- Plant, Soil and Microbial Sciences Department, Michigan State University, East Lansing, MI, United States
There is widespread recognition that a narrow crop base has inherent vulnerabilities. Crop diversification is one strategy that can help enhance human health, environmental sustainability and resilience of farming communities—yet lock-in mechanisms have mediated against such diversification. This mini-review considers inadvertent negative impacts on crop diversity of policies that favor a few, highly annual crops. Priorities of agricultural research and government institutions such as Public Distribution Systems promote production of a few determinant cereal species, and do not consider the ecosystem service functions associated with diverse growth types (e.g., long duration, indeterminant, and perennial crops). Genetic improvement of fields crops has prioritized short maturity cycles, calorie production, and inadvertently, this may lead to high consumption of water and nutrients. Such crops are highly productive; however, they “lock-in” dependence on fossil fuels and chemical pest regulation. Further, early duration crops have modest root systems, and are short-statured. This limits generation of co-products, such as fodder, fuel wood, leafy vegetables, and soil amelioration. Research gaps and next steps are proposed to address this challenge, including: (1) investigation of adoption barriers and opportunities in order to foster diverse crop growth types and “bright spots” of agroecosystem diversity, (2) changing metrics for assessing system performance, to consider nutrient-enrichment, multipurpose properties and ecosystem services in agricultural policy, and (3) investment in developing perennial and multipurpose grain crops, and plant-facilitated nutrient accessing mechanisms. Enhanced resilience in agriculture requires greater attention to promotion of crop diversity, including functional diversity and socio-economic innovations.
Introduction
Modern, intensified agriculture is highly productive of calories, and provides essential services for many economies. At the same time, environmental costs include the loss of 70% of insect biodiversity in one recent study (Sánchez-Bayo and Wyckhuys, 2019), the wide-spread challenge of nutrient loss from agricultural systems (Bowles et al., 2018), and a sizeable role for this sector in greenhouse gas emissions (Robertson et al., 2000). Agricultural sustainability challenges such as these have risen in tandem with what are increasingly simplified forms of production systems in many parts of the world (Steffen et al., 2015; O'Brien et al., 2019). A handful of crops have come to dominate (Ramankutty et al., 2018). This constricted genetic base is vulnerable to epidemics, and has been shown to reduce biocontrol pest regulation (Landis et al., 2008; Hufford et al., 2019). There are a number of socio-economic factors that reinforce this narrow range of crops, in what has become a “lock-in trap,” as explored here (Oliver et al., 2018). A lock-in trap is a societal situation that is resistant to change, due to high connectivity among factors that reinforce each other through feedback loops, often to the determinant of many stakeholders and environmental sustainability. Examples in the literature include unsustainable use of fisheries and management of resources on agricultural lands in Australia (Allison and Hobbs, 2004; Laborde et al., 2016). I consider here how investment in subsidized markets, and research, may have inadvertently reinforced large-scale production of highly annual, calorie producing crops to the determinant of diversity in crop types.
This mini-review focuses on an overlooked attribute of this lock-in trap, that the small number of crop species that dominate agricultural landscapes today have similar, highly annual growth traits. That is, a short-statured and determinant growth habit dominates modern crop varieties. This is at the expense of maintaining a diversity of multifunctional traits and indeterminant growth types. Modern varieties of row crop species are overwhelming determinant in terms of growth type. For example, tomato [Solanum lycopersicum (L.)] has many perennial and indeterminant traits, as seen in wild relatives; yet over the last 80 years varieties for field crop production have been bred for annual traits (Barrios-Masias and Jackson, 2014). Another example is soybean [Glycine max (L.)], where there is a body of literature documenting through retrospective analysis how plant traits have changed over a century of crop improvement. Plant breeders, from Canada to China, have developed short-statured and high-oil content varieties from multi-use varieties that were grown historically for forage and seed (Bruce et al., 2019). Today modern varieties are highly annual and short in stature (Wang et al., 2016).
It is understandable why plant breeders have entered into the pursuit of maximizing calorie production through developing highly annual traits including high allocation toward reproduction. Yet, there are tradeoffs associated with this headlong pursuit of one plant type, and this includes the neglect of long duration, multipurpose and semi-perennial growth types which have increasingly become marginalized (Snapp et al., 2019b). This is important, because there may well be an increased vulnerability that is an inadvertent consequence of an agricultural food system that relies on a few plant growth types (Lin, 2011). The limited production of co-products such as vegetation that can be used as a forage, and roots for soil enhancement; these are additional inadvertent consequences of privileging annual crop traits. The final section of this paper considers research gaps that could help diversify crop growth types and promote multi-functional agriculture, for enhanced resilience in a rapidly changing world.
Lock-In Trap in India
India provides an instructive example of a socio-ecological system that inadvertently promotes a few crop types, reviewed here to provide context to the on-going controversary associated with simplified production systems, which persist despite their critics. This has a genesis in the Green Revolution (Pingali, 2012). There have been a wide range of policy and technological interventions in India to improve access to high-calorie foods, from public distribution institutional interventions to crop improvement. These will be explored here. A key government policy that impacts crop production patterns is the India Public Distribution System (PDS), and related subsidies (Chakraborty and Sarmah, 2019). The India PDS is a distribution system that moves wheat and rice grain throughout the country, and subsidies access to this food in poverty stricken areas (Saini and Ahlawat, 2019). This has provided a large and consistent market for wheat-rice and rice-rice systems (two cereals per year). One unintended consequence of the privileging of these high calorie producing crops appears to be the decline in production area associated with numerous alternate crops (Figure 1). Causal attribution is not possible here, and many other factors may be important. For example, in India and many countries, production of wheat and rice is highly supported through investment in genetics, agronomic advice and subsidized fertilizer and irrigation.
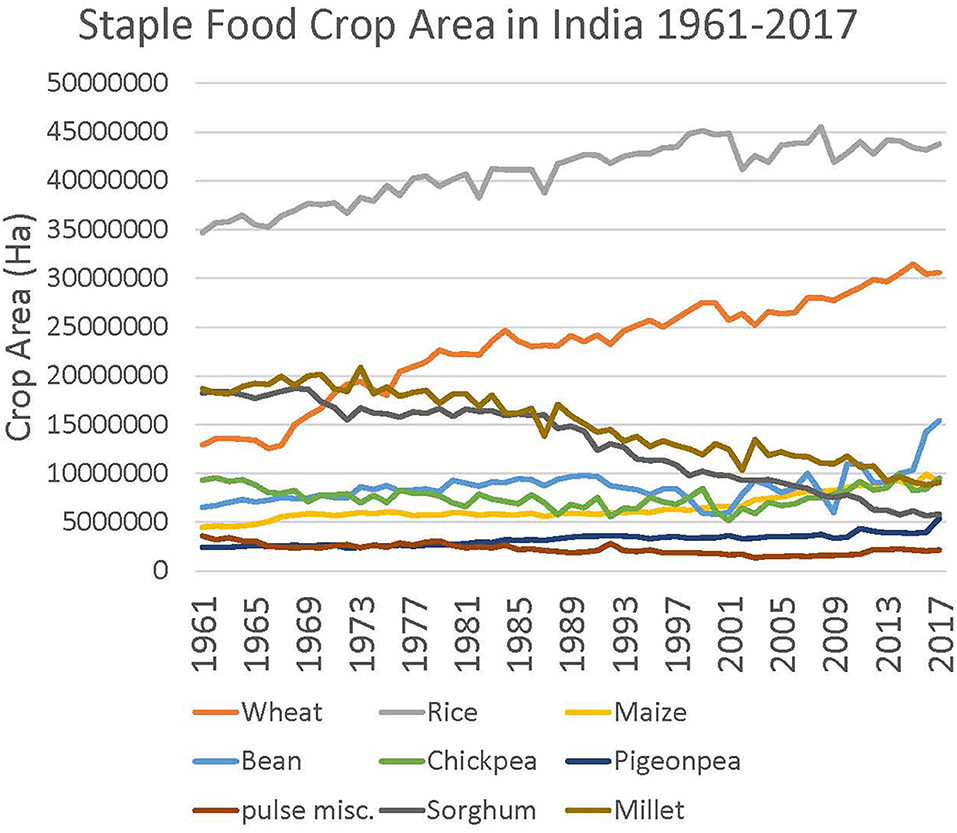
Figure 1. India production area associated with nine major food crop categories (FAOSTAT, 2020).
There are a wide range of policy and institutional innovations associated with the India Public Distribution System. The India PDS redistributes the large volumes of high-calorie grain produced in the Indo-Gangetic plain of Northern India to reach poverty stricken households (Ahluwalia, 1993). The PDS has functioned for over three decades to deliver food, wheat and rice primarily, at highly subsidized prices, to food insecure populations. It has been critiqued as ineffective in achieving that goal and there is a large literature on how to improve the food safety net goals of PDS interventions (Ramaswami and Balakrishnan, 2002; Chakraborty and Sarmah, 2019). At the same time, there has been little attention to the impact of PSD on agricultural production systems and farmer crop choice. One study found that PDS had an impact on the production side of the equation, as this state institution was shown to consistently buy and distribute poor quality grain, relative to wheat grain quality in the private sector (Ramaswami and Balakrishnan, 2002).
Consumer demand also plays a role in the dominance of a few crops. This is in addition to the role of market distortions, as many crops can't compete in terms of being a consumer-favored source of highly inexpensive calories (Davis et al., 2018). That is, many crops that are modest producers of calories have been relegated to “minor” status, such as millet or sorghum. Yet, such crops are nutrient dense, providing important sources of micro-nutrients, and provide other ecosystem services as well, such as conservation of water (deFries et al., 2016). If appreciation of the unique nutritional advantages of a diverse diet were more widespread, this could potentially lead to greater consumer demand. Nutritional education, and appreciation of traditional diets, have been proposed as means to promote minor crops, and indeed has led to resurgence in demand for sorghum in some urban markets (Minnaar et al., 2017; Rao et al., 2017).
The privileging calorie production through a focus on rice and wheat has commendable food security intentions. There is broad consensus of an increasing worldwide demand for calorie, which is associated with ecosystem disservices such as carbon loss (Johnson et al., 2014); however, there are many inefficiencies of current food systems that raise the apparent demand for calories, including that cereals are widely fed to livestock (Ritchie et al., 2018). Further, the calorie production aspect of food security has recently been moderated through attention to the “hidden hunger” associated with requirements for protein and micronutrients (Gödecke et al., 2018).
The net effect of promoting high-calorie producing crops appears to have been the prevention of other crops being sown. As shown in Figure 1, agricultural statistics reflect a steady expansion in wheat and rice production areas across India over the last six decades. At the same time, the area sown in cereals such as millet and sorghum have decreased dramatically. It is instructive to consider the production area of maize, which was once a minor crop in India. This crop is a champion at translating nitrogenous fertilizers into calorie-rich grain, if given sufficient nutrients and water (Sinclair and Horie, 1989). Maize production has grown markedly in recent decades (Figure 1). This is suggestive that it is not just wheat and rice that are favored by policies in India, there may be an overall privileging of crops with the highly-annual growth type that goes with the ability to translate fertilizer into grain, for high calorie production. This has an environmental cost as production of maize is often associated with high use of fertilizers, irrigation, and pesticides (Maggi et al., 2019).
Another case in point is that of pulse production, which has generally stagnated in India. In many markets the price of pulses has climbed, leading to reduced availability and consumption declines among poorer households in India (Rajuladevi, 2001). Pulses have historically been important source of protein. Recent analyses have highlighted that legume crops can play a key role in environmental security as well as promoting human health (Foyer et al., 2016). Yet pulses have often been under-invested in, including limited agricultural research dollars relative to cereals (Pachico, 2014). Pulses such as common bean remain with moderate yield potential and poor grain quality traits such as cooking times and digestibility (Cichy et al., 2015), and a recent review found that investment in genetic improvement of pulses has been almost nil in African agricultural development (Snapp et al., 2019a).
One way out of this lock-in trap in India has been proposed in the literature. It involves an update to the metrics for agricultural system performance (deFries et al., 2015). That is explored by Davis et al. (2018), regarding the impact of a change in agricultural policy to consider nutrition and other ecosystem services, in addition to focusing on calories. Sorghum and millet diversification of Indian rice-wheat systems is explored by these studies, which are suggestive that calories could be maintained, while substantial conservation of water achieved. All of this could be combined with enhanced production of micronutrients if diversified cropping system patterns were supported by the Indian government. These are modeling based estimates, yet they take into account that crop nutritional quality varies greatly with species, and that valuing zinc, iron, calcium, and protein would in turn support high crop diversity. Crop species vary in their impact on natural resources, thus diversification could lessen the “fossil” water withdraws that threaten the sustainability of agriculture in India today (Davis et al., 2018). Environmental services may be related in large part to the genetic variation associated with minor crops, which often include a tremendous diversity of growth types, such as early maturation, as well as long duration (Bezançon et al., 2009). Sorghum genotypes for example include land races which can be grown for 2 or 3 years, through cutting back the stems after the initial harvest, so as to produce deep root systems, soil conservation, and multiple harvests of vegetation for livestock feed, and construction purposes (Rogé et al., 2016).
Lock-in Trap of Simplification in Crop Growth Types
The narrowing of crop species diversity has been accompanied by a reduction in the diversity of functional plant types. Consider for example the grain crops cowpea, pigeonpea, rice, sorghum, and soybean, all of which at one time had tremendous variety in growth type and stature. Cultivars of these species historically included long duration, indeterminant growth habits [e.g., tall-statured types among cereals, and viney, prostrate types among legumes (Rogé et al., 2016; Snapp et al., 2019b)]. Semi-perennial management of sorghum and rice is still occasionally performed, as these crops can be grown as ratoons (Larkin et al., 2014). Yet, crop improvement efforts overwhelming focus on maximizing yield, and selection for plant traits such as determinant, annual growth habit, and a high harvest index (Sinclair, 2019). Harvest index refers to the ratio of commodity biomass (usually grain) that is produced per total plant biomass. A synchronous, determinant growth habit is also favored, which is compatible with mechanized harvest. In sum, traits prioritized in improved, modern varieties of food crops include rapid, early shoot growth, a modest root system, and annualized, highly determinant reproduction.
Grass species are suited to producing large amounts of grain, through early, rapid growth and a starchy endosperm. This has led to cereal production being prioritized by many agricultural policy and crop improvement efforts. Other species produce seed with nutritionally high-quality biochemical traits, which can be metabolically expensive to produce (Tian and Bekkering, 2019). This constrains the yield potential of such crops, as is notable for legume crops which in addition support a symbiotic association with bacteria, at some metabolic expense. A study in Europe found evidence for about 30% higher biomass production in systems that had minimal vs high legume presence (Iannetta et al., 2016). At the same time, nitrogen fertilizer requirements were high for the low legume systems. There may be additional tradeoffs associated with prioritizing plant traits of rapid growth and early maturation, as these can constrain tissue quality due the limited time for uptake and integration of nutrients (Figure 2). Tradeoff expression, however, is expected to vary with crop species, and plant breeding programs. A historical analysis of soybean varieties, for example, found that nitrogen concentration of the seed has remained stable whereas phosphorus concentration has declined (Balboa et al., 2018). The yield potential enhancement of modern maize varieties, on the other hand, has been associated with clear declines in nitrogen concentration (Scott et al., 2006).
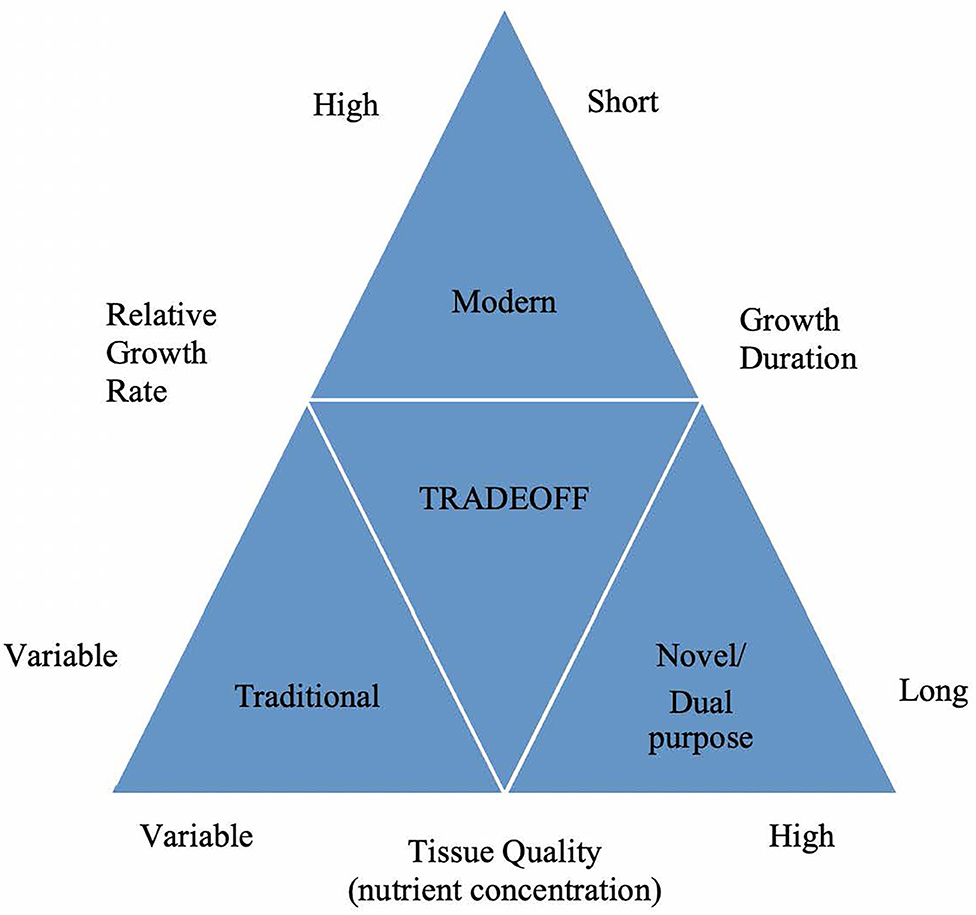
Figure 2. A conceptual diagram of tradeoffs associated with plant traits associated with the rate and duration of growth, and tissue quality. Modern, improved cultivars of food crops are generally associated with relatively rapid growth rates and short duration, to achieve early vigor, and a high crop yield. Traditional varieties vary markedly in relationship to these traits, and novel plant breeding efforts have emphasized long duration of growth for dual purpose use, or high nutrient concentration for biofortification.
Consequences of the Dominance of Rapid Maturation Crops
The emphasis on short-stature, and determinant growth types, continues today. This is indicated by priorities of plant breeders in Africa and South Asia (Snapp et al., 2019b). Modern varieties of pigeopea and sorghum for example have been bred to be as much as a meter shorter than many land races. An annual-centric focus in crop improvement is not consistent with the environmental and market context faced by many small-scale farmers. Indeed, smallholder farms are rarely chemical-intensive and often rely on indeterminacy to tolerate pests, as multiple flowering periods can reduce the negative impact of pest predation (Dube and Fanadzo, 2013). There is need for diversity in crop types. For example, many modern varieties provide traits such as early maturation and high grain yield. Yet, at the same time, smallholder farmers also require tall-statured varieties to escape to some extent grazing by free roaming livestock, and to produce in addition to grain, stems and stalks for fuel, and construction purposes (Orr et al., 2015; Rogé et al., 2017).
Consideration of the impact of a variety grown on the entire farming system is important in the context of smallholder farm livelihoods (McDonald et al., 2019). That is, the impact of a crop species on soil resources, and the stability of associated crops that are frequently grown in mixed production systems. For example, semi-perennial crops in rotation with cereals has been shown to increase the stability of crop production (Snapp et al., 2010; Chimonyo et al., 2019). Further, long duration, tall-statured and indeterminant types of crops produce copious amounts of vegetation which can be used for forage, or soil building purposes, and are valued co-products on many farms (Singh et al., 2003). Farmer-preferred varieties of pigeonpea in Malawi, for example, are associated with production of livestock feed, fuel wood, and soil fertility, as well as grain (Orr et al., 2015; Grabowski et al., 2019). Yet, modern varieties of pigeonpea recently released in Southern and East Africa emphasize short duration and short-stature, with few co-products (Snapp et al., 2019b).
Long duration, and semi-perennial properties have been associated with many traditional varieties of food crops, which often include a diversity of plant growth habits. There is little consideration in the literature of the opportunity costs of prioritizing instead a narrow range of crop growth types in modern crop improvement, that of rapid growth and early maturity. There may be steep tradeoffs with nutrient concentration, as illustrated in Figure 2. Farmer interest in diversity of crop growth types, and functions, is indicated by the persistence around the globe of traditional varieties, and agronomic practices such as polycultures and ratooning (which support multiple harvests from the same plant, and high biomass production). Perennial, indeterminant traits support multiple flushes of flowers and vegetation that can play a key role in resilience to stress, and are associated with deep root systems (Kell, 2012). Root system carbon inputs are an important soil carbon sequestration mechanism. Indeed, perennial and long duration crop traits have been highlighted as the chief means to support soil biological processes, and derived ecosystem services including crop health, nutrient provisioning and water regulation (Rasche et al., 2017).
Discussion
Evidence is growing of the inadvertent consequences of policy, agronomy and modern genetics that privilege short-duration field crops. A recent study in the Great Plains of the USA illustrates this (O'Brien et al., 2019). In tandem with simplification of crops grown to primarily corn and soybean, and associated loss of winter cereals that provided semi-perennial cover, the authors observed marked declines in peak river flows, and substantial increases in chemical inputs for crop production. Further studies of landscape consequences are needed, but it is reasonable to infer that crops that grow fast and have a high harvest index will not have been selected for simultaneous investment in root systems and biochemical properties that repel pests—thus might be expected to require large amounts of nutrients and water, and may be pest susceptible. Maize is clearly a case in point, being globally associated with nitrogenous fertilizer and pesticide use (Maggi et al., 2019).
To bring back ecosystem services in agricultural landscapes there are many efforts to diversify annual field crops through use of cover crops (Snapp et al., 2005). There are also strategies being investigated add diversity to arable fields through agroforestry and plantings of prairie strips (Lin, 2011; Leakey, 2017; Schulte et al., 2017). These are important research areas. However, such approaches focus on diversification in the off-season, or at the margins of fields. The primary land use remains in simplified rotations and monocultures of annual field crops, which constrains opportunities for diversification and for remediation of environmental problems.
A radical approach to expanding the range of growth types cultivated for food has been proposed, that of developing perennial grain crops (Glover et al., 2010; Kane et al., 2016). This strategy remains overall a theoretical concept, in that there are very few examples of perennial grain varieties being released or adopted, and studies have raised concerns about the economics within the current agricultural policy context (Bell et al., 2008; Snapp et al., 2019b). Yet a perennial growth type could potentially deliver a broad range of environmental services and should not be overlooked (Larkin et al., 2014; Sprunger et al., 2019). There are a number of traditional African farming systems that rely to varying degrees on semi-perennial grain crops such as sorghum and pigeonpea that can be ratooned (Rogé et al., 2016). Insufficient calorie production potential has been raised as a critique of perennial grain crops. Yet, there is evidence that photosynthetic capacity can be upregulated, and that this is associated with perennial traits, thus there may be unexplored genetic potential to expand calories produced in perennial crop types (Jaikumar et al., 2013). Maximizing grain yield is not the only goal in agricultural production, as stability of yield and environmental long-term resource conservation are also widely valued, and thus there is growing evidence that mixture of growth types would have value among modern crop varieties, including perennial and semi-perennial traits (Snapp et al., 2019b).
Ways Forward
Three research gaps are identified here, as initial steps to address the lock-in trap of monocultural production. It will also be important to support an enabling environment for crop diversity, which may involve scholars engaged in activism, and private-public partnerships (Jordan et al., 2020). An inspirational example is the Green Lands Blue Waters network in the US Midwest that promotes continuous living cover for a more diverse, sustainable agriculture (https://greenlandsbluewaters.org/). Prairie strip research partnerships and collaborations with farmers is another such example (Atwell et al., 2010). As is legume-based farming, promoted in Europe through participatory networks and attention to the agricultural policy framework (Mawois et al., 2019).
A major research gap is the lack of an evidence-base that documents barriers and opportunities associated with growing diverse crops, and how these operate at different scales, from farm, community, region to national. There are marked declines in production of legume crops, suggestive of steep barriers to production. For example, several recent studies have reviewed the multifunctional roles that legume crops play in Europe, and the societal price paid in the form of fertilizer dependency and associated environmental disservices (Iannetta et al., 2016; Mawois et al., 2019). In an agricultural market context where stable, high grain yields and certainty in access to markets are all important to economic viability of farm enterprises, legume production is often perceived as not meeting any of these goals (Mawois et al., 2019).
There has been limited studies of adoption among species that are categorized as alternative or minor crops. For example, international agricultural centers have invested in a handful of studies on legume variety adoption, in contrast to hundreds on cereal variety adoption (Snapp et al., 2019a). One methodological approach would be to study the “bright spots” where crop diversity has flourished, as well as “dark spots” where diversity is highly limited (Frei et al., 2018).
Research is needed on how to incentivize diversity in agriculture, through performance criteria. Change in the criteria for assessing performance of cropping systems could markedly alter how genetic and agronomic success is judged, and how agricultural policies are framed. The example presented earlier for India is a case in point, diversification of wheat and rice with millet and sorghum crops would be promoted if the political metrics included water conservation or nutrient enriched grain (Davis et al., 2018). The global case for inclusion of legume crops has also been based largely on metrics that consider human health and environmental conservation (Foyer et al., 2016). Valuation of ecosystem services, and consideration of sustainable development goals are major subjects of major research inquiry (Wood et al., 2018), yet there are gaps in terms of specifics and attention to how these could be operationalized to inform agricultual development and policies. Sustainability intensification assessment is one approach that provides a practical example of how to visualize tradeoffs among indicators and assess overall performance and potential contributions of agricultural technologies (Smith et al., 2017).
Research gaps include projects that will require a long-term perspective and a massive investment of resources. This is the nature of efforts to breed crops with new plant traits including perennial growth habits to support ecosystem services (Glover et al., 2010). Diversity of growth types would be maintained if crop breeding efforts also included intermediate multipurpose types [e.g., semi-perennial shrubs and vines (Snapp et al., 2019b)]. Enhanced ratoon ability could for example provide copious amounts of fodder and grain under drought stress, yet this has rarely been researched as part of genetic improvement or agronomic programs. A related area of research is that of developing crops that facilitate associated microbial symbioses that enhance availability of sparingly soluble phosphorus through biologically-enhanced mobilization, that fix substantial amounts of nitrogen, and that promote soil carbon accrual. There are well-documented examples of germplasm that supports all three processes, including in pigeonpea (Cajanus cajan) and lupin (Lupinus albus) (Garland et al., 2018).
In conclusion, crop diversity documentation requires a major effort as an evidence base for all three research gaps. This to characterize phenotypes and utilization in traditional agriculture, to help move forward efforts on multiple fronts. In addition, genetic throughput mechanisms need to be developed for the variety of crop traits discussed here. This requires attention to farmer-valued traits and how to characterize them through participatory breeding, as well as traditional phenotyping. Addressing these and related research gaps should not be overlooked in the search to enhance agricultural ecosystem services and sustainability.
Author Contributions
SS conceptualized, conducted, and wrote the review.
Funding
MSU, including the MSU Ag Bio Research station, provided support for the author of this review.
Conflict of Interest
The author declares that the research was conducted in the absence of any commercial or financial relationships that could be construed as a potential conflict of interest.
Acknowledgments
Thank you to my graduate students who are a never ending source of inspiration, agroecology scholars, colleagues, farmers and friends around the world, and to my amazing wife and family whom put up with a distracted manner when I'm writing, thinking, or trying to do both at once.
References
Ahluwalia, D. (1993). Public distribution of food in India: coverage, targeting and leakages. Food Policy 18, 33–54. doi: 10.1016/0306-9192(93)90095-S
Allison, H. E., and Hobbs, R. J. (2004). Resilience, adaptive capacity, and the “Lock-in Trap” of the Western Australian agricultural region. Ecol. Soc. 9:3. doi: 10.5751/ES-00641-090103
Atwell, R. C., Schulte, L. A., and Westphal, L. M. (2010). How to build multifunctional agricultural landscapes in the US Corn Belt: add perennials and partnerships. Land Use Policy 27, 1082–1090. doi: 10.1016/j.landusepol.2010.02.004
Balboa, G. R., Sadras, V. O., and Ciampitti, I. A. (2018). Shifts in soybean yield, nutrient uptake, and nutrient stoichiometry: a historical synthesis-analysis. Crop Sci. 58, 43–54. doi: 10.2135/cropsci2017.06.0349
Barrios-Masias, F. H., and Jackson, L. E. (2014). California processing tomatoes: morphological, physiological and phenological traits associated with crop improvement during the last 80 years. Eur. J. Agron. 53, 45–55. doi: 10.1016/j.eja.2013.11.007
Bell, L. W., Byrne, F., Ewing, M. A., and Wade, L. J. (2008). A preliminary whole-farm economic analysis of perennial wheat in an Australian dryland farming system. Agric. Syst. 96, 166–174. doi: 10.1016/j.agsy.2007.07.007
Bezançon, G., Pham, J. L., Deu, M., Vigouroux, Y., Sagnard, F., Mariac, C., et al. (2009). Changes in the diversity and geographic distribution of cultivated millet [Pennisetum glaucum (L.) R. Br.] and sorghum [Sorghum bicolor (L.) Moench] varieties in Niger between 1976 and 2003. Genet. Resour. Crop Evol. 56, 223–236. doi: 10.1007/s10722-008-9357-3
Bowles, T. M., Atallah, S. S., Campbell, E. E., Gaudin, A. C., Wieder, W. R., and Grandy, A. S. (2018). Addressing agricultural nitrogen losses in a changing climate. Nat. Sustain. 1, 399–408. doi: 10.1038/s41893-018-0106-0
Bruce, R. W., Grainger, C. M., Ficht, A., Eskandari, M., and Rajcan, I. (2019). Trends in soybean trait improvement over generations of selective breeding. Crop Sci. 59, 1870–1879. doi: 10.2135/cropsci2018.11.0664
Chakraborty, S., and Sarmah, S. P. (2019). India 2025: the public distribution system and national food security act 2013. Dev. Pract. 29, 230–249. doi: 10.1080/09614524.2018.1527290
Chimonyo, V. G. P., Snapp, S. S., and Chikowo, R. (2019). Grain legumes increase yield stability in maize based cropping systems. Crop Sci. 59, 1222–1235. doi: 10.2135/cropsci2018.09.0532
Cichy, K. A., Wiesinger, J. A., and Mendoza, F. A. (2015). Genetic diversity and genome-wide association analysis of cooking time in dry bean (Phaseolus vulgaris L.). Theor. Appl. Genet. 128, 1555–1567. doi: 10.1007/s00122-015-2531-z
Davis, K. F., Chiarelli, D. D., Rulli, M. C., Chhatre, A., Richter, B., Singh, D., et al. (2018). Alternative cereals can improve water use and nutrient supply in India. Sci. Adv. 4:eaao1108. doi: 10.1126/sciadv.aao1108
deFries, R., Fanzo, J., Remans, R., Palm, C., Wood, S., and Anderman, T. L. (2015). Metrics for land-scarce agriculture. Science 349, 238–240. doi: 10.1126/science.aaa5766
deFries, R., Mondal, P., Singh, D., Agrawal, I., Fanzo, J., Remans, R., et al. (2016). Synergies and trade-offs for sustainable agriculture: nutritional yields and climate-resilience for cereal crops in Central India. Glob. Food Secur. 11, 44–53. doi: 10.1016/j.gfs.2016.07.001
Dube, E., and Fanadzo, M. (2013). Maximising yield benefits from dual-purpose cowpea. Food Secur. 5, 769–779. doi: 10.1007/s12571-013-0307-3
FAOSTAT. (2020). Available online at: http://www.fao.org/faostat/en/#home
Foyer, C. H., Lam, H. M., Nguyen, H. T., Siddique, K. H., Varshney, R. K., Colmer, T. D., et al. (2016). Neglecting legumes has compromised human health and sustainable food production. Nat. Plants 2:16112. doi: 10.1038/nplants.2016.112
Frei, B., Renard, D., Mitchell, M. G., Seufert, V., Chaplin-Kramer, R., Rhemtulla, J. M., et al. (2018). Bright spots in agricultural landscapes: identifying areas exceeding expectations for multifunctionality and biodiversity. J. Appl. Ecol. 55, 2731–2743. doi: 10.1111/1365-2664.13191
Garland, G., Bünemann, E. K., Oberson, A., Frossard, E., Snapp, S., Chikowo, R., et al. (2018). Phosphorus cycling within soil aggregate fractions of a highly weathered tropical soil: a conceptual model. Soil Biol. Biochem. 116, 91–98. doi: 10.1016/j.soilbio.2017.10.007
Glover, J. D., Reganold, J. P., Bell, L. W., Borevitz, J., Brummer, E. C., Buckler, E. S., et al. (2010). Increased food and ecosystem security via perennial grains. Science 328, 1638–1639. doi: 10.1126/science.1188761
Gödecke, T., Stein, A. J., and Qaim, M. (2018). The global burden of chronic and hidden hunger: trends and determinants. Glob. Food Secur. 17, 21–29. doi: 10.1016/j.gfs.2018.03.004
Grabowski, P., Olabisi, L. S., Adebiyi, J., Waldman, K., Richardson, R., Rusinamhodzi, L., et al. (2019). Assessing adoption potential in a risky environment: the case of perennial pigeonpea. Agric. Syst. 171, 89–99. doi: 10.1016/j.agsy.2019.01.001
Hufford, M. B., Berny Mier y Teran, J. C., and Gepts, P. (2019). Crop biodiversity: an unfinished magnum opus of nature. Annu. Rev. Plant Biol. 70, 727–751. doi: 10.1146/annurev-arplant-042817-040240
Iannetta, P. P., Young, M., Bachinger, J., Bergkvist, G., Doltra, J., Lopez-Bellido, R. J., et al. (2016). A comparative nitrogen balance and productivity analysis of legume and non-legume supported cropping systems: the potential role of biological nitrogen fixation. Front. Plant Sci. 7:1700. doi: 10.3389/fpls.2016.01700
Jaikumar, N. S., Snapp, S. S., and Sharkey, T. D. (2013). Life history and resource acquisition: photosynthetic traits in selected accessions of three perennial cereal species compared with annual wheat and rye. Am. J. Bot. 100, 2468–2477. doi: 10.3732/ajb.1300122
Johnson, J. A., Runge, C. F., Senauer, B., Foley, J., and Polasky, S. (2014). Global agriculture and carbon trade-offs. Proc. Natl. Acad. Sci. U. S. A. 111, 12342–12347. doi: 10.1073/pnas.1412835111
Jordan, N., Bybee-Finley, A., Gutknecht, J., Hunter, M., Krupnik, T. J., Pittelkow, C. M., et al. (2020). To meet grand challenges, agricultural scientists must engage in the politics of constructive collective action. Crop Sci. doi: 10.1002/csc2.20318
Kane, D. A., Roge, P., and Snapp, S. S. (2016). A systematic review of perennial staple crops literature using topic modeling and bibliometric analysis. PLoS ONE 11:155788. doi: 10.1371/journal.pone.0155788
Kell, D. B. (2012). Large-scale sequestration of atmospheric carbon via plant roots in natural and agricultural ecosystems: why and how. Philos. Trans. R Soc. B Biol. Sci. 367, 1589–1597. doi: 10.1098/rstb.2011.0244
Laborde, S., Fernández, A., Phang, S. C., Hamilton, I. M., Henry, N., Jung, H. C., et al. (2016). Social-ecological feedbacks lead to unsustainable lock-in in an inland fishery. Glob. Environ. Change 41, 13–25. doi: 10.1016/j.gloenvcha.2016.08.004
Landis, D. A., Gardiner, M. M., van der Werf, W., and Swinton, S. M. (2008). Increasing corn for biofuel production reduces biocontrol services in agricultural landscapes. Proc. Natl. Acad. Sci. U. S. A. 105, 20552–20557. doi: 10.1073/pnas.0804951106
Larkin, P. J., Newell, M. T., Hayes, R. C., Aktar, J., Norton, M. R., Moroni, S. J., et al. (2014). Progress in developing perennial wheats for grain and grazing. Crop Pasture Sci. 65, 1147–1164. doi: 10.1071/CP13330
Leakey, R. (2017). Multifunctional Agriculture: Achieving Sustainable Development in Africa. London: Academic Press.
Lin, B. B. (2011). Resilience in agriculture through crop diversification: adaptive management for environmental change. BioScience 61, 183–193. doi: 10.1525/bio.2011.61.3.4
Maggi, F., Tang, F. H., la Cecilia, D., and McBratney, A. (2019). PEST-CHEMGRIDS, global gridded maps of the top 20 crop-specific pesticide application rates from 2015 to (2025). Sci. Data 6, 1–20. doi: 10.1038/s41597-019-0169-4
Mawois, M., Vidal, A., Revoyron, E., Casagrande, M., Jeuffroy, M. H., and Le Bail, M. (2019). Transition to legume-based farming systems requires stable outlets, learning, and peer-networking. Agron. Sustain. Dev. 39:1–14. doi: 10.1007/s13593-019-0559-1
McDonald, C., Corfield, J., MacLeod, N., and Lisson, S. (2019). Enhancing the impact and sustainability of development strategies with smallholder farmers: participatory engagement, whole farm modelling and farmer-led on-farm research. Int. J. Agric. Sustain. 17, 445–457. doi: 10.1080/14735903.2019.1689063
Minnaar, A., Taylor, J. R., Haggblade, S., Kabasa, J. D., and Ojijo, N. K. (2017). “Food science and technology curricula in Africa: meeting Africa's new challenges,” in Global Food Security and Wellness, ed. G. Barbosa-Cánovas (New York, NY: Springer), 247–276. doi: 10.1007/978-1-4939-6496-3_13
O'Brien, P. L., Hatfield, J. L., Dold, C., Kistner-Thomas, E. J., and Wacha, K. M. (2019). Cropping pattern changes diminish agroecosystem services in North and South Dakota, USA. Agron. J. 112, 1–24. doi: 10.1002/agj2.20001
Oliver, T. H., Boyd, E., Balcombe, K., Benton, T. G., Bullock, J. M., Donovan, D., et al. (2018). Overcoming undesirable resilience in the global food system. Glob. Sustain. 1:E9. doi: 10.1017/sus.2018.9
Orr, A., Kambombo, B., Roth, C., Harris, D., and Doyle, V. (2015). Adoption of integrated food-energy systems: improved cookstoves and pigeonpea in southern Malawi. Exp. Agric. 51, 191–209. doi: 10.1017/S0014479714000222
Pachico, D. (2014). “Towards appraising the impact of legume research: a synthesis of evidence,” in Standing Panel on Impact Assessment (SPIA), CGIAR Independent Science and Partnership Council (ISPC) (Rome), p. 39.
Pingali, P. L. (2012). Green revolution: impacts, limits, and the path ahead. PNAS 109, 12302–12308. doi: 10.1073/pnas.0912953109
Rajuladevi, A. K. (2001). Food poverty and consumption among landless labour households. Econ. Polit. Wkly. 36, 2656–2664. Available online at:https://www.jstor.org/stable/4410857?seq=1
Ramankutty, N., Mehrabi, Z., Waha, K., Jarvis, L., Kremen, C., Herrero, M., et al. (2018). Trends in global agricultural land use: implications for environmental health and food security. Annu. Rev. Plant Biol. 69, 789–815. doi: 10.1146/annurev-arplant-042817-040256
Ramaswami, B., and Balakrishnan, P. (2002). Food prices and the efficiency of public intervention: the case of the public distribution system in India. Food Policy 27, 419–436. doi: 10.1016/S0306-9192(02)00047-7
Rao, D., Bharti, N., and Srinivas, K. (2017). Reinventing the commercialization of sorghum as health and convenient foods: issues and challenges. Indian J. Econ. Dev. 13, 1–10. doi: 10.5958/2322-0430.2017.00001.4
Rasche, F., Blagodatskaya, E., Emmerling, C., Belz, R., Musyoki, M. K., and Martin, K. (2017). A preview of perennial grain agriculture: knowledge gain from biotic interactions in natural and agricultural ecosystems. Ecosphere 8:e02048. doi: 10.1002/ecs2.2048
Ritchie, H., Reay, D. S., and Higgins, P. (2018). Beyond calories: a holistic assessment of the global food system. Front. Sustain. Food Syst. 2:1–12. doi: 10.3389/fsufs.2018.00057
Robertson, G. P., Paul, E. A., and Harwood, R. R. (2000). Greenhouse gases in intensive agriculture: contributions of individual gases to the radiative forcing of the atmosphere. Science 289, 1922–1925. doi: 10.1126/science.289.5486.1922
Rogé, P., Diarisso, T., Diallo, F., Boir,é, Y., Goïta, D., Peter, B., et al. (2017). Perennial grain crops in the West Soudanian Savanna of Mali: perspectives from agroecology and gendered spaces. Int. J. Agric. Sustain. 15, 555–574. doi: 10.1080/14735903.2017.1372850
Rogé, P., Snapp, S., Kakwera, M. N., Mungai, L., Jambo, I., and Peter, B. (2016). Ratooning and perennial staple crops in Malawi. A review. Agron. Sustain. Dev. 36:50. doi: 10.1007/s13593-016-0384-8
Saini, K. S., and Ahlawat, S. (2019). Food policy in India: a historical appraisal. Asian J. Multidimensional Res. 8, 234–242. doi: 10.5958/2278-4853.2019.00235.0
Sánchez-Bayo, F., and Wyckhuys, K. A. (2019). Worldwide decline of the entomofauna: a review of its drivers. Biol. Conserv. 232, 8–27. doi: 10.1016/j.biocon.2019.01.020
Schulte, L. A., Niemi, J., Helmers, M. J., Liebman, M., Arbuckle, J. G., James, D. E., et al. (2017). Prairie strips improve biodiversity and the delivery of multiple ecosystem services from corn–soybean croplands. Proc. Natl. Acad. Sci. U. S. A. 114, 11247–11252. doi: 10.1073/pnas.1620229114
Scott, M. P., Edwards, J. W., Bell, C. P., Schussler, J. R., and Smith, J. S. (2006). Grain composition and amino acid content in maize cultivars representing 80 years of commercial maize varieties. Maydica 51, 417–423.
Sinclair, T. R. (2019). The biological yield and harvest index of cereals as agronomic and plant breeding criteria. Adv. Agron. 28, 361–405. doi: 10.2135/cropsci2018.10.0645
Sinclair, T. R., and Horie, T. (1989). Leaf nitrogen, photosynthesis, and crop radiation use efficiency: a review. Crop Sci. 29, 90–98. doi: 10.2135/cropsci1989.0011183X002900010023x
Singh, B. B., Ajeigbe, H. A., Tarawali, S. A., Fernandez-Rivera, S., and Abubakar, M. (2003). Improving the production and utilization of cowpea as food and fodder. Field Crops Res. 84, 169–177. doi: 10.1016/S0378-4290(03)00148-5
Smith, A., Snapp, S., Chikowo, R., Thorne, P., Bekunda, M., and Glover, J. (2017). Measuring sustainable intensification in smallholder agroecosystems: a review. Glob. Food Secur. 12, 127–138. doi: 10.1016/j.gfs.2016.11.002
Snapp, S., Rogé, P., Okori, P., Chikowo, R., Peter, B., and Messina, J. (2019b). Perennial grains for Africa: possibility or pipedream? Exp. Agric. 55, 251–272. doi: 10.1017/S0014479718000066
Snapp, S. S., Blackie, M. J., Gilbert, R. A., Bezner-Kerr, R., and Kanyama-Phiri, G. Y. (2010). Biodiversity can support a greener revolution in Africa. Proc. Natl. Acad. Sci. U. S. A. 107, 20840–20845. doi: 10.1073/pnas.1007199107
Snapp, S. S., Cox, C. M., and Peter, B. G. (2019a). Multipurpose legumes for smallholders in sub-Saharan Africa: identification of promising “scale out” options. Glob. Food Secur. 23, 22–32. doi: 10.1016/j.gfs.2019.03.002
Snapp, S. S., Swinton, S. M., Labarta, R., Mutch, D. R., Black, J. R., Leep, R., et al. (2005). Evaluating benefits and costs of cover crops for cropping system niches. Agron. J. 97, 322–332. doi: 10.2134/agronj2005.0322a
Sprunger, C. D., Culman, S. W., Peralta, A. L., DuPont, S. T., Lennon, J. T., and Snapp, S. S. (2019). Perennial grain crop roots and nitrogen management shape soil food webs and soil carbon dynamics. Soil Biol. Biochem. 137:107573. doi: 10.1016/j.soilbio.2019.107573
Steffen, W., Richardson, K., Rockström, J., Cornell, S. E., Fetzer, I., Bennett, E. M., et al. (2015). Planetary boundaries: guiding human development on a changing planet. Science 347:1259855. doi: 10.1126/science.1259855
Tian, L., and Bekkering, C. S. (2019). Thinking outside of the cereal box: breeding underutilized (pseudo) cereals for improved human nutrition. Front. Genet. 10:1289. doi: 10.3389/fgene.2019.01289
Wang, C., Wu, T., Sun, S., Xu, R., Ren, J., Wu, C., et al. (2016). Seventy-five years of improvement of yield and agronomic traits of soybean cultivars released in the Yellow-Huai-Hai river valley. Crop Sci. 56, 2354–2364. doi: 10.2135/cropsci2015.10.0618
Keywords: multipurpose, lock-in trap, growth habit, ecosystem services, green revolution, crop diversification
Citation: Snapp S (2020) A Mini-Review on Overcoming a Calorie-Centric World of Monolithic Annual Crops. Front. Sustain. Food Syst. 4:540181. doi: 10.3389/fsufs.2020.540181
Received: 03 March 2020; Accepted: 08 October 2020;
Published: 30 October 2020.
Edited by:
Timothy Bowles, University of California, Berkeley, United StatesReviewed by:
Justin Andrew Johnson, University of Minnesota Twin Cities, United StatesStephen A. Wood, The Nature Conservancy, United States
Copyright © 2020 Snapp. This is an open-access article distributed under the terms of the Creative Commons Attribution License (CC BY). The use, distribution or reproduction in other forums is permitted, provided the original author(s) and the copyright owner(s) are credited and that the original publication in this journal is cited, in accordance with accepted academic practice. No use, distribution or reproduction is permitted which does not comply with these terms.
*Correspondence: Sieglinde Snapp, snapp@msu.edu