- 1Texas A&M AgriLife Research, Vernon Center, TX, United States
- 2Department of Rangeland, Wildlife and Fisheries Management, Texas A&M University, College Station, TX, United States
- 3Department of Ecology and Conservation Biology, Texas A&M University, College Station, TX, United States
Ruminants including domestic livestock, have been accused of causing damaging impacts on the global environment and human well-being. However, with appropriate management, ruminant livestock can play a significant role in efforts to reverse environmental damages caused by human mismanagement and neglect. Worldwide, at least one billion people living in grazing ecosystems depend on them for their livelihoods, usually through livestock production, and for other ecosystem services that affect human well-being. For long-term rangeland sustainability and ecological resilience, agricultural production policies are urgently needed globally to transform current damaging industrial inorganic input agricultural practices to resource conservation practices that enhance ecosystem function. This is supported by evidence that farmers and ranchers who apply regenerative management practices to restore ecosystem functionality create sustainable, resilient agroecosystems cost-effectively. With enhanced management of grazing resources, domesticated ruminants can be used to produce higher permanent soil cover of litter and plants, which are effective in reducing soil erosion and increasing net biophysical carbon accumulation. Incorporating forages and ruminants into regeneratively managed cropping systems can also elevate soil organic carbon and improve soil ecological function and reduce production costs by eliminating the use of annual tillage, inorganic fertilizers and biocides. Ecosystem services that are enhanced using regenerative land management include soil stabilization and formation, water infiltration, carbon sequestration, nutrient cycling and availability, biodiversity, and wildlife habitat, which cumulatively result in increased ecosystem and economic stability and resilience. Scientists partnering with farmers and ranchers around the world who have improved their land resource base and excel financially have documented how such land managers produce sound environmental, social, and economic outcomes. Many of these producers have used Adaptive Multi-Paddock (AMP) grazing management as a highly effective approach for managing their grazing lands sustainably. This approach uses short-duration grazing periods, long adaptively varied post-grazing plant recovery periods requiring multiple paddocks per herd to ensure adequate residual biomass, and adjustment of animal numbers as environmental and economic conditions change. Using this approach, farmers and ranchers have achieved superior ecosystem and profitability outcomes. This manuscript summarizes the use of AMP grazing as regenerative tool for grazed and rotationally cropped lands.
Introduction
For the continued delivery of essential ecosystem services supporting the livelihoods of people living in grassland and savanna ecosystems, it is critical to maintain or enhance the productive capacity and resilience (Frank et al., 1998; Janzen, 2010). Such ecosystem services include the maintenance of stable and productive soils, the delivery of clean water, the sustenance of functional soil-biosphere hydrological cycles, and plants, animals and other organisms that support ecosystem function and human livelihoods and well-being. Agro-ecosystems that include grazing are more productive, stable and resilient when the soil is fully functional biologically, and they provide greater earnings and more abundant ecosystem services (Teague et al., 2013).
While developments in knowledge and technology have greatly increased agricultural productivity, inadequate attention has been given to long-term sustainability of natural resources and ecosystem services provided by agroecosystems. Through repeated soil tillage, artificial fertilizer application, and widespread biocide use, grazing and cropping agriculture is globally implicated in negative impacts on land resources and climate (MEA, 2005; Delgado et al., 2011). Carbon levels in most agricultural soils have declined in the last 100 years (Lal, 2004). In a global analysis, Sanderman et al. (2017) found that the largest SOC losses coincide with cropping regions but grazing, especially in arid and semiarid regions that are globally more extensive, was responsible for at least half of the total SOC loss. Biomes that are predominantly grasslands and savannas lost more SOC than the cropland and crop/natural vegetation categories, and the regions that have lost the most SOC relative to historic levels include the rangelands of Argentina, southern Africa and Australia. Such massive soil carbon losses have led to the degradation of soil structure, productivity and resilience as well as their capacity to infiltrate, filter and retain surface water, which collectively cool soils. These effects have led to impoverishment and loss of soil, disrupted hydrological and biogeochemical processes, contamination of water bodies by fertilizer and biocide runoff, loss of biodiversity, excessive water use and aquifer depletion, and increased greenhouse gas (GHG) emissions implicated in climate change as noted in the reviews by Lal (2003) and Janzen (2010).
Ecologically sensitive management of soil and appropriate inclusion of perennial forages and ruminants in mixed crop and grazing agro-ecosystems has been demonstrated to decrease the problems associated with current tillage and inorganic chemical input-based cropping and feedlot-based livestock production systems. Permanent cover of forage plants is critical for reducing soil erosion and, when ruminants consuming only grazed forages are included and managed appropriately, results in carbon sequestration that exceeds ruminant carbon emissions (Stanley et al., 2018). Regeneratively managed agro-ecosystems incorporating forages and ruminants minimizes the damage of tillage and inorganic fertilizers and biocides, elevates soil organic carbon, improves soil ecological function, and enhances biodiversity Janzen, 2010; Delgado et al., 2011; Gattinger et al., 2012; Aguilera et al., 2013). The innovative regenerative grazing management we refer to as adaptive multi-paddock grazing (AMP) has been operational for four decades in many parts of the world. Positive ecological and economic results have been achieved by AMP managers in various climatic areas ranging from mean annual precipitation of arid (200 mm) to humid (+2,000 mm) regions where grazing is practiced in North America, South America, Hawaii, central and southern Africa, Australia and New Zealand. This has been independently documented by Montgomery (2017) and Massy (2018). These are recent comprehensive global reviews on the subject.
In this paper we describe historical effects of herbivory on grasslands, characteristics of alternative grazing management approaches to restoring grassland health, biological drivers and causal mechanisms in grazing ecosystems, and limitations of past research. We emphasize the importance of collaborating with exemplary ranchers and farmers to understand grazing management strategies that lead to cost-effective restoration of ecological function and ecosystem services in agricultural systems which support sustainable income in a variable environment. Throughout we focus on how different strategies affect ecosystem functionality, productivity, and sustainability by modifying soil processes that underlie water and nutrient cycling and plant growth (Teague et al., 2013).
Historical Herbivory Effects on Grasslands
Grasslands co-evolved with herbivores since the late Mesozoic Era as complex, dynamic ecosystems comprised of grasses, soil biota, grazers, and predators (Retallack, 2013). Spatial and temporal variation in the grazing landscapes caused large concentrated herds of grazing ungulates to move regularly to satisfy water and nutrient requirements and to avoid recently grazed and fouled areas, and in response to their social organization and the influences of fire, predation, hunting and herding (Bailey and Provenza, 2008; Provenza, 2008). Such periodic concentrated herbivory led to relatively short periods of heavy and uniform use of grass species as animals moved across the landscape. These periods of intense herbivory were generally followed by periods of herbivore absence during which defoliated plants regrew before the grazers returned to the area. Early hunter gatherers increasingly affected the landscape by deliberately burning areas to open them up, to drive wild animals toward hunting parties, and to attract wild grazers to recently burned areas (Pyne, 2001).
This resulted in grazed ecosystems that are complex and highly resilient and sustain considerably higher levels of herbivory and animal biomass than other terrestrial habitats (Stuart Hill and Mentis, 1982; Frank et al., 1998). The interaction of fluctuating climatic conditions, fire and grazing created the resilient and dynamic networks of organisms capable of responding to episodic biophysical events, and ecosystems that never reach a steady-state or climax seral stage but rather such periodic disturbances rejuvenated and transformed grasslands, including soil structure and nutrients, plant species composition, structure and biodiversity (Rice and Parenti, 1978; Pickett and White, 1985; Hulbert, 1988).
In the evolution of grassland and savanna ecosystems, synergistic interactions between soil fungi and microbes, plants, and various associated animal life forms resulted in the biosequestering of atmospheric carbon into stable soil carbon pools; these enhanced the productivity, resilience, hydrology and carbon capture capacity of these soils and the balance between carbon accumulation and oxidation rates (Frank and Groffman, 1998; Altieri, 1999; Van der Heijden et al., 2008; De Vries et al., 2012; Morriën et al., 2017). These high carbon soils have high water holding capacity, which can extend the longevity and area of green photosynthesizing leaves, and elevated evapotranspiration of water vapor and substantial latent heat fluxes that govern 95% of the earth's heat dynamics and hydrological cooling of earth and its climate (Veizer et al., 2000; Ferguson and Veizer, 2007; Pokorný et al., 2010). While these integrated biophysical systems (soils, hydrology, plants and animals) resulted in carbon sequestration rates that exceeded oxidation rates, human agricultural activities including repeated burning and tilling, burning and overgrazing have led to the reversal of the soil carbon dynamic and the depletion of accumulated soil carbon.
With the arrival of European settlers in North and South America, Africa and Australia, migratory free-ranging herds of large grazing ungulates and transhumance livestock herding were increasingly replaced by sedentary domestic livestock production, land was increasingly subdivided into fenced landholdings and predators were widely exterminated (Oesterheld et al., 1992; Provenza, 2008). Under private land tenure, grasslands were traditionally used for livestock production by allowing grazing animals to disperse freely within individual landholdings. This grazing management approach is generally referred to as season-long continuous grazing (CG) and generally leads to long-term concentration of grazers on preferred areas (especially lower lying, flatter areas with more palatable and easily accessible plants near water resources) and repeated use of preferred forage species (Fuls, 1992; Kellner and Bosch, 1992; Teague et al., 2004). Such long-lasting, selective herbivory on individual properties has led to localized overgrazing, proliferation of less palatable grass species and woody plants, increased bare areas and, ultimately, the decline in the ecological functionality of grazed landscapes (Briggs et al., 2005; Archer et al., 2017). Continuous concentrated grazing can also lead to collapse of soil aggregation and structure, lower surface water infiltration rates, less plant-available soil water, and increased surface water runoff, soil erosion, nutrient movement to downslope water bodies, and ultimately eutrophication and impairment of freshwater sources (Thurow, 1991; Burkart and Stoner, 2002; Babiker et al., 2004; Webber et al., 2010). In the last 70 years, increasingly industrialized grazing and cropping practices have greatly accelerated these effects by applying excessive grazing pressure under continuous grazing practices combined with the overuse of fire, tillage, inorganic fertilizers, biocides, and irrigation that collectively oxidize soil carbon (Delgado et al., 2011).
Characteristics of Alternative Grazing Management Approaches
Two alternative grazing management approaches to continuous grazing (CG) have been proposed to mitigate long-term overuse of preferred areas and grasses and to simulate the historic patterns of periodic concentration of grazing followed by post-grazing herbaceous recovery periods (Figure 1). The first approach is pyric herbivory (PH), which combines rotational patch burning as the primary mechanism for concentrating grazing animals and moving their impact across the landscape, with constant continuous stocking over the entire management unit (Fuhlendorf et al., 2006). The second approach is adaptive multi-paddock (AMP) grazing, which uses multiple paddocks per herd with the goal of managing grazing resources to improve ecological function (Teague et al., 2013). This is achieved by adjusting animal numbers to match available forage, using short grazing periods, leaving sufficient post-herbivory plant residue for regrowth, and providing long recovery periods to adaptively accommodate intra- and inter-seasonal variation in herbaceous plant growth. It is important to emphasize that AMP grazing is not equivalent to rotational grazing, which is a generic term used for diverse grazing management approaches that subdivide the grazing area into any number of paddocks that are grazed sequentially using pre-determined grazing periods.
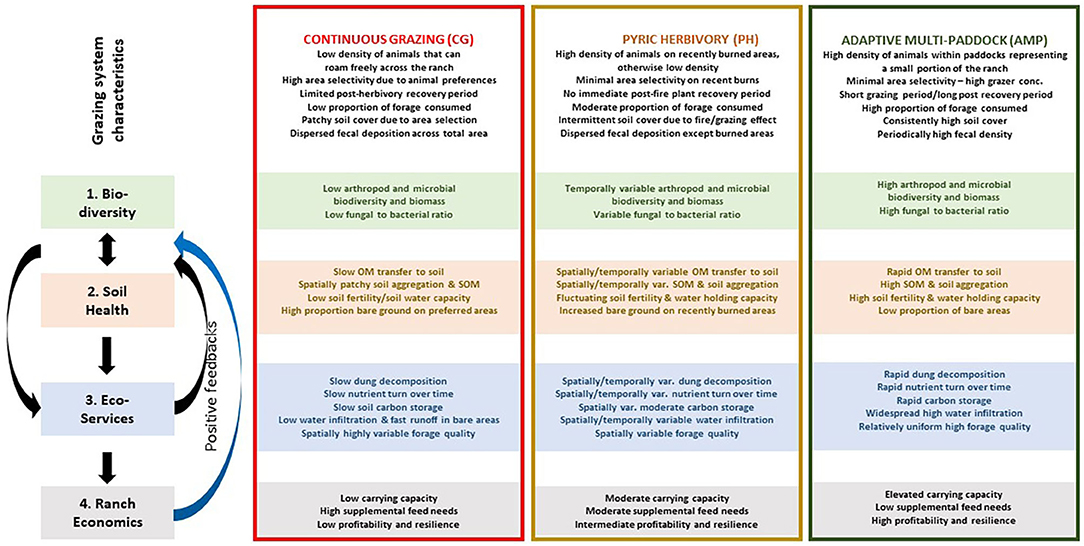
Figure 1. Postulated effects of alternative grazing management approaches on productivity and biodiversity, soil health, ecosystem services and ranch economics (Frank et al., 1998; Teague et al., 2013; Jakoby et al., 2015; Savory and Butterfield, 2016; Park et al., 2017; Stanley et al., 2018; Dowhower et al., 2019; Pecenka and Lundgren, 2019).
Periodic concentrated herbivory is likely to affect the whole management unit of a ranch in various ways depending on the periodicity of repeated herbivory. Under CG, long-term overutilization of preferred areas tends to lead to decrease in biodiversity, organic matter transfer to soils, nutrient cycling, ground cover and high-quality forage and therefore loss of topsoil and productivity. By contrast, it has been claimed that under PH and AMP grazing, periodic concentration of grazing animals followed by subsequent extended post-defoliation recovery periods may lead to net ecosystem benefits including increased soil carbon, nutrient cycling, soil microbial function, above and below ground biodiversity, surface water infiltration, rooting depth, plant cover, herbaceous biomass, and ultimately ranch profitability (Jakoby et al., 2014, 2015; Martin et al., 2014). The primary difference between the two approaches is the double defoliation effect of fire followed by grazing in PH compared to defoliation by herbivores only with AMP grazing, leading to the intended outcomes of greater spatial heterogeneity across the landscape in PH grazing and more uniform forage utilization across the landscape in AMP. Therefore, the relative net benefits under these different management choices need to be determined at the spatial and temporal scales of managed landscapes and not simply in short-term plot treatments that do not reflect real world conditions with which farmers and ranchers have to contend (Teague et al., 2013).
Management decisions affect how grasslands sequester atmospheric carbon and provide other essential ecosystem services, including soil water retention, herbaceous biomass production, and, therefore, support ranch profitability. For long-term sustainability of rangelands livestock producers need to adopt grazing management practices that improve soil and ecosystem function and resilience (Havstad et al., 2007). To achieve this goal, they should aim to increase solar energy capture, water infiltration and retention, soil organic matter accumulation, nutrient cycling, and the above and below ground biodiversity needed for ecosystems to function properly (Teague et al., 2013). PH grazing has been shown to have periodic, patchy low ground cover with nutritious regrowth in burned areas, which herbivores preferentially graze, leading to heterogenous grass cover with underutilized, lower quality forage in unburned areas, and mixed biodiversity and structural diversity across the landscape (Fuhlendorf et al., 2006). By contrast, AMP grazing has the potential to reverse causal mechanisms of ecosystem degradation operating under CG by decreasing bare ground, increasing water infiltration rates and soil water storage capacity and reducing surface water runoff and therefore soil and nutrient losses, increasing fungal to bacterial ratios, and increasing soil carbon, and ultimately restoring productive herbaceous plant communities by reducing the ability of herbivores to select only the most nutritious grass species (Sovell et al., 2000; Webber et al., 2010; Delgado et al., 2011; Teague et al., 2011, 2013; Weltz et al., 2011).
Biological Drivers and Causal Mechanisms in Grazing Ecosystems
Rangeland productivity and resilience to environmental variability are a function of soil health and microbial functional diversity (Plassart et al., 2008; Morriën et al., 2017). High soil microbial biomass contributes to improved soil aggregation, porosity, water infiltration rates and water holding capacity, and more rapid soil carbon and nutrient turnover (Coleman and Crossley, 1996; Six et al., 2004). In turn, soil health, which is a collective term for these factors, is a more reliable predictor of herbaceous productivity than land use history (De Vries et al., 2013) or clay mineralogy (Kallenbach et al., 2016). Microbial carbon plays a disproportionately large role in soil aggregate formation (Bardgett and McAlister, 1999; De Vries et al., 2013), which affects soil porosity, aeration, water infiltration rate and water holding capacity.
While the greatest limiting factor in grazing land ecosystems is the infiltration and retention of surface water in the soil (Thurow, 1991), optimal ecosystem function also requires efficient solar energy capture, soil organic matter accumulation, efficient nutrient cycling, and high levels of below and above ground biodiversity (Teague et al., 2013; Savory and Butterfield, 2016). Soil health is mediated by the interdependence of soil microbes, fungi, insects, plants and animals. Plants support microbial life by supplying carbohydrates, root exudates and detritus upon which microbes feed, while benefiting from nutrient release resulting from interactions among soil archaea, bacteria, fungi, and other microbial and eukaryotic species. Therefore, the way in which plants are managed and utilized in grazing can directly affect the associated ecosystems because the energy driving ecological functions is derived predominantly through the conversions of solar energy to carbohydrates by photosynthesizing plants.
The functions provided by the synergistic networks of soil organisms include: soil aggregation and stabilization (Van der Heijden et al., 2008); aeration and water holding capacity (Altieri, 1999); nutrient cycling, accumulation and retention (Green et al., 2008; Khidir et al., 2008); biotic and abiotic stress tolerance (De Vries et al., 2012) and buffering the impact of environmental factors on plants (Van der Heijden et al., 2008). Importantly, arbuscular mycorrhizal fungi (AMF) are keystone species in many terrestrial ecosystems, particularly grasslands, because they can enhance plant diversity, mediate interactions among plants and other microbes, and enhance plants' access to nutrients (Averill et al., 2014). Additionally, symbiosis between AMF, rhizobia and leguminous plants can enhance photosynthesis by 50% (Kashuk et al., 2009), and AMF contributes directly to the soil organic matter through secretion of soil glycoproteins that increases soil aggregate stability, thereby enhancing soil aeration and surface water infiltration (Rillig, 2004).
Improvement in grassland soil organismal composition enhances carbon cycling and nitrogen cycling to increase hydrological function and biological fertility (Altieri, 1999; Van der Heijden et al., 2008; De Vries et al., 2012). At high microbial densities and species biodiversity, symbiotic interactions among species can lead to enhanced biological outcomes via the phenomenon of quorum sensing (Nealson et al., 1970). Grazing management strategies aimed at restoring soil function tend to expand below ground microbial networks and increase the efficiency of nutrient cycling and carbon uptake by diversifying the composition and activities of fungi Ngumbi and Kloepper, 2016; Slade et al., 2016; Morriën et al., 2017. Other key biological drivers of ecosystem function that can be enhanced by adjusting grazing management to optimize benefits provided by arthropods, such as dung beetles, and earthworms. Such beneficial management can strongly benefit soil structure and ecological functions (Herrick and Lal, 1995; Richardson and Richardson, 2000; Wardle and Bardgett, 2004; Blouin et al., 2013; Pecenka and Lundgren, 2019).
Livestock production systems that use artificial inputs (e.g., inorganic fertilizers, pesticides and livestock medications) or grazing practices that result in inadequate post-herbivory ground cover, impair soil organisms, their interactions and their functions, diminish the ecological functions they perform, and reduce the delivery of ecosystem services (Iglesias et al., 2006; LaCanne and Lundgren, 2018). By contrast, under appropriate management, a grass-fed ruminant enterprise that avoids the use of damaging inputs can also be ecologically beneficial and regenerative by supporting the mechanisms that lead to increased soil health to provide the nutritional needs of plants and livestock.
Limitations of Past Research and Learning From Outstanding Managers
To succeed in the short and long term, ranchers need to achieve high levels of soil and vegetation function, animal performance, and profit within the constraints of the inherent landscape heterogeneity of their unique properties. This requires monitoring the responses of the ecosystems they manage to their operational practices at diverse temporal scales. Critically, in dynamic climatic and economic environments, ranchers must also manage adaptively in order to achieve their desired outcomes, avoid management decisions that create negative impacts on their land, and maximize the positive soil-based interactions that enhance soil health. A primary reason why grazing management researchers have failed to achieve similar results to outstanding grazing managers using adaptive, regenerative AMP rotational grazing management practices (Briske et al., 2008) is that they have not adapted grazing treatments to achieve the best possible ecological and economic results (Teague et al., 2013; Teague, 2015).
The majority of earlier grazing studies found little difference between rotational grazing and lightly stocked continuous grazing (Briske et al., 2008, 2011). However, this conclusion is largely based on reductionist grazing experiments that were not adaptively managed to specifically achieve best outcomes under changing conditions and, therefore, they do not reflect the successes that have been achieved with AMP grazing on many commercial ranches (Teague et al., 2013). The potential of multi-paddock grazing can only be achieved in field studies if they are managed adaptively over multiple years based on protocols that have produced successful results in commercial ranch settings (Teague and Barnes, 2017). Long-term management of an area to improve soil and ecosystem function can produce entirely different results than an area that has been conventionally managed and has not enhanced ecosystem services. An experiment that does not take such factors into account will likely provide results that do not reflect potential improvements in long term management outcomes.
Most grazing research on rangelands has been too short-term and too small-scale to identify longer-term unintended consequences or the management implications of the experimental grazing treatments (Teague et al., 2013). For the sake of repeatability and scientific rigor and due to budgetary constraints, grazing studies have generally examined rigidly applied treatments at spatial and temporal scales that preclude the evaluation of adaptive management possibilities within the context of commercial-scale operations. Most studies have been conducted at small spatial scales that force animals to graze evenly and, therefore, prevent the heterogeneity of grazing that leads to long-term grassland degradation under continuous grazing at commercial scales (Teague and Barnes, 2017). As a result, much grazing systems research has shown that all forms of “rotational” grazing management (“systems”) produce limited or no improvement in grazing resources compared to continuously grazed areas. The relatively few studies that have incorporated realistic context of scale and complexity, coupled with a well-planned adaptive application of treatment, have shown numerous benefits of AMP grazing over continuous grazing (Teague et al., 2013).
Most rangeland grazing research has been too short-term and small-scale to identify longer-term consequences or the management implications of the experimental grazing treatments (Teague et al., 2013). Generally, this research has been conducted over 2 to 3 years in small paddock trials that inhibit heterogeneous grazing patterns, which lead to long-term grassland degradation under continuous grazing at commercial ranch scales (Teague and Barnes, 2017). Additionally, short-term grazing experiments do not overcome the legacy effects of previous grazing management and, for the sake of repeatability and scientific rigor, such studies have generally examined a limited number of rigidly applied treatments that preclude the evaluation of adaptive management effects (Van der Ploeg et al., 2006). As a result, short-term, small-scale grazing systems research has not shown substantial environmental changes and led to the erroneous conclusion that all forms of “rotational” grazing management (“systems”) produce limited or no improvement in grazing resources compared to continuously grazed areas (Briske et al., 2008). Grazing affects many ecosystem variables at different temporal and spatial scales; these variables include but are not limited to soils organic matter, soil microbes, arthropods, vegetation, and non-livestock herbivores. Differentially lagged grazing effects on such variables necessitates consistent application of grazing management treatments for 5 years, 10–15 years or decades in humid, mesic and dry environments, respectively, to capture diverse environmental changes at the landscape level (Franzluebbers and Stuedemann, 2010). The relatively few studies that have incorporated realistic contexts of scale and complexity, coupled with a well-planned adaptive application of treatment, have shown numerous benefits of AMP grazing over continuous grazing, even where the latter is practiced at low stocking rates (Earl and Jones, 1996; Murphy, 1998; Gerrish, 2004; Jacobo et al., 2006; Provenza, 2008; Ferguson et al., 2013; Teague et al., 2013; Flack, 2016; Rowntree et al., 2016; Wang et al., 2016; Dowhower et al., 2019).
To help livestock producers achieve superior grazing management results, integrative and multidisciplinary research is needed to understand how they can achieve desired ecological, economic, and social goals on their landscapes in changing environments. Research is also needed to test hypotheses of causal above- and below-ground biological mechanisms underpinning responses to different grazing management approaches (Teague, 2015). To understand these critical elements of superior grazing management requires conducting research with innovative land managers on real operations, applying adaptive treatments, and combining detailed field experimentation with embedded, small scale, reductionist experiments in the context of the management options being studied, and simulation modeling approaches (Teague et al., 2013; Jakoby et al., 2014, 2015; Martin et al., 2014; Müller et al., 2014; Wang et al., 2016; Park et al., 2017).
Collaborating with ranchers who have simultaneously improved the biophysical conditions of their environments as the basis for achieving superior economic returns in different ecological and cultural settings is fundamentally important to learn how to improve the three-part components of operational sustainability—ecological, economic, and social (Van der Ploeg et al., 2006). Not only have such producers developed improved management protocols, but they have effectively managed cash flows and learned how to avoid unintended consequences while transitioning from non-sustainable traditional to regenerative production systems that improve soil health on their land (Teague et al., 2013). Moreover, such producers achieve best management outcomes by combining goal directed adaptiveness with a deep understanding and observations of the response dynamics of biophysical processes on their land to alternative management interventions.
A lack of systems training precludes many agricultural and ecological researchers from being able to adequately manage research projects to demonstrate the best possible outcomes of innovative management options (Van der Ploeg et al., 2006). In contrast, ranchers are less constrained by strictures of conventional scientific research protocols and are more likely to apply adaptive learning principles to test different combinations of practices and approaches within realistic whole-ranch systems (Teague, 2015). Working with leading ranchers can facilitate development of more sustainable agricultural practices for several reasons (Van der Ploeg et al., 2006), including: (i) addressing questions at commercial ranch scale; (ii) using a whole-system framework to integrate component science elements; (iii) incorporating pro-active management to achieve desired goals under changing circumstances; and (iv) identifying emergent properties and unintended consequences; and (vi) extend the usefulness of information developed in research to land managers.
A tool that enhances understanding and provides a solid theoretical base for all scientific investigations is systems simulation modeling. This approach facilitates understanding biophysical processes and management hypotheses at the landscape scale and testing them against observed results (Díaz-Solís et al., 2009; Teague et al., 2009). However, it is essential to constantly check model outputs with data from field experiments from collaborating commercial ranching operations to ensure the models are well-grounded in quantified long-term treatment responses and that their outputs are not extrapolated beyond real-world outcomes.
Managing Grazing to Restore Ecological Function and Ecosystem Services
Using well-planned and adaptively managed multi-paddock grazing management protocols is the key to sustainable use of grazing lands and recovery from degradation and involves using short grazing periods, retaining sufficient litter and plant cover to protect the soil and allow rapid plant regrowth. Such management allows adequate time for grazed plants to regrow and adjusting stock numbers to match available forage biomass ensures available forage always provides for ecosystem function, adequate animal nutrition and avoids unnecessary costs (Earl and Jones, 1996; Jacobo et al., 2006; Provenza, 2008; Ferguson et al., 2013; Teague et al., 2013; Wang et al., 2016).
AMP grazing facilitates the adjustment of these elements to avoid soil loss, strengthen soil and ecological function, increase herbaceous plant biomass and minimize the increase in unproductive species composition, leading to the achievement of desired resource and financial outcomes. In grasslands and savannas, the application of regenerative high-density AMP grazing management has been shown globally to be capable of reversing degradation emanating from the long-term practice of continuous heavily-stocked grazing (Gerrish, 2004; Teague et al., 2011, 2013; Jakoby et al., 2014, 2015; Savory and Butterfield, 2016). Additionally, in semiarid areas where this regenerative grazing management has been practiced for decades, improvements have been observed in biodiversity including pollinators, plant productivity, litter cover, nitrogen-fixing legumes, re-perennialization of ephemeral streams and watershed function (National Research Council., 2002).
Where scientists have worked with ranchers practicing AMP grazing to regenerate soil health and ecosystem functionality, positive resource and economic outcomes have been reported (Teague et al., 2013; Savory and Butterfield, 2016; Teague and Barnes, 2017). Specifically, studies in Argentina, Australia, Germany, Southern Africa, and the USA have reported positive resource and economic results from regenerative ecological grazing when research incorporates the following four factors. The research, (i) was conducted at the scale of ranching operations; (ii) grazing resource utilization was adjusted proactively as growing conditions changed to achieve desired ecosystem and production goals; (iii) when grazing treatments had been applied for sufficiently long time periods to overcome biophysical response lags and to incorporate intra- and inter-seasonal variations in diverse environmental factors, and (iv) parameters indicating change in ecosystem function and not just production outputs were measured and acted upon (Earl and Jones, 1996; Murphy, 1998; Gerrish, 2004; Jacobo et al., 2006; Müller et al., 2007; Provenza, 2008; Teague et al., 2011, 2013; Jakoby et al., 2014, 2015; Martin et al., 2014; Müller et al., 2014; Flack, 2016; Wang et al., 2016).
AMP grazing protocols were specifically designed to emulate ecosystem processes that evolved in response to intense, periodic herbivory by large herds of ungulate grazers and they have been effective in reversing the damage caused by continuous grazing in a timely and cost-effective manner (Gerrish, 2004; Teague et al., 2011, 2013; Wang et al., 2016). The use of AMP grazing principles increases livestock carrying capacity over time while improving ecological function, as paddock number increases. However, this is not intensive grazing but intensive management of grazing (Dowhower et al., 2019) as it reverses the impacts of intensive grazing by specifically avoiding overstocking of, and overgrazing by, livestock in an adaptive manner in response to variable weather (Jakoby et al., 2014, 2015; Teague et al., 2015; Wang et al., 2018). In combination, these actions result in light to moderate grazing impact on herbaceous plants, the soil and the ecological functions they perform (Teague et al., 2013; Jakoby et al., 2014; Teague and Barnes, 2017; Dowhower et al., 2019).
While some have touted the advantages of PH, for enhancing the biodiversity of certain categories of species, notably grassland birds many of which have become substantially threatened and endangered (Fuhlendorf et al., 2006), from an ecosystem sustainability perspective, the temporal juxtaposition of fire and grazing can have negative outcomes. The reasons for this are that frequent fire decreases vegetation cover which leads to increased soil surface temperatures that, in turn, can lead to disruption of the hydrological cycle, soil compaction, nutrient losses via runoff, and volatilization and leaching (Wright and Bailey, 1982). Continuous livestock grazing reduces grass biomass and creates patchy vegetation interspersed with bare soil. With the patch burning and continuous grazing that characterize PH grazing, the newly burned patches each attract heavier use while relieving previously burned patches of defoliation to allow some recovery. However, heavy grazing by livestock on burned patches and underutilization of grasses in unburned areas can reduce overall biomass production and (by design) lead to patchy vegetation, which is interspersed with bare ground. In contrast, rotational grazing in general and AMP grazing in particular, provide longer more substantive, extended recovery time periods after burning and grazing. This results in less bare ground and more plant cover to lower soil surface temperatures, enhance soil carbon to maintain or improve healthy soil hydrological characteristics, while maintaining or enhancing productive herbaceous species composition on both burned and unburned areas (Teague et al., 2008, 2010).
One disadvantage of frequent burning in PH grazing is that, while burned vegetation and ground cover can recover within the year of burning in wetter areas, in semi-arid rangelands where droughts occur frequently it may take several years of average or above average rainfall for full soil function and herbaceous vegetation recovery. When drought conditions precede or follow the application of fire, bare ground, annual forbs and grasses, and even woody plants may increase at the expense of productive perennial grasses that may require 3–5 years or longer to recover after fire (Wright and Bailey, 1982; Teague et al., 2008). This is not the case in AMP grazing where burned areas are not immediately subject to grazing but are provided sufficient time to recover if fire is applied. In summary, AMP grazing offers substantial benefits over CG and PH for reducing weather-related production risks, especially after fire. It also facilitates the attainment of ranchers' goals to improve ecological function of their production base in order to enhance the delivery of ecosystem services, economic returns and long-term sustainability of their ranching operations.
Managing for Multi-Functionality in Agricultural Systems
How well-grassland and savanna ecosystems are managed to regenerate soil and ecosystem function governs our future. Carbon rich soils benefit all terrestrial ecosystems, and managing to increase soil carbon is fundamental to improving ecosystem services including water infiltration and retention; soil retention; soil nutrient cycling and retention; biodiversity enhancement including fungi, microbes, insects, plants, and animals; wildlife nutrition and habitat; livestock forage; all of which can help support ranch profits and resilience in changing climates. To achieve this, agriculture needs to change from traditional high-input cropping and grazing practices to low-input practices that increase atmospheric carbon sequestration via photosynthesis and increase soil microbes that enhance soil water and nutrient cycles and that minimize soil carbon release back into the atmosphere. This will require increasing the amount of land under perennial or opportunistic plant growth, increase seasonal longevity of plant photosynthesis associated with enhancing carbon sequestration, and the degree to which sequestered carbon is converted into stable soil organic carbon, and not oxidized back into CO2 by burning or oxidation (Delgado et al., 2011).
While people have minimal capacity to control non-anthropogenic drivers of environmental change (Plimer, 2009), human impact on the environment caused by unsustainable agricultural practices can, in many cases where excessive soil loss has not occurred, be addressed by reversing the degradation of the landscapes by switching from extractive and environmentally harmful practices and inputs to regenerative grazing and cropping practices (Delgado et al., 2011; Teague et al., 2013). This will require using management practices that effectively and efficiently restore hydrological cycles, soil health and the delivery of critical ecosystem services.
The water cycle is the thermostat of global climate change governing massive energy changes required to melt polar ice, water evaporation and precipitation (Shaviv and Veizer, 2003), and the carbon cycle is affected by the water cycle (Plimer, 2009). Atmospheric water vapor and droplets are the most prevalent and potent GHG and the most limiting commodity for plant growth production that provides food for animals and people.
Practices that can be adopted to substantially enhance soil carbon include regenerative cropping, regenerative grazing in cropped areas and grasslands, restoration of shelter trees in grazing lands, and reduction of rangeland wildfires (Delgado et al., 2011; Teague et al., 2013). The key to optimizing ecological function and reversing degradation caused by previous mismanagement in both cropping and grazing systems is use of management practices that elevate soil health. Specifically, soil ecological function is maintained by minimizing bare ground by maintaining plant and litter cover throughout the year, using perennial rather than annual plants, using diverse species mixes and cover crops, managing grazing to promote the most productive plants, maximizing plant growth days each year, eliminating high impact tillage practices, using organic soil amendments, and minimizing artificial inorganic fertilizer and biocides use, (Delgado et al., 2011; Teague et al., 2011; Gattinger et al., 2012; Aguilera et al., 2013).
The water cycle and vegetation play a fundamental role in maintaining local climate through energy transfers in the process of evapotranspiration (ET); specifically, the interaction of water and plants dampen temperature maxima resulting from intense solar radiation (Pokorný et al., 2010). Given water vapor's potency as a heat absorber, evapotranspiration from plants transfers heat from the earth's surface into the atmosphere, thereby cooling the surface, and accounts for about 24 % of natural global hydrological cooling (Pokorný et al., 2010). Modification of landscapes by agriculture, overgrazing, deforestation, wetland drainage, and urbanization that remove transpiring vegetation diminishes the self-regulatory damping of solar radiation and temperatures by healthy ecosystems. For evapotranspiration to deliver substantial cooling requires extensive ground cover of actively growing plants to maintain a healthy hydrological cycle (Ferguson and Veizer, 2007). Elevation of soil carbon enhances both surface water infiltration, soil moisture holding capacity and soil fertility needed to support plant growth and high green leaf area that increase evapotranspiration and plant biomass.
Soil health, defined as the continued capacity of soil to function as a vital living ecosystem, determines the quantity and quality of ecosystem services delivered for the benefit of current and future generations. Paleo records provide evidence that management of grassland agroecosystems can create a large C sink (Retallack, 2013). Equally, changing management approaches in ruminant-based production chains can improve soil health and thereby create net C sinks (Wang et al., 2015; Rowntree et al., 2016; Stanley et al., 2018). Given that most agricultural producers have not used conservation practices outlined by Delgado et al. (2011), applying such practices more broadly could lead to substantial soil health improvements and, therefore, a significant increase in C sink (Conant and Elliott, 2001; Liebig et al., 2010; Teague et al., 2011; Machmuller et al., 2015; Dowhower et al., 2019; Hillenbrand et al., 2019). To determine what management changes will lead to soil carbon gains, it is necessary to include all production chain elements that affect the carbon footprint of the entire system under review (Teague et al., 2016), including the beneficial ecosystem services that well-managed grazing systems can provide.
Combining crop rotation with livestock grazing can enhance soil function and health (Delgado et al., 2011). Row crop rotations that include legumes, and cover and forage crops can produce permanent ground cover and increase soil carbon, water infiltration and fertility. Furthermore, grazing livestock can accelerate nutrient cycling through the decomposition of residual aboveground biomass (Teague et al., 2016). Including forage and grazing animals in cropping systems by sowing winter crops into permanent summer pastures significantly reduces the damaging effects of many current cultivation and management practices, including loss of soil and SOC, and GHG emissions. This management is particularly effective and necessary where soil erosion potential is moderate to high (Delgado et al., 2011). Similarly, using a grazing-cropping rotation with perennial pasture or rangeland on cropland vulnerable to degradation in the Australian ley farming systems is very effective in reducing the overall crop and livestock-associated carbon footprint (Carberry et al., 1996). Adoption of such low-cost management strategies promotes the restoration of perennial grasses without limiting crop production and is an economically viable strategy for regenerating degraded land (Millar and Badgery, 2009). Pasture-cropping, developed by Australian farmer Colin Seis, is a strategy that integrates livestock and AMP grazing to provide optimum levels of defoliation and plant recovery between grazing bouts, with direct seeding of annual crops into perennial grass communities during dormancy in a rotation that maintains or strengthens the perennial herbaceous base (Millar and Badgery, 2009; White, 2012). Ley and pasture-cropping facilitate year-round, actively growing groundcover and greatly extend plant growth days, improve soil structure, soil nutrients and soil organic carbon, reduce wind and water erosion and weed competition, and improve biodiversity and ecological resilience, even under drought stress.
While some studies claim that finishing beef cattle on grain-based feeds lowers GHG emissions per kilogram beef produced to give a lower carbon footprint compared to grass-finished beef such studies do not take into account the full carbon footprint of the different production chains (Teague et al., 2016; Stanley et al., 2018). Although grain finishing reduces overall production time to slaughter and lowers enteric fermentation during this time (Stackhouse-Lawson et al., 2012; Capper and Bauman, 2013) such calculations do not take into account the negative carbon footprint associated with the full grazing on perennial pastures in the production chain of grass-fed beef. Life cycle analyses that include all GHG emissions associated with the production of grain-based feeds, including the production and application of inorganic fertilizers and irrigation water to produce grain, show that the C footprint as well as soil erosion associated with grain-finished beef substantially exceeds the C footprint of grass-finished beef (Teague et al., 2016; Stanley et al., 2018). Additionally, C sequestered by plants grazed by cattle exceeds the enteric emissions of the grazing animals (Wang et al., 2015; Rowntree et al., 2016). The C footprint of the beef production chain can be substantially reduced when ruminants are finished on forages and grains produced using regenerative cropping practices that have a negative C footprint (Gattinger et al., 2012; Aguilera et al., 2013). If combined with regenerative AMP grazing, the whole production chain could result in substantive increases in soil carbon levels and associated ecological benefits.
Managing to Achieve Sustainable Income Goals in a Variable Environment
For continuously grazed rangeland, to achieve sustainable goals it is extremely important to choose an appropriate business management goal structure (Teague et al., 2009). Earning capacity can be four times higher for rangeland in excellent condition than in poor condition (Teague et al., 2009), however, under continuous grazing, profit is maximized at stocking rates that are higher than those that would maintain or increase long-term range condition due to negative impacts on the land from patch overgrazing. To prevent overgrazing and long-term degradation of continuously grazed areas, using light stock rates has been identified as an essential management choice (Briske et al., 2008); however, this incurs an opportunity cost due to lower livestock production and, therefore, income generation. The spatial model of Jakoby et al. (2015) identified several viable low risk management choices are possible with continuous grazing or few paddocks per herd, but they require relatively low stocking rates that result in low productivity and economic returns. Periodic resting and rotational grazing (in particular AMP grazing) have been shown to have considerable potential for decreasing negative grazing impacts in environments where there is considerable area selective grazing and vegetation regrowth is relatively slow (Snyman, 1998; Teague et al., 2004; Müller et al., 2007).
Research incorporating protocols that have provided desired resource and economic improvements by conservation ranchers have substantiated the positive soil and ecosystem results reported by regenerative livestock producers. Jakoby et al. (2014) determined that grazing management that used a large number of paddocks per herd to ensure short grazing periods and adequate post-grazing plant recovery facilitated resource improvement and produced optimal economic results. However, economic risk decreased only with management adjustments that accounted for forage quality in each paddock and seasonality over the modeled landscape (Jakoby et al., 2015). Similarly, Teague et al. (2015) found that excessively long grazing or recovery periods resulted in poorer animal performance and plant recovery, which leads to negative economic consequences, as acknowledged by consultants working with ranchers (Walt Davis1, Dave Pratt2, Dr. Allen Williams3 pers. com.).
The use of many paddocks per herd combined with adaptive stocking management is less sensitive to overstocking than constant stocking and with appropriate management increases ecological function (Jakoby et al., 2015; Teague et al., 2015). The advantage of AMP over continuous grazing is less critical at low stocking rates, it becomes increasingly essential as stock numbers increase, to improve net economic returns. Similarly, at the scale of commercial ranches, Wang et al. (2016) demonstrated that, AMP grazing with short periods of grazing and sufficiently long periods of post grazing recovery improved grass composition and productivity, and livestock dry matter consumption relative to continuous grazing, especially with heavier stocking rates and lower initial standing crop and forage composition. Conversely, the advantages of AMP grazing are less evident with favorable rainfall conditions, light stocking, low levels of undesirable plants, and when short post-grazing recovery periods are provided. Overall, these studies have found that under both low- and high-risk management strategies, AMP grazing using large paddock numbers improved resource condition, increased the likelihood of attaining a minimum income goal, lowered income variability, and produced superior economic returns on investment (Jakoby et al., 2015).
Conclusions
To ensure long-term sustainability and ecological resilience of agroecosystems, agricultural management decisions needs to be guided by policies that encourage cropping and grazing protocols that regenerate soil and ecosystem function in uncertain, variable, and rapidly changing climates. With appropriate management of grazing and cropping enterprises, soil ecological functions can be regenerated or enhanced to improve essential ecosystem services that support human well-being, while simultaneously reducing the use of costly and potentially damaging artificial inputs. Regenerating soil health and ecosystem function can be achieved using conservation agricultural practices to support ecologically healthy resilient agroecosystems, improve net profitability, and enhance watershed hydrological function.
Research conducted on managed landscapes shows that AMP grazing and regenerative forage-cropping strategies incorporating short periods of high-density grazing with long recovery periods, cropping rotations that include forages and grazing ruminants while eliminating or substantially reducing soil tillage, inorganic fertilizers and biocides can regenerate soil and ecosystem function on commercial-scale landscapes. Affected ecosystem services include solar energy capture, soil carbon accumulation, soil formation and stabilization, surface water infiltration, soil-biosphere cooling, nutrient cycling and retention, plant biomass production, biodiversity, and wildlife habitat. An increase in permanent cover of forage plants is highly effective in reducing soil erosion and increasing the infiltration of precipitation into the soil. Additionally, grass-fed livestock produced using well-managed AMP grazing can result in a net carbon sink. Similarly, incorporating forages with ruminants in rotational cropping systems can regenerate soil ecological functions in agro-ecosystems and elevate soil C. Using goal-oriented planning and monitoring protocols to reverse damages created by poor grazing, tillage, inorganic fertilizer, and biocide application practices, biodiversity and wildlife habitat can also be effectively facilitated when included in management goals.
To eliminate the damaging effects of current agricultural practices and to restore soil and ecosystem function and resilience it will be essential to change current unsustainable and costly and damaging high-input agricultural practices to low-input regenerative practices. A key to regenerating ecosystem services provided by grazing lands as the base to improve landowner incomes is to adopt well-planned and adaptively managed AMP grazing as it provides substantive advantages over CG as well as PH with continuous stocking. To promote continuous light grazing in the hope that it will minimize the negative historical impacts of poor grazing practices may, at best, sustain or perhaps slightly improve the degraded grazing land resource. However, CG or PH will not likely produce adequate economic returns to encourage managers to adopt land management practices that reverse soil degradation and will, therefore, not likely increase food production or profit potential. On the other hand, appropriately managed AMP grazing can not only support higher livestock production per hectare and secondary production efficiency, but also improve soil ecological function and, therefore, provision of ecosystem services and profits from grazing ecosystems (Jakoby et al., 2015).
To increase the scale of adoption of land management practices documented to improve soil health, scientists will need to collaborate with managers who have shown how to improve their natural resource base to prosper financially to identify management factors that lead to land improvement and to achieve sound environmental, economic and social benefits. In areas where cropping is not possible due to climate, edaphic or topographic constraints, grazing of livestock in a manner that enhances soil health will reduce agriculture's C footprint substantially. Ruminant livestock are an important tool not only for livelihoods of people living in such regions but also for achieving sustainable agriculture where cropping is feasible if appropriate regenerative grazing and cropping management is practiced. Doing so can increase atmospheric C sequestered and soil carbon capture to more than offset ruminant GHG emissions, and that improves ecosystem services that are essential for long-term human well-being.
Author Contributions
RT was invited to write the paper, conceived the contents, and wrote the paper. UK co-wrote and edited the paper and contributed content to each section of the paper. All authors contributed to the article and approved the submitted version.
Conflict of Interest
The authors declare that the research was conducted in the absence of any commercial or financial relationships that could be construed as a potential conflict of interest.
Footnotes
1. ^Walt Davis, Davis Consulting, Available online at: https://waltdavisranch.com/
2. ^Dave Pratt, Ranch Management Consultants, Available online at: https://www.ranchmanagement.com/
3. ^Dr. Allen Williams, Livestock Management Consultants, LLC, Available online at: https://joyce-farms.com/
References
Aguilera, E., Lassaletta, L., Gattinger, A., and Gimeno, B. S. (2013). Managing soil carbon for climate change mitigation and adaptation in mediterranean cropping systems: a meta-analysis. Agric. Ecosys. Environ. 168, 25–36. doi: 10.1016/j.agee.2013.02.003
Altieri, M. A. (1999). The ecological role of biodiversity in agroecosystems. Agric. Ecosyst. Environ. 74, 19–31. doi: 10.1016/B978-0-444-50019-9.50005-4
Archer, S. R., Andersen, E. M., Predick, K. I., Schwinning, S., Steidl, R. J., and Woods, S. R. (2017). Woody plant encroachment: causes and consequences. in Rangeland Systems, Springer Series on Environmental Management. ed. D. Briske (New York, NY: Springer), 25–84. doi: 10.1007/978-3-319-46709-2_2
Averill, C., Turner, B. L., and Finzi, A. C. (2014). Mycorrhiza-mediated competition between plants and decomposers drives soil carbon storage. Nature 505, 543–545. doi: 10.1038/nature12901
Babiker, I. S., Mohamed, M. A. A., Terao, H., Kato, K., and Ohta, K. (2004). Assessment of groundwater contamination by nitrate leaching from intensive vegetable cultivation using geographical information system. Environ. Int. 29, 1009–1017. doi: 10.1016/S0160-4120(03)00095-3
Bailey, D. W., and Provenza, F. D. (2008). “Mechanisms determining large-herbivore distribution,” in Resource Ecology: Spatial and Temporal Dynamics of Foraging, eds H. T. T. Prins, and F. van Langevelde (Dordrecht: Springer), 7–28.
Bardgett, R. D., and McAlister, E. (1999). The measurement of soil fungal: bacterial biomass ratios as an indicator of ecosystem self-regulation in temperate meadow grasslands. Biol. Fert. Soil 29, 282–290. doi: 10.1007/s003740050554
Blouin, M., Hodson, M. E., Delgado, E. A., Bakerd, G. L., Brussaard, K. R., et al. (2013). A review of earthworm impact on soil function and ecosystem services. Euro. J. Soil Sci. 64, 161–182. doi: 10.1111/ejss.12025
Briggs, J. M., Knapp, A. K., Blair, J. M., Heisler, J. L., Hoch, G. A., Lett, M. S., et al. (2005). An ecosystem in transition: causes and consequences of the conversion of mesic grassland to shrubland. BioSci. 55, 243–254. doi: 10.1641/0006-3568(2005)055[0243:AEITCA]2.0.CO;2
Briske, D., Derner, J., Brown, J., Fuhlendorf, S., et al. (2008). Benefits of rotational grazing on rangelands: an evaluation of the experimental evidence. Range. Ecol. Manage. 61, 3–17. doi: 10.2111/06-159R.1
Briske, D. D., Sayre, N. F., Huntsinger, L., Fernandez-Gimenez, M., Budd, B., and Derner, J. D. (2011). Origin, persistence and resolution of the rotational grazing debate: integrating human dimensions into rangeland research. Range. Ecol. Manage. 64, 325–334. doi: 10.2111/REM-D-10-00084.1
Burkart, M. R., and Stoner, J. D. (2002). Nitrate in aquifers beneath agricultural systems. Water Sci. Tech. 45, 19–29. doi: 10.2166/wst.2002.0195
Capper, J. L., and Bauman, D. E. (2013). The role of productivity in improving the environmental sustainability of ruminant production systems. Ann. Rev. An. Biosc. 1, 469–489. doi: 10.1146/annurev-animal-031412-103727
Carberry, P. S., McCown, R. L., Muchow, R. C., Dimes, J. P., Probert, M. E., Poulton, P. L., et al. (1996). Simulation of a legume ley farming system in northern Australia using the agricultural production systems simulator. Austr. J. Exp. Ag. 36, 1037–1048. doi: 10.1071/EA9961037
Conant, R. T., Paustian, K., and Elliott, E. T. (2001). Grassland management and conversion into grasslands: effects on soil carbon. Ecol. Appl. 11, 343–355. doi: 10.2307/3060893
De Vries, F. T., Bloem, J., Quirk, H., Stevens, C. J., Bol, R., and Bardgett, R. D. (2012). Extensive management promotes plant and microbial nitrogen retention in temperate grassland. PLoS ONE 7:51201. doi: 10.1371/journal.pone.0051201
De Vries, F. T., Thébault, E., Liirie, M., Birkhofer, K., Tsiafouli, M. A., Bjørnlund, L., et al. (2013). Soil food web properties explain ecosystem services across European land use systems. Proc. Natl. Acad. Sci. U.S.A. 110, 14296–14301. doi: 10.1073/pnas.1305198110
Delgado, J. A., Groffman, P. M., Nearing, M. A., Goddard, T., Reicosky, D., Lal, R., et al. (2011). Conservation practices to mitigate and adapt to climate change. J. Soil Water Conserv. 66, 118A−29A. doi: 10.2489/jswc.66.4.118A
Díaz-Solís, H., Kothmann, M. M., Grant, W. E., Teague, W. R., and Díaz-García, J. A. (2009). Adaptive management of stocking rates to reduce effects of drought on cow–calf production systems in semi-arid rangelands. AG Syst. 100, 43–50. doi: 10.1016/j.agsy.2008.12.007
Dowhower, S. L., Teague, W. R., Casey, K. D., and Daniel, R. (2019). Soil greenhouse gas emissions as impacted by soil moisture and temperature under continuous and holistic planned grazing in native tallgrass prairie. Agric. Ecosyst. Environ. 286:106647. doi: 10.1016/j.agee.2019.106647
Earl, J. M., and Jones, C. E. (1996). The need for a new approach to grazing management-is cell grazing the answer? Range J. 18, 327–350. doi: 10.1071/RJ9960327
Ferguson, B. G., Diemont, S. A. W., Alfaro-Arguello, R., Martin, J. F., Nahed-Toral, J., Álvarez-Solís, D., et al. (2013). Sustainability of holistic and conventional cattle ranching in the seasonally dry tropics of Chiapas, Mexico. Agric. Syst. 120, 38–48. doi: 10.1016/j.agsy.2013.05.005
Ferguson, P. R., and Veizer, J. (2007). Coupling of water and carbon fluxes via the terrestrial biosphere and its significance to the earth's climate system. J. Geophys. Res. 112. doi: 10.1029/2007JD008431
Flack, S. (2016). The Art and Science of Grazing: How Grass Farmers Can Create Sustainable Systems for Healthy Animals and Farm Ecosystems. Vermont: Chelsea Green, 230.
Frank, D. A., and Groffman, P. A. (1998). Ungulate vs. landscape control of soil C and N processes in grasslands of Yellowstone National Park. Ecology 79, 2229–2241.
Frank, D. A., McNaughton, S. J., and Tracy, B. F. (1998). The ecology of the earth's grazing ecosystems. BioScience 48, 513–521. doi: 10.2307/1313313
Franzluebbers, A. J., and Stuedemann, J. A. (2010). Surface soil changes during twelve years of pasture management in the southern piedmont USA. S. Sci. Soc. Am. J. 74, 2131–2141. doi: 10.2136/sssaj2010.0034
Fuhlendorf, S. D., Harrell, W. C., Engle, D. M., Hamilton, R. G., Davis, C. A., and Leslie, D. M. (2006). Should heterogeneity be the basis for conservation? Grassland bird response to fire and grazing. Ecol. Appl. 16, 1706–1716. doi: 10.1890/1051-0761(2006)016[1706:SHBTBF]2.0.CO;2
Fuls, E. R. (1992). Ecosystem modification created by patch-overgrazing in semi-arid grassland. J. Arid Environ. 23, 59–69. doi: 10.1016/S0140-1963(18)30541-X
Gattinger, A., Muller, A., Haeni, M., Skinner, C., Fliessbach, A., Buchmann, N., et al. (2012). Enhanced top soil carbon stocks under organic farming. Proc. Natl. Acad. Sci. U.S.A. 109, 18226–18231. doi: 10.1073/pnas.1209429109
Gerrish, J. (2004). Management Intensive Grazing: the Grassroots of Grass Farming. Chelsea Green, VIC: Green Park Press, 314.
Green, L. E., Porras-Alfaro, A., and Sinsabaug, R. L. (2008). Translocation of nitrogen and carbon integrates biotic crust and grass production in desert grassland. J. Ecol. 96, 1076–1085. doi: 10.1111/j.1365-2745.2008.01388.x
Havstad, K. M., Peters, D. P. C., Skaggs, R., Brown, J., Bestelmeyer, B., Fredrickson, E., et al. (2007). Ecological services to and from rangelands of the United States. Ecol. Econ. 64, 261–268. doi: 10.1016/j.ecolecon.2007.08.005
Herrick, J. E., and Lal, R. (1995). Soil physical property changes during dung decomposition in a tropical pasture. S. Sci. Soc. Am. J. 59, 908–912. doi: 10.2136/sssaj1995.03615995005900030040x
Hillenbrand, M., Thompson, R., Wang, F., Apfelbaum, S., and Teague, R. (2019). Impacts of holistic lanned grazing with bison compared to continuous grazing with cattle in South Dakota shortgrass prairie. Agric. Ecosyst. Environ. 279, 156–168. doi: 10.1016/j.agee.2019.02.005
Hulbert, L. C. (1988). Causes of fire effects in tallgrass prairie. Ecology 69, 46–58. doi: 10.2307/1943159
Iglesias, L. E., Saumell, C. A., Fernández, A. S., Lifschitz, A. L., Rodríguez, E. M., Steffan, P. E., et al. (2006). Environmental impact of ivermectin excreted by cattle treated in autumn on dung fauna and degradation of faeces on pasture. Parasitol. Res. 100, 93–102. doi: 10.1007/s00436-006-0240-x
Jacobo, E. J., Rodríguez, A. M., Bartoloni, N., and Deregibus, V. A. (2006). Rotational grazing effects on rangeland vegetation at a farm scale. Range. Ecol. Manag. 59, 249–257. doi: 10.2111/05-129R1.1
Jakoby, O., Quaas, M. F., Baumgärtner, S., and Frank, K. (2015). Adapting livestock management to spatio-temporal heterogeneity in semi-arid rangelands. J. Environ. Manage. 162, 179–189. doi: 10.1016/j.jenvman.2015.07.047
Jakoby, O., Quaas, M. F., Müller, B., Baumgärtner, S., and Frank, K. (2014). How do individual farmers' objectives influence the evaluation of rangeland management strategies under a variable climate? J. Appl. Ecol. 51, 483–493. doi: 10.1111/1365-2664.12216
Janzen, H. H. (2010). What place for livestock on a re-greening earth? Anim. Feed Sci. Technol. 166–167, 783–796. doi: 10.1016/j.anifeedsci.2011.04.055
Kallenbach, C. M., Frey, S. D., and Grand, A. S. (2016). Direct evidence for microbial-derived soil organic matter formation and its ecophysiological controls. Nat. Comm. 7:13630. doi: 10.1038/ncomms13630
Kashuk, G., Kuyper, T. W., Leffelaar, P. A., Hungria, M., and Giller, K. E. (2009). Are the rates of photosynthesis stimulated by the carbon sink strength of rhizobial and arbuscular mycorrhizal symbioses? Soil Biol. Biochem. 41, 1233–1244. doi: 10.1016/j.soilbio.2009.03.005
Kellner, K., and Bosch, O. J. H. (1992). Influence of patch formation in determining stocking rate for southern African grasslands. J. Arid. Environ. 22, 99–105. doi: 10.1016/S0140-1963(18)30661-X
Khidir, H. H., Eudy, D. M., Porras-Alfaro, A., Herrera, J., Natvig, D. O., and Sinsabaugh, R. L. (2008). A general suite of fungal endophytes dominate the roots of two dominant grasses in a semiarid grassland. J. Arid Environ. 74, 35–42. doi: 10.1016/j.jaridenv.2009.07.014
LaCanne, C. E., and Lundgren, J. G. (2018). Regenerative agriculture: merging food production and natural resource conservation in a profitable business model. PeerJ 6:4428. doi: 10.7717/peerj.4428
Lal, R. (2003). Soil erosion and the global carbon budget. Environ. Int. 29, 437–450. doi: 10.1016/S0160-4120(02)00192-7
Lal, R. (2004). Soil carbon sequestration impacts on global climate change and food security. Science 304, 1623–1627. doi: 10.1126/science.1097396
Liebig, M. A., Gross, J. R., Kronberg, S. L., Phillips, R. L., and Hanson, J.D. (2010). Grazing management contributions to net global warming potential: a long-term evaluation in the northern great plains. J. Environ. Qual. 39, 799–809. doi: 10.2134/jeq2009.0272
Machmuller, M. B., Kramer, M. G., Cyle, T. K., Hill, N., Hancock, D., and Thompson, A. (2015). Emerging land use practices rapidly increase soil organic matter. Nat. Commun. 6:6995. doi: 10.1038/ncomms7995
Martin, R., Müller, B., Linstädter, A., and Frank, K. (2014). How much climate change can pastoral livelihoods tolerate? Modelling rangeland use and evaluating risk. Glob. Environ. Change 24, 183–192. doi: 10.1016/j.gloenvcha.2013.09.009
Massy, C. (2018). Call of the Reed Warbler: A New Agriculture, A New Earth. White River Junction, VT: Chelsea Green Publishing.
MEA (2005). Millennium Ecosystem Assessment. Ecosystems and Human Well-being: Synthesis. Washington, DC: Island Press.
Millar, G. D., and Badgery, W. B. (2009). Pasture cropping: anew approach to integrate crop and livestock farming systems. An. Prod. Sci. 49, 777–787. doi: 10.1071/AN09017
Montgomery, D. R. (2017). Growing a Revolution: Bringing Our Soils Back to Life. New York, NY: W.W. Norton & Company.
Morriën, E., Hannula, S. E., Snoek, L. B., Helmsing, N. R., Zweers, H., de Hollander, M., et al. (2017). Soil networks become more connected and take up more carbon as nature restoration progresses. Nat. Comm. 8:14349. doi: 10.1038/ncomms14349
Müller, B., Frank, K., and Wissel, C. (2007). Relevance of rest periods in nonequilibrium rangeland systems: a modelling analysis. Agric. Syst. 92, 295–317. doi: 10.1016/j.agsy.2006.03.010
Müller, B., Schulzem, J., Kreuer, D., Linstädter, A., Frank, K. (2014). How to avoid unsustainable side effects of managing climate risk in drylands - the supplementary feeding controversy. Agric. Syst. 139, 153–165. doi: 10.1016/j.agsy.2015.07.001
Murphy, B. (1998). Greener Pasture on Your Side of the Fence: Better Farming Voisin Management-Intensive Grazing, 4th ed. Colchester, VT: Arriba Publishers. 388.
National Research Council. (2002). Riparian Areas: Functions and Strategies for Management. Washington, DC: National Academic Press, 415.
Nealson, K. H., Platt, T., and Hastings, J. W. (1970). Cellular control of the synthesis and activity of the bacterial luminescent system. J. Bacteriol. 104, 313–322. doi: 10.1128/JB.104.1.313-322.1970
Ngumbi, E., and Kloepper, J. (2016). Bacterial-mediated drought tolerance: current and future prospects. Appl. Soil Ecol. 105, 109–125. doi: 10.1016/j.apsoil.2016.04.009
Oesterheld, M., Sala, O. E., and McNaughton, S. J. (1992). Effect of animal husbandry on herbivore carrying capacity at the regional scale. Nature 356, 234–236. doi: 10.1038/356234a0
Park, J., Ale, S., and Teague, W. R. (2017). Simulated water quality effects of alternate grazing management practices at the ranch and watershed scales. Ecol. Mod. 360, 1–13. doi: 10.1016/j.ecolmodel.2017.06.019
Pecenka, J. R., and Lundgren, J. G. (2019). Effects of herd management and the use of ivermectin on dung arthropod communities in grasslands. Basic Appl. Ecol. 40, 1–11. doi: 10.1016/j.baae.2019.07.006
Pickett, S. T. A., and White, P. S. (1985). The Ecology of Natural Disturbance and Patch Dynamics. Boston: Academic Press.
Plassart, P., Vinceslas, M. A., Gangneux, C., Mercier, A., Barray, S., and Laval, K. (2008). Molecular and functional responses of soil microbial communities under grassland restoration. Agric. Ecosyst. Environ. 127, 286–293. doi: 10.1016/j.agee.2008.04.008
Plimer, I. (2009). Heaven and Earth: Global Warming, the Missing Science. Ballan, VIC: Connor Court Publishing. 130.
Pokorný, J., Brom, J., Cermák, J., Hesslerová, P., Huryna, H., Nadezhdina, N., et al. (2010). Solar energy dissipation and temperature control by water and plants. Int. J. Water. 5, 311–336. doi: 10.1504/IJW.2010.038726
Provenza, F. D. (2008). What does it mean to be locally adapted and who cares anyway? J. Anim. Sci. 86, 271–284. doi: 10.2527/jas.2007-0468
Retallack, G. J. (2013). Global cooling by grassland soils of the geological past and near future. Anim. Rev. Earth Planet Sci. 41, 69–86. doi: 10.1146/annurev-earth-050212-124001
Rice, E. L., and Parenti, R. L. (1978). Causes of decreases in productivity in undisturbed tall grass prairie. Am. J. Bot. 65, 1091–1097. doi: 10.1002/j.1537-2197.1978.tb06175.x
Richardson, P. Q., and Richardson, R. H. (2000). Dung beetles improve the soil community in Texas and Oklahoma. Ecol. Rest. 18, 116–117.
Rillig, M. C. (2004). Arbuscular mycorrhizae and terrestrial ecosystem processes. Ecol. Lett. 7, 740–754. doi: 10.1111/j.1461-0248.2004.00620.x
Rowntree, J. E., Ryals, R., DeLonge, M. S., Teague, W. R., Chiavegato, M. B., Byck, P., et al. (2016). Potential mitigation of midwest grass-finished beef production emissions with soil carbon sequestration in the United States of America. Future of Food J. Food Agric. Soc. 4, 31–38.
Sanderman, J., Hengl, T., and Fiske, G. J. (2017). Soil carbon debt of 12,000 years of human land use. Proc. Natl. Acad. Sci. U.S.A. 114, 9575–9580. doi: 10.1073/pnas.1706103114
Shaviv, N. J., and Veizer, J. (2003). Celestial driver of Phanerozoic climate. Geol. Soc. Am. Today 13, 4–10. doi: 10.1130/1052-5173(2003)013<0004:CDOPC>2.0.CO;2
Six, J., Bossuyt, H., Degryze, S., and Denef, K. (2004). A history of research on the link between (micro)aggregates, soil biota, and soil organic matter dynamics. Soil Till. Res. 79, 7–31. doi: 10.1016/j.still.2004.03.008
Slade, E. M., Riutta, T., Roslin, T., and Tuomisto, H. L. (2016). The role of dung beetles in reducing greenhouse gas emissions from cattle farming. Nat. Sci. Rep. 6:18140. doi: 10.1038/srep22683
Snyman, H. A. (1998). Dynamics and sustainable utilization of rangeland ecosystems in arid and semi-arid climates of southern Africa. J. Arid Environ. 39, 645–666. doi: 10.1006/jare.1998.0387
Sovell, L. A., Vondracek, B., Frost, J. A., and Mumford, K. G. (2000). Impacts of rotational grazing and riparian buffers on physio-chemical and biological characteristics of Southeastern Minnesota, USA, Streams. Environ. Manage. 26, 629–641. doi: 10.1007/s002670010121
Stackhouse-Lawson, K. R., Rotz, C. A., Oltjen, J. W., and Mitloehner, F. M. (2012). Carbon footprint and ammonia emissions of California beef production systems. J. An. Sci. 90, 4641–4655. doi: 10.2527/jas.2011-4653
Stanley, P. L., Rowntree, J. E., Beede, D. K., DeLonge, M. S., and Hamm, M. W. (2018). Impacts of soil carbon sequestration on life cycle greenhouse gas emissions in Midwestern USA beef finishing systems. Agric. Syst. 162, 249–258. doi: 10.1016/j.agsy.2018.02.003
Stuart Hill, G., and Mentis, M. (1982). Co-evolution of African grasses. Proc. Grassl. Soc. Southern Afr. 17, 122–128.
Teague, R., Grant, W., and Wang, H. (2015). Assessing optimal configurations of multi-paddock grazing strategies in tallgrass prairie using a simulation model. J. Environ. Manage. 150, 262–273. doi: 10.1016/j.jenvman.2014.09.027
Teague, R., Provenza, F., Kreuter, U., Steffens, T., and Barnes, M. (2013). Multi-paddock grazing on rangelands: why the perceptual dichotomy between research results and rancher experience? J. Environ. Manage. 128, 699–717. doi: 10.1016/j.jenvman.2013.05.064
Teague, W. R. (2015). Toward restoration of ecosystem function and livelihoods on grazed agroecosystems. Crop Sci. 55, 2550–2556. doi: 10.2135/cropsci2015.06.0372
Teague, W. R., Apfelbaum, S., Lal, R., Kreuter, U. P., Rowntree, J., Davies, C. A., et al. (2016). The role of ruminants in reducing agriculture's carbon footprint in North America. J. Soil Water Cons. 71, 156–164. doi: 10.2489/jswc.71.2.156
Teague, W. R., and Barnes, M. (2017). Grazing management that regenerates ecosystem function and grazingland livelihoods. Afr. J. Range For. Sci. 34, 77–86. doi: 10.2989/10220119.2017.1334706
Teague, W. R., Dowhower, S. L., Baker, S. A., Ansley, R. J., Kreuter, U. P., Conover, D. M., et al. (2010). Soil and herbaceous plant responses to summer patch burns under continuous and rotational grazing. Agric. Ecosyst. Environ. 137, 113–123. doi: 10.1016/j.agee.2010.01.010
Teague, W. R., Dowhower, S. L., Baker, S. A., Haile, N., DeLaune, P. B., and Conover, D. M. (2011). Grazing management impacts on vegetation, soil biota and soil chemical, physical and hydrological properties in tall grass prairie. Agric. Ecosyst. Environ. 141, 310–322. doi: 10.1016/j.agee.2011.03.009
Teague, W. R., Dowhower, S. L., and Waggoner, J. A. (2004). Drought and grazing patch dynamics under different grazing management. J. Arid Environ. 58, 97–117. doi: 10.1016/S0140-1963(03)00122-8
Teague, W. R., Duke, S. E., Waggoner, J. A., Dowhower, S. L., and Gerrard, S. A. (2008). Rangeland vegetation and soil response to summer patch fires under continuous grazing. Arid Land Res. Manage. 22, 228–241. doi: 10.1080/15324980802183210
Teague, W. R., Kreuter, U. P., Grant, W. E., Diaz-Solis, H., and Kothmann, M. M. (2009). Economic implications of maintaining rangeland ecosystem health in a semi-arid savanna. Ecol. Econ. 68, 1417–1429. doi: 10.1016/j.ecolecon.2008.10.014
Thurow, T. (1991). “Hydrology and erosion,” in Grazing Management: An Ecological Perspective, eds. R. K. Heitschmidt and J. W. Stuth (Portland, OR: Timberland Press) 141–159.
Van der Heijden, M. G. A., Bardgett, R. D., and Van Straalen, N. M. (2008). The unseen majority: soil microbes as drivers of plant diversity and productivity in terrestrial ecosystems. Ecol. Lett. 11, 296–310. doi: 10.1111/j.1461-0248.2007.01139.x
Van der Ploeg, J. D., Verschuren, P., Verhoeven, F., and Pepels, J. (2006). Dealing with novelties: a grassland experiment reconsidered. J. Environ. Pol. Plan. 8, 199–218. doi: 10.1080/15239080600915568
Veizer, J., Godderis, Y., and Francois, L. M. (2000). Evidence for decoupling of atmospheric CO2 and global climate during the phanerozoic eon. Nature 408, 689–701. doi: 10.1038/35047044
Wang, T., Teague, W. R., and Park, S. C. (2016). Evaluation of continuous and multipaddock grazing on vegetation and livestock performance - a modeling approach. Range. Ecol. Manage. 69, 457–464. doi: 10.1016/j.rama.2016.07.003
Wang, T., Teague, W. R., Park, S. C., and Bevers, S. (2015). GHG mitigation potential of different grazing strategies in the United States Southern great plains. Sustainability 7, 13500–13521. doi: 10.3390/su71013500
Wang, T., Teague, W. R., Park, S. C., and Bevers, S. (2018). Evaluating long-term economic and ecological consequences of continuous and multi-paddock grazing - a modeling approach. Agric. Syst. 165, 197–207. doi: 10.1016/j.agsy.2018.06.012
Wardle, D. A., and Bardgett, R. D. (2004). Human-induced changes in large herbivorous mammal density: the consequences for decomposers. Front. Ecol. 2, 145–153. doi: 10.1890/1540-9295(2004)002[0145:HCILHM]2.0.CO;2
Webber, D. F., Mickelson, S. K., Ahmed, S. I., Russell, J. R., Powers, W. J., Schultz, R. C., et al. (2010). Livestock grazing and vegetative filter strip buffer effects on runoff sediment, nitrate, and phosphorus losses. J. Soil Water Cons. 65, 34–41. doi: 10.2489/jswc.65.1.34
Weltz, M. A., Jolley, L., Goodrich, D., Boykin, K., Nearing, M., Stone, J., et al. (2011). Techniques for assessing the environmental outcomes of conservation practices applied to rangeland watersheds. J. Soil Water Cons. 66, 154A−162A. doi: 10.2489/jswc.66.5.154A
Keywords: ecosystem services, grazing management practices, regenerative agriculture, soil health, sustainable capitalism
Citation: Teague R and Kreuter U (2020) Managing Grazing to Restore Soil Health, Ecosystem Function, and Ecosystem Services. Front. Sustain. Food Syst. 4:534187. doi: 10.3389/fsufs.2020.534187
Received: 11 February 2020; Accepted: 19 August 2020;
Published: 29 September 2020.
Edited by:
Gary S. Kleppel, University at Albany, United StatesReviewed by:
Lisa Rachel Norton, UK Centre for Ecology and Hydrology (UKCEH), United KingdomCharles John Massy, Australian National University, Australia
Copyright © 2020 Teague and Kreuter. This is an open-access article distributed under the terms of the Creative Commons Attribution License (CC BY). The use, distribution or reproduction in other forums is permitted, provided the original author(s) and the copyright owner(s) are credited and that the original publication in this journal is cited, in accordance with accepted academic practice. No use, distribution or reproduction is permitted which does not comply with these terms.
*Correspondence: Richard Teague, cnRlYWd1ZSYjeDAwMDQwO2FnLnRhbXUuZWR1