA be classified and which business models are more common in the different regions of the Global North?
6) Which are the most relevant farming purposes of UA?
In order to answer the research questions, the research methodology combined an extensive literature review on ES provided, functions, enabling factors, cropping system typologies and associated business models observed in UA projects in the Global North, altogether with the implementation and classification of a database of regional case studies. The literature review was performed using scientific journal databases, including Google Scholar® and SCOPUS®. Search strings were compiled by integrating selected keywords including “urban farming,” “urban and periurban agriculture AND/OR horticulture,” “urban food security,” “urban food safety,” “horticultural therapy,” “multifunctional agriculture,” “ecosystem services,” “social function,” “urban regeneration,” “urban planning,” “urban land,” “vacant land,” “eco-efficiency assessment,” “life cycle assessment (LCA),” “social-life cycle assessment (S-LCA),” “social justice,” “urban green infrastructures,” “life cycle costing (LCC),” “urban ecology,” “urban biodiversity,” “food contaminants,” “urban pollution,” “urban heat island,” “allotment gardens,” “community gardens,” “rooftop agriculture AND/OR farming AND/OR garden AND/OR greenhouse,” “hydroponics,” “plant factories with artificial lighting (PFAL),” “vertical farms,” “urban beekeeping,” “urban peasant,” “business model,” “farming purpose,” “entrepreneurial urban agriculture.” Within each combined search, selected articles from first 50 results for each keyword search were used for the study, altogether with snowball sampling (e.g., from references and citations included in already identified documents) additional articles already familiar to the authors and those suggested during the review process. The inventory of regional case studies in the Global North was implemented using internet search (through Google®), of selected keywords (including multiple combined searches for words “urban farming,” “urban agriculture,” “community supported agriculture,” “vertical farm,” “indoor farm,” “rooftop garden,” “community garden,” “rooftop greenhouse,” “allotment garden,” “periurban farm,” “solidarity buying group,” “farmers market,” “workshop”), eventually translated in national languages through online translators. Among identified projects, those with available information (e.g., online or responding to contact e-mails) were integrated in the study, accounting for a total of 470 projects, out of which, 417 declared their cultivated area (m2). The results emerged from both the literature survey and the implemented case study database are discussed in the following sections. Classes for cultivated area were then created, diversifying projects based on area below 500 m2, between 501 and 1,000 m2, between 1,001 and 5,000 m2, between 5,001 and 25,000 m2, between 25,001 and 100,000 m2 and those above 100,000 m2 (10 ha). Contingency tables were used to analyze the relationships among class of projects dimension and business model typologies (n = 447), farming purposes (n = 470), city typology (n = 470), city density (n = 470), or city climate (n = 470). According to Magrefi et al. (2018), business models were classified in cost-reduction, differentiation, diversification, share economy, experience and experimental. Farming purposes were classified in commercial, image, innovation, social and educational and urban living quality (Thomaier et al., 2015). Cities were classified according to urban population in six categories (Dijkstra and Poelman, 2012): Small (S) for cities with a population ≤ 100,000 inhabitants; Medium (M) for cities with a population ranging 100,001 to 250,000 inhabitants; Large (L) for cities with a population ranging 250,001 to 500,000 inhabitants; Extra-large (XL) for cities with a population ranging 500,001 to 1,000,000 inhabitants; Megacity (XXL) for cities with a population ranging 1,000,001 to 5,000,000; (Global city) for cities with a population ≥ 5,000,0001 inhabitants. Cities were also classified according to city density in three categories (Saldivar-Sali, 2010): “LOW density” for cities with a population density ≤ 5,000 inhabitants km−2; “MEDIUM” density for cities with a population density ranging 5,001 to 15,000 inhabitants km−2; “HIGH density” for cities with a population density ≥ 15,001 inhabitants km−2. Finally, city climate was classified according to Koppen's classification in tropical, temperate and continental.
Chi-square test was used to assess the null hypothesis (i.e., the independence of the two categorical descriptors) comparing observed and expected frequencies. When Chi-square test resulted significant, a standardized Pearson residuals analysis as a measure of the strength of the difference between observed and expected values was performed (Agresti, 2003). Standardized residuals are useful as they enhance the detection of the cells that mainly contribute to the value. Additionally, a Bonferroni adjustment was applied by dividing the significance level by the number of cells in the contingency table in order to compensate for potential type 1 family wide errors (Garcia-Perez and Nunez-Anton, 2003).
Defining Urban Agriculture (UA)
UA has been defined by the Food and Agriculture Organization of the United Nations as the “plant cultivation and animal rearing (including aquaculture) within cities and towns and in their immediate surroundings” (Drechsel and Kunze, 2001). While providing for both food and non-food products for household consumption as well as income generation, UA also includes all related activities (production and sale of agricultural inputs and processing and marketing of products) (Mougeot, 2000). Being a complementary activity to the dominant agricultural production taking place in the countryside, UA overall increases the efficiency of the food system. UA allows for producing several typologies of crops (cereals, roots and vegetables, fruits, herbs, ornamentals, trees) and livestock (poultry, rabbits, goats, sheep, cattle, pigs, guinea pigs, fish) or animal-based products (meat, eggs, milk). However, in most cases it is represented by horticultural crops (vegetables, aromatic and medicinal plants, flowers and ornamentals, fruit and wood trees) grown in small fields or gardens (Orsini et al., 2013). Within a recent debate on how to define UA in developed economies, definitions building on its main features were elaborated, including where it is conducted (spatial dimension), what it produces (functional dimension), why it takes place (motivational dimension), where its produce is consumed (market dimension), how it generates (origin dimension), or by whom it is performed (actor dimension) (Vejre et al., 2016). Furthermore, as within UA projects in the Global North, externalities and non-food products may take over the primary driver of farming, their classification may either rely on the adopted business strategy (Pölling et al., 2015) or the main farming purpose (Thomaier et al., 2015), as further detailed in the following sections.
Ecosystem Services (ES) Associated With UA
As farming takes place closer to cities or even inside them, several differences from conventional agriculture arise, translating into both advantages and limitations (Table 1), whose perception among societal groups and initiatives may largely vary (Sanyé-Mengual et al., in review). It appears that, according to Sanyé-Mengual et al. (2019), in order to be viable among the three sustainability dimensions (social, economic and environmental) and overcome the constraints related to the urban environment, the development of UA needs to combine both social and technological innovations. As a result, urban farming must bring together the existing knowledge and advances from the traditional agricultural sector with a set of new skills, technologies, tools and strategies allowing to target a diversified set of ES, falling into four main categories (TEEB, 2010):
(a) provisioning services: services that describe the material or energy output from ecosystems, including food, raw materials, water and medicinal plants (Pourias et al., 2015).
(b) regulating services: services that ecosystems provide by acting as regulators (e.g., regulating the quality of air and soil, storing greenhouse gases, or providing flood and disease control, Camps-Calvet et al., 2016).
(c) habitat services: services that ecosystems provide by the maintenance of genetic biodiversity and offering habitat for species (Lin et al., 2015).
(d) cultural services: services which represent the non-material flow of benefits from ecosystems to humans, including recreation, mental and physic health, tourism, spiritual experiences, amenity or social inclusion (Camps-Calvet et al., 2016).
All of them are strictly connected with and contribute to the functionality and environmental sustainability of the city. Moreover, since UA experiences primarily target specific ES, a classification based on the farming purpose has been recently elaborated (Thomaier et al., 2015), which distinguishes UA projects in five main categories (image, commercial, urban living quality, innovation, or social-education).
Urban Food and Nutrition Security
Among provisioning services supplied by UA, the most acknowledged is associated with food production and supply. Indeed, while estimates for the potential UA contribution to food security (Orsini et al., 2013) and sovereignty (García-Sempere et al., 2019) are available for several cities in developing countries, a lower number of studies addressed the quantification of urban food production in cities from richer economies, where aims of UA are mainly associated with environmental and social functionalities. Nonetheless, food production potential of UA in the Global North has raised interest in response to economic crises (e.g., after 2007, Colasanti et al., 2012) or as a tool to mitigate the effects of food deserts on the health status of the poorest strata of the population (Meenar and Hoover, 2012; McClintock et al., 2013). The emergent phenomenon of new peasantry as a response to growing urban poverty has also lead to new forms of UA, where innovation takes place in both businesses and land-use models, as occurred for instance in Detroit, Michigan (USA) (Draus et al., 2014), Berlin (Germany) (Clausen, 2015) and Yokohama (Japan) (Ikejima, 2016). Accordingly, several studies targeted in the last couple of decades the quantification of potential food production of UA in cities of North America, Europe, Asia and Oceania (Table 2), although estimates most often built on scenarios rather than actually measured data.
Health
Horticulture is a discipline that has great therapeutic potential, and its role in human well-being was well-explained by Diane Relf in her “Human Issues in Horticulture,” which examined “the other side of horticulture” (Relf, 1992). Since then, horticulture's therapeutic roles were increasingly studied and debated, and the definitions and methodologies that use horticulture as support in therapeutic processes of physical and/or mental rehabilitation were elaborated (Relf and Dorn, 1995; Burls, 2008). A distinction was made between Horticultural Therapy and Therapeutic Horticulture. Horticultural Therapy is defined as a process through which plants, gardening activities and the simple contact with nature are used as tools in therapy and rehabilitation programs conducted by a therapist. It is an active process in which horticulture is used as support for other rehabilitation interventions. Therapeutic Horticulture is instead defined as a process that uses plants and the relationship with them to create or improve people's physical, psychological and social well-being. It is a process in which the plant plays a central role but in which specific therapeutic objectives are not pursued (Shoemaker and Diehl, 2010).
The therapeutic role of horticulture is based on the general positive psychological and physiological actions promoted by all sensations and emotions that arise from contact with nature, especially in those contexts (e.g., a walk in a park, taking care of a vegetable garden, the presence and sight of plants and flowers) in which the relationship between man and nature does not have the character of a working commitment (Ulrich, 1984; Ulrich et al., 1991; Kaplan, 1995; Thwaites et al., 2005; Mattson, 2010). Although scientific evidence of the positive effects of gardening on blood pressure, body temperature, brain activity, immune system response and psychological sphere are only recent (Coleman and Mattson, 1995; Liu et al., 2004; Park et al., 2004; Sugimoto et al., 2006; Gonzalez et al., 2009; Park and Mattson, 2009; Kam and Siu, 2010), the intuitions about the beneficial effects of horticulture on human health are much older. More than 2,000 years ago the Chinese Taoists built gardens in the belief that the environment had beneficial effects on health. In Europe, therapeutic activities related to horticulture have been documented as beneficial already in the seventeenth century in Spanish psychiatric hospitals, whilst in America Benjamin Rush mentioned the practice of horticulture and gardening as a remedy for anxiety or phobic disorders or, more generally, against depression, in the eighteenth century (Smith, 1998). Nowadays, all over the world, horticulture is a consolidated and recognized practice for the treatment of a wide range of disorders in therapeutic programs. Furthermore, it is integrated in the aims and fields of activities of numerous associations such as the American Horticultural Therapy Association founded in 1973 (www.ahta.org), the Thrive founded in 1978 in England (www.thrive.org.uk), the Canadian Horticultural Therapy Association founded in 1987 (www.chta.ca), the Japanese Horticultural Therapy Society founded in 1996 (www.jhts.jp), the German Association for Horticulture and Therapy founded in 2001 (www.ggut.org), the Horticultural Therapy Swiss association established in 2004 (www.horticulturaltherapy.ch), and the Therapeutic Horticulture Australia (www.tha.org.au).
Social Inclusion and Justice
Within the sustainability rhetoric of UA (Tornaghi, 2014), social inclusion and justice have been commonly linked to UA initiatives. As a matter of fact, some initiatives have started as a reaction to urban policies, the marginality of neighborhoods or to economic crises (Anguelovski, 2013; Camps-Calvet et al., 2015; Gasperi et al., 2016; Reynolds and Cohen, 2016; Calvet-Mir and March, 2017). Accordingly, the regeneration of unused urban spaces and the creation of community networks to manage and access food production resources are seen as an opposition to the capitalistic framework of conventional food production (McClintock, 2010). Gardens can be a place where “collective efficacy” flourishes (Teig et al., 2009), where citizens can create community and empower themselves toward conflicts resolution and rights claiming. Such characteristics support the UA contribution to community improvement, including social inclusion and empowerment (Armstrong, 2000; Saldivar-Tanaka and Krasny, 2004; Wakefield et al., 2007; Teig et al., 2009; Taylor and Taylor Lovell, 2014; Camps-Calvet et al., 2015).
Notwithstanding the potential social benefits, society's impact mainly relies on the typology of initiative, as already reported for rooftop agriculture (Specht et al., 2017). Sanyé-Mengual et al. (2019) observed that socially innovative UA activities contributed to a larger diversity of social benefits than technologically-innovative ones. UA grassroots initiatives (e.g., community gardens, squatting gardening) commonly focus on enhancing social inclusion and justice, such as improving food access for low-income citizens, thereby creating socially inclusive spaces. On the contrary, within commercial initiatives, economic profit stands as main driver, while specific social aspects are usually sidelined (Poulsen, 2017).
Nonetheless, UA initiatives can also generate negative social impacts. Some bottom-up initiatives claiming social inclusion and justice have become places of injustice and exclusion, where elites can displace low-income and culturally-diverse citizens (Anguelovski, 2015). Access to UA programs can be imbalanced, accentuating social exclusion and injustice among the community instead of closing the gap between citizens with different economic and cultural backgrounds (Cohen and Reynolds, 2015; Reynolds and Cohen, 2016). On the other hand, when UA experiences are implemented by local governments in a purely top-down process without including the citizens through participatory approaches, ineffective and unsuccessful projects can appear, negatively affecting the local community (Gasperi et al., 2016). As an emerging new urban space typology, urban gardens can also ultimately contribute to urban green gentrification, as observed in Barcelona (Anguelovski et al., 2018).
Ecological Aspects
UA is strongly related to some regulating and habitat ES. Genetic agro-biodiversity is strictly linked to food security (Thrupp, 2000; Frison et al., 2011). In a survey on UA projects in 10 European countries (Pölling et al., 2016a), it was observed that about half of the considered cases promoted biodiversity preservation, by cultivating more than thirty crop types and varieties. Alternatively, limited biodiversity was only observed within intensive monocultural farms, including vine growers or greenhouses. UA also intrinsically foster biodiversity: more than 1,000 plant species were recorded in 267 private gardens in London (Loram et al., 2008) and 440 different species have been found in a single 400 m2 allotment garden in Stockholm (Colding et al., 2006). Small and widely diversified urban crop systems also increase the vegetative complexity of the cities and can have positive effects on animal biodiversity, providing suitable habitats for the microbiological fauna (Tresch et al., 2019), invertebrates (Halaj et al., 2000; Sperling and Lortie, 2010), birds (Andersson et al., 2007), and mammals (Baker and Harris, 2007).
UA systems can have a relevant impact on the provision of arthropod mediated regulating ES, such as natural pest control and pollination (Shrewsbury and Leather, 2012; Lin et al., 2015; Bazzocchi, 2020). Allotment gardens often exhibit a rich abundance of flowering plants, supporting urban pollinators for long periods: at least a quarter of all known bee species in Vienna are hosted by communal gardens (Lanner et al., 2019) and 54 species (13% of the recorded New York State bee fauna) were found in few community gardens located in heavily developed neighborhoods in New York City (Matteson et al., 2008). Importance of increased floral resource availability and plant structural diversity of urban agro-systems has been demonstrated to maintain and promote presence of ladybugs (Bazzocchi et al., 2017), main agents of natural pest control.
In addition to habitat quality, habitat connectivity is a key factor. Some studies suggest that proximity to natural habitat can increase bees abundance and pollination success for a wide range of crop species (Ricketts et al., 2008). Agro-ecological corridors, exploiting a network of small, natural habitat fragments and cultivated patches across urban areas may affect the ability of beneficial insects (both pollinators and pest natural enemies) to persist in the urban landscape (Breuste et al., 2008; Burkman and Gardiner, 2014; Bazzocchi, 2020). On the other hand, potential disservices might come from UA and must be considered. Intensive UA, which can be characterized by pesticide application, extensive pruning, and frequent mowing, would presumably have a negative impact on biodiversity and ES. Moreover, not all biodiversity is necessarily “desired”: some pests and pathogens are polyphagous and can benefit from vegetational diversification, and potentially dangerous mosquitos can proliferate due to the presence of stagnant water for irrigation (Winkler et al., 2010).
Economic and Environmental Sustainability
In recent years, research studies aimed at evaluating the environmental impact of UA initiatives have flourished, with a number of reference studies being released, with a main focus on Mediterranean Europe, including Spain (e.g., Sanyé-Mengual et al., 2015a) and Italy (e.g., Sanyé-Mengual et al., 2015b), also thanks to associated European (e.g., SustUrbanFoods, FewMeter, UrbanGreenTrain, and FoodE projects) and National (e.g., FertileCity project) fundings on the topic. Literature on impact assessment of UA systems often refer to innovative solutions and technologies, including aquaponics (e.g., Forchino et al., 2018), mushroom cultivation (Aubry and Daniel, 2017), rooftop farms and greenhouses (Sanyé-Mengual et al., 2015a,b; Grard et al., 2018; Sanjuan-Delmás et al., 2018), indoor and vertical farms (Liaros et al., 2016; Martin and Molin, 2019; Martin et al., 2019; Pennisi et al., 2019a,b), or resource use efficiency including energy (Nadal et al., 2017), organic waste (Dorr et al., 2017), or water (Opher et al., 2018). Nevertheless, applied studies on more traditional systems also exists, as for the case of urban gardens in Italy (Sanyé-Mengual et al., 2018a), USA (Algert et al., 2014) and Canada (CoDyre et al., 2015), or periurban commercial farms in The Netherlands (Benis and Ferrão, 2018). Besides evaluating existing strategies, some of these studies also indicate alternative management scenarios, including crop input and management, resource origin and management structures (Sanyé-Mengual et al., 2015b; Martin and Molin, 2019; Pennisi et al., 2019c). Nevertheless, each study's peculiarities and uniqueness (which relate to both the UA project itself and the specificities of the city where it takes place), often limit the possibility to drive general conclusions and implement widely applicable policy tools (Sanyé-Mengual et al., 2019). Indeed, by combining the available body of evidences, it emerges that environmental sustainability shall be specifically targeted by a series of actions, which can be summarized as follows:
- Cradle-To-Grave studies are very limited in number. Mostly, available literature concentrated to certain production stages and often excluded initial investments or infrastructural elements. More comprehensive research is needed to compare sustainability among urban and rural agriculture, with adequate emphasis on all stages associated with food production, transformation and distribution (Sanyé-Mengual et al., 2013). Such life cycle approach has been highlighted in the recently published Farm to Fork Strategy (EC, 2020).
- Available evidence is limited to specific case studies, often experimental or small-scale, and geographical areas, preventing the existing literature's capacity to draft conclusions on the actual contribution of UA in sustainability terms (Sanyé-Mengual et al., 2018c). Such aspect limits the capacity to define how UA can be framed in policy-making.
- The economic sphere and sustainability of newly born UA projects in the Global North still needs to be confirmed. Further studies should specifically target the identification of economic viability of innovative experiences, also through adequate valorisation of the ecosystem and environmental services they supply to the urban fabric (Sanyé-Mengual et al., 2018b). On the other hand, in order to elaborate viable strategies for UA in the Global North it becomes crucial that labor costs are included in the overall economic balance even when information are limited (Love et al., 2015) or associated costs could pose a risk on the financial viability of the experience (Algert et al., 2014).
- Cross sectorial studies are needed. Impact assessment needs to be comprehensive and shall not disregard any element within the three sustainability spheres (economic, environmental and social). Integrated tools—e.g., combining Life Cycle Analysis (LCA) with Life Cycle Costing (LCC) or Social Life Cycle Analysis (S-LCA)—are needed in order to compile a comprehensive and reliable vision on UA experiences. Economic analysis should also make use of financial tools including determination of Net Present Value (NPV), Internal Rate of Return (IRR) and Payback Period (PP). S-LCA may allow to better identify which variables—especially when economic figures associated to mere food production and marketing are non-sufficient to ensure viability—should also be accounted for in the evaluation of services provided by UA experiences (Sanyé-Mengual et al., 2018b).
- Environmental studies may provide functional tools to UA entrepreneurs and local policy makers through the creation of alternative scenarios (Martin and Molin, 2019). They allow to identify how local resources availability (including raw materials, energy or labor) may affect a certain project's sustainability, or how alternative management strategies may result in avoided impact. Overall, scenarios allow to widen the applicability of the study and offer adequate policy tools.
Factors That Affect Development and Diffusion of UA
Growth and diffusion of UA may be hindered by factors that range from regulatory frameworks, access to land and its potential contamination, the local climatic conditions and resource availability. Each of these hindering factors will be targeted and discussed in the following sub-sections.
Laws and Regulations
Western World cities are densely populated and Europe is one of the most densely urbanized continents in the World, where, between 2012 and 2018, 539 km2 of land is yearly transformed into housing, industries, roads or recreational uses (EEA, 2019). At the same time, the amount of green space per city dweller for many European cities remains below the minimum standard suggested by the World Health Organization (EEA, 2012). The percentage of green space in the cities of EU varies from 3 to 4 m2 per person (Reggio Calabria, Italy) to more than 300 m2 per person (e.g., Liège, Belgium, Oulu, Finland, and Valenciennes, France) (Fuller and Gaston, 2009). In this context, within the EU, strategies that aim at reusing and regenerating the so-called vacant land (Gasperi et al., 2016) have become key elements for territorial development and urban planning (EEA, 2015). They foster the sustainable use of the land by providing green habitats and peaceful places to promote respect for urban heritage (EC, 2010). These policies are geared toward a more sustainable and efficient use of resources, recognizing that land is a limited and declining resource, subject to competing pressures from urbanization, infrastructure, increased food, fiber and fuel production and supply of key ES (Gasperi et al., 2016).
While policies tend to promote green spaces and UA in the city carried out for ecological-environmental, aesthetic-recreational, and social-educational purposes (urban farming as a tool for social inclusion, intercultural dialogue and job creation such as in Bologna, Oslo, Barcelona or Paris), the same cannot be said for UA oriented toward food production. Apparently, while cross-sectoral innovation blooms, taking the form of new technologies (e.g., vertical farms, rooftop greenhouses), production and management models (e.g., community-based agriculture) or supply chain (e.g., solidarity buying groups, farmers markets), the integration of UA within the food system is slowed down by the lack of National and European policies and strategic frameworks (Fox-Kämper et al., 2018). Meanwhile, food consumption becomes accounted for as main driver of EU citizens' environmental impact (Sala et al., 2019), and, within the EU Green Deal, the improvement of the sustainability of the food system paves the way for the upcoming Farm to Fork strategy (EC, 2020), where UA may find appropriate ground for evolution.
Notwithstanding that more than 200 World Cities signed the Milan Food Policy Pact in 2015 (Filippini et al., 2019), a general and consistent uptake at global level is still lacking. A legislative and regulatory environment enabling to ease the establishment and management of small-scale and citizen-driven UA initiatives is needed, overarching the economic, environmental and social functions that sustainable food systems may play. Policies often underestimate the ES associated with multifunctional UA, overall resulting in a limited support to initiatives that in turn, as previously described, would provide climate change prevention and resilience, job creation and social inclusion. Furthermore, given that UA initiatives' long-term success is hindered by both the lack of training and the difficulties in formal community engagement (Ochoa et al., 2019), appropriate policies for awareness creation should be fostered. Accordingly, in recent years, some municipalities have integrated support policies for UA, as in the case of Paris (Colle et al., 2017) or Barcelona (Giacchè et al., 2016) as further described in the coming sections.
Land Access
Today, in developed countries about 20% of the global irrigated cropland is located in cities or within 20 km from the urban centers, where also 44% of the global rainfed agriculture occurs (Thebo et al., 2014). In urban settings, cropping intensity (expressing the amount of crops cultivated within a year in a specific plot) was also found to be higher in urban areas (1.48) (Thebo et al., 2014) than in rural areas (1.12) (Portmann et al., 2010), suggesting more intense rotations in the former, a phenomenon that has also been associated with higher population density (Ellis et al., 2013). In general, agricultural activities' success is correlated with the farm size (Hansson, 2007), given the reduced costs of production that can be achieved when economy of scale can take place (Bertoni and Cavicchioli, 2016). In cities, however, a major constraint limiting the development of UA projects is associated with land access, given that generally any other activity has a greater and faster return of investment (Wästfelt and Zhang, 2016), and the fact that UA still struggles to have a recognized economic role (Specht et al., 2016). Consistently, in USA, grassroot UA initiatives were shown to occur in districts with highest land value, a phenomenon that was linked with smaller plot size (Rogus and Dimitri, 2015) and therefore limited income perspectives (Centrone Stefani et al., 2018).
On the other hand, as cities grow, the phenomenon of urban sprawl is generally observed, with periurban areas characterized by dispersed, scarcely planned and low-density settings (Jaeger et al., 2010), where several unused or empty lots are usually found and named as vacant lands (Gasperi et al., 2016). In response to the economic and financial crisis started in 2007 (Heath, 2001), further voids in the urban fabric have emerged, including abandoned industrial districts, but also public buildings (e.g., dismissed army barracks, hospitals and schools) (Frumkin, 2003). In many cities, UA explores potential new uses for these vacant lands (Gasperi et al., 2016), by colonizing brownfields, empty rooftops or taking place within abandoned buildings. Municipal plans that foster urban regeneration through UA are also issued, providing land use rights to urban gardeners as for the case of the Green Thumb agency in New York (USA) (Smith and Kurtz, 2003), the Pla Buits in Barcelona (Spain) (Giacchè et al., 2016) or the Parisculteurs initiative in Paris (France) (Colle et al., 2017). Although UA projects are consequently sprouting in marginal urban lands, their long-term sustainability is posed at risk by factors that include small plot size (Ernwein, 2014), limited time concessions for land-use (Tornaghi, 2012), potential contamination risks (Vittori Antisari et al., 2015), space accessibility (Tornaghi, 2017) and distance from potential consumers (Ancion et al., 2019).
Environmental Contamination
When plants are grown within cities' highly anthropogenic environment, questions may arise on the potential contamination risks associated (Hursthouse and Leitão, 2016). While most commonly experienced contaminants are heavy metals or metalloids (B, As, Cd, Cr, Cu, Mo, Mn, Pb, Zn, Hg), a growing concern is also linked to specific localized components (e.g., selenium, or radioactive isotopes) and organic compounds (e.g., polycyclic aromatic hydrocarbons, poly-chloro-biphenyls, pesticides, dioxins and furans) (Megson et al., 2011; Mitchell et al., 2014). In the last couple of decades, the comprehension of how contamination originates and the possible strategies to tackle associated human health hazards was targeted by a relevant body of research activities (Table 3). It emerges that, within urban environments, contamination hazard generally varies according to the location where horticulture takes place, being higher in proximity of pollution sources (e.g., main roads, or within former industrial districts) (Hursthouse and Leitão, 2016) or as a consequence of the background geology of the site (Jean-Soro et al., 2014). Heavy metal deposition from nearby roads was however shown to decrease through distancing (e.g., by 25 m, Reinirkens, 1996), elevation (e.g., in rooftop gardens) or inclusion of vegetated barriers (Vittori Antisari et al., 2015). Conduction of accurate soil analyses and quantification of hazard risk (e.g., through application of contamination indexes) is generally recommended before starting the cultivation (Hough et al., 2004). Whenever contamination risk is confirmed, however, strategies may be set in place in order to limit the hazard, including integration of soil amendments or adoption of agronomic practices to reduce plant uptake of the contaminants (Table 3). Whenever UA takes place in potentially contaminated sites, the integration of peat or potting soil may also be an option to overtake contamination (Pennisi et al., 2016, 2017), although at the expenses of increased associated environmental impacts (Dahlin et al., 2019), which on a large-scale could pose at risk the overall sustainability of UA (Meharg, 2016). Alternatives to commercial/potting soils should therefore be considered, these including among others composted urban waste (Shrestha et al., 2020), spent coffee grounds (Cervera-Mata et al., 2019) or biochar (Song et al., 2020), assuming they do not contain further contaminants and are suitable for plant cultivation (Beniston and Lal, 2012; Hardgrove and Livesley, 2016).
Climatic Conditions
UA is playing a crucial function on the city environmental sustainability. Based on Koppel's climate classification, world global north areas are mainly located within two main climate groups (temperate, C, and continental, D) based on seasonal precipitation and temperature patterns. The temperate climate (C) is characterized by coldest month averaging temperature between 0 and 18°C, with at least 1 month averaging above 10°C. Continental (D) climate displays at least 1 month averaging below 0°C and at least 1 month averaging higher than 10°C (Kottek et al., 2006). Most updated climate models foresee that extreme heatwaves will become more frequent and more intensive also in the Global North due to climate change (Huttner et al., 2009). The development of green spaces and infrastructures reduces urban heat island effects, mitigates rainwater impacts, and improves urban climatic metabolism (Ackerman et al., 2014). Indeed, functionality of green infrastructures is highly connected to plant vegetation status and management. A study carried out in Freiburg (Germany) (Huttner et al., 2009) reported that when natural soils in the urban and periurban areas are not covered with vegetation or not wetted by irrigation or rainfall during heatwaves, their effects on the microclimate are comparable to those from asphalted roads, leading to higher radiative temperatures.
Urban climate is also affected by the city size and the population density. Urban environments in the Global North are today experiencing growing population trends. In Europe, more than 70% of the population is living in urban areas today, and according to updated predictions, this number is likely to increase above 80% by 2050 (Kabisch and Haase, 2011). Intensified urbanization combined with the highest frequency of heavy rainfall are the leading causes of amplified peak flows and increased flood risk in the cities worldwide (Chang and Franczyk, 2008). Major cities in Europe are characterized by elevated (50–75% or even higher) fractions of impervious surfaces (such as roads, buildings, parking lots etc.), which translate into reduced water drainage and elevated risk of pluvial flooding (Du et al., 2015). Urban vegetation and well-planned UA spaces (including green roofs and parks) can significantly improve stormwater management decreasing the impact on the surface of heavy rain and providing run-off regulation and cooling through evapotranspiration (Orsini et al., 2014; Langemeyer and Latkowska, 2016).
Urban Resources
UA may substantially contribute to fostering sustainable resource use within the city metabolism, particularly with reference to water, mineral nutrients and energy fluxes. In cities, water for irrigation generally comes from municipal supply systems, leading to environmental and agronomic concerns (Wortman and Lovell, 2013). Indeed, alternative sources may include both rainwater harvesting and greywater or wastewater regeneration. In a GIS-based study it was recently estimated that if rainfall would be harvested from rooftops of nearby buildings and conveyed toward vegetable gardens in Rome, water saving could be in measure of 20 to 40% (Lupia et al., 2017). Theoretical scenarios of implementing rooftop greenhouses on retail parks roofs in different world cities (including Barcelona, Lisbon, Utrecht, and Rotterdam) indicated that crop water requirements could be satisfied by rainwater harvesting from the greenhouse roof (Sanyé-Mengual et al., 2018d).
The use of regenerated greywater may also significantly reduce the water footprint of UA, although concerns associated with pH or salinity may arise (Li F. et al., 2009), altogether with non-balanced mineral contents or microbiological load (Hanjra et al., 2012). With reference to the mineral nutrition of crops, the integration of composting of the organic waste and pruning residues was shown to markedly contribute to the urban horticultural production, although sanitary aspects shall be carefully considered in order to avoid risks associated with both microbiological load and heavy metal contamination (Brown and Jameton, 2000). Studies have demonstrated the viability of employing urban compost in substrate mixtures for UA (Grard et al., 2018). Moreover, as ambient CO2 concentrations can be up to 80 ppm greater in urban areas relative to adjacent rural areas due to the combustion of fossil fuels, increased photosynthetic rates may also be experienced in UA (Ziska and Bunce, 2007). With reference to the urban energy balance, the distribution and presence of green infrastructures throughout the urban fabric may significantly reduce the so-called urban heat island effect, both resulting in improved city liveability and reduced heat associated mortality during warmer seasons (Qiu et al., 2013). On a smaller scale, when building-integrated agriculture takes place (e.g., in rooftop greenhouses), an integrated building metabolism was shown to improve water, energy and carbon fluxes, while also supplying a range of ES (Piezer et al., 2019).
Main Typologies of UA Systems
In recent years, also in northern global areas, UA has been considered a strategy to contribute to food security and city environmental sustainability (Taylor and Taylor Lovell, 2014). Within the coming sections, established typologies of UA systems popular in Global North are described (Table 4), mainly focussing on allotment gardens, extensive periurban farms and community gardens. Furthermore, this section will introduce some innovative growing systems specifically developed for the urban environment. These range from building-integrated agriculture systems, taking place on building rooftops (e.g., rooftop gardens and greenhouses) or even inside them (e.g., indoor or vertical farms with artificial lighting). Furthermore, the section also explores new crops that are increasingly adopted in UA, thanks to the market opportunities provided by the urban environment and the proximity to consumers.
Allotment Gardens
Private and public urban gardens for vegetables production are widespread all over the world. Historically, allotment gardens were set up with the primary goal to mitigate poverty by providing fresh food among factory workers during the industrial revolution or later during wars and depression times (Barthel et al., 2013). Their relevance was dramatically increased during the first half of the twentieth century, during the two World Wars, when agricultural products could not easily reach the city markets and were sold at elevated prices or on the black market. Consequently, foodstuffs' production, especially fruit and vegetables, became essential for the survival of cities' inhabitants. Urban gardening was then considered as a patriotic act, enabling to feed citizen and the army, while governmental propaganda called for action in the so-called “war gardens” or “victory gardens” (Miller, 2003; Lawson, 2004). As a result, in those years, the number of vegetable gardens rose dramatically in almost all cities touched by the war where not only family and urban gardens but also public parks and roadways' edges were cultivated. During the conflict, areas destroyed by bombing were also used for growing crops. After the war, reconstruction activities began: jobs, industries, cities were growing, the price of building land dramatically raised and the phenomenon of allotments gardens significantly decreased. But the gardens did not disappear; they moved from the city center to the suburbs and frequently reappeared as squatting. They were commonly associated with the concurrent migration process experienced from the rural areas to the city's outer skirts (Tei et al., 2009). Then, since the 1980s, a “renaissance” of UA has been noticed. Urban gardens originally aimed at ensuring food security evolved, addressing other key roles (ecological-environmental, aesthetic-recreational, social-educational, therapeutic) in relation to the changed economic and socio-cultural conditions (Crouch, 2000; Wells, 2000; Hynes and Howe, 2004; Tei et al., 2009; Meneghello et al., 2016; Righetto et al., 2016).
During the last 50 years, the local municipalities promoted the establishment of urban gardens by providing the land, establishing a water system, and eventually fencing the area. In most cases, urban allotment gardens are organized in associations or committees for decision making (Bell et al., 2016). Sometimes, they also appear to be integrated within urban agricultural parks, as for the cities of Rome and Barcelona (Colantoni et al., 2017). Allotment gardeners are generally requested to pay a small rent for the plot and attend specific association duties. Production is intended exclusively for self-consumption or limited to donation, as in most cases the sale is not allowed by municipal regulations (Barthel et al., 2013). Today food production is not anymore the only primary purpose but also other functionalities are acknowledged, including aesthetic-recreational and educational (Wells, 2000), social (Tei et al., 2009), or therapeutic (Crouch, 2000). Also in Italy these types of gardens have evolved in their form in the last decades (La Malfa et al., 2009), mainly under the framework of the Italian association for recreation, culture and gardens (Associazione Nazionale dei Centri Sociali Ricreativi Culturali ed Orti—ANCeSCAO), that provides gardeners with administrative and insurance support (Gasperi et al., 2012) and today accounts for more than 360,000 members, and manages 1,400 social centers and 22,000 vegetable gardens. Similar organizations are found in Germany such us Kleingaerten and Schrebergaerten (Drescher, 2001), Real Food Wythenshawe in UK (Bell et al., 2016), Gezonde Gronden in The Netherlands (van der Schans, 2010), Pispala allotment in Finland (Bell et al., 2016), and ROD Obroncow Pokoju in Poland (Bell et al., 2016) and have proven to be a useful means for learning democratic rules as well (Gasperi et al., 2012).
Extensive Periurban Farms
In recent years, the relevance of urban and periurban farming in terms of food production in cities and their contribution to food security has been a matter of extensive research. Whether or not to include periurban farms as a part of UA has been discussed in several ways, with most authors suggesting their inclusion and adopting the more general definition of Urban and Periurban Agriculture (UPA) (van der Schans and Wiskerke, 2012; Mok et al., 2014) or metropolitan agriculture (Heimlich, 1989), both synonyms of the more general concept of UA adopted in the present manuscript. While UA's primary purpose is still meeting food needs mainly at the household level (Petts, 2005), extensive periurban agriculture can provide more substantial quantities and has broader distribution pathways, allowing for significant contributions in terms of food supply at city level in the Global North. Extensive periurban agriculture farms nowadays provide goods and services both for the local and global markets (Opitz et al., 2016). These farms emerge within the “transition area” between urban and rural environments, characterized by lower population density with lesser infrastructures and buildings, whilst concurrently featuring a more limited land availability for agriculture use as compared to rural areas (Allen, 2003; Piorr et al., 2011).
In extensive periurban farms, multifunctionality at the farm level appears, with farms providing not only agricultural goods and food, but also services to the community as well as public functionalities (Le and Dung, 2018). Representative case studies of periurban farms are found within metropolitan areas in several cities of the Global North. In Bologna (Italy), Spazio Battirame (https://www.etabeta.coop/spaziobattirame/), is a place of socio-recreational and educational activities created and developed as an urban regeneration project by the social cooperative Eta Beta (Gasperi et al., 2016). In Spazio Battirame, cultural events, handcraft laboratories and concerts are organized, while organic vegetables are produced over 4 hectares of open-fields and marketed through solidarity buying groups and participation in weekly farmer's markets. A professional kitchen serves a bar-restaurant and hosts cooking courses and food-related activities. The project has a strong social connotation, and functions include inclusive job creation, education and training, urban regeneration and sustainable growth (Cavallo and Rainieri, 2018). In the fringes of the city of Angers (France), proximity agriculture takes place at Le jardin de l'avenir (The future garden, https://www.jardindelavenir.fr/). The farm, extended over almost 9 hectares, operates on a pick-your-own scheme, where local residents may access the farm and harvest fruits and vegetables based on their needs and then weight and pay them at the counter. The farm is managed following principles of organic farming and permaculture, while environmental sustainability is also targeted through the co-generation of electricity for the farm needs and the local energy supplier. A similar scheme is adopted by the farm Hof Mertin (Germany), placed in the densely urbanized and industrial region of the Ruhr (Pölling et al., 2017). The farm (https://hof-mertin.de/) extends over around 120 hectares, out of which 40 are devoted to strawberry cultivation and integrated in a pick-your-own scheme or used for educational or socio-recreational workshops. In Ontario (Canada), a survey involving 21 periurban farmers highlighted that, while proximity to city may open up new marketing opportunities, the overall sustainability of the sector strongly depends on the existence of infrastructural and policy measures to link UPA with the local market (Akimowicz et al., 2016). It appears that while periurban farmers may benefit from the nearly rural conditions of their environment, a set of diversified and adaptive strategies must be integrated in order to attract local citizens and involve them into alternative food networks, as also evidenced in a recent study in the city of Barcelona (Spain) (Paül and McKenzie, 2013).
Urban Community Gardens
The term “community garden” refers to “open spaces which are managed and operated by members of the local community where food or flowers are cultivated” (Holland, 2004; Pudup, 2008). Nowadays, community gardens are growing in popularity in response to the shift toward cooperative forms of spatial design and land-use, and reflect the shift from government to governance including changing roles, responsibilities and impact of government agencies and local citizens (Rosol, 2010). They can involve a wide range of groups such as schools, prisons, youth, the elderly, hospitals, and neighborhood residents (Pudup, 2008; Teig et al., 2009). Different studies emphasized that community gardens are not only a source of food but provide other benefits, such as community building, education, and promoting health (Turner, 2011). Indeed, the most common motivation to take part of a community garden by citizens are: to consume fresh foods, social development or cohesion such as community building and culture exchange, to improve health among members and to make or save money by eating from the garden or selling the produce (Guitart et al., 2012). It was recently estimated that 86% of community gardens in USA were used to grow food, flowers and native vegetation. The same study also revealed that 82% of community gardens were operated by non-profit organizations, including cultural and neighborhood groups (Guitart et al., 2012). Further research studies confirm that community garden members are rather heterogeneous in terms of education, age, gender and financial aspect and usually lack previous gardening experience (Bell et al., 2016).
Community gardens can also be classified based on their own government structures (Fox-Kämper et al., 2018). Nettle (2016) observed that community gardens can be classified as either top-down or bottom-up governance structures depending on who initiated them. McGlone et al. (1999) noted the difference between gardens that were managed by external professionals (top-down) and those that were managed by community members including professionals (bottom-up). Top-down community gardens are implemented with the help of enabling legislation passed by local or central government (Nettle, 2016) and external/private officials carry out the management of the garden in order to meet government-set outcomes (McGlone et al., 1999). On the other hand, bottom-up community gardens build upon a direct involvement of the local community. Indeed, in the latter case, the community garden is planned and devised through collaboration by community groups (Okvat and Zautra, 2014) as well as the implementation with the help of enabling legislation passed by local or central governments (Nettle, 2016). The community also collaborates to devise a management scheme for the garden (McGlone et al., 1999). Among the most famous cases of community gardening in the Global North may be found several initiatives in the city of Berlin (Germany), including Allmende Kontor (https://www.allmende-kontor.de/) in the former Tempelhof airport, Ton Steine Garten (http://gaerten-am-mariannenplatz.blogspot.com/) in the Kreuzberg area or experiences of community entrepreneurship found in both Prinzessinengarten (https://prinzessinnengarten.net/) and Himmelbeet (https://himmelbeet.de/) (Wunder, 2013; Bradley and Hedrén, 2014).
Rooftop Farms
Within cities, plant cultivation on the rooftops of buildings has been recently gaining global attention (Orsini et al., 2017). It may take place both in protected (rooftop greenhouse) and non-protected (open-air rooftop farm) conditions (Sanyé-Mengual et al., 2015b). Among growing technologies, soil-based (e.g., soil-filled containers) or soil-less (e.g., hydroponics, aquaponics) systems are commonly adopted (Nasr et al., 2017). Due to the peculiar specificities of the environment where it takes place, rooftop agriculture may be constrained by the rooftop structural loading capacity, its accessibility to people and to agricultural input and tools, and the elevate solar radiation and temperature ranges (Caputo et al., 2017). On the other hand, benefits may be associated with rooftop gardening, including potential energy saving up to 15% thanks to the thermal insulation provided by the green cover (Wong et al., 2003). Besides, when a rooftop greenhouse is present, further advantages may be associated with its integration within the building metabolism (e.g., in terms of energetic fluxes and both water and carbon recirculation) (Sanyé-Mengual et al., 2015b).
The majority of rooftop agriculture projects is represented by open-air rooftop farms or gardens that use low-tech systems such as raised beds filled with soil (Thomaier et al., 2015). While the absence of physical barriers may be associated with higher exposure to the atmospheric pollutants' deposition, the higher elevation and the adoption of hydroponics were shown to generally limit the contamination risk in rooftop farms (see section Environmental Contamination, Vittori Antisari et al., 2015). On the other hand, rooftop greenhouses are generally associated with sophisticated technologies, targeting both increased production capacity and resource use efficiency (Sanyé-Mengual et al., 2015b). While open-air rooftop agriculture is widely adopted in the Global South, high tech commercial rooftop farms generally take place in North America, Asia and Europe in the form of business-oriented start-ups with economic profitability as the first aim (Specht et al., 2015). However, rooftop farms are also often associated with non-profit aims, e.g., the amelioration of urban living quality or communities' involvement in social, recreational and educational activities (Thomaier et al., 2015). Furthermore, rooftop cultivation can also represent a marketing action for hotels and restaurants, which offering fresh and self-produced products to customers can ameliorate their image and gain preference among the public (Thomaier et al., 2015).
Rooftop farms may either be located in existing buildings or integrated in new constructions (Buehler and Junge, 2016). In the former case, higher costs for refitting, less rational use of the rooftop space and a limited range of applicable cultivating techniques are commonly experienced (Caputo et al., 2017). Nevertheless, due to a slow uptake of rooftop farming technologies among estate operators and building companies, adaptation of existing buildings still represents the majority of rooftop farming projects (Thomaier et al., 2015).
Vertical Farms With Artificial Lighting
One of the most technologically oriented growing solution for cities is the use of plant factories with artificial lighting (PFALs). PFALs are cultivation systems where the environmental factors (e.g., air temperature and humidity, light, CO2 concentration) are controlled by minimizing exchanges between indoor and outdoor environments, thanks to the adoption of insulated cultivation room where a minimum amount of air and heat is exchanged with the outside (Kozai and Niu, 2016). The enclosed system also enables the farm to achieve resilience to extreme events and easier control of pest and pathogens as compared to more traditional cultivation systems (Kozai, 2019). Nevertheless, it has also been suggested that when pest outbreaks take place in PFALs, their impact may be dramatic, due to the combined effects of the relative proximity of plants (both in vertical and horizontal dimensions), as well as the intense air circulation fluxes needed to guarantee environmental uniformity (Roberts et al., 2020).
Among the advantages of PFALs, one extremely relevant in the urban environment is the reduced pressure on land, obtained by exploring the vertical direction through multilayers cultivation systems fed by artificial lighting devices (Beacham et al., 2019). Besides, the benefits associated with hydroponics and possible de-humidification and water recovery from the internal atmosphere allow for elevated water use efficiency (Pennisi et al., 2020a), in the range of 30–50-folds the measured values in greenhouses and open-field cultivation (Kozai, 2013). On the other hand, the elevated energetic requirements (mainly due to electricity consumption associated with artificial lighting, Paucek et al., 2020) are still hindering the large-scale applicability of these systems (Kozai, 2019). Indeed, while technology is rapidly evolving, strategies for reducing the environmental burdens associated with PFALs are also being identified (Son et al., 2016; Martin and Molin, 2019; Pennisi et al., 2019c, 2020c; Orsini et al., 2020) and will likely foster the large-scale application of these technologies in the near future. To date, the most common plant species grown in PFALs are leafy vegetables (e.g., lettuce, basil, microgreens), medicinal plants (e.g., cannabis or other crops used in the preparation of pharmaceutical, herbal or cosmetic products), small fruit (e.g., berries), edible flowers and seedlings (e.g., grafted vegetable) (Kozai, 2013).
Beyond Vegetables: Alternative Farm Products in Urban Environments
UA takes many forms and involves a diversity of actors and products. Among new forms of UA, microgreens cultivation is gaining relevance and popularity in the Global North. Initiated in the early 80s in California, microgreens are tiny edible greens harvested just after the first set of true leaves, known for their high rate of antioxidants and micronutrients. Because of their limited space needs and their elevated water use efficiency (Durham, 2017), microgreens are well-adapted to UA and, as a matter of fact, many worldwide urban PFALs and vertical farms are dedicated to or include microgreens cultivation (Kozai, 2018; Butturini and Marcelis, 2020). Furthermore, their post-harvest storage requirements may be substantially reduced when they are grown in proximity to consumers, an important feature given their limited shelf-life (Durham, 2017). Alternatives to the conventional purchasing and large-scale retail trade are the sale to-order (e.g., Brooklyn Grange rooftop garden in New York City) and the “in-store farming” through modular automated incubators that can be placed in a variety of customer-facing city locations (Butturini and Marcelis, 2020). Homemade self-production is also greatly increasing. Similarly to microgreens, edible flowers are also gaining relevance in UA projects, where they find commercial uses thanks the growing interest from chefs and top-class restaurants, their elevated nutritional properties and their limited shelf-life (Mlcek and Rop, 2011).
Another growing strategy in UA is associated with food-forestry (crop and animal farming coupled with cultivation of woody perennial plants). Strategic combination of fruit- and nut- producing trees and herbaceous crops meets a multifunctional role of UA contributing to provisioning (food production) and regulating ES (carbon storage, runoff management, air quality improvement, soil erosion control, climate mitigation) (Clark and Nicholas, 2013). Urban food-forestry projects have been described for at least 37 cities in USA (Clark and Nicholas, 2013), 47 municipalities in Canada (Konijnendijk and Park, 2020) and a growing number of cities in Europe (Park et al., 2019). Related to urban forestry is the concept of urban foraging. The renewed interest for the harvesting of forest and rural edible wild species is also becoming popular in many worldwide urban contexts of developed countries (Shackleton et al., 2017; Konijnendijk and Park, 2020).
Aquaponics combines fish farming in smart water environment (aquaculture) and soilless plant production systems (hydroponics). The system is based on a closed water cycle in which fish dejections become inputs for plants development thanks to nitrifying bacteria's action, whereas plants act as filter to clean the water, which can be re-circulated back to the fish tank. Economic and ecological (primarily because the decrease in freshwater availability) sustainability of aquaponics is under investigation (Quagrainie et al., 2018). Interestingly, aquaponic systems can be combined with building-integrated wastewater management in cities (Steglich et al., 2020). An important pilot case is the Berlin Roof Water Farm (RWF) in which gray water, treated and mixed with rainwater, is used to irrigate the rooftop aquaponics system, and black water (rich in nutrients) is processed into a liquid fertilizer for UA purpose (Steglich et al., 2020).
A systemic and multifunctional approach is also at the base of the integration of microalgae production systems into innovative urban infrastructures. Production of microalgae as food for humans and feeds for animal and fish (e.g., Spirulina) or as energy-based biomass, can outperform other renewable resources with their potential to absorb CO2, recycle wastewater, and release O2 (Peruccio and Vrenna, 2019).
Urban bee keeping has been established in ancient times within the Mediterranean basin (Mavrofridis, 2015). Bees (both wild and reared) easily adapt to the urban environment thanks to the warmer temperatures, a wider variety of plants for pollination and foraging, and lower level of pesticide pollution in comparison with agricultural landscapes (Blum, 2017; Hall et al., 2017). Born as an activity linked to urban ecology and the decline of pollinators and honeybees' populations (Lebuhn et al., 2013), urban beekeeping has recently boomed in popularity. Beehives can be found in many cities of the Global North (e.g., New York City, London, Berlin), including in private (e.g., hotels) and public (e.g., operas) buildings, mainly motivated by the cultural and experimental interests of city dwellers (McCallum and Benjamin, 2012). Although, there is a substantial lack of quantitative data on the production and marketing of honey and other beehive products (e.g., wax, propolis, venom) coming from urban beekeeping, economic value of urban apiculture is rising, also in relation to educational and recreational side activities. Some concerns have however been raised on the possible negative effects that domesticated beekeeping could have on the native urban bee fauna, mainly related to competition for flora and disease spreading (Mallinger et al., 2017).
The UA Economic Dimension: Toward a Classification of Business Models (BMs)
The narrative of economic development in cities from the Global North has recently started to associate UA to key sustainability indicators. The definition of economic viability of UA experiences is however a complex and multifaceted exercise. Financial performances of UA are often benefitting from both external funding and availability of unpaid/voluntary workforce, which often follow alternative, non-capitalist economic logics, as recently analyzed in UA projects in Boston (Massachusetts, USA) (Biewener, 2016). Consistently, a comparative study integrating qualitative and quantitative data from self-harvesting, intercultural and community gardens in Germany revealed that participants are often more concerned on benefits than costs and that sharing and self-governance are predominant ambitions over economic viability (Krikser et al., 2019). Economic indicators and employment opportunities are also highly variable among UA projects (mainly due to the economy of scale and mechanization), as observed in Denver (Colorado, USA) (Fisher and Karunanithi, 2014). Nevertheless, and despite the potential role UA may play toward social and economic justice, policies and financial support often tend to concentrate on UA economic competitiveness, perceived as indicator of enduring and sustainable urban planning (Walker, 2016). Accordingly, and as UA assume growing economic relevance, several attempts have also been made to classify its emerging business models (BMs) (van der Schans, 2010; Liu, 2015; Pölling et al., 2016b; van der Schans et al., 2016). While some models are recurring within all existing literature (e.g., cost-reduction BM, differentiation BM, and diversification BM), emerging strategies are also being integrated as they become commonly adopted. This include the so-called innovative operations (Liu, 2015, now more commonly referred to as experimental BM), but also “the commons” (van der Schans et al., 2016, hereby referred to as share economy BM) and the experience BM (van der Schans et al., 2016).
In the present paper, reference will be made to the more recent classification of BMs in urban farming projects resulting from the EU project Urban Green Train (Urban Green Education for Enterprising Agricultural Innovation) (Magrefi et al., 2018) (Table 5).
Cost-Reduction BM
Cost-reduction BM includes farms that build their success on reducing costs associated with crop production. As for traditional agriculture, reducing costs and increasing profit through efficient economy of scale may also prove viable in urban environments (Zasada, 2011). For instance, it is the case of greenhouse farms in the periurban fringes that benefit from the increased market opportunities provided by the proximity of the consumers (Péron and Geoffriau, 2007). The economic viability of proximity farms may also benefit from in-farm shops, participation in farmers market, or integration in consumer delivery schemes, as for the cases of the so-called solidarity buying groups (Opitz et al., 2016), where direct delivery at distribution points within the city is practiced. In this last option, users often purchase a fixed amount of fruits and vegetables, whose composition will reflect the seasonal availability of locally produced goods (Vogl et al., 2003). Cost-reduction farms often evolve toward other business models in order to take benefit from the existing and multiple marketing offer (e.g., services associated with food distribution and marketing, as for the following BM categories) that the city can provide (Gasperi et al., 2016). Benefits associated with the proximity from consumers can be associated with reductions in requirements for transport, packaging (Sanyé-Mengual et al., 2013), as well as reducing food losses (Dimitri et al., 2016).
Diversification BM
Diversification BM includes farms that produce a diversified variety of products and services. There are two main categories of diversified urban farms, depending on their original core business. The first typology encompasses those cases where farmers may decide to integrate additional products and services to their main agricultural production. These may be urban peasants that integrate their food production and marketing with services (Dixon et al., 2007). Alternatively, there may be the case of the so-called “new farmers,” represented by entrepreneurs, private companies or non-for-profit bodies that have their core business in other sectors and start to explore agriculture in the urban settings. Under this category often fall socially involved institutions (including those providing job opportunities to disadvantaged users, Gasperi et al., 2016), which initially started in other sectors (e.g., handcrafting, catering) and more recently also engaged in agricultural activities thanks to the grown public awareness of sustainable food systems (Sanyé-Mengual et al., 2018b). A further classification is adopted to identify whether the diversification stands on a business-to-business (B2B) scheme (e.g., when electricity is produced through solar panels installed in the farm premises and energy is sold to the local energy supplier, Nelkin and Caplow, 2007, or when local compost plants that process urban bio-waste supply the organic matter that is then used for plant cultivation, Deelstra and Girardet, 2000), or on a business-to-consumer (B2C) scheme (e.g., when additional services are provided to the final users, including horse-riding farms, agro-tourism and educational farms, Pölling et al., 2017).
Differentiation BM
Differentiation BM includes farms that differentiate themselves from the competitors for the uniqueness of their specific product or production protocol. Urban farms that operate into the differentiation BM may concentrate on a specific niche product (e.g., an ancient tomato cultivar, van der Schans, 2010), or a special production factor specifically available in the city (e.g., rainwater collected from neighboring buildings and used for plant irrigation), a special strategy to target the consumer (e.g., pick-your-own fields, Vogl et al., 2003, or rent-a-field schemes, Pölling et al., 2016b) or determinate standards for food production (e.g., an organic or biodynamics certification scheme, Beauchesne and Bryant, 1999). Interestingly, when vertical integration is set in place (e.g., by implementing transparent, reliable and personal relationships between producers and consumers), differentiated farms may benefit from important market opportunities. These may reflect in more traditional B2C schemes, but also in B2B commercial agreements, where restaurants, canteens or food festivals are engaged in promoting locally-produced food (Pölling et al., 2016a).
Share-Economy BM
Share-economy BM includes collectively managed projects where the production risks are shared within a community. From an economic dimension, the share-economy BM entails for the highest level of innovation. They originate from the concept of “commons,” bringing together communities into collaborative efforts toward the achievement of a shared objective. In France known as AMAP (Tang et al., 2019), elsewhere generally referred to as Community Supported Agriculture (CSA) schemes (van der Schans et al., 2016), they generally originate and grow from grassroots experiences of groups of activists and environmentally concerned citizens. In these experiences, citizens move from consumer's concept and become so-called prosumers, capable of influencing the structure and overall sustainability of their food systems. A crucial element (that constitutes the main evolution from the previously described farmers markets or solidarity buying groups) stands in the recognition that agriculture plays a main functional role in the society and that responsibility of food systems sustainability shall be distributed. Accordingly, the production risk (which is also being exacerbated by price fluctuations in a global market and uncertainty of production in response to climate change) is distributed among the different food stakeholders rather than placed only on farmers (Pölling et al., 2016a). Beside CSA schemes, citizens are also engaging in collective actions in the Global North, including the establishment of so-called Food Policy Councils, where active citizenships results in modifying public procurement schemes (e.g., in schools and prisons), as occurred in the city of Berlin, where the establishment of the local Ernahrungsrat (Food Policy Council) in 2015, significantly contributed to the creation of a food strategy and a dedicated municipal office (Berlin Isst so—Unsere Ernahrungsstrategie) devoted to improve sustainability of the food system (Braun et al., 2018).
Experience BM
Experience BM includes projects where the revenues are mainly associated with marketing a specific experience rather than a farm product per-se (Pölling et al., 2015). The BM targets the growing necessity in urban citizens to reconnect with nature and experience traditional cooking recipes (e.g., recovering traditional ways to process a tomato sauce, or hand-making of pasta) or learn gardening skills (e.g., recognizing wild edible species, or acquiring synergistic or permacultural cropping techniques) (Pölling et al., 2017). Experience may take place in the form of intensive workshops (as for the “kill-your-own chicken” workshops organized at Nettle Farm, Rhode Island, USA, La Bibioteca, Fermo, Italy, or Uit Je Eigen Stad in Rotterdam, The Netherlands, Gustafsson and Olsson, 2016), but also as non-organized activities that are made accessible within the farm (e.g., sensorial paths or pick-your-own fields, Yoshida et al., 2019).
Experimental BM
Experimental BM includes projects that retain a high level of innovation, generally linked to new food producing technologies or adaptation of existing solutions to the urban environment. Innovation may fall within the production technology (e.g., indoor vertical farms, rooftop greenhouses or aquaponics, Calone et al., 2019), but also in the processing stage (e.g., through integration of urban waste flows or the set-up of circular schemes, Pulighe and Lupia, 2019) or in the functions (e.g., regeneration of abandoned districts or brownfields revitalisation, Gasperi et al., 2016). In these systems, technology is often at beta stage, and the project sustainability often benefits from available public or private funding for research and innovation activities (O'Sullivan et al., 2019).
Building an Inventory of UA Projects in the Global North
To date, a comprehensive census of UA projects and initiatives in the Global North has not yet been compiled, although some attempts of building local inventories exist, mainly in the framework of national and international projects. Entrepreneurial UA projects in Europe were recently listed in two highly comprehensive inventories in the framework of both the COST project TD1106 Urban Agriculture Europe (http://www.urban-agriculture-europe.org, Pölling et al., 2016b) and the Eramus+ project Urban Green Train (https://site.unibo.it/urbangreentrain/en/, Renting et al., 2016), altogether with classifications based on adopted business models. Similarly, a list of urban municipal gardens was compiled in the framework of the COST project TU1201 Allotment Gardens in European Cities (https://www.urbanallotments.eu/, Bell et al., 2016). Educational school gardens were also analyzed within the Erasmus+ project GardensToGrow (http://www.gardenstogrow.eu/, Pennisi et al., 2020b). The sustainability assessment of UA projects was mainly targeted within the H2020 MSCA project SustUrbanFoods (https://susturbanfoods.com/, Sanyé-Mengual et al., 2019) and the ClimateKIC action UrbaClim (http://www2.agroparistech.fr/Projet-URBACLIM.html, Lelièvre and Clérino, 2018). Similar research is also conducted within the JPI Urban Europe project FEW-Meter (http://www.fewmeter.org/en/home/), which targets assessment of resource use in UA projects in both Europe and North America (Ponizy et al., 2018). Moving to North America, among the mostly acknowledged projects, CarrotCity (https://www.ryerson.ca/carrotcity/), evolved from a book that aimed to compile a comprehensive database of UA experiences (Nasr and Komisar, 2014) and allowed for the creation of a mobile exhibition of featured case studies, which between 2009 and 2015 was displayed in cities across North America, Europe, Africa and Asia. Within the present manuscript a comprehensive inventory of UA projects built on all abovementioned databases was compiled (Supplementary Table 1), comprising all projects established in countries with developed economies (United Nations, 2020). The search provided no queries associated with some countries within the list, namely Finland, Luxemburg, Croatia, Cyprus, Estonia, Hungary, Latvia, Lithuania, Malta, Romania and New Zealand. Nevertheless, a total inventory of 470 UA projects (respectively, 288 in Europe, 97 in North America, 5 in Asia, and 80 in Oceania) was compiled, whose main features (including area, farming purpose and business models adopted) are hereby summarized (Figure 2). For comparative purposes, the main farming purpose and main business model were reported for each project, although these can be combined with other farming purposes and business models. The database shall be considered as updated at May 2020. Out of the 470 cases considered, surface data on the cultivated area was provided by 417 cases.
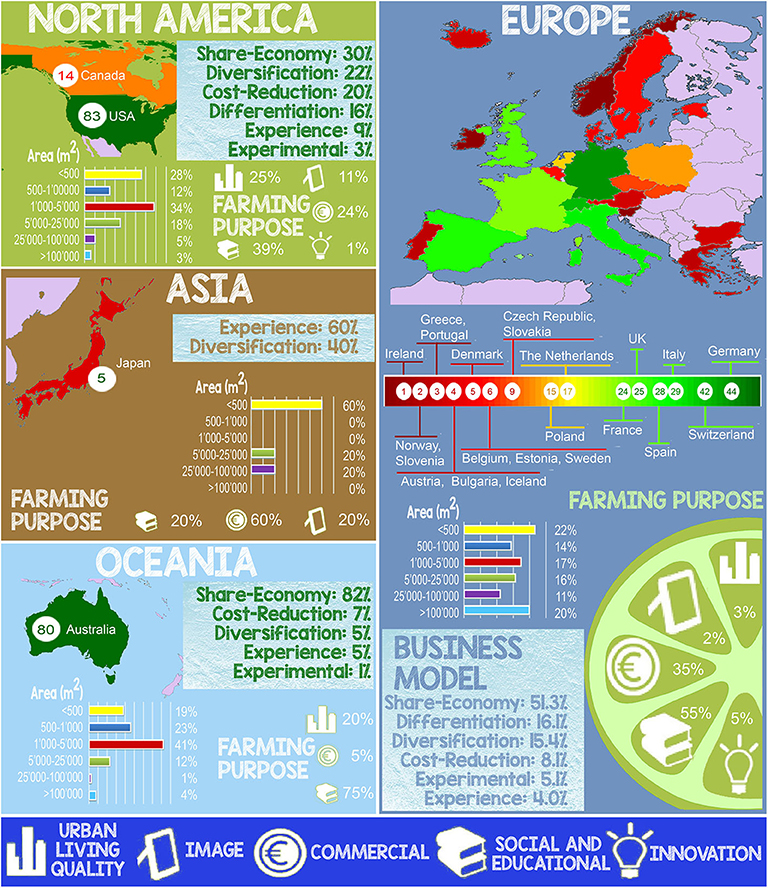
Figure 2. Infographics on the inventory of UA projects in the Global North countries. In white circles the number of cases per country, also reflected within country color over the heatmap (e.g., red 0–10 projects per country, orange-yellow 10–20 projects per country, green more than 20 projects per country). In each World Region, figures on farm area, business model and farming purpose are integrated. Area charts represent the distribution frequency in the different size classes. Business models are classified according to Pölling et al. (2015) in the six categories: cost reduction, diversification, differentiation, share economy, experience, and experimental and placed in order of frequency. Farming purposes are classified accordingly to Thomaier et al. (2015) in five categories: urban living quality, image, commercial, social and educational, innovation. Sample composed of 417 UA projects.
Statistically significant association [X2 (25) = 92.568, p < 0.000] between projects dimension class and business model typologies was observed (Figure 3A, n = 399). Share-economy business model is highly (>49% of the total) diffuse in small projects with a surface area lower than 5,000 m2, while in general experience and experimental business models are less frequent for all the considered projects dimension class. From the standardized residual analyses, it emerged that diversification business model was more common (38.7%) for projects with a surface area ranging 25,001 to 100,000 m2 (see Supplementary Table 2) as compared to the other projects dimension classes, while differentiation business model resulted more represented in the biggest project dimensions category (surface area > 100,000 m2), where, on the other hand, share-economy business model resulted statistically underrepresented.
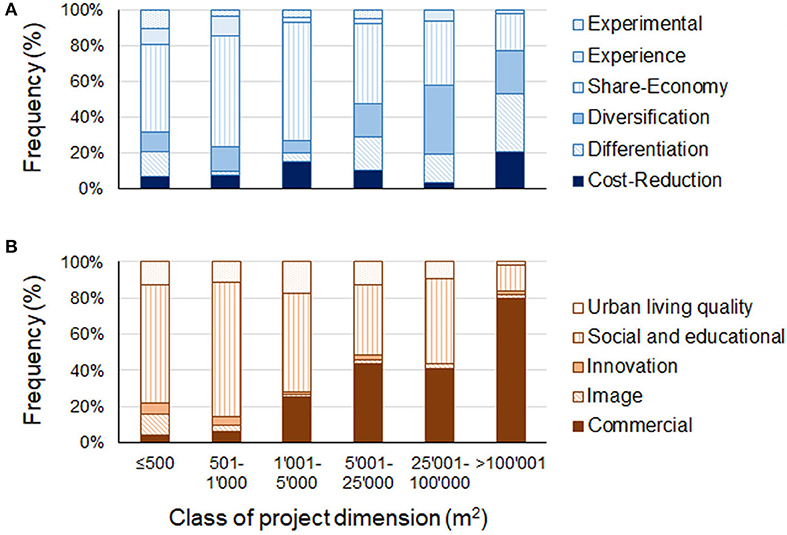
Figure 3. Relative distribution frequency (%) of business models (A, Pölling et al., 2015, n = 399) and farming purposes (B, Thomaier et al., 2015, n = 407) with respect to the class of project dimension among the case studies included in the database.
A statistically significant association [X2 (20) = 137.519, p < 0.000] between class of projects dimension and farming purposes was observed (Figure 3B, n = 407). In general, it was observed that social and educational purpose is highly represented (>54% of the total) in small projects with a surface area lower than 5,000 m2, while for the biggest project category purpose is more common (80% of the total). From the standardized residual analyses, it resulted that projects with commercial purpose were underrepresented in small project category (surface area ≤ 1,000 m2), but more common in projects belonging to the highest projects dimension class, trend which resulted completely inversed for social and educational projects (see Supplementary Table 3). Furthermore, also projects with image purpose resulted overrepresented in the smallest project dimension class.
Contrarily, chi-square test did not show a statistically significant relation between class of projects dimension and cities population [X2 (25) = 41.639, p = 0.05, n = 417], between class of projects dimension and category of city density [X2 (10) = 12.157, p = 0.275, n = 386] and between class of projects dimension and city climate [X2 (115) = 20.543, p = 0.152, n = 417] (data not shown).
Conclusions
Within the present review paper, 470 UA projects distributed across different world regions of the so-called Global North are identified and classified, according to their main business models, farming purposes and surface covered (Figure 2). UA's main ecosystems services in the Global North span from food provision to health functions, social inclusion and justice and contribution to ecological and environmental sustainability. Main factors that affect UA development and diffusion include the existing legal framework, land access, contamination risks, local climatic conditions and resource availability. A diversified number of typologies of farming systems were observed, including allotment gardens, extensive periurban farms, urban community gardens, rooftop farms and indoor vertical farms, as well as specific systems associated with the production of niche food products (e.g., microgreens, aquaponics, urban honey). With reference to farm size, in all world regions considered, a large share of UA projects operate on small surface (<1,000 m2). Larger farms (e.g., above 10 ha), represent a fifth of the cases in Europe, whereas about 3–4% in both America and Oceania. Among business model strategies, in Europe, North America and Oceania, the largest share of UA projects followed share-economy BM, but diversification and experience business models were also found in all world regions. Specifically, share-economy business model resulted to be diffuse in projects with small surface area (<5,000 m2), while differentiation and diversification were the predominant business models in the biggest project dimensions category (e.g., above 10 ha) (Figure 3A). Among farming purposes, social and educational farming were the most frequent cases in Europe, Oceania and North America, while commercial projects were indeed predominant within the few cases reported in Japan. Considering farming purposes in relation to project surface areas, it emerged that commercial projects were underrepresented in small project category (≤ 1,000 m2) but the most common among largest projects, contrarily to social and educational projects which resulted more common in small projects but quite rare for big (above 10 ha) projects (Figure 3B). Looking at city population, city density and city climate, no statistically significant relations were highlighted with project surface area categories. The collected data may allow for further design and implementation of successful UA experiences, while also fostering cross-pollination among initiatives and enabling the environment for sustainable urban farming. It overall emerges that, although with smaller figures in terms of food production capacity as compared with rural agriculture, the UA sector has a clear potential in fostering food security in time of emergency (e.g., in response to pandemics or extreme climate events), as well as promoting the overall city sustainability (with the associated benefits in terms of reduced environmental footprint, social justice, ecology and microclimate). However, further research effort is needed to substantiate the estimated potential with actual figures at city scale and enable the environment for the implementation of appropriate legal frameworks and guidelines toward large-scale diffusion of sustainable UA initiatives. Moreover, the application of the hereby adopted methodologies and classifications to UA projects from the Global South could also allow for comparative assessment of successful strategies.
Author Contributions
FO drafted the paper and coordinated the preparation of the manuscript and data visualization. GP, NM, and ES-M created the inventory of case studies and contributed to sections Ecosystem Services (ES) Associated with UA, Factors That Affect Development and Diffusion of UA, and Main Typologies of UA Systems. GB contributed to section Ecological Aspects and Building an Inventory of UA Projects in the Global North. AM contributed to section Introduction. GG contributed to sections Introduction, Defining Urban Agriculture (UA), Health, Laws and Regulations, and Main Typologies of UA Systems. All authors critically revised the manuscript.
Funding
The research leading to this publication has received funding from the European Union's Horizon 2020 research and innovation programme under grant agreement No. 862663. The publication reflects the author's views. The Research Executive Agency (REA) is not liable for any use that may be made of the information contained therein.
Conflict of Interest
The authors declare that the research was conducted in the absence of any commercial or financial relationships that could be construed as a potential conflict of interest.
Supplementary Material
The Supplementary Material for this article can be found online at: https://www.frontiersin.org/articles/10.3389/fsufs.2020.562513/full#supplementary-material
References
Abd El-Azeem, S. A. M., Ahmad, M., Usman, A. R. A., Kim, K. R., Oh, S. E., Lee, S. S., et al. (2013). Changes of biochemical properties and heavy metal bioavailability in soil treated with natural liming materials. Environ. Earth Sci. 70, 3411–3420. doi: 10.1007/s12665-013-2410-3
Aben, R., and de Wit, S. (1999). The Enclosed Garden: History and Development of the Hortus Conclusus and its Reintroduction into the Present-Day Urban Landscape. Rotterdam: 010 Publishers.
Ackerman, K., Conard, M., Culligan, P., Plunz, R., Sutto, M. P., and Whittinghill, L. (2014). Sustainable food systems for future cities: the potential of urban agriculture. Econ. Soc. Rev. 45, 189–206.
Akimowicz, M., Cummings, H., and Landman, K. (2016). Green lights in the greenbelt? A qualitative analysis of farm investment decision-making in peri-urban Southern Ontario. Land Use Policy 55, 24–36. doi: 10.1016/j.landusepol.2016.03.024
Algert, S. J., Baameur, A., and Renvall, M. J. (2014). Vegetable output and cost savings of community gardens in San Jose, California. J. Acad. Nutr. Diet. 114, 1072–1076. doi: 10.1016/j.jand.2014.02.030
Allen, A. (2003). Environmental planning and management of the peri-urban interface: perspectives on an emerging field. Environ. Urban 15, 135–148. doi: 10.1630/095624703101286402
Ancion, N., Morel-Chevillet, G., Rovira Val, M. R., Schreier, F., Solecki, B., Zita, N., et al. (2019). The Case of the Bankruptcy of Urban-Farmers in The Hague. Groof Analysis. Available online at: https://orbi.uliege.be/retrieve/390326/407573/Urban%20farmers%20Bankruptcy-GROOF%20report-Uli%C3%A8ge.pdf (accessed October 27, 2020).
Andersson, E., Barthel, S., and Ahrné, K. (2007). Measuring social–ecological dynamics behind the generation of ecosystem services. Ecol. Appl. 17, 1267–1278. doi: 10.1890/06-1116.1
Anguelovski, I. (2013). From environmental trauma to safe haven: place attachment and place remaking in three marginalized neighborhoods of Barcelona, Boston, and Havana. City Commun. 12, 211–237. doi: 10.1111/cico.12026
Anguelovski, I. (2015). Alternative food provision conflicts in cities: contesting food privilege, injustice, and whiteness in Jamaica Plain, Boston. Geoforum 58, 184–194. doi: 10.1016/j.geoforum.2014.10.014
Anguelovski, I., Connolly, J. J., Masip, L., and Pearsall, H. (2018). Assessing green gentrification in historically disenfranchised neighborhoods: a longitudinal and spatial analysis of Barcelona. Urban Geogr. 39, 458–491. doi: 10.1080/02723638.2017.1349987
Armstrong, D. (2000). A survey of community gardens in upstate New York: implications for health promotion and community development. Health Place 6, 319–327. doi: 10.1016/S1353-8292(00)00013-7
Aubry, C., and Daniel, A. C. (2017). “Innovative commercial urban agriculture in the Paris metropolitan area,” in Toward Sustainable Relations Between Agriculture and the City, eds C. T. Soulard, C. Perrin, and E. Valette (Cham: Springer), 147–162.
Austruy, A., Shahid, M., Xiong, T., Castrec, M., Payre, V., Khan Niazi, N., et al. (2014). Mechanisms of metal-phosphates formation in the rhizosphere soils of pea and tomato: environmental and sanitary consequences. J. Soils Sediments 14, 666–678. doi: 10.1007/s11368-014-0862-z
Baker, P. J., and Harris, S. (2007). Urban mammals: what does the future hold? An analysis of the factors affecting patterns of use of residential gardens in Great Britain. Mam. Rev. 37, 297–315. doi: 10.1111/j.1365-2907.2007.00102.x
Barthel, S., Parker, J., and Ernstson, H. (2013). Food and green space in cities: a resilience lens on gardens and urban environmental movements. Urban Stud. 52, 1321–1338. doi: 10.1177/0042098012472744
Bazzocchi, G. (2020). “Pest management for urban agriculture,” in Achieving Sustainable Urban Agriculture, ed J. S. C. Wiskerke (Cambridge: Burleigh Dodds Science Publishing), 199–219.
Bazzocchi, G., Pennisi, G., Frabetti, A., Orsini, F., and Gianquinto, G. (2017). Abundance, migration and distribution of Coccinella septempunctata (Coleoptera: Coccinellidae) in a highly biodiverse urban garden. Acta Hortic. 1189, 501–504. doi: 10.17660/ActaHortic.2017.1189.100
Beacham, A. M., Vickers, L. H., and Monaghan, J. M. (2019). Vertical farming: a summary of approaches to growing skywards. J. Hortic. Sci. Biotech. 94, 277–283. doi: 10.1080/14620316.2019.1574214
Beauchesne, A., and Bryant, C. (1999). Agriculture and innovation in the urban fringe: the case of organic farming in Quebec. Tijdschr Econ. Soc. Geogr. 90, 320–328. doi: 10.1111/1467-9663.00073
Bell, S., Fox-Kämper, R., Keshavarz, N., Benson, M., Caputo, S., Noori, S., et al. (2016). Urban Allotment Gardens in Europe. London: Routledge.
Benis, K., and Ferrão, P. (2018). Commercial farming within the urban built environment–taking stock of an evolving field in northern countries. Glob. Food Sec. 17, 30–37. doi: 10.1016/j.gfs.2018.03.005
Beniston, J., and Lal, R. (2012). “Improving soil quality for urban agriculture in the North Central US.” in Carbon Sequestration in Urban Ecosystems, eds R. Lal and B. Augustin (Dordrecht: Springer), 279–313.
Bertoni, D., and Cavicchioli, D. (2016). Farm succession, occupational choice and farm adaptation at the rural-urban interface: the case of Italian horticultural farms. Land Use Policy 57, 739–748. doi: 10.1016/j.landusepol.2016.07.002
Biewener, C. (2016). Paid work, unpaid work, and economic viability in alternative food initiatives: reflections from three Boston urban agriculture endeavors. J. Agric. Food Syst. Comm. Dev. 6, 35–53. doi: 10.5304/jafscd.2016.062.019
Blum, J. (2017). Where is the UK's pollinator biodiversity? The importance of urban areas for flower-visiting insects. Proc. R. Soc. B. 282:20142849. doi: 10.1098/rspb.2014.2849
Bradley, K., and Hedrén, J. (2014). Green Utopianism: Perspectives, Politics and Micro-Practices. New York, NY: Routledge.
Braun, C., Rombach, M., Häring, A., and Bitsch, V. (2018). A local gap in sustainable food procurement: organic vegetables in Berlin's school meals. Sustainability 10:4245. doi: 10.3390/su10114245
Breuste, J., Niemel,ä, J., and Snep, R. P. (2008). Applying landscape ecological principles in urban environments. Land. Ecol. 23, 1139–1142. doi: 10.1007/s10980-008-9273-0
Brown, C., and Miller, S. (2008). The impacts of local markets: a review of research on farmers markets and community supported agriculture (CSA). Am. J. Agric. Econ. 90, 1298–1302. doi: 10.1111/j.1467-8276.2008.01220.x
Brown, K., and Jameton, A. (2000). Public health implications of urban agriculture. J. Public Health Policy 21, 20–39. doi: 10.2307/3343472
Bryld, E. (2003). Potentials, problems, and policy implications for urban agriculture in developing countries. Agric. Hum. Value 20, 79–86. doi: 10.1023/A:1022464607153
Buehler, D., and Junge, R. (2016). Global trends and current status of commercial urban rooftop farming. Sustainability 8:1108. doi: 10.3390/su8111108
Burkman, C. E., and Gardiner, M. M. (2014). Urban greenspace composition and landscape context influence natural enemy community composition and function. Biol. Cont. 75, 58–67. doi: 10.1016/j.biocontrol.2014.02.015
Burls, A. P. (2008). Seeking nature: a contemporary therapeutic environment. Int. J. Ther. Commun. 29, 228–44.
Butturini, M., and Marcelis, L. F. (2020). “Vertical farming in Europe: present status and outlook,” in Plant Factory: An Indoor Vertical Farming System for Efficient Quality Food Production, eds T. Kozai, G. Niu, and M. Takagaki (Cambridge: Academic Press), 77–91.
Calone, R., Pennisi, G., Morgenstern, R., Sanyé-Mengual, E., Lorleberg, W., Dapprich, P., et al. (2019). Improving water management in European catfish recirculating aquaculture systems through catfish-lettuce aquaponics. Sci. Total Environ. 687, 759–767. doi: 10.1016/j.scitotenv.2019.06.167
Calvet-Mir, L., and March, H. (2017). Crisis and post-crisis urban gardening initiatives from a Southern European perspective: the case of Barcelona. Eur. Urban Reg. Stud. 26, 97–112. doi: 10.1177/0969776417736098
Camps-Calvet, M., Langemeyer, J., Calvet-Mir, L., and Gómez-Baggethun, E. (2016). Ecosystem services provided by urban gardens in Barcelona, Spain: insights for policy and planning. Environ. Sci. Policy 62, 14–23. doi: 10.1016/j.envsci.2016.01.007
Camps-Calvet, M., Langemeyer, J., Calvet-Mir, L., Gómez-Baggethun, E., and March, H. (2015). Sowing resilience and contestation in times of crises: the case of urban gardening movements in Barcelona. Partecip. Conf. 8, 417–442. doi: 10.1285/i20356609v8i2p417
Caputo, S., Iglesias, P., and Rumble, H. (2017). “Design of rooftop agriculture systems – elements of rooftop agriculture design,” in Rooftop Urban Agriculture, eds F. Orsini, M. Dubbeling, H. De Zeeuw, and G. Gianquinto (Cham: Springer), 39–59.
Cavallo, M., and Rainieri, G. (2018). “Metropolitan agriculture and social involvement. An international debate,” in Metropolitan Agriculture and Natural-Based Solutions, eds M. Cavallo and S. Spillare (Milano: Franco Angeli), 101–110.
Centrone Stefani, M., Orsini, F., Magrefi, F., Sanyé-Mengual, E., Pennisi, G., Michelon, N., et al. (2018). “Toward the creation of urban foodscapes: case studies of successful urban agriculture projects for income generation, food security, and social cohesion,” in Urban Horticulture, ed D. Nandwani (Cham: Springer), 91–106.
Cervera-Mata, A., Navarro-Alarcón, M., Delgado, G., Pastoriza, S., Montilla-Gómez, J., Llopis, J., et al. (2019). Spent coffee grounds improve the nutritional value in elements of lettuce (Lactuca sativa L.) and are an ecological alternative to inorganic fertilizers. Food Chem. 282, 1–8. doi: 10.1016/j.foodchem.2018.12.101
Chang, H., and Franczyk, J. (2008). Climate change, land-use change, and floods: toward an integrated assessment. Geogr. Comp. 2, 1549–1579. doi: 10.1111/j.1749-8198.2008.00136.x
Charlesworth, S., de Miguel, E. A., and Ordoñez, A. (2011). A review of the distribution of particulate trace elements in urban terrestrial environments and its application to considerations of risk. Environ. Geochem. Health 33, 103–123. doi: 10.1007/s10653-010-9325-7
Clark, K. H., and Nicholas, K. A. (2013). Introducing urban food forestry: a multifunctional approach to increase food security and provide ecosystem services. Landscape Ecol. 28, 1649–1669. doi: 10.1007/s10980-013-9903-z
Clausen, M. (2015). Urban agriculture between pioneer use and urban land grabbing: the case of “Prinzessinnengarten” Berlin. Cities Environ. 8:15.
CoDyre, M., Fraser, E. D. G., and Landman, K. (2015). How does your garden grow? An empirical evaluation of the costs and potential of urban gardening. Urban For. Urban Green. 14, 72–79. doi: 10.1016/j.ufug.2014.11.001
Cofie, O., Bradford, A. A., and Dreschel, P. (2006). “Recycling of urban organic waste for urban agriculture,” in Cities Farming for the Future: Urban Agriculture for Green and Productive Cities, ed. R. van Veenhuizen (Leusden: RUAF), 207–229.
Cohen, N., and Reynolds, K. (2015). Resource needs for a socially just and sustainable urban agriculture system: lessons from New York City. Renew. Agric. Food Syst. 30, 103–114. doi: 10.1017/S1742170514000210
Colantoni, A., Pili, S., Mosconi, E. M., Poponi, S., Cecchini, M., Doria, P., and Salvati, L. (2017). Metropolitan agriculture, socio-demographic dynamics and the food-city relationship in southern Europe. Curr. Policy Econ. Eur. 28, 301–324. Available online at: https://core.ac.uk/download/pdf/188826516.pdf
Colasanti, K. J. A., Hamm, M. W., and Litjens, C. M. (2012). The city as an “Agricultural Powerhouse”? Perspectives on expanding urban agriculture from Detroit, Michigan. Urban Geogr. 33, 348–369. doi: 10.2747/0272-3638.33.3.348
Colding, J., Lundberg, J., and Folke, C. (2006). Incorporating green area user groups in urban ecosystem management. AMBIO J. Hum. Environ. 35, 237–244. doi: 10.1579/05-A-098R.1
Coleman, C., and Mattson, R. H. (1995). Influence of foliage plants on human stress during thermal biofeedback training. HortTechnology 5, 137–140. doi: 10.21273/HORTTECH.5.2.137
Colle, M., Daniel, A. C., and Aubry, C. (2017). Call for projects “Parisculteurs”: catalyst for urban agriculture development on rooftops in Paris. Acta Hortic. 1215, 147–152. doi: 10.17660/ActaHortic.2018.1215.28
Crouch, D. (2000). Reinventing allotments for the twenty-first century: the UK experience. Acta Hortic. 523, 135–142. doi: 10.17660/ActaHortic.2000.523.18
Cunningham, S. D., Berti, W. R., and Huang, J. W. (1995). Phytoremediation of contaminated soils. Trends Biotechnol. 13, 393–397. doi: 10.1016/S0167-7799(00)88987-8
Dahlin, J., Beuthner, C., Halbherr, V., Kurz, P., Nelles, M., and Herbes, C. (2019). Sustainable compost and potting soil marketing: private gardener preferences. J. Clean. Prod. 208, 1603–1612. doi: 10.1016/j.jclepro.2018.10.068
de Bon, H., Parrot, L., and Moustier, P. (2010). Sustainable urban agriculture in developing countries. A review. Agron Sust. Dev. 30, 21–32. doi: 10.1051/agro:2008062
de Zeeuw, H., Van Veenhuizen, R., and Dubbeling, M. (2011). The role of urban agriculture in building resilient cities in developing countries. J. Agric. Sci. 149:153. doi: 10.1017/S0021859610001279
Deelstra, T., and Girardet, H. (2000). “Urban agriculture and sustainable cities,” in Growing Cities, Growing Food, eds N. Bakker, M. Dubbeling, S. Gündel, U. Sabel-Koshella, and H. de Zeeuw (Feldafing: Zentralstelle für Ernährung und Landwirtschaft), 43–66.
Depietri, Y., Renaud, F. G., and Kallis, G. (2012). Heat waves and floods in urban areas: a policy-oriented review of ecosystem services. Sustain. Sci. 7, 95–107. doi: 10.1007/s11625-011-0142-4
Dijkstra, L., and Poelman, H. (2012). Cities in Europe: the new OECD-EC definition. Region. Focus 1, 1–13.
Dimitri, C., Oberholtzer, L., and Pressman, A. (2016). Urban agriculture: connecting producers with consumers. Br. Food J. 118, 603–617. doi: 10.1108/BFJ-06-2015-0200
Ding, C., Zhang, T., Wang, X., Zhou, F., Yang, Y., and Yin, Y. (2013). Effects of soil type and genotype on lead concentration in rootstalk vegetables and the selection of cultivars for food safety. J. Environ. Man. 122, 8–14. doi: 10.1016/j.jenvman.2013.02.026
Dixon, J., Omwega, A. M., Friel, S., Burns, C., Donati, K., and Carlisle, R. (2007). The health equity dimensions of urban food systems. J. Urb. Health 84, 118–129. doi: 10.1007/s11524-007-9176-4
Donadieu, P. (2006). Landscape urbanism in Europe: from brownfields to sustainable urban development. J. Lands. Archit. 1, 36–45. doi: 10.1080/18626033.2006.9723371
Dorr, E., Sanyé-Mengual, E., Gabrielle, B., Grard, B. J., and Aubry, C. (2017). Proper selection of substrates and crops enhances the sustainability of Paris rooftop garden. Agron. Sustain. Dev. 37:51. doi: 10.1007/s13593-017-0459-1
Draus, P. J., Roddy, J., and McDuffie, A. (2014). ‘We don't have no neighbourhood': advanced marginality and urban agriculture in Detroit. Urban Stud. 51, 2523–2538. doi: 10.1177/0042098013506044
Drechsel, P., and Kunze, D. (2001). Waste Composting for Urban and Peri-Urban Agriculture: Closing the Rural-Urban Nutrient Cycle in Sub-Saharan Africa. Wallingford, CT: CABI.
Drescher, A. A. W. (2001). “The German allotment gardens-a model for poverty alleviation and food security in Southern African Cities,” in Proceedings of the Sub-Regional Expert Meeting on Urban Horticulture (Stellenbosch: University of Stellenbosch).
Du, S., Shi, P., Van Rompaey, A., and Wen, J. (2015). Quantifying the impact of impervious surface location on flood peak discharge in urban areas. Nat. Hazard 76, 1457–1471. doi: 10.1007/s11069-014-1463-2
Dubbeling, M. (2014). Urban agriculture as a climate change and disaster risk reduction strategy. UA Mag. 27, 3–7. Available online at: https://journals.openedition.org/factsreports/5650
Durham, S. (2017). Which minerals are in microgreens? Agric. Res. Mag. 65, 1–3. Available online at: https://agresearchmag.ars.usda.gov/2017/apr/microgreens/
EC (2010). Making Our Cities Attractive and Sustainable. How the EU Contributes to Improving the Urban Environment. Brussels: Publications Office of the European Union. Available online at: https://ec.europa.eu/environment/europeangreencapital/wp-content/uploads/2011/04/Making-our-cities-attractive-and-sustainable.pdf
EC (2020). A Farm to Fork Strategy for a Fair, Healthy and Environmentally-Friendly Food System. Brussels: Publications Office of the European Union. Available online at: https://eur-lex.europa.eu/legal-content/EN/TXT/HTML/?uri=CELEX:52020DC0381andfrom=EN
Edelstein, M., and Ben-Hur, M. (2018). Heavy metals and metalloids: sources, risks and strategies to reduce their accumulation in horticultural crops. Sci. Hortic. 234, 431–444. doi: 10.1016/j.scienta.2017.12.039
EEA (2012). Climate Change, Impacts and Vulnerability in Europe 2012: An Indicator-Based Report. Luxembourg: Office for Official Publications of the European Union. Available online at: https://www.eea.europa.eu/publications/climate-impacts-and-vulnerability-2012
EEA (2015). Urban Sustainability Issues. What is a Resource-Efficient City? Luxembourg: Office for Official Publications of the European Union. Available online at: https://www.eea.europa.eu/publications/resource-efficient-cities
EEA (2019). Land Take in Europe. Luxembourg: Office for Official Publications of the European Union. Available online at: https://www.eea.europa.eu/data-and-maps/indicators/land-take-3/assessment
El Hamiani, O., El Khalil, H., Lounatea, K., Sirguey, C., Hafidi, M., Bitton, G., et al. (2010). Toxicity assessment of garden soils in the vicinity of mining areas in Southern Morocco. J. Hazards Mater. 177, 755–761. doi: 10.1016/j.jhazmat.2009.12.096
Ellis, E. C., Kaplan, J. O., Fuller, D. Q., Vavrus, S., Klein Goldewijk, K., and Verburg, P. H. (2013). Used planet: a global history. Proc. Natl. Acad. Sci. U.S.A. 110, 7978–7985. doi: 10.1073/pnas.1217241110
Ernwein, M. (2014). Framing urban gardening and agriculture: on space, scale and the public. Geoforum 56, 77–86. doi: 10.1016/j.geoforum.2014.06.016
Filippini, R., Mazzocchi, C., and Corsi, S. (2019). The contribution of urban food policies toward food security in developing and developed countries: a network analysis approach. Sustain. Cities Soc. 47:101506. doi: 10.1016/j.scs.2019.101506
Fisher, S., and Karunanithi, A. (2014). “Contemporary comparative LCA of commercial farming and urban agriculture for selected fresh vegetables consumed in Denver, Colorado,” in Proceedings of the 9th International Conference on Life Cycle Assessment in the Agri-Food Sector, eds R. Schenck, and D. Huizenga (San Francisco, CA: American Center for Life Cycle Assessment), 405–414.
Forchino, A. A., Gennotte, V., Maiolo, S., Brigolin, D., Mélard, C., and Pastres, R. (2018). Eco-designing Aquaponics: a case study of an experimental production system in Belgium. Proc. CIRP 69, 546–550. doi: 10.1016/j.procir.2017.11.064
Fox-Kämper, R., Wesener, A., Münderlein, D., Sondermann, M., McWilliam, W., and Kirk, N. (2018). Urban community gardens: an evaluation of governance approaches and related enablers and barriers at different development stages. Land. Urban Plan. 170, 59–68. doi: 10.1016/j.landurbplan.2017.06.023
Frison, E. A., Cherfas, J., and Hodgkin, T. (2011). Agricultural biodiversity is essential for a sustainable improvement in food and nutrition security. Sustainability 3, 238–253. doi: 10.3390/su3010238
Frumkin, H. (2003). Healthy places: exploring the evidence. Am. J. Public Health 93, 1451–1456. doi: 10.2105/AJPH.93.9.1451
Fuller, R. A., and Gaston, K. J. (2009). The scaling of green space coverage in European cities. Biol. Lett. 5, 352–355. doi: 10.1098/rsbl.2009.0010
Gallaher, C. M., Kerr, J. M., Njenga, M., Karanja, N. K., and WinklerPrins, A. M. (2013). Urban agriculture, social capital, and food security in the Kibera slums of Nairobi, Kenya. Agric. Hum. Value 30, 389–404. doi: 10.1007/s10460-013-9425-y
García, R., and Millán, E. (1994). Heavy metals contents from road soils in Guipúzcoa (Spain). Sci. Total Environ. 146, 157–161. doi: 10.1016/0048-9697(94)90232-1
Garcia-Perez, M. A., and Nunez-Anton, V. (2003). Cellwise residual analysis in two-way contingency tables. Educ. Psychol. Meas. 63, 825–839. doi: 10.1177/0013164403251280
García-Sempere, A., Morales, H., Hidalgo, M., Ferguson, B. G., Rosset, P., and Nazar-Beutelspacher, A. (2019). Food Sovereignty in the city?: A methodological proposal for evaluating food sovereignty in urban settings. Agroecol. Sustain. Food Syst. 43, 1145–1173. doi: 10.1080/21683565.2019.1578719
Gasperi, D., Pennisi, G., Rizzati, N., Magrefi, F., Bazzocchi, G., Mezzacapo, U., et al. (2016). Towards regenerated and productive vacant areas through urban horticulture: lessons from Bologna, Italy. Sustainability 8:1347. doi: 10.3390/su8121347
Gasperi, D., Bazzocchi, G., Bertocchi, I., Ramazzotti, S., and Gianquinto, G. (2012). The multifunctional role of urban gardens in the twentieth century. the Bologna case study. Acta Hortic. 1093, 91–98. doi: 10.17660/ActaHortic.2015.1093.9
Gerster-Bentaya, M. (2013). Nutrition-sensitive urban agriculture. Food Secur. 5, 723–737. doi: 10.1007/s12571-013-0295-3
Ghosh, A. K., Bhatt, M. A., and Agrawal, H. P. (2012). Effect of long-term application of treated sewage water on heavy metal accumulation in vegetables grown in Northern India. Environ. Monit. Assess. 184, 1025–1036. doi: 10.1007/s10661-011-2018-6
Giacchè, G., Ejderyan, O., Salomon Cavin, J., Lardon, S., and Mumenthaler, C. (2016). “Exploring the diversity of actors in Urban Agriculture,” in Urba Agriculture Europe, eds F. Lohrberg, L. Liçka, L. Scazzosi, and A. Timpe, 58–62. Available online: https://www.jovis.de/en/books/details/product/urban-agriculture-europe.html
Goldstein, B. P., Hauschild, M. Z., Fernández, J. E., and Birkved, M. (2017). Contributions of local farming to urban sustainability in the Northeast United States. Environ. Sci. Technol. 51, 7340–7349. doi: 10.1021/acs.est.7b01011
Gonzalez, M. T., Hartig, T., Patil, G. G., Martinsen, E. W., and Kirkevold, M. (2009). Therapeutic horticulture in clinical depression: a prospective study. Res. Theor. Nurs. Pract. 23, 312–328. doi: 10.1891/1541-6577.23.4.312
Grant, C. A., Clarke, J. M., Duguid, S., and Chaney, R. L. (2008). Selection and breeding of plant cultivars to minimize cadmium accumulation. Sci. Total Environ. 390, 301–310. doi: 10.1016/j.scitotenv.2007.10.038
Grard, B. J. P., Chenu, C., Manouchehri, N., Houot, S., Frascaria-Lacoste, N., and Aubry, C. (2018). Rooftop farming on urban waste provides many ecosystem services. Agron. Sustain. Dev. 38:2. doi: 10.1007/s13593-017-0474-2
Grewal, S. S., and Grewal, P. S. (2012). Can cities become self-reliant in food? Cities 29, 1–11. doi: 10.1016/j.cities.2011.06.003
Guitart, D., Pickering, C., and Byrne, J. (2012). Past results and future directions in urban community gardens research. Urban For. Urban Green. 11, 364–373. doi: 10.1016/j.ufug.2012.06.007
Gustafsson, M., and Olsson, M. (2016). Metropolitan foodscapes with multifunctional land use [Dissertation/Master's thesis]. Malmo: Swedish University of Agricultural Sciences.
Halaj, J., Ross, D. W., and Moldenke, A. R. (2000). Importance of habitat structure to the arthropod food-web in Douglas-fir canopies. Oikos 90, 139–152. doi: 10.1034/j.1600-0706.2000.900114.x
Hall, D. M., Camilo, G. R., Tonietto, R. K., Ollerton, J., Ahrn,é, K., Arduser, M., et al. (2017). The city as a refuge for insect pollinators. Conserv. Biol. 31, 24–29. doi: 10.1111/cobi.12840
Hamilton, A. J., Burry, K., Mok, H. F., Barker, S. F., Grove, J. R., and Williamson, V. G. (2014). Give peas a chance? Urban agriculture in developing countries. A review. Agron. Sustain. Dev. 34, 45–73. doi: 10.1007/s13593-013-0155-8
Hanjra, M. A., Blackwell, J., Carr, G., Zhang, F., and Jackson, T. M. (2012). Wastewater irrigation and environmental health: implications for water governance and public policy. Int. J. Hyg. Environ. Health 215, 255–269. doi: 10.1016/j.ijheh.2011.10.003
Hansen, C. W., Jensen, P. S., and Skovsgaard, C. V. (2015). Modern gender roles and agricultural history: the Neolithic inheritance. J. Econ. Growth 20, 365–404. doi: 10.1007/s10887-015-9119-y
Hansson, H. (2007). Strategy factors as drivers and restraints on dairy farm performance: evidence from Sweden. Agric. Syst. 94, 726–737. doi: 10.1016/j.agsy.2007.03.002
Hardgrove, S. J., and Livesley, S. J. (2016). Applying spent coffee grounds directly to urban agriculture soils greatly reduces plani growth. Urban For. Urban Green 18, 1–8. doi: 10.1016/j.ufug.2016.02.015
Heath, T. (2001). Adaptive re-use of offices for residential use: the experiences of London and Toronto. Cities 18, 173–184. doi: 10.1016/S0264-2751(01)00009-9
Heckler, S. A. (2012). A right to farm in the city: providing a legal framework for legitimizing urban farming in American cities. Value UL Rev. 47:217. Available online at: https://scholar.valpo.edu/vulr/vol47/iss1/6
Heimlich, R. E. (1989). Metropolitan agriculture: farming in the city's shadow. J. Am. Plan. Assess. 55, 457–466. doi: 10.1080/01944368908975434
Holland, L. (2004). Diversity and connections in community gardens: a contribution to local sustainability. Local Environ. 9, 285–305. doi: 10.1080/1354983042000219388
Hough, R. L., Breward, N., Young, S. D., Crout, N. M., Tye, A. M., Moir, A. M., et al. (2004). Assessing potential risk of heavy metal exposure from consumption of home-produced vegetables by urban populations. Environ. Health Perspect. 112, 215–221. doi: 10.1289/ehp.5589
Hursthouse, A. S., and Kowalczyk, G. (2009). Transport and dynamics of toxic pollutants in the natural environment and their effect on human health: research gaps and challenge. Environ. Geochem. Health 31, 165–187. doi: 10.1007/s10653-008-9213-6
Hursthouse, A. S., and Leitão, T. E. (2016). “Environmental pressures on and the status of urban allotments,” in Urban Allotment Gardens in Europe, eds S. Bell, R. Fox-Kämper, N. Keshavarz, M. Benson, S. Caputo and S. Noori (London: Routledge), 164–186.
Huttner, S., Bruse, M., Dostal, P., and Katzschner, A. (2009). “Strategies for mitigating thermal heat stress in central European cities: the project Klimes,” in 7th International Conference on Urban Climate, 3 July, 2020 (Yokohama). Available online at: http://www.ide.titech.ac.jp/~icuc7/extended_abstracts/pdf/382880-4-090514012244-002.pdf (accessed June 29, 2009).
Hynes, H. P., and Howe, G. (2004). Urban horticulture in the contemporary United States: personal and community benefits. Acta Hort. 643, 171–181. doi: 10.17660/ActaHortic.2004.643.21
Ikejima, Y. (2016). “The reality of food deserts in a large Japanese city and their resolution using urban agriculture,” in Food Security and Food Safety for the Twenty-First Century, ed S. Hongladarom (Singapore: Springer), 205–216.
Isager, S., and Skydsgaard, J. E. (2013). Ancient Greek Agriculture: an Introduction. London: Routledge.
Jaeger, J. A. G., Bertiller, R., Schwick, C., and Kienast, F. (2010). Suitability criteria for measures of urban sprawl. Ecol. Indic. 10, 397–406. doi: 10.1016/j.ecolind.2009.07.007
Janoš, P., Vávrová, J., Herzogová, L., and Pilaøová, V. (2010). Effects of inorganic and organic amendments on the mobility (leachability) of heavy metals in contaminated soil: a sequential extraction study. Geoderma 159, 335–341. doi: 10.1016/j.geoderma.2010.08.009
Jean-Soro, L., Le Guern, C., Bechet, B., Lebeau, T., and Ringeard, M. F. (2014). Origin of trace elements in an urban garden in Nantes, France. J. Soils Sediment 15, 1802–1812. doi: 10.1007/s11368-014-0952-y
Kabisch, N., and Haase, D. (2011). Diversifying european agglomerations: evidence of urban population trends for the 21st century. Pop. Space Place 17, 236–253. doi: 10.1002/psp.600
Kam, M. C. Y., and Siu, A. M. H. (2010). Evaluation of a horticultural activity programme for persons with psychiatric illness. Hong Kong J. Occup. Theor. 20, 80–86. doi: 10.1016/S1569-18611170007-9
Kaplan, S. (1995). The restorative benefits of nature: toward an integrative framework. J. Environ. Psychol. 15, 169–183. doi: 10.1016/0272-4944(95)90001-2
Keshavarz, N., and Bell, S. (2016). “A history of urban gardens in Europe,” in Urban Allotment Gardens in Europe, eds S. Bell, R. Fox- Kämper, N. Keshavarz, M. Benson, S. Caputo and S. Noori (London: Routledge), 30–54.
Konijnendijk, C. C., and Park, H. (2020). “Optimising urban forestry: the food connection,” in Achieving Sustainable Urban Agriculture, ed J. S. C. Wiskerke (Cambridge: Burleigh Dodds Science Publishing), 353–368.
Kottek, M., Grieser, J., Beck, C., Rudolf, B., and Rubel, F. (2006). World map of the Köppen-Geiger climate classification updated. Meteor. Zeits 15, 259–263. doi: 10.1127/0941-2948/2006/0130
Kozai, T. (2013). Resource use efficiency of closed plant production system with artificial light: Concept, estimation and application to plant factory. Proc. Jpn. Acad. 89, 447–461. doi: 10.2183/pjab.89.447
Kozai, T. (2019). Towards sustainable plant factories with artificial lighting (PFALs) for achieving SDGs. Int. J. Agric. Biol. Eng. 12, 28–37. doi: 10.25165/j.ijabe.20191205.5177
Kozai, T., and Niu, G. (2016). “Role of the plant factory with artificial lighting (PFAL) in urban areas,” in Plant Factory: an Indoor Vertical Farming System for Efficient Quality Food Production, eds T. Kozai, G. Niu and M. Takagaki (Cambridge: Academic Press), 7–33.
Krikser, T., Zasada, I., and Piorr, A. (2019). Socio-economic viability of urban agriculture—a comparative analysis of success factors in Germany. Sustainability 11:1999. 10.3390/su11071999 doi: 10.3390/su11071999
La Malfa, G., Branca, F., Tribulato, A., and Romano, D. (2009). New trends in Mediterranean urban vegetable gardening. Acta Hortic. 881, 131–136. doi: 10.17660/ActaHortic.2010.881.13
La Rosa, D., Barbarossa, L., Privitera, R., and Martinico, F. (2014). Agriculture and the city: a method for sustainable planning of new forms of agriculture in urban contexts. Land Use Policy 41, 290–303. doi: 10.1016/j.landusepol.2014.06.014
Lal, R. (2020). Home gardening and urban agriculture for advancing food and nutritional security in response to the COVID-19 pandemic. Food Secur. 12, 1–6. doi: 10.1007/s12571-020-01058-3
Langemeyer, J., and Latkowska, M. J. (2016). “Ecosystem services from urban gardens,” in Urban Allotment Gardens in Europe, eds S. Bell, R. Fox-Kämper, N. Keshavarz, M. Benson, S. Caputo and S. Noori (London: Routledge), 137–163.
Lanner, J., Kratschmer, S., Petrović, B., Gaulhofer, F., Meimberg, H., and Pachinger, B. (2019). City dwelling wild bees: how communal gardens promote species richness. Urban Ecosyst. 23, 271–288. doi: 10.1007/s11252-019-00902-5
Lawson, L. (2004). The planner in the garden: a historical view into the relationship between planning and community gardens. J. Plan. Hist. 3, 151–176. doi: 10.1177/1538513204264752
Le, N. P., and Dung, N. M. (2018). Multifunctionality of peri-urban agriculture: a case study in Trau Quy Commune, Hanoi City. Int. J. Rural Dev. 2, 8–19. doi: 10.22161/ijreh.2.4.2
Lebuhn, G., Droege, S., Connor, E. F., Gemmill-Herren, B., Potts, S. G., Minckley, R. L., et al. (2013). Detecting insect pollinator declines on regional and global scales. Conserv. Biol. 27, 113–120. doi: 10.1111/j.1523-1739.2012.01962.x
Lelièvre, A., and Clérino, P. (2018). “Developing a tool to evaluate the sustainability of intra-urban farms,” in 13th European International Farming Systems Association (IFSA) Symposium, Farming Systems: Facing Uncertainties and Enhancing Opportunities (Chania). Available online at: https://hal.archives-ouvertes.fr/hal-02318311/document (accessed July 15, 2018).
Li, F., Wichmann, K., and Otterpohl, R. (2009). Evaluation of appropriate technologies for grey water treatments and reuses. Water Sci. Technol. 59, 249–260. doi: 10.2166/wst.2009.854
Li, H., Shi, W. Y., Shaob, H. B., and Shaob, M. A. (2009). The remediation of the lead-polluted garden soil by natural zeolite, J. Hazard. Mater. 169, 1106–1111. doi: 10.1016/j.jhazmat.2009.04.067
Liaros, S., Botsis, K., and Xydis, G. (2016). Technoeconomic evaluation of urban plant factories: the case of basil (Ocimum basilicum). Sci. Total Environ. 554, 218–227. doi: 10.1016/j.scitotenv.2016.02.174
Lin, B. B., Philpott, S. M., and Jha, S. (2015). The future of urban agriculture and biodiversity-ecosystem services: challenges and next steps. Basic Appl. Ecol. 16, 189–201. doi: 10.1016/j.baae.2015.01.005
Liu, M., Mattson, R. H., and Kim, E. (2004). Influences of lavender fragrance and cut flower arrangements on cognitive performance. Int. J. Aromat. 14, 169–174. doi: 10.1016/j.ijat.2004.09.015
Liu, S. (2015). Business characteristics and business model classification in urban agriculture [Dissertation/Master's thesis]. Wageningen: Wageningen University and Research Centre.
Liu, T., Yang, M., Han, Z., and Ow, D. W. (2016). Rooftop production of leafy vegetables can be profitable and less contaminated than farm-grown vegetables. Agron. Sustain. Dev. 36:41. doi: 10.1007/s13593-016-0378-6
Loram, A., Warren, P. H., and Gaston, K. J. (2008). Urban domestic gardens (XIV): the characteristics of gardens in five cities. Environ. Manag. 42, 361–376. doi: 10.1007/s00267-008-9097-3
Love, D. C., Uhl, M. S., and Genello, L. (2015). Energy and water use of a small-scale raft aquaponics system in Baltimore, Maryland, United States. Aquacult. Eng. 68, 19–27. doi: 10.1016/j.aquaeng.2015.07.003
Lupia, F., Baiocchi, V., Lelo, K., and Pulighe, G. (2017). Exploring rooftop rainwater harvesting potential for food production in urban areas. Agriculture 7:46. doi: 10.3390/agriculture7060046
Magrefi, F., Geoffriau, E., Kahane, R., Pölling, B., Orsini, F., Pennisi, G., et al. (2018). Training pioneering entrepreneurs in urban agriculture: a model of curriculum based on the URBAN GREEN TRAIN project experience. Acta Hortic. 1215, 433–438. doi: 10.17660/ActaHortic.2018.1215.78
Mallinger, R. E., Gaines-Day, H. R., and Gratton, C. (2017). Do managed bees have negative effects on wild bees?: A systematic review of the literature. PLoS ONE 12:e0189268. doi: 10.1371/journal.pone.0189268
Mancarella, S., Pennisi, G., Gasperi, D., Loges, V., do Nascimento, C. W. A., Vittori Antisari, L., et al. (2017). Metal(oid)s contamination in rural and urban vegetable gardens of Teresina (Brazil). Acta Hortic. 1189, 465–468. doi: 10.17660/ActaHortic.2017.1189.92
Mancarella, S., Pennisi, G., Gasperi, D., Marchetti, L., Loges, V., Orsini, F., et al. (2016). Antimony accumulation risk in lettuce grown in Brazilian urban gardens. EQA Intern. J. Environ. Qual. 20, 35–47. doi: 10.6092/issn.2281-4485/6306
Marchetti, L., Piovene, C., Cesarali, A., Bertocchi, I., Orsini, F., and Gianquinto, G. (2015). “Greenhousing”: integrating low-input simplified hydroponics for roof gardening in bologna's public housing. Acta Hortic. 1093, 99–106. doi: 10.17660/ActaHortic.2015.1093.10
Martin, M., and Molin, E. (2019). Environmental assessment of an urban vertical hydroponic farming system in Sweden. Sustainability 11:4124. doi: 10.3390/su11154124
Martin, M., Poulikidou, S., and Molin, E. (2019). Exploring the environmental performance of urban symbiosis for vertical hydroponic farming. Sustainability 11:6724. doi: 10.3390/su11236724
Mason, B., Rufí-Salís, M., Parada, F., Gabarrell, X., and Gruden, C. (2019). Intelligent urban irrigation systems: Saving water and maintaining crop yields. Agric. Water Manag. 226:105812. doi: 10.1016/j.agwat.2019.105812
Masson-Minock, M., and Stockmann, D. (2010). Creating a legal framework for urban agriculture: lessons from Flint, Michigan. J. Agric. Food Syst. Commun. Dev. 1, 91–104. doi: 10.5304/jafscd.2010.012.007
Matteson, K. C., Ascher, J. S., and Langellotto, G. A. (2008). Bee richness and abundance in New York City urban gardens. Ann. Entomol. Soc. Am. 101, 140–150. doi: 10.1603/0013-8746(2008)101[140:BRAAIN]2.0.CO;2
Mattson, R. H. (2010). Biofeedback evidence of social and psychological health benefits provided by plants and flowers in urban environments. Acta Hortic. 881, 751–757. doi: 10.17660/ActaHortic.2010.881.124
McCallum, B., and Benjamin, A. (2012). Bees in the City: the Urban Beekeepers' Handbook. London: Guardian Society.
McClintock, N. (2010). Why farm the city? Theorizing urban agriculture through a lens of metabolic rift. Cambridge J. Reg. Econ. Soc. 3, 191–207. doi: 10.1093/cjres/rsq005
McClintock, N., Cooper, J., and Khandeshi, S. (2013). Assessing the potential contribution of vacant land to urban vegetable production and consumption in Oakland, California. Landscape Urban Plan. 111, 46–58. doi: 10.1016/j.landurbplan.2012.12.009
McDougall, R., Kristiansen, P., and Rader, R. (2019). Small-scale urban agriculture results in high yields but requires judicious management of inputs to achieve sustainability. Proc. Natl Acad. Sci U.S.A. 116, 129–134. doi: 10.1073/pnas.1809707115
McDougall, R., Rader, R., and Kristiansen, P. (2020). Urban agriculture could provide 15% of food supply to Sydney, Australia, under expanded land use scenarios. Land Use Policy 94:104554. doi: 10.1016/j.landusepol.2020.104554
McGlone, P., Dobson, B., Dowler, E., and Nelson, M. (1999). Food Projects and How They Work. York: York Publishing Services Ltd.
Meenar, M. R., and Hoover, B. M. (2012). Community food security via urban agriculture: Understanding people, place, economy, and accessibility from a food justice perspective. J. Agric. Food Syst. Commun. Dev. 3, 143–160. doi: 10.5304/jafscd.2012.031.013
Megson, D., Dack, S., and Moore, M. (2011). Limitations of the CLEA model when assessing human health risks from dioxins and furans in soil at an allotments site in Rochdale, NW England. J. Environ. Monitor. 13, 1983–1990. doi: 10.1039/c1em10096c
Meharg, A. A. (2016). Perspective: city farming needs monitoring. Nature 531:S60. doi: 10.1038/531S60a
Meneghello, F., Marcassa, G., Koch, I., Sgaravatti, P., Piccolomini, B., Righetto, C., et al. (2016). Garden therapy in neurorehabilitation: well-being and skills improvement. Acta Hortic. 1121, 13–18. doi: 10.17660/ActaHortic.2016.1121.3
Miller, C. (2003). In the sweat of our brow: citizenship in American domestic practice during WWII—victory gardens. J. Am. Cult. 26, 395–409. doi: 10.1111/1542-734X.00100
Mitchell, R. G., Spliethoff, H. M., Ribaudo, L. N., Lopp, D. M., Shayler, H. A., Marquez-Bravo, L. G., et al. (2014). Lead (Pb) and other metals in New York city community garden soils: factors influencing contaminant distributions. Environ. Pollut. 187, 162–169. doi: 10.1016/j.envpol.2014.01.007
Mlcek, J., and Rop, O. (2011). Fresh edible flowers of ornamental plants–a new source of nutraceutical foods. Trends Food Sci. Technol. 22, 561–569. doi: 10.1016/j.tifs.2011.04.006
Mok, H. F., Williamson, V. G., Grove, J. R., Burry, K., Barker, S. F., and Hamilton, A. J. (2014). Strawberry fields forever? Urban agriculture in developed countries: a review. Agron. Sustain. Dev. 34, 21–43. doi: 10.1007/s13593-013-0156-7
Molle, F., and Berkoff, J. (2009). Cities versus agriculture: a review of intersectoral water re-allocation. Nat. Resour. Forum 33, 6–18. doi: 10.1111/j.1477-8947.2009.01204.x
Mougeot, L. J. (2000). Urban Agriculture: Definition, Presence, Potentials and Risks, and Policy Challenges. Cities Feeding People Series. Rept. 31. Available online at: https://idl-bnc-idrc.dspacedirect.org/bitstream/handle/10625/26429/117785.pdf?sequence=12 (accessed October 27, 2020).
Nadal, A., Llorach-Massana, P., Cuerva, E., López-Capel, E., Montero, J. I., Josa, A., Rieradevall, J., and Royapoor, M. (2017). Building-integrated rooftop greenhouses: An energy and environmental assessment in the mediterranean context. Appl. Energy 187, 338–351. doi: 10.1016/j.apenergy.2016.11.051
Nasr, J., and Komisar, J. (2014). “Displaying urban agriculture: from garden city to carrot city,” in Exhibitions and the Development of Modern Planning Culture, eds R. Freestone and M. Amati (Farnham: Ashgate Publishing Limited), 277–292.
Nasr, J., Komisar, J., and De Zeeuw, H. (2017). “The status and challenges of rooftop agriculture – a Panorama of rooftop agriculture types,” in Rooftop Urban Agriculture, eds F. Orsini, M. Dubbeling, H. de Zeeuw and G. Gianquinto (Cham: Springer), 9–29.
Nelkin, J., and Caplow, T. (2007). Sustainable controlled environment agriculture for urban areas. Acta Hortic. 801, 449–456. doi: 10.17660/ActaHortic.2008.801.48
Ochoa, J., Sanyé-Mengual, E., Specht, K., Fernandez, J. A., Banon, S., Orsini, F., et al. (2019). Sustainable community gardens require social engagement and training: a users' needs analysis in Europe. Sustainability 11:3978. doi: 10.3390/su11143978
Okvat, H. A., and Zautra, A. J. (2014). “Sowing seeds of resilience: community gardening in a post-Disaster context,” in Greening in the Red Zone. Disaster, Resilience and Community Greening, eds K. G. Tidball, and M. E. Krasny (Dordrecht: Springer), 73–90.
Opher, T., Friedler, E., and Shapira, A. (2018). Comparative life cycle sustainability assessment of urban water reuse at various centralization scales. Int J. Life Cycle Assess. 24, 1319–1332. doi: 10.1007/s11367-018-1469-1
Opitz, I., Berges, R., Piorr, A., and Krikser, T. (2016). Contributing to food security in urban areas: differences between urban agriculture and peri-urban agriculture in the Global North. Agric. Hum. Values 33, 341–358. doi: 10.1007/s10460-015-9610-2
Orsini, F., Dubbeling, M., de Zeeuw, H., and Gianquinto, G. (2017). Rooftop Urban Agriculture. Cham: Springer.
Orsini, F., Gasperi, D., Marchetti, L., Piovene, C., Draghetti, S., Ramazzotti, S., et al. (2014). Exploring the production capacity of rooftop gardens (RTGs) in urban agriculture: the potential impact on food and nutrition security, biodiversity and other ecosystem services in the city of Bologna. Food Secur. 6, 781–792. doi: 10.1007/s12571-014-0389-6
Orsini, F., Kahane, R., Nono-Womdim, R., and Gianquinto, G. (2013). Urban agriculture in the developing world. A review. Agron. Sustain. Dev. 33, 695–720. doi: 10.1007/s13593-013-0143-z
Orsini, F., Pennisi, G., Zulfiqar, F., and Gianquinto, G. (2020). Sustainable use of resources in plant factories with artificial lighting (PFALs). Eur. J. Hortic. Sci. 85:297–309. doi: 10.17660/eJHS.2020/85.5.1
O'Sullivan, C. A., Bonnett, G. D., McIntyre, C. L., Hochman, Z., and Wasson, A. P. (2019). Strategies to improve the productivity, product diversity and profitability of urban agriculture. Agric. Syst. 174, 133–144. doi: 10.1016/j.agsy.2019.05.007
Park, H., Kramer, M., Rhemtulla, J. M., and Konijnendijk, C. C. (2019). Urban food systems that involve trees in Northern America and Europe: a scoping review. Urban For. Urban Gree. 45:126360. doi: 10.1016/j.ufug.2019.06.003
Park, S., and Mattson, R. H. (2009). Therapeutic influences of plants in hospital rooms on surgical recovery. Hort. Sci. 44, 102–105. doi: 10.21273/HORTSCI.44.1.102
Park, S., Mattson, R. H., and Kim, E. (2004). Pain tolerance effects of ornamental plants in a simulated hospital patient room. Acta Hortic. 639, 241–247. doi: 10.17660/ActaHortic.2004.639.31
Partalidou, M., and Anthopoulou, T. (2017). Urban allotment gardens during precarious times: from motives to lived experiences. Sociol. Rural. 57, 211–228. doi: 10.1111/soru.12117
Pascale, L., Orsini, F., and Gianquinto, G. (2015). AGRIlive: modular hydroponic online supported gardens for zero km agriculture. Acta Hortic. 1093, 1007–1012. doi: 10.17660/ActaHortic.2015.1093.7
Paucek, I., Appolloni, E., Pennisi, G., Quaini, S., Gianquinto, G., and Orsini, F. (2020). LED Lighting systems for horticulture: business growth and global distribution. Sustainability 12:7516. doi: 10.3390/su12187516
Paül, V., and McKenzie, F. H. (2013). Peri-urban farmland conservation and development of alternative food networks: insights from a case-study area in metropolitan Barcelona (Catalonia, Spain). Land Use Policy 30, 94–105. doi: 10.1016/j.landusepol.2012.02.009
Peck, S. (2003). Towards an integrated green roof infrastructure evaluation for Toronto. Green Roof Infrastruct. Monit. 5, 4–7.
Pennisi, G., Blasioli, S., Cellini, A., Maia, L., Crepaldi, A., Braschi, I., et al. (2019a). Unravelling the role of red:blue LED lights on resource use efficiency and nutritional properties of indoor grown sweet basil. Front. Plant Sci. 10:305. doi: 10.3389/fpls.2019.00305
Pennisi, G., Gasperi, D., Mancarella, S., Vittori Antisari, L., Vianello, G., Orsini, F., et al. (2017). Soilless cultivation in urban gardens for reduced potentially toxic elements (PTEs) contamination risk. Acta Hortic. 1189, 377–382. doi: 10.17660/ActaHortic.2017.1189.72
Pennisi, G., Magrefi, F., Michelon, N., Bazzocchi, G., Maia, L., Orsini, F., et al. (2020b). Promoting Education and Training in Urban Agriculture Building on International Projects at the Research Centre on Urban Environment for Agriculture and Biodiversity. ISHS Acta Horticulturae.
Pennisi, G., Orsini, F., Blasioli, S., Cellini, A., Crepaldi, A., Braschi, I., et al. (2019b). Resource use efficiency of indoor lettuce (Lactuca sativa L.) cultivation as affected by red:blue ratio provided by LED lighting. Nat. Sci. Rep. 9, 1–11. doi: 10.1038/s41598-019-50783-z
Pennisi, G., Orsini, F., Gasperi, D., Mancarella, S., Sanoubar, R., Vittori Antisari, L., et al. (2016). Soilless system on peat reduce trace metals in urban grown food: unexpected evidence for a soil origin of plant contamination. Agron. Sustain. Dev. 36, 1–11. doi: 10.1007/s13593-016-0391-9
Pennisi, G., Orsini, F., Landolfo, M., Pistillo, A., Crepaldi, A., Nicola, S., et al. (2020c). Optimal photoperiod for indoor cultivation of leafy vegetables and herbs. Eur. J. Hortic. Sci. 85, 329–338. doi: 10.17660/eJHS.2020/85.5.4
Pennisi, G., Pistillo, A., Orsini, F., Gianquinto, G., Fernandez, J. A., Crepaldi, C., et al. (2020a). Improved Red and Blue ratio in LED lighting for indoor cultivation of basil. Acta Hortic. 1271, 115–118. doi: 10.17660/ActaHortic.2020.1271.16
Pennisi, G., Sanyé-Mengual, E., Orsini, F., Crepaldi, A., Nicola, S., Ochoa, J., et al. (2019c). Modelling environmental burdens of indoor-grown vegetables and herbs as affected by red and blue LED lighting. Sustainability 11:4063. doi: 10.3390/su11154063
Pérez-Esteban, J., Escolástico, C., Masaguer, A., Vargas, C., and Moliner, A. (2014). Soluble organic carbon and pH of organic amendments affect metal mobility and chemical speciation in mine soils, Chemosphere 103, 164–171. doi: 10.1016/j.chemosphere.2013.11.055
Péron, J. Y., and Geoffriau, E. (2007). Characteristics and sustainable development of peri-urban vegetable production in Europe. Acta Hortic. 762, 159–170. doi: 10.17660/ActaHortic.2007.762.16
Peruccio, P. P., and Vrenna, M. (2019). Design and microalgae. Sustainable systems for cities. AGATHÓN|. Int. J. Art. Des. Educ. 6, 218–227. doi: 10.19229/2464-9309/6212019
Petts, J. (2005). “The economics of urban and peri-urban agriculture.” in CPULs, Continuous Productive Urban Landscapes–Designing Urban Agriculture for Sustainable Cities. eds A. Viljoen, K. Bohn, A. Howe (Oxford: Architectural Press. Elsevier), 65–77.
Piezer, K., Petit-Boix, A., Sanjuan-Delmás, D., Briese, E., Celik, I., Rieradevall, J., et al. (2019). Ecological network analysis of growing tomatoes in an urban rooftop greenhouse. Sci. Total Environ. 651, 1495–1504. doi: 10.1016/j.scitotenv.2018.09.293
Piorr, A., Ravetz, J, and Tosics, I. (2011). Peri-urbanisation in Europe: Towards European Policies to Sustain Urban–Rural Futures. Frederiksberg: Forest and Landscape.
Pölling, B., Alfranca, O., Alves, E., Andersson, G., Branduini, P., Deborah, E. L., et al. (2016a). “Creating added value: societal benefits of urban agriculture,” in Urban Agriculture Europe, eds F. Lohrberg, L. Lička, L. Scazzosi and A. Timpe (Berlin: Jovis Publishers), 92–100.
Pölling, B., Lorleberg, W., Orsini, F., Magrefi, F., Hoekstra, F., Renting, H., et al. (2015). “Business models in Urban Agriculture answering cost pressures and societal needs,” in 2nd International Conference on Agriculture in an Urbanizing Society Reconnecting Agriculture and Food Chains to Societal Needs (Rome).
Pölling, B., Mergenthaler, M., and Lorleberg, W. (2016b). Professional urban agriculture and its characteristic business models in Metropolis Ruhr, Germany. Land Use Policy 58, 366–379. doi: 10.1016/j.landusepol.2016.05.036
Pölling, B., Sroka, W., and Mergenthaler, M. (2017). Success of urban farming's city-adjustments and business models—findings from a survey among farmers in Ruhr Metropolis, Germany. Land Use Policy 69, 372–385. doi: 10.1016/j.landusepol.2017.09.034
Ponizy, L., Fox-Kämper, R., Caputo, S., Cohen, N., Newell, J., Grard, B., et al. (2018). “The FEW-meter an integrative model to measure and improve urban agriculture, shifting it towards circular urban metabolism,” in Proceedings of the ESP Europe Regional Conference Ecosystem Services in a Changing World: Moving from Theory to Practice (San Sebastian).
Portmann, F. T., Siebert, S., and Döll, P. (2010). MIRCA2000—global monthly irrigated and rainfed crop areas around theyear 2000: a new high-resolution data set for agricultural and hydrological modelling. Glob. Biogeochem. Cycles 24. doi: 10.1029/2008GB003435
Poulsen, M. N. (2017). Cultivating citizenship, equity, and social inclusion? Putting civic agriculture into practice through urban farming. Agric. Hum. Values 34, 135–148. doi: 10.1007/s10460-016-9699-y
Poulsen, M. N., McNab, P. R., Clayton, M. L., and Neff, R. A. (2015). A systematic review of urban agriculture and food security impacts in low-income countries. Food Policy 55, 131–146. doi: 10.1016/j.foodpol.2015.07.002
Pourias, J., Duchemin, E., and Aubry, C. (2015). Products from urban collective gardens: food for thought or for consumption? Insights from Paris and Montreal. J. Agric. Food Syst. Commun. Dev. 5, 175–199. doi: 10.5304/jafscd.2015.052.005
Pudup, M. (2008). It takes a garden: cultivating citizen-subjects in organized garden projects. Geoforum 39, 1228–1240. doi: 10.1016/j.geoforum.2007.06.012
Pulighe, G., and Lupia, F. (2019). Multitemporal geospatial evaluation of urban agriculture and (non)-sustainable food self-provisioning in Milan, Italy. Sustainability 11:1846. doi: 10.3390/su11071846
Qiu, G., Li, H., Zhang, Q., Wan, C., Liang, X., and Li, X. (2013). Effects of evapotranspiration on mitigation of urban temperature by vegetation and urban agriculture. J. Integr. Agric. 12, 1307–1315. doi: 10.1016/S2095-3119(13)60543-2
Quagrainie, K. K., Flores, R. M. V., Kim, H. J., and McClain, V. (2018). Economic analysis of aquaponics and hydroponics production in the US Midwest. J. Appl. Aquac. 30, 1–14. doi: 10.1080/10454438.2017.1414009
Reinirkens, P. (1996). Analysis of emissions through traffic volume in roadside soils and their effects on seepage water. Sci. Total Environ. 189, 361–369. doi: 10.1016/0048-9697(96)05232-1
Relf, D. (1992). Human issues in horticulture. HortTechnology 2, 159–171. doi: 10.21273/HORTTECH.2.2.159
Relf, D., and Dorn, S. (1995). Horticulture: meeting the needs of special populations. HortTechnology 5, 94–103. doi: 10.21273/HORTTECH.5.2.94
Renting, H., Hoekstra, F., Dubbeling, M., Geoffriau, E., Kahane, R., Pölling, B., et al. (2016). New Urban Agriculture Initiatives Toward a Mindset Change. Available online at: https://agritrop.cirad.fr/583920/1/UGT_New%20UA%20initiatives%20toward%20mindset%20change_2016.pdf (accessed October 27, 2020).
Reynolds, K., and Cohen, N. (2016). Beyond the Kale: Urban Agriculture and Social Justice Activism in New York City. Athens: University of Georgia Press.
Ricketts, T. H., Regetz, J., Steffan-Dewenter, I., Cunningham, S. A., Kremen, C., Bogdanski, A., et al. (2008). Landscape effects on crop pollination services: are there general patterns? Ecol. Lett. 11, 499–515. doi: 10.1111/j.1461-0248.2008.01157.x
Righetto, C., Gianquinto, G., Orsini, F., Meneghello, F., Marcassa, G., Koch, I., et al. (2016). Realization of a neuro-rehabilitation therapeutic garden: design criteria and horticultural choices. Acta Hortic. 1121, 51–57. doi: 10.17660/ActaHortic.2016.1121.9
Roberts, J. M., Bruce, T. J., Monaghan, J. M., Pope, T. W., Leather, S. R., and Beacham, A. M. (2020). Vertical farming systems bring new considerations for pest and disease management. Ann. Appl. Biol. 176, 226–232. doi: 10.1111/aab.12587
Rogus, S., and Dimitri, C. (2015). Agriculture in urban and peri-urban areas in the United States: highlights from the census of agriculture. Renew. Agr. Food Syst. 30, 64–78. doi: 10.1017/S1742170514000040
Rosol, M. (2010). Public participation in post-Fordist urban green space governance: The case of community gardens in Berlin. Int. J. Urban Reg. Res. 34, 548–563. doi: 10.1111/j.1468-2427.2010.00968.x
Rufí-Salís, M., Petit-Boix, A., Villalba, G., Sanjuan-Delmás, D., Parada, F., Ercilla-Montserrat, M., et al. (2020). Recirculating water and nutrients in urban agriculture: an opportunity towards environmental sustainability and water use efficiency? J. Clean. Prod. 261, 121213. doi: 10.1016/j.jclepro.2020.121213
Sala, S., Benini, L., Beylot, A., Castellani, V., Cerutti, A., Corrado, S., et al. (2019). Consumption and Consumer Footprint: Methodology and Results. Indicators and Assessment of the Environmental Impact of European Consumption. Luxembourg: Publications Office of the European Union.
Saldivar-Sali, A. N. D. (2010). A global typology of cities: classification tree analysis of urban resource consumption [Doctoral dissertation], Massachusetts Institute of Technology.
Saldivar-Tanaka, L., and Krasny, M. E. (2004). Culturing community development, neighborhood open space, and civic agriculture: the case of Latino community gardens in New York City. Agric. Hum. Values 21, 399–412. doi: 10.1023/B:AHUM.0000047207.57128.a5
Sanjuan-Delmás, D., Llorach-Massana, P., Nadal, A., Ercilla-Montserrat, M., Muñoz, P., Montero, J. I., et al. (2018). Environmental assessment of an integrated rooftop greenhouse for food production in cities. J. Clean. Prod. 177, 326–337. doi: 10.1016/j.jclepro.2017.12.147
Sanyé-Mengual, E., Cerón-Palma, I., Oliver-Sol,à, J., Montero, J. I., and Rieradevall, J. (2013). Environmental analysis of the logistics of agricultural products from roof top greenhouses in Mediterranean urban areas. J. Sci. Food Agric. 93, 100–109. doi: 10.1002/jsfa.5736
Sanyé-Mengual, E., Cerón-Palma, I., Oliver-Sol,à, J., Montero, J. I., and Rieradevall, J. (2015c). Integrating horticulture into cities: a guide for assessing the implementation potential of Rooftop Greenhouses (RTGs) in industrial and logistics parks. J. Urban Aff. 22, 87–111. doi: 10.1080/10630732.2014.942095
Sanyé-Mengual, E., Gasperi, D., Michelon, N., Orsini, F., Ponchia, G., and Gianquinto, G. (2018a). Eco-Efficiency assessment and food security potential of home gardening: a case study in Padua, Italy. Sustainability 10:2124. doi: 10.3390/su10072124
Sanyé-Mengual, E., Martinez-Blanco, J., Finkbeiner, M., Cerdà, M., Camargo, M., Ometto, A. R., et al. (2018d). Urban horticulture in retail parks: Environmental assessment of the potential implementation of rooftop greenhouses in European and South American cities. J. Clean. Prod. 172, 3081–91. doi: 10.1016/j.jclepro.2017.11.103
Sanyé-Mengual, E., Oliver-Sol,à, J., Montero, J. I., and Rieradevall, J. (2015a). An environmental and economic life cycle assessment of rooftop greenhouse (RTG) implementation in Barcelona, Spain. Assessing new forms of urban agriculture from the greenhouse structure to the final product level. Int. J. Life Cycle Assess. 20, 350–366. doi: 10.1007/s11367-014-0836-9
Sanyé-Mengual, E., Orsini, F., and Gianquinto, G. (2018b). Revisiting the sustainability concept of urban food production from a stakeholders' perspective. Sustainability 10:2175. doi: 10.3390/su10072175
Sanyé-Mengual, E., Orsini, F., Oliver-Sol,à, J., Rieradevall, J., Montero, J. I., and Gianquinto, G. (2015b). Techniques and crops for efficient rooftop gardens in Bologna, Italy. Agron. Sustain. Dev. 35, 1477–1488. doi: 10.1007/s13593-015-0331-0
Sanyé-Mengual, E., Specht, K., Grapsa, E., Orsini, F., and Gianquinto, G. (2019). How can innovation in urban agriculture contribute to sustainability? A characterization and evaluation study from five Western European cities. Sustainability 11:4221. doi: 10.3390/su11154221
Sanyé-Mengual, E., Specht, K., Krikser, T., Vanni, C., Pennisi, G., Orsini, F., et al. (2018c). Social acceptance and perceived ecosystem services of urban agriculture in southern Europe: the case of Bologna, Italy. PLoS ONE 13:e0200993. doi: 10.1371/journal.pone.0200993
Shackleton, C. M., Hurley, P. T., Dahlberg, A. C., Emery, M. R., and Nagendra, H. (2017). Urban foraging: a ubiquitous human practice overlooked by urban planners, policy, and research. Sustainability 9:1884. doi: 10.3390/su9101884
Shoemaker, C. A., and Diehl, E. R. M. (2010). The practice and profession of horticultural therapy in the United States. Acta Hortic. 954, 161–163. doi: 10.17660/ActaHortic.2012.954.20
Shrestha, P., Small, G. E., and Kay, A. (2020). Quantifying nutrient recovery efficiency and loss from compost-based urban agriculture. PLoS ONE 15:e0230996. doi: 10.1371/journal.pone.0230996
Shrewsbury, P. M., and Leather, S. R. (2012). “Using biodiversity for pest suppression in urban landscapes,” in Biodiversity and Insect Pests: Key issues for Sustainable Management, eds G. M. Gurr, S. D. Wratten, and W. E. Snyder (Hoboken, NJ: Wiley-Blackwell), 293–308.
Smith, C. M., and Kurtz, H. E. (2003). Community gardens and politics of scale in New York City. Geogr. Rev. 93, 193–212. doi: 10.1111/j.1931-0846.2003.tb00029.x
Smith, D. J. (1998). Horticultural therapy: the garden benefits everyone. J. Psychosoc. Nurs. Ment. Health Serv. 36, 14–21. doi: 10.3928/0279-3695-19981001-12
Son, J. E., Kim, H. J., and Ahn, T. I. (2016). “Hydroponic systems,” in Plant Factory: an Indoor Vertical Farming System for Efficient Quality Food Production, eds T. Kozai, G. Niu and M. Takagaki (Cambridge: Academic Press), 213–221.
Song, S., Arora, S., Laserna, A. K. C., Shen, Y., Thian, B. W., Cheong, J. C., and Ok, Y. S. (2020). Biochar for urban agriculture: impacts on soil chemical characteristics and on Brassica rapa growth, nutrient content and metabolism over multiple growth cycles. Sci. Total Environ. 727:138742. doi: 10.1016/j.scitotenv.2020.138742
Specht, K., Reynolds, K., and Sanyé-Mengual, E. (2017). Community and social justice aspects of rooftop agriculture. in Rooftop Urban Agriculture, eds F. Orsini, M. Dubbeling, H. de Zeeuw and G. Gianquinto (Cham: Springer), 277–290.
Specht, K., Siebert, R., Thomaier, S., Freisinger, U. B., Sawicka, M., Dirich, A., et al. (2015). Zero-acreage farming in the city of Berlin: an aggregated stakeholder perspective on potential benefits and challenges. Sustainability 7, 4511–4523. doi: 10.3390/su7044511
Specht, K., Weith, T., Swoboda, K., and Siebert, R. (2016). Socially acceptable urban agriculture businesses. Agron. Sustain. Dev. 36:17. doi: 10.1007/s13593-016-0355-0
Sperling, C. D., and Lortie, C. J. (2010). The importance of urban backgardens on plant and invertebrate recruitment: a field microcosm experiment. Urban Ecosyst. 13, 223–235. doi: 10.1007/s11252-009-0114-y
Steenbergen, C. M., and Reh, W. (2003). Architecture and Landscape: the Design Experiment of the Great European Gardens and Landscapes. Berlin: Birkhäuser.
Steglich, A., Bürgow, G., and Million, A. (2020). “Optimising aquaculture/aquaponics in urban agriculture: developing rooftop water farms” in Achieving Sustainable Urban Agriculture, 408. Available online at: https://shop.bdspublishing.com/store/bds/detail/product/3-190-9781786766441
Sugimoto, M., Cho, H., and Mattson, R. H. (2006). Bio-monitoring human heart rate and caloric expenditure responses to horticultural activity. J. Ther. Hortic. 16, 20–27. Available online at: https://www.ahta.org/journal-of-therapeutic-horticulture-16—2005
Tang, H., Liu, Y., and Huang, G. (2019). Current status and development strategy for community-supported agriculture (CSA) in China. Sustainability 11:3008. doi: 10.3390/su11113008
Tang, J. C., Zhu, W. Y., Kookana, R., and Katayama, A. (2013). Characteristics of biochar and its application in remediation of contaminated soil. J. Biosci. Bioeng. 116, 653–659. doi: 10.1016/j.jbiosc.2013.05.035
Taylor, J. R., and Taylor Lovell, S. (2014). Urban home food gardens in the Global North: research traditions and future directions. Agric. Hum. Values 31, 285–305. doi: 10.1007/s10460-013-9475-1
TEEB (2010). The Economics of Ecosystems and Biodiversity: Ecological and Economic Foundations. London: Routledge.
Tei, F., Benincasa, P., Farneselli, M., and Caprai, M. (2009). allotment gardens for senior citizens in Italy: current status and technical proposals. Acta Hortic. 881, 91–96. doi: 10.17660/ActaHortic.2010.881.8
Teig, E., Amulya, J., Bardwell, L., Buchenau, M., Marshall, J., and Jill, S. (2009). Collective efficacy in Denver, Colorado: strengthening neighbourhoods and health through community gardens. Health Place 15, 1115–1122. doi: 10.1016/j.healthplace.2009.06.003
Thebo, A. L., Drechsel, P., and Lambin, E. F. (2014). Global assessment of urban and peri-urban agriculture: irrigated and rainfed croplands. Environ. Res. Lett. 9:114002. doi: 10.1088/1748-9326/9/11/114002
Thomaier, S., Specht, K., Henckel, D., Dierich, A., Siebert, R., Freisinger, U. B., et al. (2015). Farming in and on urban buildings: present practice and specific novelties of zero-acreage farming (ZFarming). Renew. Agric. Food Syst. 30, 43–54. doi: 10.1017/S1742170514000143
Thrupp, L. A. (2000). Linking agricultural biodiversity and food security: the valuable role of agrobiodiversity for sustainable agriculture. Int. Aff. 76, 283–297. doi: 10.1111/1468-2346.00133
Thwaites, K., Helleur, E., and Simkins, I. (2005). Restorative urban open space: Exploring the spatial configuration of human emotional fulfilment in urban open space. Landsc. Res. 30, 525–548. doi: 10.1080/01426390500273346
Tornaghi, C. (2012). “Public space, urban agriculture and the grassroots creation of new commons: lessons and challenges for policy makers,” in Sustainable Food Planning: Evolving Theory and Practice, eds A. Viljoen and S. C. Johannes (Wageningen: Wageningen Academic Publishers), 3–28.
Tornaghi, C. (2014). Critical geography of urban agriculture. Prog. Hum. Geogr. 38, 551–567. doi: 10.1177/0309132513512542
Tornaghi, C. (2017). Urban agriculture in the food-disabling city: (re)-defining urban food justice, reimagining a politics of empowerment. Antipode 49, 781–801. doi: 10.1111/anti.12291
Tresch, S., Frey, D., Le Bayon, R. C., Zanetta, A., Rasche, F., Fliessbach, A., et al. (2019). Litter decomposition driven by soil fauna, plant diversity and soil management in urban gardens. Sci. Total Environ. 658, 1614–1629. doi: 10.1016/j.scitotenv.2018.12.235
Turner, B. (2011). Embodied connections: sustainability, food systems and community gardens. Local Environ. 16, 509–522. doi: 10.1080/13549839.2011.569537
Ukwattage, N. L., Ranjith, P. G., and Bouazza, M. (2013). The use of coal combustion fly ash as a soil amendment in agricultural lands (with comments on its potential to improve food security and sequester carbon). Fuel 109, 400–408. doi: 10.1016/j.fuel.2013.02.016
Ulrich, R. S. (1984). View through a window may influence recovery from surgery. Science 224, 420–421. doi: 10.1126/science.6143402
Ulrich, R. S., Simons, R. F., Losito, B. D., Miles, M. A., and Zelson, M. (1991). Stress recovery during exposure to natural and urban environments. J. Environ. Psychol. 11, 210–230. doi: 10.1016/S0272-4944(05)80184-7
United Nations (2020). World Economic Situation and Prospects. United Nations publication. Available online at: https://www.un.org/development/desa/dpad/wp.content/uploads/sites/45/WESP2020_FullReport.pdf
van der Schans, J. W. (2010). Urban agriculture in the Netherlands. Urban Agric. Mag. 24, 40–42. Available online at: https://ruaf.org/what-we-do/urban-agriculture-magazine/
van der Schans, J. W., Lorleberg, W., Alfranca-Burriel, O., Alves, E., Andersson, G., Branduini, P., et al. (2016). “It is a business! business models in urban agriculture,” in Urban Agriculture Europe, eds F. Lohrberg, L. Lička, L. Scazzosi and A. Timpe (Berlin: Jovis Publishers), 82–91.
van der Schans, J. W., and Wiskerke, J. S. C. (2012). “Urban agriculture in developed economies,” in Sustainable Food Planning: Evolving Theory and Practice, eds A. Viljoen and S. C. Johannes (Wageningen: Wageningen Academic Publishers), 245–258.
Vejre, H., Eiter, S., Hernandez-Jimenez, V., Lohrberg, F., Loupa-Ramos, I., Recasens, X., et al. (2016). “Can agriculture be urban?,” in Urban Agriculture Europe, eds F. Lohrberg, L. Lička, L. Scazzosi and A. Timpe (Berlin: Jovis Publishers), 18–21.
Vitiello, D., and Wolf-Powers, L. (2014). Growing food to grow cities? The potential of agriculture for economic and community development in the urban United States. Commun. Dev. J. 49, 508–523. doi: 10.1093/cdj/bst087
Vittori Antisari, L., Orsini, F., Marchetti, L., Vianello, G., and Gianquinto, G. (2015). Heavy metal accumulation in vegetables grown in urban gardens. Agron. Sustain. Dev. 35, 1139–1147. doi: 10.1007/s13593-015-0308-z
Vogl, C. R., Axmann, P., and Vogl-Lukasser, B. (2003). Urban organic farming in Austria with the concept of Selbsternte (“self-harvest”): an agronomic and socio-economic analysis. Renew. Agric. Food Syst. 19, 67–79. doi: 10.1079/RAFS200062
Wakefield, S., Yeudall, F., Taron, C., Reynolds, J., and Skinner, A. (2007). Growing urban health: community gardening in South-East Toronto. Health Promot. Int. 22, 92–101. doi: 10.1093/heapro/dam001
Walker, S. (2016). Urban agriculture and the sustainability fix in Vancouver and Detroit. Urban Geogr. 37, 163–182. doi: 10.1080/02723638.2015.1056606
Wästfelt, A., and Zhang, Q. (2016). Reclaiming localisation for revitalising agriculture: a case study of peri-urban agricultural change in Gothenburg Sweden. J. Rural Stud. 47, 172–185. doi: 10.1016/j.jrurstud.2016.07.013
Wells, N. (2000). At home with nature: effects of “greenness” on children's cognitive functioning. Environ. Behav. 32, 775–795. doi: 10.1177/00139160021972793
Winkler, K., Wäckers, F. L., Termorshuizen, A. J., and van Lenteren, J. C. (2010). Assessing risks and benefits of floral supplements in conservation biological control. BioControl 55, 719–727. doi: 10.1007/s10526-010-9296-8
Wong, N. H., Cheong, D. K. W., Yan, H., Soh, J., Ong, C. L., and Sia, A. (2003). The effects of rooftop garden on energy consumption of a commercial building in Singapore. Energy Build. 35, 353–64. doi: 10.1016/S0378-7788(02)00108-1
Wortman, S. E., and Lovell, S. T. (2013). Environmental challenges threatening the growth of urban agriculture in the United States. J. Environ. Qual. 42, 1283–1294. doi: 10.2134/jeq2013.01.0031
Wunder, S. (2013). Learning for Sustainable Agriculture: Urban Gardening in Berlin. Ecologic, Berlin. Available online at: https://www.ecologic.eu/sites/files/publication/2014/wunder-13-learning-for-sustainable-agriculture-urban-gardening-in-berlin.pdf (accessed October 27, 2020).
Yang, Z., Cai, J., and Sliuzas, R. (2010). Agro-tourism enterprises as a form of multi-functional urban agriculture for peri-urban development in China. Habitat Int. 34, 374–385. doi: 10.1016/j.habitatint.2009.11.002
Yi-Zhang, C., and Zhangen, Z. (2000). “Shanghai: trends towards specialised and capital intensive urban agriculture,” in Growing Cities Growing Food: Urban Agriculture on the Policy Agenda, eds N. Bakker, M. Dubbeling, S. Guendel, U. Sabel Koschella, H. de Zeeuw (Feldafing: Deutsche stiftung für internationale entwicklung), 467–475.
Yoshida, S., Yagi, H., Kiminami, A., and Garrod, G. (2019). Farm diversification and sustainability of multifunctional peri-urban agriculture: entrepreneurial attributes of advanced diversification in Japan. Sustainability 11:2887. doi: 10.3390/su11102887
Zasada, I. (2011). Multifunctional peri-urban agriculture—a review of societal demands and the provision of goods and services by farming. Land Use Policy 28, 639–648. doi: 10.1016/j.landusepol.2011.01.008
Zhang, X. K., Wang, H. L., He, L. Z., Lu, K. P., Sarmah, A., Li, J. W., et al. (2013). Using biochar for remediation of soils contaminated with heavy metals and organic pollutants. Environ. Sci. Pollut. Res. 20, 8472–8483. doi: 10.1007/s11356-013-1659-0
Keywords: urban horticulture, green infrastructure (GI), vertical farm, rooftop agriculture, ecosystem service, ecology, business models, circular cities
Citation: Orsini F, Pennisi G, Michelon N, Minelli A, Bazzocchi G, Sanyé-Mengual E and Gianquinto G (2020) Features and Functions of Multifunctional Urban Agriculture in the Global North: A Review. Front. Sustain. Food Syst. 4:562513. doi: 10.3389/fsufs.2020.562513
Received: 15 May 2020; Accepted: 20 October 2020;
Published: 16 November 2020.
Edited by:
Gaston Small, University of St. Thomas, United StatesReviewed by:
Theresa Wei Ying Ong, Dartmouth College, United StatesMohsen Aboulnaga, Cairo University, Egypt
Joan Rieradevall, Institut de Ciència i Tecnologia Ambientals (ICTA), Spain
Copyright © 2020 Orsini, Pennisi, Michelon, Minelli, Bazzocchi, Sanyé-Mengual and Gianquinto. This is an open-access article distributed under the terms of the Creative Commons Attribution License (CC BY). The use, distribution or reproduction in other forums is permitted, provided the original author(s) and the copyright owner(s) are credited and that the original publication in this journal is cited, in accordance with accepted academic practice. No use, distribution or reproduction is permitted which does not comply with these terms.
*Correspondence: Francesco Orsini, Zi5vcnNpbmkmI3gwMDA0MDt1bmliby5pdA==